- 1Department of Vascular Surgery, Second Hospital of Shanxi Medical University, Taiyuan, China
- 2Department of Vascular Surgery, First Hospital of Shanxi Medical University, Taiyuan, China
Background: Previous research has hinted at a crucial link between gut microbiota and arterial embolism and thrombosis, yet the causal relationship remains enigmatic. To gain a deeper understanding, we aimed to comprehensively explore the causal relationship and elucidate the impact of the gut microbiota on the risk through a two-sample Mendelian randomization (MR) study.
Methods: Genetic instrumental variables for gut microbiota were identified from a genome-wide association study (GWAS) of 18,340 participants. Summary statistics for IBS were drawn from a GWAS including 1,076 cases and 381,997 controls. We used the inverse-variance weighted (IVW) method as the primary analysis. To test the robustness of our results, we further performed the weighted median method, MR-Egger regression, and MR pleiotropy residual sum and outlier test.
Results: We identified three bacterial traits that were associated with the risk of arterial embolism and thrombosis: odds ratio (OR): 1.58, 95% confidence interval (CI): 1.08–2.31, p = 0.017 for genus Catenibacterium; OR: 0.64, 95% CI: 0.42–0.96, p = 0.031 for genus Dialister; and OR: 2.08, 95% CI: 1.25–3.47, p = 0.005 for genus Odoribacter. The results of sensitivity analyses for these bacterial traits were consistent (P<0.05).
Conclusion: Our systematic analyses provided evidence to support a potential causal relationship between several gut microbiota taxa and the risk of arterial embolism and thrombosis. More studies are required to show how the gut microbiota affects the development of arterial embolism and thrombosis.
Introduction
Thrombosis, characterized by localized blood clotting, can occur within either the arterial or venous circulation, exerting significant medical implications (Mackman, 2008). Acute arterial thrombosis serves as the primary etiology for the majority of myocardial infarctions (heart attacks) and approximately 80% of strokes, constituting the leading cause of mortality in developed nations (Mackman, 2008; GBD Collaborators, 2021). Furthermore, acute thrombotic diseases collectively represent the foremost cause of global mortality and contribute significantly to the global disease burden, as assessed by disability-adjusted life years (Wendelboe and Raskob, 2016; St Germain et al., 2023). Given the substantial morbidity and mortality linked to acute thrombosis, there is an urgent imperative to enhance our comprehension of its pathogenesis and establish effective prevention strategies.
The human gut harbors trillions of microbial cells, comprising a vital component of our healthy physiological ecosystem, encompassing bacteria, fungi, archaea, and viral communities, collectively referred to as the “microbiota” (Tang et al., 2017). Alterations in the composition and dysregulation of the gut microbiota, known as dysbiosis, have been implicated in the onset of various diseases (Tang et al., 2017). In recent years, there has been a surge of interest in the interaction between the gut microbiota and the host. A mounting body of evidence indicates that the gut microbiota plays a pivotal role in human health and disease, encompassing arterial embolism and thrombosis (Koren et al., 2011; Tang et al., 2017, 2019). For example, some studies have reported cases implicating Dialister in the occurrence of arterial embolism and thrombosis (Pierre Lepargneur et al., 2006; Koren et al., 2011). However, these observational findings are susceptible to unmeasured confounding factors and may not accurately depict the relationship between gut microbiota and arterial embolism and thrombosis. Furthermore, reverse causation could introduce bias if alterations or cessation of the gut microbiota were influenced by the onset or progression of arterial embolism and thrombosis.
Mendelian randomization (MR) studies have been widely applied in etiological research for diseases in recent years. In the absence of randomized controlled trials, MR is the most persuasive strategy for exploring causality between exposure and outcomes (Zuccolo and Holmes, 2017). MR mimics randomized controlled trials by using single-nucleotide polymorphisms (SNPs) related to exposure as instrumental variables (IVs) (Hemani et al., 2018). This IV approach replicates randomization as SNPs are randomly allocated to offspring at conception, largely avoiding confounding factors, and individual factors such as sex and age are unlikely to bias causal effects(Burgess et al., 2013; Richmond and Davey Smith, 2022). Similarly, due to the formation of genotypes occurring before disease onset, errors related to reverse causality in MR studies are also unlikely (Burgess et al., 2013). In this study, we chose 196 gut microbiotas as exposures and arterial embolism as the outcome for MR analysis. Our objective was to investigate the potential causal relationship between gut microbiota, aiming to establish a theoretical foundation for further research into the intricate mechanisms and risk factors associated with arterial embolism and thrombosis.
Materials and methods
Study design
An effective MR study should adhere to three assumptions: (1) a robust and strong correlation between the IVs and the exposure factor; (2) independence between the IVs and confounding factors that influence the “exposure-outcome” relationship; and (3) genetic variation can only affect the occurrence of the outcome through the exposure factor and not through other pathways (Davies et al., 2018).
Gut microbiota was selected as the exposure, while arterial embolism and thrombosis of the lower extremity artery served as the outcome. All statistics involved in the analysis were derived from publicly available genome-wide association studies (GWASs) (Supplementary Table S1). SNPs associated with gut microbial taxa and gut microbial metabolites were extracted as IVs. Based on the summary-level data from GWAS of gut microbiota and arterial embolism and thrombosis, we conducted a two-sample MR analysis. The flowchart of the study is shown in Figure 1.
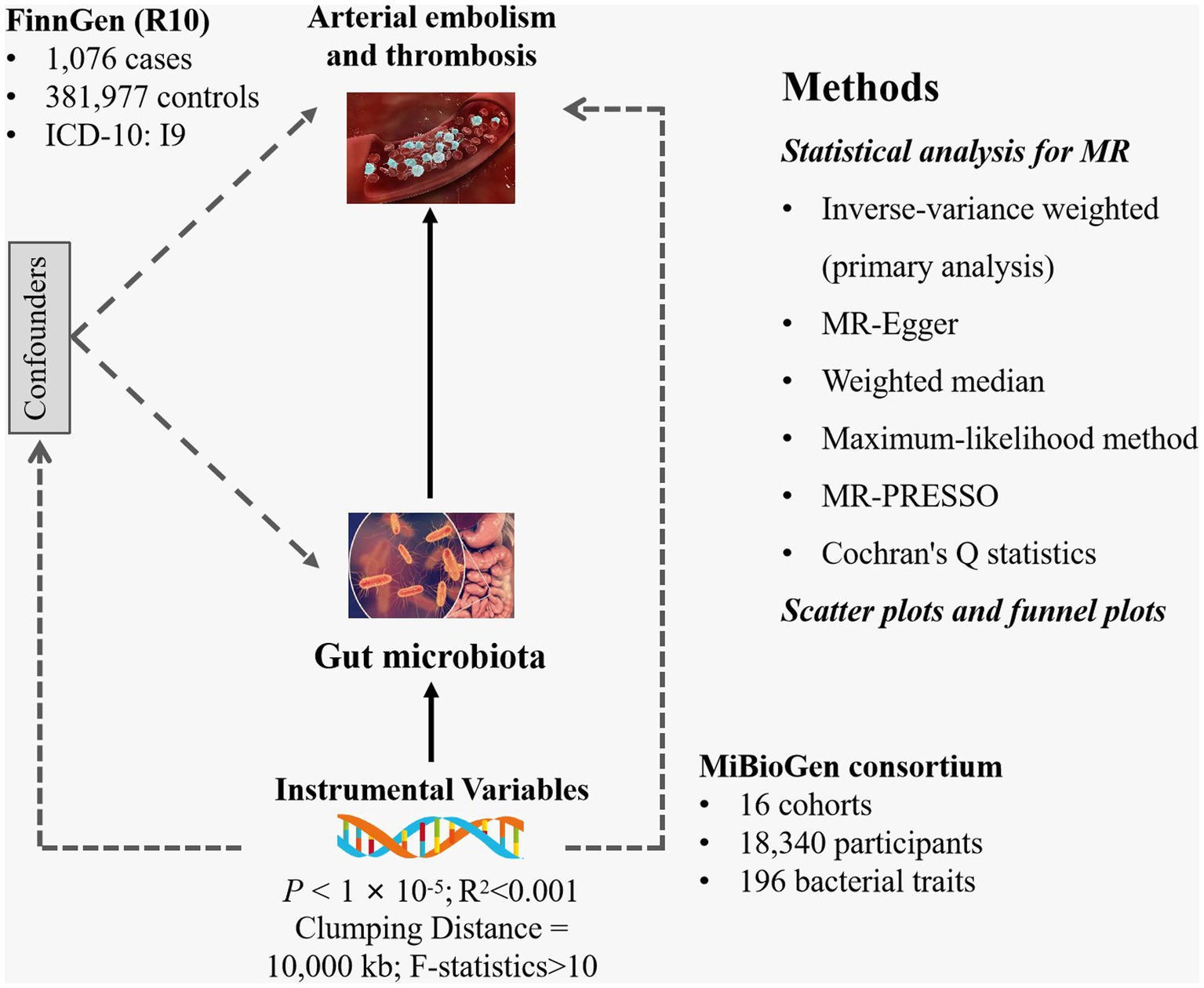
Figure 1. Study design of the present MR study of the associations between gut microbiota and arterial embolism and thrombosis of the lower extremity artery. MR, Mendelian randomization; SNP, single-nucleotide polymorphism.
Exposure data of gut microbiota
The comprehensive GWAS summary statistics on the microbiome are mainly derived from the international consortium MiBioGen (Kurilshikov et al., 2021). This consortium incorporates data from 16 cohorts in different countries, including 18,340 participants with 24S rRNA gene sequencing profiles and genotyping data (Kurilshikov et al., 2021). The large-scale, multi-ethnic, whole-genome meta-analysis examines the association between common human genetic variations and the gut microbiome (Kurilshikov et al., 2021). The summary data include 211 gut microbial taxa. Relevant GWAS data can be accessed at https://mibiogen.gcc.rug.nl/. This represents the largest genome-wide meta-analysis conducted to date on the microbiome (Kurilshikov et al., 2021).
Outcome data of arterial embolism and thrombosis of lower extremity artery
The summary data for arterial embolism and thrombosis of the lower extremity artery were retrieved from FinnGen’s online database (Kurki et al., 2023). This is a unique study combining genotype data from the Finnish Biobank and digital healthcare data from the Finnish Health Registry for both participants aged 18 years and older residing in Finland adjusting for age, sex, genetic relatedness, genotyping batch, and principal components (Kurki et al., 2023). Within this GWAS for Arterial embolism and thrombosis of lower extremity artery, total 383,053 European participants were included, with 381,977 controls and 1,076 Arterial embolism and thrombosis of lower extremity artery cases which were diagnosed according to the International Classification of Diseases codes (ICD-10: I9) (Kurki et al., 2023).
Genetic instruments selection and harmonization
We first removed 15 bacterial traits without a specific name, leaving 196 bacterial traits, including 9 phylum, 16 class, 20 order, 32 family, and 119 genus (Liu et al., 2022). To guarantee the reliability and precision of the outcomes, the SNPs underwent a thorough quality assessment to yield compliant IVs. The principles guiding the selection of SNPs were outlined as follows: Firstly, the SNPs must exhibit a strong correlation with the exposure; second, the SNPs must be unrelated to any confounders; and finally, the SNPs must be associated with the outcomes mediated by the exposures (Davies et al., 2018). To achieve a more comprehensive result, a genome-wide statistical significance threshold of p < 1 × 10−5 was chosen for selecting eligible IVs (Liu et al., 2022). Then, to eliminate linkage disequilibrium (LD), a clumping method with r2 = 0.001 and kb = 10,000 was applied. Finally, the F-statistics were calculated to assess the strength of the selected SNPs using the formula: R2 = 2 MAF (1 − MAF) × β2, F = R2 (n-k-1) / k(1-R2) (Palmer et al., 2012; Kamat et al., 2019). In this formula, “MAF” is the minor allele frequency of SNPs used as IVs, R2 is the fraction of variability explained by each SNP, N is the GWAS sample size, and K is the number of SNPs. MAF is the A F statistic of 10 indicates that there is no convincing evidence of instrument bias (Georgakis et al., 2019).
Statistical analysis
We used the inverse-variance weighted (IVW) method as the primary analysis. Then we applied a range of sensitivity analyses to assess the robustness of the IVW findings against potential violations, including MR-Egger, weighted median, maximum-likelihood method, and MR pleiotropy residual sum and outlier (MR-PRESSO) analyses. IVW utilizes a meta-analytic approach to pool the Wald estimates for each SNP, which is the most popular and reliable MR analysis technique (Burgess et al., 2013). MR-Egger is used to account for the uncorrelated pleiotropy of SNPs as IVs (Bowden et al., 2015). Weighted median method ensures reliable causal effect estimates if over half its weight derives from valid IVs (Bowden et al., 2016). The maximum-likelihood method was used, which may display a more credible association result when measurement error existed in the SNP-exposure effects (Hemani et al., 2018). For sensitivity analysis, Cochran’s Q statistic was utilized to assess heterogeneity, with a p-value of <0.05 regarded as significant heterogeneity (Burgess et al., 2017). If there is existing heterogeneity, random-effect IVW is performed instead of fixed-effect IVW (Burgess et al., 2017). MR-Egger is used to account for the uncorrelated pleiotropy of SNPs as IVs (Bowden et al., 2015). To exclude the effect of horizontal pleiotropy, MR-Egger regression was used to assess pleiotropy through the intercept term. An intercept p-value of <0.05 implied the presence of horizontal pleiotropy (Bowden et al., 2015). Moreover, the MR-PRESSO method was performed to remove possible horizontal pleiotropic outliers. After removing the outliers, the data were subsequently re-analyzed (Verbanck et al., 2018). Additionally, we used scatter plots and funnel plots to detect whether there were existing outliers and evident heterogeneity.
The association between gut microbiota and arterial embolism and thrombosis was represented using odds ratios (ORs) along with 95% confidence intervals (CIs). A p-value of <0.05 was considered the significance threshold. All the analyses were conducted by applying packages “TwoSampleMR,” “MRPRESSO,” and “MendelianRandomization” in R version 4.1.3. The analysis codes are shown in Supplementary Table S1.
Results
Selection of instrumental variables
After removing palindromic SNPs from a large-scale GWAS, we initially identified 2,699 SNPs related to 196 gut microbiota (with a locus-wide significance level of p < 1 × 10−5) (Supplementary Tables S2, S3). For IVs used for gut microbiota, the F-statistics ranged from 21.8 to 142.0, which were all above 10, suggesting weak instrument bias was less likely (Supplementary Table S2). We observed statistically significant evidence for a potential causal association between 11 gut microbiota and the risk of AS using the IVW method, including one class, one family, and nine genera. The details of SNPs used for bacterial traits are listed in Supplementary Table S3, and the results of the associations between the 11 bacterial traits and the risk of arterial embolism and thrombosis are presented in Figure 2 and Supplementary Table S4.
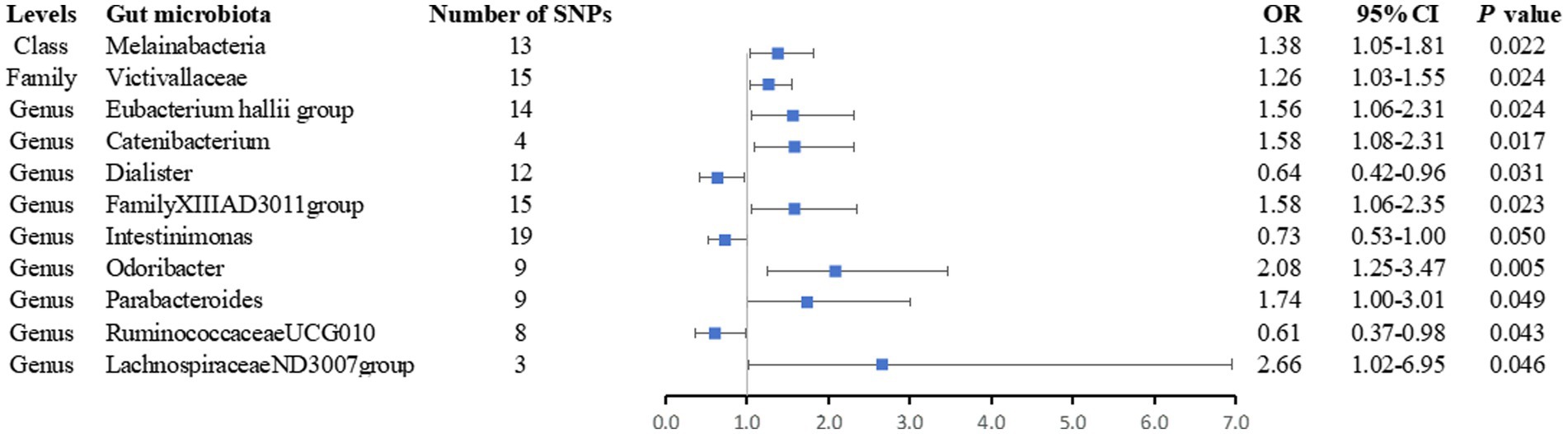
Figure 2. Forest plot of the associations between genetically determined 11 bacterial traits and arterial embolism and thrombosis of lower extremity artery risk. CI, confidence interval; OR, odds ratio; SNP, single-nucleotide polymorphism.
Overall, we found that genus Catenibacterium was positively associated with the risk of arterial embolism and thrombosis of the lower extremity artery by the IVW method (OR: 1.58, 95% CI: 1.08–2.31, p = 0.017) (Figure 3). Using alternative MR methods, we observed similar results. For genus Catenibacterium, a similar effect estimate was obtained in the weighted median method (OR: 1.66, 95% CI: 1.05–2.62, p = 0.031) and the maximum-likelihood method (OR: 1.60, 95% CI: 1.08–2.37, p = 0.020). MR-PRESSO test did not detect any outliers, and the association estimate was similar (OR: 1.58, 95% CI: 1.20–2.08, p = 0.046). Little evidence of directional pleiotropy was detected by MR-Egger regression (P intercept = 0.352).
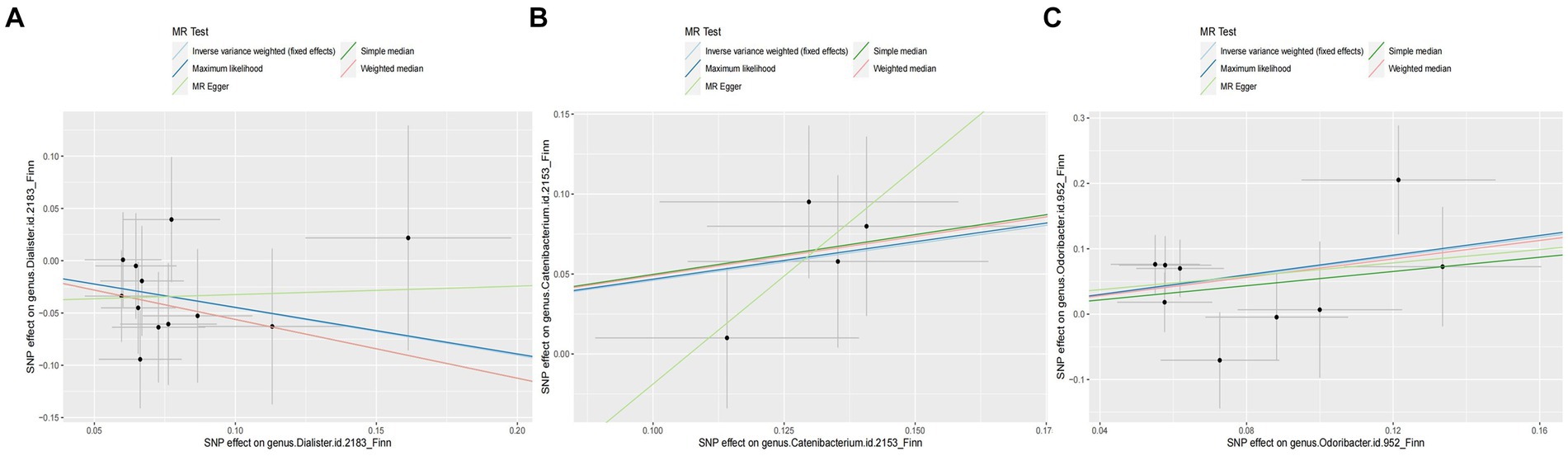
Figure 3. Scatter plot of the associations of genetic variants with three bacterial traits and the risk of arterial embolism and thrombosis. MR, mendelian randomization; SNP, single-nucleotide polymorphism. (A) SNP effect on genus Dialister; (B) SNP effect on genus Catenibacterium; (C) SNP effect on genus Odoribacter.
In addition, we also observed that genus Odoribacter was positively associated with the risk of arterial embolism and thrombosis of the lower extremity artery by IVW method (OR: 2.08, 95% CI: 1.25–3.47, p = 0.005) (Figure 3). The sensitivity analysis results were consistent (OR: 2.30, 95% CI: 1.13–4.69, p = 0.021 for weighted median method; OR: 2.14, 95% CI: 1.26–3.62, p = 0.005 for maximum-likelihood method). MR-PRESSO test found no potential outlier, and the association remained statistically significant (OR: 2.08, 95% CI: 1.25–3.49, p = 0.005). MR-Egger regression did not suggest directional pleiotropy (P intercept = 0.803).
However, genus Dialister was negatively associated with the risk of arterial embolism and thrombosis of the lower extremity artery by IVW (OR: 0.64, 95% CI: 0.42–0.96, p = 0.031) (Figure 3). Similar associations were observed using the weighted median method (OR: 0.57, 95% CI: 0.34–0.96, p = 0.035), maximum-likelihood method (OR: 0.64, 95% CI: 0.43–0.98, p = 0.038), and MR-PRESSO test (OR: 0.64, 95% CI: 0.47–0.85, p = 0.012). The intercepts of MR-Egger regression did not significantly deviate from zero for them (P intercept = 0.536).
Finally, we noticed that the rest of the six bacterial traits were associated with a higher risk of arterial embolism and thrombosis of the lower extremity artery in the IVW method (OR: 1.38; 95% CI: 1.05–1.81; p = 0.022 for class Melainabacteria; OR: 1.26; 95% CI: 1.03–1.55; p = 0.024 for family Victivallaceae; OR: 1.56; 95% CI: 1.06–2.31; p = 0.024 for genus Eubacterium hallii group OR: 1.58; 95% CI: 1.06–2.35; p = 0.023 for genus FamilyXIIIAD3011group; OR: 1.74; 95% CI: 1.00–3.01; p = 0.049 for genus Parabacteroides; and OR: 2.66; 95% CI: 1.02–6.95; p = 0.046 for genus LachnospiraceaeND3007group) while two were associated with a lower risk (OR: 0.73; 95% CI: 0.53–1.00; p = 0.050 for genus Intestinimonas and OR: 0.61; 95% CI: 0.37–0.98; p = 0.012 for genus RuminococcaceaeUCG010).
Discussion
To our knowledge, this is the first MR study that integrates gut microbiota, arterial embolism, and thrombosis of the lower extremity artery. This two-sample MR study identified a total of 11 bacterial taxa, including class Melainabacteria, family Victivallaceae, genus Eubacterium hallii group, genus Catenibacterium, Dialister, FamilyXIIIAD3011 group, Intestinimonas, Odoribacter, Parabacteroides, RuminococcaceaeUCG010, and LachnospiraceaeND3007 group might have a causal relationship with the risk of arterial embolism and thrombosis of the lower extremity artery. However, sensitivity analyses using different MR methods and restricted IV sets demonstrated three bacterial taxa, Catenibacterium, Dialister, and Odoribacter, associated with the risk of arterial embolism and thrombosis of the lower extremity artery. These results will contribute to further exploration of the roles of gut microbiota and blood metabolites in the process of arterial embolism and thrombosis of the lower extremity artery. Furthermore, they provide a reference for future intervention measures and the development of potential therapeutic targets.
A two-sample MR study found 17 bacterial traits and their association with the risk of venous thromboembolism, including family Victivallaceae (Wang et al., 2024), which was consistent with our study’s finding of an increased risk of arterial embolism. However, another two-sample MR study found seven causal associations between genetic liability in the gut microbiota and lower extremity deep vein thrombosis (LEDVT) (Hirai et al., 2022). These findings did not align with the results of our study, which could be attributed to differences in the study populations.
The genus Dialister has also been identified as a protective factor against arterial embolism and thrombosis in the lower extremity artery. For instance, dysbiosis in Dialister has been associated with various diseases, including thrombosis (Pierre Lepargneur et al., 2006; Xu et al., 2024). Another study has also identified that an increase in Actinobacteria would decrease the risk of thrombosis (Yang et al., 2022). In addition, the abundance of Dialister showed significant alterations in healthy controls and cardiovascular and cerebrovascular diseases (Ji et al., 2017; Chang et al., 2021; Han et al., 2021). The genus Dialister may produce molecules with immunoregulatory and anti-inflammatory functions, such as short-chain fatty acids (SCFAs), indoles and their derivatives, and secondary bile acids. These molecules could potentially regulate the immune system by inhibiting the activity of immune cells, thus modulating the intensity and direction of immune responses, which may mitigate the risk of arterial embolism and thrombosis (Sun et al., 2022). The potential causal relationship observed in this study between Dialister and arterial embolism and thrombosis underscores the significance of Dialister in the development of these conditions.
The bacterial taxa genus Catenibacterium and Odoribacter were found to be positively associated with the risk of arterial embolism and thrombosis in this study. However, to date, there have been no studies reporting alterations in the genus Catenibacterium in patients with arterial embolism and thrombosis. We noticed an increasing trend for the genus Catenibacterium and Odoribacter in stroke cases compared to healthy controls (Li et al., 2019; Yu et al., 2023; Yuan et al., 2023). It has been shown that Catenibacterium and Odoribacter could be linked to obesity (Pinart et al., 2021), which is known to increase the risk of arterial embolism. While this study is the first to suggest a potential causal relationship between the genera Catenibacterium and Odoribacter and the risk of arterial embolism and thrombosis, further research is warranted to elucidate the underlying biological mechanisms.
There are potential explanations for how the gut microbiota influences arterial embolism and thrombosis. The intestinal microbiota is segregated from the host by the mucus layer and a specialized epithelial lining (Johansson et al., 2011; Duerr and Hornef, 2012). The epithelium must meet precise criteria to shield the host from foreign antigens while simultaneously permitting substances to enter the portal circulation for energy metabolism and sufficient nutrient provision (Lingaraju et al., 2015). The gut–vascular barrier, in addition to the epithelial barrier, must also meet these requirements (Spadoni et al., 2015; Thevaranjan et al., 2017). It is noteworthy that the gut microbiota not only influences the permeability of the intestinal vasculature but also has the capability to induce the development of complex capillary networks and lacteals within the villus structures of the small intestine, facilitating nutrient absorption (Stappenbeck et al., 2002; Reinhardt et al., 2012; Bernier-Latmani et al., 2015). In addition, there is a close relationship between the gut microbiota and the immune system (Haak et al., 2018; Wiersinga and van der Poll, 2022). The gut microbiota interacts intricately with the host’s immune system, influencing immune responses through interactions with immune cells. Specific components of the microbial community can activate immune cells, leading to inflammatory responses and immune reactions closely associated with the occurrence and progression of arterial embolism and thrombosis. Notably, the rampant and indiscriminate use of antibiotics has resulted in heightened resistance to antimicrobial drugs, which in turn disrupts the gut microbiota of the host organism (Dorobanțu et al., 2022; Păduraru et al., 2022). It is pertinent to consider whether these antibiotics could impact the 11 gut microorganisms identified in the study, potentially influencing thrombogenesis.
Our MR study offers several advantages. First, we examined the causal effect of each biological level of gut microbiota on arterial embolism and thrombosis, ranging from the genus to the phylum level. This approach contributes to elucidating the mechanisms and interactions between the gut microbiota and host immunity, facilitating a comprehensive assessment of the influence of various bacterial traits. Additionally, the genetic variants of the gut microbiota were sourced from the largest GWAS available, enhancing the credibility of our findings. However, our MR study also has limitations. First, while we identified causal associations from exposure to outcomes, the magnitude of these associations may not be accurately gauged. Further research is necessary to validate these findings. Second, the use of multiple statistical corrections may have been overly stringent and conservative, potentially leading to the oversight of bacterial traits with a causal association with sepsis. Therefore, given biological plausibility, we did not consider multiple testing results. Third, although the majority of participants in our study with gut microbiota data were of European descent, a small portion of microbiological data originated from other racial backgrounds, potentially confounding our estimates. Fourth, we used a less strict threshold (p < 1 × 10−5) for horizontal pleiotropy examination and sensitivity analysis. While this allowed for the identification of a broader range of associations, it also increased the risk of detecting false positives. Fifth, this study did not collect information on the stage, duration, and severity of arterial embolism and thrombosis to further analyze the effect of gut microbes on different stages of arterial embolism and thrombosis. Sixth, we could not rule out the possibility of residual confounding by some uncontrolled factors (e.g., viruses and fungi), which may bias the gut microbiota and influence arterial embolism and thrombosis.
Ultimately, our study is confined to data analysis, lacking direct experimentation in animal models and validation in patient cohorts. Further confirmation of our conclusions through subsequent experiments is imperative. In summary, our study reaffirms the causal effects of gut microbiota on the risk of arterial embolism and thrombosis. Three bacterial taxa—the genera Catenibacterium, Dialister, and Odoribacter—identified in this study may be causally linked to the risk of arterial embolism and thrombosis. These findings may offer insights into the pathogenesis and potential novel treatments for arterial embolism and thrombosis.
Data availability statement
Publicly available datasets were analyzed in this study. This data can be found at: Finngen R10 (https://r10.finngen.fi/); MiBioGen (https://mibiogen.gcc.rug.nl/) for releasing the GWAS summary statistics.
Author contributions
Y-BS: Data curation, Formal analysis, Investigation, Methodology, Writing – original draft, Writing – review & editing. H-LD: Data curation, Formal analysis, Investigation, Methodology, Supervision, Writing – original draft. W-KC: Data curation, Formal analysis, Investigation, Writing – original draft, Writing – review & editing. YZ: Data curation, Formal analysis, Investigation, Methodology, Writing – review & editing. H-JJ: Data curation, Investigation, Methodology, Writing – original draft. J-KL: Conceptualization, Data curation, Investigation, Methodology, Writing – original draft. SY: Investigation, Methodology, Supervision, Validation, Writing – review & editing.
Funding
The author(s) declare that no financial support was received for the research, authorship, and/or publication of this article.
Acknowledgments
The authors appreciate the Finngen R10, MiBioGen, and FHS Offspring Cohort studies for releasing the GWAS summary statistics.
Conflict of interest
The authors declare that the research was conducted in the absence of any commercial or financial relationships that could be construed as a potential conflict of interest.
Publisher’s note
All claims expressed in this article are solely those of the authors and do not necessarily represent those of their affiliated organizations, or those of the publisher, the editors and the reviewers. Any product that may be evaluated in this article, or claim that may be made by its manufacturer, is not guaranteed or endorsed by the publisher.
Supplementary material
The Supplementary material for this article can be found online at: https://www.frontiersin.org/articles/10.3389/fmicb.2024.1396699/full#supplementary-material
References
Bernier-Latmani, J., Cisarovsky, C., Demir, C. S., Bruand, M., Jaquet, M., Davanture, S., et al. (2015). DLL4 promotes continuous adult intestinal lacteal regeneration and dietary fat transport. J. Clin. Invest. 125, 4572–4586. doi: 10.1172/JCI82045
Bowden, J., Davey Smith, G., and Burgess, S. (2015). Mendelian randomization with invalid instruments: effect estimation and bias detection through egger regression. Int. J. Epidemiol. 44, 512–525. doi: 10.1093/ije/dyv080
Bowden, J., Davey Smith, G., Haycock, P. C., and Burgess, S. (2016). Consistent estimation in Mendelian randomization with some invalid instruments using a weighted median estimator. Genet. Epidemiol. 40, 304–314. doi: 10.1002/gepi.21965
Burgess, S., Butterworth, A., and Thompson, S. G. (2013). Mendelian randomization analysis with multiple genetic variants using summarized data. Genet. Epidemiol. 37, 658–665. doi: 10.1002/gepi.21758
Burgess, S., Small, D. S., and Thompson, S. G. (2017). A review of instrumental variable estimators for Mendelian randomization. Stat. Methods Med. Res. 26, 2333–2355. doi: 10.1177/0962280215597579
Chang, Y., Woo, H. G., Jeong, J. H., Kim, G. H., Park, K. D., and Song, T. J. (2021). Microbiota dysbiosis and functional outcome in acute ischemic stroke patients. Sci. Rep. 11:10977. doi: 10.1038/s41598-021-90463-5
Davies, N. M., Holmes, M. V., and Davey Smith, G. (2018). Reading Mendelian randomisation studies: a guide, glossary, and checklist for clinicians. BMJ 362:k601. doi: 10.1136/bmj.k601
Dorobanțu, F. R., Hodoșan, V., Tîrb, A. M., Zaha, D. C., Galușca, D., Pop, N. O., et al. (2022). Pattern of newborn antibiotic use in a tertiary level maternity for five years. Pharmacophore 13, 57–63. doi: 10.51847/pq4Px0rkxG
Duerr, C. U., and Hornef, M. W. (2012). The mammalian intestinal epithelium as integral player in the establishment and maintenance of host-microbial homeostasis. Semin. Immunol. 24, 25–35. doi: 10.1016/j.smim.2011.11.002
GBD Collaborators (2021). Global, regional, and national burden of stroke and its risk factors, 1990-2019: a systematic analysis for the global burden of disease study 2019. Lancet Neurol. 20, 795–820. doi: 10.1016/S1474-4422(21)00252-0
Georgakis, M. K., Gill, D., Rannikmäe, K., Traylor, M., Anderson, C. D., Lee, J. M., et al. (2019). Genetically determined levels of circulating cytokines and risk of stroke. Circulation 139, 256–268. doi: 10.1161/CIRCULATIONAHA.118.035905
Haak, B. W., Prescott, H. C., and Wiersinga, W. J. (2018). Therapeutic potential of the gut microbiota in the prevention and treatment of Sepsis. Front. Immunol. 9:2042. doi: 10.3389/fimmu.2018.02042
Han, Y., Gong, Z., Sun, G., Xu, J., Qi, C., Sun, W., et al. (2021). Dysbiosis of gut microbiota in patients with acute myocardial infarction. Front. Microbiol. 12:680101. doi: 10.3389/fmicb.2021.680101
Hemani, G., Zheng, J., Elsworth, B., Wade, K. H., Haberland, V., Baird, D., et al. (2018). The MR-base platform supports systematic causal inference across the human phenome. eLife 7:e34408. doi: 10.7554/eLife.34408
Hirai, J., Kuruma, T., Sakanashi, D., Kuge, Y., Kishino, T., Shibata, Y., et al. (2022). Lemierre syndrome due to Dialister pneumosintes: a case report. Infect. Drug Resist. 15, 2763–2771. doi: 10.2147/IDR.S359074
Ji, W., Zhu, Y., Kan, P., Cai, Y., Wang, Z., Wu, Z., et al. (2017). Analysis of intestinal microbial communities of cerebral infarction and ischemia patients based on high throughput sequencing technology and glucose and lipid metabolism. Mol. Med. Rep. 16, 5413–5417. doi: 10.3892/mmr.2017.7227
Johansson, M. E., Ambort, D., Pelaseyed, T., Schütte, A., Gustafsson, J. K., Ermund, A., et al. (2011). Composition and functional role of the mucus layers in the intestine. Cell. Mol. Life Sci. 68, 3635–3641. doi: 10.1007/s00018-011-0822-3
Kamat, M. A., Blackshaw, J. A., Young, R., Surendran, P., Burgess, S., Danesh, J., et al. (2019). PhenoScanner V2: an expanded tool for searching human genotype-phenotype associations. Bioinformatics 35, 4851–4853. doi: 10.1093/bioinformatics/btz469
Koren, O., Spor, A., Felin, J., Fåk, F., Stombaugh, J., Tremaroli, V., et al. (2011). Human oral, gut, and plaque microbiota in patients with atherosclerosis. Proc. Natl. Acad. Sci. USA 108, 4592–4598. doi: 10.1073/pnas.1011383107
Kurilshikov, A., Medina-Gomez, C., Bacigalupe, R., Radjabzadeh, D., Wang, J., Demirkan, A., et al. (2021). Large-scale association analyses identify host factors influencing human gut microbiome composition. Nat. Genet. 53, 156–165. doi: 10.1038/s41588-020-00763-1
Kurki, M. I., Karjalainen, J., Palta, P., Sipilä, T. P., Kristiansson, K., Donner, K. M., et al. (2023). FinnGen provides genetic insights from a well-phenotyped isolated population. Nature 613, 508–518. doi: 10.1038/s41586-022-05473-8
Li, N., Wang, X., Sun, C., Wu, X., Lu, M., Si, Y., et al. (2019). Change of intestinal microbiota in cerebral ischemic stroke patients. BMC Microbiol. 19:191. doi: 10.1186/s12866-019-1552-1
Lingaraju, A., Long, T. M., Wang, Y., Austin, J. R. 2nd, and Turner, J. R. (2015). Conceptual barriers to understanding physical barriers. Semin. Cell Dev. Biol. 42, 13–21. doi: 10.1016/j.semcdb.2015.04.008
Liu, B., Ye, D., Yang, H., Song, J., Sun, X., Mao, Y., et al. (2022). Two-sample Mendelian randomization analysis investigates causal associations between gut microbial genera and inflammatory bowel disease, and specificity causal associations in ulcerative colitis or Crohn's disease. Front. Immunol. 13:921546. doi: 10.3389/fimmu.2022.921546
Mackman, N. (2008). Triggers, targets and treatments for thrombosis. Nature 451, 914–918. doi: 10.1038/nature06797
Păduraru, L., Jurca, A. D., Zaha, D. C., Dorobanțu, F. R., Hasan, M. A., and Popoviciu, M. S. (2022). Aetiology of bacterial infections and antibiotic resistance in pediatric patients from a Romanian hospital. Pharmacophore 13, 77–83. doi: 10.51847/r2fEu5aJXs
Palmer, T. M., Lawlor, D. A., Harbord, R. M., Sheehan, N. A., Tobias, J. H., Timpson, N. J., et al. (2012). Using multiple genetic variants as instrumental variables for modifiable risk factors. Stat. Methods Med. Res. 21, 223–242. doi: 10.1177/0962280210394459
Pierre Lepargneur, J., Dubreuil, L., and Levy, J. (2006). Isolation of Dialister pneumosintes isolated from a bacteremia of vaginal origin. Anaerobe 12, 274–275. doi: 10.1016/j.anaerobe.2006.07.004
Pinart, M., Dötsch, A., Schlicht, K., Laudes, M., Bouwman, J., Forslund, S. K., et al. (2021). Gut microbiome composition in obese and non-obese persons: a systematic review and Meta-analysis. Nutrients 14:12. doi: 10.3390/nu14010012
Reinhardt, C., Bergentall, M., Greiner, T. U., Schaffner, F., Ostergren-Lundén, G., Petersen, L. C., et al. (2012). Tissue factor and PAR1 promote microbiota-induced intestinal vascular remodelling. Nature 483, 627–631. doi: 10.1038/nature10893
Richmond, R. C., and Davey Smith, G. (2022). Mendelian randomization: concepts and scope. Cold Spring Harb. Perspect. Med. 12:a040501. doi: 10.1101/cshperspect.a040501
Spadoni, I., Zagato, E., Bertocchi, A., Paolinelli, R., Hot, E., Di Sabatino, A., et al. (2015). A gut-vascular barrier controls the systemic dissemination of bacteria. Science (New York, N.Y.) 350, 830–834. doi: 10.1126/science.aad0135
St Germain, L., De Paula, E., Ay, C., Barco, S., Cesarman-Maus, G., Connors, J. M., et al. (2023). Moving against thrombosis: ISTH recognizes 10th anniversary of world thrombosis day and the leaders in the field who led the way - in memory of Claire McLintock, MD, world thrombosis day steering committee vice chair 2019 to 2022. J. Thromb. Haemost. 21, 2311–2312. doi: 10.1016/j.jtha.2023.06.006
Stappenbeck, T. S., Hooper, L. V., and Gordon, J. I. (2002). Developmental regulation of intestinal angiogenesis by indigenous microbes via Paneth cells. Proc. Natl. Acad. Sci. USA 99, 15451–15455. doi: 10.1073/pnas.202604299
Sun, W., Du, D., Fu, T., Han, Y., Li, P., and Ju, H. (2022). Alterations of the gut microbiota in patients with severe chronic heart failure. Front. Microbiol. 12:813289. doi: 10.3389/fmicb.2021.813289
Tang, W. H. W., Bäckhed, F., Landmesser, U., and Hazen, S. L. (2019). Intestinal microbiota in cardiovascular health and disease: JACC state-of-the-art review. J. Am. Coll. Cardiol. 73, 2089–2105. doi: 10.1016/j.jacc.2019.03.024
Tang, W. H., Kitai, T., and Hazen, S. L. (2017). Gut microbiota in cardiovascular health and disease. Circ. Res. 120, 1183–1196. doi: 10.1161/CIRCRESAHA.117.309715
Thevaranjan, N., Puchta, A., Schulz, C., Naidoo, A., Szamosi, J. C., Verschoor, C. P., et al. (2017). Age-associated microbial Dysbiosis promotes intestinal permeability, systemic inflammation, and macrophage dysfunction. Cell Host Microbe 21, 455–466.e4. doi: 10.1016/j.chom.2017.03.002
Verbanck, M., Chen, C. Y., Neale, B., and Do, R. (2018). Detection of widespread horizontal pleiotropy in causal relationships inferred from Mendelian randomization between complex traits and diseases. Nat. Genet. 50, 693–698. doi: 10.1038/s41588-018-0099-7
Wang, J., Fei, B., and Wang, C. (2024). Gut microbiota and venous thromboembolism: a two-sample Mendelian randomization study Gut microbiota and venous thromboembolism. doi: 10.21203/rs.3.rs-3834627/v1
Wendelboe, A. M., and Raskob, G. E. (2016). Global burden of thrombosis: epidemiologic aspects. Circ. Res. 118, 1340–1347. doi: 10.1161/CIRCRESAHA.115.306841
Wiersinga, W. J., and van der Poll, T. (2022). Immunopathophysiology of human sepsis. EBioMedicine 86:104363. doi: 10.1016/j.ebiom.2022.104363
Xu, Q., Fang, J., Wang, Y., Lang, D., and Xu, B. (2024). The causal relationship between gut microbiota and lower extremity deep vein thrombosis combined with pulmonary embolism. doi: 10.21203/rs.3.rs-3938899/v1
Yang, M., Luo, P., Zhang, F., Xu, K., Feng, R., and Xu, P. (2022). Large-scale correlation analysis of deep venous thrombosis and gut microbiota. Front. Cardiovasc. Med. 9:1025918. doi: 10.3389/fcvm.2022.1025918
Yu, S., Chen, J., Zhao, Y., Liao, X., Chen, Q., Xie, H., et al. (2023). Association analysis of the gut microbiota in predicting outcomes for patients with acute ischemic stroke and H-type hypertension. Front. Neurol. 14:1275460. doi: 10.3389/fneur.2023.1275460
Yuan, S., Li, Y., Wang, L., Xu, F., Chen, J., Levin, M. G., et al. (2023). Deciphering the genetic architecture of atrial fibrillation offers insights into disease prediction, pathophysiology and downstream sequelae. medRxiv. 25:2023.07.20.23292938. doi: 10.1101/2023.07.20.23292938
Keywords: arterial embolism and thrombosis, gut microbiota, Mendelian randomization, causal relationship, single-nucleotide polymorphism
Citation: Shi Y-B, Dong H-L, Chang W-K, Zhao Y, Jin H-J, Li J-K and Yan S (2024) Genetic evidence for a causal link between gut microbiota and arterial embolism and thrombosis: a two-sample Mendelian randomization study. Front. Microbiol. 15:1396699. doi: 10.3389/fmicb.2024.1396699
Edited by:
Na Wu, Peking University People's Hospital, ChinaReviewed by:
Marcos Edgar Herkenhoff, University of São Paulo, BrazilGeorge Grant, Independent Researcher, Aberdeen, United Kingdom
Cosmin Mihai Vesa, University of Oradea, Romania
Copyright © 2024 Shi, Dong, Chang, Zhao, Jin, Li and Yan. This is an open-access article distributed under the terms of the Creative Commons Attribution License (CC BY). The use, distribution or reproduction in other forums is permitted, provided the original author(s) and the copyright owner(s) are credited and that the original publication in this journal is cited, in accordance with accepted academic practice. No use, distribution or reproduction is permitted which does not comply with these terms.
*Correspondence: Sheng Yan, 578115246@qq.com