- 1National Center of Technology Innovation for Pigs, Chongqing, China
- 2Chongqing Academy of Animal Science, Chongqing, China
- 3College of Life Sciences, Southwest University of Science and Technology, Mianyang, Sichuan, China
Introduction: As a symbiotic probiotic for the host, Clostridium butyricum (CB) has the potential to strengthen the body’s immune system and improve intestinal health. However, the probiotic mechanism of CB is not completely understood. The Clostridium butyricum CBX 2021 strain isolated by our team from a health pig independently exhibits strong butyric acid production ability and stress resistance. Therefore, this study comprehensively investigated the efficacy of CBX 2021 in pigs and its mechanism of improving pig health.
Methods: In this study, we systematically revealed the probiotic effect and potential mechanism of the strain by using various methods such as microbiome, metabolites and transcriptome through animal experiments in vivo and cell experiments in vitro.
Results: Our in vivo study showed that CBX 2021 improved growth indicators such as daily weight gain in weaned piglets and also reduced diarrhea rates. Meanwhile, CBX 2021 significantly increased immunoglobulin levels in piglets, reduced contents of inflammatory factors and improved the intestinal barrier. Subsequently, 16S rRNA sequencing showed that CBX 2021 treatment implanted more butyric acid-producing bacteria (such as Faecalibacterium) in piglets and reduced the number of potentially pathogenic bacteria (like Rikenellaceae RC9_gut_group). With significant changes in the microbial community, CBX 2021 improved tryptophan metabolism and several alkaloids synthesis in piglets. Further in vitro experiments showed that CBX 2021 adhesion directly promoted the proliferation of a porcine intestinal epithelial cell line (IPEC-J2). Moreover, transcriptome analysis revealed that bacterial adhesion increased the expression of intracellular G protein-coupled receptors, inhibited the Notch signaling pathway, and led to a decrease in intracellular pro-inflammatory molecules.
Discussion: These results suggest that CBX 2021 may accelerate piglet growth by optimizing the intestinal microbiota, improving metabolic function and enhancing intestinal health.
Introduction
A healthy gastrointestinal tract is the cornerstone for improving animal growth performance and overall health. However, in modern intensive livestock production, weaned piglets experience drastic changes in diet, environment, and management that can disrupt normal GI function, resulting in delayed growth and compromised health (Tang X. P. et al., 2022). Multiple evidence suggests that probiotics can improve intestinal health in weaned piglets, thereby promoting body growth and overall health (Mun et al., 2021; Tang et al., 2021).
Clostridium butyricum (CB) serves as an effective probiotic for restoring the disrupted intestinal microbiota in livestock due to weaning stress. Additionally, CB has been proven to alleviate diarrhea and enhance growth performance (Lu et al., 2020; Ye et al., 2022). Extensive evidence shows that butyric acid, the major functional metabolite produced by CB bacteria, repairs intestinal mucosa damage, maintains intestinal immune homeostasis, and alleviates inflammation in the body (Abdelqader and Al-Fataftah, 2016; Chen G. X. et al., 2018). In recent years, CB has become popular in animal husbandry production as a probiotic preparation for its beneficial characteristics. Despite ongoing research, there is still much to uncover about the specific mechanisms through which CB regulates intestinal health and promotes growth.
Screening and confirming a suitable bacterial strain is crucial for the development of microbial ecological preparations due to the significant differences in physiological characteristics among the different strains from different sources. Our team isolated the Clostridium butyricum CBX 2021 strain from a healthy adult pig, which has shown a high capability for producing butyric acid. The strain was found to produce endospores and have a butyric acid production of 2.15 mg/mL during the exponential growth phase. Additionally, it is non-pathogenic to animals and can effectively restore antibiotic-induced intestinal dysbiosis and damage in mice by rebuilding the gut microbiota and optimizing metabolic function (Liu et al., 2023). Thus, it appears that CBX 2021 shows promise for application in the field of feed probiotics. However, the impact and mechanism of this strain on pig health, especially intestinal health, are currently unclear.
This study examines the impacts of the CBX 2021 strain on the growth and overall health of piglets. Various methods, including microbiome, metabolomics, and transcriptome analysis, were used in both in vivo and in vitro experiments to systematically reveal the potential mechanisms of its probiotic effects. The study provides guidance for developing and using new micro-ecological products.
Materials and methods
Bacteria and its preparation
Clostridium butyricum CBX 2021 strain was isolated and screened from the intestines of a healthy 6-month-old boar in our laboratory, and is currently stored at the Guangdong Microbial Culture Collection Center (GDMCC No.62503).
The CBX 2021 strain was incubated under anaerobic environment at 37°C for 12 h in reinforced Clostridium medium. For animal administration, the harvested bacterial solution (3 × 108 CFU/mL) obtained at this time point was orally administered to weaned piglets. For cell culture assays, the bacterial solution was centrifuged at 5,000 × g at 4°C for 10 min to collect the bacteria. Then, the bacteria were resuspended in DMEM/F12 cell culture medium without penicillin/streptomycin (1.5 × 108 CFU/mL) for cell experiments.
Animal experiment design and sample collection
The experiment included forty-eight 28-day-old healthy piglets (8.16 ± 0.77 kg) split evenly into 2 groups, with 6 replicates per group and 4 piglets per replicate. The control (CON) group piglets were administered a 5 mL sterile saline orally, while the CB group piglets were given a 5 mL CBX 2021 bacterial solution (with an effective bacterial count of 3 × 108 CFU/mL) orally once daily. The experiment lasted for 28 days and utilized a commercially available, standard-formulated feed. The composition and nutritional levels of it are displayed in Supplementary Table S1.
After the experiment concluded, one piglet from each replicate was chosen at random to gather fresh fecal samples for 16S rRNA sequencing analysis. Meanwhile, the venous blood of piglets was collected. After the blood samples stood overnight, the serum was collected by centrifugation at 3,000 × g at 4°C for a duration of 15 min and stored at −80°C for subsequent analysis of immune, growth-related hormones, and intestinal permeability.
Growth performance
All piglets were weighed on an empty stomach on d 0 and 28, and the daily feed intake for each replicate was noted daily. These values are utilized in the computation of the average daily gain (ADG), average daily feed intake (ADFI), and feed-to-gain ratio (F/G).
Monitor the fecal consistency of each piglet during the trial, and document the number of piglets with diarrhea. Assess the piglet feces according to the following scoring standards reported in previous studies and calculate the frequency and index of diarrhea (Hung et al., 2019).
Blood biochemical indicators
As per the manufacturer’s guidelines, the ELISA kit (Enzyme-linked Biotechnology Co., Ltd., Shanghai, China) was utilized to measure serum immune indicators: immunoglobulin A (IgA), IgG, and IgM, as well as interleukin-1β (IL-1β), IL-10, and tumor necrosis factor-α (TNF-α); growth-related hormone indicators: growth hormone (GH), gastrin (GAS), ghrelin (GHRL), peptide YY (PYY), and glucagon-like peptide-1 (GLP-1); intestinal permeability indicators: D-lactic acid (DLA) and diamine oxidase (DAO).
Fecal microbiome analysis
As previously mentioned, we extracted and purified total genomic DNA from piglet fecal samples (Wang J. L. et al., 2016). The quantitative PCR products were then examined through the Illumina NovaSeq platform for sequencing at Beijing Biocloud Biotechnology Co., Ltd. (Beijing, China1) via paired-end sequencing. The raw data can be found in the NCBI Sequence Read Archive (SRA) database and accessed using the login number PRJNA947735.
Sequencing reads were denoised using the DADA2 method and clustered into amplicon sequence variants (ASVs). The SILVA 138 database was used as a reference for classifying and annotating each representative sequence of the ASVs using a naive Bayes classifier, with a 70% confidence threshold. The unweighted unifrac distance algorithm was used for principal coordinate analysis (PCoA) at the bacterial genus level. ANOSIM function was used for statistical analysis. Student’s t-test or Mann–Whitney U test was used to analyze the differences in microbial distribution at phylum and genus level. Additionally, Linear discriminant analysis (LDA) effect size (LEfSe) was employed to identify biomarkers (from family to genus level), with a significance threshold set at an LDA value of ≥3.0.
Serum metabolomics analysis
The serum samples from the piglets were sent to Beijing Biocloud Biotechnology Co., Ltd. (Beijing, China) for metabolite extraction, detection and analysis. The Progenesis QI software was utilized to process the raw data, including peak detection, calibration, and other essential operations for data processing. Upon peak area normalization, the processed data were exported for further analysis. The orthogonal partial least squares discriminant analysis (OPLS-DA) was utilized to compare and identify the overall metabolic variations in the CON and CB groups. Differential metabolites were chosen based on VIP values >1 in the OPLS-DA model and p-value <0.01. The pathway enrichment analysis of the differential metabolites was then conducted using the Kyoto Encyclopedia of Genes and Genomes (KEGG) database. The Spearman correlation algorithm was applied to analyze the relationships among the top 20 bacterial genera, differential metabolites, and serum biochemical indicators, generating correlation matrices to assess their associations.
Cell and cell culture
IPEC-J2 cells were graciously provided by Dr. Yongjiang Wu (Chongqing University of Arts and Sciences, Chongqing, China). The cells were grown in DMEM/F12 medium supplemented with 10% fetal bovine serum (Gibco, Paisley, USA), 1% penicillin/streptomycin (Solarbio, Beijing, China), and 1% insulin-transferrin-selenium (Sigma-Aldrich, MO, USA). And they were incubated in biochemical incubators (5% CO2, 37°C).
Determination of the adhesion ability of the CBX 2021 strain to IPEC-J2 cells
IPEC-J2 cells were inoculated in a 6-well plate (5 × 105 cells/ well), and divided into 2 groups after the confluence reached 70–80%. CB group was exposed to CBX 2021 bacterial suspension (1.5 × 108 CFU/mL, 2 ml/well), and CON group was treated with an equivalent volume of DMEM/F12 medium, with three replicates in each group. After incubation at 37°C for 4, 6, and 8 h, the cells were treated according to the standard procedure. Subsequently, they underwent fixation with 4% neutral formaldehyde for 15 min before being subjected to gram staining. The samples were examined under inverted fluorescence microscope with magnification of ×40 for analysis. Lastly, 25 cells were randomly selected, and the average number of CBX 2021 bacterial adhesion on visible cell surfaces was calculated and documented through photography.
IPEC-J2 cell proliferation assay
IPEC-J2 cells were inoculated in a 96-well plate (1 × 104 cells/ well) and grew to the above degree. The cells were classified into 3 distinct groups: the blank group (without cells), CON group, and CB group. Each group had 3 replicate wells (100 μl/ well). After the cells were incubated for 2, 4, 6, 8, and 12 h, the old medium was aspirated and DMEM/F12 medium with 10% CCK-8 was added (100 μl/ well) within the specified culture time, followed by 2 h of additional incubation. Then, OD values at 450 nm were determined using plate reader, and the cell proliferation activity was calculated at each culture time point to ascertain the optimal time for coculture of the bacterial strain and cells.
Determination of inflammatory factor secretion in IPEC-J2 cells
Cell processing and grouping were as described in the above adhesion experiment. After incubation for 6 h, the coculture supernatant was gathered to measure the secretion levels of IL-1β and IL-10 using porcine ELISA kits as per the manufacturer’s guidelines.
RNA-seq and gene expression analysis
Cell processing and grouping were as described in the above adhesion experiment, and each group had 5 replicates. After incubation for 6 h, cell digestion and collection were performed using 0.25% pancreatase-EDTA (Sigma-Aldrich, MO, USA). The cell samples were then sent to Hangzhou Lianchuan Biotechnology Co., Ltd. (Hangzhou, China) for total RNA isolation, quality control, cDNA library construction, and transcriptome sequencing. The raw data can be found in the NCBI SRA database and accessed using the login number PRJNA948058.
The raw sequencing data underwent quality control, which involved removing reads that had low-quality bases and adapter contamination. Fragment per kilobase per million mapped reads were used to determine gene expression levels. DESeq2 was applied for the differential expression analysis, with the false discovery rate (FDR) controlled through the Benjamini-Hochberg procedure. The screening condition of differentially expressed genes (DEGs) was FDR < 0.05 and |log2(Fold change)| > 1. Additionally, the enrichment analyses were conducted using the OmicStudio cloud platform, including gene ontology (GO) term, KEGG pathway, and gene set enrichment analysis (GSEA). GSEA analysis relied on the GO database and involved calculating a normalized enrichment score (NES) for each gene set through 1,000 permutations of the genome. Gene sets with |NES| > 1 and FDR < 0.05 were considered significantly enriched in the GSEA analysis.
Quantitative real-time PCR
The previously mentioned RNA samples underwent reverse transcription to generate cDNA, utilizing a commercially available reverse transcription kit (Yeasen, Shanghai, China) in a two-step reaction. Then, we followed the instructions provided by the manufacturer of the fluorescent quantitative PCR assay kit (Yeasen, Shanghai, China) in order to conduct our experiments. The 10 μl reaction mixture was prepared containing 1 μl of cDNA, 0.2 μl of upstream and downstream primer, 3.6 μl of RNA-free water, and 5 μl of SYBR fluorescent dye. The mixture underwent cDNA amplification and fluorescence signal collection using a real-time fluorescence quantitative PCR instrument (Thermo Scientific, Wilmington, DE, USA). The amplification program was performed as instructed. In this study, the PCR amplification efficiency was always between 95 and 100%, so the 2-∆∆CT method was used to analyze the relative mRNA expression levels of the target genes mRNA (Livak and Schmittgen, 2001). A single internal reference gene GAPDH was selected for normalization based on previous studies (Wang H. et al., 2019; Wu et al., 2021; Li et al., 2023). Primers utilized in this research were synthesized by Shanghai Sangon Biotech Co., Ltd. (Shanghai, China) (Supplementary Table S2).
Statistical analysis
After the data were preliminarily organization in Excel 2021, SPSS 26.0 software was used for statistical analysis. The Shapiro–Wilk test is used to evaluate whether the data obeys normality. Student’s t-test was conducted for the data that followed normal distribution and showed homogeneity of variance, while Mann–Whitney U test was used for non-parametric analysis when variance was unequal. The results are expressed as means ± SEM. Statistical significance was defined as p < 0.05 (*), p < 0.01 (**), and p < 0.001 (***). A significant trend was noted when 0.05 ≥ p > 0.10. Data visualization was carried out using GraphPad Prism 9.0 software.
Results
Supplementation with CBX 2021 improved the growth performance in weaned piglets
Table 1 presents the growth performance results of piglets. The CB piglets showed a 10.10% increase in body weight after 28 days compared to the CON piglets (p = 0.06). The ADG of CB treated pigs significantly increased by 24.95%, and F/G obviously reduced by 15.82% (p < 0.05). However, no difference in ADFI was witnessed between CON and CB piglets (p > 0.05). Compared to the CON group, CB piglets experienced a significant reduction in diarrhea frequency and diarrhea index by 36.15 and 40.74%, respectively (p < 0.05). The findings indicate that CBX 2021 improves the growth performance of piglets and helps manage post-weaning diarrhea.
Research suggests that there is a strong correlation between peripheral hormone secretion and improved growth performance (Li et al., 2021). Thus, we analyzed the serum levels of growth- and appetite-related hormones in piglets (Table 2). Compared with CON piglets, the of GH and GAS levels in the CB piglets increased by 6.33 and 7.74%, respectively (p < 0.05). The level of GHRL, a key appetite-stimulating hormone, showed a trend of increase by 3.90% (p = 0.06). Elevated levels of GAS and GHRL expression play a significant role in stimulating digestive fluid secretion, which is vital for increasing feed intake and conversion (Mazzoni et al., 2022; Rodríguez-Viera et al., 2022). However, there were no notable variations in the concentrations of the anorectic hormones PYY and GLP-1 between the piglets in the two groups (p > 0.05). The results indicate that CBX 2021 may positively impact the growth and development of piglets by enhancing endocrine hormone levels.
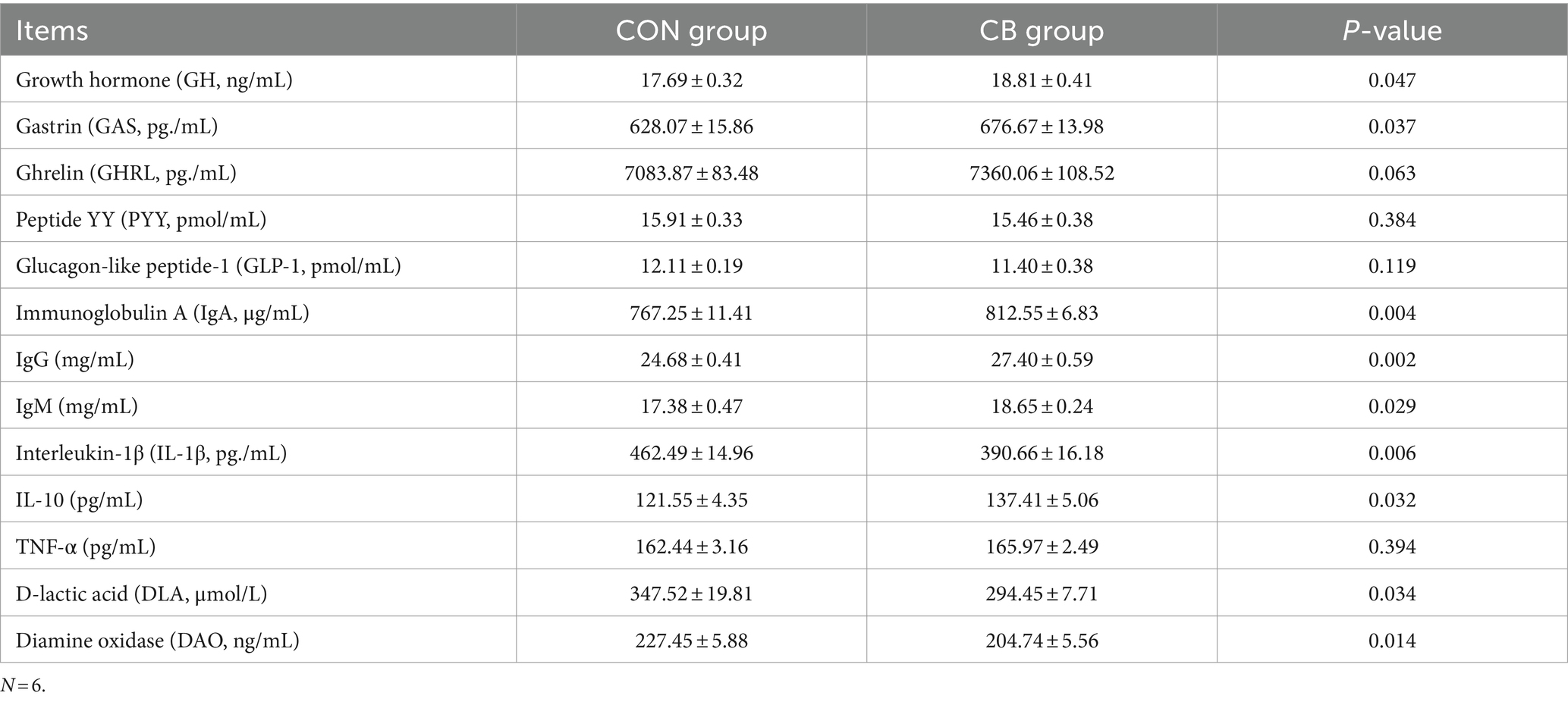
Table 2. Effect of Clostridium butyricum CBX 2021 on blood biochemical indicators in weaned piglets.
Supplementation with CBX2021 enhanced the immunity and intestinal barrier function in weaned piglets
The serum immunoglobulin levels in Table 2 reflect the humoral immune response level. The CB group piglets showed a significant increase of 5.90, 11.02, and 7.31% in IgA, IgG, and IgM levels, respectively, compared with CON piglets (p < 0.05).
Furthermore, we assessed serum levels of inflammatory mediators as indicators of weaning stress-induced inflammation within the body. The study revealed that compared with CON piglets, IL-10 content of CB piglets increased by 13.05%, and IL-1β content decreased by 15.53% (p < 0.05). However, there was no significant difference in TNF-α level between the two groups (p > 0.05).
The impact of CBX 2021 on piglet’ intestinal permeability was studied by measuring serum DLA levels and DAO activity. Elevated levels of DLA and DAO are typically indicative of heightened intestinal permeability (Cai et al., 2019). The findings indicated that the DLA level and DAO activity in the CB piglets were significantly reduced by 15.27 and 9.98%, respectively (p < 0.05) compared with CON piglets.
To sum up, the results indicate that CBX 2021 effectively enhanced immune response, strengthened intestinal barrier, and alleviated inflammatory stress in weaned piglets. This may lead to an overall improvement in the animals’ health status.
Supplementation with CBX 2021 optimized the fecal microbiota in weaned piglets
A balanced microbial community structure contributes to immune system development and intestinal health (Wang et al., 2018; Wu et al., 2023). Here, we conducted an analysis on the diversity and structure of fecal microbiota by 16S rDNA sequencing to evaluate the gut bacterial community of piglets. The PCoA scatter plot, which illustrates the β diversity of microbiota, showing that the microbial distribution of the two groups of piglets is obviously different (R = 0.670, p = 0.002, Figure 1A). This result indicated that supplementation with CBX 2021 obviously altered the composition of microbial community in piglet feces.
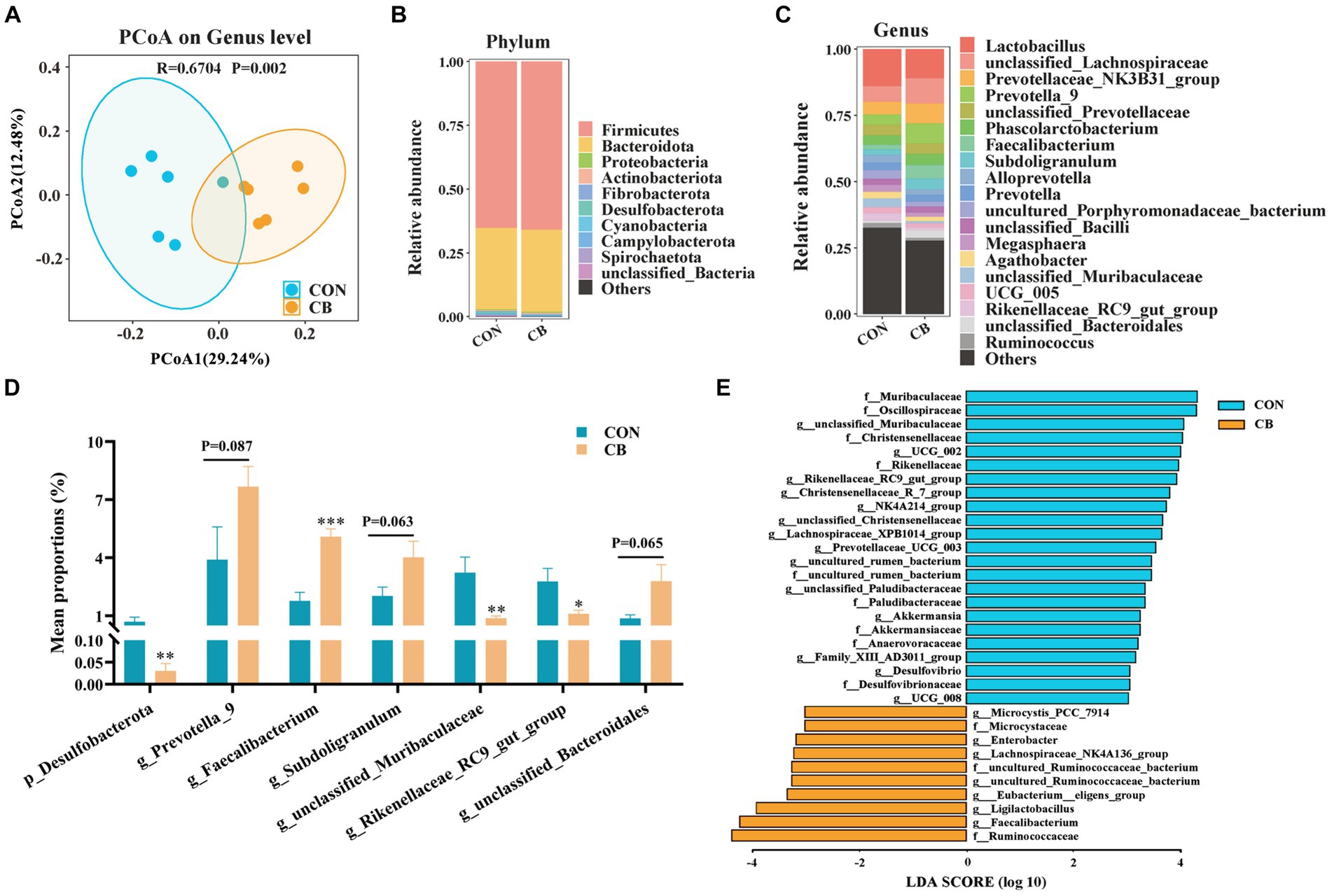
Figure 1. Effects of Clostridium butyricum CBX 2021 on fecal microbiota in weaned piglets (n = 6). (A) Principal coordinate analysis (PCoA) scatter diagram based on genus level. Column chart of species composition at the phylum (B) and genus (C) level. (D) Histogram of bacterial differences at the phylum and genus level. (E) Linear discriminant analysis (LDA) histogram of differential microbial community in the CON and CB group. Data are displayed as the mean ± SEM. *** Indicates p < 0.001, ** indicates p < 0.01, * indicates p < 0.05, and 0.05 ≥ p > 0.10 indicates a significant trend in comparison with the CON piglets.
Subsequently, an analysis of the bacterial population structure was conducted, focusing specifically on phylum and genus levels (Figures 1B,C). A combined total of 12 bacterial phyla were recognized in both groups of piglets. Firmicutes and Bacteroidota were the main phyla among them, comprising over 97% of the total abundance (Figure 1B). Notably, the abundance of Desulfobacterota was significantly decreased by CBX 2021 treatment (p < 0.05, Figure 1D). Figure 1C illustrates the relative abundance of the top 20 genera. Faecalibacterium, a butyrate producer, had a higher relative abundance in piglets receiving CBX 2021 intervention than CON piglets (p < 0.05). Additionally, there was an increasing trend in the number of helpful bacteria, particularly Prevotella_9 (p = 0.087), Subdoligranulum (p = 0.063), and unclassified_Bacteroidales (p = 0.055), with their numbers 1.97-, 1.99-, and 4.65-fold higher than those of the CON piglets, respectively (Figure 1D). Furthermore, the addition of CBX 2021 caused a substantial drop in the number of proinflammatory bacteria, including unclassified_Muribaculaceae and Rikenellaceae_RC9_gut_group (p < 0.05, Figure 1D). The findings suggest that exogenous administration of CBX2021 had a positive impact on the gut microbiota as a whole.
LEfSe analysis was used to study the key bacterial species that drive variations within the microbial population. As shown in Figure 1E, a total of 33 different taxa were found in both groups (LDA score ≥ 3.0). In the CB piglets, seven genera, including Faecalibacterium, Ligilactobacillus, Eubacterium_eligens_group, and Lachnospiraceae_NK4A136_group, were significantly enriched and could serve as biomarker genera. Furthermore, CON piglets exhibited 14 characteristic genera, such as unclassified_Muribaculaceae, Rikenellaceae_RC9_gut_group, Desulfovibrio, and Family_XIII_AD3011_group.
Supplementation with CBX 2021 altered the serum metabolome in weaned piglets
LC-Q/TOF-MS technology was employed to examine the serum metabolome changes in weaned piglets treated with CBX 2021.The OPLS-DA score plots clearly showed clear separation between the two groups, suggesting that CBX 2021 significantly influenced the serum metabolite composition in piglets (Figures 2A,B). The high statistical values of R2Y (explanatory ability parameter) and Q2 (predictive ability parameter) in the score plots demonstrated that the models had good applicability and predictability. Furthermore, in the permutation tests of both ion modes, the intercepts of the Q2Y fitted regression lines with the vertical axis were below 0, confirming the reliability of the OPLS-DA model and its suitability for identifying differential metabolites between groups (Figures 2C,D).
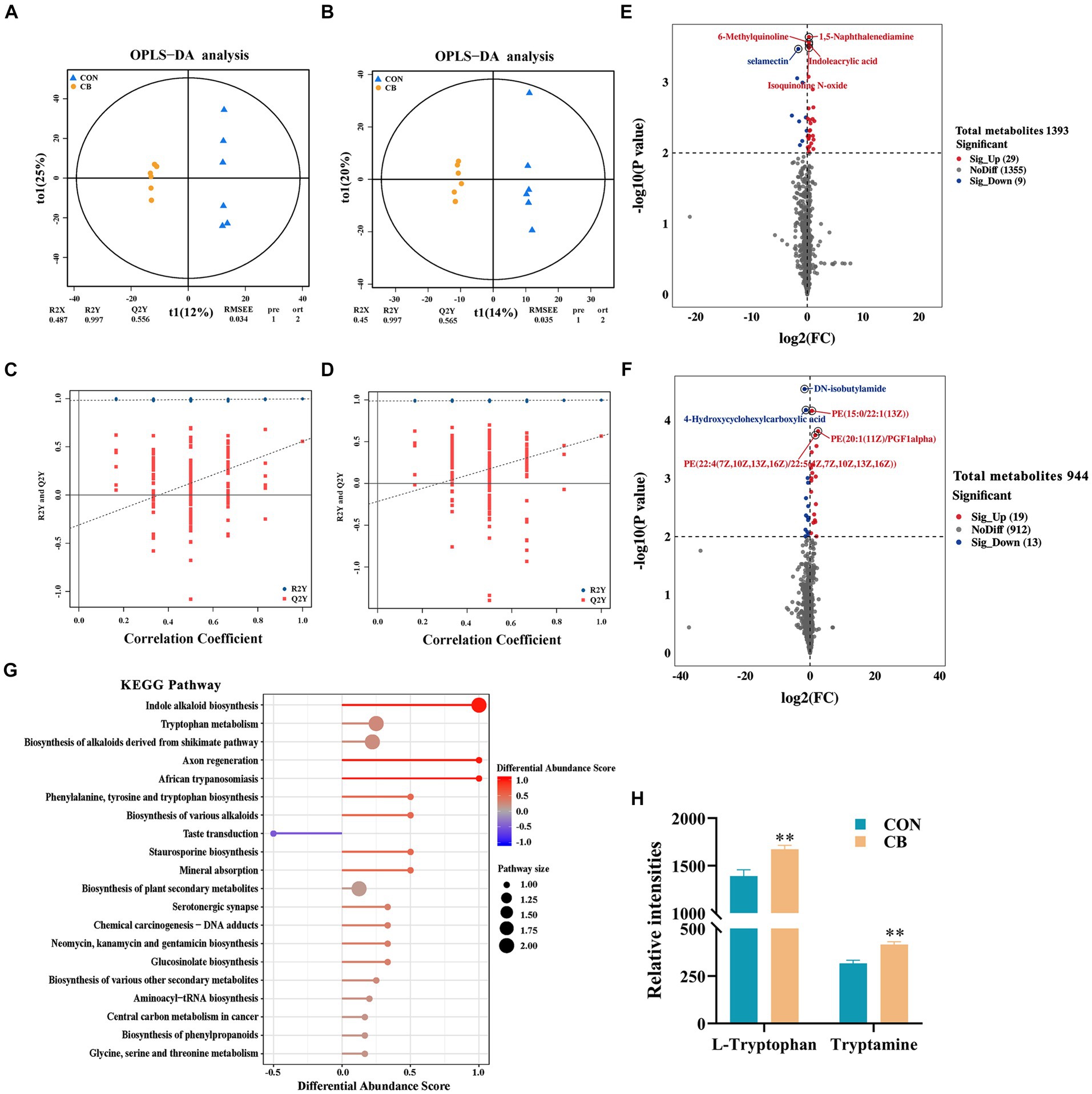
Figure 2. Effects of Clostridium butyricum CBX 2021 on serum metabolome in weaned piglets (n = 6). Orthogonal partial least squares discriminant analysis (OPLS-DA) score chart under positive (A) and negative (B) ion modes. Permutation test chart under positive (C) and negative (D) ion modes. Difference statistics volcano plot under positive (E) and negative (F) ion modes. (G) KEGG enrichment pathway diagram of different metabolites in two groups of piglets. (H) Histogram of the differential metabolites (L-tryptophan and tryptamine) that cause KEGG enrichment. Data are displayed as the mean ± SEM. ** Indicates p < 0.01 in comparison with the CON piglets.
A total of 70 differential metabolites were screened between the two groups of piglets, with 38 being positive ions and 32 being negative ions (Figures 2E,F and Supplementary Table S3). In the positive ion mode, the contents of 29 metabolites, such as 1,5-naphthalenediamine, 6-methylquinoline, indoleacrylic acid, and isoquinoline N-oxide, were significantly higher in the CB piglets than CON piglets, while the contents of 9 metabolites, such as selamectin, were significantly lower (p < 0.01). In the negative ion mode, the contents of 19 metabolites, such as PE(22:4(7Z,10Z,13Z,16Z)/22:5(4Z,7Z,10Z,13Z,16Z)), PE(20:1(11Z)/PGF1alpha), and PE(15:0/22:1(13Z)), showed a significant increase, while the contents of 13 metabolites, such as DN-isobutylamide and 4-hydroxycyclohexylcarboxylic acid, exhibited a significant decrease (p < 0.01).
Subsequently, an analysis of KEGG pathway enrichment was conducted on all differential metabolites found in the piglets from both groups. As shown in Figure 2G, CBX 2021 highly affected 5 pathways, including indole alkaloid biosynthesis, and tryptophan metabolism (p < 0.05). The significant increase in L-tryptophan and tryptamine mediated by CBX 2021 mainly contributed to the enrichment of these pathways (p < 0.05, Figure 2H). These outcomes show that CBX 2021 modulated tryptophan metabolism and biosynthesis of various alkaloids by altering the levels of metabolites, thus affecting the metabolic function of weaned piglets.
Correlation analysis of microbiota, differential metabolites and blood biochemical indicators
Metabolomics is considered an important tool for elucidating potential interactions between the microbiota and host phenotypes. Therefore, this study investigated the correlations between the top 20 bacterial genera and serum differential metabolites, along with the relationships between these metabolites and blood indicators. Figure 3A shows that in the CB piglets, upregulated genera (Faecalibacterium, unclassified_Bacteroidales, and Subdoligranulum) and downregulated genera (unclassified_Muribaculaceae and Rikenellaceae_RC9_gut_group) were highly correlated with differential metabolites. Specifically, Faecalibacterium showed positive correlations with upregulated metabolites in the CB piglets; these metabolites included 6-methylquinoline, indoleacrylic acid, isoquinoline N-oxide, L-tryptophan, and tryptamine (p < 0.05). Faecalibacterium exhibited negative correlations with downregulated metabolites in the CB piglets; these metabolites included selamectin, 4-hydroxycyclohexylcarboxylic acid, and DN-isobutylamide (p < 0.05). Interestingly, unclassified_Bacteroidales and Subdoligranulum showed similar trends to that of Faecalibacterium, while unclassified_Muribaculaceae and Rikenellaceae_RC9_gut_group showed opposite trends.
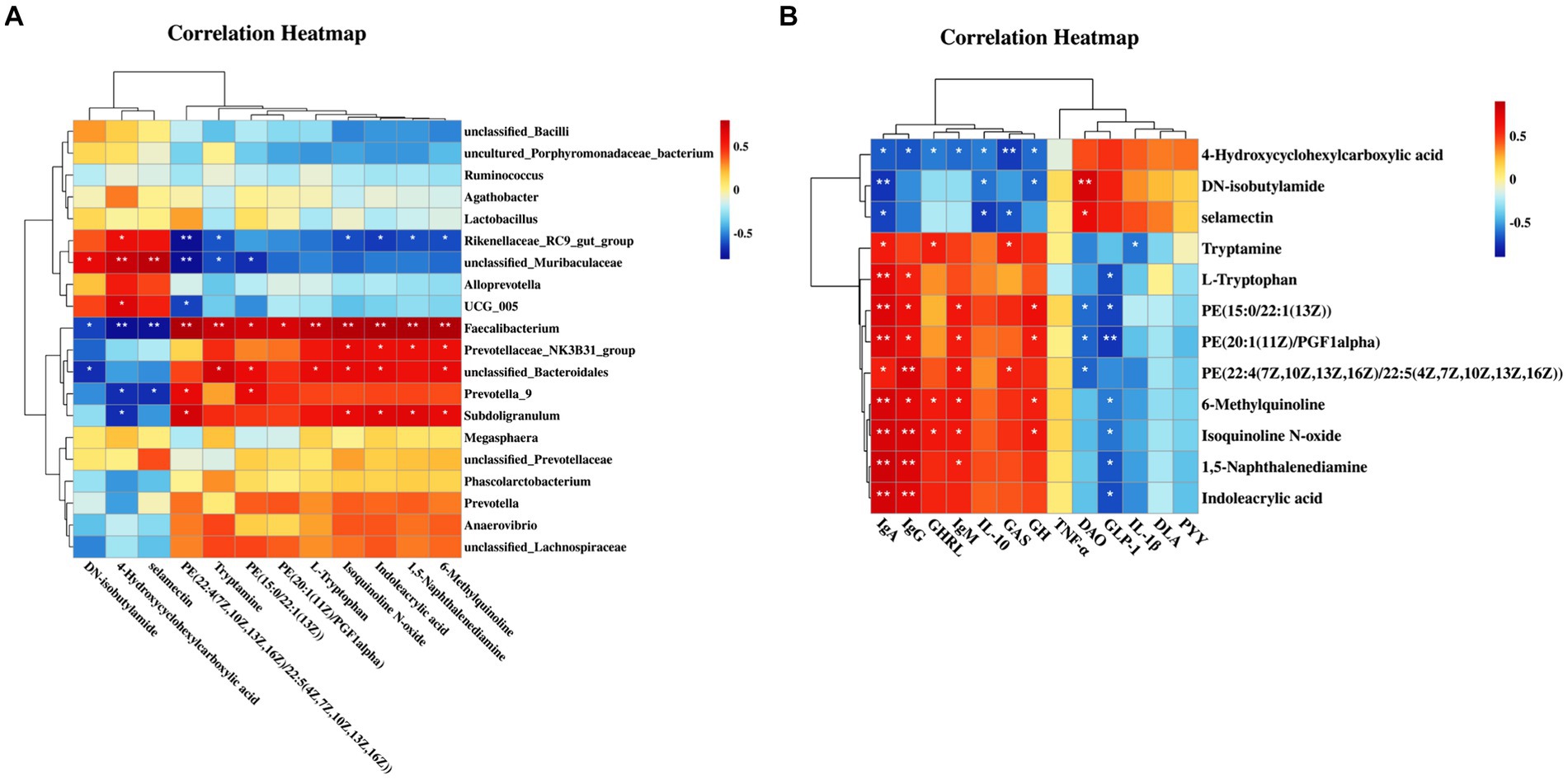
Figure 3. Correlation of microbiota, metabolites, and blood biochemical indicators (n = 6). (A) Heatmap of correlations between the top 20 bacterial genera and serum differential metabolites; The abscissa is the fecal bacteria with relative abundance of top 20; The ordinate is 12 serum differential metabolites. (B) Heatmap of correlations between serum differential metabolites and blood indicators; The abscissa is 12 serum differential metabolites; The ordinate is the blood biochemical indicators (immunoglobulin A/G/M, ghrelin, interleukin-10, growth hormone, gastrin, tumor necrosis factor-α, Glucagon-like peptide-1, diamine oxidase, interleukin-1β, peptide YY, D-lactic acid).
The correlation analysis results between differential metabolites and blood indicators are shown in Figure 3B. Serum immunoglobulins showed positive correlations with a majority of the upregulated metabolites in the CB piglets (p < 0.05), while they showed negative correlations with downregulated metabolites, especially 4-hydroxycyclohexylcarboxylic acid (p < 0.05). Regarding inflammatory factors, IL-1β was negatively correlated with tryptamine, and IL-10 was negatively correlated with selamectin, 4-hydroxycyclohexylcarboxylic acid, and DN-isobutylamide (p < 0.05). Notably, growth-related hormones, including GH, GHRL, and GAS, were consistent with the trend of immunoglobulin. Furthermore, in terms of indicators reflecting intestinal permeability, serum DAO activity showed positive correlations with the content of DN-isobutylamide and selamectin (p < 0.05), and it showed negative correlations with the content of PE(22:4(7Z,10Z,13Z,16Z)/22:5(4Z,7Z,10Z,13Z,16Z)), PE(20:1(11Z)/PGF1alpha), and PE(15:0/22:1(13Z)) (p < 0.05).
The aforementioned findings indicate that alteration in gut microbiota composition mediated by CBX 2021 intervention may positively influence hormone levels, immune responses and gut barrier function by regulating metabolic function, ultimately improving growth, development and overall health of piglets.
Adhesion of CBX 2021 promoted the proliferation and reduced the inflammatory response in IPEC-J2 cells
Adhesion of strains to the surface of intestinal epithelial cells (IEC) is crucial for achieving their maximum probiotic effect (Kainulainen et al., 2015). Therefore, we assessed the ability of the CBX 2021 strain to adhere to IPEC-J2 cells in this study. The results, shown in Figures 4A,B, indicate that after 2 h of co-culture, there was almost no bacterial adhesion. After 4 h of co-culture, the average bacteria amount of adhesion was 50.04 ± 2.74 CFU/cell. After 6 h of co-culture, the average bacterial adhesion decreased to 33.92 ± 2.48 CFU/cell. When co-cultured for 8 h, there was almost no detectable bacterial adhesion. These findings indicate that the adhesion capability of CBX 2021 to IPEC-J2 cells is time-dependent, reaching its peak at approximately 4 h of co-culture.
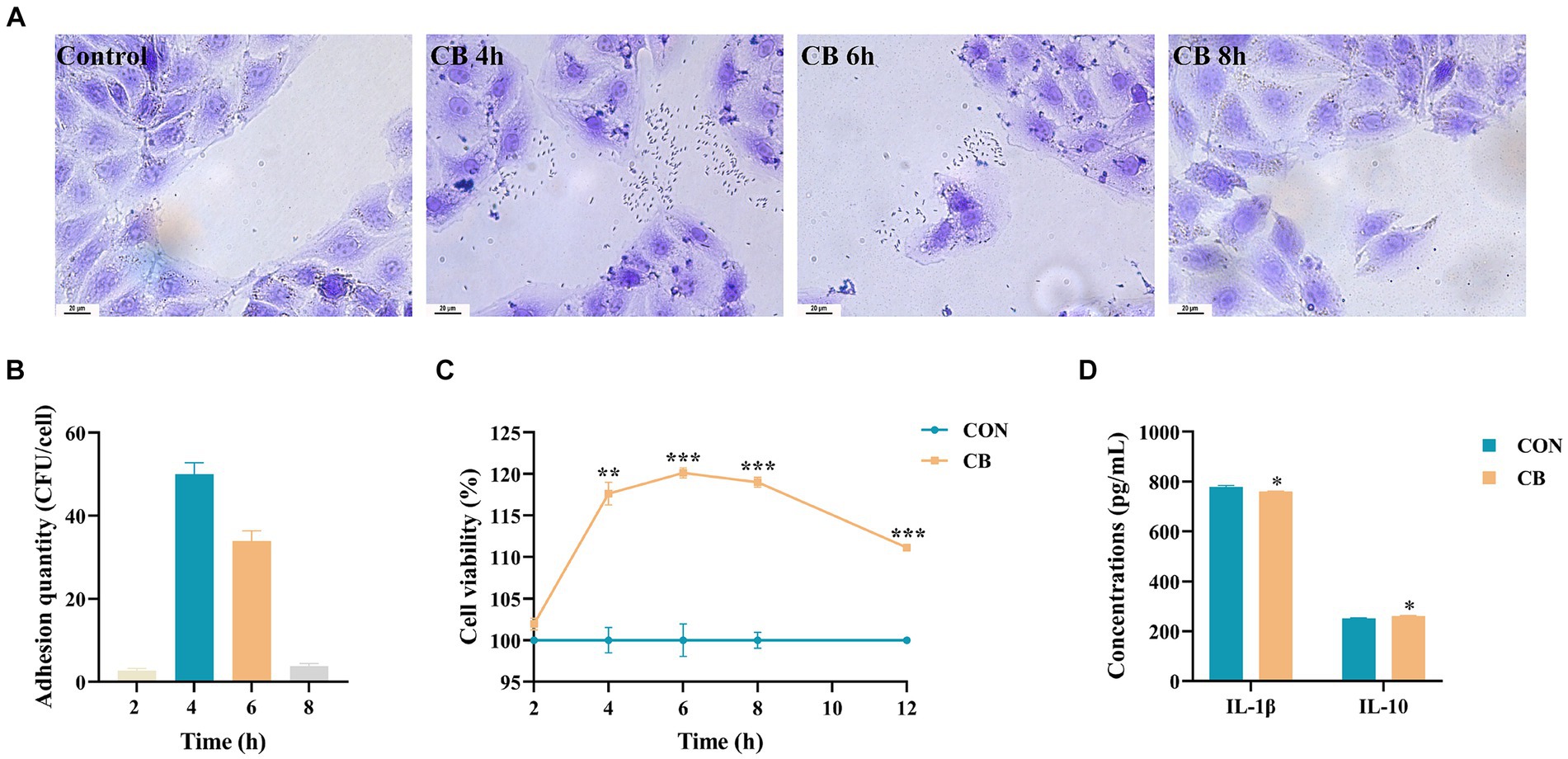
Figure 4. Effects of Clostridium butyricum CBX 2021 adhesion on the proliferation and inflammatory factors in IPEC-J2 cells (n = 25 or 3). (A) Gram staining microscopy of IPEC-J2 cells (scale bar = 20 μm). (B) Adhesion quantity of the CBX 2021 strain cocultured with IPEC-J2 cells at different time. (C) Line chart of cell proliferation activity. (D) Histogram showing the difference in inflammatory cytokine levels in the cell supernatant between two groups. Data are displayed as the mean ± SEM. *** Indicates p < 0.001, ** indicates p < 0.01, and * indicates p < 0.05 in comparison with the CON group.
The integrity of the intestinal barrier is dependent on the proliferation of IEC (Xie et al., 2019). Therefore, we investigated the impact of CBX 2021 adhesion on the proliferation activity of IPEC-J2 cells. The results in Figure 4C indicate that the strain adhesion did not significantly affect cell viability through 2 h of co-culture (p > 0.05). However, between 4 to 12 h of co-culture, CBX 2021 demonstrated a significant proliferative effect on IPEC-J2 cells (p < 0.01). As the co-culture time increased, cell viability gradually increased and reached its peak at 6 h, with a value of 120.14%. Subsequently, with a further increase in time, cell viability slightly declined, reaching 111.15% at 12 h. However, the CB group cells still demonstrated a significant proliferative effect on the cells at this time point (p < 0.001) compared to the CON group cells. The outcomes suggest that CBX 2021 significantly promoted IPEC-J2 cells proliferation, reaching peak activity after a 6-h co-culture. Therefore, a 6-h co-culture time was chosen for subsequent experiments.
To assess the potential anti-inflammatory properties of CBX 2021 in the intestine, we also analyzed the levels of inflammatory mediators in the cell supernatant. The data shows that CBX 2021 effectively decreased IL-1β levels, while enhancing IL-10 secretion in IPEC-J2 cells following 6 h of stimulation, (p < 0.05, Figure 4D). These findings are consistent with the in vivo experimental results, and thus suggesting that CBX 2021 can reduce the inflammatory response of IEC.
Adhesion of CBX 2021 altered inflammation and immune regulatory genes in IPEC-J2 cells
To delve deeper into the molecular mechanisms responsible for reducing inflammation in IPEC-J2 cells through CBX 2021 adhesion, RNA-seq analysis was conducted to confirm changes in the relative gene expression. The PCA scatter plot indicates that the two groups of cells formed distinct clusters, suggesting significant differences in gene expression profiles (Figure 5A). A total of 1892 DEGs were distinguished between the CON and CB groups. Among them, 1,387 genes, including TCIM, ZC3H12A, NFKBIE, and GPR20, showed a marked increase in the CB group compared to the CON group, while 505 genes, including KEAP1, PANK1, and CDCA7, were significantly downregulated (Figure 5B). The heatmap was generated using the top 100 genes with the smallest adjust p-value (Supplementary Figure S1). It is obvious that cell samples within the same group have high repeatability in gene expression. Notably, the TCIM and ZC3H12A genes were mapped to GO database and involved in various immune-related pathways. For instance, the TCIM gene was implicated in negative regulation of the Notch signaling pathway. The ZC3H12A gene is involved in activating immune response signals and negatively regulating the production of IL-1β, IL-6, and TNF. The GPR20 gene is involved in the G protein-coupled receptor (GPCR) signaling pathway (Supplementary Table S4).
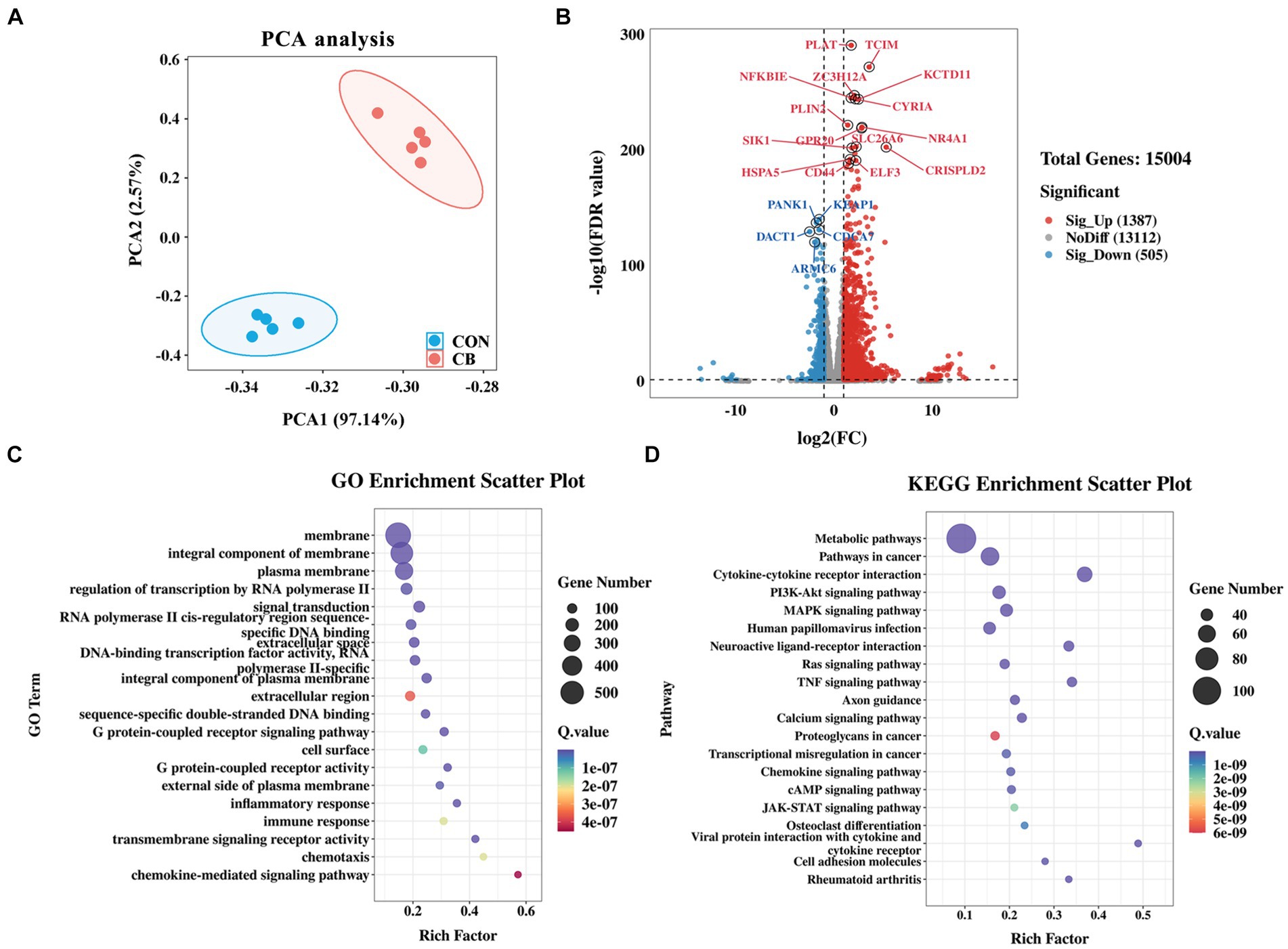
Figure 5. Effects of Clostridium butyricum CBX 2021 adhesion on the transcriptomics in IPEC-J2 cells (n = 5). (A) Principal component analysis (PCA) plot between the CON and CB RNA-seq samples. (B) The volcano plot displays differentially expressed genes (DEGs) from CON vs. CB. Bubble diagram of GO (C) and KEGG (D) enrichment analysis of differentially expressed genes.
Subsequently, enrichment analyses were carried out for all DEGs using GO and KEGG databases. The findings showed that numerous DEGs were significantly enriched in GPCR signaling pathway, inflammatory and immune response, which are related to cellular health and stress adaptation (Figure 5C). The KEGG pathway analysis revealed multiple immune and inflammatory pathways, including cytokine-cytokine receptor interaction (52 DEGs), PI3K-Akt signaling pathway (44 DEGs), JAK–STAT signaling pathway (23 DEGs), were altered by the adhesion of CBX 2021 (Figure 5D). By screening for these DEGs, we found that IL10RA and IL22RA1, which are anti-inflammatory cytokine receptors, were significantly upregulated in the CB-treated cells. In contrast, the pro-inflammatory gene IL17D was significantly downregulated in the cells.
To further reveal the details of changes in cellular immunity and inflammatory response, GESA was conducted using the Go database on all gene sets of the CON and CB groups. As shown in Figures 6A–F, adhesion of CBX 2021 significantly enhanced immune response (ES = 0.58, NES = 2.26), GPCR activity (ES = 0.55, NES = 2.17), and GPCR signaling pathway (ES = 0.55, NES = 2.27) in the cells. In addition, CBX 2021 was able to improve the negative regulation for the Notch signaling pathway (ES = 0.66, NES = 1.85), the negative regulation of the production of IL-6 (ES = 0.67, NES = 1.94) and the negative regulation of tumor necrosis factor (ES = 0.60, NES = 1.80). The changes in these gene sets supported the fact that CBX 2021 decreased pro-inflammatory cytokines like IL-1β in IPEC-J2 cells. It is obvious that this result interprets the molecular pathway for CBX adhesion to reduce cellular inflammation at the gene level.
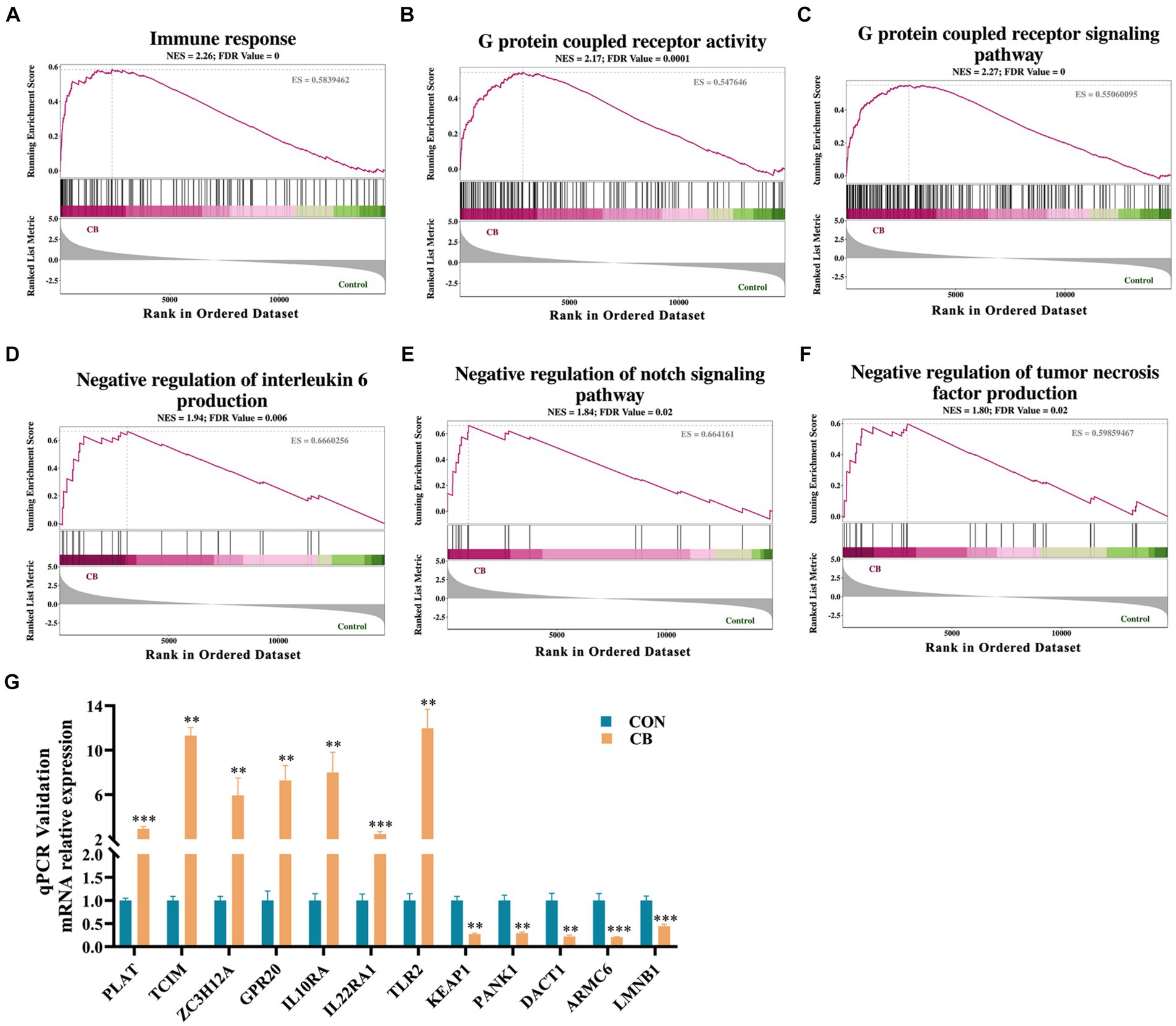
Figure 6. Gene set enrichment analysis (GSEA) pathway analysis and qRT-PCR validation (A–F) GSEA signal pathway analysis. (G) Histograms of target gene expression levels showed by qRT-PCR. Data are displayed as the mean ± SEM. *** Indicates p < 0.001 and ** indicates p < 0.01 in comparison with the CON group.
Next, the transcriptomic result was validated by using qRT-PCR method. Twelve genes (PLAT, TCIM, ZC3H12A, GPR20, IL10RA, IL22RA1, TLR2, KEAP1, PANK1, DACT1, ARMC6, and LMNB1) were subjected to qRT-PCR analysis. The findings indicated a high degree of concordance between the qRT-PCR data and transcriptomics results and thus confirming the reliability of the RNA sequencing (Figure 6G).
Discussion
Weaning is often associated with impaired immune and metabolic function, compromised small intestinal structure, and dysbacteriosis, leading to postweaning diarrhea and growth retardation. In the context of feed antibiotic bans, CB, an important member of the animal gut symbiotic bacteria, helps to alleviate weaning stress and improve overall health (Chen L. et al., 2018). In this study, we demonstrated that porcine-derived CBX 2021, which has independent intellectual property rights, had the ability to enhance growth rate and lower diarrhea frequency in piglets. This is achieved by modulating gut microbiota structure, optimizing metabolic functions, reducing intestinal permeability, enhancing intestinal and serum immune functions, and promoting IPEC-J2 cell proliferation.
In our study, the experiment demonstrated that supplementing CBX 2021 improved piglets’ growth performance. This aligns with earlier studies suggesting that CB can improve the well-being of weaned piglets, Ira rabbits and calves (Li et al., 2019; Ye et al., 2022; Wu et al., 2023). Clearly, these reports also reflect that improving intestinal health is effective in alleviating the growth stress in animals caused by weaning. In addition, the enhancement in growth performance may be attributed not solely to the amelioration in peripheral hormone levels, but also to the reduction in intestinal permeability, which contributes to improved digestion and absorption of nutrients within the intestine (Guilloteau et al., 2006; Goes et al., 2022; Lee et al., 2022). Post-weaning diarrhea, primarily resulting from an imbalance in intestinal microbiota and the rapid proliferation of pathogens, is the chief contributor to growth retardation in animals (Rhouma et al., 2017). Research findings have indicated a strong correlation between reduction of diarrhea and the subsequent improvement in growth performance, and factors such as immune, intestinal barrier integrity, gut microbiota, and bacterial metabolism (Lu et al., 2020; Zhang R. Q. et al., 2021; Yu et al., 2022; Chen et al., 2023). In this research, CBX 2021 administration effectively reduced postweaning diarrhea in piglets, confirming the efficacy of Clostridium butyricum in improving the gut microbiota and restoring gut health. Thus, the overall health and development of the CB piglets was better than that of the CON piglets.
CBX 2021 effectively enhanced immunity and reduced inflammation in piglets. Specifically, on the one hand, the increased serum immunoglobulin concentration of CB piglets suggests that the ability to resist pathogen infection may be stronger. This observation aligns with previous research (Zhang et al., 2014; Han et al., 2020; Wang et al., 2021). A previous study showed that CB can promote IgA secretion by inducing an increase in IL-17-producing CD4+ T cells (Hagihara et al., 2021). However, we found that the improvement in immunity may be related to the upregulated metabolites mediated by CBX 2021, including phosphatidylethanolamine (PE), L-tryptophan, and bacterial tryptophan metabolites. PE supplementation has been reported to effectively restore impaired humoral immune function (Fu et al., 2021). L-tryptophan and its derivatives have demonstrated roles in modulating host immunity and maintaining intestinal balance (Gao et al., 2018). Therefore, CBX 2021 may promote immunoglobulin secretion by positively regulating the metabolite profile. On the other hand, CBX 2021 significantly reduced IL-1β levels and promoted IL-10 production in piglets mirroring the results reported by Li et al. (2018). IL-1β is a typical inflammatory mediator, and its overexpression can damage the animal intestine (Kruse et al., 2008). Conversely, IL-10 serves as an anti-inflammatory regulator that not only inhibits the overexpression of proinflammatory factors, but also regulates intestinal homeostasis during immune defense processes (Gao et al., 2012; Zigmond et al., 2014; Xu S. Q. et al., 2020). Generally speaking, CB primarily regulates the secretion of inflammatory cytokines through Toll-like receptor (TLR) pathways, such as TLR4/NF-κB pathway and TLR2/MyD88-independent pathway (Hayashi et al., 2013; Wang et al., 2022).Then, we further uncovered the potential molecular pathways that contribute to anti-inflammatory properties of CBX 2021 through transcriptomics analysis. Interestingly, GSEA intuitively showed that CBX 2021 enhanced GPCR activity and its signaling pathway, while downregulating the Notch signaling pathway and the production of proinflammatory factors (IL-6 and TNF-α).
Research indicates that elevated levels of Notch signaling within the intestine can suppress goblet cell differentiation, causing a rise in intestinal permeability and invasion of exogenous harmful substances, thereby inducing inflammation (Xie et al., 2020). Probiotics, such as Bacillus subtilis RZ001 and Lactobacillus acidophilus LA85, can repair the intestinal barrier and alleviate inflammation through inhibiting the Notch signaling pathway (Li et al., 2020; Xue et al., 2022). These studies were strongly supported in the current research. Notably, GPCRs have the potential to regulate immune activity and anti-inflammatory properties. For example, the researcher discovered that activation of GPR39 led to an increase in IL-10 release, which ultimately reduced inflammation in macrophages (Muneoka et al., 2018). Furthermore, activation of GPR109A has been shown to inhibit downstream Akt and NF-κB p65 phosphorylation in macrophages, thereby maintaining the barrier integrity and suppressing colonic inflammation (Chen G. X. et al., 2018). Therefore, it is speculated that CBX 2021 may reduce inflammation by activating the GPCR pathway and downregulating the Notch signaling pathway, thereby effectively reducing intestinal diseases such as diarrhea. Moreover, we hypothesized that after CBX 2021 adheres to cells, it may induce changes in cellular inflammation levels and gene expression profiles through its cell wall component, lipoteichoic acid (LTA). Research has found that Clostridium butyricum LTA can affect intracellular inflammatory responses by mediating NF-κB and ERK pathways (Wang H. et al., 2016). However, this is merely a preliminary guess, and we plan to further explore this mechanism through additional experiments in future research.
Weaning-induced inflammation often disrupts intestinal barrier function, leading to negative impacts on growth, development and general well-being (Stewart et al., 2017). In the present research, CBX 2021 reduced intestinal permeability and ameliorated weaning-induced intestinal barrier damage by reducing DLA and DAO levels. This is similar to reports in broiler chickens afflicted with Escherichia coli K88 and rats experiencing pancreatitis (Zhang et al., 2016; Zhao et al., 2020). Reducing intestinal permeability has proven to enhance piglet growth performance and reduce the incidence of diarrhea (Huang et al., 2015). And the recovery of intestinal barrier function is related to the cytokine levels and the GPCR pathway activated by CB (Lee, 2015; Ariyoshi et al., 2021). With respect to cytokines, proinflammatory cytokines can impair intestinal tight junctions and increase permeability, whereas anti-inflammatory cytokines protect and maintain intestinal barrier integrity (Lee, 2015). CBX 2021 did indeed induce positive changes in cytokine levels. In addition, we found that the improvement in intestinal permeability may be related to CBX 2021-mediated increases in a number of PE metabolites. PE is a vital constituent of cell membranes and has been demonstrated to play a significant role in repairing damaged intestinal cells damaged intestinal cells (Sawai et al., 2001).
Based on this, we further explained the potential mechanism of CBX2021 at the cellular level. The outcomes indicated that the adhesion of the CBX 2021 strain significantly promoted the proliferation of IPEC-J2 cells. Intestinal cell proliferation is a major driving force for intestinal growth and development, which is beneficial for repairing damaged intestinal barriers and preventing intestinal infections (Wang J. L. et al., 2019). Previous research has shown that stimulating PI3K/Akt and GPR84 pathway can induce the multiplication of mammary epithelial cells and promote mammary gland development (Meng et al., 2017). In our study, CBX 2021 significantly enhanced GPCR activity and its signaling pathway, as well as regulated the PI3K/Akt signaling pathway. Therefore, CBX 2021 might promote intestinal cell proliferation by modulating the GPCR and PI3K/Akt signaling pathways, thereby exerting probiotic effects on piglet intestinal structure.
Undoubtedly, CBX2021 has a regulatory effect on piglets by inducing changes in their gut microbiota. In our research, CBX2021 optimized the composition and structure of the fecal microorganism in weaned piglets. Notably, CBX 2021 significantly decreased the number of proinflammatory bacteria, including unclassified_Muribaculaceae, Rikenellaceae_RC9_gut_group, and Family_XIII_AD3011_group. Studies have indicated that the lower the abundance of these bacteria, the less the content of pro-inflammatory factors including IL-1β, IL-5 and IL-6 (Cai et al., 2021; Zhang L. et al., 2021; Zhang et al., 2022). The decrease in IL-1β content observed in this study is consistent with these findings. Additionally, CBX 2021 markedly decreased the abundance of Desulfovibrio. The bacterium secretes toxic metabolites that harm IEC and immune function (Verstreken et al., 2012; Kushkevych et al., 2018). It is speculated that the decrease in these bacteria may be one of the reasons for the decrease in the incidence of diarrhea in piglets.
In addition, CBX 2021 enriched many functional bacteria that produce butyric acid, including Faecalibacterium, [Eubacterium]_eligens_group, and Lachnospiraceae_NK4A136_group. These bacteria serve as anti-inflammatory regulators and enhance intestinal barrier function (Dou et al., 2020; Xu J. H. et al., 2020; Tang S. S. et al., 2022). For instance, Faecalibacterium exerts anti-inflammatory actions by suppressing histone deacetylase activity through producing butyric acid (Zhou et al., 2018). The Lachnospiraceae_NK4A136_group has been shown to promote the expression of the tight junction protein claudin-1, which is negatively correlated with intestinal permeability and serum LPS levels (Ma et al., 2020). Additionally, CBX 2021 promoted the growth of helpful bacteria, including Prevotella_9 and Ligilactobacillus. Notably, Prevotella_9 has demonstrated a positive relationship with weight, ADG, and ADFI, and a negative relationship with diarrhea incidence (Hung et al., 2019; Yang et al., 2021). Therefore, CBX 2021 may have beneficial effects on weaned piglets by modulating the gut microbiota.
Alterations in the gut microbiome undoubtedly result in changes in metabolic function and levels of metabolites (Ariyoshi et al., 2021). These metabolites act as messengers of the intestinal microbiota, directly regulating various physiological processes in the host. CBX 2021 significantly enhanced the biosynthesis of various alkaloids, including indole alkaloids, and tryptophan metabolism. Studies have demonstrated that indole alkaloids exhibit a range of advantageous effects, such as anti-inflammatory, anti-pathogenic bacteria, and antioxidant properties (Song et al., 2018; Hu et al., 2021). Therefore, it is reasonable to believe that the reduction in proinflammatory mediators and harmful bacteria in the intestines of weaned piglets may be partly associated with these effects. Tryptophan and its derivatives, including kynurenine, indole, indoleacrylic acid, and tryptamine, play important roles in inflammatory responses (Kim et al., 2010; Bhattarai et al., 2020; Liu et al., 2022; Duan et al., 2023). Indoleacrylic acid, for example, can promote IL-10 production and enhance intestinal epithelial barrier function, resulting in anti-inflammatory effects (Wlodarska et al., 2017). In addition to regulating inflammation, tryptophan metabolism is also important for maintaining immune homeostasis, protecting intestinal integrity, and promoting animal growth (Yao et al., 2011; Liang et al., 2018; Seo and Kwon, 2023). It is worth noting that we found that the levels of L-tryptophan and tryptamine were positively correlated with the abundance of Faecalibacterium and unclassified_Bacteroidales. Therefore, CBX 2021 may boost tryptophan metabolism through the increased proliferation of these bacteria. This can positively regulate inflammation, immune function, and intestinal barrier function, ultimately promoting healthy growth of piglets.
Conclusion
In conclusion, exogenous administration of CBX 2021 effectively reduced postweaning diarrhea and accelerated growth of piglets through enhancing immune function and intestinal barrier integrity. The mechanism is mainly related to the improvement of intestinal health by CBX 2021. CBX 2021 not only optimized gut microbiota structure and improved bacterial metabolic functions in piglets, and also directly promoted epithelial cell proliferation and reduced inflammation by altering cellular gene expression. Thus, the current research reveals the probiotic mechanism of Clostridium butyricum and establishes a theoretical foundation for the potential application of CBX 2021.
Data availability statement
The raw 16S rRNA sequencing data and transcriptome sequencing data presented in the study are deposited in the NCBI Sequence Read Archive (SRA), accession number PRJNA947735 and PRJNA948058.
Ethics statement
The animal study was approved by Ethics Committee of National Center of Technology Innovation for Pigs. The study was conducted in accordance with the local legislation and institutional requirements.
Author contributions
XL: Writing – review & editing, Writing – original draft, Visualization, Validation, Supervision, Methodology, Investigation, Formal analysis, Data curation, Conceptualization. XQ: Writing – review & editing, Validation, Methodology, Investigation, Funding acquisition, Formal analysis, Data curation. YY: Writing – review & editing, Validation, Methodology, Investigation, Formal analysis, Data curation. JW: Writing – review & editing, Validation, Methodology, Investigation, Formal analysis, Data curation. QW: Writing – review & editing, Validation, Methodology, Investigation, Funding acquisition. JL: Writing – review & editing, Validation, Methodology, Investigation. JH: Writing – review & editing, Validation, Investigation, Conceptualization. FY: Writing – review & editing, Validation, Investigation, Conceptualization. ZL: Writing – review & editing, Writing – original draft, Validation, Supervision, Investigation, Funding acquisition, Conceptualization. RQ: Writing – review & editing, Writing – original draft, Validation, Supervision, Resources, Project administration, Methodology, Investigation, Funding acquisition, Conceptualization.
Funding
The author(s) declare that financial support was received for the research, authorship, and/or publication of this article. This work was jointly supported by the National Natural Science Foundation of China (U21A20245 and 32272830), the Strategic Priority Research Program of the National Center of Technology Innovation for Pigs (NCTIP-XD/B04), and the Financial Resourced Program of Chongqing (23509J).
Conflict of interest
The authors declare that the research was conducted in the absence of any commercial or financial relationships that could be construed as a potential conflict of interest.
Publisher’s note
All claims expressed in this article are solely those of the authors and do not necessarily represent those of their affiliated organizations, or those of the publisher, the editors and the reviewers. Any product that may be evaluated in this article, or claim that may be made by its manufacturer, is not guaranteed or endorsed by the publisher.
Supplementary material
The Supplementary material for this article can be found online at: https://www.frontiersin.org/articles/10.3389/fmicb.2024.1394332/full#supplementary-material
SUPPLEMENTARY FIGURE S1 | Cluster analysis of the top 100 DEGs with the smallest P-value.
Footnotes
References
Abdelqader, A., and Al-Fataftah, A.-R. (2016). Effect of dietary butyric acid on performance, intestinal morphology, microflora composition and intestinal recovery of heat-stressed broilers. Livest. Sci. 183, 78–83. doi: 10.1016/j.livsci.2015.11.026
Ariyoshi, T., Hagihara, M., Tomono, S., Eguchi, S., Minemura, A., Miura, D., et al. (2021). Clostridium butyricum MIYAIRI 588 modifies bacterial composition under antibiotic-induced dysbiosis for the activation of interactions via lipid metabolism between the gut microbiome and the host. Biomedicines 9, 1065–1083. doi: 10.3390/biomedicines9081065
Bhattarai, Y., Jie, S., Linden, D. R., Ghatak, S., Mars, R. A. T., Williams, B. B., et al. (2020). Bacterially derived tryptamine increases mucus release by activating a host receptor in a mouse model of inflammatory bowel disease. iScience 23, 101798–101823. doi: 10.1016/j.isci.2020.101798
Cai, J. R., Chen, H., Weng, M. L., Jiang, S. Y., and Gao, J. (2019). Diagnostic and clinical significance of serum levels of D-lactate and diamine oxidase in patients with Crohn's disease. Gastroent. Res. Pract. 2019, 8536952–8536959. doi: 10.1155/2019/8536952
Cai, B. N., Pan, J. Y., Chen, H., Chen, X., Ye, Z. Q., Yuan, H. B., et al. (2021). Oyster polysaccharides ameliorate intestinal mucositis and improve metabolism in 5-fluorouracil-treated S180 tumour-bearing mice. Carbohyd. Polym. 256, 117545–117554. doi: 10.1016/j.carbpol.2020.117545
Chen, L., Li, S., Zheng, J., Li, W. T., Jiang, X. M., Zhao, X. L., et al. (2018). Effects of dietary Clostridium butyricum supplementation on growth performance, intestinal development, and immune response of weaned piglets challenged with lipopolysaccharide. J. Anim. Sci. Biotechnol 9, 62–75. doi: 10.1186/s40104-018-0275-8
Chen, G. X., Ran, X., Li, B., Li, Y. H., He, D. W., Huang, B. X., et al. (2018). Sodium butyrate inhibits inflammation and maintains epithelium barrier integrity in a TNBS-induced inflammatory bowel disease mice model. EBioMedicine 30, 317–325. doi: 10.1016/j.ebiom.2018.03.030
Chen, J., Xu, X. W., Kang, J. X., Zhao, B. C., Xu, Y. R., and Li, J. L. (2023). Metasilicate-based alkaline mineral water confers diarrhea resistance in maternally separated piglets via the microbiota-gut interaction. Pharmacol. Res. 187, 106580–106593. doi: 10.1016/j.phrs.2022.106580
Dou, X. J., Gao, N., Yan, D., and Shan, A. S. (2020). Sodium butyrate alleviates mouse colitis by regulating gut microbiota dysbiosis. Animals (Basel) 10, 1154–1164. doi: 10.3390/ani10071154
Duan, X. Y., Luan, Y. M., Wang, Y. C., Wang, X. L., Su, P., Li, Q. W., et al. (2023). Tryptophan metabolism can modulate immunologic tolerance in primitive vertebrate lamprey via IDO-kynurenine-AHR pathway. Fish Shellfish Immun. 132, 108485–108492. doi: 10.1016/j.fsi.2022.108485
Fu, G. T., Guy, C. S., Chapman, N. M., Palacios, G., Wei, J., Zhou, P. P., et al. (2021). Metabolic control of TFH cells and humoral immunity by phosphatidylethanolamine. Nature 595, 724–729. doi: 10.1038/s41586-021-03692-z
Gao, Q. X., Qi, L. L., Wu, T. X., and Wang, J. B. (2012). An important role of interleukin-10 in counteracting excessive immune response in HT-29 cells exposed to Clostridium butyricum. BMC Microbiol. 12, 100–107. doi: 10.1186/1471-2180-12-100
Gao, J., Xu, K., Liu, H. N., Liu, G., Bai, M. M., Peng, C., et al. (2018). Impact of the gut microbiota on intestinal immunity mediated by tryptophan metabolism. Front. Cell Infect. Mi 8, 13–34. doi: 10.3389/fcimb.2018.00013
Goes, E. C., Dal Pont, G. C., Maiorka, A., Bittencourt, L. C., Bortoluzzi, C., Fascina, V. B., et al. (2022). Effects of a microbial muramidase on the growth performance, intestinal permeability, nutrient digestibility, and welfare of broiler chickens. Poultry Sci. 101, 102232–102240. doi: 10.1016/j.psj.2022.102232
Guilloteau, P., Le Meuth-Metzinger, V., Morisset, J., and Zabielski, R. (2006). Gastrin, cholecystokinin and gastrointestinal tract functions in mammals. Nutr. Res. Rev. 19, 254–283. doi: 10.1017/s0954422407334082
Hagihara, M., Ariyoshi, T., Kuroki, Y., Eguchi, S., Higashi, S., Mori, T., et al. (2021). Clostridium butyricum enhances colonization resistance against Clostridioides difficile by metabolic and immune modulation. Sci. Rep. 11, 15007–15021. doi: 10.1038/s41598-021-94572-z
Han, Y. S., Tang, C. H., Li, Y., Yu, Y., Zhan, T. F., Zhao, Q. Y., et al. (2020). Effects of dietary supplementation with Clostridium butyricum on growth performance, serum immunity, intestinal morphology, and microbiota as an antibiotic alternative in weaned piglets. Animals (Basel) 10, 2287–2303. doi: 10.3390/ani10122287
Hayashi, A., Sato, T., Kamada, N., Mikami, Y., Matsuoka, K., Hisamatsu, T., et al. (2013). A single strain of Clostridium butyricum induces intestinal IL-10-producing macrophages to suppress acute experimental colitis in mice. Cell Host Microbe 13, 711–722. doi: 10.1016/j.chom.2013.05.013
Hu, Y., Chen, S. L., Yang, F., and Dong, S. (2021). Marine indole alkaloids-isolation, structure and bioactivities. Mar. Drugs 19, 658–708. doi: 10.3390/md19120658
Huang, C., Song, P. X., Fan, P. X., Hou, C. L., Thacker, P., and Ma, X. (2015). Dietary sodium butyrate decreases postweaning diarrhea by modulating intestinal permeability and changing the bacterial communities in weaned piglets. J. Nutr. 145, 2774–2780. doi: 10.3945/jn.115.217406
Hung, D. Y., Cheng, Y. H., Chen, W. J., Hua, K. F., Pietruszka, A., Dybus, A., et al. (2019). Bacillus licheniformis-fermented products reduce diarrhea incidence and alter the fecal microbiota community in weaning piglets. Animals (Basel) 9, 1145–1159. doi: 10.3390/ani9121145
Kainulainen, V., Tang, Y., Spillmann, T., Kilpinen, S., Reunanen, J., Saris, P. E., et al. (2015). The canine isolate Lactobacillus acidophilus LAB20 adheres to intestinal epithelium and attenuates LPS-induced IL-8 secretion of enterocytes in vitro. BMC Microbiol. 15, 4–11. doi: 10.1186/s12866-014-0337-9
Kim, C. J., Kovacs-Nolan, J. A., Yang, C., Archbold, T., Fan, M. Z., and Mine, Y. (2010). L-tryptophan exhibits therapeutic function in a porcine model of dextran sodium sulfate (DSS)-induced colitis. J. Nutr. Biochem. 21, 468–475. doi: 10.1016/j.jnutbio.2009.01.019
Kruse, R., Essen-Gustavsson, B., Fossum, C., and Jensen-Waern, M. (2008). Blood concentrations of the cytokines IL-1beta, IL-6, IL-10, TNF-alpha and IFN-gamma during experimentally induced swine dysentery. Acta Vet. Scand. 50, 32–38. doi: 10.1186/1751-0147-50-32
Kushkevych, I., Dordević, D., Vítězová, M., and Kollár, P. (2018). Cross-correlation analysis of the Desulfovibrio growth parameters of intestinal species isolated from people with colitis. Biologia 73, 1137–1143. doi: 10.2478/s11756-018-0118-2
Lee, S. H. (2015). Intestinal permeability regulation by tight junction: implication on inflammatory bowel diseases. Intest. Res. 13, 11–18. doi: 10.5217/ir.2015.13.1.11
Lee, L. R., Holman, A. E., Li, X., Vasiljevski, E. R., O'Donohue, A. K., Cheng, T. L., et al. (2022). Combination treatment with growth hormone and zoledronic acid in a mouse model of Osteogenesis imperfecta. Bone 159, 116378–116389. doi: 10.1016/j.bone.2022.116378
Li, H. H., Jiang, X. R., and Qiao, J. Y. (2021). Effect of dietary Bacillus subtilis on growth performance and serum biochemical and immune indexes in weaned piglets. J. Appl. Anim. Res. 49, 83–88. doi: 10.1080/09712119.2021.1877717
Li, H. H., Li, Y. P., Zhu, Q., Qiao, J. Y., and Wang, W. J. (2018). Dietary supplementation with Clostridium butyricum helps to improve the intestinal barrier function of weaned piglets challenged with enterotoxigenic Escherichia coli K88. J. Appl. Microbiol. 125, 964–975. doi: 10.1111/jam.13936
Li, J., Xie, K., Yang, J., Zhang, J., Yang, Q., Wang, P., et al. (2023). S100A9 plays a key role in Clostridium perfringens beta2 toxin-induced inflammatory damage in porcine IPEC-J2 intestinal epithelial cells. BMC Genomics 24:16. doi: 10.1186/s12864-023-09118-6
Li, Y. R., Zhang, T. X., Guo, C. C., Geng, M., Gai, S. L., Qi, W., et al. (2020). Bacillus subtilis RZ001 improves intestinal integrity and alleviates colitis by inhibiting the notch signalling pathway and activating ATOH-1. Pathog. Dis. 78:ftaa016. doi: 10.1093/femspd/ftaa016
Li, W. X., Zhou, S., Zhang, L. Y., Wang, N., Sun, Y. K., and Yonggen, Z. (2019). Effects of Clostridium butyricum on growth performance, serum biochemical indices, antioxidant capacity and immune function of weaning calves. Chin. J. Anim. Nutr. 31, 369–377. doi: 10.3969/j.issn.1006-267x.2019.01.044
Liang, H. W., Dai, Z. L., Kou, J., Sun, K. J., Chen, J. Q., Yang, Y., et al. (2018). Dietary L-tryptophan supplementation enhances the intestinal mucosal barrier function in weaned piglets: implication of tryptophan-metabolizing microbiota. Int. J. Mol. Sci. 20, 20–32. doi: 10.3390/ijms20010020
Liu, X., Qiu, X. Y., Yang, Y., Wang, J., Wang, Q., Liu, J. B., et al. (2023). Alteration of gut microbiome and metabolome by Clostridium butyricum can repair the intestinal dysbiosis caused by antibiotics in mice. iScience 26, 106190–106208. doi: 10.1016/j.isci.2023.106190
Liu, G. M., Tao, J. Y., Lu, J. J., Jia, G., Zhao, H., Chen, X. L., et al. (2022). Dietary tryptophan supplementation improves antioxidant status and alleviates inflammation, endoplasmic reticulum stress, apoptosis, and pyroptosis in the intestine of piglets after lipopolysaccharide challenge. Antioxidants (Basel) 11, 872–883. doi: 10.3390/antiox11050872
Livak, K. J., and Schmittgen, T. D. (2001). Analysis of relative gene expression data using real-time quantitative PCR and the 2−ΔΔCT method. Methods 25, 402–408. doi: 10.1006/meth.2001.1262
Lu, J. J., Yao, J. Y., Xu, Q. Q., Zheng, Y. X., and Dong, X. Y. (2020). Clostridium butyricum relieves diarrhea by enhancing digestive function, maintaining intestinal barrier integrity, and relieving intestinal inflammation in weaned piglets. Livest. Sci. 239, 104112–104119. doi: 10.1016/j.livsci.2020.104112
Ma, L. Y., Ni, Y. H., Wang, Z., Tu, W. Q., Ni, L. Y., Zhuge, F., et al. (2020). Spermidine improves gut barrier integrity and gut microbiota function in diet-induced obese mice. Gut Microbes 12, 1832857–1832819. doi: 10.1080/19490976.2020.1832857
Mazzoni, M., Zampiga, M., Clavenzani, P., Lattanzio, G., Tagliavia, C., and Sirri, F. (2022). Effect of chronic heat stress on gastrointestinal histology and expression of feed intake-regulatory hormones in broiler chickens. Animal 16, 100600–100609. doi: 10.1016/j.animal.2022.100600
Meng, Y. Y., Zhang, J., Zhang, F. L., Ai, W., Zhu, X. T., Shu, G., et al. (2017). Lauric acid stimulates mammary gland development of pubertal mice through activation of GPR84 and PI3K/Akt signaling pathway. J. Agric. Food Chem. 65, 95–103. doi: 10.1021/acs.jafc.6b04878
Mun, D., Kyoung, H., Kong, M., Ryu, S., Jang, K. B., Baek, J., et al. (2021). Effects of Bacillus-based probiotics on growth performance, nutrient digestibility, and intestinal health of weaned pigs. J. Anim. Sci. Technol. 63, 1314–1327. doi: 10.5187/jast.2021.e109
Muneoka, S., Goto, M., Kadoshima-Yamaoka, K., Kamei, R., Terakawa, M., and Tomimori, Y. (2018). G protein-coupled receptor 39 plays an anti-inflammatory role by enhancing IL-10 production from macrophages under inflammatory conditions. Eur. J. Pharmacol. 834, 240–245. doi: 10.1016/j.ejphar.2018.07.045
Rhouma, M., Fairbrother, J. M., Beaudry, F., and Letellier, A. (2017). Post weaning diarrhea in pigs: risk factors and non-colistin-based control strategies. Acta Vet. Scand. 59, 31–49. doi: 10.1186/s13028-017-0299-7
Rodríguez-Viera, L., Martí, I., Martínez, R., Perera, E., Estrada, M. P., Mancera, J. M., et al. (2022). Feed supplementation with the GHRP-6 peptide, a ghrelin analog, improves feed intake, growth performance and aerobic metabolism in the gilthead sea bream Sparus aurata. Fishes (Basel) 7, 31–43. doi: 10.3390/fishes7010031
Sawai, T., Drongowski, R. A., Lampman, R. W., Coran, A. G., and Harmon, C. M. (2001). The effect of phospholipids and fatty acids on tight-junction permeability and bacterial translocation. Pediatr. Surg. Int. 17, 269–274. doi: 10.1007/s003830100592
Seo, S. K., and Kwon, B. (2023). Immune regulation through tryptophan metabolism. Exp. Mol. Med. 55, 1371–1379. doi: 10.1038/s12276-023-01028-7
Song, L. L., Mu, Y. L., Zhang, H. C., Wu, G. Y., and Sun, J. Y. (2018). A new indole alkaloid with anti-inflammatory from the branches of Nauclea officinalis. Nat. Prod. Res. 34, 2283–2288. doi: 10.1080/14786419.2018.1536130
Stewart, A. S., Pratt-Phillips, S., and Gonzalez, L. M. (2017). Alterations in intestinal permeability: the role of the leaky gut in health and disease. J. Equine Vet. 52, 10–22. doi: 10.1016/j.jevs.2017.02.009
Tang, S. S., Liang, C. H., Liu, Y. L., Wei, W., Deng, X. R., Shi, X. Y., et al. (2022). Intermittent hypoxia is involved in gut microbial dysbiosis in type 2 diabetes mellitus and obstructive sleep apnea-hypopnea syndrome. World J. Gastroenterol. 28, 2320–2333. doi: 10.3748/wjg.v28.i21.2320
Tang, X. P., Xiong, K. N., Fang, R. J., and Li, M. J. (2022). Weaning stress and intestinal health of piglets: a review. Front. Immunol. 13, 1042778–1042791. doi: 10.3389/fimmu.2022.1042778
Tang, Q. S., Yi, H. B., Hong, W. B., Wu, Q. W., Yang, X. F., Hu, S. L., et al. (2021). Comparative effects of L. plantarum CGMCC 1258 and L. reuteri LR1 on growth performance, antioxidant function, and intestinal immunity in weaned pigs. Front. Vet. Sci. 8, 728849–728858. doi: 10.3389/fvets.2021.728849
Verstreken, I., Laleman, W., Wauters, G., and Verhaegen, J. (2012). Desulfovibrio desulfuricans bacteremia in an immunocompromised host with a liver graft and ulcerative colitis. J. Clin. Microbiol. 50, 199–201. doi: 10.1128/JCM.00987-11
Wang, T. H., Fu, J., Xiao, X., Lu, Z. Q., Wang, F. Q., Jin, M. L., et al. (2021). CBP22, a novel bacteriocin isolated from Clostridium butyricum ZJU-F1, protects against LPS-induced intestinal injury through maintaining the tight junction complex. Mediat. Inflamm. 2021, 8032125–8032136. doi: 10.1155/2021/8032125
Wang, J., Ji, H. F., Wang, S. X., Liu, H., Zhang, W., Zhang, D. Y., et al. (2018). Probiotic Lactobacillus plantarum promotes intestinal barrier function by strengthening the epithelium and modulating gut microbiota. Front. Microbiol. 9, 1953–1966. doi: 10.3389/fmicb.2018.01953
Wang, J., Qi, L., Mei, L., Wu, Z., and Wang, H. (2016). C. butyricum lipoteichoic acid inhibits the inflammatory response and apoptosis in HT-29 cells induced by S. aureus lipoteichoic acid. Int. J. Biol. Macromol. 88, 81–87. doi: 10.1016/j.ijbiomac.2016.03.054
Wang, K. X., Wang, K., Wang, J. R., Yu, F., and Ye, C. (2022). Protective effect of Clostridium butyricum on Escherichia coli-induced endometritis in mice via ameliorating endometrial barrier and inhibiting inflammatory response. Microbiol. Spectr. 10, 1–12. doi: 10.1128/spectrum.03286-22
Wang, L. X., Yan, S. L., Li, J. Z., Li, Y. L., Ding, X. Q., Yin, J., et al. (2019). Rapid communication: the relationship of enterocyte proliferation with intestinal morphology and nutrient digestibility in weaning piglets. J. Anim. Sci. 97, 353–358. doi: 10.1093/jas/sky388
Wang, J. L., Zhang, T., Shen, X. T., Liu, J., Zhao, D. L., Sun, Y. W., et al. (2016). Serum metabolomics for early diagnosis of esophageal squamous cell carcinoma by UHPLC-QTOF/MS. Metabolomics 12, 116–125. doi: 10.1007/s11306-016-1050-5
Wang, H., Zong, Q., Wang, S., Zhao, C., Wu, S., and Bao, W. (2019). Genome-wide DNA methylome and transcriptome analysis of porcine intestinal epithelial cells upon deoxynivalenol exposure. J. Agric. Food Chem. 67, 6423–6431. doi: 10.1021/acs.jafc.9b00613
Wlodarska, M., Luo, C., Kolde, R., d’Hennezel, E., Annand, J. W., Heim, C. E., et al. (2017). Indoleacrylic acid produced by commensal Peptostreptococcus species suppresses inflammation. Cell Host Microbe 22, 25–37.e6. doi: 10.1016/j.chom.2017.06.007
Wu, Q., Cui, D., Chao, X., Chen, P., Liu, J., Wang, Y., et al. (2021). Transcriptome analysis identifies strategies targeting immune response-related pathways to control Enterotoxigenic Escherichia coli infection in porcine intestinal epithelial cells. Front. Veter. Sci. 8, 677897–677912. doi: 10.3389/fvets.2021.677897
Wu, J. M., Wang, J. P., Lin, Z. S., Liu, C. C., Zhang, Y. C., Zhang, S. M., et al. (2023). Clostridium butyricum alleviates weaned stress of piglets by improving intestinal immune function and gut microbiota. Food Chem. 405, 135014–135026. doi: 10.1016/j.foodchem.2022.135014
Xie, S., Jiang, L., Wang, M. J., Sun, W. J., Yu, S. Y., Turner, J. R., et al. (2020). Cadmium ingestion exacerbates Salmonella infection, with a loss of goblet cells through activation of notch signaling pathways by ROS in the intestine. J. Hazard. Mater. 391, 122262–122273. doi: 10.1016/j.jhazmat.2020.122262
Xie, S., Zhao, S. Y., Jiang, L., Lu, L. H., Yang, Q., and Yu, Q. H. (2019). Lactobacillus reuteri stimulates intestinal epithelial proliferation and induces differentiation into goblet cells in young chickens. J. Agr. Food Chem. 67, 13758–13766. doi: 10.1021/acs.jafc.9b06256
Xu, S. Q., Bao, W. J., Men, X. L., Liu, Y., Sun, J., Li, J., et al. (2020). Interleukin-10 protects schwann cells against advanced glycation end products-induced apoptosis via NF-κB suppression. Exp. Clin. Endocrinol. Diabetes 128, 89–96. doi: 10.1055/a-0826-4374
Xu, J. H., Liang, R. R., Zhang, W., Tian, K. Y., Li, J. Y., Chen, X. M., et al. (2020). Faecalibacterium prausnitzii-derived microbial anti-inflammatory molecule regulates intestinal integrity in diabetes mellitus mice via modulating tight junction protein expression. J. Diabetes 12, 224–236. doi: 10.1111/1753-0407.12986
Xue, L. Y., Li, Z. Q., Xue, J. B., Wang, H. T., Wu, T., Liu, R., et al. (2022). Lactobacillus acidophilus LA85 ameliorates cyclophosphamide-induced immunosuppression by modulating notch and TLR4/NF-κB signal pathways and remodeling the gut microbiota. Food Funct. 13, 8107–8118. doi: 10.1039/d1fo04331e
Yang, Y., Liu, Y. D., Liu, J., Wang, H. Z., Guo, Y. L., Du, M., et al. (2021). Composition of the fecal microbiota of piglets at various growth stages. Front. Vet. Sci. 8, 661671–661681. doi: 10.3389/fvets.2021.661671
Yao, K., Fang, J., Yin, Y. L., Feng, Z. M., Tang, Z. R., and Wu, G. Y. (2011). Tryptophan metabolism in animals: important roles in nutrition and health. Front. Biosci. 3, 286–297. doi: 10.2741/s152
Ye, X. X., Li, K. Y., Li, Y. F., Lu, J. N., Guo, P. T., Liu, H. Y., et al. (2022). The effects of Clostridium butyricum on Ira rabbit growth performance, cecal microbiota and plasma metabolome. Front. Microbiol. 13, 974337–974351. doi: 10.3389/fmicb.2022.974337
Yu, X. R., Cui, Z. C., Qin, S. K., Zhang, R. Q., Wu, Y. P., Liu, J. S., et al. (2022). Effects of Bacillus licheniformis on growth performance, diarrhea incidence, antioxidant capacity, immune function, and fecal microflora in weaned piglets. Animals (Basel) 12, 1609–1621. doi: 10.3390/ani12131609
Zhang, L., Cao, G. T., Zeng, X. F., Zhou, L., Ferket, P. R., Xiao, Y. P., et al. (2014). Effects of Clostridium butyricum on growth performance, immune function, and cecal microflora in broiler chickens challenged with Escherichia coli K88. Poult. Sci. 93, 46–53. doi: 10.3382/ps.2013-03412
Zhang, L., Jing, J. R., Han, L., Wang, J. Y., Zhang, W., Liu, Z. Y., et al. (2021). Characterization of gut microbiota, metabolism and cytokines in benzene-induced hematopoietic damage. Ecotoxicol. Environ. Saf. 228, 112956–112967. doi: 10.1016/j.ecoenv.2021.112956
Zhang, R. Q., Zhang, H. R., Liu, J. S., Zeng, X. F., Wu, Y. P., and Yang, C. M. (2021). Rhamnolipids enhance growth performance by improving the immunity, intestinal barrier function, and metabolome composition in broilers. J. Sci. Food Agr. 102, 908–919. doi: 10.1002/jsfa.11423
Zhang, L., Zhang, L. L., Zhan, X. A., Zeng, X. F., Zhou, L., Cao, G. T., et al. (2016). Effects of dietary supplementation of probiotic, Clostridium butyricum, on growth performance, immune response, intestinal barrier function, and digestive enzyme activity in broiler chickens challenged with Escherichia coli K88. J. Anim. Sci. Biotechnol. 7, 3–11. doi: 10.1186/s40104-016-0061-4
Zhang, H. Q., Zuo, Y. W., Zhao, H. C., Zhao, H., Wang, Y. T., Zhang, X. Y., et al. (2022). Folic acid ameliorates alcohol-induced liver injury via gut–liver axis homeostasis. Front. Nutr. 9, 989311–989326. doi: 10.3389/fnut.2022.989311
Zhao, H. B., Jia, L., Yan, Q. Q., Deng, Q., and Wei, B. (2020). Effect of Clostridium butyricum and butyrate on intestinal barrier functions: study of a rat model of severe acute pancreatitis with intra-abdominal hypertension. Front. Physiol. 11, 561061–561073. doi: 10.3389/fphys.2020.561061
Zhou, L. X., Zhang, M. M., Wang, Y. M., Dorfman, R. G., Liu, H., Yu, T., et al. (2018). Faecalibacterium prausnitzii produces butyrate to maintain Th17/Treg balance and to ameliorate colorectal colitis by inhibiting histone deacetylase 1. Inflamm. Bowel Dis. 24, 1926–1940. doi: 10.1093/ibd/izy182
Keywords: Clostridium butyricum CBX 2021, intestinal microbiota, intestinal health, weaned piglets, IPEC-J2 cells
Citation: Liu X, Qiu X, Yang Y, Wang J, Wang Q, Liu J, Huang J, Yang F, Liu Z and Qi R (2024) Uncovering the mechanism of Clostridium butyricum CBX 2021 to improve pig health based on in vivo and in vitro studies. Front. Microbiol. 15:1394332. doi: 10.3389/fmicb.2024.1394332
Edited by:
François J. M. A. Meurens, University of Montreal, CanadaReviewed by:
Jie Yin, Hunan Agricultural University, ChinaShenglan Hu, Guangdong Academy of Agricultural Sciences, China
Copyright © 2024 Liu, Qiu, Yang, Wang, Wang, Liu, Huang, Yang, Liu and Qi. This is an open-access article distributed under the terms of the Creative Commons Attribution License (CC BY). The use, distribution or reproduction in other forums is permitted, provided the original author(s) and the copyright owner(s) are credited and that the original publication in this journal is cited, in accordance with accepted academic practice. No use, distribution or reproduction is permitted which does not comply with these terms.
*Correspondence: Zuohua Liu, bGl1enVvaHVhNjZAMTYzLmNvbQ==; Renli Qi, cWlyZW5saTE5ODJAMTYzLmNvbQ==