- 1Université Lyon 1, CNRS, VetAgroSup, Laboratoire de Biométrie et Biologie Evolutive, UMR 5558, Villeurbanne, France
- 2INSA Lyon, INRAE, BF2I, UMR203, Villeurbanne, France
- 3Izinovation SAS, Lyon, France
- 4Institut Universitaire de France, Paris, France
The common bed bug, Cimex lectularius, is a hemipteran insect that feeds only on blood, and whose bites cause public health issues. Due to globalization and resistance to insecticides, this pest has undergone a significant and global resurgence in recent decades. Blood is an unbalanced diet, lacking notably sufficient B vitamins. Like all strict hematophagous arthropods, bed bugs host a nutritional symbiont supplying B vitamins. In C. lectularius, this nutritional symbiont is the intracellular bacterium Wolbachia (wCle). It is located in specific symbiotic organs, the bacteriomes, as well as in ovaries. Experimental depletion of wCle has been shown to result in longer nymphal development and lower fecundity. These phenotypes were rescued by B vitamin supplementation. Understanding the interaction between wCle and the bed bug may help to develop new pest control methods targeting the disruption of this symbiotic interaction. The objective of this work was thus to quantify accurately the density of wCle over the life cycle of the host and to describe potential associated morphological changes in the bacteriome. We also sought to determine the impact of sex, feeding status, and aging on the bacterial population dynamics. We showed that the relative quantity of wCle continuously increases during bed bug development, while the relative size of the bacteriome remains stable. We also showed that adult females harbor more wCle than males and that wCle relative quantity decreases slightly in adults with age, except in weekly-fed males. These results are discussed in the context of bed bug ecology and will help to define critical points of the symbiotic interaction during the bed bug life cycle.
1 Introduction
Bed bugs have undergone a major and worldwide resurgence in the number of infestations during the last decades (Doggett and Lee, 2023) due to globalization (Štefka et al., 2022), urbanization (Hwang et al., 2005), and selection of insecticide resistance (Dang et al., 2017). Bed bugs are mainly found in habitats associated with humans (Parola and Izri, 2020): individual or collective housing, habitats linked to tourism (e.g., hotels, seasonal rentals) or health (e.g., hospitals, retirement homes), but also places associated with culture (e.g., movie theaters) or transportation (e.g., trains). Bites lead to itchy lesions and rashes, and infestations can cause sleep disturbances, psychological distress, and stigma (Doggett et al., 2012). Thus, bed bugs are a growing health and socio-economic burden requiring new, alternative methods to insecticides to control their populations.
The common bed bug, Cimex lectularius, is a strict hematophagous pest insect. This hemimetabolous insect undergoes five nymphal stages before the final molt to adulthood. Each instar requires a blood meal to proceed to the molting in 1 week, and both sexes bite at the adult stage. Like other strict hematophagous insects or species living on a nutritionally unbalanced diet (Janson et al., 2008; Rio et al., 2017; Duron and Gottlieb, 2020), bed bugs have evolved a long-term association with endosymbionts providing essentials nutrients that they lack in their diet, notably sufficient B vitamins. As far as known, C. lectularius microbiota is composed of one to three endosymbionts, one of them being an obligate nutritional symbiont. The obligate endosymbiont is Wolbachia (wCle) (Sakamoto and Rasgon, 2006) and provides two essential B vitamins (i.e., biotin and riboflavin) (Hosokawa et al., 2010; Nikoh et al., 2014; Hickin et al., 2022). The experimental depletion of wCle results in a decrease in the number of laid eggs, a decrease in hatching rate, an increase in nymphal development, and a smaller adult size (Chang, 1974; Hosokawa et al., 2010; Hickin et al., 2022). The two facultative endosymbionts are a γ-proteobacterial endosymbiont (Hypša and Aksoy, 1997; Hosokawa et al., 2010) of the Symbiopectobacterium family, known as BEV-like, for “Bacterial symbiont of Euscelidius variegatus” (Degnan et al., 2011; Nadal-Jimenez et al., 2022) and a torix Rickettsia (Potts et al., 2020; Thongprem et al., 2020; Pilgrim et al., 2021). Little is known about these facultative endosymbionts, but their effect on fitness appears limited (Hosokawa et al., 2010; Thongprem et al., 2020). All three endosymbionts are present in the ovaries, which ensure their vertical transmission through eggs (Hosokawa et al., 2010; Thongprem et al., 2020). Endosymbionts are also observed in specialized host cells called bacteriocytes. These cells form oblong-shaped organs, the bacteriomes, which are localized on the left and right side of the insect, between the fourth and fifth abdominal tergites (Hertig and Wolbach, 1924; Chang and Musgrave, 1973; Hosokawa et al., 2010; Thongprem et al., 2020).
Disrupting the nutritional symbiosis with the obligate endosymbiont wCle could therefore be a promising control method, for instance by inducing a break-down in symbiosis or a stop in metabolic supplementation. To develop such a control strategy, it is essential to understand the dynamics of wCle throughout the insect development, and as a function of sex and feeding. Previous studies have reported the presence of wCle in the eggs, the first instars, and in the adults of both sexes (Hosokawa et al., 2010; Thongprem et al., 2020). wCle relative quantity was shown to be higher in the fifth instar stage than in the first nymphal stage and to decrease in adult females starved for at least 21 days (Fisher et al., 2018). These results raise the question of the impact of development and natural aging (independent of starvation), but also of the dynamics of wCle in males. Here, we thus present a detailed characterization of the interaction between C. lectularius and its nutritional obligatory endosymbiont wCle using cohorts. We describe the dynamics of wCle load in C. lectularius, and the effect of feeding, at all nymphal stages. We also document the development of the bacteriome during nymphal development. Finally, we quantify the evolution of the relative quantity of wCle after adult emergence and specifically test the impacts of sex, aging, and starvation on the relative quantity of wCle in adults.
2 Methods
2.1 Insects, feeding and rearing
The “F4” strain of C. lectularius was sampled in London (UK) in 2008 (Thongprem et al., 2020). Isofemale lines were created immediately after sampling and were reared on human blood in Oliver Otti’s lab (Dresden, Germany) until 2021. For our experiments, we used three of these isofemale lines, provided by O. Otti: F4-V1, F4-V6, and F4-V48. These lines were confirmed by PCR to be negative for torix Rickettsia and are positive for BEV-like. We fed bed bugs on the Hemotek® system with human blood provided by the Etablissement Français du Sang [blood group: O or A with 17 IU.mL Sodium Heparin (BD Vacutainer); temperature: 36.5 ± 0.5°C; membrane: parafilm®]. Colonies were maintained in round plastic jars containing corrugated cardboard harborage shelters (41 mm × 30 mm), at 24°C, 60% relative humidity, and with a photoperiod of 12 L:12D.
2.2 Cohort generation and sampling for bacterial dynamics in nymphs
Couples from either F4-V1, F4-V6, and F4-V48 lines were fed weekly on 1 mL of human blood for 30 min. The cohorts were generated as follows: fed couples were isolated in 24-well plates to mate and oviposit for 5 days, then males were removed. After 2 weeks, females were removed, and nymphs were gathered in round plastic jars containing corrugated cardboard harborage shelters. For each cohort and each nymphal stage, unfed (UF) nymphs (1 to 3 nymphs per cohort) were sampled from the first nymphs hatched (#1 in Supplementary Figure S1) and the remaining nymphs were fed. Nymphs that did not feed were discarded. Sampling was completed at each nymphal stage by collecting nymphs one-day post-feeding (1DPF, #2 in Supplementary Figure S1), and five-days post-feeding (5DPF, #3 in Supplementary Figure S1). Molting to the next instar occurred 1 week after the last meal; nymphs that did not molt were discarded, and samplings were performed (UF, 1DPF, 5DPF) as described previously for the following stages (second, third, fourth, and fifth instar). Bed bugs were frozen immediately after collection and kept at −20°C until DNA extraction. A total of 168 nymphs were sampled, originating from 6 cohorts (1 F4-V1, 2 F4-V6, and 3 F4-V48).
2.3 Cohort generation and sampling for bacterial dynamics in adults
Nymphs at the fifth instar were taken from either F4-V1, F4-V6, and F4-V48 lines and were fed on 1 mL of human blood for 30 min. To quantify Wolbachia density over the nymph-to-adult transition, 5DPF fifth instars were sampled for both sexes, the sex being determined at that stage by a combination of approaches described previously in Langer et al. (2020). One week after the fifth instar feeding, residual nymphs were discarded.
To determine the effect of sex, feeding, and aging on Wolbachia density, 1DPF male and female adults were sampled, and populations were divided equally into two round plastic jars. One jar was fed weekly (condition: weekly feeding), while the other one was not fed (condition: starvation after feeding on day 1). Each week, for 4 weeks, males and females of both jars were sampled at the date corresponding to 1DPF for the fed population (Supplementary Figure S2). Bed bugs were frozen immediately after collection and kept at −20°C until DNA extraction. A total of 146 fifth instars and adults were sampled, originating from 6 cohorts (1 F4-V1, 2 F4-V6, and 3 F4-V48).
2.4 DNA extraction
DNA was extracted using the MACHEREY-NAGEL NucleoSpin® 96 Tissue kit, following the protocol for genomic DNA from tissue, except for small individuals (i.e., from first to third instars). Small instar samples were placed in 0.2-mL tubes with three 1.5-mm stainless steel beads while large instar samples were placed in 2-mL tubes with one 5-mm diameter bead. All samples were left for 15 min at −80°C before being ground with a Tissue Lyzer (Retsch, Qiagen) for 2 min at 20 Hz. The following steps of the protocol followed the supplier’s recommendations, except for the small individuals. Indeed, for small individuals, the volume of lysis and wash buffers was divided by 3 compared to the recommendations and compared to the samples considered as large (i.e., from the fourth nymphal stage to the adult stage). For all samples, pre-lysis was carried out for 2 h at 56°C, washes were performed under vacuum, while elution was performed by centrifugation at 5600 g for 2 min with 100 μL of elution buffer. Eluted DNA was stored at −20°C until qPCR quantification.
2.5 Detection of symbionts using PCR amplification
Conventional PCR was used to verify the presence/absence of symbionts. The wCle 16S rRNA gene [INT2F, INT2R; 136 bp (Sakamoto and Rasgon, 2006)], the γ-proteobacteria 16S rRNA gene [BEV_F, BEV_R; 420 bp (Hosokawa et al., 2010)], the torix Rickettsia gltA gene [RiGltA405_F, RiGltA1193_R; 786 bp (Pilgrim et al., 2017)], and the C. lectularius ribosomal protein (RPL18) gene [RPL18F, RPL18R; 137 bp (Fisher et al., 2018)] were PCR-amplified as follows on a subset of unfed fifth instars (n = 10) and on one control sample known to be infected by the three symbionts. A 25-μL reaction containing 2.5 μL 10X DreamTaq® Green Buffer, 0.5 μL of dNTP (10 μM), 0.1 μL of DreamTaq® DNA Polymerase (5 U/μL), 0.5 μL of each primer set (10 μM) (primer sequences in Supplementary Table S1), and 2 μL of template DNA (previously diluted to 1/20) was prepared. All PCR reactions were performed in a Bio-Rad C1000 Touch™ thermal cycler with the following program: an initial denaturation step at 95°C for 5 min, followed by 35 cycles of 95°C for 30 s, Tm °C for 30 s and 72°C for 1 min. A final extension step of 72°C for 5 min was included. To visualize the amplicons, 5 μL of the PCR products were electrophoresed at 100 mV for 25 min (TBE 0.5X, 1% agarose, 0.5% BIOTIUM GelRed® Nucleic Acid Gel Stain).
2.6 Wolbachia quantification using real-time PCR
A real-time quantitative PCR (qPCR) duplex assay targeting the wCle-16S rDNA and the bed bug RPL18 gene was used to obtain relative quantification of wCle in each bed bug. The 10-μL reaction mix contained 5 μL SsoAdvanced™ Universal Probe Supermix (Bio-Rad), 500 nM of each forward and reverse primer, 300 nM of each probe, and 2 μL of extracted DNA previously diluted to 1/20. We used primers and probes previously described (Supplementary Table S1). The probes were provided by IDT DNA Technologies and included two quenchers, Iowa Black® at the 3′ end, and another internal quencher called ZEN™. Each probe also had a specific fluorophore at the 5’ end, FAM for wCle, and HEX for the RPL18 gene of C. lectularius. Real-time qPCR was performed in QuantStudio 6 Flex™ (Applied Biosystems) with the following program: an initial denaturation step at 95°C for 30 s, followed by 40 cycles of 95°C for 10 s, 60°C for 30 s and 72°C for 30 s. The qPCR assay efficiency (primer efficiency in Supplementary Table S1) was determined and confirmed in every run by constructing a standard curve using serial dilutions of a purified and quantified amplicon of each target. In addition, the specificity and absence of inhibitors in the samples were assessed upstream using dilutions of DNA extracts produced under the same conditions.
2.7 Statistical analysis of the relative quantity of Wolbachia
Each qPCR measurement was made in duplicate. Values for which the delta of quantification cycles (Cq) between replicates was greater than 0.5 cycles or the values of Cq were higher than 35 cycles were discarded [wCle-16S: 7.14% discarded (n = 12), RPL18: 5.36% discarded (n = 9; 8 in common with wCle-16S)]. Most of these discarded samples were young nymphal stages (71.42% of discarded are first instars).
The relative quantity (RQ) of Wolbachia within the insect was calculated based on equation one of Pfaffl (2001) where the ratio between the quantity of insect gene (reference) and wCle gene (target) relied on the PCR efficiency (E) and the number of Cq:
We used the R software (version 4.1.0) for all analyses (R Core Team, 2024) and the ggplot2 R package (version 3.4.4) for all plots (Wickham, 2016). To test the effect of different factors (i.e., development, feeding, sex) and their interactions on relative wCle quantities, we analyzed log10-transformed data using a linear mixed effect model with the lme4 R package (Bates et al., 2015).
For nymphal (N) samples, we tested the following model: lmer [log10(RQ) ~ Age_N + Feeding_State + Age_N:Feeding_State + (1|Cohort)], where Age_N is a quantitative variable associated to nymphal development (weeks), Feeding_State is a qualitative factor associated to blood feeding status (UF/1DPF/5DPF), and Cohort is a random factor linked to replicate sampling (A to F). Unfed (UF) first instars are considered as references in the model.
For adult (A) samples, because the feeding factor varies only after the adult’s first blood meal, we first tested on fifth instars 5DPF and adults 7DPF of both sexes (n = 26) the effect of development (fifth instar to adult) on the relative quantity in wCle in both sexes, with the following model: lmer [log10(RQ) ~ Age + Sex + Sex:Age + (1|Cohort)], using the fifth instar females as reference. We then tested on adults only the effect of aging, blood feeding, and sex, with the following model: lmer [log10(RQ) ~ Age_A + Sex + Feeding:Age_A + Sex:Age_A + Feeding:Sex:Age_A + (1|Cohort)], using the weekly fed-first week females as reference. Age and Age_A are quantitative variables associated with development and aging, respectively; Sex is a qualitative factor (female, male); Feeding is a qualitative factor associated to blood feeding status (fed, unfed), whose dynamics depends on the aging/duration of starvation; and Cohort is a random factor linked to replicate sampling (G to L).
Normality and homoscedasticity were checked graphically on residuals for each fitted model. Residuals were also checked for homogeneity of variance. Statistics for global effects of factors and their interactions are reported in Supplementary Tables S2, S4, S5. Model coefficients are reported with their mean ± confidence intervals (0.95). Interaction effects can be interpreted as an additive effect compared with the reference. Because calculating p-values from linear mixed effect model is complex and subject to controversy (Wasserstein and Lazar, 2016; Wasserstein et al., 2019), p-values estimated using the lmerTest R package (Kuznetsova et al., 2017) are only specified in Supplementary Tables S2, S4, S5.
2.8 Wolbachia localization using Fluorescence In situ Hybridization (FISH)
We documented the localization of wCle for each nymphal stage after molting (10 nymphs per instar) in the F4-V48 line, using the FISH technique (Thongprem et al., 2020). Tissues were dissected in 1X PBS and preserved immediately in Carnoy solution (chloroform: ethanol: glacial acetic acid = 6:3:1) overnight. All samples were cleared by incubation in 6% H2O2 in ethanol until the body was transparent, i.e., for at least 24 h for first and second instars, and up to 5 weeks for third to fifth instars. We then used a tungsten micro-needle to make micropores in the nymph cuticle to allow the fluorescence probes to diffuse through the cuticle during the hybridization step. The samples were hybridized by incubating the tissues overnight in a hybridization buffer (20 mM Tris–HCl pH 8.0, 0.9 M NaCl, 0.01% Sodium dodecyl sulfate 30% formamide) with 10 pmol/mL of rRNA specific probes for wCle (Hosokawa et al., 2010; probe sequences in Supplementary Table S1). After incubation, tissues were washed in buffer (0.3 M NaCl, 0.03 M sodium citrate, 0.01% sodium dodecyl sulfate) and mounted onto a slide using fluoro Gel with DABCO (Electron Microscopy Science) as a mounting medium. Slides were then observed under Leica THUNDER Imager 3D Assay epifluorescence microscope.
2.9 Evolution of bacteriome size over development
Length of bacteriome and thorax were measured on ImageJ 1.53c (Schneider et al., 2012) taking the maximum length (Lb) and width (Wb) of both bacteriomes, and the length (Lp) and width (Wp) at the middle of the pronotum. Relative bacteriome sizes were calculated by the ratio between pronotum estimated area (Lp ×Wp) and the mean estimated area (π × (1/2) Lb × (1/2) Wb) of both bacteriomes. We used the R software (version 4.1.0) for all analyses and plots (R Core Team, 2024).
To test the effect of age on absolute area of the bacteriome, we analyzed data using a Linear Model (LM). We tested the following model: lm (log10(Mean_area_bact) ~ Stage), where Stage is a qualitative factor associated to nymphal development (first to fifth instar) and Mean_area_bact is the mean of the estimated area of both bacteriomes in a nymph.
To test the effect of the age on the relative area of the bacteriome, we analyzed data using the following model: lm (Mean_ratio_area ~ Stage), where Mean_ratio_area is the mean of the estimated area of both bacteriomes relatively to the estimated area of the thorax in the nymph.
Normality and homoscedasticity were checked graphically on residuals for each fitted model. Residuals were checked for homogeneity of variance. LM statistics were given for global effects of factors. To compare the means of either the area or the relative size of the bacteriome between stages, we performed post-hoc Tukey tests (see Supplementary Table S3 for the complete output of each model).
2.10 Characterization of cryofixed bacteriomes using electro-microscopy (EM)
We performed the following manipulations at the Centre Technologique des Microstructures (CTμ, Université Claude Bernard Lyon 1, France). To avoid losing the samples during the procedure, bacteriomes of fifth instar females were dissected in PBS together with the proximal ovary and the surrounding cuticula. Samples were placed into specimen carriers previously covered with 0.5% lecithin in chloroform. Carriers were directly loaded into the HPM100 high pressure freezer and fast frozen. After freezing, samples were placed into a Leica EM AFS II Freeze substitution machine and incubated at -90°C for 30 h in substitution buffer (1% osmium tetroxide, 0.25% uranyl acetate, 0.5% glutaraldehyde, 1.5% H2O in acetone) and then gradually heated to -30°C (+5°C/h) and maintained at that temperature for 24 h. Samples were washed with -30°C cold acetone and then gradually heated to 20°C (+10°C/h). Samples were placed in 25% Epon™ (Epoxy substitute embedding medium kit from Sigma-Aldrich®) in acetone for 3 h, 50% Epon™ in acetone for 17 h, 75% Epon™ in acetone for 3 h, then in three different baths of pure Epon™ for a total of 24 h. Infiltration and embedding in 1.7% benzyl dimethyl amine in Epon™ resin were performed during 4 days at 60°C.
Sectioning was performed on Leica EM UC7 ultramicrotome using a diamond knife (Diatome) and mounted on uncoated copper grids. Ultra-thin sections (70 nm) for Transmission Electro-microscopy (TEM) were observed at 120 keV using a JEOL 1400 electron microscope. Electron micrographs of standard sections were taken with GATAN DigitalMicrograph software (Pleasanton, CA) and further analyzed using ImageJ 1.53c.
3 Results
3.1 The relative quantity of wCle increases exponentially over nymphal development
To analyze the dynamics of the obligatory endosymbiont wCle during nymphal development, we measured by qPCR duplex assay the relative quantity of wCle in 157 nymphs at different stages of development. wCle quantity was below the level of detection in 2.55% of the samples (n = 4), which were all first instars. We tested the effect of development and feeding status on wCle relative quantity on the remaining 153 samples, considering the variability observed between cohorts as a random effect in the model (Supplementary Figure S3).
The log10-transformed relative quantity of wCle linearly increased with age (Figure 1) with a slope equal to 0.68 ± 0.11 (confidence interval at 0.95), equivalent to an increase by 4.68 of the relative quantity of wCle at each nymphal stage; the log10-transformed absolute quantity of wCle, as measured by quantification of its 16S rDNA, increased ~2 times more strongly than that of the bed bug gene RPL18 genes (Supplementary Figure S4). This result confirms an increase in the wCle quantity relative to the number of host cells over nymphal development.
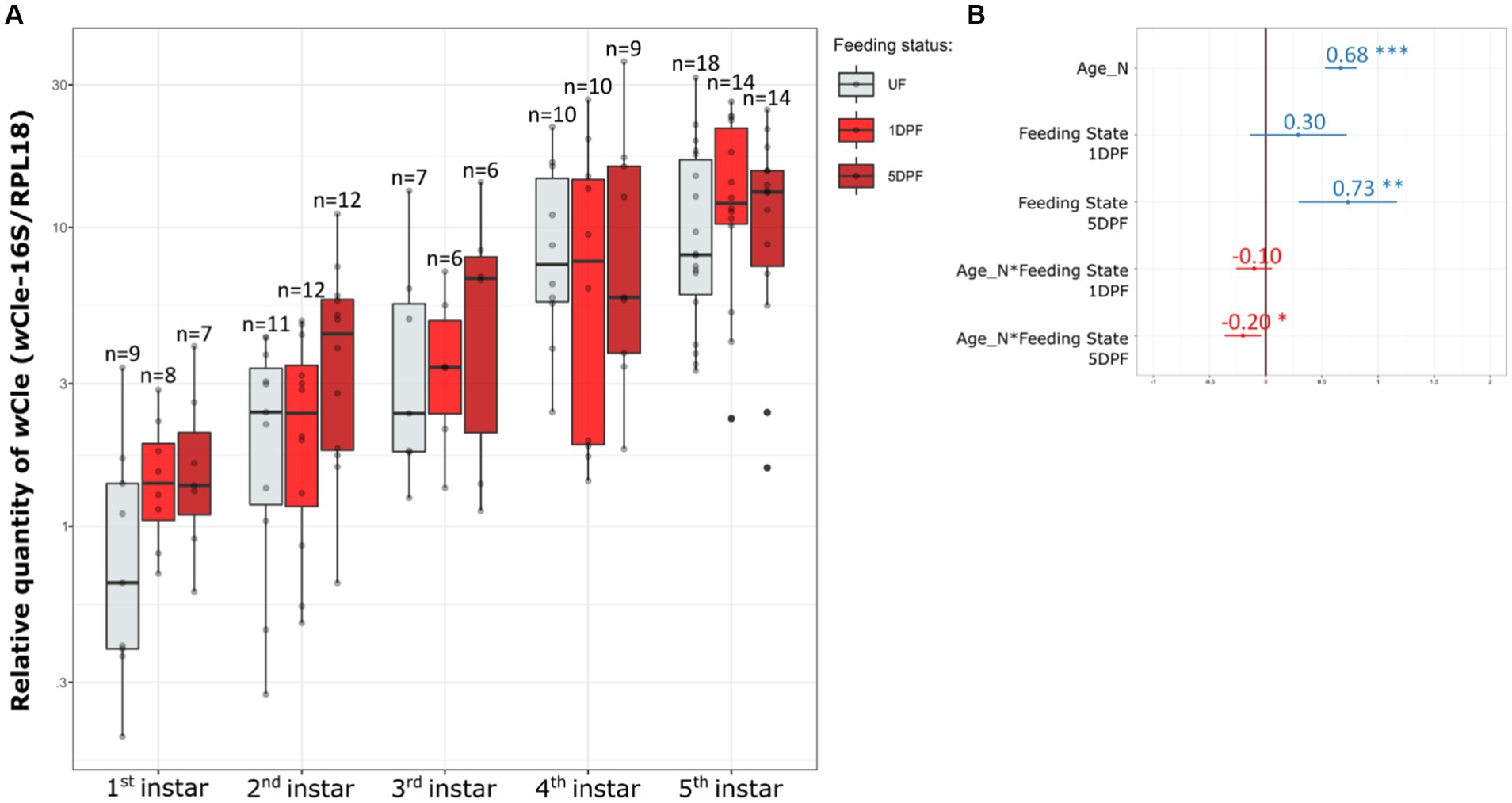
Figure 1. Dynamics of the wCle relative quantity in nymphal bed bugs over development and associated statistics. (A) wCle relative quantities of unfed (UF) newly molted nymphs, 1-day post-feeding nymphs (1DPF), and 5-day post-feeding nymphs (5DPF) are represented as boxplots for each nymphal instar. Each boxplot represents n = 6–18 individual nymphs (1 to 3 sampled nymphs per cohort). (B) Resume statistics of the mixed model log10(RQ) ~ Age_N + Feeding_State + Age_N:Feeding_State + (1|Cohort), where Age_N is a quantitative factor (number of weeks) and Feeding_State a qualitative factor (UF, 1DPF, 5DPF, with UF set as reference). Estimate values and their associated confidence intervals are indicated on the panel (blue when positive effect, red when negative effect). As example for this analysis: log10(RQ) increases by 0.68 ± 0.11 each week for UF individuals, log10(RQ) increases by 0.73 ± 0.45 between the UF reference and 5DPF, and log10(RQ) increases by 0.68–0.20 = 0.48 each week for 5DPF individuals. For detailed statistics, see Supplementary Table S2.
The blood feeding status also impacted the relative wCle quantity, with an increase of 0.73 ± 0.45 on log10-transformed relative quantity (i.e., equivalent to an increase by 5.37 of the relative quantity) between unfed and 5DPF (Days Post Feeding) nymphs. This positive impact of blood feeding on the wCle relative quantity dimmed as the nymph was growing (see interaction Age_N*Feeding State 5DPF Figure 1B).
3.2 The relative size of the bacteriome remains stable during nymphal development
To determine if the increase in wCle relative quantity is associated with a change in the bacteriome shape or an over-growth of endosymbiont within the bacteriome, we performed Fluorescence In situ Hybridization (FISH) using probes targeting the endosymbiont 16S rRNA. wCle was detected in bacteriomes of each sex at all instars during nymphal development (Figures 2A–F). All the bacteriocytes observed within a bacteriome were infected, and their cytoplasm was packed with wCle (Figures 2A–E; Supplementary Figure S5). TEM observation performed on fifth instar females confirmed that wCle exhibits a three-layer membrane presumably composed of the two membranes of the symbiont and an individual vacuolar membrane of insect origin similar to what has been described 50 years ago (Chang and Musgrave, 1973) (Figure 2G).
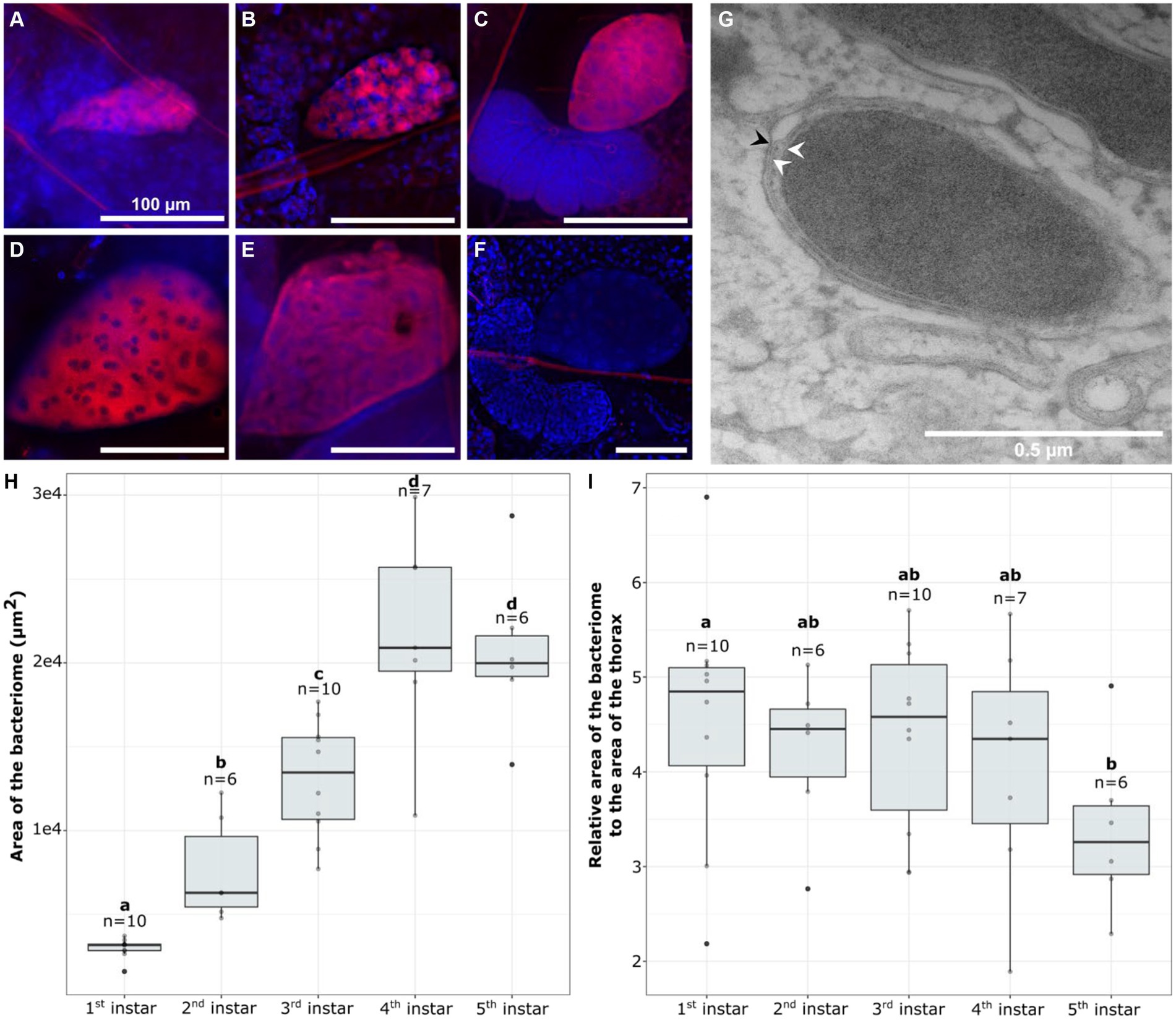
Figure 2. Visualization of the bacteriome and endosymbiont dynamics over bed bug development. (A–F) FISH visualization of wCle in bacteriomes (A) first instar; (B) second instar; (C) third instar; (D) fourth instar; (E) fifth instar; (F) Negative control (without probe) of a third instar bacteriome. In red: wCle; in blue: bacteriocyte nuclei (DAPI). Scale bars: 100 μm. (G) TEM of wCle in a fifth instar female. White arrows show the two inner membranes of the endosymbiont, while the black one shows the peripheric third vacuolar membrane. (H,I) Dynamics of the bacterial size over development: (H) absolute area or (I) relative area of the bacteriome at each nymphal instar (first to fifth). Each boxplot represents n = 6–10 individual nymphs (F4-V48 line). Boxplots sharing the same letter are not significantly different (t-test, p > 0.05). For statistics, see Supplementary Table S3.
To determine if the increase in wCle relative quantity is associated with an increase in symbiotic organ size, we measured the size of the bacteriome in 39 nymphs (n = 6–10/stage). While the bacteriome absolute area doubled at each stage between the first and fourth instars (Figure 2H), its relative area to the thorax remained unchanged between the different developmental stages (Figure 2I).
3.3 The dynamics of wCle is positively impacted by feeding in males but not in females
We first aimed to determine whether the increase in Wolbachia relative quantity during nymphal development persists after the last molt (Figures 3A–D). We thus measured the relative quantity of wCle in fifth instars (5DPF) and newly emerged adults (7DPF) a few days after feeding (data used symbolized by * on Figure 3C (females) and Figure 3D (males); statistics: Figure 3E). We showed a significant increase with development, as log10(RQ) of adult females increased by 0.63 ± 0.42 (equivalent to an increase by 4.57 of the relative quantity) compared to their fifth instar counterparts. No significant effect of sex on bacterial relative quantity was detected in this developmental window.
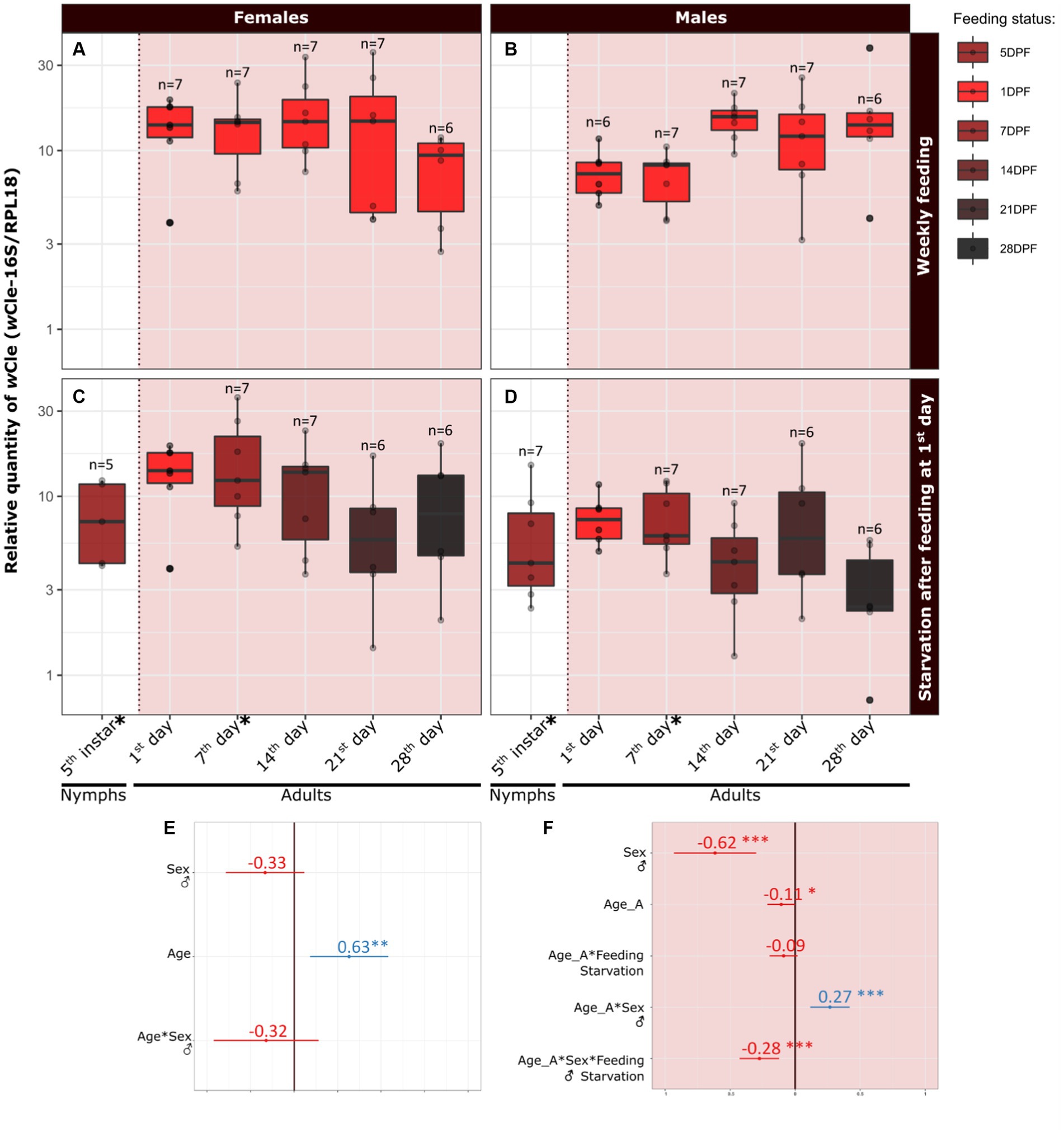
Figure 3. Dynamics of the relative quantity of wCle from the fifth instar to adulthood, and during 5 weeks of adulthood. Concerning the relative quantity dynamics during last metamorphosis (i.e., between fifth instar (5DPF) and adulthood (7DPF) according to sex), the dataset used is marked with an asterisk on panels C and D. This first dataset was analyzed using a linear mixed model: log10(RQ) ~ Age + Sex + Sex:Age + (1|Cohort), where Age is a quantitative factor (weeks) and Sex a qualitative factor (female, male; female set as reference). Resume statistics are indicated on panel (E) Concerning the relative quantity dynamics in adult bed bugs according to sex, aging and feeding, the dataset used is presented in the red squares on the right of the dashed lines: relative quantity of wCle in (A) females and (B) males fed every week (collection: 1DPF), or in (C) females and (D) males starved after the first-week meal (collected concomitantly with fed individuals). This latter dataset was analyzed using a linear mixed model log10(RQ) ~ Age_A + Sex + Feeding:Age_A + Sex:Age_A + Feeding:Sex:Age_A + (1|Cohort), where Age_A is a quantitative factor (weeks), Sex a qualitative factor (female set as reference) and Feeding is a qualitative factor (weekly fed, starved; weekly fed set as reference). Resume statistics are indicated on panel (F). Each boxplot represents n = 6–7 individuals. Estimate values and their associated confidence intervals are indicated on panels E and F, respectively for each statistical analysis (blue when positive effect, red when negative effect). For detailed statistics, see Supplementary Tables S4, S5.
We then focused on adults and determined the effect of sex, aging, and feeding on the relative quantity of wCle. We chose a weekly feeding protocol to mimic the natural feeding rate of bed bugs (Reinhardt and Siva-Jothy, 2007; Saveer et al., 2021). We thus measured the relative quantity of wCle weekly during 28 days after emergence, in weekly fed or starved adults of each sex (total = 119 adults) and analyzed data using a global statistical model (Figure 3F). The relative quantity of wCle slightly decreased with age in females for both feeding conditions (slope on log10-transformed data = −0.11 equivalent to a decrease of 0.78 each week; Figures 3A,C). In males, while the relative quantity of wCle also decreased with time in starved males (slope on log10-transformed data = 0.16–0.28 = −0.12, equivalent to a decrease of 0.76 each week; Figure 3D), it increased with time in weekly-fed males (slope on log10-transformed data = 0.27–0.11 = 0.16, equivalent to an increase of 1.44 each week; Figure 3B), showing complex interactions between sex, age, and feeding conditions. Overall, males had nevertheless significantly less wCle than females, as log10(RQ) of males was 0.62 ± 0.31 times less the one of females (equivalent to a 4,2-fold difference in relative quantity, Figures 3A–D,F). The log10-transformed absolute quantities of Wolbachia showed similar patterns (Supplementary Figure S6).
4 Discussion
In this study, we described the dynamics of wCle, a nutritional obligatory symbiont of bed bugs. We observed that: (a) the largest increase in relative wCle quantity occurred during the nymphal development; (b) this increase was exponential and positively impacted by the development of nymphal instars and the feeding status; (c) the size of the bacteriome increased isometrically with the development of the nymph; (d) except for continuously fed males, wCle relative quantity decreased slightly during the first 4 weeks of adulthood, independently of the feeding status.
This study shows that the increase in wCle relative quantity was exponential over nymphal development. Once adulthood was reached, wCle relative quantity started to slightly decrease, except in weekly-fed males. These data complete and corroborate the results obtained by Fisher et al. (2018), which showed that wCle relative quantity was higher in the fifth nymphal stage than in the first nymphal stage, but did not precisely quantify the dynamic between these two instars. Their data indicated an increase of ~1.6-fold in wCle relative quantity between the end of the first instar and the start of the fifth instar in Jersey City and Harold Harlan strains. The increase was stronger in our F4 strain, with a ~ 6.4-fold increase in relative quantity. In the adult stage, our data confirm the decrease they reported in starved females. However, a stronger tendency was observed in Fisher et al.’s work, as titers in three-week starved females approached the low titer observed in first instars, while in our study, the decrease in the wCle titer in four-week starved females only approached the titer measured in fifth instars. Fisher et al. (2018) observed that one of their two strains retained more wCle through starvation than the other. One possible explanation could be differences in the way strains respond to starvation, as we used here the F4 strain (Thongprem et al., 2020) that is different from the strains used in the work of Fisher et al. (2018).
In this study, the increase in wCle relative quantity in nymphs is associated with an increase in the absolute bacteriome size, but not in its relative size. These observations suggest an increase in the number of bacteria in each bacteriome and could result from two non-exclusive mechanisms: an increase in the bacteriocyte number, or an increase in the bacteriocyte size. The respective contribution of these two alternatives is difficult to evaluate in a species such as the bed bug. Indeed, bacteriocytes form very cohesive bacteriomes and it would require dissociating the organ in order to count and measure bacteriocytes. An increase in endosymbiont relative quantity from egg to adult stage (i.e., along nymphal, larval or pupal stages, depending on the insects) has been reported in several other nutritional endosymbiotic associations, such as the tsetse fly Glossinidia glossina/Wigglesworthia glossinidia (Rio et al., 2006), the cereal weevil Sitophilus oryzae/Sodalis pierantonius (Vigneron et al., 2014), or the pea aphid Acyrtosiphon pisum/Buchnera aphidicola (Simonet et al., 2016). In the pea aphid and the weevil, this increase was associated with a change in the number of the bacteriocytes or bacteriomes. In the A. pisum/B. aphidicola association, where bacteriocytes do not group into a cohesive bacteriome (Buchner, 1965; Douglas, 1989), the increase in the endosymbiont load during development was shown to correlate with both an increase in the quantity and in the size of bacteriocytes (Simonet et al., 2016, 2018). While bacteriocyte mitotic activity has not yet been reported, bacteriocyte enlargement and polyploidy has been proposed in several models to participate to bacteriome growth (Koch, 1959; Douglas, 1989; Moran et al., 1998; Baumann et al., 2006; Orr-Weaver, 2015; Alarcón et al., 2022; Nozaki and Shigenobu, 2022). Further experiments are needed to analyze the precise cellular mechanism of bacteriome growth in the bed bug. Alternatively, the increase in bacterial relative density could be linked to an increase in bacterial load in ovaries during development. To go further, it would be interesting to perform individual bacterial quantifications in bacteriomes and ovaries/testis. Unfortunately, it is technically very difficult to sex bed bugs and dissect bacteriomes in the early nymphal stages.
We noticed that blood intake had a positive effect (after 5 DPF) on the wCle relative quantity in nymphs. This could indicate that blood ingestion brings profuse nutritional elements for wCle proliferation. Based on this and previous works (Hosokawa et al., 2010; Nikoh et al., 2014; Hickin et al., 2022), the following succession of critical steps in the interaction could be considered: i) blood ingestion allows the increase in bacterial load, ii) this increase allows higher provision of B vitamins by the host, iii) the host molt once a critical threshold in B vitamin is reached, one week post blood-feeding. However, measuring the dynamic of wCle in week-starved nymphs will be required to demonstrate that it is indeed the blood ingestion that enhances the developmental increase in wCle, and that it is not simply due to aging. In the strict hematophagous hemipteran Rhodnius prolixus, the blood-meal stimulates the molt through humoral factors and neuronal signals generated by stretch receptors in the gut (Adams, 1999; Lange et al., 2022). Hence, another possibility would be that blood feeding in bedbugs impacts both the insect hormonal signaling regulating the molt and the bacterial load, coordinating an increase in bacterial quantity with the molting process.
After the increase in the wCle relative quantity in nymphs up to adult emergence, its decrease in both weekly fed and starved females is intriguing. The decrease observed in starved females does not reach the first instar’s quantity of wCle like in Fisher et al. (2018). Because wCle-synthetized B vitamins are necessary for fecundity and egg viability, we could expect either an increase or at least a steadiness in wCle relative quantity in sexually mature fed females [i.e., ~5 days post emergence (Mellanby, 1939; Johnson, 1941; Davis, 1964)]. While the same pattern was observed between starved adults and weekly fed females, the slow decrease of wCle could have different origins. In starved adults, nutritional scarcity could prevent the allocation of sufficient resources to endosymbiont growth or maintenance. Additionally, nutritional stress could trigger active mechanisms of endosymbiont elimination, including recycling of the symbionts for nutritive needs. For example, starved adults could engage in wCle lysis by autophagy to retrieve nutrients through endosymbiont digestion during periods of nutritional scarcity. This process, known as wolbophagy, drives the elimination of the few damaged Wolbachia in healthy cells under stress conditions, and has been described in Drosophila (Deehan et al., 2021; Hargitai et al., 2022) but not in Wolbachia-nutritional endosymbioses. Drastic endosymbiont clearance by autophagy has been described in several associations, such as in some aphids (Hinde, 1971), the whitefly (Wang et al., 2022), or the carpenter ant (Gonçalves et al., 2020). This phenomenon is particularly exemplified in cereal weevils where the endosymbiont S. pierantonius grows dynamically during early adulthood, and provides essential amino acids for the host cuticle synthesis (Vigneron et al., 2014; Dell’Aglio et al., 2023). However, these gut-associated endosymbionts are eliminated through apoptotic and autophagic mechanisms, starting 1 week after adult emergence, when the cuticle is fully synthetized and functional (Vigneron et al., 2014). The moderate decrease in wCle quantity we observed along 4 weeks of adulthood in the bed bug could be the result of a moderate and progressive wolbophagy, which does not exclude a more drastic elimination later in the life cycle. To note, the mechanisms of transfer of nutrients from the endosymbionts to the host, especially B vitamins, remain unidentified so far in the bed bug-wCle association, and could themselves rely on moderate wolbophagy, allowing the host to maintain a wCle population while “harvesting” the B vitamins from the endosymbionts accordingly to its physiological needs.
Surprisingly, the weekly fed females also present a slow decrease of wCle in the 4 weeks of observation, despite having abundant nutritional resources. However, this apparent abundance of nutritional resources must be put in perspective of their physiological needs. Indeed, weekly blood-feeding increases the mating rate (Mellanby, 1939; Johnson, 1941; Saveer et al., 2021). Mating is traumatic in bed bugs (Stutt and Siva-Jothy, 2001; Reinhardt et al., 2003; Reinhardt and Siva-Jothy, 2007); a high mating rate can thus enhance their induced immunity (Siva-jothy et al., 2019) and potentially impact wCle density regulation. Weekly blood-feeding also increases the number of laid eggs (Mellanby, 1939; Johnson, 1941; Saveer et al., 2021). This increased reproductive activity (mating and egg production) of weekly-fed females likely requires a higher metabolic investment that could constrain the resource allocation to the endosymbionts despite the recurrent host feeding. Finally, a part of wCle could be “lost” in the vertical transmission to the eggs. Counting the number of laid eggs per female and correlating them with the remaining quantity of endosymbionts in the mother’s ovaries could be a way to confirm the potential “loss” of wCle quantity by the mothers to the benefit of the transmission to progeny.
We also analyzed the wCle dynamics in males, which had not been previously studied. Surprisingly, after emergence, weekly-fed males exhibited an increasing dynamics of wCle relative quantity that contrasts with the decrease in wCle quantities observed in starved males, but also in starved or weekly-fed females. This increasing quantity in weekly fed males is even more intriguing in males, in which the impact of an elimination of wCle has a very limited effect on life history traits, such as the thorax size (Hickin et al., 2022). One hypothesis to explain the contrasting dynamics between weekly fed females and males could be that, while they both have access to food, they do likely not have similar metabolic needs, given the very costly investment the females make in reproduction. Therefore, wCle could maintain and proliferate more effectively in weekly-fed males due to a higher availability of metabolic resources in these individuals.
Altogether, our main hypothesis to explain the regulation of wCle behind the observed dynamics in adults would be that, in nutritional abundance conditions like weekly feeding, wCle can proliferate to fulfill the host’s needs. In contrast, during critical steps of host development, when the host’s metabolic needs are higher (i.e., mating, starvation, egg formation), the symbiotic cost is in detriment of wCle, whose quantity decreases. This decrease in quantity could be the result of either a decrease in proliferation due to nutritional scarcity, or an active recycling of endosymbionts by the host for example by wolbophagy. However, as described in other symbiotic associations, regulation of both wCle location and load could also involve active immune mechanisms such as production of reactive oxygen species (ROS) (Pan et al., 2012; Zug and Hammerstein, 2015) or antimicrobial peptides (AMP) (Login et al., 2011) by the host.
5 Conclusion
This work shows a dynamics of wCle that is positively impacted by blood ingestion during nymphal stages and in the adult males. This description of the dynamics of wCle along the life cycle of its host allows to unveil that feeding is a critical step in the interaction, which should be considered in future research to pinpoint the best stages to decipher the molecular dialog between partners and envision the development of new symbiocides.
Data availability statement
The raw data supporting the conclusions of this article will be made available by the authors, without undue reservation. Access to raw data and scripts: https://zenodo.org/records/10817678.
Ethics statement
The manuscript presents research on animals that do not require ethical approval for their study.
Author contributions
MP: Data curation, Formal analysis, Investigation, Methodology, Writing – original draft, Writing – review & editing. ER: Resources, Writing – review & editing. HH: Data curation, Methodology, Writing – review & editing, Investigation. SB: Methodology, Visualization, Writing – review & editing, Investigation. M-LD-M: Methodology, Writing – review & editing. AH: Conceptualization, Funding acquisition, Writing – review & editing. RL: Funding acquisition, Project administration, Supervision, Writing – review & editing. FV: Conceptualization, Funding acquisition, Project administration, Supervision, Validation, Writing – original draft, Writing – review & editing. AZ-R: Conceptualization, Funding acquisition, Project administration, Supervision, Validation, Writing – original draft, Writing – review & editing, Methodology. NK: Conceptualization, Formal analysis, Funding acquisition, Project administration, Supervision, Validation, Writing – original draft, Writing – review & editing, Data curation, Methodology.
Funding
The author(s) declare financial support was received for the research, authorship, and/or publication of this article. This work was funded by the CIFRE contract n°2019/1626, the Pack Ambition Recherche Rhone-Alpes SymBed (20 009583 01), the ANR PRC FBI (ANR-21-CE35-0011), the Scientific Breakthrough Project Micro-be-have (Microbial impact on insect behavior) of Université de Lyon within the program ‘Investissements d’Avenir’ (ANR-11-IDEX-0007; ANR-16-IDEX-0005), and the Institut Universitaire de France (AZ-R, junior member).
Acknowledgments
We thank Oliver Otti for providing us bed bugs. We thank the Symbiotron platform from the FR3728 BioEEnViS (and especially Angelo Jacquet) and the Equipex+ InfectioTron (ANR-21-ESRE-0023) for facilities and equipment for rearing and experimentation on bed bugs. TEM imaging was performed at Centre Technologique des Microstructures EZUS LYON - Université Claude Bernard Lyon1 (CTμ), and we thank Lucie Geay for the ultra-thin sectioning of the tissues. We thank the members of MP thesis comity (Olivier Duron, Delphine Destoumieux-Garzon and François Leulier) for fruitful discussions.
Conflict of interest
MP and RL were employed by Izinovation SAS, a company working on protection against invasive species, pests, pathogens and in the field of plant health.
The remaining authors declare that the research was conducted in the absence of any commercial or financial relationships that could be construed as a potential conflict of interest.
Publisher’s note
All claims expressed in this article are solely those of the authors and do not necessarily represent those of their affiliated organizations, or those of the publisher, the editors and the reviewers. Any product that may be evaluated in this article, or claim that may be made by its manufacturer, is not guaranteed or endorsed by the publisher.
Supplementary material
The Supplementary material for this article can be found online at: https://www.frontiersin.org/articles/10.3389/fmicb.2024.1386458/full#supplementary-material
References
Adams, T. S. (1999). Hematophagy and hormone release. Ann. Entomol. Soc. Am. 92, 1–13. doi: 10.1093/aesa/92.1.1
Alarcón, M. E., Polo, P. G., Akyüz, S. N., and Rafiqi, A. M. (2022). Evolution and ontogeny of bacteriocytes in insects. Front. Physiol. 13:1034066. doi: 10.3389/fphys.2022.1034066
Bates, D., Mächler, M., Bolker, B., and Walker, S. (2015). Fitting linear mixed-effects models using lme4. J. Stat. Softw. 67, 1–48. doi: 10.18637/jss.v067.i01
Baumann, P., Moran, N. A., and Baumann, L. (2006). “Bacteriocyte-associated endosymbionts of insects” in The Prokaryotes. eds. M. Dworkin, S. Falkow, E. Rosenberg, K. -H. Schleifer, and E. Stackebrandt (New York, NY: Springer)
Buchner, P. (1965) Endosymbiosis of animals with plant microorganisms. Inderscience Publishers. Geneva
Chang, K. P. (1974). Effects of elevated temperature on the mycetome and symbiotes of the bed bug Cimex lectularius (Heteroptera). J. Invertebr. Pathol. 23, 333–340. doi: 10.1016/0022-2011(74)90098-6
Chang, K. P., and Musgrave, A. J. (1973). Morphology, histochemistry, and ultrastructure of mycetome and its rickettsial symbiotes in Cimex lectularius L. Can. J. Microbiol. 19, 1075–1081. doi: 10.1139/m73-171
Dang, K., Doggett, S. L., Veera Singham, G., and Lee, C. Y. (2017). Insecticide resistance and resistance mechanisms in bed bugs, Cimex spp. (Hemiptera: Cimicidae). Parasit. Vectors 10, 1–31. doi: 10.1186/s13071-017-2232-3
Davis, N. T. (1964). Studies of the reproductive physiology of Cimicidae (Hemiptera)-I. Fecundation and egg maturation. J. Insect Physiol. 10, 947–963. doi: 10.1016/0022-1910(64)90083-6
Deehan, M., Lin, W., Blum, B., Emili, A., and Frydman, H. (2021). Intracellular density of wolbachia is mediated by host autophagy and the bacterial cytoplasmic incompatibility gene cifb in a cell type-dependent manner in drosophila melanogaster. MBio 12, 1–19. doi: 10.1128/mBio.02205-20
Degnan, P. H., Bittleston, L. S., Hansen, A. K., Sabree, Z. L., Moran, N. A., and Almeida, R. P. P. (2011). Origin and examination of a leafhopper facultative endosymbiont. Curr. Microbiol. 62, 1565–1572. doi: 10.1007/s00284-011-9893-5
Dell’Aglio, E., Lacotte, V., Peignier, S., Rahioui, I., Benzaoui, F., Vallier, A., et al. (2023). Weevil carbohydrate intake triggers endosymbiont proliferation: a trade-off between host benefit and endosymbiont burden. MBio 14, 1–14. doi: 10.1128/mbio.03333-22
Doggett, S. L., Dwyer, D. E., Peñas, P. F., and Russell, R. C. (2012). Bed bugs: clinical relevance and control options. Clin. Microbiol. Rev. 25, 164–192. doi: 10.1128/CMR.05015-11
Doggett, S. L., and Lee, C. Y. (2023). Historical and contemporary control options against bed bugs, Cimex spp. Annu. Rev. Entomol. 68, 169–190. doi: 10.1146/annurev-ento-120220-015010
Douglas, A. E. (1989). Mycetocyte symbiosis in insects. Biol. Rev. 64, 409–434. doi: 10.1111/j.1469-185X.1989.tb00682.x
Duron, O., and Gottlieb, Y. (2020). Convergence of nutritional symbioses in obligate blood feeders. Trends Parasitol. 36, 816–825. doi: 10.1016/j.pt.2020.07.007
Fisher, M. L., Watson, D. W., Osborne, J. A., Mochizuki, H., Breen, M., and Schal, C. (2018). Growth kinetics of endosymbiont Wolbachia in the common bed bug, Cimex lectularius. Sci. Rep. 8, 11444–11449. doi: 10.1038/s41598-018-29682-2
Gonçalves, W. G., Fernandes, K. M., Silva, A. P. A., Gonçalves, D. G., Fiaz, M., and Serrão, J. E. (2020). Ultrastructure of the Bacteriocytes in the midgut of the carpenter ant Camponotus rufipes: endosymbiont control by autophagy. Microsc. Microanal. 26, 1236–1244. doi: 10.1017/S1431927620024484
Hargitai, D., Kenéz, L., al-Lami, M., Szenczi, G., Lőrincz, P., and Juhász, G. (2022). Autophagy controls Wolbachia infection upon bacterial damage and in aging Drosophila. Front. Cell Dev. Biol. 10:976882. doi: 10.3389/fcell.2022.976882
Hertig, M., and Wolbach, S. B. (1924). Studies on Rickettsia-like micro-organisms in insects. J. Med. Res. 44, 329–374.7.
Hickin, M. L., Kakumanu, M. L., and Schal, C. (2022). Effects of Wolbachia elimination and B-vitamin supplementation on bed bug development and reproduction. Sci. Rep. 12, 10270–10214. doi: 10.1038/s41598-022-14505-2
Hinde, R. (1971). The control of the mycetome symbiotes of the aphids Brevicoryne brassicae Myzus persicae, and Macrosiphum rosae. J. Insect Physiol. 17, 1791–1800. doi: 10.1016/0022-1910(71)90076-X
Hosokawa, T., Koga, R., Kikuchi, Y., Meng, X. Y., and Fukatsu, T. (2010). Wolbachia as a bacteriocyte-associated nutritional mutualist. PNAS 107, 769–774. doi: 10.1073/pnas.0911476107
Hwang, S. W., Svoboda, T. J., de Jong, I. J., Kabasele, K. J., and Gogosis, E. (2005). Bed bug infestations in an urban environment. Emerg. Infect. Dis. 11, 533–538. doi: 10.3201/eid1104.041126
Hypša, V., and Aksoy, S. (1997). Phylogenetic characterization of two transovarially transmitted endosymbionts of the bedbug Cimex lectularius (Heteroptera: Cimicidae). Insect Mol. Biol. 6, 301–304. doi: 10.1046/j.1365-2583.1997.00178.x
Janson, E. M., Stireman, J. O., Singer, M. S., and Abbot, P. (2008). Phytophagous insect-microbe mutualisms and adaptive evolutionary diversification. Evolution 62, 997–1012. doi: 10.1111/j.1558-5646.2008.00348.x
Johnson, C. G. (1941). The ecology of the bed-bug, cimex lectularius L., in Britain: report on research, 1935–1940. J. Hyg. 41, 345–461. doi: 10.1017/S0022172400012560
Koch, A. (1959). Intracellular symbiosis in insects. Ann. Rev. Microbiol. 14, 121–140. doi: 10.1146/annurev.mi.14.100160.001005
Kuznetsova, A., Brockhoff, P. B., and Christensen, R. H. B. (2017). lmerTest package: tests in linear mixed effects models. J. Stat. Softw. 82, 1–26. doi: 10.18637/JSS.V082.I13
Lange, A. B., Leyria, J., and Orchard, I. (2022). The hormonal and neural control of egg production in the historically important model insect, Rhodnius prolixus: a review, with new insights in this post-genomic era. Gen. Comp. Endocrinol. 321-322:114030. doi: 10.1016/j.ygcen.2022.114030
Langer, L., Froschauer, C., and Reinhardt, K. (2020). Sex differences in bedbug nymphs, Cimex lectularius. J. Appl. Entomol. 144, 838–843. doi: 10.1111/jen.12823
Login, F. H., Balmand, S., Vallier, A., Vincent-Monégat, C., Vigneron, A., Weiss-Gayet, M., et al. (2011). Antimicrobial peptides keep insect endosymbionts under control (method). Science 334, 362–365. doi: 10.1126/science.1209728
Mellanby, K. (1939). Fertilization and egg production in the bed-bug, Cimex lectularius L. Parasitology 31, 193–199. doi: 10.1017/S0031182000012750
Moran, N. A., Telang, A., and Smith, M. (1998). Symbionts of insects, a variety of insect groups harbor ancient prokaryotic endosymbionts. Bioscience 48, 295–304. doi: 10.1007/0-387-30741-9_16
Nadal-Jimenez, P., Siozios, S., Halliday, N., Cámara, M., and Hurst, G. D. D. (2022). Symbiopectobacterium purcellii, gen. Nov., sp. nov., isolated from the leafhopper Empoasca decipiens. Int. J. Syst. Evol. Microbiol. 72, 1–13. doi: 10.1099/ijsem.0.005440
Nikoh, N., Hosokawa, T., Moriyama, M., Oshima, K., Hattori, M., and Fukatsu, T. (2014). Evolutionary origin of insect-Wolbachia nutritional mutualism. Proc. Natl. Acad. Sci. 111, 10257–10262. doi: 10.1073/pnas.1409284111
Nozaki, T., and Shigenobu, S. (2022). Ploidy dynamics in aphid host cells harboring bacterial symbionts. Sci. Rep. 12, 9111–9112. doi: 10.1038/s41598-022-12836-8
Orr-Weaver, T. L. (2015). When bigger is better: the role of polyploidy in organogenesis. Trends Genet. 31, 307–315. doi: 10.1016/j.tig.2015.03.011
Pan, X., Zhou, G., Wu, J., Bian, G., Lu, P., Raikhel, A. S., et al. (2012). Wolbachia induces reactive oxygen species (ROS)-dependent activation of the toll pathway to control dengue virus in the mosquito Aedes aegypti. Proc. Natl. Acad. Sci. USA 109, E23–E31. doi: 10.1073/pnas.1116932108
Parola, P., and Izri, A. (2020). Clinical Practice: Clinical Practice. N. Engl. J. Med. 382, 2230–2237. doi: 10.1056/NEJMcp1905840
Pfaffl, M. W. (2001). A new mathematical model for relative quantification in real-time RT–PCR. Nucleic Acids Res. 29, 45e–445e. doi: 10.1093/nar/29.9.e45
Pilgrim, J., Ander, M., Garros, C., Baylis, M., Hurst, G. D. D., and Siozios, S. (2017). Torix group Rickettsia are widespread in Culicoides biting midges (Diptera: Ceratopogonidae), reach high frequency and carry unique genomic features. Environ. Microbiol. 19, 4238–4255. doi: 10.1111/1462-2920.13887
Pilgrim, J., Thongprem, P., Davison, H. R., Siozios, S., Baylis, M., Zakharov, E. V., et al. (2021). Torix Rickettsia are widespread in arthropods and reflect a neglected symbiosis. GigaScience 10, 1–19. doi: 10.1093/gigascience/giab021
Potts, R., Molina, I., Sheele, J. M., and Pietri, J. E. (2020). Molecular detection of Rickettsia infection in field-collected bed bugs. New Microbes New Infect. 34:100646. doi: 10.1016/j.nmni.2019.100646
R Core Team (2024). R: a language and environment for statistical computing. Available at: (https://www.R-project.org/).
Reinhardt, K., Naylor, R., and Siva-Jothy, M. T. (2003). Reducing a cost of traumatic insemination: female bedbugs evolve a unique organ. Proc. R. Soc. B Biol. Sci. 270, 2371–2375. doi: 10.1098/rspb.2003.2515
Reinhardt, K., and Siva-Jothy, M. T. (2007). Biology of the bed bugs (Cimicidae). Annu. Rev. Entomol. 52, 351–374. doi: 10.1146/annurev.ento.52.040306.133913
Rio, R. V. M., Attardo, G. M., and Weiss, B. L. (2017). Grandeur alliances: symbiont metabolic integration and obligate arthropod hematophagy. Physiol. Behav. 176, 139–148. doi: 10.1016/j.pt.2016.05.002.Grandeur
Rio, R. V. M., Wu, Y. N., Filardo, G., and Aksoy, S. (2006). Dynamics of multiple symbiont density regulation during host development: tsetse fly and its microbial flora. Proc. R. Soc. B Biol. Sci. 273, 805–814. doi: 10.1098/rspb.2005.3399
Sakamoto, J. M., and Rasgon, J. L. (2006). Geographic distribution of Wolbachia infections in Cimex lectularius (Heteroptera: Cimicidae). J. Med. Entomol. 43, 696–700. doi: 10.1603/0022-2585(2006)43[696:GDOWII]2.0.CO;2
Saveer, A. M., DeVries, Z., Santangelo, R. G., and Schal, C. (2021). Mating and starvation modulate feeding and host-seeking responses in female bed bugs, Cimex lectularius. Sci. Rep. 11:1915. doi: 10.1038/s41598-021-81271-y
Schneider, C. A., Rasband, W. S., and Eliceiri, K. W. (2012). NIH image to ImageJ: 25 years of image analysis. Nat. Methods 9, 671–675. doi: 10.1038/nmeth.2089
Simonet, P., Duport, G., Gaget, K., Weiss-Gayet, M., Colella, S., Febvay, G., et al. (2016). Direct flow cytometry measurements reveal a fine-tuning of symbiotic cell dynamics according to the host developmental needs in aphid symbiosis. Sci. Rep. 6:19967. doi: 10.1038/srep19967
Simonet, P., Gaget, K., Balmand, S., Ribeiro Lopes, M., Parisot, N., Buhler, K., et al. (2018). Bacteriocyte cell death in the pea aphid / Buchnera symbiotic system. PNAS 115, E1819–E1828. doi: 10.1073/pnas.1720237115
Siva-jothy, M. T., Zhong, W., Naylor, R., Heaton, L., Hentley, W., and Harney, E. (2019). Female bed bugs (Cimex lectularius L) anticipate the immunological consequences of traumatic insemination via feeding cues. PNAS 116, 14682–14687. doi: 10.1073/pnas.1904539116
Štefka, J., Votýpka, J., Lukeš, J., and Balvín, O. (2022). Cimex lectularius and Cimex hemipterus (bed bugs). Trends Parasitol. 38, 919–920. doi: 10.1016/j.pt.2022.04.006
Stutt, A. D., and Siva-Jothy, M. T. (2001). Traumatic insemination and sexual conflict in the bed bug Cimex lectularius. Proc. Natl. Acad. Sci. USA 98, 5683–5687. doi: 10.1073/pnas.101440698
Thongprem, P., Evison, S. E. F., Hurst, G. D. D., and Otti, O. (2020). Transmission, tropism, and biological impacts of Torix Rickettsia in the common bed bug Cimex lectularius (Hemiptera: Cimicidae). Front. Microbiol. 11:608763. doi: 10.3389/fmicb.2020.608763
Vigneron, A., Masson, F., Vallier, A., Balmand, S., Rey, M., Vincent-Monégat, C., et al. (2014). Insects recycle endosymbionts when the benefit is over. Curr. Biol. 24, 2267–2273. doi: 10.1016/j.cub.2014.07.065
Wang, Y., Li, C., Yan, J.-Y., Wang, T.-Y., Yao, Y.-L., Ren, F.-R., et al. (2022). Autophagy regulates white fl y-symbiont metabolic interactions. Appl. Environ. Microbiol. 88, 1–19. doi: 10.1128/AEM.02089-21
Wasserstein, R. L., and Lazar, N. A. (2016). The ASA statement on p-values: context, process, and purpose. Am. Stat. 70, 129–133. doi: 10.1080/00031305.2016.1154108
Wasserstein, R. L., Schirm, A. L., and Lazar, N. A. (2019). Moving to a world beyond “p < 0.05”. Am. Stat. 73, 1–19. doi: 10.1080/00031305.2019.1583913
Wickham, H. (2016). ggplot2 elegant graphics for data analysis. Available at: http://www.springer.com/series/6991
Keywords: Cimex lectularius, Wolbachia, nutritional symbiosis, host-symbiont interaction, bacterial growth dynamics, development, bacteriome
Citation: Poulain M, Rosinski E, Henri H, Balmand S, Delignette-Muller M-L, Heddi A, Lasseur R, Vavre F, Zaidman-Rémy A and Kremer N (2024) Development, feeding, and sex shape the relative quantity of the nutritional obligatory symbiont Wolbachia in bed bugs. Front. Microbiol. 15:1386458. doi: 10.3389/fmicb.2024.1386458
Edited by:
Yuval Gottlieb, Hebrew University of Jerusalem, IsraelReviewed by:
Jeremy Foster, New England Biolabs, United StatesHabib Ali, Khwaja Fareed University of Engineering and Information Technology (KFUEIT), Pakistan
Xiao-Li Bing, Nanjing Agricultural University, China
Copyright © 2024 Poulain, Rosinski, Henri, Balmand, Delignette-Muller, Heddi, Lasseur, Vavre, Zaidman-Rémy and Kremer. This is an open-access article distributed under the terms of the Creative Commons Attribution License (CC BY). The use, distribution or reproduction in other forums is permitted, provided the original author(s) and the copyright owner(s) are credited and that the original publication in this journal is cited, in accordance with accepted academic practice. No use, distribution or reproduction is permitted which does not comply with these terms.
*Correspondence: Marius Poulain, bWFyaXVzLnBvdWxhaW5Ab3V0bG9vay5mcg==; Anna Zaidman-Rémy, YW5uYS56YWlkbWFuQGluc2EtbHlvbi5mcg==; Natacha Kremer, bmF0YWNoYS5rcmVtZXJAY25ycy5mcg==