- 1Department of Obstetrics & Gynecology, Hunan Provincial Maternal and Child Health Care Hospital, Changsha, Hunan, China
- 2National Health Commission Key Laboratory for Birth Defect Research and Prevention, Hunan Provincial Maternal and Child Health Care Hospital, Changsha, Hunan, China
- 3Key Lab of Molecular Virology and Immunology, Shanghai Institute of Immunity and Infection, Chinese Academy of Sciences, Shanghai, China
- 4Key Laboratory of Multi-Cell Systems, Shanghai Institute of Biochemistry and Cell Biology, Center for Excellence in Molecular Cell Science, Chinese Academy of Sciences, Shanghai, China
- 5Department of Obstetrics and Gynecology, 3rd Xiangya Hospital, Central South University, Changsha, Hunan, China
Chlamydia trachomatis is responsible for infections in various mucosal tissues, including the eyes, urogenital, respiratory, and gastrointestinal tracts. Chronic infections can result in severe consequences such as blindness, ectopic pregnancy, and infertility. The underlying mechanisms leading to these diseases involve sustained inflammatory responses, yet thorough comprehension of the underlying mechanisms remains elusive. Chlamydial biologists employ in multiple methods, integrating biochemistry, cell biology, and genetic tools to identify bacterial factors crucial for host cell interactions. While numerous animal models exist to study chlamydial pathogenesis and assess vaccine efficacy, selecting appropriate models for biologically and clinically relevant insights remains a challenge. Genital infection models in animals have been pivotal in unraveling host-microbe dynamics, identifying potential chlamydial virulence factors influencing genital pathogenicity. However, the transferability of this knowledge to human pathogenic mechanisms remains uncertain. Many putative virulence factors lack assessment in optimal animal tissue microenvironments, despite the diverse chlamydial infection models available. Given the propensity of genital Chlamydia to spread to the gastrointestinal tract, investigations into the pathogenicity and immunological impact of gut Chlamydia become imperative. Notably, the gut emerges as a promising site for both chlamydial infection vaccination and pathogenesis. This review elucidates the pathogenesis of Chlamydia infections and delineates unique features of prevalent animal model systems. The primary focus of this review is to consolidate and summarize current animal models utilized in Chlamydia researches, presenting findings, discussions on their contributions, and suggesting potential directions for further studies.
1 Introduction
Chlamydia, predominantly represented by Chlamydia pneumoniae (C.p) and Chlamydia trachomatis (C.t), constitutes a significant portion of human infections. The escalating impact of C.t-mediated diseases emphasizes the urgency for innovative interventions alongside existing public health measures (de la Maza et al., 2017; Gottlieb and Johnston, 2017).
The successful transformation of C.t using recombinant plasmids from its own endogenous plasmid has accelerated the study of plasmid-encoded factors crucial for chlamydial pathogenicity (Ding et al., 2013; Gong et al., 2013; Song et al., 2013). The development of genetic tools has expanded exploration into chromosome-encoded factors, resulting in numerous genetically defined mutants (Brothwell et al., 2018; Putman et al., 2019). The ongoing optimization of these genetic tools calls for a thoughtful discussion on selecting the most suitable models for evaluating these mutants. Despite the availability of various animal models to study chlamydial pathogenic mechanisms and assess vaccine efficacy, refining, and choosing the most appropriate models for obtaining biologically and clinically relevant information remains a challenge.
Our aim is to clarify the general pathogenic characteristics of chlamydial infections and navigate the intricacies of selecting appropriate model systems, recognizing their inherent limitations, to extract biologically and clinically relevant insights. Lastly, we will elaborate on the newly proposed hypothetical model for Chlamydia genital-gut interaction to offer a more comprehensive understanding of chlamydial pathogenesis and insights for future research.
We believe in the importance of selecting robust models with appropriate infection routes, coupled with well-matched mutants. This strategic approach enhances our ability to identify tissue-dependent C.t virulence determinants, unravel mechanisms driving site-specific immunity and pathology, and pinpoint site-specific factors that may either contribute to or impede local immunity.
2 Genital animal models of Chlamydia infection
2.1 Non-human primate trachoma and NHP genital tract models
After Tang successfully isolated C.t organisms from human ocular tissues (Tang et al., 1957), attempts were made to infect monkey eyes with human isolates to meet Koch's postulates criteria for trachoma causation (Wang, 1999). Various NHP species exhibited induced ocular inflammatory pathologies similar to those in humans, confirming causation and establishing a valuable NHP ocular model for studying trachoma pathogenesis and vaccine evaluation.
A whole C.t organism-based vaccine induced protective immunity against conjunctivitis in monkeys, but immunity waned within a year. Notably, C.t serovar A, capable of inducing robust inflammatory pathology in monkeys, failed when deficient in the chlamydial plasmid (Kari et al., 2011). The plasmid's role in human ocular infection and pathogenesis needs further investigation, but its correlation with high antibody titers to plasmid-encoded Pgp3 suggests potential contributions to chlamydial pathogenicity in human ocular tissue (Winstanley et al., 2017; Wiegand et al., 2018).
The primate trachoma model revealed persistent live organism shedding from serovar A-infected monkeys' ocular tissue for up to 7 weeks, with inflammatory pathologies lasting up to 15 weeks (Kari et al., 2011). In contrast, plasmid-free serovar A-infected monkeys experienced brief shedding, clearing infection within 3 weeks without significant pathology. This underscores the plasmid's necessity for C.t colonization and pathogenicity in ocular tissue, consistent with its prevalence in human C.t isolates. Moreover, plasmid-free serovar A-inoculated monkeys were protected against both infection and pathology induced by wild-type serovar A, demonstrating the feasibility of developing a live attenuated C.t vaccine (de la Maza et al., 2017; Zhu et al., 2018). Interestingly, ocular protective immunity in macaques was found to be CD8+ T cell-dependent, though its role against C.t infections at other sites remains undetermined (Olivares-Zavaleta et al., 2014).
Over the years, various NHP species, including pigtail and rhesus macaques, have been employed to model C.t genital tract infections (Bell et al., 2011). The genital tracts of female pigtail macaques share many similarities with those of women, including the length of the menstrual cycle, reproductive tract anatomy, cervical tissue cellular structure, and vaginal microflora. In a recent study of C.t pathogenicity in pigtail macaques, six macaques received a cervical inoculation of C.t and were observed and sampled at weekly intervals (Patton et al., 2018). These macaques developed mild to moderate infection and disease. Remarkably, parallel animals inoculated with the same strain of C.t but deficient in the plasmid developed similar infection and disease endpoints (Patton et al., 2018), and these results were reproducible in rhesus macaques (Qu et al., 2015). Evidently, the plasmid is not essential for C.t infection in primate genital tracts, contrasting with its requirement in the ocular C.t challenge model. A baboon cervical infection model exists but may face limitations due to cost and availability issues (Bell et al., 2011).
2.2 Mouse model of C.t genital infection
Despite C.t not naturally infecting rodents, mice serve as essential models for exploring chlamydial pathogenic mechanisms (Zhong, 2018) and evaluating vaccine candidates (de la Maza et al., 2017). The efficiency of creating mutations in C.t serovar L2 has led to extensive assessments in mice, including L2 mutants; other C.t organisms, such as serovar D with or without genetic mutations, have also been studied in the murine model (Sturdevant et al., 2010).
However, C.t encounters challenges overcoming murine innate immunity, resulting in its swift elimination from the mouse genital tract (Sturdevant and Caldwell, 2014). In contrast, intravaginal inoculation with Chlamydia muridarum (C.m) effectively establishes productive infection and induces upper genital tract pathology, resembling observations in C.t-infected women during laparoscopy (Sun et al., 2015). This robust model, along with genetic manipulation of C.m, has significantly advanced our understanding of chlamydial pathogenesis.
Following Tang's isolation of C.t from human ocular tissues (Tang et al., 1957), mice immunized with killed C.t prevented toxicity from intravenous injection of live C.t, aiding in classifying 'trachoma virus strains' and studying IFNγ's role in chlamydial infection. However, this model lacked relevance to C.t pathogenicity in humans.
To gain more pertinent information on C.t pathogenicity, intravaginal inoculation into mice was deemed necessary. Unfortunately, C.t fails to induce lasting pathology in the mouse upper genital tract, despite lower genital tract infections (Sturdevant et al., 2010; Eko et al., 2014). This is partly due to C.t's inability to overcome innate immunity in the mouse genital tract. Female C3H/HeJ mice, with a natural toll-like receptor 4 gene mutation, show increased susceptibility to C.t in the genital tract (Sturdevant et al., 2010). Improving the mouse intravaginal infection model by genetically increasing susceptibility to C.t colonization is desired.
The intrabursal model, requiring survival surgery and overcoming physiological barriers, contrasts with the preferred transcervical inoculation with C.t due to its less invasiveness. Transcervical inoculation with an inactivated C.t vaccine strain showed protection against live C.t challenge infection (Stary et al., 2015). Additionally, transcervical inoculation with live C.t serovar D induced tubal infertility in C57 wild-type and HLA-DR4 transgenic mice, serving as a potentially useful model for studying C.t pathogenesis and evaluating human-relevant chlamydial vaccines (Pal et al., 2018).
Following the successful transformation of the C.t LGV L2 strain, a series of L2 mutants deficient in plasmid genes were created and assessed in mice (Ramsey et al., 2014). Pgp3-deficient L2 showed attenuation in infecting the mouse lower genital tracts and inducing inflammatory pathology. An L2 strain deficient in expressing the chlamydial protease-like activity factor (CPAF) was isolated from L2 mutagenesis libraries. CPAF-deficient L2 revealed the critical role of CPAF in chlamydial survival in mice. However, the L2 genital infection model has limitations for modeling C.t infection in humans, as both the infection and pathology were transient even with wild-type L2.
2.3 Mouse model of genital C.m infection
The mouse cervicovaginal infection model with C.m has been crucial for unraveling chlamydial pathogenic mechanisms and host immune responses (Cheng et al., 2008). Utilizing C.m, chosen for its genomic similarity to C.t, has mirrored upper genital tract pathology observed in women infected with C.t. Evaluation of mouse genital tracts revealed gross pathologies, such as hydrosalpinx (Sun et al., 2015), aligning with medically relevant endpoints seen in C.t-infected women (Budrys et al., 2012).
Upon intravaginal inoculation, C.m ascends the mouse genital tract, triggering acute inflammation manifesting as pyosalpinx detectable macroscopically between 2 and 3 weeks. Some pyosalpinxes transform into hydrosalpinx by the 4th week, potentially leading to long-lasting infertility (Zhang et al., 2014). Long-lasting hydrosalpinx in the C.m mouse model mirrors fibrotic pathologies seen in women with tubal factor infertility (Budrys et al., 2012). The chlamydial plasmid, particularly Pgp3, plays a pivotal role in inducing hydrosalpinx. Deficiency in Pgp3 mirrors plasmid-deficiency effects, emphasizing its major contribution to the overall pathogenic process (Zhong, 2018). And Chromosomal proteins TC0237/TC0668 also contribute to hydrosalpinx induction in the mouse genital tract (Chen et al., 2015; Conrad et al., 2016).
With these models, various mechanisms have been identified that influence chlamydial induction of upper genital tract pathology (Murthy et al., 2011; Frazer et al., 2013). Despite these advancements, our understanding is still incomplete, particularly regarding the mechanisms sustaining the persistence of long-lasting pathogenic tubal fibrosis after Chlamydia clearance from the oviduct infection site in animal genital models.
3 Gastrointestinal Chlamydia infection model
While Chlamydia is primarily recognized as a genital tract pathogen, its regular detection in the human gastrointestinal (GI) tracts (Peters et al., 2014; Craig et al., 2015) introduces a layer of complexity. This finding aligns with observations of C.t infecting human enteroendocrine cells (Dlugosz et al., 2014). Remarkably, individuals engaging in oral/anal sex or those abstaining from such behaviors both show C.t presence in their rectal swabs (Peters et al., 2014), suggesting potential routes of C.t transmission from genital to GI tracts beyond sexual behavior.
The mechanism by which C.t enters the GI tract, whether through sexual behaviors or alternative pathways, raises a critical question: can GI tract C.t influence C.t pathogenicity in the genital tract (Bavoil et al., 2017). This inquiry becomes essential due to the frequent detection of C.t in the human GI tract. Addressing this question in human subjects would necessitate extensive clinical investigations, potentially involving therapeutic interventions. As an alternative approach, evaluating the impact of GI tract Chlamydia on genital tract pathogenicity can be explored using the murine model of CM induction of hydrosalpinx (Tian et al., 2023).
The successful transformation of C.m by has provided a valuable tool for real-time monitoring of live chlamydial infection in mice (Campbell et al., 2014). This tool has revealed the swift ascent of vaginal C.m to the oviduct, its subsequent spread to the GI tract, and its ability to establish long-lasting colonization within the GI tract (Zhang et al., 2015). In the context of mice, chlamydial long-lasting colonization in the gut denotes the enduring presence and survival of Chlamydia within the GI tract over an extended period. While a clear definition in human hosts is currently lacking, the persistence of Chlamydia in the large intestine of mice can extend for hundreds of days (>50 weeks), significantly surpassing the duration observed in the genital tract. Chlamydial colonization in the GI tract depends on its overcoming mucosal barriers, utilizing chromosomal genes to evade IFNγ from innate lymphoid cells (Zhong, 2021). Remarkably, the spread from the genital tract to the GI tract appears independent of the oral/anorectal route, as evidenced by experiments with mice wearing restraining Elizabethan collars and housed in net-bottom cages to prevent coprophagy. Instead, this spreading seems to be dependent on multiple pathways (Zhang et al., 2015). The research indicates potential pathways for hematogenous Chlamydia to reach the large intestine lumen after genital infection dissemination, involving both spleen-to-stomach and liver-to-intestine routes (Zhou et al., 2021). Additionally, other studies suggest that genital Chlamydia can also be transported to the gut by host cells (Howe et al., 2019). Upon arrival in the GI tract, C.m does not autoinoculate extra-GI tissues (Wang et al., 2016), challenging the hypothesis that chlamydial organisms in the GI tract could act as a reservoir for auto-inoculating the genital tract (Yeruva et al., 2013). Despite the absence of autoinoculation, the correlation between chlamydial spreading from the genital to GI tracts and pathogenicity in the upper genital tract has led to the proposition of a Two-Hit model as a chlamydial pathogenic mechanism (Tian et al., 2020).
In this Two-Hit model (Figure 1), the first hit is attributed to genital C.m ascending infection, where C.m invades oviduct epithelial cells and induces tubal inflammation. This initial hit may cause epithelial damage, triggering tissue repair responses, including fibrosis. While the fibrotic response is typically transient and halts upon the restoration of oviduct function, the second hit comes into play when genital C.m spreads to the GI tract. Here, C.m organisms residing in the GI tract for extended periods induce pro-fibrotic lymphocytes, which, when recruited into the oviduct previously affected by C.m ascending infection, act as the second hit. And the nature of the second hit might be GI related lymphocytes, such as CD8+T cells (Tian et al., 2021). These GI tract-derived C.m-specific profibrotic lymphocytes may convert the initially transient tubal repairing fibrotic response into a long-lasting tubal fibrotic blockade (Tian et al., 2020). The 2-hit model aligns with the concept that responses induced by gut bacteria can impact tissues beyond the GI tract. Conversely, if a naive mouse is exposed to C.m in the GI tract first, it essentially becomes immune to subsequent C.m exposure in extra-GI tissues (Wang et al., 2018; Zhu et al., 2018). The site of the first exposure to C.m may therefore determine the consequential outcome. It is essential to note that the contribution of gut Chlamydia to upper genital pathology has only been tested in mice under specific experimental conditions. Thus, the Two-Hit Model remains a hypothesis requiring further exploration in human studies.
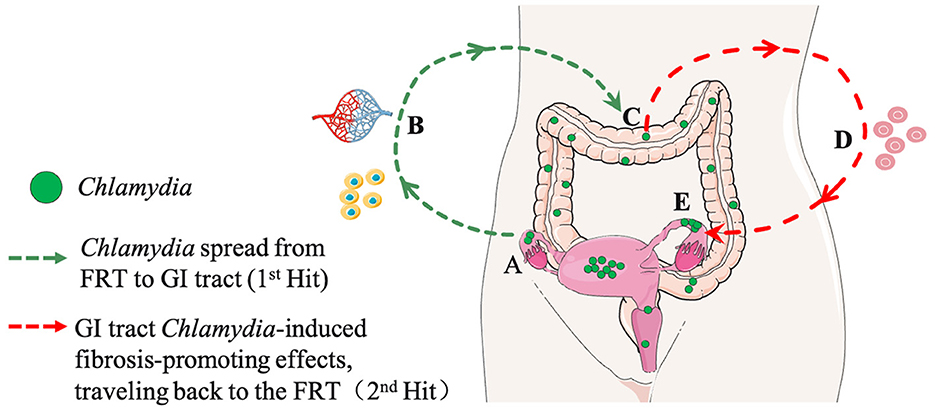
Figure 1. A hypothetical model for Chlamydia pathogenesis: interactions between the genital and gastrointestinal (GI) tracts (A Two-Hit Model). (A) First Hit: Chlamydia ascends the female reproductive tract (FRT), invading oviduct epithelial cells, inducing acute inflammation, and resulting in the clearance of tubal infection, epithelial damage, and transient fibrotic responses. (B) Spreading Routes: Chlamydia spreads from the FRT to the GI tract through multiple pathways, including blood circulation and host cells. (C) GI Tract Colonization: Chlamydia further spreads and colonizes the large intestine. (D) Second Hit: The colonized gut act as a reservoir for continuous exposure, perpetuating its impact on FRT pathogenicity. This contributes to sustained inflammation, pro-fibrotic responses, and effects on the FRT. (E) Long-Term Consequences: The enduring impact from GI tract on tubal fibrosis in the FRT may contribute to infertility and other reproductive complications. Portions of the figure utilized images from Servier Medical Art, licensed under Creative Commons Attribution 3.0 Unported License.
While C.t is frequently detected in the human GI tracts, the impact of human GI tract C.t on C.t pathogenicity in the genital tract remains unclear. Exploring the current studies in human contexts could provide valuable insights into this aspect.
4 Discussion
The review emphasizes challenges in modeling chlamydial infections and the limitations of current animal models (Table 1). Host-specific adaptation poses a critical challenge, hindering lasting fibrosis induction in murine genital tracts by genitourinary C.t serovars. While IFNγ-mediated immunity is crucial for both human C.t and murine C.m infections, distinct mechanisms highlight complex host-pathogen interactions.
Tissue tropisms of C.t serovars in humans raise questions about factors influencing tissue-specific evolution. Chlamydial spreading patterns within and between hosts, coupled with infection dose and frequency considerations, add complexity to modeling disease outcomes. NHP and rodent models, valuable in certain contexts, cannot precisely replicate human disease, emphasizing the need for further exploration.
The discussion introduces the GI tract perspective, emphasizing Chlamydia's interaction with different anatomical sites. C.m spread from genital to GI tract prompts consideration of GI Chlamydia's impact on genital tract pathogenicity. The Two-Hit model in murine studies provides a framework, but exploring diverse animal models beyond mice is imperative. Examining different species can offer insights into varied responses and consequences, enhancing our understanding of Chlamydia pathogenesis.
In conclusion, complexities in modeling Chlamydia infections underscore the need for diverse animal models and continued exploration, especially in non-murine species, to capture nuanced host-pathogen interactions in different anatomical sites and broaden our understanding of GI tract Chlamydia.
Author contributions
QT: Funding acquisition, Writing – original draft, Writing – review & editing. TZ: Funding acquisition, Writing – original draft, Writing – review & editing. CS: Writing – review & editing. ZH: Writing – review & editing. YH: Writing – review & editing. JW: Writing – review & editing. LW: Writing – review & editing. XS: Writing – review & editing.
Funding
The author(s) declare that financial support was received for the research, authorship, and/or publication of this article. This work was supported by the National Natural Science Foundation of China for the Youth (32100162) to QT and (32000138) to TZ, the Natural Science Foundation of Hunan Province, China (2022JJ70092 and 2023JJ40355), the Innovation Platform and Talent Program of Hunan Province, China (2021SK4021), and the Rui Xin project of Hunan Provincial Maternal and Child Health Care Hospital (2021RX08, 2021RX10, and 2023RX03).
Conflict of interest
The authors declare that the research was conducted in the absence of any commercial or financial relationships that could be construed as a potential conflict of interest.
Publisher's note
All claims expressed in this article are solely those of the authors and do not necessarily represent those of their affiliated organizations, or those of the publisher, the editors and the reviewers. Any product that may be evaluated in this article, or claim that may be made by its manufacturer, is not guaranteed or endorsed by the publisher.
References
Bavoil, P. M., Marques, P. X., Brotman, R., and Ravel, J. (2017). Does active oral sex contribute to female infertility? J. Infect. Dis. 216, 932–935. doi: 10.1093/infdis/jix419
Bell, J. D., Bergin, I. L., Harris, L. H., Chai, D., Mullei, I., Mwenda, J., et al. (2011). The effects of a single cervical inoculation of Chlamydia trachomatis on the female reproductive tract of the baboon (Papio anubis). J. Infect. Dis. 204, 1305–1312. doi: 10.1093/infdis/jir541
Brothwell, J. A., Muramatsu, M. K., Zhong, G., and Nelson, D. E. (2018). Advances and obstacles in the genetic dissection of chlamydial virulence. Curr. Top. Microbiol. Immunol. 412, 133–158. doi: 10.1007/82_2017_76
Budrys, N. M., Gong, S., Rodgers, A. K., Wang, J., Louden, C., Shain, R., et al. (2012). Chlamydia trachomatis antigens recognized in women with tubal factor infertility, normal fertility, and acute infection. Obstet. Gynecol. 119, 1009–1016. doi: 10.1097/AOG.0b013e3182519326
Campbell, J., Huang, Y., Liu, Y., Schenken, R., Arulanandam, B., and Zhong, G. (2014). Bioluminescence imaging of Chlamydia muridarum ascending infection in mice. PLoS ONE 9:e101634. doi: 10.1371/journal.pone.0101634
Chen, C., Zhou, Z., Conrad, T., Yang, Z., Dai, J., Li, Z., et al. (2015). In vitro passage selects for Chlamydia muridarum with enhanced infectivity in cultured cells but attenuated pathogenicity in mouse upper genital tract. Infect. Immun. 83, 1881–1892. doi: 10.1128/IAI.03158-14
Cheng, W., Shivshankar, P., Li, Z., Chen, L., Yeh, I. T., and Zhong, G. (2008). Caspase-1 contributes to Chlamydia trachomatis-induced upper urogenital tract inflammatory pathologies without affecting the course of infection. Infect. Immun. 76, 515–522. doi: 10.1128/IAI.01064-07
Conrad, T. A., Gong, S., Yang, Z., Matulich, P., Keck, J., Beltrami, N., et al. (2016). The chromosome-encoded hypothetical protein TC0668 Is an upper genital tract pathogenicity factor of Chlamydia muridarum. Infect. Immun. 84, 467–479. doi: 10.1128/IAI.01171-15
Craig, A. P., Kong, F. Y., Yeruva, L., Hocking, J. S., Rank, R. G., Wilson, D. P., et al. (2015). Is it time to switch to doxycycline from azithromycin for treating genital chlamydial infections in women? Modelling the impact of autoinoculation from the gastrointestinal tract to the genital tract. BMC Infect. Dis. 15:200. doi: 10.1186/s12879-015-0939-3
de la Maza, L. M., Zhong, G., and Brunham, R. C. (2017). Update on Chlamydia trachomatis vaccinology. Clin. Vacc. Immunol. 24, e00543-16. doi: 10.1128/CVI.00543-16
Ding, H., Gong, S., Tian, Y., Yang, Z., Brunham, R., and Zhong, G. (2013). Transformation of sexually transmitted infection-causing serovars of chlamydia trachomatis using Blasticidin for selection. PLoS ONE 8:e80534. doi: 10.1371/journal.pone.0080534
Dlugosz, A., Muschiol, S., Zakikhany, K., Assadi, G., D'amato, M., and Lindberg, G. (2014). Human enteroendocrine cell responses to infection with Chlamydia trachomatis: a microarray study. Gut Pathog. 6:24. doi: 10.1186/1757-4749-6-24
Eko, F. O., Mania-Pramanik, J., Pais, R., Pan, Q., Okenu, D. M., Johnson, A., et al. (2014). Vibrio cholerae ghosts (VCG) exert immunomodulatory effect on dendritic cells for enhanced antigen presentation and induction of protective immunity. BMC Immunol. 15:584. doi: 10.1186/s12865-014-0056-x
Frazer, L. C., Sullivan, J. E., Zurenski, M. A., Mintus, M., Tomasak, T. E., Prantner, D., et al. (2013). CD4+ T cell expression of MyD88 is essential for normal resolution of Chlamydia muridarum genital tract infection. J. Immunol. 191, 4269–4279. doi: 10.4049/jimmunol.1301547
Gong, S., Yang, Z., Lei, L., Shen, L., and Zhong, G. (2013). Characterization of Chlamydia trachomatis plasmid-encoded open reading frames. J. Bacteriol. 195, 3819–3826. doi: 10.1128/JB.00511-13
Gottlieb, S. L., and Johnston, C. (2017). Future prospects for new vaccines against sexually transmitted infections. Curr. Opin. Infect. Dis. 30, 77–86. doi: 10.1097/QCO.0000000000000343
Howe, S. E., Shillova, N., and Konjufca, V. (2019). Dissemination of Chlamydia from the reproductive tract to the gastro-intestinal tract occurs in stages and relies on Chlamydia transport by host cells. PLoS Pathog. 15:e1008207. doi: 10.1371/journal.ppat.1008207
Kari, L., Whitmire, W. M., Olivares-Zavaleta, N., Goheen, M. M., Taylor, L. D., Carlson, J. H., et al. (2011). A live-attenuated chlamydial vaccine protects against trachoma in nonhuman primates. J. Exp. Med. 208, 2217–2223. doi: 10.1084/jem.20111266
Murthy, A. K., Li, W., Chaganty, B. K., Kamalakaran, S., Guentzel, M. N., Seshu, J., et al. (2011). Tumor necrosis factor alpha production from CD8+ T cells mediates oviduct pathological sequelae following primary genital Chlamydia muridarum infection. Infect. Immun. 79, 2928–2935. doi: 10.1128/IAI.05022-11
Olivares-Zavaleta, N., Whitmire, W. M., Kari, L., Sturdevant, G. L., and Caldwell, H. D. (2014). CD8+ T cells define an unexpected role in live-attenuated vaccine protective immunity against Chlamydia trachomatis infection in macaques. J. Immunol. 192, 4648–4654. doi: 10.4049/jimmunol.1400120
Pal, S., Tifrea, D. F., Zhong, G., and De La Maza, L. M. (2018). Transcervical inoculation with chlamydia trachomatis induces infertility in HLA-DR4 transgenic and wild-type mice. Infect. Immun. 86, 10–1128. doi: 10.1128/IAI.00722-17
Patton, D. L., Sweeney, Y. C., Baldessari, A. E., Cles, L., Kari, L., Sturdevant, G. L., et al. (2018). The Chlamydia trachomatis plasmid and CT135 virulence factors are not essential for genital tract infection or pathology in female pig-tailed macaques. Infect. Immun. 86, 10–1128. doi: 10.1128/IAI.00121-18
Peters, R. P., Dubbink, J. H., Van Der Eem, L., Verweij, S. P., Bos, M. L., Ouburg, S., et al. (2014). Cross-sectional study of genital, rectal, and pharyngeal Chlamydia and gonorrhea in women in rural South Africa. Sex Transm. Dis. 41, 564–569. doi: 10.1097/OLQ.0000000000000175
Putman, T., Hybiske, K., Jow, D., Afrasiabi, C., Lelong, S., Cano, M. A., et al. (2019). ChlamBase: a curated model organism database for the Chlamydia research community. Database 2019:baz041. doi: 10.1093/database/baz091
Qu, Y., Frazer, L. C., O'connell, C. M., Tarantal, A. F., Andrews, C. W. Jr., O'connor, S. L., et al. (2015). Comparable genital tract infection, pathology, and immunity in rhesus macaques inoculated with wild-type or plasmid-deficient chlamydia trachomatis serovar D. Infect. Immun. 83, 4056–4067. doi: 10.1128/IAI.00841-15
Ramsey, K. H., Schripsema, J. H., Smith, B. J., Wang, Y., Jham, B. C., O'hagan, K. P., et al. (2014). Plasmid CDS5 influences infectivity and virulence in a mouse model of Chlamydia trachomatis urogenital infection. Infect. Immun. 82, 3341–3349. doi: 10.1128/IAI.01795-14
Song, L., Carlson, J. H., Whitmire, W. M., Kari, L., Virtaneva, K., Sturdevant, D. E., et al. (2013). Chlamydia trachomatis plasmid-encoded Pgp4 is a transcriptional regulator of virulence-associated genes. Infect. Immun. 81, 636–644. doi: 10.1128/IAI.01305-12
Stary, G., Olive, A., Radovic-Moreno, A. F., Gondek, D., Alvarez, D., Basto, P. A., et al. (2015). VACCINES. A mucosal vaccine against Chlamydia trachomatis generates two waves of protective memory T cells. Science 348:aaa8205. doi: 10.1126/science.aaa8205
Sturdevant, G. L., and Caldwell, H. D. (2014). Innate immunity is sufficient for the clearance of Chlamydia trachomatis from the female mouse genital tract. Pathog. Dis. 72, 70–73. doi: 10.1111/2049-632X.12164
Sturdevant, G. L., Kari, L., Gardner, D. J., Olivares-Zavaleta, N., Randall, L. B., Whitmire, W. M., et al. (2010). Frameshift mutations in a single novel virulence factor alter the in vivo pathogenicity of Chlamydia trachomatis for the female murine genital tract. Infect. Immun. 78, 3660–3668. doi: 10.1128/IAI.00386-10
Sun, X., Yang, Z., Zhang, H., Dai, J., Chen, J., Tang, L., et al. (2015). Chlamydia muridarum induction of glandular duct dilation in mice. Infect. Immun. 83, 2327–2337. doi: 10.1128/IAI.00154-15
Tang, F. F., Huang, Y. T., Chang, H. L., and Wong, K. C. (1957). Isolation of trachoma virus in chick embryo. J. Hyg. Epidemiol. Microbiol. Immunol. 1, 109–120.
Tian, Q., Zhang, T., Wang, L., Ma, J., and Sun, X. (2023). Gut dysbiosis contributes to chlamydial induction of hydrosalpinx in the upper genital tract. Front. Microbiol. 14:1142283. doi: 10.3389/fmicb.2023.1142283
Tian, Q., Zhou, Z., Wang, L., Abu-Khdeir, A. H., Huo, Z., Sun, X., et al. (2020). Gastrointestinal coinfection promotes chlamydial pathogenicity in the genital tract. Infect. Immun. 88, 10–1128. doi: 10.1128/IAI.00905-19
Tian, Q., Zhou, Z., Wang, L., Sun, X., Arulanandam, B., Xu, D., et al. (2021). Gastrointestinal chlamydia-induced CD8(+) T cells promote chlamydial pathogenicity in the female upper genital tract. Infect. Immun. 89:e0020521. doi: 10.1128/IAI.00205-21
Wang, L., Zhang, Q., Zhang, T., Zhang, Y., Zhu, C., Sun, X., et al. (2016). The Chlamydia muridarum organisms fail to auto-inoculate the mouse genital tract after colonization in the gastrointestinal tract for 70 days. PLoS ONE 11:e0155880. doi: 10.1371/journal.pone.0155880
Wang, L., Zhu, C., Zhang, T., Tian, Q., Zhang, N., Morrison, S., et al. (2018). Nonpathogenic colonization with chlamydia in the gastrointestinal tract as oral vaccination for inducing transmucosal protection. Infect. Immun. 86, 10-1128. doi: 10.1128/IAI.00630-17
Wang, Y. (1999). Etiology of trachoma: a great success in isolating and cultivating Chlamydia trachomatis. Chin. Med. J. 112, 938–941.
Wiegand, R. E., Cooley, G., Goodhew, B., Banniettis, N., Kohlhoff, S., Gwyn, S., et al. (2018). Latent class modeling to compare testing platforms for detection of antibodies against the Chlamydia trachomatis antigen Pgp3. Sci. Rep. 8:4232. doi: 10.1038/s41598-018-22708-9
Winstanley, C. E., Ramsey, K. H., Marsh, P., and Clarke, I. N. (2017). Development and evaluation of an enzyme-linked immunosorbent assay for the detection of antibodies to a common urogenital derivative of Chlamydia trachomatis plasmid-encoded PGP3. J. Immunol. Methods 445, 23–30. doi: 10.1016/j.jim.2017.03.002
Yeruva, L., Spencer, N., Bowlin, A. K., Wang, Y., and Rank, R. G. (2013). Chlamydial infection of the gastrointestinal tract: a reservoir for persistent infection. Pathog. Dis. 68, 88–95. doi: 10.1111/2049-632X.12052
Zhang, H., Zhou, Z., Chen, J., Wu, G., Yang, Z., Zhou, Z., et al. (2014). Lack of long-lasting hydrosalpinx in A/J mice correlates with rapid but transient chlamydial ascension and neutrophil recruitment in the oviduct following intravaginal inoculation with Chlamydia muridarum. Infect. Immun. 82, 2688–2696. doi: 10.1128/IAI.00055-14
Zhang, Q., Huang, Y., Gong, S., Yang, Z., Sun, X., Schenken, R., et al. (2015). In vivo and ex vivo imaging reveals a long-lasting chlamydial infection in the mouse gastrointestinal tract following genital tract inoculation. Infect. Immun. 83, 3568–3577. doi: 10.1128/IAI.00673-15
Zhong, G. (2018). Chlamydia spreading from the genital tract to the gastrointestinal tract - a two-hit hypothesis. Trends Microbiol. 26, 611–623. doi: 10.1016/j.tim.2017.12.002
Zhong, G. (2021). Chlamydia overcomes multiple gastrointestinal barriers to achieve long-lasting colonization. Trends Microbiol. 29, 1004–1012. doi: 10.1016/j.tim.2021.03.011
Zhou, Z., Tian, Q., Wang, L., Xue, M., Xu, D., and Zhong, G. (2021). Chlamydia spreads to the large intestine lumen via multiple pathways. Infect. Immun. 89:e0025421. doi: 10.1128/IAI.00254-21
Keywords: chlamydia, chlamydia pathogenesis, animal models, genital tract infections, gastrointestinal tract infections
Citation: Tian Q, Zhang T, Shu C, Han Z, Huang Y, Wan J, Wang L and Sun X (2024) Diverse animal models for Chlamydia infections: unraveling pathogenesis through the genital and gastrointestinal tracts. Front. Microbiol. 15:1386343. doi: 10.3389/fmicb.2024.1386343
Received: 15 February 2024; Accepted: 18 March 2024;
Published: 28 March 2024.
Edited by:
Volker Winstel, TWINCORE, Centre for Experimental and Clinical Infection Research, GermanyReviewed by:
Chuan Wang, University of South China, ChinaCopyright © 2024 Tian, Zhang, Shu, Han, Huang, Wan, Wang and Sun. This is an open-access article distributed under the terms of the Creative Commons Attribution License (CC BY). The use, distribution or reproduction in other forums is permitted, provided the original author(s) and the copyright owner(s) are credited and that the original publication in this journal is cited, in accordance with accepted academic practice. No use, distribution or reproduction is permitted which does not comply with these terms.
*Correspondence: Qi Tian, qi-tian@hotmail.com; Tianyuan Zhang, zty1911@126.com
†These authors have contributed equally to this work and share first authorship