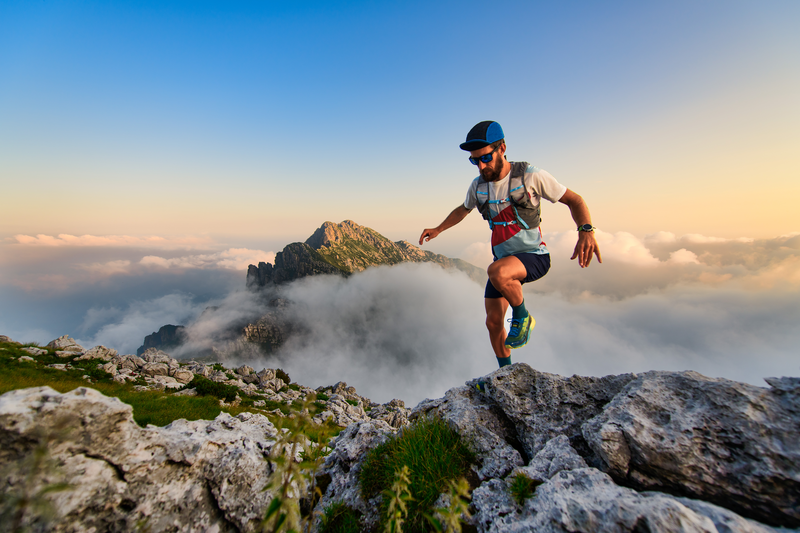
94% of researchers rate our articles as excellent or good
Learn more about the work of our research integrity team to safeguard the quality of each article we publish.
Find out more
SYSTEMATIC REVIEW article
Front. Microbiol. , 10 May 2024
Sec. Antimicrobials, Resistance and Chemotherapy
Volume 15 - 2024 | https://doi.org/10.3389/fmicb.2024.1385475
This article is part of the Research Topic New Therapeutic Strategies Against Carbapenem-Resistant Gram-negative Bacteria View all 14 articles
Systematic review registration: Gram-negative bacteria have been one of the most studied classes in the field of microbiology, especially in the context of globally alarming antimicrobial resistance levels to these pathogens over the course of the past decades. With high numbers of these microorganisms being described as multidrug-resistant (MDR), or even extended-drug-resistant (XDR) bacteria, specialists in the field have been struggling to keep up with higher prevalence of difficult-to-treat infections caused by such superbugs. The FDA approval of novel antimicrobials, such as cefiderocol (FDC), ceftolozane/tazobactam (C/T), ceftazidime/avibactam (CZA), imipenem/relebactam (IMR), sulbactam/durlobactam (SUL-DUR) and phase 3 clinical trials’ results of aztreonam/avibactam (ATM-AVI) has proven that, while all these substances provide encouraging efficacy rates, antibiotic resistance keeps up with the pace of drug development. Microorganisms have developed more extensive mechanisms of resistance in order to target the threat posed by these novel antimicrobials, thus equiring researchers to be on a constant lookout for other potential drug candidates and molecule development. However, these strategies require a proper understanding of bacterial resistance mechanisms to gain a comprehensive outlook on the issue. The present review aims to highlight these six antibiotic agents, which have brought hope to clinicians during the past decade, discussing general properties of these substances, as well as mechanisms and patterns of resistance, while also providing a short overview on further directions in the field.
https://www.crd.york.ac.uk/prospero/#searchadvanced, Identifier CRD42024505832.
Emergent antimicrobial resistance stands as a global public health issue that burdens clinicians, patients, and healthcare systems overall. Since the market approval of penicillin more than 80 years ago, the field of antibiotics has been in constant change, however, so was antibiotic resistance. Nowadays, it is thought that antimicrobial resistance lies as the cause of more than 700.000 yearly deaths globally, while hospital-acquired infection levels continue to rise in many parts of the world (Uddin et al., 2021). The last decades have witnessed a decreased interest of pharmaceutical companies regarding the development of novel antimicrobials, mostly due to the cost of production and poor return of investment in comparison with other drugs treating chronic disorders, for instance. Thus, the focus shifted toward the development of antimicrobial combinations, in addition to ongoing progress in the field of monoclonal antibodies and immunotherapy (Hutchings et al., 2019). Products such as cefiderocol (FDC), ceftolozane/tazobactam (C/T), ceftazidime/avibactam (CZA), imipenem/relebactam (IMR) aztreonam/avibactam (ATM-AVI) or sulbactam/durlobactam (SUL-DUR) have brought hope to both clinicians and the patients’ community by their promising efficacy rates in the case of difficult-to-treat infections. However, with most of these infections being caused by multidrug-resistant (MDR) or extended-drug-resistant (XDR) bacteria, these microorganisms have evolved in terms of resistance mechanisms even against these antimicrobial agents (Wang et al., 2020; Mushtaq et al., 2021; Teo et al., 2021; Gaibani et al., 2022; Karakonstantis et al., 2022). This review aims to briefly characterize these substances, highlighting the current patterns and mechanisms of resistance encountered in vitro.
The literature review was conducted independently by the authors in November–December 2023 based on the PRISMA 2020 guideline (Page et al., 2021). Articles from all years were sourced on PubMed. Systematic reviews, meta-analyses and clinical trials studying the desired 6 antimicrobials were included. General data about these antimicrobials, as well as resistance profiles of different Gram-negative pathogen strains against these agents have been studied. In addition, a search on PubChem has been conducted in order to obtain the chemical structures of the studied antibiotics. Moreover, a comprehensive assessment of clinical trials involving these antibiotics has been conducted using clinicaltrials.gov. Exclusion criteria were studies focusing only on pharmacokinetics and/or safety, articles presenting the antimicrobials in correlation with other bacterial pathogens. Keywords used in the search were ‘antimicrobial resistance,’ ‘ceftazidime/avibactam,’ ‘cefiderocol,’ ‘ceftolozane/tazobactam,’ ‘imipenem/relebactam,’ ‘aztreonam/avibactam,’ ‘sulbactam/durlobactam.’ The search formula used was “(antimicrobial resistance) AND [(ceftazidime/avibactam) OR (cefiderocol) OR (ceftolozane/tazobactam) OR (imipenem/relebactam) OR (aztreonam/avibactam) OR (sulbactam/durlobactam)].” Additionally, 10 articles have been selected for presenting further research directions, however the exclusion criteria were maintained. Search results were analyzed by all authors. PRISMA guidelines were followed, and bias risk was not assessed. In total, 1,653 records have been found, out of which 96 have been included as the references of this paper, following the screening criteria. This review has also been included in the International prospective register of systematic reviews (PROSPERO), with the registration ID CRD42024505832. The full flow of the scientific data collection can be consulted in Figure 1.
FDC stands as an improved siderophore cephalosporin, sharing structural assets with both ceftazidime (shown in Figure 2), with which it shares the same C-7 side chain, and cefepime, from which it borrows the pyrrolidinium group on the C-3 chain, which translates into an improved stability level against β-lactamases such as KPC (Klebsiella pneumoniae carbapenemase), NDM (New Delhi metallo-β-lactamase), OXA (oxacillin carbapenemase) or VIM (Verona integron-encoded metallo-β-lactamase) (Table 1). In the case of the C-7 side chain, FDC showcases groups responsible for providing a certain level of stability against bacterial β-lactamases (the oxime and dimethyl groups), as well as an acidic component conferring improved permeability of outer membranes. The innovative structural feature is a chlorocatechol nucleus on the C-3 side chain, which gives cefiderocol the ability to chelate iron. Thus, FDC uses specific iron active transporters in microorganisms to pass through the outer membrane, in a mechanism known as a ‘Trojan horse’ strategy (Sato and Yamawaki, 2019; Karakonstantis et al., 2022). Moreover, besides being stable against the action of β-lactamases, FDC shows high affinity for penicillin binding proteins (PBPs), especially PBP3 but also Klebsiella pneumoniae PBP2 and Pseudomonas aeruginosa PBP1a (Ito et al., 2018). Thus, FDC manages to interfere with cell wall synthesis, resulting in cellular apoptosis (Sato and Yamawaki, 2019).
Figure 2. Chemical structures of cefiderocol (A), ceftolozane/tazobactam (B), ceftazidime/avibactam (C), aztreonam (D), imipenem/relebactam (E), and sulbactam/durlobactam (F) (Avycaz, 2024; Aztreonam, 2024; Cefiderocol, 2024; Ceftolozane-Tazobactam, 2024; Durlobactam, 2024; Imipenem, 2024; Relebactam, 2024; Sulbactam, 2024).
Table 1. Action comparison of FDC (cefiderocol), C/T (ceftolozane/tazobactam), CZA (ceftazidime/avibactam), IMR (imipenem/relebactam), ATM-AVI (aztreonam/avibactam), SUL- DUR (sulbactam/durlobactam) against selected β-lactamases.
C/T is a molecular association between a cephalosporin, ceftolozane, and a β-lactamase inhibitor, tazobactam. Ceftolozane is structurally similar to ceftazidime (shown in Figure 2) however showcasing increased stability against AmpC (cephalosporinase class C) β-lactamases – mediated hydrolysis, benefiting from a heavier side chain, which is vital when encountering Pseudomonas aeruginosa resistance mechanisms. Tazobactam, on the other hand, is a β-lactamase inhibitor with a β-lactam structural core, making it inefficient against some carbapenemases encountered in novel MDR/XDR bacteria, such as NDM-1, KPC-2, KPC-3 or OXA-48 (Table 1; Van Duin and Bonomo, 2016; Teo et al., 2021).
CZA is constituted of a third-generation cephalosporin, ceftazidime, a molecule sharing chemical characteristics with ceftolozane (shown in Figure 2), and avibactam, a β-lactamase inhibitor which does not belong to the β-lactam class, being a diazabicyclooctane substance. In addition to the properties of ceftazidime, which are similar to the other novel-generation cephalosporins, avibactam creates a reversible covalent bond with the serine residues belonging to the β-lactamase active center, which provides it with extended stability against β-lactamases, even against KPC-2, KPC-3 or OXA-48. However, it is not effective against metallo-β-lactamase (IMP, VIM, NDM) producing pathogens, due to the structural characteristics of their active sites, which do not contain serine residues (Table 1; Van Duin and Bonomo, 2016; Mosley et al., 2016; Gaibani et al., 2022).
IMR combines imipenem, a carbapenem which interferes with the process of cellular wall synthesis by inactivating PBPs contained by the membrane of the bacterial cell, with relebactam, another diazabicyclooctane similar to avibactam (shown in Figure 2), which limits the efflux of the complex via a side chain that presents positive charging. While shown to be effective against the likes of KPC or AmpC, relebactam has been demonstrated to be inactivated by class B metallo-β-lactamases (IMP, NDM, VIM), also showing limited action against OXA-48-like producing pathogens (Table 1; Heo, 2021; Gaibani et al., 2022; O’Donnell and Lodise, 2022).
ATM-AVI is a molecular association currently in development, which combines aztreonam, a β-lactam agent stable against metallo-β-lactamase mediated hydrolysis, but otherwise susceptible to being degraded by ESBLs, KPCs or AmpCs, and avibactam, a diazabicyclooctane, which, by being effective against serine-containing β-lactamases, is considered to bring the final antimicrobial agent – ATM-AVI to a maximum level of effectiveness against pathogens producing most types of β-lactamases (Table 1; Mushtaq et al., 2021; Sader et al., 2023).
SUL-DUR, which has recently been approved by the FDA after successful results of phase III clinical trials, brings together two β-lactamase inhibitors – Sulbactam, one of the older antimicrobial agents, a penicillanic acid with limited anti-β-lactamase activity and Durlobactam, a synthetic diazabicyclooctane inhibiting class A, C and D β-lactamases. In terms of target sites, SUL targets PBP1a, PBP1b and PBP3, while PBP2 stands as DUR’s target. Together, they stand as a molecular association with efficient inhibitory activity against class A, C and D β-lactamase-producing Acinetobacter baumannii strains (Table 1; Keam, 2023; Karruli et al., 2023).
Since the 1940s, development of antimicrobials helped medicine evolve beyond the treatment of infectious diseases, also enabling now common practices, such as invasive maneuvers, improve their safety and efficacy. However, bacteria fought back bringing us to the present public health concern that antimicrobial resistance represents, which combines with decreasing interest for novel antibiotic development on the pharmaceutical companies’ side, mainly due to increased research costs and rapid emergence of resistance, leading to low economic turnover. In 2017, a priority list of pathogens for which new antimicrobials’ research and development is needed was released by the WHO, placing carbapenem-resistant Enterobacteriaceae as third in the ranking for critical pathogens (World Health Organization, 2017). In accordance with these reports, the second half of the last decade has brought fruitful updates on the approval of novel antimicrobial agents used for the treatment of infections due to MDR/XDR Gram-negative bacteria, especially complicated urinary tract infections (cUTIs), complicated intra-abdominal infections (cIAIs), hospital-acquired pneumonia (HAP) and, respectively, ventilator-associated pneumonia (VAP) (Naseer et al., 2021; Yusuf et al., 2021). Moreover, CZA has also been approved for usage in the case of pediatric patients (older than 3 months) suffering from cUTIs and cIAIs, used in co-therapy with metronidazole (Bassetti et al., 2020). In addition, 2023 witnessed the FDA approval of SUL-DUR for adult patients suffering from Acinetobacter baumannii HAP/VAP (Keam, 2023). Nonetheless, ATM-AVI currently stands as a very promising candidate for FDA approval, with recent phase-3 clinical trials showcasing effectiveness and good tolerance levels when tested for the treatment of cIAI, HAP and VAP (Carmeli et al., 2023). The FDA approval timeline and indications for the 6 antimicrobials in our paper can be found in Table 2. However, these antimicrobials are not intended for firstline usage, due to the secondary risk of decreased efficacy caused by emergent resistance. Four of them (FDC, CZA, C/T and IMR) are classified in the ‘reserve’ group of antibiotics by the WHO, thus should be treated as ‘last resort’ options. ATM-AVI and SUL-DUR have not been included on this list due to their approval happening after the release of the WHO 2021 AWaRe classification (World Health Organization, 2021).
Table 2. FDA approval timeline and indications for cefiderocol, ceftolozane/tazobactam, ceftazidime/avibactam, imipenem/relebactam, aztreonam/avibactam, sulbactam/durlobactam including approval extensions for different pathologies and, in the case of ceftolozane/tazobactam, extension for pediatric use.
Multiple clinical trials have been conducted in patients suffering from cUTI, cIAI, HAP/VAP and even sepsis, focusing on the effectiveness of these antimicrobials in real-life scenarios, as well as comparing clinical and microbiological results with those of antibiotics that have been used in clinical practice beforehand. Comparative results present the fact that all these novel antimicrobials are non-inferior to previous therapies considered to be the best available. The full listing of these trials can be found in Table 3. In addition, we aim to discuss each antimicrobial’s particularities against MDR/XDR microorganisms below, as shown by previously conducted studies, both in vitro and in vivo.
Table 3. Results of clinical trials showing non-inferior or improved favorable clinical response rates of patients to treatment with FDC, C/T, CZA, IMR, ATM-AVI, and SUL-DUR as compared with other antimicrobials.
While clinical trials reported in Table 3 prove that FDC is non-inferior to its comparators, there have also been smaller studies focusing on its antimicrobial properties against MDR/XDR Gram-negative bacteria. For instance, a 2023 in vitro study conducted in the United Arab Emirates revealed that FDC has been highly efficient (97.9% efficacy) against MDR and XDR Klebsiella pneumoniae isolates, including carbapenemase producers and double carbapenemase-producers (NDM and OXA-48-like) (Daoud et al., 2023). Similar studies indicated that FDC has potent antimicrobial activity against the vast majority of isolates, including MDR and carbapenem-non-susceptible strains (Jacobs et al., 2018; Kazmierczak et al., 2019). The ARGONAUT-I study exhibited consistent susceptibility levels to FDC in multiple bacterial species, including non-fermenters: 97.0% in Acinetobacter baumannii complex strains, 100% amongst Pseudomonas aeruginosa and Stenotrophomonas maltophilia isolates (Jacobs et al., 2018).
While C/T has received approval for several pathologies caused by Gram-negative pathogens, it is most commonly referred to as a viable treatment alternative for MDR/XDR Pseudomonas aeruginosa strains. Resistance patterns to C/T, as to most antimicrobials, vary from country to country, but recently published studies report effectiveness in more than 75% of cases (Venuti et al., 2023; Karlowsky et al., 2024; Mendes Pedro et al., 2024). However, a 2023 multicenter study focusing on various infections caused by MDR Pseudomonas aeruginosa (HAP, VAP, wound infections, UTI, IAI, catheter-related BSI) showcases that C/T does not provide significant differences regarding clinical outcome, when compared to CZA (Almangour et al., 2023). Additionally, an earlier multicentric study centering around gram-negative bacteria causing pneumonia concluded that, while C/T and CZA showed similar, encouraging susceptibility rates against Pseudomonas aeruginosa (96.0% for CZA and 95.9% for C/T), CZA was significantly more efficient against MDR Enterobacterales (99.2% susceptible to CZA, while only 53.8% susceptible to C/T) (Sader et al., 2020).
In addition to the 10 clinical trials concerning CZA included in Table 3, other studies reported significant efficacy of CZA against carbapenem-resistant and MDR Gram-negative pathogens. Wilson et al., focusing on CZA’s activity against Pseudomonas aeruginosa strains, concluded in a meta-analysis that this molecular association imposed a positive clinical outcome in 73% of infections with MDR or carbapenem-resistant Pseudomonas aeruginosa as etiologic agent (Wilson et al., 2021). Moreover, a study published in 2020 proved that over 90% of KPC-2 producing Klebsiella pneumoniae strains were susceptible to CZA, as well as this antimicrobial proving efficient against carbapenem-resistant Pseudomonas aeruginosa (Yang et al., 2020).
Data from published studies suggest that, while IMR proves as an efficient alternative when tackling MDR Enterobacterales (non-Morganellaceae Enterobacterales) such as Escherichia coli, K. pneumoniae, Enterobacter spp., Citrobacter spp. or Serratia spp., with a susceptibility rate of over 89% against these pathogens, it has shown low activity levels when encountering MBL-producing Enterobacterales, while also posing limited action against OXA-48-like producing Gram-negatives (Karlowsky et al., 2023). Moreover, IMR has proved inefficient against carbapenem-resistant Acinetobacter baumannii (CRAB), consistent with previous reports of CRAB resistance to imipenem (Mansour et al., 2021). Thus, IMR is now considered a viable treatment alternative when coming across several MDR Enterobacterales, unless encountering bacteria producing MBL or OXA-48 as an enzymatic resistance mechanism.
The last years have seen multiple reports attempting to characterize the combination of aztreonam with avibactam in multiple clinical contexts. Nonetheless, reports show impressive susceptibility rates of Gram-negative bacteria to ATM-AVI (>97%), with this feature preserved in the MDR and XDR subgroups (Wise et al., 2023). However, with MDR/XDR/PDR microorganisms evolving in terms of resistance mechanisms, there is the need for more research in order to appropriately determine the activity of ATM-AVI against highly resistant strains. For instance, a 2023 study conducted in China concluded that ATM-AVI is indeed more efficient than CZA in MBL-producing XDR/PDR Pseudomonas aeruginosa isolates, but these microorganisms could potentially carry rare MBL encoding genes, thus it remains to be seen whether or not these resistance genes will become more commonly encountered in futurely described isolates (Kang et al., 2023). Other, smaller studies focused on more diverse Gram-negative microorganisms, such as E. coli, K. pneumoniae, Enterobacter spp., Proteus mirabilis or Morganella morganii. For instance, a 2021-published study assessing MBL-producing Gram-negative strains discovered that the addition of avibactam to aztreonam in previously not-susceptible strains results in significant in-vitro antimicrobial activity against 85% of strains (Bhatnagar et al., 2021).
SUL-DUR remains a highly efficient treatment alternative when treating infections caused by Acinetobacter baumannii. In a study characterizing globally collected strains from 2016 to 2017, SUL-DUR proved susceptible in over 97% of isolates, far more efficient compared to sulbactam alone (less than 50% susceptibility of isolates) (Karlowsky et al., 2022). When considering CRAB, SUL-DUR still shows promising results, with over 70% of CRAB isolates proving susceptible (Findlay et al., 2022).
Gram-negative pathogens exhibit resistance via 3 main mechanisms (enzyme-mediated antibiotic inactivation, structural changes of antimicrobial targets and cell permeability changes), closely linked to the active targets of antimicrobials and bacterial cell structure. Resistance can be intrinsic, when linked to chromosomal abnormalities in the bacterial genome, or acquired, via bacterial communication through transposable genetic elements, such as transferable plasmids (Breijyeh et al., 2020).
In accordance with the Ambler classification, there are 4 main categories of β-lactamases: A (which includes KPC and CTX-M, among others), B (which are known as the metallo-β-lactamases, such as IMP, VIM or NDM), C (AmpC and extended-spectrum variants), and D (which includes OXA). From a structural point of view, A, C and D groups contain serine residues at the enzyme’s active core, while class B showcases ions of zinc (Sawa et al., 2020). In this case, antimicrobial activity is diminished through the changes of structure in the enzymatic amino-acid chains, these mutant enzymes showcasing insertions or, most frequently, substitutions (Wang et al., 2020). Amino-acid substitutions have been comprehensively studied during the past years, consistent with increasing development in peptide engineering, in order to provide a better understanding of their role in antimicrobial interactions, especially concerning CZA and ATM-AVI. Notably, the amino-acid substitutions which can occur in the Ω-loop of β-lactamases, a structure playing a vital role in the catalytic activity of the enzyme, are linked to increased resistance to ceftazidime, via a mechanism known as ‘covalent trapping,’ leading to much faster hydrolysis of the substrate (Levitt et al., 2012). More specifically, studies focusing on the Asp179Asn substitution in the structure of KPC-2 confirmed that this mutation increases the MIC of CZA to these bacterial strains, as well as the fact that the addition of avibactam to ceftazidime does in fact increase efficacy against KPC-2 mediated resistance, but it remained insufficient to overcome the resistance conferred by the Asp179 mutations (Barnes et al., 2017). However, the same study showcased that ATM-AVI is efficient against these mutations-carrying variants. Another study revealed that there are no less than 65 structurally different KPC variants harboring resistance to CZA, with the majority of them (43/65) showcasing mutations in the aforementioned Ω-loop and 63% of them (41/65) also presenting insertions or deletions (Hobson et al., 2022). Moreover, resistance to carbapenems has been increasingly reported in AmpC presenting Enterobacterales (Breijyeh et al., 2020).
Penicillin-binding proteins (PBPs) are protein structures that play a key role in bacterial wall synthesis, which makes them the target sites of FDC, C/T, CZA, IMR ATM-AVI and SUL-DUR. These structures, which can be divided into two categories taking into account their molecular weight - low-molecular weight PBPs and high-molecular weight PBPs, are inhibited by the antimicrobial substances they come in contact with, thus inhibiting the formation of peptidoglycan, a key component of the bacterial cell wall (Levitt et al., 2012). Structural changes at the PBP level have been reportedly linked to increased MIC levels, Alm et al. suggesting that a PBP3 four amino acid insertion is linked to decreased susceptibility to ATM-AVI of Escherichia coli (Alm et al., 2015). Moreover, studies show that the same mutation in E. coli isolates leads to elevated MIC levels of CZA (Wang et al., 2020). Additionally, mutations of PBP2, PBP3 and, morerarely, PBP1a and PBP1b are considered the most commonly encountered resistance pathways to SUL-DUR (Principe et al., 2022; Karruli et al., 2023). Ultimately, PBP-mediated resistance has been frequently highlighted in recent research, with most antimicrobials preferentially targeting one PBP with higher affinity, hence the antimicrobial combinations we have discussed having been constructed in order to inhibit more PBPs for utmost efficiency. The literature is scarce in describing the frequency of these structural changes’ occurrence, as it most certainly may be a multifactorial characteristic, however researchers consistently report that these modifications greatly impact the efficacy rates of antimicrobials (Sethuvel et al., 2023). Another potential mechanism of resistance is considered to be mutations in the structures of siderophore receptors1or iron transporters. These phenomena are of utmost importance when it comes to bacterial resistance against cefiderocol, due to its chemical properties.
Several studies have described the issue, highlighting multiple genes involved in iron transport pathways and the structure of the siderophore receptor (for instance: cirA, pirA, other TonB-dependent receptor genes, etc.) which can be potentially mutated or underexpressed, thus reducing intracellular uptake of cefiderocol and improving bacterial resistance (Domingues et al., 2023). While it is unsurprising that disturbances in iron metabolic pathway interact with cefiderocol’s antimicrobial activity, there have been studies reporting contradictory results, which imply the need for future biomolecular research highlighting the role of these structural changes in cefiderocol resistance (Karakonstantis et al., 2022).
Porins are outer membrane proteins that play a huge role in cellular permeability regulation, allowing hydrophilic substances to enter the cell via passive transport, necessary for cellular processes. Moreover, porins are also closely linked with the peptidoglycan in the bacterial wall structure, adding to their undeniable role in outer cover stability. Gram-negative bacteria present 5 porin types: OmpA, OmpC, OmpF, OmpW, OmpX. The loss of these porins and structural mutations have been shown to decrease antibiotic susceptibility in microorganisms presenting variants of these proteins, thus elevated MICs or even resistance rates have been observed in the case of most antimicrobials, including carbapenems, β-lactamases or third generation cephalosporins. Moreover, it has been shown that interactions between these porins also contribute to the development of resistance (Zhou et al., 2023). More specifically, taking Klebsiella pneumoniae as an example, outer membrane proteins OmpK35 and OmpK36 play a very important role in antimicrobial resistance, as there have been reported strong correlations between single or double deletions and increases in MIC levels against multiple antimicrobials (Tsai et al., 2011). Furthermore, it has been shown that homologous outer membrane proteins in different bacterial species present different characteristics. For example, Sugawara et al. discovered that OmpK35 and OmpK36 provide more efficient diffusion of β-lactams through the bacterial membrane than E. coli OmpF and OmpC, by creating channels with increased permeability and size (Sugawara et al., 2016).
Other bacterial components that contribute to permeability regulations are efflux pumps. While they can be linked to physiological processes, such as the elimination of cellular metabolites created on the course of respiration or the excretion of siderophores, bacterial-originating iron chelators, an essential adaptive mechanism to low-iron environments, efflux pumps also serve as a way of excreting antimicrobial substances, thus contributing to increased resistance. While they can either be chromosomally encoded or acquired via interbacterial communication, by transposons or plasmids, these structures are linked to complex intracellular regulation systems, grouping themselves into bacterial efflux systems, which can either excrete a specific antimicrobial, or more classes. For instance, efflux pumps belonging to the RND (resistance nodulation cell division) family excrete β-lactams, fluoroquinolones and linezolid, among others, while structures such as TetA are specific for tetracycline (Sharma et al., 2019; Nishino et al., 2021).
While these novel antimicrobial agents still present favorable effectiveness against a wide range of microorganisms, evolving resistance is a thing the healthcare community should be constantly aware of. Resistance mechanisms need to be further studied in order to be properly explored as potential targets for future therapeutic methods. Besides this, there is a constant need for novel strategies to be developed and, while antibiotic production finds itself at low levels, the focus is switching toward modern methods, such as antimicrobial combinations, immunotherapy, or molecule modeling. Considering the changes in the targets of antimicrobials, novel molecule development should be centered around updating stereospecific binding structures. Moreover, efflux pumps inhibitors are slowly shown as effective adjuvants or alternatives to antimicrobial agents in infections caused by resistant pathogens (Sharma et al., 2019). Nevertheless, the field of molecular science has been in constant evolution and novel approaches have shown promising results. One example of these is the development of dendrimers, nano-sized molecules previously researched for cancer treatment, which have recently been tested for antimicrobial activity via PBP affinity, showcasing promising results (Ahmed et al., 2016). Their silver salt structures have been increasingly studied in order to produce updated molecules, with improved MIC reduction capabilities, via elevated cationic characteristics through extra amino acid conjugation (Schito et al., 2021). More recently, molecular biology has further investigated the field of peptide-derived antibiotic development, which shall open a gateway to new-antimicrobial class discoveries in the future, by addressing the mechanisms of resistance that have been previously encountered, thus enabling the engineering of new means-of-action agents (Upert et al., 2021). After a study conducted both in vitro and on mouse models, Zosurabalpin now stands as a clinical candidate for the treatment of severe infections caused by CRAB. By successfully inhibiting the transport of lipopolysaccharide (LPS), more exactly the LptB2FGC complex, this drug provides alterations to the structural integrity of the bacterial cell. This discovery shines new light on how targeting LPS should be a viable strategy for treatment development against XDR/PDR (pandrug-resistant) microorganisms, however more extensive studies need to be performed (Pahil et al., 2024; Zampaloni et al., 2024).
Another interest-worthy field of advancements is represented by the usage of bacteriophages in order to efficiently treat these infections. These agents essentially act via a number of mechanisms, for instance pore creation and enzyme-mediated degradation of the bacterial peptidoglycan, resulting in bacterial cell lysis. While under development for human use, studies involving animal models have shown promising results, thus by adapting these treatments for human usage via improving pharmaceutical properties, we could see important progress in the field in the upcoming years (Zagaliotis et al., 2022).
Nonetheless, it is worth mentioning that there are several other antimicrobials undergoing testing for MDR Gram-negative organisms, such as cefepime/zidebactam or fosfomycin (Tirlangi et al., 2023; Meschiari et al., 2024). Also, while polymyxin analogs have not been previously taken into account when discussing the development of novel antimicrobials, there has been an increased interest in polymyxin engineering via amino-acid substitutions, thus it will be interesting to see how these substances will be able to perform in vivo, combined with the usage of machine learning and/or artificial intelligence applied to structuring of polymyxin analogs and other bioactive components (Li et al., 2021).
Several limitations, although frequently encountered in other systematic review papers, need to be taken into consideration. Firstly, we have included only one scientific database into our research process. Even though we consider it to be the most appropriate for conducting a systematic review on our topic, some relevant information found in other databases might have been overlooked. Moreover, the quality of clinical trials included has not been assessed, as their selection was made based on relevance.
In a world which finds itself in continuous change, antimicrobial resistance still poses a consistent challenge for the healthcare & patients’ communities alike. The six antimicrobials that we have covered in this review are meant to be viable alternatives to the treatment schemes of MDR/XDR/PDR microorganism-borne infections. However, we believe that, in the light of recent emerging resistance of bacteria to these antibiotic agents, susceptibility rates need to be continuously monitored, in order to obtain an appropriate outlook on the course of treatment in each case. Additionally, more in-depth research is needed in order to fully understand the mechanisms of resistance against novel antimicrobials, as well as for finding updated means of tackling it. Nonetheless, antibiotic drug discovery should remain a priority for the healthcare industry in order for novel agents to become potential candidates for clinical usage, as well as alternative molecules arising from the studied means of resistance to be further developed.
The original contributions presented in the study are included in the article/supplementary material, further inquiries can be directed to the corresponding author.
MD: Conceptualization, Data curation, Formal analysis, Investigation, Methodology, Resources, Validation, Visualization, Writing – original draft, Writing – review & editing. DT: Conceptualization, Data curation, Formal analysis, Investigation, Methodology, Supervision, Validation, Visualization, Writing – review & editing.
The author(s) declare that financial support was received for the research, authorship, and/or publication of this article. This work was supported by the University of Medicine and Pharmacy Carol Davila, through the institutional program Publish not Perish.
The authors declare that the research was conducted in the absence of any commercial or financial relationships that could be construed as a potential conflict of interest.
All claims expressed in this article are solely those of the authors and do not necessarily represent those of their affiliated organizations, or those of the publisher, the editors and the reviewers. Any product that may be evaluated in this article, or claim that may be made by its manufacturer, is not guaranteed or endorsed by the publisher.
MDR, multidrug-resistant; XDR, extended-drug-resistant; PDR, pandrug-resistant; CZA, ceftazidime/avibactam; IMR, imipenem/relebactam; C/T, ceftolozane/tazobactam; FDC, cefiderocol; ATM-AVI, aztreonam/avibactam; SUL-DUR, sulbactam/durlobactam; cUTIs, complicated Urinary Tract Infections; cIAIs, complicated Intra-Abdominal Infections; HAP, hospital-acquired pneumonia; VAP, ventilator-associated pneumonia; KPC, Klebsiella pneumoniae carbapenemase; OXA, oxacillin carbapenemase; VIM, Verona integron-encoded metallo-β-lactamase; NDM, New Delhi metallo-β-lactamase; IMP, imipenemase; AmpC, cephalosporinase class C; CRAB, carbapenem-resistant Acinetobacter baumannii; PBP, penicillin-binding protein; ESBL, extended-spectrum β-lactamase; FDA, US Food and Drug Administration; MIC, minimum inhibitory concentration; BSI, bloodstream infections.
Ahmed, S., Vepuri, S. B., Ramesh, M., Kalhapure, R., Suleman, N., and Govender, T. (2016). In silico characterization of the binding affinity of dendrimers to penicillin-binding proteins (Pbps): can pbps be potential targets for antibacterial dendrimers? Appl. Biochem. Biotechnol. 178, 1546–1566. doi: 10.1007/s12010-015-1967-6
Alm, R. A., Johnstone, M. R., and Lahiri, S. D. (2015). Characterization of Escherichia coli NDM isolates with decreased susceptibility to aztreonam/avibactam: role of a novel insertion in PBP3. J. Antimicrob. Chemother. 70, 1420–1428. doi: 10.1093/jac/dku568
Almangour, T. A., Ghonem, L., Alassiri, D., Aljurbua, A., Al Musawa, M., Alharbi, A., et al. (2023). Ceftolozane-Tazobactam versus ceftazidime-avibactam for the treatment of infections caused by multidrug-resistant Pseudomonas aeruginosa: a multicenter cohort study. Antimicrob. Agents Chemother. 67:e0040523. doi: 10.1128/aac.00405-23
Arakawa, S., Kawahara, K., Kawahara, M., Yasuda, M., Fujimoto, G., Sato, A., et al. (2019). The efficacy and safety of tazobactam/ceftolozane in Japanese patients with uncomplicated pyelonephritis and complicated urinary tract infection. J. Infect. Chemother. 25, 104–110. doi: 10.1016/j.jiac.2018.10.009
Avycaz (2024). Available at: https://pubchem.ncbi.nlm.nih.gov/compound/90643431, https://pubchem.ncbi.nlm.nih.gov/compound/Avycaz (Accessed January 18, 2024)
Aztreonam (2024). Available at: https://pubchem.ncbi.nlm.nih.gov/compound/5742832 (Accessed January 18, 2024).
Barnes, M. D., Winkler, M. L., Taracila, M. A., Page, M. G., Desarbre, E., Kreiswirth, B. N., et al. (2017). Klebsiella pneumoniae Carbapenemase-2 (KPC-2), substitutions at ambler position Asp179, and resistance to ceftazidime-avibactam: unique antibiotic-resistant phenotypes emerge from β-lactamase protein engineering. mBio 8:e00528-17. doi: 10.1128/mBio.00528-17
Bassetti, M., Echols, R., Matsunaga, Y., Ariyasu, M., Doi, Y., Ferrer, R., et al. (2021). Efficacy and safety of cefiderocol or best available therapy for the treatment of serious infections caused by carbapenem-resistant gram-negative bacteria (credible-cr): a randomised, open-label, multicentre, pathogen-focused, descriptive, phase 3 trial. Lancet Infect. Dis. 21, 226–240. doi: 10.1016/S1473-3099(20)30796-9
Bassetti, M., Peghin, M., Mesini, A., and Castagnola, E. (2020). Optimal management of complicated infections in the pediatric patient: the role and utility of ceftazidime/avibactam. Infect Drug Resist. 13, 1763–1773. doi: 10.2147/IDR.S209264
Bhatnagar, A., Boyd, S., Sabour, S., Bodnar, J., Nazarian, E., Peinovich, N., et al. (2021). Aztreonam-avibactam susceptibility testing program for metallo-beta-lactamase-producing enterobacterales in the antibiotic resistance laboratory network, March 2019 to December 2020. Antimicrob. Agents Chemother. 65:e0048621. doi: 10.1128/AAC.00486-21
Bradley, J. S., Broadhurst, H., Cheng, K., Mendez, M., Newell, P., Prchlik, M., et al. (2019a). Safety and efficacy of ceftazidime-avibactam plus metronidazole in the treatment of children ≥ 3 months to < 18years with complicated intra-abdominal infection: results from a phase 2, randomized, controlled trial. Pediatr. Infect. Dis. J. 38, 816–824. doi: 10.1097/INF.0000000000002392
Bradley, J. S., Roilides, E., Broadhurst, H., Cheng, K., Huang, L.-M., MasCasullo, V., et al. (2019b). Safety and efficacy of ceftazidime–avibactam in the treatment of children ≥ 3 months to <18 years with complicated urinary tract infection: results from a phase 2 randomized, controlled trial. Pediatr. Infect. Dis. J. 38, 920–928. doi: 10.1097/INF.0000000000002395
Breijyeh, Z., Jubeh, B., and Karaman, R. (2020). Resistance of gram-negative bacteria to current antibacterial agents and approaches to resolve it. Molecules 25:1340. doi: 10.3390/molecules25061340
Carmeli, Y., Armstrong, J., Laud, P. J., Newell, P., Stone, G., Wardman, A., et al. (2016). Ceftazidime- avibactam or best available therapy in patients with ceftazidime-resistant Enterobacteriaceae and 40 Pseudomonas aeruginosa complicated urinary tract infections or complicated intra-abdominal infections (reprise): a randomised, pathogen-directed, phase 3 study. Lancet Infect. Dis. 16, 661–673. doi: 10.1016/S1473-3099(16)30004-4
Carmeli, Y., Cisneros, J.-M., Paul, M., Daikos, G. L., Wang, M., Cisneros, J. T., et al. (2023). 2893 a. efficacy and safety of aztreonam-avibactam for the treatment of serious infections due to gram-negative bacteria, including metallo-β-lactamase-producing pathogens: phase 3 revisit study. Open forum. Infect. Dis. 10:ofad500.2476. doi: 10.1093/ofid/ofad500.2476
Cefiderocol (2024). Available at: https://pubchem.ncbi.nlm.nih.gov/compound/77843966 (Accessed January 18, 2024).
Ceftolozane-Tazobactam (2024). Available at: https://pubchem.ncbi.nlm.nih.gov/compound/165413180 (Accessed January 18, 2024).
Chaftari, A.-M., Hachem, R., Malek, A. E., Mulanovich, V. E., Szvalb, A. D., Jiang, Y., et al. (2022). A prospective randomized study comparing ceftolozane/tazobactam to standard of care in the management of neutropenia and fever in patients with hematological malignancies. Open Forum Infect. Dis. 9:ofac079. doi: 10.1093/ofid/ofac079
Daoud, L., Al-Marzooq, F., Ghazawi, A., Anes, F., and Collyns, T. (2023). High efficacy and enhanced synergistic activity of the novel siderophore-cephalosporin cefiderocol against multidrug-resistant and extensively drug-resistant Klebsiella pneumoniae from inpatients attending a single hospital in the United Arab Emirates. J. Infect. Public Health 16, 33–44. doi: 10.1016/j.jiph.2023.11.003
Domingues, S., Lima, T., Saavedra, M. J., and Da Silva, G. J. (2023). An overview of Cefiderocol’s therapeutic potential and underlying resistance mechanisms. Life 13:1427. doi: 10.3390/life13071427
Durlobactam (2024). Available at: https://pubchem.ncbi.nlm.nih.gov/compound/89851852 (Accessed January 18, 2024).
Findlay, J., Poirel, L., Bouvier, M., and Nordmann, P. (2022). In vitro activity of sulbactam-durlobactam against carbapenem-resistant Acinetobacter baumannii and mechanisms of resistance. J. Glob. Antimicrob. Resist. 30, 445–450. doi: 10.1016/j.jgar.2022.05.011
Gaibani, P., Giani, T., Bovo, F., Lombardo, D., Amadesi, S., Lazzarotto, T., et al. (2022). Resistance to ceftazidime/avibactam, meropenem/vaborbactam and imipenem/relebactam in gram-negative mdr bacilli: molecular mechanisms and susceptibility testing. Antibiotics 11:628. doi: 10.3390/antibiotics11050628
Heo, Y.-A. (2021). Imipenem/cilastatin/relebactam: a review in gram-negative bacterial infections. Drugs 81, 377–388. doi: 10.1007/s40265-021-01471-8
Hobson, C. A., Pierrat, G., Tenaillon, O., Bonacorsi, S., Bercot, B., Jaouen, E., et al. (2022). Klebsiella pneumoniae Carbapenemase variants resistant to ceftazidime-avibactam: an evolutionary overview. Antimicrob. Agents Chemother. 66:e0044722. doi: 10.1128/aac.00447-22
Hutchings, M. I., Truman, A. W., and Wilkinson, B. (2019). Antibiotics: past, present and future. Curr. Opin. Microbiol. 51, 72–80. doi: 10.1016/j.mib.2019.10.008
Imipenem (2024) Available at: https://pubchem.ncbi.nlm.nih.gov/compound/104838 (Accessed January 18, 2024).
Ito, A., Sato, T., Ota, M., Takemura, M., Nishikawa, T., Toba, S., et al. (2018). In vitro antibacterial properties of Cefiderocol, a novel Siderophore cephalosporin, against gram-negative Bacteria. Antimicrob. Agents Chemother. 62:e01454-17. doi: 10.1128/AAC.01454-17
Jackson, C.-C. A., Newland, J., Dementieva, N., Lonchar, J., Su, F.-H., Huntington, J. A., et al. (2023). Safety and efficacy of ceftolozane/tazobactam plus metronidazole versus meropenem from a phase 2, randomized clinical trial in pediatric participants with complicated intra-abdominal infection. Pediatr. Infect. Dis. J. 42, 557–563. doi: 10.1097/INF.0000000000003911
Jacobs, M. R., Abdelhamed, A. M., Good, C. E., Rhoads, D. D., Hujer, K. M., Hujer, A. M., et al. (2018). ARGONAUT-I: activity of Cefiderocol (S-649266), a Siderophore cephalosporin, against gram-negative Bacteria, including Carbapenem-resistant nonfermenters and Enterobacteriaceae with defined extended-Spectrum β-lactamases and Carbapenemases. Antimicrob. Agents Chemother. 63, e01801–e01818. doi: 10.1128/AAC.01801-18
Kang, Y., Xie, L., Yang, J., and Cui, J. (2023). Optimal treatment of ceftazidime- avibactam and aztreonam-avibactam against bloodstream infections or lower respiratory tract infections caused by extensively drug-resistant or pan drug-resistant (XDR/PDR) Pseudomonas aeruginosa. Front. Cell. Infect. Microbiol. 13:1023948. doi: 10.3389/fcimb.2023.1023948
Karakonstantis, S., Rousaki, M., and Kritsotakis, E. I. (2022). Cefiderocol: systematic review of mechanisms of resistance, heteroresistance and in vivo emergence of resistance. Antibiotics 11:723. doi: 10.3390/antibiotics11060723
Karlowsky, J. A., Hackel, M. A., McLeod, S. M., and Miller, A. A. (2022). In vitro activity of Sulbactam-Durlobactam against global isolates of Acinetobacter baumannii-calcoaceticus complex collected from 2016 to 2021. Antimicrob. Agents Chemother. 66:e0078122. doi: 10.1128/aac.00781-22
Karlowsky, J. A., Lob, S. H., Bauer, K. A., Esterly, J., Siddiqui, F., Young, K., et al. (2024). Activity of ceftolozane/tazobactam, imipenem/relebactam and ceftazidime/avibactam against clinical gram-negative isolates-SMART United States 2019-21. JAC Antimicrob Resist 6:dlad152. doi: 10.1093/jacamr/dlad152
Karlowsky, J. A., Wise, M. G., Chen, W. T., Siddiqui, F., Young, K., Motyl, M. R., et al. (2023). Susceptibility of gram-negative isolates collected in South Korea to imipenem/relebactam and comparator agents-SMART 2018-21. JAC Antimicrob. Resist. 6:dlad149. doi: 10.1093/jacamr/dlad149
Karruli, A., Migliaccio, A., Pournaras, S., Durante-Mangoni, E., and Zarrilli, R. (2023). Cefiderocol and sulbactam-durlobactam against carbapenem-resistant Acinetobacter baumannii. Antibiotics 12:1729. doi: 10.3390/antibiotics12121729
Kaye, K. S., Shorr, A. F., Wunderink, R. G., Du, B., Poirier, G. E., Rana, K., et al. (2023). Efficacy and safety of sulbactam–durlobactam versus colistin for the treatment of patients with serious infections caused by Acinetobacter baumannii–calcoaceticus complex: a multicentre, randomised, active-controlled, phase 3, non-inferiority clinical trial (attack). Lancet Infect. Dis. 23, 1072–1084. doi: 10.1016/S1473-3099(23)00184-6
Kazmierczak, K. M., Tsuji, M., Wise, M. G., Hackel, M., Yamano, Y., Echols, R., et al. (2019). In vitro activity of cefiderocol, a siderophore cephalosporin, against a recent collection of clinically relevant carbapenem-non-susceptible gram-negative bacilli, including serine carbapenemase- and metallo-β-lactamase-producing isolates (SIDERO-WT-2014 study). Int. J. Antimicrob. Agents 53, 177–184. doi: 10.1016/j.ijantimicag.2018.10.007
Keam, S. J. (2023). Sulbactam/durlobactam: first approval. Drugs 83, 1245–1252. doi: 10.1007/s40265-023-01920-6
Kohno, S., Bando, H., Yoneyama, F., Kikukawa, H., Kawahara, K., Shirakawa, M., et al. (2021). The safety and efficacy of relebactam/imipenem/cilastatin in Japanese patients with complicated intra- abdominal infection or complicated urinary tract infection: a multicenter, open-label, noncomparative phase 3 study. J. Infect. Chemother. 27, 262–270. doi: 10.1016/j.jiac.2020.09.032
Kollef, M. H., Novácˇek, M., Kivistik, Ü., Réa-Neto, Á., Shime, N., Martin-Loeches, I., et al. (2019). Ceftolozane–tazobactam versus meropenem for treatment of nosocomial pneumonia (aspect-np): a randomised, controlled, double-blind, phase 3, non-inferiority trial. Lancet Infect. Dis. 19, 1299–1311. doi: 10.1016/S1473-3099(19)30403-7
Levitt, P. S., Papp-Wallace, K. M., Taracila, M. A., Hujer, A. M., Winkler, M. L., Smith, K. M., et al. (2012). Exploring the role of a conserved class a residue in the ω-loop of kpc-2 β-lactamase. J. Biol. Chem. 287, 31783–31793. doi: 10.1074/jbc.M112.348540
Li, J., Guan, D., Chen, F., Shi, W., Lan, L., and Huang, W. (2021). Total and Semisyntheses of Polymyxin analogues with 2-Thr or 10-Thr modifications to decipher the structure-activity relationship and improve the antibacterial activity. J. Med. Chem. 64, 5746–5765. doi: 10.1021/acs.jmedchem.0c02217
Lucasti, C., Hershberger, E., Miller, B., Yankelev, S., Steenbergen, J., Friedland, I., et al. (2014). Multicenter, double-blind, randomized, phase ii trial to assess the safety and efficacy of ceftolozane-tazobactam plus metronidazole compared with meropenem in adult patients with complicated intra-abdominal infections. Antimicrob. Agents Chemother. 58, 5350–5357. doi: 10.1128/AAC.00049-14
Lucasti, C., Popescu, I., Ramesh, M. K., Lipka, J., and Sable, C. (2013). Comparative study of the efficacy and safety of ceftazidime/avibactam plus metronidazole versus meropenem in the treatment of complicated intra-abdominal infections in hospitalized adults: results of a randomized, double-blind, phase II trial. J. Antimicrob. Chemother. 68, 1183–1192. doi: 10.1093/jac/dks523
Lucasti, C., Vasile, L., Sandesc, D., Venskutonis, D., McLeroth, P., Lala, M., et al. (2016). Phase 2, dose- ranging study of relebactam with imipenem-cilastatin in subjects with complicated intra-abdominal infection. Antimicrob. Agents Chemother. 60, 6234–6243. doi: 10.1128/AAC.00633-16
Mansour, H., Ouweini, A. E. L., Chahine, E. B., and Karaoui, L. R. (2021). Imipenem/cilastatin/relebactam: a new carbapenem β-lactamase inhibitor combination. Am. J. Health Syst. Pharm. 78, 674–683. doi: 10.1093/ajhp/zxab012
Mazuski, J. E., Gasink, L. B., Armstrong, J., Broadhurst, H., Stone, G. G., Rank, D., et al. (2016). Efficacy and safety of ceftazidime-avibactam plus metronidazole versus meropenem in the treatment of complicated intra-abdominal infection: results from a randomized, controlled, double-blind, phase 3 program. Clin. Infect. Dis. 62, 1380–1389. doi: 10.1093/cid/ciw133
Mendes Pedro, D., Paulo, S. E., Santos, C. M., Fonseca, A. B., Melo Cristino, J., Pereira, Á. A., et al. (2024). Extensively drug-resistant Pseudomonas aeruginosa: clinical features and treatment with ceftazidime/avibactam and ceftolozane/tazobactam in a tertiary care university hospital center in Portugal - a cross-sectional and retrospective observational study. Front. Microbiol. 15:1347521. doi: 10.3389/fmicb.2024.1347521
Meschiari, M., Faltoni, M., Kaleci, S., Tassoni, G., Orlando, G., Franceschini, E., et al. (2024). Intravenous Fosfomycin in combination regimens as a treatment option for difficult-to-treat Infenctions due to multidrug-resistant gram-negative organisms: a real-life experience. Int. J. Antimicrob. Agents 63:107134. doi: 10.1016/j.ijantimicag.2024.107134
Mikamo, H., Monden, K., Miyasaka, Y., Horiuchi, T., Fujimoto, G., Fukuhara, T., et al. (2019). The efficacy and safety of tazobactam/ceftolozane in combination with metronidazole in Japanese patients with complicated intra-abdominal infections. J. Infect. Chemother. 25, 111–116. doi: 10.1016/j.jiac.2018.10.012
Mosley, J. F. 2nd, Smith, L. L., Parke, C. K., Brown, J. A., Wilson, A. L., and Gibbs, L. V. (2016). Ceftazidime-avibactam (Avycaz): for the treatment of complicated intra-abdominal and urinary tract infections. P T. 41, 479–483.
Motsch, J., Murta De Oliveira, C., Stus, V., Köksal, I., Lyulko, O., Boucher, H. W., et al. (2020). Restore-Imi 1: a multicenter, randomized, double-blind trial comparing efficacy and safety of imipenem/relebactam vs colistin plus imipenem in patients with imipenem-nonsusceptible bacterial infections. Clin. Infect. Dis. 70, 1799–1808. doi: 10.1093/cid/ciz530
Mushtaq, S., Vickers, A., Ellaby, N., Woodford, N., and Livermore, D. M. (2021). Selection and characterization of mutational resistance to aztreonam/avibactam in β-lactamase-producing Enterobacterales. J. Antimicrob. Chemother. 77, 98–111. doi: 10.1093/jac/dkab346
Naseer, S., Weinstein, E. A., Rubin, D. B., Suvarna, K., Wei, X., Higgins, K., et al. (2021). Us food and drug administration (Fda): benefit-risk considerations for cefiderocol (Fetroja®). Clin. Infect. Dis. 72, e1103–e1111. doi: 10.1093/cid/ciaa1799
Nishino, K., Yamasaki, S., Nakashima, R., Zwama, M., and Hayashi-Nishino, M. (2021). Function and inhibitory mechanisms of multidrug efflux pumps. Front. Microbiol. 12:737288. doi: 10.3389/fmicb.2021.737288
O’Donnell, J. N., and Lodise, T. P. (2022). New perspectives on antimicrobial agents: imipenem-relebactam. Antimicrob. Agents Chemother. 66:e0025622. doi: 10.1128/aac.00256-22
Page, M. J., McKenzie, J. E., Bossuyt, P. M., Boutron, I., Hoffmann, T. C., Mulrow, C. D., et al. (2021). The PRISMA 2020 statement: an updated guideline for reporting systematic reviews. BMJ 372:n71. doi: 10.1136/bmj.n71
Pahil, K. S., Gilman, M. S. A., Baidin, V., Clairfeuille, T., Mattei, P., Bieniossek, C., et al. (2024). A new antibiotic traps lipopolysaccharide in its intermembrane transporter. Nature 625, 572–577. doi: 10.1038/s41586-023-06799-7
Portsmouth, S., Van Veenhuyzen, D., Echols, R., Machida, M., Ferreira, J. C. A., Ariyasu, M., et al. (2018). Cefiderocol versus imipenem-cilastatin for the treatment of complicated urinary tract infections caused by gram-negative uropathogens: a phase 2, randomised, double-blind, non-inferiority trial. Lancet Infect. Dis. 18, 1319–1328. doi: 10.1016/S1473-3099(18)30554-1
Principe, L., Di Bella, S., Conti, J., Perilli, M., Piccirilli, A., Mussini, C., et al. (2022). Acinetobacter baumannii resistance to sulbactam/durlobactam: a systematic review. Antibiotics 11:1793. doi: 10.3390/antibiotics11121793
Qin, X., Tran, B. G., Kim, M. J., Wang, L., Nguyen, D. A., Chen, Q., et al. (2017). A randomised, double-blind, phase 3 study comparing the efficacy and safety of ceftazidime/avibactam plus metronidazole versus meropenem for complicated intra-abdominal infections in hospitalised adults in Asia. Int. J. Antimicrob. Agents 49, 579–588. doi: 10.1016/j.ijantimicag.2017.01.010
Relebactam (2024). Available at: https://pubchem.ncbi.nlm.nih.gov/compound/44129647 (Accessed January 18, 2024).
Roilides, E., Ashouri, N., Bradley, J. S., Johnson, M. G., Lonchar, J., Su, F.-H., et al. (2023). Safety and efficacy of ceftolozane/tazobactam versus meropenem in neonates and children with complicated urinary tract infection, including pyelonephritis: a phase 2, randomized clinical trial. Pediatr. Infect. Dis. J. 42, 292–298. doi: 10.1097/INF.0000000000003832
Sader, H. S., Castanheira, M., Kimbrough, J. H., Kantro, V., and Mendes, R. E. (2023). Aztreonam/avibactam activity against a large collection of carbapenem-resistant Enterobacterales (Cre) collected in hospitals from Europe, Asia and Latin America (2019–21). JAC Antimicrob. Resist. 5:dlad032. doi: 10.1093/jacamr/dlad032
Sader, H. S., Flamm, R. K., Carvalhaes, C. G., and Castanheira, M. (2020). Comparison of ceftazidime-avibactam and ceftolozane-tazobactam in vitro activities when tested against gram-negative bacteria isolated from patients hospitalized with pneumonia in United States medical centers (2017-2018). Diagn. Microbiol. Infect. Dis. 96:114833. doi: 10.1016/j.diagmicrobio.2019.05.005
Sagan, O., Yakubsevitch, R., Yanev, K., Fomkin, R., Stone, E., Hines, D., et al. (2020). Pharmacokinetics and tolerability of intravenous sulbactam-durlobactam with imipenem-cilastatin in hospitalized adults with complicated urinary tract infections, including acute pyelonephritis. Antimicrob. Agents Chemother. 64:e01506-19. doi: 10.1128/AAC.01506-19
Sato, T., and Yamawaki, K. (2019). Cefiderocol: discovery, chemistry, and in vivo profiles of a novel siderophore cephalosporin. Clin. Infect. Dis. 69, S538–S543. doi: 10.1093/cid/ciz826
Sawa, T., Kooguchi, K., and Moriyama, K. (2020). Molecular diversity of extended-spectrum β-lactamases and carbapenemases, and antimicrobial resistance. J. Intensive Care 8:13. doi: 10.1186/s40560-020-0429-6
Schito, A. M., Schito, G. C., and Alfei, S. (2021). Synthesis and antibacterial activity of cationic amino acid-conjugated dendrimers loaded with a mixture of two triterpenoid acids. Polymers 13:521. doi: 10.3390/polym13040521
Sethuvel, D. P. M., Bakthavatchalam, Y. D., Karthik, M., Irulappan, M., Shrivastava, R., Periasamy, H., et al. (2023). β-Lactam resistance in ESKAPE pathogens mediated through modifications in penicillin-binding proteins: an overview. Infect. Dis. Ther. 12, 829–841. doi: 10.1007/s40121-023-00771-8
Sharma, A., Gupta, V., and Pathania, R. (2019). Efflux pump inhibitors for bacterial pathogens: from bench to bedside. Indian J. Med. Res. 149, 129–145. doi: 10.4103/ijmr.IJMR_2079_17
Sims, M., Mariyanovski, V., McLeroth, P., Akers, W., Lee, Y.-C., Brown, M. L., et al. (2017). Prospective, randomized, double-blind, phase 2 dose-ranging study comparing efficacy and safety of imipenem/cilastatin plus relebactam with imipenem/cilastatin alone in patients with complicated urinary tract infections. J. Antimicrob. Chemother. 72, 2616–2626. doi: 10.1093/jac/dkx139
Sugawara, E., Kojima, S., and Nikaido, H. (2016). Klebsiella pneumoniae Major Porins OmpK35 and OmpK36 allow more efficient diffusion of β-lactams than their Escherichia coli homologs OmpF and OmpC. J. Bacteriol. 198, 3200–3208. doi: 10.1128/JB.00590-16
Sulbactam (2024). Available at: https://pubchem.ncbi.nlm.nih.gov/compound/130313 (Accessed January 18, 2024).
Sun, Y., Fan, J., Chen, G., Chen, X., Du, X., Wang, Y., et al. (2022). A phase III, multicenter, double-blind, randomized clinical trial to evaluate the efficacy and safety of ceftolozane/tazobactam plus metronidazole versus meropenem in Chinese participants with complicated intra-abdominal infections. Int. J. Infect. Dis. 123, 157–165. doi: 10.1016/j.ijid.2022.08.003
Teo, J. Q.-M., Lim, J. C., Tang, C. Y., Lee, S. J.-Y., Tan, S. H., Sim, J. H.-C., et al. (2021). Ceftolozane/tazobactam resistance and mechanisms in carbapenem-nonsusceptible pseudomonas aeruginosa. mSphere 6:e01026-20. doi: 10.1128/mSphere.01026-20
Tirlangi, P. K., Wanve, B. S., Dubbudu, R. R., Yadav, B. S., Kumar, L. S., Gupta, A., et al. (2023). Successful use of Cefepime-Zidebactam (WCK 5222) as a salvage therapy for the treatment of disseminated extensively drug-resistant New Delhi Metallo-β-lactamase-producing Pseudomonas aeruginosa infection in an adult patient with acute T-cell leukemia. Antimicrob. Agents Chemother. 67:e0050023. doi: 10.1128/aac.00500-23
Titov, I., Wunderink, R. G., Roquilly, A., Rodríguez Gonzalez, D., David-Wang, A., Boucher, H. W., et al. (2021). A randomized, double-blind, multicenter trial comparing efficacy and safety of imipenem/cilastatin/relebactam versus piperacillin/tazobactam in adults with hospital-acquired or ventilator-associated bacterial pneumonia (restore-Imi 2 study). Clin. Infect. Dis. 73, e4539–e4548. doi: 10.1093/cid/ciaa803
Torres, A., Zhong, N., Pachl, J., Timsit, J.-F., Kollef, M., Chen, Z., et al. (2018). Ceftazidime-avibactam versus meropenem in nosocomial pneumonia, including ventilator-associated pneumonia (reprove): a randomised, double-blind, phase 3 non-inferiority trial. Lancet Infect. Dis. 18, 285–295. doi: 10.1016/S1473-3099(17)30747-8
Tsai, Y. K., Fung, C. P., Lin, J. C., Chen, J. H., Chang, F. Y., Chen, T. L., et al. (2011). Klebsiella pneumoniae outer membrane porins OmpK35 and OmpK36 play roles in both antimicrobial resistance and virulence. Antimicrob. Agents Chemother. 55, 1485–1493. doi: 10.1128/AAC.01275-10
Uddin, T. M., Chakraborty, A. J., Khusro, A., Zidan, B. R. M., Mitra, S., Emran, T. B., et al. (2021). Antibiotic resistance in microbes: history, mechanisms, therapeutic strategies and future prospects. J. Infect. Public Health 14, 1750–1766. doi: 10.1016/j.jiph.2021.10.020
Upert, G., Luther, A., Obrecht, D., and Ermert, P. (2021). Emerging peptide antibiotics with therapeutic potential. Med. Drug Discov. 9:100078. doi: 10.1016/j.medidd.2020.100078
Van Duin, D., and Bonomo, R. A. (2016). Ceftazidime/avibactam and ceftolozane/tazobactam: second-generation β-lactam/β-lactamase inhibitor combinations. Clin. Infect. Dis. 63, 234–241. doi: 10.1093/cid/ciw243
Vazquez, J. A., González Patzán, L. D., Stricklin, D., Duttaroy, D. D., Kreidly, Z., Lipka, J., et al. (2012). Efficacy and safety of ceftazidime–avibactam versus imipenem–cilastatin in the treatment of complicated urinary tract infections, including acute pyelonephritis, in hospitalized adults: results of a prospective, investigator-blinded, randomized study. Curr. Med. Res. Opin. 28, 1921–1931. doi: 10.1185/03007995.2012.748653
Venuti, F., Gaviraghi, A., De Nicolò, A., Stroffolini, G., Longo, B. M., Di Vincenzo, A., et al. (2023). Real-life experience of continuously infused Ceftolozane/Tazobactam in patients with bronchiectasis and multidrug-resistant Pseudomonas aeruginosa infection in the outpatient setting. Antibiotics 12:1214. doi: 10.3390/antibiotics12071214
Wagenlehner, F. M., Sobel, J. D., Newell, P., Armstrong, J., Huang, X., Stone, G. G., et al. (2016). Ceftazidime-avibactam versus doripenem for the treatment of complicated urinary tract infections, including acute pyelonephritis: recapture, a phase 3 randomized trial program. Clin. Infect. Dis. 63, 754–762. doi: 10.1093/cid/ciw378
Wang, Y., Wang, J., Wang, R., and Cai, Y. (2020). Resistance to ceftazidime–avibactam and underlying mechanisms. J. Glob. Antimicrob. Resist. 22, 18–27. doi: 10.1016/j.jgar.2019.12.009
Wilson, G. M., Fitzpatrick, M., Walding, K., Gonzalez, B., Schweizer, M. L., Suda, K. J., et al. (2021). Meta-analysis of clinical outcomes using ceftazidime/avibactam, Ceftolozane/Tazobactam, and Meropenem/Vaborbactam for the treatment of multidrug-resistant gram-negative infections. Open Forum Infect. Dis. 8:ofaa651. doi: 10.1093/ofid/ofaa651
Wise, M. G., Karlowsky, J. A., Mohamed, N., Kamat, S., and Sahm, D. F. (2023). In vitro activity of aztreonam-avibactam against Enterobacterales isolates collected in Latin America, Africa/Middle East, Asia, and Eurasia for the ATLAS global surveillance program in 2019-2021. Eur. J. Clin. Microbiol. Infect. Dis. 42, 1135–1143. doi: 10.1007/s10096-023-04645-2
World Health Organization (2017). WHO publishes list of bacteria for which new antibiotics are urgently needed. www.who.int. Available at: https://www.who.int/news/item/27-02-2017-who-publishes-list-of-bacteria-for-which-new-antibiotics-are-urgently-needed
World Health Organization (2021). 2021 AWaRe classification. www.who.int. Available at: https://www.who.int/publications/i/item/2021-aware-classification
Wunderink, R. G., Matsunaga, Y., Ariyasu, M., Clevenbergh, P., Echols, R., Kaye, K. S., et al. (2021). Cefiderocol versus high-dose, extended-infusion meropenem for the treatment of gram-negative nosocomial pneumonia (Apeks-np): a randomised, double-blind, phase 3, non-inferiority trial. Lancet Infect. Dis. 21, 213–225. doi: 10.1016/S1473-3099(20)30731-3
Yang, Y., Guo, Y., Yin, D., Zheng, Y., Wu, S., Zhu, D., et al. (2020). In vitro activity of Cefepime-Zidebactam, ceftazidime-avibactam, and other comparators against clinical isolates of Enterobacterales, Pseudomonas aeruginosa, and Acinetobacter baumannii: results from China antimicrobial surveillance network (CHINET) in 2018. Antimicrob. Agents Chemother. 65:e01726-20. doi: 10.1128/AAC.01726-20
Yusuf, E., Bax, H. I., Verkaik, N. J., and Van Westreenen, M. (2021). An update on eight “new” antibiotics against multidrug-resistant gram-negative bacteria. JCM 10:1068. doi: 10.3390/jcm10051068
Zagaliotis, P., Michalik-Provasek, J., Gill, J. J., and Walsh, T. J. (2022). Therapeutic bacteriophages for gram-Nega- tive bacterial infections in animals and humans. Pathog. Immun. 7, 1–45. doi: 10.20411/pai.v7i2.516
Zampaloni, C., Mattei, P., Bleicher, K., Winther, L., Thäte, C., Bucher, C., et al. (2024). A novel antibiotic class targeting the lipopolysaccharide transporter. Nature 625, 566–571. doi: 10.1038/s41586-023-06873-0
Keywords: salvage therapy, antibiotic resistance, ceftazidime/avibactam, imipenem/relebactam, ceftolozane/tazobactam, cefiderocol, aztreonam/avibactam, sulbactam/durlobactam
Citation: Dan MO and Tǎlǎpan D (2024) Friends or foes? Novel antimicrobials tackling MDR/XDR Gram-negative bacteria: a systematic review. Front. Microbiol. 15:1385475. doi: 10.3389/fmicb.2024.1385475
Received: 12 February 2024; Accepted: 17 April 2024;
Published: 10 May 2024.
Edited by:
Maria Teresa Mascellino, Sapienza University of Rome, ItalyReviewed by:
Eugene A. Rogozhin, Institute of Bioorganic Chemistry (RAS), RussiaCopyright © 2024 Dan and Tǎlǎpan. This is an open-access article distributed under the terms of the Creative Commons Attribution License (CC BY). The use, distribution or reproduction in other forums is permitted, provided the original author(s) and the copyright owner(s) are credited and that the original publication in this journal is cited, in accordance with accepted academic practice. No use, distribution or reproduction is permitted which does not comply with these terms.
*Correspondence: Daniela Tǎlǎpan, ZGFuaWVsYS50YWxhcGFuQHVtZmNkLnJv
Disclaimer: All claims expressed in this article are solely those of the authors and do not necessarily represent those of their affiliated organizations, or those of the publisher, the editors and the reviewers. Any product that may be evaluated in this article or claim that may be made by its manufacturer is not guaranteed or endorsed by the publisher.
Research integrity at Frontiers
Learn more about the work of our research integrity team to safeguard the quality of each article we publish.