- 1Department of Nutrition, Faculty of Medicine, Mashhad University of Medical Sciences, Mashhad, Iran
- 2School of Chemical Engineering, University of Birmingham, Edgbaston, United Kingdom
- 3Medical Toxicology Research Center, Mashhad University of Medical Sciences, Mashhad, Iran
Introduction: Kefir beverage has beneficial microorganisms that have health-giving properties; therefore, they have a good potential to be probiotic. This study evaluated the probiotic potential, technological, and safety characteristics of Enterococcus faecalis, Lactococcus lactis, and Pichia fermentans isolated from traditional kefir beverages.
Method: First, isolates were evaluated in terms of resistance to acid, alkali, bile salts, trypsin, and pepsin of the gastrointestinal tract. The auto-aggregation and co-aggregation ability of isolates were measured using spectrophotometry. Antimicrobial activities were assayed against important food-borne pathogens using the agar well diffusion method. Moreover, gamma-aminobutyric acid (GABA) production was investigated by thin-layer chromatography (TLC).
Result: Among the isolates, P. fermentans had an 85% total survival rate, but its amount reached below 6 log CFU/ml which is considered non-resistant, and it showed the highest auto-aggregation (74.67%). Moreover, only L. lactis showed antimicrobial activity and had the highest co-aggregation with E. coli PTCC 1338 (54.33%) and L. monocytogenes ATCC 7644 (78%). Finally, an evaluation of the technological and safety characteristics of the strains showed that the strains produced GABA and were safe.
Discussion: Although the isolates were not resistant to the gastrointestinal tract, their supernatant contained valuable natural compounds, including antioxidants, GABA, and antimicrobials, which can be used to produce functional foods and medicines. In addition, other approaches, such as increasing the initial number of strains, using foods as carriers of isolates, and encapsulating the isolates, can effectively increase the survivability of isolates in the gastrointestinal tract.
1 Introduction
Although in the past healthy humans were just considered safe sources of probiotics, since FAO and WHO announced that the function of probiotics is more important than their source, scientists’ attention has been drawn to functional foods as new sources of probiotics (Guo et al., 2011; Guetouache and Guessas, 2015; LeBlanc et al., 2020; Bs et al., 2021; Bangotra et al., 2023). Among the functional foods, dairy-fermented products are consumed more, and the demand for them is higher (Nielsen et al., 2014). Kefir which is one of these products is a low-alcohol, viscous, and easily digestible carbonated beverage obtained by fermenting milk. Microorganisms that inhabit kefir grains, an insoluble protein and polysaccharide matrix, carry out the fermentation (Nielsen et al., 2014; Guetouache and Guessas, 2015; Mitra and Ghosh, 2020; Azizi et al., 2021; Touranlou et al., 2023).
A large number of these microorganisms have various merits, such as improving the immune system, preventing the growth of pathogenic microorganisms, antioxidant activity, hypocholesterolemic effect, controlling plasma glucose, antihypertensive, improving digestion, reducing the effects of obesity, reducing heart hypertrophy, and kidney hypertrophy (by producing vitamins, short-chain fatty acids, and bioactive substances like antioxidants, and gamma-aminobutyric acid) and prevent disease (by producing antimicrobial compounds) (Leite et al., 2015; Rosa et al., 2017; Mantzourani et al., 2019; Ganatsios et al., 2021; Moghimani et al., 2023). Therefore, kefir microorganisms are suitable candidates for being probiotic (Guetouache and Guessas, 2015; Gul et al., 2018; LeBlanc et al., 2020; Bs et al., 2021; Bangotra et al., 2023). In addition, Previous studies showed that the characteristics of microorganisms can be strain-dependent, so a strain-by-strain assessment of probiotic potential, health benefits, and safety of microorganisms is necessary (Leite et al., 2015; Bangotra et al., 2023; Erfani et al., 2023; Sionek et al., 2023; Zamanpour et al., 2023).
Probiotics have health-giving effects on the host when they reach the small intestine as live and active cells, for this reason, they must be resistant to the acidic and alkaline pH of the stomach, bile salts, pepsin, and pancreatin enzymes. Moreover, there are other factors besides resistance to stomach pH and bile salts to evaluate the probiotic potential, including the auto-aggregation ability for colonization in the intestine, co-aggregation ability with pathogens, and antimicrobial activity to inhibit the pathogens (Barzegar et al., 2021; Doğan and Ay, 2021; Goktas et al., 2021; Almeida et al., 2022; He et al., 2022).
Since probiotics are classified as Generally Recognized as Safe (GRAS) and Qualified Presumption of Safety (QPS) compounds, they must be checked for safety, especially Enterococcus, which is known as an opportunistic pathogen (Zendeboodi et al., 2020; Ozma et al., 2021).
Probiotics’ technological properties can be assessed to aid in their industrial application. Technological characteristics include the production of bioactive and beneficial compounds that increase cell survival rates (Zendeboodi et al., 2020; Barzegar et al., 2021). Gamma-aminobutyric acid (GABA) is one of these compounds. In the central nervous system, GABA, a four-carbon non-protein amino acid, functions as an inhibitory neurotransmitter. GABA has positive effects, such as treating insomnia, suppressing depression, improving long-term memory, and regulating blood pressure in the brain. Between the synthetic and biological methods of GABA production, biological production has received more attention due to its higher efficiency, lower cost, and environmental risks. A large group of microorganisms, including lactic acid bacteria and yeasts, can biologically produce GABA (Ribeiro et al., 2018; Ly et al., 2019; Perpetuini et al., 2020; Bs et al., 2021; Falah et al., 2021; Khanlari et al., 2021; Ghafurian Nasab et al., 2022).
Among the articles that assessed the probiotic potential of kefir’s microorganisms in various geographical regions, just Rahmani et al. (2022) assessed the probiotic potential of Iranian kefir beverage’s yeasts. This study isolated different species of yeast including Saccharomyces cerevisiae, Kluyveromyces marxianus, Pichia fermentans, and Pichia kudriavzevii that showed one strain of P. fermentans and three strains of S. cerevisiae are proper candidates as probiotic yeast (Rahmani et al., 2022). Other studies were related to Argentina, Korea, Brazil, Turkey, Malaysia, Singapore, and Mexico (Carasi et al., 2014; Leite et al., 2015; Zanirati et al., 2015; Cassanego et al., 2017; Cho et al., 2018; Azhar and Munaim, 2019; Talib et al., 2019; Yerlikaya, 2019; Akpinar and Yerlikaya, 2021; Doğan and Ay, 2021; Hurtado-Romero et al., 2021; Tan et al., 2022; Youn et al., 2022).
Hurtado-Romero et al. (2021) and Tan et al. (2022) were the only studies that examined the ability of kefir’s microorganisms to produce GABA. Hurtado-Romero et al. (2021) reported that Lactococcus. lactis (BIOTEC006, BIOTEC007, BIOTEC008), Kluyveromyces. lactis (BIOTEC009), Leuconostoc. pseudomesenteroides (BIOTEC012), and Lentilactobacillus. kefiri (BIOTEC014) isolated from Mexican kefir beverage were able to produce GABA (Hurtado-Romero et al., 2021). Tan et al. (2022) reported that just Lentilactobacillus hilgardii (Kef-w8, Kef-w9, Kef-w10) isolated from Singapore kefir had GABA synthetic genes (Tan et al., 2022).
In general, studies revealed that the microorganisms isolated from kefir beverages in different geographical regions are various and have a great potential to be probiotic (Carasi et al., 2014; Zanirati et al., 2015; Cassanego et al., 2017; Englerová et al., 2017; Cho et al., 2018; Bengoa et al., 2019; Talib et al., 2019; Akpinar and Yerlikaya, 2021). Therefore, the present study aims to assess the probiotic potential, biochemical and technological properties, and the safety of two bacterial species Lactococcus lactis, and Enterococcus faecalis, and a yeast species Pichia fermentans isolated from traditional Iranian kefir beverage.
2 Method
2.1 Study design
A schematic flow chart of the experimental procedures used to investigate the characteristics of microorganisms and evaluate the probiotic potential, technical, and safety characteristics is shown in Figure 1.
2.2 Isolation and identification
Enterococcus faecalis (Accession number PP790751), Lactococcus lactis, (Accession number PP826201) and Pichia fermentans (Accession number PP803455) were isolated and identified from Iranian milk kefir beverages in our previous study using polymerase chain reaction (PCR) (Moghimani et al., 2023).
2.3 Examination of phenotypic, biochemical, and physiological characteristics
2.3.1 Phenotypic characteristics
The morphology of colonies was examined based on colony shape, color, edge, size, bacterial cell shape, and Arrangement.
2.3.2 Biochemical and physiological characteristics
The study examined the fermentation patterns of various sugars, specifically mannitol, glucose, lactose, sucrose, and xylose, in different bacterial strains. Additionally, the bacteria were analyzed using Gram staining. For yeast, lactophenol cotton blue staining was employed. The study also included a catalase test for enzyme activity and assessed the bacteria’s ability to grow at a temperature of 45°C.
2.4 Probiotic potential
The probiotic potential of the isolates was evaluated by examining four common tests, including resistance to the gastrointestinal tract, auto-aggregation ability, co-aggregation ability with pathogens, and antimicrobial activity.
2.4.1 Resistance to the gastrointestinal tract
2.4.1.1 Resistance to different pH, bile salts, simulated gastric, and intestinal juice
2.4.1.1.1 Preparation of isolate samples
Overnight cultures were spun at 6,000 rpm for 15 min. The supernatant was discarded, and the remaining cell pellets were washed twice with phosphate-buffered saline (PBS) at a pH of 7.2. The concentration of these cell pellets was adjusted to 1.5 × 108 CFU/mL.
2.4.1.1.2 pH resistance test
One milliliter of the prepared isolates was mixed with 9 mL of PBS adjusted to different pH levels: 2.5 (simulating gastric conditions), 8 (simulating intestinal conditions), and 7 (control). These mixtures were incubated at 37°C for 3 h. The survival of cells at 0 and 3 h was assessed by cultivation on de man–rogosa–sharpe agar (MRS) and potato dextrose agar (PDA) (Ibresco) plates (Baccouri et al., 2019).
2.4.1.1.3 Bile salt resistance test
For testing resistance to bile salts, 1 mL of isolates at a concentration of 1.5 × 108 CFU/mL was combined with 9 mL of MRS (Condalab) and yeast extract peptone dextrose (YPD) (Quelab) broth containing 0.3% bile salts (Sigma-Aldrich). These were incubated at 37°C for 4 h, with cell survival analyzed at 0 and 4 h using MRS and PDA agar plates. Broths without bile salts served as controls (Baccouri et al., 2019).
2.4.1.1.4 Simulated digestive juice test
To mimic gastric juice, a solution containing 3 g/L of pepsin (Sigma-Aldrich) at pH 2.5 was prepared. For intestinal juice, a solution containing 0.15% bile salts and 0.1% pancreatin (Sigma-Aldrich) at pH 8 was used. Each isolate was first exposed to gastric juice for 3 h, centrifuged, washed with PBS, and then exposed to intestinal juice for another 3 h at 37°C. Cell survival was evaluated at 0 and 3 h post-exposure to each juice type (Barzegar et al., 2021; Afshari et al., 2022).
Results were put in the following equation to obtain the percentage of survival rates.
N1 = The number counted in the final time.
N0 = The number counted at time 0.
2.4.2 Auto-aggregation and co-aggregation ability
The overnight culture of isolates was centrifuged at 6,000 rpm for 15 min. Their supernatant was discarded and the pellets were washed twice with PBS at a pH of 7.2. Isolates with the concentration of 1.5 × 108 CFU/mL were vortexed for 10 s and incubated at 37°C for 24 h. To obtain the auto-aggregation percentage, the absorbance of isolates was measured by a spectrophotometer (Jenway, England) at 600 nm in 0, 2, 4, 6, 8, and 24 h (Lactobacillus casei PTTC 1608 was used as standard probiotic strain). Finally, the percentage of auto-aggregation was determined according to the following equation (Barzegar et al., 2021).
A0 = Absorption at 0 h.
A1 = Absorption at 2, 4, 6, 8, and 24 h.
To evaluate the co-aggregation ability, an equal amount of isolates and pathogenic bacteria, including Escherichia coli (PTCC 1338) and Listeria monocytogenes (ATCC 7644) with the concentration of 1.5 × 108 CFU/mL were prepared, mixed, and vortexed for 10 s. The absorbance of the mixture suspensions was measured at 600 nm at 0, 2, 4, 6, 8, and 24 h by a spectrophotometer (Lactobacillus casei PTTC 1608 was used as the standard probiotic strain). The percentage of Co-aggregation was calculated according to the following equation:
AX: Absorbance of each isolate at 0 h.
AY: Absorbance of pathogen 0 h.
A(X + Y): Absorbance of the mixture suspension at 2, 4, 6, 8, and 24 h.
2.4.3 Antimicrobial activity
The study assessed the antimicrobial properties of certain isolates using the agar well diffusion method on agar plates. This test was conducted against four types of bacteria: Listeria monocytogenes (ATCC 7644), Bacillus cereus (ATCC 14579), Salmonella Typhimurium (ATCC 14028), and Escherichia coli (PTCC 1338). Initially, 1.5 × 108 CFU/mL of each bacterial strain was spread on Muller Hinton agar (Condalab) plates. Subsequently, wells of 6 mm diameter were created in the agar. The cell-free supernatant (CFS) of the isolates was prepared by centrifuging their overnight cultures at 6,000 rpm for 15 min, followed by filtration through a 0.22 μm filter. 100 μL of this supernatant was then added to each well. The plates were incubated at 37°C for 24 h, with sterile distilled water serving as blank (Almeida et al., 2022).
2.5 Technological properties
2.5.1 Antioxidant activity
The antioxidant activity of the isolates was measured using a DPPH (1-diphenyl-2-picrylhydrazyl) assay. For this test, an equal volume of each isolate’s CFS was mixed with 1.5 mL of ethanolic DPPH (Sigma-Aldrich) solution (0.4 mmol). This mixture was incubated at 37°C in the dark for 1 h. The absorbance of the solution was then measured at 517 nm. The control for this test was a mixture of 1.5 mL DPPH and 1.5 mL methanol. Antioxidant activity, expressed as scavenging activity percentage, was calculated using the following formula (Archer and Halami, 2015; Sakkaa et al., 2022).
2.5.2 Screening for GABA production
The ability of the isolates to produce GABA (Gamma-Aminobutyric Acid) from monosodium glutamate (MSG) was determined using thin-layer chromatography (TLC). The isolates were grown in MRS and YDP broth with 1% MSG at 37°C for 48 h. Then, 2 μL of their CFS was applied on a silica gel TLC plate (60 F256, Sigma-Aldrich). The applied spots were positioned 2 cm from the bottom and 1 cm apart from each other and the plate edges. GABA and MSG were also applied as controls. The plate was exposed to a mobile phase consisting of butanol, acetic acid, and distilled water (5:2:2, v/v/v), and removed once the solvent front reached two-thirds of the plate height. The plate was then sprayed with a ninhydrin solution and heated at 105°C for 5 min. The retention factor (Rf) for each spot was calculated, and isolates showing the same Rf as the GABA standard were identified as GABA producers (Falah et al., 2021; Ghafurian Nasab et al., 2022; Sakkaa et al., 2022).
2.6 Safety evaluation
2.6.1 Antibiotic resistance
The antibiotic resistance of the isolates was assessed using the disk diffusion method. For this purpose, overnight cultures of the isolates, at a concentration of 1.5 × 108 CFU/mL, were spread on MRS and PDA agar plates. Antibiotic disks, including penicillin, ampicillin (10 mg per disk), erythromycin (15 mg per disk), vancomycin, chloramphenicol, and tetracycline (30 mg per disk), along with a filter paper disk as a control, were placed on the agar, ensuring they were spaced apart. The plates were then incubated at 37°C for 24 h. The diameters of the inhibition zones around each disk (ZDI values) were measured and interpreted according to the Clinical and Laboratory Standards Institute (CLSI) 2009 guidelines. The results were categorized as follows: resistant (ZDI: ≤ 15 mm), sensitive (ZDI: ≥ 21 mm), or intermediately susceptible (ZDI: 16–20 mm) (Katiku et al., 2022).
2.6.2 Hemolytic activity
To evaluate the hemolytic activity of the isolates, their overnight culture was cultured on blood agar plates (supplemented with 7% human blood), and the plates were incubated at 37°C for 48 h (Lakhlifi et al., 2023).
2.7 Statistical analysis
All data are shown as mean ± standard deviation of three independent replicates. Statistical data analysis was performed using Microsoft Excel 2016 and SPSS 16 with independent t-test, pair t-test, and one-way ANOVA followed by Tukey’s test.
3 Results and discussion
3.1 Phenotypic, biochemical, and physiological characteristics
The results are reported in Table 1. Microscopic images of microorganisms are also shown in Figure 2.
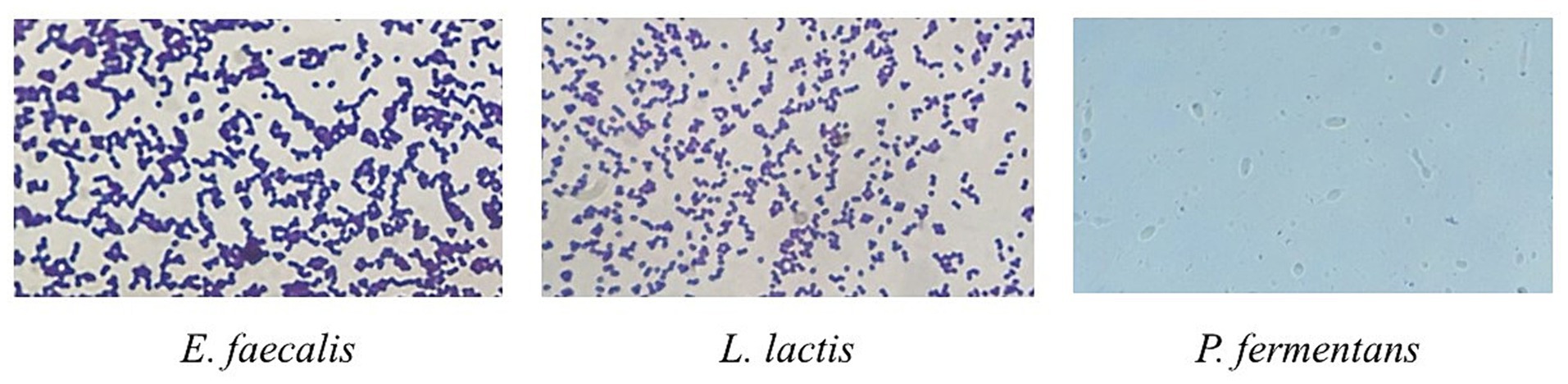
Figure 2. Microscopic image of Gram staining of E. faecalis and L. lactis strains and lactophenol cotton blue staining of P. fermentans. Bacteria were imaged at an original magnification of 1,000× and the yeast was imaged at the original magnification of 400×.
With regard to phenotypic, biochemical, and physiological characteristics of the isolates, results of the present study were consistent with previous studies, but there were some differences in sugar fermentation patterns which is because of the difference between studied strains (Leite et al, 2015; Hejazi et al., 2019; Hurtado-Romero et al, 2021). For example, Hejazi et al. (2019), reported that E. faecalis isolated from cheese was unable to ferment saccharose, while E. faecalis in the present study fermented it (Hejazi et al., 2019).
3.2 Probiotic potential
3.2.1 Resistance to the gastrointestinal tract
3.2.1.1 Resistance to different pH, bile salts, and simulated gastric and intestinal juice
Since an important principle about the effectiveness of probiotics is that they have to reach the target organ—the large intestine—alive and reproducible, they must be able to cope with the high acidity and alkaline pH, bile salts as well as gastric and intestinal juice in the digestive tract (Barzegar et al., 2021).
The resistance of isolates to different pH (2.5, 8, and 7), bile salts, and gastric and intestinal juice are shown in Tables 2–5, respectively. According to the results, E. faecalis and L. lactis could not tolerate the harsh conditions of the digestive tract, while P. fermentans tolerated these conditions with a total survival rate of 85%.
Multiple studies indicate that the ability of different bacterial isolates to withstand conditions in the gastrointestinal tract is influenced by several factors, including the acidity (pH), bile salt concentration, digestive enzymes (pepsin and pancreatin), incubation duration, and the specific strain of the bacteria (Leite et al., 2015; Baccouri et al., 2019; Merchán et al., 2020; Rahmani et al., 2022; Tan et al., 2022; Kanak et al., 2023). Our study specifically examined how isolates react to a pH level of 2.5 over a 3-h period, as these conditions closely resemble the average acidity and food retention time in the human stomach. However, other research, such as Leite et al. (2015), has found that certain strains like L. lactis from Brazilian kefir can endure a pH of 3 for up to 3 h and a bile salt concentration of 0.3% for an hour (Leite et al., 2015). Additionally, the final concentration of P. fermentans fell below 106 CFU/mL, which is insufficient for probiotics to effectively benefit the host Therefore, it’s important to consider both the survival rate and final concentration of the bacteria.
Despite the fact that the isolates in our study could not withstand the aforementioned digestive conditions, it should be noted that their survival could be enhanced by using a food matrix like kefir, which is easily digested and does not remain in the stomach for long. Other potential solutions include encapsulation and increasing the initial quantity of the probiotics (Barzegar et al., 2021).
3.2.2 Auto-aggregation and co-aggregation ability
Auto-aggregation refers to the potential of cells to assemble themselves, involving complex interactions with cell surface components or secreted factors. On the other hand, co-aggregation is when cells adhere to pathogens, aided by protein compounds on their surfaces. Both mechanisms serve as antimicrobial strategies: auto-aggregation prevents pathogen attachment, while co-aggregation exposes pathogens more effectively to probiotic antimicrobial agents like bacteriocins (Hurtado-Romero et al., 2021; Rahmani et al., 2022; Kanak et al., 2023).
As illustrated in Figure 3, all isolates exhibited a significant increase in auto-aggregation over time (p-value < 0.05), reaching 64–73% after 24 h. P. fermentans demonstrated the highest level of auto-aggregation, surpassing even the standard probiotic strain L. casei PTTC 1608, while L. lactis showed the lowest. However, the differences in auto-aggregation between E. faecalis, L. lactis, and the standard probiotic strain were not statistically significant (p-value > 0.05).
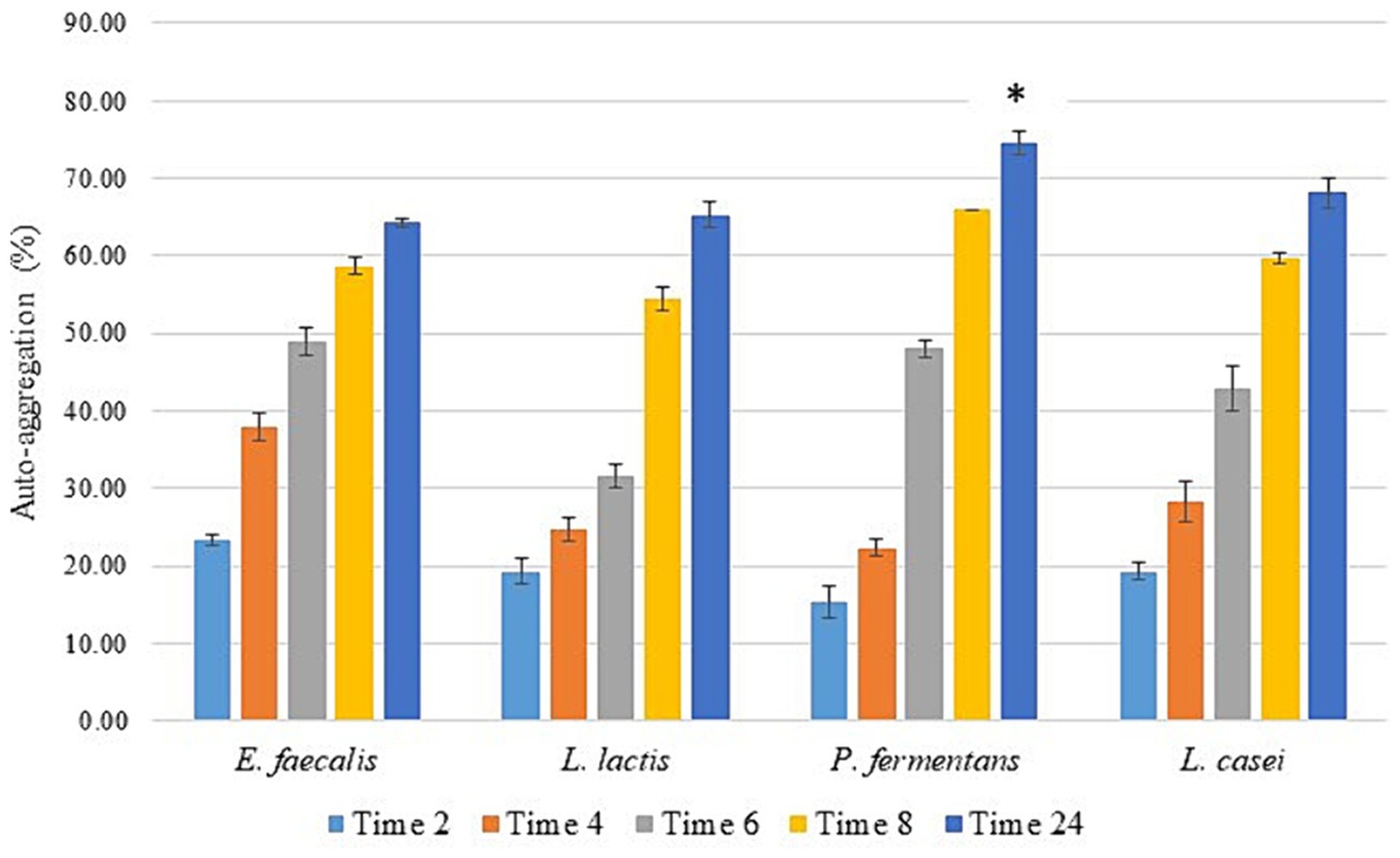
Figure 3. The percentage of auto-aggregation of the isolates as the average of three replicates with standard deviation at 2, 4, 6, 12, and 24 h of incubation at 37°C. L. casei was considered the standard probiotic strain. *indicates that P. fermentans had significantly the highest auto-aggregation activity among the strains and the standard strain after 24 h (p-value < 0.05).
Various articles have demonstrated that the auto-aggregation of different probiotic strains is approximately 30–96% with an average of 62.6%, which increases over time. Therefore, the isolates of this study had a high percentage of auto-aggregation (Ogunremi et al., 2015; Baccouri et al., 2019; Kondrotiene et al., 2020; Merchán et al., 2020; Pytka et al., 2022; Rahmani et al., 2022; Youn et al., 2022; Kanak et al., 2023).
Figure 4 shows the co-aggregation percentage of the isolates with two food-borne pathogens including E. coli and L. monocytogenes. According to it, the co-aggregation percentage of all isolates and standard probiotic strain (L. casei PTTC 1608) with L. monocytogenes was significantly (p-value < 0.05) higher than E. coli. The co-aggregation of standard probiotic strain was significantly (p-value < 0.05) higher with both pathogens compared to all the isolates. The percentage of co-aggregation with both pathogens for the isolates increased significantly over time (p-value < 0.05).
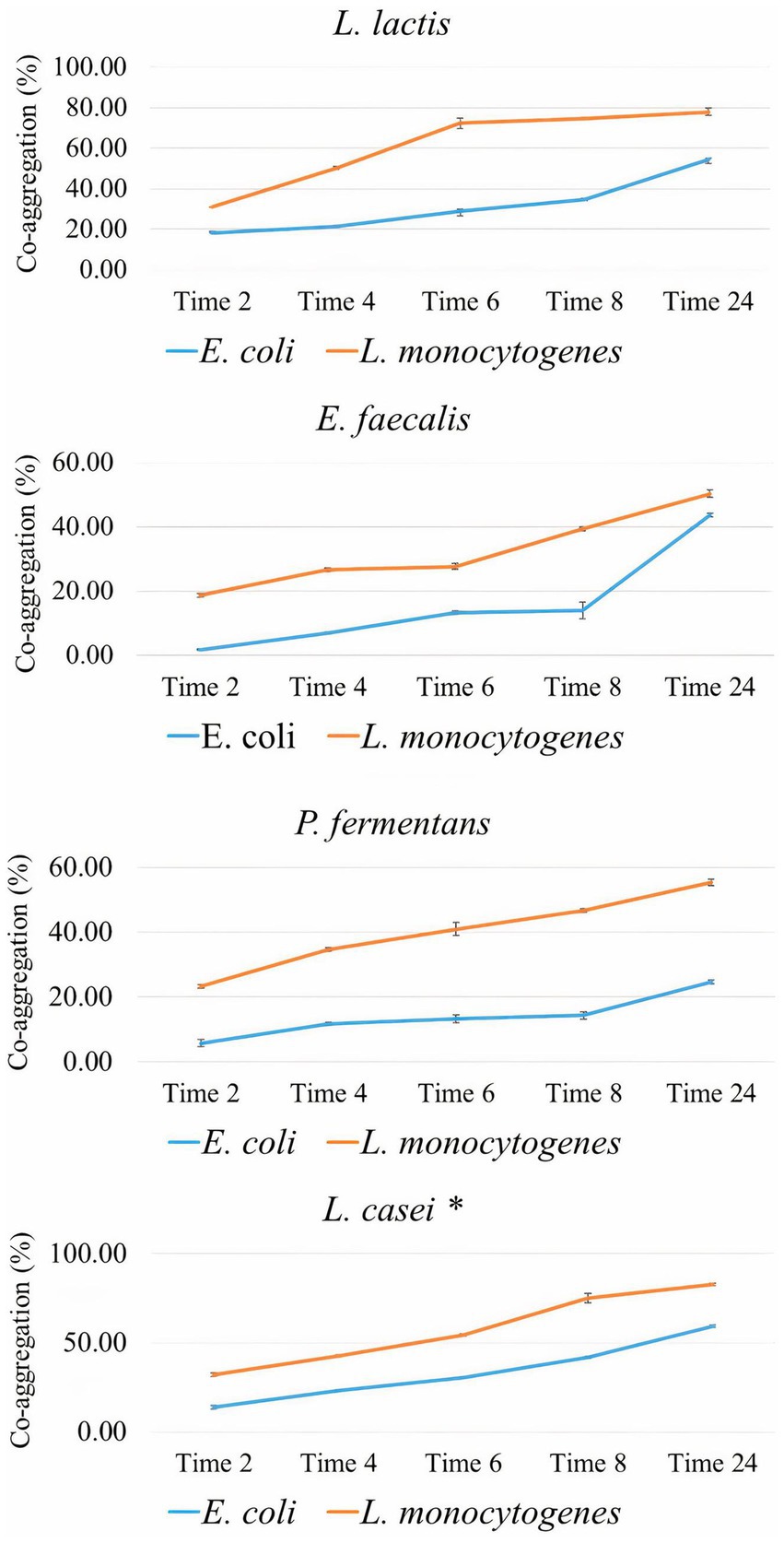
Figure 4. The percentage of co-aggregation of the isolates with E. coli and L. monocytogenes as the average of three replicates with standard deviation at 2, 4, 6, 12, and 24 h of incubation at 37°C. L. casei was considered the standard probiotic strain. *indicates that L. casei had significantly the highest co-aggregation activity with both food-borne pathogenes among the strains and the standard strain after 24 h (p-value < 0.05).
However, the present study demonstrated that the percentage of co-aggregation of all isolates with Gram-positive pathogen (L. monocytogenes) was significantly higher than with Gram-negative pathogen (E. coli). Results of previous studies did not show a relationship between the percentage of co-aggregation and Gram stain of the pathogen (Nami et al., 2019; Pytka et al., 2022; Kanak et al., 2023; Yang et al., 2023). Research evidence has shown that the co-aggregation percentage is dependent only on incubation time, probiotics, and pathogen strain (Kanak et al., 2023).
3.2.3 Antimicrobial activity
The antimicrobial property of probiotics is due to their ability to produce compounds such as organic acids (especially lactic and acetic acids), polyamines, proteases, and bacteriocins (Rahmani et al., 2022).
Table 6 and Figure 5 show the antimicrobial activity of the isolates. According to them, E. faecalis and P. fermentans had no inhibitory effect while L. lactis had an antimicrobial effect on all pathogens studied. Among the pathogens, L. lactis had the most inhibitory effect on L. monocytogenes (p-value < 0.05) and its inhibitory effect on the other pathogens was not significantly different (p-value > 0.05).

Table 6. The diameter of the inhibition zone of the isolates against the pathogens of L. monocytogenes, B. cereus, S. typhimurium, and E. coli.
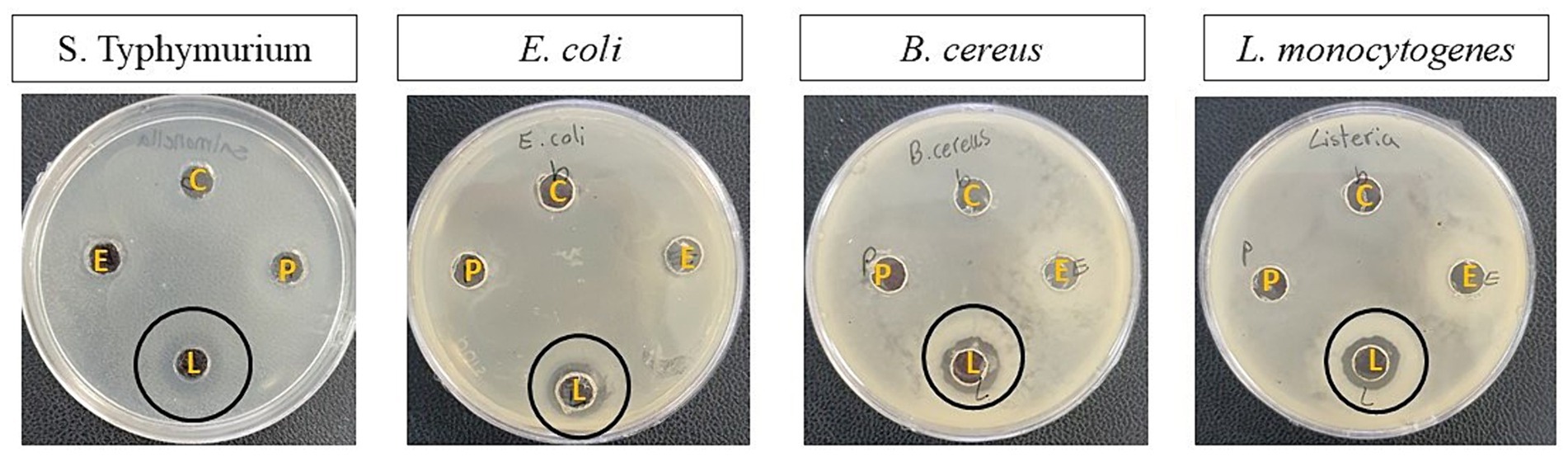
Figure 5. The antimicrobial activity of the isolates against S. typhimurium, E. coli, B. cereus, and L. monocytogenes. The letters B, E, L, and P represent blank (Sterile distilled water), E. faecalis, L. lactis, and P. fermentans, respectively.
Studies show that the antimicrobial property of probiotics is very different even in the same species and it depends on probiotics’ by-products and pathogen strains (Merchán et al., 2020; Hurtado-Romero et al., 2021; Rahmani et al., 2022; Tan et al., 2022). For instance, the results of the present study were not consistent with Hurtado-Romero et al. (2021)’s findings because L. lactis strains isolated from Brazilian kefir had no antimicrobial activity against the E. coli, S. typhi, and S. aureus (Hurtado-Romero et al., 2021).
The findings of the present study were similar to Rahmani et al. (2022) who reported P. fermentans strains isolated from Iranian kefir did not have any antimicrobial effect on S. enterica, E. coli, E. faecalis, S. aureus, and Pseudomonas aeruginosa. Merchán et al. (2020) also showed that P. fermentans strains isolated from cheese had no or very weak antimicrobial effect on the studied pathogens (Merchán et al., 2020; Rahmani et al., 2022).
Although scientific evidence has shown that the inhibitory effect of yeast is less than lactic acid bacteria, those yeast strains that cannot produce antimicrobial metabolites can prevent pathogen growth through other abilities such as auto-aggregation and co-aggregation (Rahmani et al., 2022).
3.3 Technological properties
3.3.1 Antioxidant activity
Probiotics have the ability to release bioactive substances with antioxidant qualities that shield the body from oxidative stress, a condition that is directly linked to a number of illnesses, including aging, Parkinson’s disease, diabetes, and cancer (Lakhlifi et al., 2023). The antioxidant activity of probiotics is strain-dependent and there are various methods to evaluate it. Using the DPPH free radical is one of the typical ways to assess the antioxidant activity of microorganisms. This method is based on DPPH reduction in methanol by taking hydrogen from an antioxidant to form DPPH-H (Ogunremi et al., 2015; Baccouri et al., 2019).
As shown in Figure 6, the CFS of the isolates showed a great ability to scavenge DPPH. Among the isolates, the scavenging activity of E. faecalis and P. fermentans was significantly higher than L. lactis (p-value < 0.05).
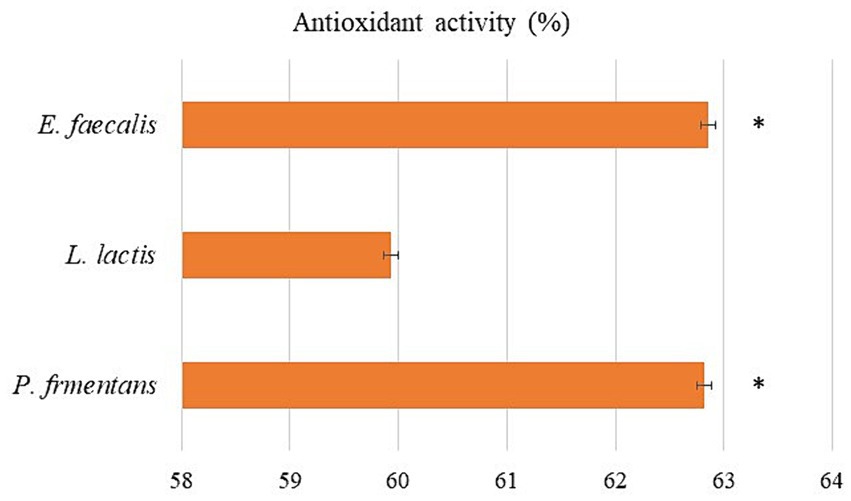
Figure 6. The antioxidant activity of the isolates as the average of three replicates with standard deviation. *indicates significance (p-value < 0.05).
Probiotic bacteria produce metabolites including glutathione, vitamins, and phenolic compounds such as carotenoids, which can prevent the production of free radicals or even destroy them, while the antioxidant activity of probiotic yeasts is mostly because of the presence of large amounts of beta-glucan in their cell walls (Amaretti et al., 2013; Ogunremi et al., 2015; Kotowicz et al., 2019; Hsu and Chou, 2021).
3.3.2 Screening for GABA production
In this study, the GABA-producing potential of the isolates from MSG was investigated by TLC. The results in Figure 7 showed that the RF of all isolates was equal to the GABA standard RF (RF = 0.75) and the diameter of the spot for E. faecalis, L. lactis, and P. fermentans was 6, 7, and 9 mm, respectively, which qualitatively shows that P. fermentans had produced more GABA.
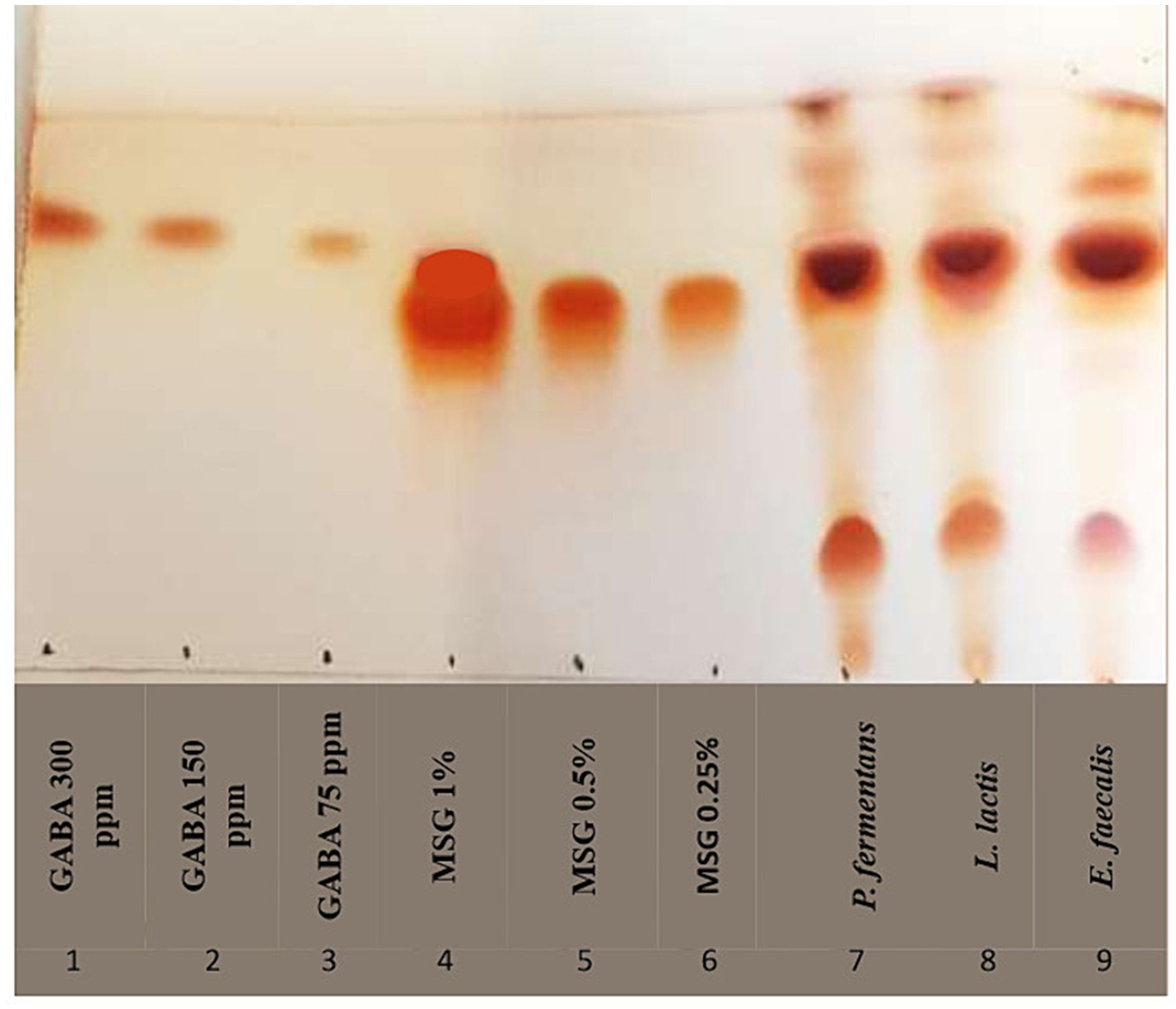
Figure 7. TLC chromatogram of GABA production of representative strains. As controls, lanes one to three and four to six contain varying quantities of gamma-aminobutyric acid (GABA) and monosodium glutamate (MSG), respectively; lanes seven through nine represent the isolates.
To the best of our knowledge, our study is the first study that has reported GABA production by E. faecalis. Franciosi et al. (2015) reported E. faecalis isolated from cheese was unable to produce GABA and the rest of the studies investigated other strains of Enterococcus, which demonstrated that E. faecium and E. avium were able to produce GABA (Tamura et al., 2010; Franciosi et al., 2015; Bs et al., 2021; Sakkaa et al., 2022). Moreover, P. fermentans was investigated for the first time in terms of GABA production in this study, while, previous studies showed that other Pichia species, including P. Kudriavzevii, P. silvicola, P. Guilliermondii, and P. scolyti had been able to produce GABA (Guo et al., 2011; Han and Lee, 2017; Li et al., 2022).
Psychobiotics are living bacteria that have directly and indirectly positive effects on the function of neurons by colonizing in the large intestine. Therefore, the production of GABA as a neurotransmitter is considered a psychobiotic property. Since GABA is regarded as a bioactive substance that supports health and is helpful for the development of foods for specified health uses (FOSHU), the food industry is primarily interested in its production especially by GABA-producing microorganisms because produce natural GABA (Martirosyan and Singh, 2015; Diez-Gutiérrez et al., 2020). For example, a germination technique was used by Cáceres et al. (2017) and Cho and Lim (2016) to increase the amount of GABA in brown rice, while El-Fattah et al. (2018) created functional yogurt that is high in bioactive compounds, including GABA (Cho and Lim, 2016; Cáceres et al., 2017; El-Fattah et al., 2018).
3.4 Safety evaluation
Since humans and animals consume probiotics, they should be safe and were assessed in this term. There are many doubts about the use of Enterococci bacteria as probiotics (Barzegar et al., 2021). Although Enterococci bacteria are not yet GRAS, in contrast to other LAB genera, and they are the main cause of nosocomial infections, previous studies have shown that some Enterococci bacteria such as E. faecalis, E. faecium, and E. durans have been approved as probiotics. For these reasons, before introducing a novel, potentially probiotic Enterococcus strain into functional food, its safety should be determined. A certain Enterococcus strain must be non-pathogenic, genetically stable, devoid of virulence and antibiotic resistance genes, particularly for vancomycine, in order to be considered safe. On the other hand, various Enterococcus species are part of the normal flora in the colon and their main pathogenicity is outside the digestive tract, therefore its oral consumption does not normally cause any problems (Iqbal et al., 2017; Baccouri et al., 2019; Nascimento et al., 2019; Nami et al., 2019; Kim et al., 2022; Sakkaa et al., 2022; Kanak et al., 2023).
3.4.1 Antibiotic resistance
Although antibiotics are effective treatments for bacterial diseases, the indiscriminate use of broad-spectrum antibiotics has caused antibiotic resistance in some pathogens. The transmission of antibiotic-resistant genes by these pathogens in the food chain is very dangerous for human health. Therefore, probiotics should not be resistant to antibiotics (Azhar and Munaim, 2019; Hurtado-Romero et al., 2021; Kim et al., 2022).
The antibiotic resistance results summarized in Figure 8 show that E. faecalis was resistant only to ampicillin and L. lactis to ampicillin and vancomycin, while the yeast strain was resistant to most antibiotics.
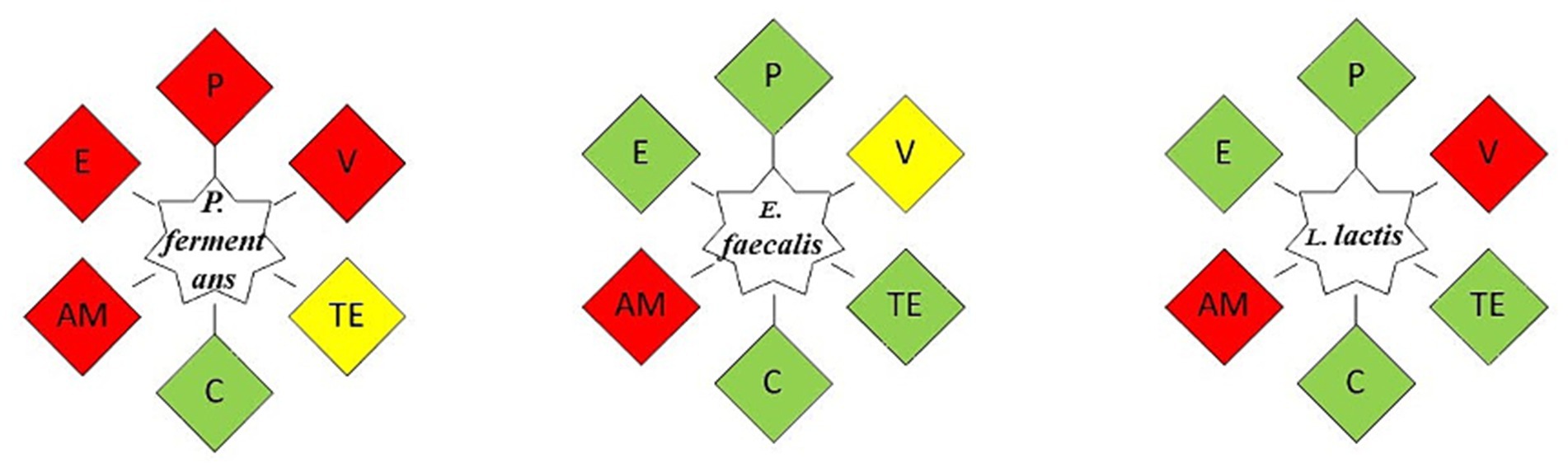
Figure 8. The antibiotic resistance of the isolates. As an indicator of the isolate’s susceptibility to the intended antibiotic, green represents its sensitivity to it, yellow indicates its relative resistance to it, and red shows its resistance. P, Penicillin; V, Vancomycin; TE, Tetracycline; C, Chloramphenicol; AM, Ampicillin; E, Erythromycin.
Since antibiotic resistance varies depending on the strain, many articles have revealed different results about it. Moreover, the source of antibiotic resistance genes is another factor that can influence antibiotic resistance; if it is intrinsic, it cannot be passed on, which is a quality that may be desired; More specifically, this property enables probiotics to restore the gut microbiota during or following antibiotic therapy; but, if it is acquired, it poses a risk of spreading to other microorganisms. Although the isolates in this investigation did not exhibit phenotypic resistance to the majority of antibiotics, it is crucial to look into the existence of antibiotic resistance genes in future research and, in the following phase, determine whether these genes are inherent or acquired (Azhar and Munaim, 2019; Hurtado-Romero et al., 2021).
3.4.2 Hemolytic activity
Hemolysins are protein enzymes or non-protein toxins that cause cellular disruption; This mechanism involves creating pores in the cell membrane. There are three types of hemolysis generated by bacteria: alpha (α), beta (β), and gamma (γ). Alpha hemolysis is the relative lysis of red blood cells that results in the colony area turning green following incubation. Gamma hemolysis does not cause hemolysis. On the other hand, in β hemolysis, the red blood cells undergo complete lysing and following incubation, the colony turns transparent. Therefore probiotics must be hemolysin-free (Kim et al., 2022; Rahmani et al., 2022).
None of the isolates showed β-hemolysis after 24 h, which is consistent with the results of Baccouri et al. (2019), Kanak et al. (2023), Yang et al. (2023), and Rahmani et al. (2022).
4 Limitations
• Failure to investigate virulence factors, especially in the E. faecalis strain.
5 Conclusion
In the contemporary global landscape, there is a marked and increasing interest in the production and consumption of functional foods, attributed to their health benefits. This study delves into the realm of kefir, a widely acclaimed functional beverage, renowned for its unique properties. Research has consistently linked the therapeutic qualities of kefir to the diverse microorganisms present within kefir grains. Our investigation focused on analyzing microorganisms isolated from Iranian kefir, scrutinizing their probiotic potential, technological merits, and safety attributes.
While these isolates displayed limited resistance to the conditions of the digestive tract, they exhibited promising results in several other key areas. Notably, the Cell-Free Supernatant (CFS) of these isolates was found to contain antioxidant compounds and Gamma-Aminobutyric Acid (GABA), a compound of significant value. These components present exciting opportunities for the extraction and development of novel functional food products. Furthermore, the CFS of Lactococcus lactis demonstrated a potent inhibitory effect on four common food-borne pathogens, highlighting its potential as a natural antimicrobial agent. This is particularly relevant given the current high demand for such natural compounds in the food industry.
Considering the limited digestive tract resistance of these isolates, the study proposes two strategic approaches to enhance their efficacy. First, the use of encapsulation techniques involving biomaterials could offer better protection to the probiotics to tackle the harsh conditions of the digestive tract. Secondly, the development of more robust and targeted delivery systems is suggested. Such systems could significantly improve the stability and survival rate of these microorganisms, ensuring that they retain their beneficial properties throughout the digestive process. This dual approach could be pivotal in maximizing the therapeutic potential of kefir-derived probiotics, thereby contributing to the broader field of functional food development.
Data availability statement
The raw data supporting the conclusions of this article will be made available by the authors, without undue reservation.
Author contributions
MM: Data curation, Formal analysis, Investigation, Methodology, Software, Writing – original draft. HO: Methodology, Writing – review & editing. MH: Methodology, Resources, Writing – review & editing. AA: Conceptualization, Funding acquisition, Project administration, Resources, Supervision, Validation, Visualization, Writing – review & editing.
Funding
The author(s) declare that financial support was received for the research, authorship, and/or publication of this article. This work was supported by Mashhad University of Medical Sciences, Mashhad, Iran, grant No 4010383.
Acknowledgments
We would like to thank Mashhad University of Medical Sciences, Mashhad, Iran for their financial support.
Conflict of interest
The authors declare that the research was conducted in the absence of any commercial or financial relationships that could be construed as a potential conflict of interest.
Publisher’s note
All claims expressed in this article are solely those of the authors and do not necessarily represent those of their affiliated organizations, or those of the publisher, the editors and the reviewers. Any product that may be evaluated in this article, or claim that may be made by its manufacturer, is not guaranteed or endorsed by the publisher.
Supplementary material
The Supplementary material for this article can be found online at: https://www.frontiersin.org/articles/10.3389/fmicb.2024.1385301/full#supplementary-material
References
Afshari, A., Hashemi, M., Tavassoli, M., Eraghi, V., and Noori, S. M. A. (2022). Probiotic bacteria from 10 different traditional Iranian cheeses: isolation, characterization, and investigation of probiotic potential. Food Sci. Nutr. 10, 2009–2020. doi: 10.1002/fsn3.2817
Akpinar, A., and Yerlikaya, O. (2021). Some potential beneficial properties of Lacticaseibacillus paracasei subsp. paracasei and Leuconostoc mesenteroides strains originating from raw milk and kefir grains. J. Food Process. Preserv. 45:e15986. doi: 10.1111/jfpp.15986
Almeida, M. E., Wallace Felipe, B., Pessoa, A. C., Melgaço, C., Ramos, L. P., Rezende, R. P., et al. (2022). In vitro selection and characterization of probiotic properties in eight lactobacillus strains isolated from cocoa fermentation. An. Acad. Bras. Cienc. 94:e20220013. doi: 10.1590/0001-3765202220220013
Amaretti, A., Di Nunzio, M., Pompei, A., Raimondi, S., Rossi, M., and Bordoni, A. (2013). Antioxidant properties of potentially probiotic bacteria: in vitro and in vivo activities. Appl. Microbiol. Biotechnol. 97, 809–817. doi: 10.1007/s00253-012-4241-7
Archer, A. C., and Halami, P. M. (2015). Probiotic attributes of Lactobacillus fermentum isolated from human feces and dairy products. Appl. Microbiol. Biotechnol. 99, 8113–8123. doi: 10.1007/s00253-015-6679-x
Azhar, M. A., and Munaim, M. S. A. (2019). Identification and evaluation of probiotic potential in yeast strains found in kefir drink samples from Malaysia. Int. J. Food Eng. 15:20180347. doi: 10.1515/ijfe-2018-0347
Azizi, N. F., Kumar, M. R., Yeap, S. K., Abdullah, J. O., Khalid, M., Omar, A. R., et al. (2021). Kefir and its biological activities. Food Secur. 10:1210. doi: 10.3390/foods10061210
Baccouri, O., Boukerb, A. M., Farhat, L. B., Zébré, A., Zimmermann, K., Domann, E., et al. (2019). Probiotic potential and safety evaluation of Enterococcus faecalis OB14 and OB15, isolated from traditional tunisian testouri cheese and rigouta, using physiological and genomic analysis. Front. Microbiol. 10:881. doi: 10.3389/fmicb.2019.00881
Bangotra, R., Kour, B., and Bajaj, B. K. (2023). Probiotic functional attributes of lactic acid bacteria from indigenously fermented milk product kalarei uct kalarei. Ind. J. Exp. Biol. 61, 546–557. doi: 10.56042/ijeb.v61i07.3116
Barzegar, H., Behbahani, B. A., and Falah, F. (2021). Safety, probiotic properties, antimicrobial activity, and technological performance of Lactobacillus strains isolated from Iranian raw milk cheeses. Food Sci. Nutr. 9, 4094–4107. doi: 10.1002/fsn3.2365
Bengoa, A. A., Iraporda, C., Acurcio, L. B., de Cicco, S. H., Sandes, K. C., Guimarães, G. M., et al. (2019). Physicochemical, immunomodulatory and safety aspects of milks fermented with Lactobacillus paracasei isolated from kefir. Food Res. Int. 123, 48–55. doi: 10.1016/j.foodres.2019.04.041
Bs, S., Thankappan, B., Mahendran, R., Muthusamy, G., Selta, D. R. F., and Angayarkanni, J. (2021). Evaluation of GABA production and probiotic activities of Enterococcus faecium BS5. Probiot. Antimicrob. Proteins 13, 993–1004. doi: 10.1007/s12602-021-09759-7
Cáceres, P. J., Peñas, E., Martinez-Villaluenga, C., Amigo, L., and Frias, J. (2017). Enhancement of biologically active compounds in germinated brown rice and the effect of sun-drying. J. Cereal Sci. 73, 1–9. doi: 10.1016/j.jcs.2016.11.001
Carasi, P., Jacquot, C., Romanin, D. E., Elie, A.-M., de Antoni, G. L., Urdaci, M. C., et al. (2014). Safety and potential beneficial properties of Enterococcus strains isolated from kefir. Int. Dairy J. 39, 193–200. doi: 10.1016/j.idairyj.2014.06.009
Cassanego, D., Richards, N., Valente, P., Mazutti, M., and Ramirez-Castrillon, M. (2017). Identification by PCR and evaluation of probiotic potential in yeast strains found in kefir samples in the city of Santa Maria, RS, Brazil. Food Sci. Technol. 38, 59–65. doi: 10.1590/1678-457x.13617
Cho, Y.-J., Kim, D.-H., Jeong, D., Seo, K.-H., Jeong, H. S., Lee, H. G., et al. (2018). Characterization of yeasts isolated from kefir as a probiotic and its synergic interaction with the wine byproduct grape seed flour/extract. LWT 90, 535–539. doi: 10.1016/j.lwt.2018.01.010
Cho, D.-H., and Lim, S.-T. (2016). Germinated brown rice and its bio-functional compounds. Food Chem. 196, 259–271. doi: 10.1016/j.foodchem.2015.09.025
Diez-Gutiérrez, L., San Vicente, L., Barrón, L. J. R., Villarán, M. d. C., and Chávarri, M. (2020). Gamma-aminobutyric acid and probiotics: multiple health benefits and their future in the global functional food and nutraceuticals market. J. Funct. Foods 64:103669. doi: 10.1016/j.jff.2019.103669
Doğan, M., and Ay, M. (2021). Evaluation of the probiotic potential of Pediococcus strains from fermented dairy product kefir. Czech J. Food Sci. 39, 376–383. doi: 10.17221/71/2021-CJFS
El-Fattah, A., Alaa, S. S., El-Dieb, S., and Elkashef, H. (2018). Developing functional yogurt rich in bioactive peptides and gamma-aminobutyric acid related to cardiovascular health. LWT 98, 390–397. doi: 10.1016/j.lwt.2018.09.022
Englerová, K., Nemcová, R., and Mudroňová, D. (2017). The study of the probiotic potential of the beneficial bacteria isolated from kefir grains. Folia Vet. 61, 27–37. doi: 10.1515/fv-2017-0005
Erfani, A., Shakeri, G., Moghimani, M., and Afshari, A. (2023). Specific species of probiotic bacteria as bio-preservative cultures for control of fungal contamination and spoilage in dairy products. Int. Dairy J. 151:105863. doi: 10.1016/j.idairyj.2023.105863
Falah, F., Vasiee, A., Behbahani, B. A., Yazdi, F. T., and Mortazavi, S. A. (2021). Optimization of gamma-aminobutyric acid production by Lactobacillus brevis PML1 in dairy sludge-based culture medium through response surface methodology. Food Sci. Nutr. 9, 3317–3326. doi: 10.1002/fsn3.2304
Franciosi, E., Carafa, I., Nardin, T., Schiavon, S., Poznanski, E., Cavazza, A., et al. (2015). Biodiversity and γ-aminobutyric acid production by lactic acid bacteria isolated from traditional alpine raw cow’s milk cheeses. Biomed. Res. Int. 2015, 1–11. doi: 10.1155/2015/625740
Ganatsios, V., Nigam, P., Plessas, S., and Terpou, A. (2021). Kefir as a functional beverage gaining momentum towards its health promoting attributes. Beverages 7:48. doi: 10.3390/beverages7030048
Ghafurian Nasab, A., Mortazavi, S. A., Tabatabaei Yazdi, F., and Sarabi Jamab, M. (2022). Optimization of gamma aminobutyric acid production using high pressure processing (HPP) by Lactobacillus brevis PML1. Biomed. Res. Int. 2022, 1–9. doi: 10.1155/2022/8540736
Goktas, H., Dikmen, H., Demirbas, F., Sagdic, O., and Dertli, E. (2021). Characterisation of probiotic properties of yeast strains isolated from kefir samples. Int. J. Dairy Technol. 74, 715–722. doi: 10.1111/1471-0307.12802
Guetouache, M., and Guessas, B. (2015). Characterization and identification of lactic acid bacteria isolated from traditional cheese (Klila) prepared from cows milk. Afr. J. Microbiol. Res. 9, 71–77. doi: 10.5897/AJMR2014.7279
Gul, O., Atalar, I., Mortas, M., and Dervisoglu, M. (2018). Rheological, textural, colour and sensorial properties of kefir produced with buffalo milk using kefir grains and starter culture: a comparison with cows’ milk kefir. Int. J. Dairy Technol. 71, 73–80. doi: 10.1111/1471-0307.12503
Guo, X.-f., Hagiwara, T., Masuda, K., and Watabe, S. (2011). Optimal reaction conditions for the production of γ-aminobutyric acid by the marine yeast isolate Pichia anomala MR-1 strain. Biosci. Biotechnol. Biochem. 75, 1867–1871. doi: 10.1271/bbb.100425
Han, S.-M., and Lee, J.-S. (2017). Production and its anti-hyperglycemic effects of γ-aminobutyric acid from the wild yeast strain Pichia silvicola UL6-1 and Sporobolomyces carnicolor 402-JB-1. Mycobiology 45, 199–203. doi: 10.5941/MYCO.2017.45.3.199
He, Y., Li, F., Zhang, W., An, M., Li, A., Wang, Y., et al. (2022). Probiotic potential of Bacillus amyloliquefaciens isolated from Tibetan yaks. Probiot. Antimicrob. Proteins 16, 212–223. doi: 10.1007/s12602-022-10027-5
Hejazi, M. A., Ghafouri-Fard, S., Eslami, S., Afshar, D., Barzegari, A., and Khorshidian, N. (2019). Polyphasic characterization of Enterococcus strains isolated from traditional Moghan cheese in Iran. J. Food Saf. 39:e12631. doi: 10.1111/jfs.12631
Hsu, S.-A., and Chou, J.-Y. (2021). Yeasts in fermented food and kefir: in vitro characterization of probiotic traits. J. Anim. Plant Sci. 31, 567–582. doi: 10.36899/JAPS.2021.2.0245
Hurtado-Romero, A., Del Toro-Barbosa, M., Gradilla-Hernández, M. S., Garcia-Amezquita, L. E., and García-Cayuela, T. (2021). Probiotic properties, prebiotic fermentability, and GABA-producing capacity of microorganisms isolated from Mexican milk kefir grains: a clustering evaluation for functional dairy food applications. Food Secur. 10:2275. doi: 10.3390/foods10102275
Iqbal, M., Ahmad, A., Fatima, G., and Mirza, S. (2017). Antibiotic resistance pattern in nosocomial urinary isolates of Enterococcus. Infect. Dis. J. Pak. 26, 43–47.
Kanak, E. K., Yilmaz, S. Ö., Altindiş, M., Tanriverdi, E. S., and Otlu, B. (2023). Determination of safety status and probiotic properties of Enterococcus strains isolated from traditional cheeses in Turkey. J. Appl. Microbiol. 134:lxac005. doi: 10.1093/jambio/lxac005
Katiku, M. M., Matofari, J. W., and Nduko, J. M. (2022). Preliminary evaluation of probiotic properties and safety profile of Lactiplantibacillus plantarum isolated from spontaneously fermented milk, Amabere amaruranu. Heliyon 8:e10342. doi: 10.1016/j.heliyon.2022.e10342
Khanlari, Z., Moayedi, A., Ebrahimi, P., Khomeiri, M., and Sadeghi, A. (2021). Enhancement of γ-aminobutyric acid (GABA) content in fermented milk by using Enterococcus faecium and Weissella confusa isolated from sourdough. J. Food Process. Preserv. 45:e15869. doi: 10.1111/jfpp.15869
Kim, Y. G., Choi, S.-I., Jeong, Y., and Kang, C.-H. (2022). Evaluation of safety and probiotic potential of Enterococcus faecalis MG5206 and Enterococcus faecium MG5232 isolated from kimchi, a Korean fermented cabbage. Microorganisms 10:2070. doi: 10.3390/microorganisms10102070
Kondrotiene, K., Lauciene, L., Andruleviciute, V., Kasetiene, N., Serniene, L., Sekmokiene, D., et al. (2020). Safety assessment and preliminary in vitro evaluation of probiotic potential of Lactococcus lactis strains naturally present in raw and fermented milk. Curr. Microbiol. 77, 3013–3023. doi: 10.1007/s00284-020-02119-8
Kotowicz, N., Bhardwaj, R. K., Ferreira, W. T., Hong, H. A., Olender, A., Ramirez, J., et al. (2019). Safety and probiotic evaluation of two Bacillus strains producing antioxidant compounds. Benefic. Microbes 10, 759–771. doi: 10.3920/BM2019.0040
Lakhlifi, T., El Oirdi, S., Maroui, I., Zouhair, R., and Belhaj, A. (2023). Probiotic properties and safety aspect of three antifungal lactic acid bacteria strains isolated from wheat and camel milk. Biologia 78, 1129–1139. doi: 10.1007/s11756-023-01319-4
LeBlanc, J. G., Levit, R., Savoy de Giori, G., and de Moreno de LeBlanc, A. (2020). Application of vitamin-producing lactic acid bacteria to treat intestinal inflammatory diseases. Appl. Microbiol. Biotechnol. 104, 3331–3337. doi: 10.1007/s00253-020-10487-1
Leite, A. M. O., Miguel, M. A. L., Peixoto, R. S., Patricia Ruas-Madiedo, V. M. F., Paschoalin, B. M., and Delgado, S. (2015). Probiotic potential of selected lactic acid bacteria strains isolated from Brazilian kefir grains. J. Dairy Sci. 98, 3622–3632. doi: 10.3168/jds.2014-9265
Li, Y., Wang, T., Li, S., Yin, P., Sheng, H., Wang, T., et al. (2022). Influence of GABA-producing yeasts on cheese quality, GABA content, and the volatilome. LWT 154:112766. doi: 10.1016/j.lwt.2021.112766
Ly, D., Sigrid Mayrhofer, I. B., Yogeswara, A., Nguyen, T.-H., and Domig, K. J. (2019). Identification, classification and screening for γ-amino-butyric acid production in lactic acid bacteria from Cambodian fermented foods. Biomol. Ther. 9:768. doi: 10.3390/biom9120768
Mantzourani, I., Chondrou, P., Bontsidis, C., Karolidou, K., Terpou, A., Alexopoulos, A., et al. (2019). Assessment of the probiotic potential of lactic acid bacteria isolated from kefir grains: evaluation of adhesion and antiproliferative properties in in vitro experimental systems. Ann. Microbiol. 69, 751–763. doi: 10.1007/s13213-019-01467-6
Martirosyan, D. M., and Singh, J. (2015). A new definition of functional food by FFC: what makes a new definition unique? Funct. Foods Health Dis. 5, 209–223. doi: 10.31989/ffhd.v5i6.183
Merchán, A. V., Benito, M. J., Galván, A. I., and de Herrera, S. R. (2020). Identification and selection of yeast with functional properties for future application in soft paste cheese. LWT 124:109173. doi: 10.1016/j.lwt.2020.109173
Mitra, S., and Ghosh, B. C. (2020). Quality characteristics of kefir as a carrier for probiotic Lactobacillus rhamnosus GG. Int. J. Dairy Technol. 73, 384–391. doi: 10.1111/1471-0307.12664
Moghimani, M., Salari, A., Hashemi, M., Soleimanpour, S., Ranjbar, G., and Afshari, A. (2023). Iranian traditional kefir beverage: isolation and identification of beneficial microorganisms and evaluation of antimicrobial activity against food-borne pathogens. Nutr. Food Sci. 53, 1257–1267. doi: 10.1108/NFS-01-2023-0023
Nami, Y., Bakhshayesh, R. V., Jalaly, H. M., Lotfi, H., Eslami, S., and Hejazi, M. A. (2019). Probiotic properties of Enterococcus isolated from artisanal dairy products. Front. Microbiol. 10:300. doi: 10.3389/fmicb.2019.00300
Nascimento, L. C., Casarotti, S. N., Todorov, S. D., and Penna, A. L. B. (2019). Probiotic potential and safety of enterococci strains. Ann. Microbiol. 69, 241–252. doi: 10.1007/s13213-018-1412-5
Nielsen, B., Candan Gürakan, G., and Ünlü, G. (2014). Kefir: a multifaceted fermented dairy product. Probiot. Antimicrob. Proteins 6, 123–135. doi: 10.1007/s12602-014-9168-0
Ogunremi, O. R., Sanni, A. I., and Agrawal, R. J. J. O. A. M. (2015). Probiotic potentials of yeasts isolated from some cereal-based Nigerian traditional fermented food products. J. Appl. Microbiol. 119, 797–808. doi: 10.1111/jam.12875
Ozma, M. A., Khodadadi, E., Rezaee, M. A., Kamounah, F. S., Asgharzadeh, M., Ganbarov, K., et al. (2021). Induction of proteome changes involved in biofilm formation of Enterococcus faecalis in response to gentamicin. Microb. Pathog. 157:105003. doi: 10.1016/j.micpath.2021.105003
Perpetuini, G., Tittarelli, F., Battistelli, N., Suzzi, G., and Tofalo, R. (2020). γ-Aminobutyric acid production by Kluyveromyces marxianus strains. J. Appl. Microbiol. 129, 1609–1619. doi: 10.1111/jam.14736
Pytka, M., Kordowska-Wiater, M., Wajs, J., Glibowski, P., and Sajnaga, E. (2022). Usefulness of potentially probiotic L. lactis isolates from polish fermented cow milk for the production of cottage cheese. Appl. Sci. 12:12088. doi: 10.3390/app122312088
Rahmani, B., Alimadadi, N., Attaran, B., and Nasr, S. (2022). Yeasts from Iranian traditional milk kefir samples: isolation, molecular identification and their potential probiotic properties. Lett. Appl. Microbiol. 75, 1264–1274. doi: 10.1111/lam.13794
Ribeiro, S. C., Domingos-Lopes, M. F. P., Catherine Stanton, R., Ross, P., and Silva, C. C. G. (2018). Production of-aminobutyric acid (GABA) by Lactobacillus otakiensis and other Lactobacillus sp. isolated from traditional Pico cheese. Int. J. Dairy Technol. 71, 1012–1017. doi: 10.1111/1471-0307.12527
Rosa, D. D., Dias, M. M. S., Grześkowiak, Ł. M., Reis, S. A., Conceição, L. L., and Peluzio, M. d. C. G. (2017). Milk kefir: nutritional, microbiological and health benefits. Nutr. Res. Rev. 30, 82–96. doi: 10.1017/S0954422416000275
Sakkaa, S. E., Zaghloul, E. H., and Ghanem, K. M. (2022). Psychobiotic potential of gamma-aminobutyric acid–producing marine enterococcus faecium SH9 from marine shrimp. Probiot. Antimicrob. Proteins 14, 934–946. doi: 10.1007/s12602-022-09963-z
Sionek, B., Szydłowska, A., Zielińska, D., Neffe-Skocińska, K., and Kołożyn-Krajewska, D. (2023). Beneficial Bacteria isolated from food in relation to the next generation of probiotics. Microorganisms 11:1714. doi: 10.3390/microorganisms11071714
Talib, N., Mohamad, N. E., Yeap, S. K., Hussin, Y., Aziz, M. N. M., Masarudin, M. J., et al. (2019). Isolation and characterization of Lactobacillus spp. from kefir samples in Malaysia. Molecules 24:2606. doi: 10.3390/molecules24142606
Tamura, T., Noda, M., Ozaki, M., Maruyama, M., Matoba, Y., Kumagai, T., et al. (2010). Establishment of an efficient fermentation system of gamma-aminobutyric acid by a lactic acid bacterium, Enterococcus avium G-15, isolated from carrot leaves. Biol. Pharm. Bull. 33, 1673–1679. doi: 10.1248/bpb.33.1673
Tan, L. L., Tan, C. H., Ng, N. K. J., Tan, Y. H., Conway, P. L., and Loo, S. C. J. (2022). Potential probiotic strains from milk and water kefir grains in Singapore—use for defense against enteric bacterial pathogens. Front. Microbiol. 13:857720. doi: 10.3389/fmicb.2022.857720
Touranlou, F. A., Noori, S. M. A., Salari, A., Afshari, A., and Hashemi, M. (2023). Application of kefir for reduction of contaminants in the food industry: a systematic review. Int. Dairy J. 146:105748. doi: 10.1016/j.idairyj.2023.105748
Yang, Z., Ni, L., Tian, W., and Chi, H. (2023). Screening and identification of goat-Milk-derived lactic acid Bacteria with Bacteriocin-like activity and probiotic potentials. Microorganisms 11:849. doi: 10.3390/microorganisms11040849
Yerlikaya, O. (2019). Probiotic potential and biochemical and technological properties of Lactococcus lactis ssp. lactis strains isolated from raw milk and kefir grains. J. Dairy Sci. 102, 124–134. doi: 10.3168/jds.2018-14983
Youn, H.-Y., Kim, D.-H., Kim, H.-J., Bae, D., Song, K.-Y., Kim, H., et al. (2022). Survivability of Kluyveromyces marxianus isolated from Korean kefir in a simulated gastrointestinal environment. Front. Microbiol. 13:842097. doi: 10.3389/fmicb.2022.842097
Zamanpour, S., Rezvani, R., Isfahani, A. J., and Afshari, A. (2023). Isolation and some basic characteristics of lactic acid bacteria from beetroot (Beta vulgaris L.)—a preliminary study. Canrea J. 6, 42–56. doi: 10.20956/canrea.v6i1.980
Zanirati, D. F., Abatemarco, M. Jr., Sandes, S. H. d. C., Nicoli, J. R., Nunes, Á. C., and Neumann, E. (2015). Selection of lactic acid bacteria from Brazilian kefir grains for potential use as starter or probiotic cultures. Anaerobe 32, 70–76. doi: 10.1016/j.anaerobe.2014.12.007
Keywords: kefir, food microbiology, probiotic potential, gastrointestinal tract, gamma-aminobutyric acid, thin-layer chromatography, safety characteristics, Enterococcus faecalis
Citation: Moghimani M, Onyeaka H, Hashemi M and Afshari A (2024) Evaluation of the probiotic, technological, safety attributes, and GABA-producing capacity of microorganisms isolated from Iranian milk kefir beverages. Front. Microbiol. 15:1385301. doi: 10.3389/fmicb.2024.1385301
Edited by:
Christian Ariel Lopes, Institute for Research and Development in Process Engineering, Biotechnology and Alternative Energies (CONICET PROBIEN), ArgentinaReviewed by:
Kalpana Bhatt, Purdue University, United StatesKarina Teixeira Magalhães-Guedes, Federal University of Bahia (UFBA), Brazil
María Chávarri, Tecnalia Research and Innovation, Spain
Copyright © 2024 Moghimani, Onyeaka, Hashemi and Afshari. This is an open-access article distributed under the terms of the Creative Commons Attribution License (CC BY). The use, distribution or reproduction in other forums is permitted, provided the original author(s) and the copyright owner(s) are credited and that the original publication in this journal is cited, in accordance with accepted academic practice. No use, distribution or reproduction is permitted which does not comply with these terms.
*Correspondence: Asma Afshari, YWZzaGFyaWFzQG11bXMuYWMuaXI=