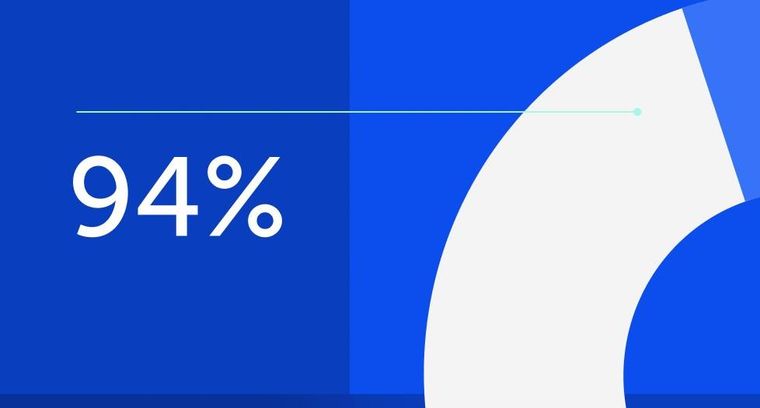
94% of researchers rate our articles as excellent or good
Learn more about the work of our research integrity team to safeguard the quality of each article we publish.
Find out more
ORIGINAL RESEARCH article
Front. Microbiol., 29 April 2024
Sec. Food Microbiology
Volume 15 - 2024 | https://doi.org/10.3389/fmicb.2024.1383779
This article is part of the Research TopicInnovations in Microbiome Applications for Health-Promoting and Sustainable Food SystemsView all 7 articles
Introduction: The effects of fructo-oligosaccharides (FOS) on atopic dermatitis (AD) have not been determined.
Methods: In a randomized, double-blind, placebo-controlled trial, children with AD aged 24 months to 17 years received either advanced FOS containing 4.25 g of 1-kestose or a placebo (maltose) for 12 weeks.
Results: The SCORAD and itching scores were reduced in patients treated with both FOS (all p < 0.01) and maltose (p < 0.05 and p < 0.01). Sleep disturbance was improved only in the FOS group (p < 0.01). The FOS group revealed a decreased proportion of linoleic acid (18:2) esterified omega-hydroxy-ceramides (EOS-CERs) with amide-linked shorter chain fatty acids (C28 and C30, all p < 0.05), along with an increased proportion of EOS-CERs with longer chain fatty acids (C32, p < 0.01).
Discussion: FOS may be beneficial in alleviating itching and sleep disturbance, as well as improving skin barrier function in children with AD.
Although the precise pathophysiology underlying atopic dermatitis (AD) remains incompletely understood, extensive studies have emphasized the significance of microbial dysbiosis and abnormalities in epidermal barrier proteins and lipids (Langan et al., 2020; Kim et al., 2023a,b). Recent investigations suggest that oral administration of prebiotics, including galactooligosaccharides (GOS) and fructo-oligosaccharides (FOS) hold promise in treating AD by serving as energy sources for gut microbes and generating short-chain fatty acids that enhance the growth of beneficial microorganisms (Kim et al., 2021; Kim S. et al., 2022; Ahn, 2023). Among FOS variants, 1-kestose (GF2), a trisaccharide formed by the combination of a fructose molecule with glucose, can lower pH in culture media and stimulate the growth of Bifidobacterium breve, B. longum, B. bifidum, and B. adolescentis more effectively than other variants (Tochio et al., 2018). While normal FOS, which is composed of 35% 1-kestose, is used widely in food products, dietary supplements, animal feed, and pharmaceuticals, kestose-enriched advanced FOS (>85% 1-kestose) has been developed as the dominant component in synthetic FOS products (Kim et al., 2021).
Despite the strong prebiotic effect of purified 1-kestose at lower doses, as well as fewer adverse reactions such as diarrhea, abdominal distention, and flatulence (Tochio et al., 2018), its therapeutic effect on AD has been investigated in few studies. Koga et al. (2016) who conducted a randomized controlled trial of 1-kestose for 6 weeks, found a significant positive correlation between increased counts of gut Faecalibacterium prausnitzii and improvements in SCORing Atopic Dermatitis (SCORAD) scores in children aged 2 to 5 years with AD. Similarly, Shibata et al. (2009) revealed lower SCORAD scores in AD patients who received 2 g of 1-kestose daily for 12 weeks compared to the placebo group in a randomized, double-blind, placebo-controlled study involving children under 3 years of age. Despite the potential beneficial effects of 1-kestose in AD treatment, previous studies lack substantial evidence due to limitations such as insufficient clinical improvement and the absence of mechanism studies (Ahn, 2023). Moreover, in Shibata et al.’s study, no significant correlation was found between improvements in SCORAD score and Bifidobacterium counts.
We previously reported the beneficial effects of GOS on skin-barrier function and AD-like skin symptoms, suggesting the possibility of direct effects from prebiotics or probiotics on AD skin (Kim S. et al., 2022). However, mechanisms that explain the direct and indirect effects of 1-kestose on human AD skin have yet to be identified. Therefore, we aimed to examine the impacts of 1-kestose on AD skin beyond the clinical aspects, including the modulation of epidermal skin barrier and lipid profiles as well as skin microbiome.
In this randomized, double-blind, placebo-controlled study, we enrolled patients aged 2–17 years diagnosed with AD based on the criteria established by Hanifin and Rajka (1980), with SCORAD scores ranging from 15 to 50. Exclusion criteria were recent administration of antibiotics, systemic corticosteroids, or phototherapy within the past 4 weeks; use of systemic immunosuppressants or biologics within the previous 4 weeks; intake of probiotics, prebiotics, or herbal medicine within the past week; use of antihistamines within the previous 2 weeks; and concurrent presence of other skin diseases or systemic illnesses.
Patients were randomly assigned to receive either advanced FOS or placebo treatments in a double-blind manner, using a computer-generated random-number list. An investigator not involved in the trial prepared the random-number list, which was then posted to the bags containing the materials. Samples of advanced FOS (composed of >85% 1-kestose) and maltose powder were provided by Food R&D, Samyang Corp (Seongnam, Korea). Over the course of 12 weeks, patients were orally administered either advanced FOS at a dosage of 2.5 g twice daily or a placebo of identical appearance and taste. During the study period, emollients (Atobarrier lotion MD, Aestura, Seoul, Korea) and topical corticosteroids (TCS; hydrocortisone 1% and prednisolone valeroacetate 0.3%) were made available during the study. To quantitatively assess their usage, the tubes containing emollients and TCS were weighed at each visit. The study was approved by the Institutional Review Board of Samsung Medical Center in Seoul, and written informed consent was obtained from parents prior to participation in the study (SMC IRB file No. 2020-11-155). Our study protocol was registered in the WHO ICTRP (International Clinical Trials Registry Platform). The registration number is KCT0006816.
Before initiation of treatment (T1) using either advanced FOS or placebo and upon completion of treatment (T2), children underwent clinical evaluations and blood tests (Figure 1). The severity of AD was assessed using the SCORAD score, ranging from 0 to 103 (European Task Force on Atopic Dermatitis, 1993). This grading system includes objective and subjective scores, evaluating pruritus and sleep disturbance on scales from 0 to 10. Measurements were performed for eosinophil counts in the peripheral blood, mRNA expression levels of fatty-acid elongation enzymes, and serum total immunoglobin E (IgE) levels determined by immunoCAP tests (ThermoFisher Scientific Inc., Waltham, MA). Skin tape stripping and skin microbiome samples were collected at T1 and T2.
Hypervariable regions V3-V4 of the 16S rRNA bacterial gene were amplified on skin samples collected and sequenced with an Illumina MiSeq platform (Illumina, San Diego, CA, USA). The 16S rRNA gene sequence data were analyzed using Quantitative Insights Into Microbial Ecology software (v1.9.1; Caporaso et al., 2010). Using qualified sequences (Phred ≥ Q20), operational taxonomic units were identified and quantified using an open reference method that mapped sequences with 97% identity to known sequences in the Greengenes database (v13.8) using UCLUST alignment algorithms and the EzBioCloud database1 (DeSantis et al., 2006; Edgar, 2010; Yoon et al., 2017).
Samples of human stratum corneum (SC) were collected from the right forearm skin using D-squame tape with a diameter of 22 mm (CuDerm, Dallas, TX). Four consecutive D-squame strips were collected from each subject. The first tape disk was discarded, and the remaining three were placed in separate Eppendorf tubes (1.5 mL). Skin ceramides were extracted from the tape-stripping samples following a modified Sadowski method (Kim B. K. et al., 2022). In brief, each tape was vortexed with 1 mL of methanol for 1 h and centrifuged at 14,000 g and 4°C for 15 min. After removing the tape and debris, the extracts from three consecutive tape strips were pooled and dried under a nitrogen stream. The dried extracts were reconstituted in 100 μL of a chloroform-methanol mixture (1:9, v/v) containing the internal standard of EO-type ceramide (EOS, d18:1/26:0/ d9-18:1) purchased from Avanti Polar Lipids (Alabaster, AL, United States). Targeted skin lipidome analysis was performed using a modified Shimadzu LCMS-8060 liquid chromatograph–mass spectrometer (Shimadzu Corporations, Kyoto, Japan; Chu et al., 2023). Skin lipids were separated on a reversed-phase Kinetex C18 column (100 × 2.1 mm, 2.6 μm; Phenomenex, Torrance, CA, USA). Mobile phases A and B consisted of 10 mM ammonium acetate in water/methanol (1/9, v/v) and 10 mM ammonium acetate in methanol/isopropanol (1/1, v/v), respectively. The gradient elution was as follows: 0 min (30% B), 0–15 min (95% B), 15–20 min (95% B), and 20–25 min (30% B). The flow rate was 0.2 mL/min. Quantitation was performed using selected reaction monitoring of the [M + H]+ ion and the related product ion for each lipid. Each lipid was quantified as the peak area ratio of the target analyte to that of the internal standard multiplied by the concentration of the standard (Suzuki et al., 2022).
Human primary keratinocytes (HEKs; Thermo Fisher Scientific, Walthamm, MA, Cat #: C0015C) were derived from neonatal foreskin and grown in serum-free EpiLife™ cell culture medium (Life Technologies, Grand Island, NY, United States) containing 0.06 mmol/L calcium chloride, 1% human keratinocyte growth supplement S7 (Life Technologies), and 1% gentamicin/amphotericin as described previously (Kim S. et al., 2022). To investigate the effects of the advanced FOS on gene expression of fatty acid elongases (ELOVLs) 1–6, ceramide synthase (CERS) 2 and 3, filaggrin (FLG), loricrin (LOR), and involucrin (IVL), HEKs were differentiated in the presence of CaCl2 (1.3 mmol/L) for 3 days. Cells were then incubated with maltose (1.0%), advanced FOS (1.0%), or a combination, interleukin (IL) 4 (5 ng/mL, R&D Systems, Minneapolis, MN, United States) and IL-13 (5 ng/mL, R&D Systems) for an additional 24 h. All reagents, including maltose, advanced FOS, and cytokines, were diluted using serum-free EpiLife medium for in vitro experiments.
RNeasy Mini Kits (Qiagen, Valencia, CA, USA) were used according to the manufacturer’s protocol to isolate RNA from keratinocytes. The RNA was reverse-transcribed into cDNA using a SuperScript VILO™ MasterMix according to the manufacturer’s protocol (Life Technologies). The cDNA was analyzed using real-time quantitative polymerase chain reaction (PCR) amplification and an ABI prism 7900 real-time PCR instrument (Applied Biosystems, Foster City, CA, United States) as described previously (Kim S. et al., 2022). Primers and probes for ELOVL1-6, CERS2, CERS3, FLG, LOR, IVL, and 18 s were purchased from Applied Biosystems and normalized to the 18 s mRNA levels.
The sample size was determined based on previous studies (Han et al., 2012; Jeong et al., 2020), estimating a difference of 6.5 in SCORAD change scores between the FOS and placebo groups. We calculated that 49 patients in each group (80% power, 5% significance, with a 20% dropout rate) would be needed. The final analysis included patients with adherence exceeding 80%, and skin samples were collected at both T1 and T2. All statistical analyses were conducted using GraphPad Prism, version 9 (San Diego, CA, United States) and SAS® (version 9.4, SAS Institute, Cary, NC, United States). Chi-squared test or Fisher’s exact test was performed to explore the difference for categorical data between the FOS and the control groups. For comparisons between the groups, independent t-test or Wilcoxon signed-rank test was used. For comparisons between T1 and T2 time points within group, paired t-test or Wilcoxon rank-sum test was applied. Statistical differences between 3 or more groups were determined using a one-way ANOVA, and significant differences were determined by a Tukey–Kramer post-hoc test. Additionally, Spearman’s correlation was used to evaluate correlations between variables. Error bars represent the SEM, and significance was set at a p value < 0.05.
Analysis was conducted on 48 patients (24 in each group), all of whom had adherence exceeding 80% and provided skin samples (Figure 1). There were no differences in sex, age, comorbid allergic diseases, family history of allergic diseases, baseline SCORAD scores, the level of total IgE and the eosinophil count between the two groups (Table 1). The total scores and the objective scores of the SCORAD at T2 were significantly decreased in both groups (all p < 0.01; Figures 2A,B). The itching scores were reduced in both the FOS and the control groups, but showed a more significant decrease in the FOS group compared to the control group (p = 0.001 vs. p = 0.043; Figure 2C). Sleep disturbance scores were significantly decreased only in the FOS group at T2 (p = 0.003), while no changes were found in the control group (p = 0.134; Figure 2D). In the FOS group, the percentage of eosinophil was significantly lower at T2 than T1 (p = 0.024), whereas no significant changes were observed in the control group (p = 0.413; Figure 2E). However, there were no significant changes in the level of total IgE in either group between T1 and T2 (Figure 2F). Similarly, there were no significant differences in the amount of emollients and topical corticosteroids used during the study period (p = 0.802 and 0.209, respectively). No serious adverse events occurred in either group, and only 1 patient in the control group dropped out due to adverse events. No adverse events related to the intervention were reported in either group.
Figure 2. Changes in total (A) and objective SCORAD (B) scores, itching scores (C), sleep disturbance scores (D), peripheral blood eosinophil percentages (E), and total IgE levels (F) at T2 compared to baseline (T1) in the FOS and control groups. *p < 0.05, **p < 0.01. FOS, fructo-oligosaccharide; IgE, immunoglobulin E; SCORAD, SCORing Atopic Dermatitis.
No significant differences were observed in the bacterial phyla between the FOS and the control group both at T1 and T2 (all p > 0.05; Figure 3). However, at the genus level, a higher abundance of Micrococcus was found in the FOS group compared to the control group at T1 (p = 0.049; Figure 4A). After 12 weeks of treatment, the FOS group showed decreased abundance of Lachnospiraceae (p = 0.040; Figure 4B) and increased abundance of Prevotella (p = 0.007; Figure 4C), while the control group revealed a decreased abundance of Methylobacterium (p = 0.008; Figure 4D). Additionally, a higher abundance of Rothia was noted in the FOS group compared to the control group at T2 (p = 0.025; Figure 4E).
Figure 3. Skin microbiome composition at the phylum levels in the FOS and control groups. FOS, fructo-oligosaccharide; B, before; A, after, F, FOS group; C, control group.
Figure 4. The change in relative abundance of Micrococcus (A), Lachnospiraceae (B), Prevotella (C), Methylobacterium (D), and Rothia (E) in the FOS and control groups. *p < 0.05, **p < 0.01. FOS, fructo-oligosaccharide.
The proportion of linoleic acid (18:2) esterified omega-hydroxy-ceramides (EOS-CERs) with amide-linked shorter chain fatty acids (C28 and C30) was decreased significantly in the FOS group (p = 0.029 and 0.013; Figure 5A). Additionally, the proportion of EOS-CERs with amide-linked longer chain fatty acids (C32) was increased in the FOS group (p = 0.010; Figure 5A). However, no significant change was observed in the proportion of EOS-CERs in the control group (Figure 5B). While no relationship between itching scores and EOS-CERs with amide-linked C28 fatty acids (p = 0.568), itching scores were positively correlated with EOS-CERs with amide-linked C30 fatty acids (p = 0.007; Figures 5C,D). The negative relationship between itching scores and the proportion of EOS-CERs with amide-linked longer-chain fatty acids (C32) was not statistically significant (p = 0.087; Figure 5E).
Figure 5. Proportion of EOS-CERs in the FOS (A) and control (B) groups and correlation between itching scores and the proportion of EOS-CERs with amide-linked C28 (C), C30 (D), and C32 (E) fatty acids in all subjects. *p < 0.05, **p < 0.01. EOS-CER, linoleic acid (18:2) esterified omega-hydroxy-ceramide; FOS, fructo-oligosaccharide.
Gene expression of ELOVL3 was significantly upregulated in HEKs treated with advanced FOS compared to those treated with media (p < 0.001) or maltose (p = 0.025; Figure 6A). Gene expression of ELOVL3 was significantly decreased by a combination of IL-4 and IL-13 as expected (p < 0.001; Figure 6A; Berdyshev et al., 2018). Gene expression of ELOVL4 was not affected by advanced FOS (Figure 6B). Likewise, gene expression of ELOVL1, 2, 5, 6, CERS2, and CERS3 was not affected by advanced FOS (Figure S1). Additionally, gene expression of ELOVL3 was significantly increased in HEKs treated with maltose compared to those treated with media alone (p = 0.039; Figure 6A). However, gene expression of ELOVL4 was decreased by maltose (p = 0.020; Figure 6B).
Figure 6. Gene expression of ELOVL3 (A), ELOVL4 (B), FLG (C), LOR (D), and IVL (E) in primary epidermal keratinocytes treated with media alone, maltose, advanced FOS, or a combination of IL-4 and IL-13. *p < 0.05, **p < 0.01, ***p < 0.001. ELOVL, elongating of very long chain fatty acids; FLG, filaggrin; FOS, fructo-oligosaccharide; IVL, involucrin; LOR, loricrin.
Moreover, gene expression of FLG and was significantly upregulated in HEKs treated with advanced FOS compared to those treated with media alone (p < 0.001) or maltose (p < 0.001; Figure 6C). Gene expression of FLG was significantly decreased by a combination of IL-4 and IL-13 as expected (Figure 6C; Howell et al., 2009). However, gene expression of LOR (Figure 6D) and IVL (Figure 6E) was not affected by maltose or advanced FOS.
In the present study, we showed that advanced FOS can alleviate symptoms and be safely consumed in children with AD. Over a 12-week treatment period, the FOS group displayed a more pronounced decrease in itching scores and sleep disturbance compared to the control group. Furthermore, the FOS group exhibited a decrease in eosinophil counts and specific alterations in the skin microbiome and epidermal lipid profiles. These findings suggest potential mechanisms underlying the observed clinical improvements.
Itching, a characteristic symptom of AD, triggers scratching, exacerbating skin lesions, and perpetuating a detrimental cycle (Langan et al., 2020). This cycle detrimentally impacts sleep quality, thereby affecting the overall well-being of children with AD and their families (Ständer et al., 2019). The substantial improvement in itching and sleep disturbances observed with FOS treatment was clinically relevant in our study, potentially preventing vicious cycles and chronicity in children with AD and positively influencing their quality of life. These clinical improvements correlate with changes in the percentage of eosinophils in peripheral blood, indicating that immunologic alteration contributes to the therapeutic effect of the prebiotics. These results correspond with those of earlier studies which reported significant improvements in itching and sleep disturbances following a 12-week intake of advanced FOS in children under 3 years of age with AD (Shibata et al., 2009). However, unlike our study, that study showed a reduction in SCORAD scores, while both the total and objective SCORAD scores were reduced in both the FOS and control groups in our current study. Discrepancies in host age, AD severity, ethnicity, or atopic characteristics might account for these differences. It is also possible that the effects observed in the control group may be attributed to the effects of maltose.
Skin barrier dysfunction is characteristic finding of AD, substantially contributing to its progression and the manifestation of pruritic symptoms (Kim and Leung, 2018; Leung et al., 2020; van den Bogaard et al., 2023). Therefore, to understand the mechanisms underlying the positive effects of advanced FOS on AD symptoms, we investigated whether advanced FOS modulates the expression of ELOVLs and epidermal barrier proteins, which are associated with skin barrier function (Kim and Leung, 2018). We found that advanced FOS increased expression of ELOVL3, which is a key enzyme for elongation of C16-C22 fatty acids (Zwara et al., 2021). It has been reported that ELOVL3-ablated mice exhibit a defect in water repulsions and increased transepidermal water loss (TEWL; Westerberg et al., 2004). Additionally, we demonstrated that advanced FOS upregulated the gene expression of FLG, a key epidermal barrier protein maintaining skin barrier function (Irvine et al., 2011; Kim and Leung, 2018). Advanced FOS may improve AD symptoms by upregulating ELOVL3 and FLG, which play important roles in skin barrier function, despite the absence of significant alterations in TEWL following administration of advanced FOS (data not shown). However, our study did not detect notable alterations in TEWL following administration of advanced FOS, suggesting that factors other than FLG and ELOVL3 contribute to TEWL changes.
Omega-O-acylceramides (AcylCERs), specific skin ceramides characterized by ultralong N-acyl chains esterified with linoleic acid, constitute 7%–12% of human SC ceramides and indispensable for the maintenance of skin barrier function (Opálka et al., 2016). Their scarcity, reduced by 15%–50% in AD patients compared to healthy individuals, significantly impacts skin barrier integrity (Janssens et al., 2012). Biosynthesis of EOS-CERs, one of the most abundant AcylCERs, is disrupted in AD keratinocytes, leading to the disorganization of formed lamellae and a disrupted skin barrier (Leung et al., 2020). Furthermore, the impact of fatty acid chain length in epidermal lipids on membrane structure and permeability emphasizes the pivotal role of disrupted skin barriers associated with irregular lipid layers in AD pathogenesis (Knox and O'Boyle, 2021). CERs containing shorter chain fatty acids rarely interact with other lipid chains, affecting barrier functions despite similar polar head groups and the ability to form hydrogen bonds with longer chain fatty acids (Ishikawa et al., 2010). In this study, proportion of EOS-CER having C28 and C30 acyl chains was significantly decreased in the FOS group, while EOS-CER with C32 acyl chain was significantly increased. However, it remains unclear how oral administration of prebiotics influences skin lipid profiles through the enzymatic pathway.
Previous studies showed an increased presence of Staphylococcus and reduced abundance of Corynebacterium and Micrococcus in AD skin compared to healthy skin (Kim et al., 2023b). Levels of long-chain unsaturated free fatty acids (FFAs) in CERs are reportedly associated with increases in the abundance of Propionibacteria and Corynebacterium in the skin of pediatric and adult AD patients, suggesting a potential interplay between SC lipids and these microbes (Baurecht et al., 2018; Kim et al., 2023b). Similarly, our study revealed changes in both SC lipid profiles and skin microbiome subsequent to the administration of advanced FOS in children with AD, although the exact sequence of these changes remains unclear. Prevotella, has been reported to exhibit a negative correlation with the severity of AD after topical corticosteroid treatment (Zheng et al., 2019). In infants with AD aged 0–1 year, the relative abundance of Rothia declined significantly with increased AD severity in the perioral skin area (Zheng et al., 2019). Our observation of an increase in Rothia abundance after 12 weeks in the FOS group suggests that kestose induces an improvement in AD by upregulating the abundance of beneficial microbes. Chng et al. reported a lower abundance of Methylobacterium in AD skin compared to healthy skin, and these bacteria showed a negative correlation with S. aureus (Chng et al., 2016; Olesen et al., 2018). In our study, Methylobacterium abundance tended to decrease in both groups after 12 weeks, with a more pronounced decrease in the control group compared to the FOS group. This reduction could be attributed to the potential impact of advanced FOS on Methylobacterium reduction, recognized as beneficial bacteria, or may be associated with S. aureus distribution, necessitating further investigation. In addition, most previous studies were based on the distribution of Lachnospiraceae in feces, and there is little research on the relationship between the distribution of Lachnospiraceae in the skin and AD. Results of animal experiments using dogs with allergies showed that the relative abundance of Lachnospiraceae on skin had a positive correlation with the percentage of CD4 + CD25 + Foxp3+ Treg cells (Rostaher et al., 2021). However, unlike the animal test results, the abundance of Lachnospiraceae was lower even when the skin condition improved after 12 weeks in our study. This suggests that the action of Lachnospiraceae may vary depending on its distribution, and that its effects differ by disease; additional research is warranted.
This study has limitations arising from the sample size and the selection of the placebo. The smaller-than-anticipated sample size may have limited our ability to detect any clinical effects of advanced FOS. Our findings revealed that maltose, which is commonly used as a placebo in randomized controlled trials assessing prebiotics, resulted in an upregulation of the expression of ELOVL3, which is a key player in skin lipid elongation, similar to kestose (Passeron et al., 2006; Shibata et al., 2009; Koga et al., 2016; Kim et al., 2021). However, in the current study, maltose increased the gene expression of ELOVL 3 like kestose, but the effect is found to be less than that of kestose. Additionally, unlike kestose, maltose reduced the gene expression of ELOVL 4, a key player in very long-chain fatty acid production (Cameron et al., 2007). This finding suggests that kestose may have greater positive effects on skin lipids compared to maltose. In the case of skin barrier proteins in the current study, maltose upregulated the gene expression of LOR in the current study, which is crosslinked to IVL, envoplakin, and periplakin scaffolds, thereby reinforcing the cornified envelope (Furue, 2020). Further investigation is necessary to elucidate determine whether maltose genuinely enhances skin barrier function. Nevertheless, advanced FOS showed had a favorable impact on alleviating itching and sleep disturbance, indicating its potential influence not only in microbial communities of the skin, but also on SC lipids responsible for maintaining skin barrier integrity.
In conclusion, the oral administration of advanced FOS demonstrated both safety and beneficial effects in itching and sleep disturbance while inducing specific alterations in the skin microbiome and epidermal lipid profiles among children with AD throughout a 12-week treatment period. This suggests that advanced FOS may be beneficial in improving skin symptoms and enhancing skin barrier function in children with AD.
The authors acknowledge that the data presented in this study must be deposited and made publicly available in an acceptable repository, prior to publication. Frontiers cannot accept a manuscript that does not adhere to our open data policies.
The studies involving humans were approved by the Institutional Review Board of Samsung Medical Center in Seoul (SMC IRB file No. 2020-11-155). The studies were conducted in accordance with the local legislation and institutional requirements. Written informed consent for participation in this study was provided by the participants’ legal guardians/next of kin.
SK: Conceptualization, Data curation, Formal analysis, Methodology, Visualization, Writing – original draft. B-GK: Formal analysis, Writing – original draft. SSa: Funding acquisition, Writing – review & editing. SP: Funding acquisition, Writing – review & editing. KR: Funding acquisition, Writing – review & editing. JL: Formal analysis, Writing – review & editing. BP: Formal analysis, Methodology, Validation, Writing – review & editing. MK: Data curation, Writing – review & editing. YK: Data curation, Writing – review & editing. JiwK: Data curation, Writing – review & editing. SSh: Data curation, Writing – review & editing. SJ: Data curation, Writing – review & editing. BK: Methodology, Writing – review & editing. JB: Formal analysis, Software, Visualization, Writing – review & editing. KA: Writing – review & editing. K-HL: Validation, Writing – original draft, Writing – review & editing. JihK: Conceptualization, Methodology, Project administration, Software, Supervision, Validation, Writing – original draft, Writing – review & editing.
The author(s) declare that financial support was received for the research, authorship, and/or publication of this article. This work was supported by Samyang Corporation, Seongnam, Korea.
The authors would like to thank Chan-Mi Moon and Younghee Chung from Multimedia Services Part, Samsung Medical Center (Seoul, Republic of Korea) for drafting the graphical abstract.
SSa, SP, and KR were employed by Samyang Corporation. JB are employed by BioEleven Co., Ltd.
The remaining authors declare that the research was conducted in the absence of any commercial or financial relationships that could be construed as a potential conflict of interest.
All claims expressed in this article are solely those of the authors and do not necessarily represent those of their affiliated organizations, or those of the publisher, the editors and the reviewers. Any product that may be evaluated in this article, or claim that may be made by its manufacturer, is not guaranteed or endorsed by the publisher.
The Supplementary material for this article can be found online at: https://www.frontiersin.org/articles/10.3389/fmicb.2024.1383779/full#supplementary-material
Ahn, K. (2023). The effect of prebiotics on atopic dermatitis. Allergy Asthma Immunol Res. 15, 271–275. doi: 10.4168/aair.2023.15.3.271
Baurecht, H., Ruhlemann, M. C., Rodriguez, E., Thielking, F., Harder, I., Erkens, A. S., et al. (2018). Epidermal lipid composition, barrier integrity, and eczematous inflammation are associated with skin microbiome configuration. J. Allergy Clin. Immunol. 141, 1668–1676.e16. doi: 10.1016/j.jaci.2018.01.019
Berdyshev, E., Goleva, E., Bronova, I., Dyjack, N., Rios, C., Jung, J., et al. (2018). Lipid abnormalities in atopic skin are driven by type 2 cytokines. JCI Insight 3:e98006. doi: 10.1172/jci.insight.98006
Cameron, D. J., Tong, Z., Yang, Z., Kaminoh, J., Kamiyah, S., Chen, H., et al. (2007). Essential role of Elovl4 in very long chain fatty acid synthesis, skin permeability barrier function, and neonatal survival. Int. J. Biol. Sci. 3, 111–119. doi: 10.7150/ijbs.3.111
Caporaso, J. G., Kuczynski, J., Stombaugh, J., Bittinger, K., Bushman, F. D., Costello, E. K., et al. (2010). QIIME allows analysis of high-throughput community sequencing data. Nat. Methods 7, 335–336. doi: 10.1038/nmeth.f.303
Chng, K. R., Tay, A. S., Li, C., Ng, A. H., Wang, J., Suri, B. K., et al. (2016). Whole metagenome profiling reveals skin microbiome-dependent susceptibility to atopic dermatitis flare. Nat. Microbiol. 1:16106. doi: 10.1038/nmicrobiol.2016.106
Chu, H., Kim, S. M., Zhang, K., Wu, Z., Lee, H., Kim, J. H., et al. (2023). Head and neck dermatitis is exacerbated by Malassezia furfur colonization, skin barrier disruption, and immune dysregulation. Front. Immunol. 14:1114321. doi: 10.3389/fimmu.2023.1114321
DeSantis, T. Z., Hugenholtz, P., Larsen, N., Rojas, M., Brodie, E. L., Keller, K., et al. (2006). Greengenes, a chimera-checked 16S rRNA gene database and workbench compatible with ARB. Appl. Environ. Microbiol. 72, 5069–5072. doi: 10.1128/AEM.03006-05
Edgar, R. C. (2010). Search and clustering orders of magnitude faster than BLAST. Bioinformatics 26, 2460–2461. doi: 10.1093/bioinformatics/btq461
European Task Force on Atopic Dermatitis (1993). Severity scoring of atopic dermatitis: the SCORAD index. Consensus report of the European task force on atopic dermatitis. Dermatology 186, 23–31. doi: 10.1159/000247298
Furue, M. (2020). Regulation of Filaggrin, Loricrin, and Involucrin by IL-4, IL-13, IL-17A, IL-22, AHR, and NRF2: pathogenic implications in atopic dermatitis. Int. J. Mol. Sci. 21:5382. doi: 10.3390/ijms21155382
Han, Y., Kim, B., Ban, J., Lee, J., Kim, B. J., Choi, B. S., et al. (2012). A randomized trial of Lactobacillus plantarum CJLP133 for the treatment of atopic dermatitis. Pediatr. Allergy Immunol. 23, 667–673. doi: 10.1111/pai.12010
Hanifin, J. M., and Rajka, G. (1980). Diagnostic features of atopic dermatitis. Acta Dermato Venereol 1980, 44–47.
Howell, M. D., Kim, B. E., Gao, P., Grant, A. V., Boguniewicz, M., DeBenedetto, A., et al. (2009). Cytokine modulation of atopic dermatitis filaggrin skin expression. J. Allergy Clin. Immunol. 124, R7–R12. doi: 10.1016/j.jaci.2009.07.012
Irvine, A. D., McLean, W. H., and Leung, D. Y. (2011). Filaggrin mutations associated with skin and allergic diseases. N. Engl. J. Med. 365, 1315–1327. doi: 10.1056/NEJMra1011040
Ishikawa, J., Narita, H., Kondo, N., Hotta, M., Takagi, Y., Masukawa, Y., et al. (2010). Changes in the ceramide profile of atopic dermatitis patients. J. Invest. Dermatol. 130, 2511–2514. doi: 10.1038/jid.2010.161
Janssens, M., van Smeden, J., Gooris, G. S., Bras, W., Portale, G., Caspers, P. J., et al. (2012). Increase in short-chain ceramides correlates with an altered lipid organization and decreased barrier function in atopic eczema patients. J. Lipid Res. 53, 2755–2766. doi: 10.1194/jlr.P030338
Jeong, K., Kim, M., Jeon, S. A., Kim, Y. H., and Lee, S. (2020). A randomized trial of Lactobacillus rhamnosus IDCC 3201 tyndallizate (RHT3201) for treating atopic dermatitis. Pediatr. Allergy Immunol. 31, 783–792. doi: 10.1111/pai.13269
Kim, J. H., Baek, J., Sa, S., Park, J., Kih, M., and Kim, W. (2021). Kestose-enriched fructo-oligosaccharide alleviates atopic dermatitis by modulating the gut microbiome and immune response. J. Funct. Foods 85:104650. doi: 10.1016/j.jff.2021.104650
Kim, S., Han, S. Y., Lee, J., Kim, N. R., Lee, B. R., Kim, H., et al. (2022). Bifidobacterium longum and galactooligosaccharide improve skin barrier dysfunction and atopic dermatitis-like skin. Allergy Asthma Immunol Res. 14, 549–564. doi: 10.4168/aair.2022.14.5.549
Kim, J., Kim, B. E., Berdyshev, E., Bronova, I., Bin, L., Bae, J., et al. (2023a). Staphylococcus aureus causes aberrant epidermal lipid composition and skin barrier dysfunction. Allergy 78, 1292–1306. doi: 10.1111/all.15640
Kim, J., Kim, B. E., Goleva, E., Berdyshev, E., Bae, J., Kim, S., et al. (2023b). Alterations of epidermal lipid profiles and skin microbiome in children with atopic dermatitis. Allergy Asthma Immunol Res. 15, 186–200. doi: 10.4168/aair.2023.15.2.186
Kim, B. E., and Leung, D. Y. M. (2018). Significance of skin barrier dysfunction in atopic dermatitis. Allergy Asthma Immunol Res. 10, 207–215. doi: 10.4168/aair.2018.10.3.207
Kim, B. K., Shon, J. C., Seo, H. S., Liu, K. H., Lee, J. W., Ahn, S. K., et al. (2022). Decrease of ceramides with long-chain fatty acids in psoriasis: possible inhibitory effect of interferon gamma on chain elongation. Exp. Dermatol. 31, 122–132. doi: 10.1111/exd.14431
Knox, S., and O'Boyle, N. M. (2021). Skin lipids in health and disease: a review. Chem. Phys. Lipids 236:105055. doi: 10.1016/j.chemphyslip.2021.105055
Koga, Y., Tokunaga, S., Nagano, J., Sato, F., Konishi, K., Tochio, T., et al. (2016). Age-associated effect of kestose on Faecalibacterium prausnitzii and symptoms in the atopic dermatitis infants. Pediatr. Res. 80, 844–851. doi: 10.1038/pr.2016.167
Langan, S. M., Irvine, A. D., and Weidinger, S. (2020). Atopic dermatitis. Lancet 396, 345–360. doi: 10.1016/S0140-6736(20)31286-1
Leung, D. Y. M., Berdyshev, E., and Goleva, E. (2020). Cutaneous barrier dysfunction in allergic diseases. J. Allergy Clin. Immunol. 145, 1485–1497. doi: 10.1016/j.jaci.2020.02.021
Olesen, C. M., Clausen, M. L., Andersen, P. S., and Agner, T. (2018). The skin microbiome in atopic dermatitis—a potential treatment target? Curr. Derm. Rep. 7, 199–208. doi: 10.1007/s13671-018-0245-6
Opálka, L., Kováčik, A., Maixner, J., and Vávrová, K. (2016). Omega-O-Acylceramides in skin lipid membranes: effects of concentration, Sphingoid Base, and model complexity on microstructure and permeability. Langmuir 32, 12894–12904. doi: 10.1021/acs.langmuir.6b03082
Passeron, T., Lacour, J. P., Fontas, E., and Ortonne, J. P. (2006). Prebiotics and synbiotics: two promising approaches for the treatment of atopic dermatitis in children above 2 years. Allergy 61, 431–437. doi: 10.1111/j.1398-9995.2005.00956.x
Rostaher, A., Rodriguez-Campos, S., Deplazes, P., Zwickl, L., Akdis, A. C., Urwyler, A., et al. (2021). Atopic dermatitis in West Highland white terriers—part III: early life peripheral blood regulatory T cells are reduced in atopic dermatitis. Vet. Dermatol. 32, 239–e63. doi: 10.1111/vde.12939
Shibata, R., Kimura, M., Takahashi, H., Mikami, K., Aiba, Y., Takeda, H., et al. (2009). Clinical effects of kestose, a prebiotic oligosaccharide, on the treatment of atopic dermatitis in infants. Clin. Exp. Allergy 39, 1397–1403. doi: 10.1111/j.1365-2222.2009.03295.x
Ständer, S., Yosipovitch, G., Bushmakin, A. G., Cappelleri, J. C., Luger, T., Tom, W. L., et al. (2019). Examining the association between pruritus and quality of life in patients with atopic dermatitis treated with crisaborole. J. Eur. Acad. Dermatol. Venereol. 33, 1742–1746. doi: 10.1111/jdv.15712
Suzuki, M., Ohno, Y., and Kihara, A. (2022). Whole picture of human stratum corneum ceramides, including the chain-length diversity of long-chain bases. J. Lipid Res. 63:100235. doi: 10.1016/j.jlr.2022.100235
Tochio, T., Kadota, Y., Tanaka, T., and Koga, Y. (2018). 1-Kestose, the smallest Fructooligosaccharide component, which efficiently stimulates Faecalibacterium prausnitzii as well as Bifidobacteria in humans. Food Secur. 7:140. doi: 10.3390/foods7090140
van den Bogaard, E. H., Elias, P. M., Goleva, E., Berdyshev, E., Smits, J. P. H., Danby, S. G., et al. (2023). Targeting skin barrier function in atopic dermatitis. J. Allergy Clin. Immunol. Pract. 11, 1335–1346. doi: 10.1016/j.jaip.2023.02.005
Westerberg, R., Tvrdik, P., Undén, A. B., Månsson, J. E., Norlén, L., Jakobsson, A., et al. (2004). Role for ELOVL3 and fatty acid chain length in development of hair and skin function. J. Biol. Chem. 279, 5621–5629. doi: 10.1074/jbc.M310529200
Yoon, S. H., Ha, S. M., Kwon, S., Lim, J., Kim, Y., Seo, H., et al. (2017). Introducing EzBioCloud: a taxonomically united database of 16S rRNA gene sequences and whole-genome assemblies. Int. J. Syst. Evol. Microbiol. 67, 1613–1617. doi: 10.1099/ijsem.0.001755
Zheng, Y., Wang, Q., Ma, L., Chen, Y., Gao, Y., Zhang, G., et al. (2019). Alterations in the skin microbiome are associated with disease severity and treatment in the perioral zone of the skin of infants with atopic dermatitis. Eur. J. Clin. Microbiol. Infect. Dis. 38, 1677–1685. doi: 10.1007/s10096-019-03598-9
Keywords: atopic dermatitis, fatty acid elongase, fructo-oligosaccharides, kestose, prebiotics
Citation: Kim S, Kang B-G, Sa S, Park SY, Ryu K, Lee J, Park B, Kwon M, Kim Y, Kim J, Shin S, Jang S, Kim BE, Bae J, Ahn K, Liu K-H and Kim J (2024) Advanced fructo-oligosaccharides improve itching and aberrant epidermal lipid composition in children with atopic dermatitis. Front. Microbiol. 15:1383779. doi: 10.3389/fmicb.2024.1383779
Received: 08 February 2024; Accepted: 09 April 2024;
Published: 29 April 2024.
Edited by:
Antonios Koutelidakis, University of the Aegean, GreeceReviewed by:
Lily Mijouin, Shiseido Europe Innovation Center, FranceCopyright © 2024 Kim, Kang, Sa, Park, Ryu, Lee, Park, Kwon, Kim, Kim, Shin, Jang, Kim, Bae, Ahn, Liu and Kim. This is an open-access article distributed under the terms of the Creative Commons Attribution License (CC BY). The use, distribution or reproduction in other forums is permitted, provided the original author(s) and the copyright owner(s) are credited and that the original publication in this journal is cited, in accordance with accepted academic practice. No use, distribution or reproduction is permitted which does not comply with these terms.
*Correspondence: Kwang-Hyeon Liu, ZHN0bGtoQGdtYWlsLmNvbQ==; Jihyun Kim, amhsb3ZlY2hpbGRAZ21haWwuY29t
†These authors have contributed equally to this work and share senior authorship
Disclaimer: All claims expressed in this article are solely those of the authors and do not necessarily represent those of their affiliated organizations, or those of the publisher, the editors and the reviewers. Any product that may be evaluated in this article or claim that may be made by its manufacturer is not guaranteed or endorsed by the publisher.
Research integrity at Frontiers
Learn more about the work of our research integrity team to safeguard the quality of each article we publish.