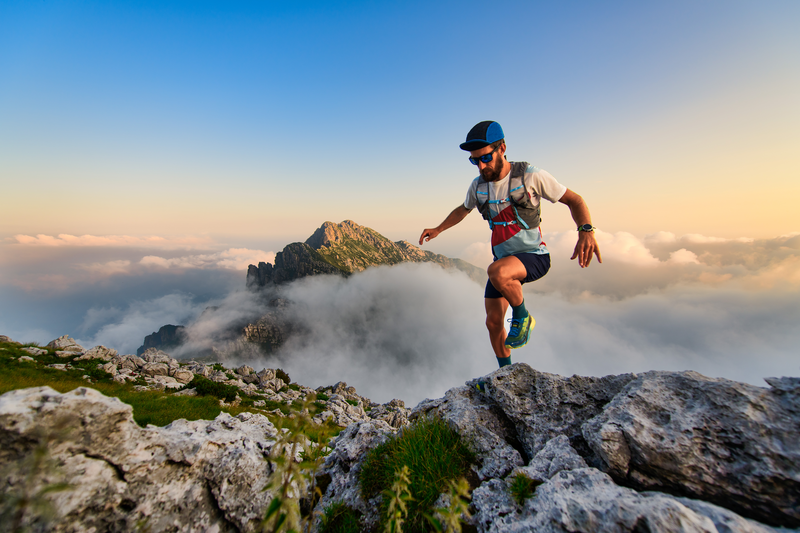
94% of researchers rate our articles as excellent or good
Learn more about the work of our research integrity team to safeguard the quality of each article we publish.
Find out more
ORIGINAL RESEARCH article
Front. Microbiol. , 02 May 2024
Sec. Virology
Volume 15 - 2024 | https://doi.org/10.3389/fmicb.2024.1373601
Introduction: There are three major categories of waterfowl parvoviruses, namely goose parvovirus (GPV), Muscovy duck parvovirus, and novel goose parvovirus (NGPV). NGPV can infect both Cherry Valley ducks and mule ducks, resulting in short beaks and dwarfism syndrome, and the incidence of short beaks and dwarfism syndrome rises annually, posing a significant threat to the waterfowl breeding and the animal husbandry. Therefore, clarifying the biological characteristics and genetic evolution of NGPV is very important for the prevention and control of NGPV.
Methods: Ducks with short beaks and dwarfism syndrome from Shandong and Henan Province were investigated by dissection and the tissue samples were collected for study. The NGPV genome was amplified by PCR, and the genome was analyzed for genetic evolution.
Results: Eight strains of NGPV were isolated, which were designated as HZ0512, HZ0527, HZ0714, HZ0723, HZ0726, HZ0811, HZ0815, and HN0403. The nucleotide homology among these strains ranged from 99.9% to 100%. The eight strains, along with other NGPVs, belong to GPV. The eight strains showed a 92.5%–98.9% nucleotide homology with the classical GPV, while a 96.0%–99.9% homology with NGPV.Therefore, it can be deduced that there have been no major mutations of NGPV in Shandong and Henan provinces in recent years.
Discussion: This study lays a theoretical foundation for further studying the genetic evolution and pathogenicity of NGPV, thereby facilitating the prevention and control of NGPV.
Waterfowl parvoviruses comprise Muscovy duck parvovirus (MDPV), goose parvovirus (GPV), and novel goose parvovirus (NGPV). These viruses exhibit similar morphological structures and physical characteristics, including a 5-kb linear genome and a non-enveloped 20-sided capsid with a diameter of 20–22 nm. The viral genome consists of two major open reading frames (ORFs) responsible for encoding nonstructural (NS) proteins and capsid proteins (VP). Notably, capsid proteins play a crucial role in determination of pathogenicity and host range (Tu et al., 2015). In addition, VP proteins can be divided into VP1, VP2, and VP3, among which VP3 is the most expressed and tunable protein among capsid proteins, and can induce antibody neutralization and provide protective immunity for waterfowl (Lian et al., 2020). The peptide chain length of VP1 is longer than that of VP2 and VP3, and the entire amino acid sequences of VP2 and VP3 are encompassed in the carboxyl terminal part of VP1 (Tsai et al., 2004).
Two major outbreaks of novel goose parvovirus were reported in Taiwan, China during 1982–1989 (Li et al., 2021). In 2015, an outbreak of “short beak and dwarfism syndrome” (SBDS), which is characterized by symptoms such as short beaks, exposed tongues, stunted growth, weak bones, and diarrhea, was reported in Cherry Valley duck flocks in China. This syndrome, first reported in the 1900s, was also observed in French mule ducks in the 1970s (Palya et al., 2009; Fu et al., 2017). The disease has an incidence rate of 10–30% and a mortality rate of 2–6%, posing a significant threat to waterfowl breeding in China (Li et al., 2018). In recent years, 53% of NGPV cases were found in Shandong, 19% in Anhui, 6% in Henan, 16% in Jiangsu, and 6% in other provinces of China (Figure 1). NGPV has been identified as the primary pathogen of this disease. NGPV is a newly discovered waterfowl parvovirus with significant differences from GPV and MDPV. GPV can infect geese and Muscovy ducks, leading to a condition known as Derzsy’s disease, and MDPV primarily infects Muscovy ducks (Glávits et al., 2005; Ji et al., 2010). Nevertheless, NGPV can infect both Cherry Valley ducks and mule ducks, resulting in SBDS. To make it worse, NGPV can co-infect with duck circovirus and duck hepatitis virus.
To clarify the biological characteristics of NGPV and its genetic evolution in specific regions of China, we isolated the strains and identified the pathogen and sequence the genomes of eight diseased Cherry Valley broiler ducks with typical SBDS symptoms. These ducks were obtained from Shandong and Henan Province. We conducted a comparative analysis of the sequences and phylogenetic development involving 47 reference strains, which allowed the elucidation of the genetic variability of NGPV in Shandong and Henan and offered valuable insights for the control and prevention of SBDS. Additionally, this study lays a foundation for further studying the genetic evolution and pathogenicity of NGPV.
Eight groups of 28-day-old Cherry Valley ducks from Shandong and Henan with typical SBDS symptoms, including ruffled feathers, stunted growth, shortened beaks, swollen tongues, and diarrhea, were enrolled. Dissection revealed swollen tongues, kidney hemorrhaging, and enlarged gallbladders in some of the sick ducks, while no significant lesions were observed in other organs. Laboratory primers were used for avian influenza virus, novel duck reovirus, Duck Tembusu virus, duck circovirus, duck astrovirus, and NGPV identification in PCR, wherein all samples were tested positive for NGPV, while the other viruses yielded negative results. The sequences of primers used for identification are shown in Table 1. These groups were denoted as HZ0512, HZ0527, HZ0714, HZ0723, HZ0726, HZ0811, HZ0815, and HN0403, respectively. Liver and spleen tissue samples were collected and stored at −80°C.
The preserved tissue samples were diluted and homogenized with 0.9% NaCl, followed by centrifugation at 12,000 rpm for 60 s at room temperature. The supernatant was collected. Additionally, viral genomic DNA extraction was conducted using the DNA extraction kit (Bio Flux, Hangzhou, China).
Five pairs of primers were designed to amplify the NGPV gene based on the primers described in the article and the NGPV genome sequence available in GenBank (Ge et al., 2017). The primer sequences are shown in Table 2.
The gene sequence of NGPV was amplified using the Taq PCR Master Mix Kit. For each reaction, 2 μL of extracted DNA, 10 μL of the Mix, 6 μL of H2O, and 1 μL of upstream and downstream primers each were added. For DNA amplification, denaturation at 95°C for 4 min was conducted, followed by denaturation at 95°C for 15 s, annealing at 57°C for 15 s, and extension at 72°C for 15 s; the extension was repeated for a total of 35 times, followed by a final extension at 72°C for 5 min. Subsequently, 10 μL of the PCR product was subjected to 1% agarose gel electrophoresis. The positive PCR products were then extracted from the gel and sent to Beijing Prime Biotechnology Co., Ltd. for sequencing. The sequencing results were analyzed by DNA Star (Version 7.1.0, Madison, United States).
Nucleotide and amino acid sequences were aligned using the Clustal W method in Megalin 7.1.0 (DNAStar Inc., Madison, United States). MEGA7 was employed to compare the gene sequences of the eight NGPV strains with the reference strains. Phylogenetic trees were developed using the Neighbor-joining method based on the aligned nucleotide sequences of NGPV strains. A total of 1,000 bootstrap replicates were involved. Sopma1 was employed to analyze the secondary structures of NS and VP proteins, and the Swiss-Model2 was employed to develop a model of the tertiary structure of VP proteins. The gene sequences used in this study are presented in Table 3.
PCR was performed on the sample ducks using the laboratory preserved Avian influenza virus, Novel duck reovirus, Duck Tembusu virus, duck circovirus, duck astrovirus, and NGPV identification primers. The results showed that only NGPV identification primers led to amplified positive bands (Figure 2). The detection rate of NGPV was 25%, among which HZ0403 was detected in ducks from Henan and other strains were detected in ducks from Shandong.
Figure 2. Results of NGPV identification. M: DL 2000 DNA maker, 1–8: Results of NGPV identification (661 bp).
The gene sequences of the eight NGPV strains isolated in this study encompassed the complete coding sequences (CDs) of two major ORFs, which encode NS and VP proteins. The NS genes had a total length of 1884 bp and are responsible for encoding 627 amino acids, while the VP genes had a total length of 2,199 bp and are responsible for encoding 732 amino acids. The eight NGPV strains isolated in this study showed a nucleotide identity of 99.9–100% and an amino acid identity of 99.5–99.9%.
The nucleotide homology of the eight NGPV strains with the MDPV reference strains, GPV nucleotides, and NGPV were 80.8–85.3%, 92.5–98.9% and 96.0–99.9%, respectively.
The results of the genome-wide phylogenetic tree analysis (Figure 3) demonstrated that waterfowl parvovirus strains can be categorized into GPV and MDPV. All NGPV strains consisted of a sub-group of the GPV group, and the eight strains isolated belonged to the NGPV group. Notably, they were the most closely related to the HN1P strain isolated from ducks from Henan.
Figure 3. Phylogenetic tree of NGPV strains obtained based on the genome nucleotide sequences. Numbers at nodes indicate bootstrap percentages obtained after 1,000 replicates. Genotypes are indicated on the right side of the trees.
The amino acid sequences of the NS proteins of the eight viruses were determined based on the nucleotide sequences of the NS gene. A phylogenetic tree was established using the amino acid sequences of these proteins and 30 reference strains (Figure 4A). Notably, the amino acid sequences of the NS proteins of the eight viruses were clustered together within the same branch as the NGPV HN1P strain. Compared with the classical GPV vaccine strain, the NS proteins of the isolated strains had 19 common mutation sites, as shown in Table 4.
Figure 4. Phylogenetic tree of NS complete amino acid gene (A), phylogenetic tree of VP complete gene amino acid (B). Numbers at nodes indicate bootstrap percentages obtained after 1,000 replicates. Genotypes are indicated on the right side of the trees.
The homology analysis revealed that the amino acid sequences of the NS proteins in the isolates shared 89.2–89.6%, 96.8–97.3%, and 96.7–100% similarities to those of MDPV, GPV and NGPV, respectively. Within the eight NGPV isolates, the homology of amino acids of the NS proteins ranged from 99.7 to 100%. Due to the high genomic similarity of NGPV isolates, the NS and VP proteins of the eight NGPV isolates showed negligible differences in secondary structure. The results of Sopma showed that the NS protein was composed of helix (39.71%), sheet (12.76%), turn (3.99%) and coil (43.54%) (Figure 5A).
Figure 5. Secondary structures of NS protein (A) and VP protein (B) predicted by the Sopma. Red indicates sheet, blue indicates helix, green indicates turn and purple indicates coil.
The amino acid sequences of the VP proteins of the eight viruses were determined based on the nucleotide sequence of the VP gene. Then, a phylogenetic tree was established by aligning these amino acid sequences based on the 30 reference sequences (Figure 4B). All isolates were within the same branch as the NGPV HN1P strain, and HZ0815, HZ0726, and HZ0723 were clustered in the same branch as the HN1P strain. Moreover, the eight isolates were not in the same branch with the classic vaccine strain VG32/1 (EU583392). A comparison of the VP proteins of the isolates and the classical GPV vaccine strains revealed 12 common mutation sites, seven of which were in the VP3 region (198–732 aa), as shown in Table 5. Additionally, nucleotide sequences of VP3 of the isolates and 7 reference strains were compared, and phylogenetic trees were established. The results showed that all isolates were only in the same branch as HN1P though their homology exceeded 98.7% (Figure 6).
Figure 6. Phylogenetic tree of VP3 complete nucleotide sequence. Numbers at nodes indicate bootstrap percentages obtained after 1,000 replicates. Genotypes are indicated on the right side of the trees.
The homology of amino acid sequences of the VP proteins of the isolates ranged from 99.7 to 100%. The isolates showed 87.3–90.8%, 94.8–98.2%, and 97.1–99.9% homologies to the MDPV, GPV, and NGPV reference strains, respectively. Determination of the secondary structure by Sopma demonstrated that the NS protein was composed of helix (13.39%), sheet (15.98%), turn (2.32%) and coil (68.31%) (Figure 5B). Swiss-Model was used for template searching and model establishment. As indicated, the VP protein of HZ0512 had the highest similarity to Hum8 capsid (59.84%), while 1-214aa had a low similarity to Hum8 capsid, and its tertiary structure could not be determined in this case. The 215–732 aa model was developed with 7ltm as the template (Figure 7).
Figure 7. Model establishment by the Swiss Model. The VP protein of HZ0512 appears as a surface form.
Waterfowl parvovirus is a highly contagious and fatal pathogen affecting goslings and ducklings. It belongs to the species of Anseriform dependoparvovirus 1, the genus of Dependoparvovirus. Waterfowl parvovirus is classified as a vesiculovirus. It has a small icosahedral capsid assembled from 32 capsids, with a diameter of 20–22 nm; it is typically characterized by a single-stranded DNA genome. Its full-length genome contains two open reading frames: the left encoding the non-structural (NS) proteins (NS1 and NS2), and the right encoding the structural proteins (VP1, VP2, and VP3). SBDS was first reported in mule ducks in France during the 1970s. It has also been found in various regions of China, including Shandong, Henan, Guangxi, and Sichuan (Matczuk et al., 2020). NGPV has been confirmed as the causative agent of SBDS (Matczuk et al., 2020). This virus can be transmitted both horizontally and vertically and can co-infect ducks with duck circovirus and duck hepatitis virus, resulting in the feather shedding syndrome (Chen et al., 2015; Matczuk et al., 2020; Yang et al., 2020). Moreover, goose parvovirus is also involved in the angel wing syndrome in Muscovy ducks, and the infected ducks would exhibit SBDS and angel wing symptoms (Sallam et al., 2022). In addition, the influence of NGPV on Cherry Valley ducks has been explored from the perspective of intestinal flora, and it has been found that NGPV could cause an imbalance of intestinal flora, accompanied by increases in cecal short-chain fatty acids (Chen et al., 2021).
Ducks with SBDS exhibit various symptoms, including short bill, protruding tongue, weak bones, and growth retardation. This condition has a prevalence of up to 30% in duck flocks, resulting in a mortality rate of 2–6%. Despite that the mortality is not high, a significant number of infected ducks experience severe growth retardation, resulting in a culling rate of about 80% (Chen et al., 2015). Consequently, SBDS poses a substantial threat to waterfowl breeding in China.
This study investigated eight NGPV strains isolated from Shandong and Henan. These strains contain two major ORFs: encoding VP proteins and encoding NS proteins. These isolates had a nucleotide homology of 100% with each other. Phylogenetic analysis based on reference strains demonstrated that the eight isolates belong to the NGPV, which is consistent with the results of the homology analysis. Additionally, these NGPV isolates were closely related to HN1P, a strain isolated from ducks in Henan, China, with a nucleotide homology of 99.9%.
The NS protein plays a crucial role in viral replication, gene expression, and apoptosis. It facilitates essential activities such as DNA replication and transcription (Brown et al., 1995). The nucleotide sequences encoding the NS protein in the eight isolates were found to have 1884 base pairs, which is consistent with previous studies. Phylogenetic analysis of the amino acid sequences of the NS proteins indicated that these isolates developed a significant branch with the NGPV reference strain. Homology analysis showed a homology of 96.8–97.3% and 96.7–100% with GPV and NGPV, respectively. Notably, the homology of the eight isolates and classical GPV and NGPV had negligible differences, making recombination events possible in our isolates. Compared with classical GPV, 19 common mutation sites were found in NS proteins of the eight isolates, among which 140aa (A-S), 159aa (L-V), and 350aa (A-V) were located near motifs associated with viral transcription (Shien et al., 2008). Previous studies have shown that the epitope of GPV NS protein is mainly located in the C-terminal (485–627aa) (Li et al., 2018). Compared with classical GPV, nine amino acid mutations were found at the C-terminal of the NS protein in NGPV. Hence, it can be speculated that the short beak symptoms and “angel wing” symptoms of ducks may be related to mutations in NS proteins.
VP proteins are involved in the synthesis of viral particle capsid proteins, assembly, and release of progeny viruses, and serve as ligands that bind to receptors on the surface of host cells, which is a critical factor in the viral infection process (Tu et al., 2015). About 80% of the VP proteins are VP3, which is the main capsid protein and protective antigen of the virus (Shen et al., 2015; Li et al., 2016). VP3 can induce antibody neutralization and provide immunity towards other waterfowl parvoviruses. It is also a prime candidate antigen for vaccine development and serum diagnostic testing. It has been demonstrated that virus-like particles based on NGPV VP2 can protect ducks from NGPV (Shang et al., 2023). The homology between the eight isolated NGPV and the reference MDPV was 87.3–90.8%; the homology between GPV and GPV was 94.8–98.2%; and the homology between NGPV and NGPV was 97.1–99.9%. The amino acid sequences of VP proteins in the isolates had a 99.9% homology with the NGPV reference strain. Therefore, it can be speculated that VP proteins are exposed to negligible variations in order to guarantee the stability of viral infection. Indeed, amino acids located at the receptor binding site of VP proteins play an important role in the host range and pathogenicity of viruses (Tu et al., 2015). Compared with classical GPV, 12 common mutation sites of VP proteins were found in the isolates, and the change of VP protein in NGPV may be related to host transfer. The seven linear immunodominant epitope fragments of GPV included 35–71aa, 123–198aa, 423–444aa, 474–491aa, 531–566aa, and 616–669aa (Yu et al., 2012). Four out of the 12 amino acid mutations in NGPV are present in these epitopes, and these mutation sites may affect the immunogenicity of NGPV.
Currently, isolation of NGPV from tissues of infected ducks remains challenging, while the incidence of SBDS rises annually, posing a significant threat to the waterfowl breeding and the animal husbandry. Given the widespread prevalence of NGPV, addressing this issue requires a two-fold approach. First, feeding management practices shall be enhanced and standards of environmental sanitation and disinfection shall be strictly followed. Second, development of NGPV vaccines shall be accelerated. While classical GPV vaccines have been reported to offer partial prevention against NGPV, they are at risk of becoming ineffective due to virus mutations. Our results indicated that the eight NGPV isolates have relatively low mutation levels, suggesting a relatively stable outbreak.
The datasets presented in this study can be found in online repositories. The names of the repository/repositories and accession number(s) can be found in the article/supplementary material.
The animal study was approved by Shandong Agricultural University Animal Care and Use Committee. The study was conducted in accordance with the local legislation and institutional requirements.
GZ: Formal analysis, Investigation, Methodology, Writing – original draft. NL: Data curation, Formal analysis, Software, Validation, Writing – original draft. XF: Data curation, Investigation, Writing – review & editing. WJ: Data curation, Investigation, Writing – review & editing. MY: Investigation, Validation, Visualization, Writing – review & editing. YL: Investigation, Validation, Visualization, Writing – review & editing. SD: Methodology, Project administration, Supervision, Writing – review & editing.
The author(s) declare financial support was received for the research, authorship, and/or publication of this article. This research was funded by Shandong Province Science and Technology Achievement Transfer and Transformation Subsidy (Shandong Chongqing Science and Technology Cooperation) Project (2022LYXZ009).
We appreciate the help of other students in the lab and the reviewers.
GZ was employed by Xintai Jianyuan Breeding Poultry Limited Company, Jiangsu Yike Food Group Limited Company.
The remaining authors declare that the research was conducted in the absence of any commercial or financial relationships that could be construed as a potential conflict of interest.
All claims expressed in this article are solely those of the authors and do not necessarily represent those of their affiliated organizations, or those of the publisher, the editors and the reviewers. Any product that may be evaluated in this article, or claim that may be made by its manufacturer, is not guaranteed or endorsed by the publisher.
Brown, K. E., Green, S. W., and Young, N. S. (1995). Goose parvovirus—an autonomous member of the Dependovirus genus? Virology 210, 283–291. doi: 10.1006/viro.1995.1345
Chen, Y., Afumba, R., Pang, F., Yuan, R., and Dong, H. (2021). Advances in research on genetic relationships of waterfowl parvoviruses. J. Vet. Res. 65, 391–399. doi: 10.2478/jvetres-2021-0063
Chen, H., Dou, Y., Tang, Y., Zhang, Z., Zheng, X., Niu, X., et al. (2015). Isolation and genomic characterization of a duck-origin GPV-related parvovirus from Cherry Valley ducklings in China. PLoS One 10:e0140284. doi: 10.1371/journal.pone.0140284
Fu, Q., Huang, Y., Wan, C., Fu, G., Qi, B., Cheng, L., et al. (2017). Genomic and pathogenic analysis of a Muscovy duck parvovirus strain causing short beak and dwarfism syndrome without tongue protrusion. Res. Vet. Sci. 115, 393–400. doi: 10.1016/j.rvsc.2017.07.006
Ge, Z., Liu, Y., Chen, S., Zhu, D., Liu, M., Wang, M., et al. (2017). Amplification and phylogenetic analysis of novel goose parvovirus Sichuan strain. J. Sichuan Agric. Univ. 35, 574–580. doi: 10.16036/j.issn
Glávits, R., Zolnai, A., Szabó, E., Ivanics, E., Zarka, P., Mató, T., et al. (2005). Comparative pathological studies on domestic geese (Anser anser domestica) and Muscovy ducks (Cairina moschata) experimentally infected with parvovirus strains of goose and Muscovy duck origin. Acta Vet. Hung. 53, 73–89. doi: 10.1556/avet.53.2005.1.8
Ji, J., Xie, Q. M., Chen, C. Y., Bai, S. W., Zou, L. S., Zuo, K. J., et al. (2010). Molecular detection of Muscovy duck parvovirus by loop-mediated isothermal amplification assay. Poult. Sci. 89, 477–483. doi: 10.3382/ps.2009-00527
Li, K. P., Hsu, Y. C., Lin, C. A., Chang, P. C., Shien, J. H., Liu, H. Y., et al. (2021). Molecular characterization and pathogenicity of the novel recombinant Muscovy duck parvovirus isolated from geese. Animals (Basel) 11:3211. doi: 10.3390/ani11113211
Li, P., Lin, S., Zhang, R., Chen, J., Sun, D., Lan, J., et al. (2018). Isolation and characterization of novel goose parvovirus-related virus reveal the evolution of waterfowl parvovirus. Transbound. Emerg. Dis. 65, e284–e295. doi: 10.1111/tbed.12751
Li, C., Liu, H., Li, J., Liu, D., Meng, R., Zhang, Q., et al. (2016). A conserved epitope mapped with a monoclonal antibody against the VP3 protein of goose parvovirus by using peptide screening and phage display approaches. PLoS One 11:e0147361. doi: 10.1371/journal.pone.0147361
Lian, C., Zhang, R., Lan, J., Yang, Y., Li, H., Sui, N., et al. (2020). Identification of a common conserved neutralizing linear B-cell epitope in the VP3 protein of waterfowl parvoviruses. Avian Pathol. 49, 325–334. doi: 10.1080/03079457.2020.1746743
Matczuk, A. K., Chmielewska-Władyka, M., Siedlecka, M., Bednarek, K. J., and Wieliczko, A. (2020). Short beak and dwarfism syndrome in ducks in Poland caused by novel goose parvovirus. Animals (Basel) 10:2397. doi: 10.3390/ani10122397
Palya, V., Zolnai, A., Benyeda, Z., Kovács, E., Kardi, V., and Mató, T. (2009). Short beak and dwarfism syndrome of mule duck is caused by a distinct lineage of goose parvovirus. Avian Pathol. 38, 175–180. doi: 10.1080/03079450902737839
Sallam, H. M., Halim Nour, A. A., and Zanaty, A. M. (2022). Involvement of goose parvovirus in induction of angel wing syndrome in Muscovy ducks. Avian Dis. 66, 373–380. doi: 10.1637/aviandiseases-D-22-00014
Shang, Y., Ma, Y., Tang, S., Chen, X., Feng, H., Li, L., et al. (2023). Virus-like particles based on the novel goose parvovirus (NGPV) VP2 protein protect ducks against NGPV challenge. Vaccines (Basel) 11:1768. doi: 10.3390/vaccines11121768
Shen, H., Zhang, W., Wang, H., Zhou, Y., and Shao, S. (2015). Identification of recombination between Muscovy duck parvovirus and goose parvovirus structural protein genes. Arch. Virol. 160, 2617–2621. doi: 10.1007/s00705-015-2541-9
Shien, J. H., Wang, Y. S., Chen, C. H., Shieh, H. K., Hu, C. C., and Chang, P. C. (2008). Identification of sequence changes in live attenuated goose parvovirus vaccine strains developed in Asia and Europe. Avian Pathol. 37, 499–505. doi: 10.1080/03079450802356979
Tsai, H. J., Tseng, C. H., Chang, P. C., Mei, K., and Wang, S. C. (2004). Genetic variation of viral protein 1 genes of field strains of waterfowl parvoviruses and their attenuated derivatives. Avian Dis. 48, 512–521. doi: 10.1637/7172
Tu, M., Liu, F., Chen, S., Wang, M., and Cheng, A. (2015). Role of capsid proteins in parvoviruses infection. Virol. J. 12:114. doi: 10.1186/s12985-015-0344-y
Yang, Y., Sui, N., Zhang, R., Lan, J., Li, P., Lian, C., et al. (2020). Coinfection of novel goose parvovirus-associated virus and duck circovirus in feather sacs of Cherry Valley ducks with feather shedding syndrome. Poult. Sci. 99, 4227–4234. doi: 10.1016/j.psj.2020.05.013
Keywords: Cherry Valley duck, novel goose parvovirus, short beak and dwarfism syndrome, mutation site, phylogenetic analysis
Citation: Zhan G, Liu N, Fan X, Jiang W, Yuan M, Liu Y and Dong S (2024) Genome cloning and genetic evolution analysis of eight duck-sourced novel goose parvovirus strains in China in 2023. Front. Microbiol. 15:1373601. doi: 10.3389/fmicb.2024.1373601
Received: 20 January 2024; Accepted: 03 April 2024;
Published: 02 May 2024.
Edited by:
Junki Maruyama, The University of Texas Medical Branch at Galveston, United StatesReviewed by:
Huapeng Feng, Zhejiang Sci-Tech University, ChinaCopyright © 2024 Zhan, Liu, Fan, Jiang, Yuan, Liu and Dong. This is an open-access article distributed under the terms of the Creative Commons Attribution License (CC BY). The use, distribution or reproduction in other forums is permitted, provided the original author(s) and the copyright owner(s) are credited and that the original publication in this journal is cited, in accordance with accepted academic practice. No use, distribution or reproduction is permitted which does not comply with these terms.
*Correspondence: Shishan Dong, ZG9uZ3NoaXNoYW5AMTYzLmNvbQ==
†These authors have contributed equally to this work
Disclaimer: All claims expressed in this article are solely those of the authors and do not necessarily represent those of their affiliated organizations, or those of the publisher, the editors and the reviewers. Any product that may be evaluated in this article or claim that may be made by its manufacturer is not guaranteed or endorsed by the publisher.
Research integrity at Frontiers
Learn more about the work of our research integrity team to safeguard the quality of each article we publish.