- 1Research Institute of Tissue Engineering and Stem Cells, Nanchong Central Hospital, The Second Clinical College of North Sichuan Medical College, Nanchong, Sichuan, China
- 2Department of Burns and Plastic Surgery, West China Hospital, Sichuan University, Chengdu, Sichuan, China
- 3Department of Dermatology, West China Hospital, Sichuan University, Chengdu, Sichuan, China
Background: Quorum sensing (QS) research stands as a pivotal and multifaceted domain within microbiology, holding profound implications across various scientific disciplines. This bibliometric analysis seeks to offer an extensive overview of QS research, covering the period from 2004 to 2023. It aims to elucidate the hotspots, trends, and the evolving dynamics within this research domain.
Methods: We conducted an exhaustive review of the literature, employing meticulous data curation from the Science Citation Index Extension (SCI-E) within the Web of Science (WOS) database. Subsequently, our survey delves into evolving publication trends, the constellation of influential authors and institutions, key journals shaping the discourse, global collaborative networks, and thematic hotspots that define the QS research field.
Results: The findings demonstrate a consistent and growing interest in QS research throughout the years, encompassing a substantial dataset of 4,849 analyzed articles. Journals such as Frontiers in Microbiology have emerged as significant contributor to the QS literature, highlighting the increasing recognition of QS's importance across various research fields. Influential research in the realm of QS often centers on microbial communication, biofilm formation, and the development of QS inhibitors. Notably, leading countries engaged in QS research include the United States, China, and India. Moreover, the analysis identifies research focal points spanning diverse domains, including pharmacological properties, genetics and metabolic pathways, as well as physiological and signal transduction mechanisms, reaffirming the multidisciplinary character of QS research.
Conclusion: This bibliometric exploration provides a panoramic overview of the current state of QS research. The data portrays a consistent trend of expansion and advancement within this domain, signaling numerous prospects for forthcoming research and development. Scholars and stakeholders engaged in the QS field can harness these findings to navigate the evolving terrain with precision and speed, thereby enhancing our comprehension and utilization of QS in various scientific and clinical domains.
Introduction
Quorum sensing (QS) is a captivating and essential cellular communication process that transcends species boundaries, involving the exchange of signaling molecules among diverse microbial communities (Grandclément et al., 2016; Prescott and Decho, 2020). It has emerged as a pivotal area of research since its discovery in the marine luminous bacterium V. fischeri in the 1970's (Arnold et al., 1972). This groundbreaking finding has propelled QS into a wide array of applications, spanning from medical therapeutics targeting bacterial virulence to environmental strategies for pollutant degradation. This phenomenon serves as the cornerstone of intricate intercellular dialogues that underpin collective behaviors within bacterial populations (Eickhoff and Bassler, 2018; Subramani and Jayaprakashvel, 2019; Wu et al., 2022). At its core, QS enables bacteria to gauge their population density and respond collectively to changing environmental conditions (Cornforth et al., 2014; Popat et al., 2015). This dynamic process has far-reaching implications across a spectrum of fields, from the formation of biofilms to the development of QS inhibitors.
Biofilms, structured communities of bacteria embedded in self-produced extracellular matrices, represent a paradigmatic outcome of QS in action (Irie and Parsek, 2008; Steinberg and Kolodkin-Gal, 2015). Bacteria coordinate their activities within biofilms, enhancing their resistance to external stresses, antibiotics, and immune responses (Fisher et al., 2017; Sharma et al., 2019). Understanding the mechanisms underlying biofilm formation is a central focus of QS research (Li et al., 2022), as it carries significant implications for infection control. Furthermore, the development of QS inhibitors has emerged as a promising strategy to disrupt bacterial communication and attenuate the virulence of pathogenic species (Tay and Yew, 2013; Scutera et al., 2014).
In the realm of practical applications, QS research spans across diverse domains, including medicine, agriculture, food science, and sewage treatment (Annous et al., 2009; Sharma and Jangid, 2014; Huang et al., 2016). In medicine, it has profound implications for combating bacterial infections, particularly those involving multidrug-resistant strains (Kiran et al., 2011; Alibi et al., 2020). Agriculture benefits from insights into plant-microbe interactions and the potential for environmentally friendly microorganism management (Berg, 2009). In food science, QS impacts food safety and preservation (Skandamis and Nychas, 2012; Li et al., 2018). Moreover, QS research has transcended disciplinary boundaries, in combination with materials science, antimicrobial coatings have been developed that possess the ability to regulate and inhibit QS, enhancing the efficiency of wastewater treatment facilities (Shrout and Nerenberg, 2012; Siddiqui et al., 2015). And QS research has transcended disciplinary boundaries, for instance, QS-triggered microbial fuel cells have showed significant application prospects in electricity generation and wastewater treatment (Monzon et al., 2016; Sivasankar et al., 2019). This multidisciplinary approach underscores the far-reaching impact of QS research and its potential to address an array of challenges across diverse fields.
Bibliometrics serves as a quantitative approach utilized to analyze and assess scientific literature by employing statistical techniques (Kim et al., 2020). This methodology is instrumental in identifying research focal points and emerging trends within a given field, offering valuable insights into its current status and providing direction for future research initiatives. As an illustration, researchers have effectively employed bibliometric analysis to explore the evolving research landscape concerning subjects like microneedles, chronic wounds and transdermal drug delivery (Chen et al., 2022, 2023a,b). Providing an impartial portrayal of research trends, bibliometrics analysis plays a crucial role in guiding and molding the course of future research endeavors.
This investigation aims to comprehensively assess the existing body of literature concerning QS, utilizing a bibliometric analysis approach. The objective is to enhance understanding within the QS research domain by employing co-word biclustering analysis. The expected outcomes of this study are poised to assist researchers in achieving a more precise and efficient grasp of QS research trends and advancements.
Methods
Data source and search methods
We conducted a search on the Science Citation Index Extension (SCI-E) within the Web of Science (WOS) database, using the following search criteria: articles with “quorum sensing” in the title (TI) and written in English, covering the period from January 1, 2004, to December 31, 2023. A total of 5,528 articles were retrieved. Subsequently, we excluded specific types of literature, such as early access, book chapters, news items, corrections, proceedings papers, letters, editorial materials, and meeting abstracts, and used Photoshop V2020 to produce Figure 1. For co-word clustering analysis, we employed the retrieval function provided by the NCBI for Medical Subject Heading (MeSH) terms, with the analysis period set from 2004 to 2023 and “quorum sensing” designated as the MeSH term. All publications were retrieved as of December 21, 2023, to minimize potential biases stemming from database updates.
Data collection
Two independent reviewers assessed the search outcomes, considering various factors including the H-index, citation count, author details, geographical location, publication date, and title. These reviewers achieved a substantial overall agreement ratio of 0.90. In cases where disparities in assessments arose, a third reviewer was consulted to make the final determination regarding data inclusion. The data used for analysis was imported into two separate platforms: an online Bibliometrics analysis platform (http://bibliometric.com/) and VOSviewer V1.6.17 (Leiden University, Leiden, the Netherlands), both using the “Tab Delimited File” format. For CiteSpace V 6.2.R4 (Drexel University, Philadelphia, PA, United States), the data was uploaded in the “Plain Text File” format. Furthermore, MeSH terms obtained from NCBI were initially formatted as “PubMed” and subsequently input into BICOMB V2.02 for analysis. Subsequently, gCLUTO V1.0 (Graphical Clustering Toolkit) was employed to visualize biclustering based on the co-word matrix file generated during the investigation.
Data investigation
Bibliometric analysis and geographical distribution
We utilized the Bibliometrics online analysis platform to visualize international collaborations and contributions at national/regional levels. VOSviewer was employed for creating a clear clustering visualization, with a primary focus on evaluating inter-agency cooperation among institutions. Additionally, we employed the number of journals published as the standard for density clustering visualization. Within VOSviewer, we selected the “bibliographic data-based map creation” data format and specified “Co-authorship” as the analysis category, resulting in the generation of the “Density Visualization” graphic. Furthermore, CiteSpace was utilized to forecast future research directions, identify research trends, and pinpoint emerging keywords over time. To ensure data accuracy, we recommended applying the “Remove duplicates” function before configuring keywords as node types, with “the number of states” set to 2. Journal impact factors (IF) were determined by referencing the 2023 Journal Citation Report (JCR).
Co-word biclustering analysis
We utilized BICOMB and gCLUTO to perform biclustering analysis on significant MeSH terms and MeSH subheadings, with the objective of identifying key research focal points. In the BICOMB software, we formatted the data in the “PubMed” style and imported the relevant MeSH term data. By using the “Extract” function, we configured it to include both the main topic and sub-topic. Subsequently, specialized software was employed to convert the primary MeSH terms into a matrix, resulting in a co-word matrix highlighting frequently occurring MeSH terms. This co-word matrix was then input into gCLUTO, where we defined parameters, including the “Number of Clusters,” and selected the “Repeated Bisection” as the cluster method. Finally, matrix and mountain visualization techniques were applied to present the outcomes of the biclustering analysis, offering valuable insights into research hotspots within the QS field.
Results
Investigation of publications output
As per our inclusion criteria, a combined total of 4,367 research articles and 482 review articles pertaining to QS were identified (Figure 1). An analysis of publication trends from 2004 to 2023 reveals a consistent growth pattern, with a notable upsurge in 2017 (Figure 2). It is worth noting that, as of the present date, the count of articles published in 2023 has reached 394, signifying nearly 4-fold increase compared to the number published in 2004. The lower number of publications in 2023 compared to 2022 could be attributed to the data collection not encompassing the entire year and potential delays in the online publication of some works.
The contributions of nations and institutions to global publications
The exploration of QS spans across a diverse spectrum of 101 countries and regions. Upon data import, a thermal map (Figure 3) vividly illustrates that these articles predominantly concentrate in East Asia, North America, and Western Europe. Significantly, the United States (n = 1,292) emerges as the foremost contributor, followed by China (n = 1,048) and India (n = 514; Table 1). Figure 4 showcases the publication growth trajectories of major countries. An analysis of collaboration among countries and regions indicates that there is a close level of cooperation between the United States and China (Figure 5). Centrality serves as a pivotal indicator of a country's global collaboration involvement, with elevated centrality values indicating a more substantial impact. The results indicate that the United States exhibits the highest centrality (center = 0.51), followed closely by China (0.32) and England (0.25). Among research institutions, the top five comprise University of Nottingham (n = 129), Center National De La Recherche Scientifique (n = 124), Princeton University (n = 112), University of Wisconsin System (n = 90), and Howard Hughes Medical Institute (n = 89; Table 1). Employing VOSviewer, we scrutinized inter-agency collaborations through co-authorship and visualized these alliances as density clusters (Figure 6). This analysis of inter-agency partnerships reveals 10 distinct clusters, each represented by a unique color. Within the same color cluster, the level of collaboration among institutions is relatively high, exemplified by the joint publication of documents with shared affiliations and instances where a scholar holds concurrent positions across different institutions.
Journals publishing research on quorum sensing
A total of 1,003 scholarly journals have made substantial contributions to disseminating QS -related research. Figure 7 provides an overview of journals excelling in this research domain. Among the 4,849 articles included in the QS database, 987 (comprising 20.35%) found their place in the top 10 journals (Table 2). Notably, Frontiers in Microbiology has the highest average citation number, closely followed by Journal of Bacteriology and PLoS ONE, collectively representing 9.3% of all articles in this field. Noteworthy among journals with more than 10 publications, Nature Microbiology (IF: 28.3), trailed by Nature Communications (IF: 16.6) and Nucleic Acids Research (IF: 14.9), all maintaining prestigious Q1 classification according to the JCR 2023 standard.
Authors' contributions to quorum sensing research
A total of 16,828 distinct authors made active contributions to this investigation, with Table 3 presenting the top 10 most prolific researchers. Noteworthy among them are Bassler, Bonnie L. from Princeton University and Howard Hughes Medical Institute, USA, renowned for her groundbreaking work in elucidating the molecular mechanisms of quorum sensing in bacteria; Williams, Paul from University of Nottingham, England, who has significantly advanced our understanding of bacterial biofilm formation and antibiotic resistance through his research on quorum sensing; and Blackwell, Helen E. from University of Wisconsin Madison, USA, acclaimed for her innovative synthesis of small molecules that modulate bacterial quorum sensing, all of whom stand as leading contributors in the field of microbiology and infectious disease control. Furthermore, we conducted an examination of highly cited articles within the QS research domain, with Table 4 showcasing the top 10 articles. Among these, the study titled “Quorum sensing: Cell-to-cell communication in bacteria” authored by Bassler et al., and published in Annual Review of Cell and Developmental Biology in 2005 (n = 2,676), emerges as the most frequently cited research (Table 4).
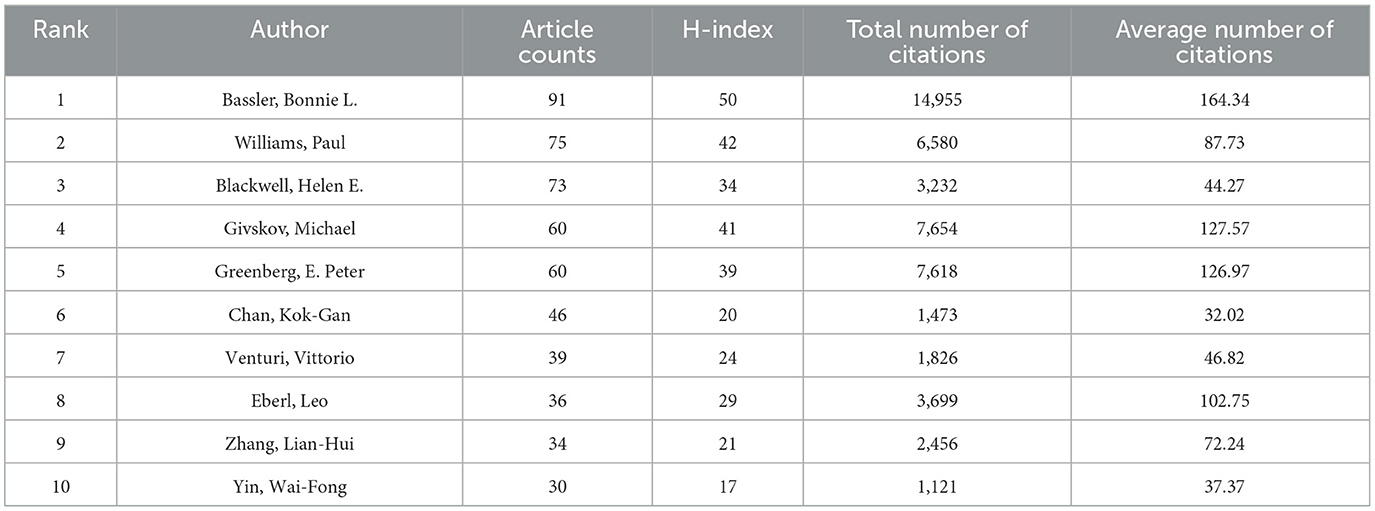
Table 3. The top 10 most productive authors contributed to publications in quorum sensing (2004–2023).
Research hotspots of quorum sensing
We utilized CiteSpace for the extraction of keywords from a dataset encompassing 4,849 literature sources. This process led to the identification of the top 25 dynamic burst words, covering the period from 2004 to 2023, shedding light on the evolving trends in research hotspots (Figure 8). Furthermore, our analysis revealed a total of 2,707 significant MeSH terms/MeSH subheadings, with a collective frequency of 9,543 instances. High-frequency terms, defined according to the G-index criteria, were those recurring more than 31 times (Table 5).
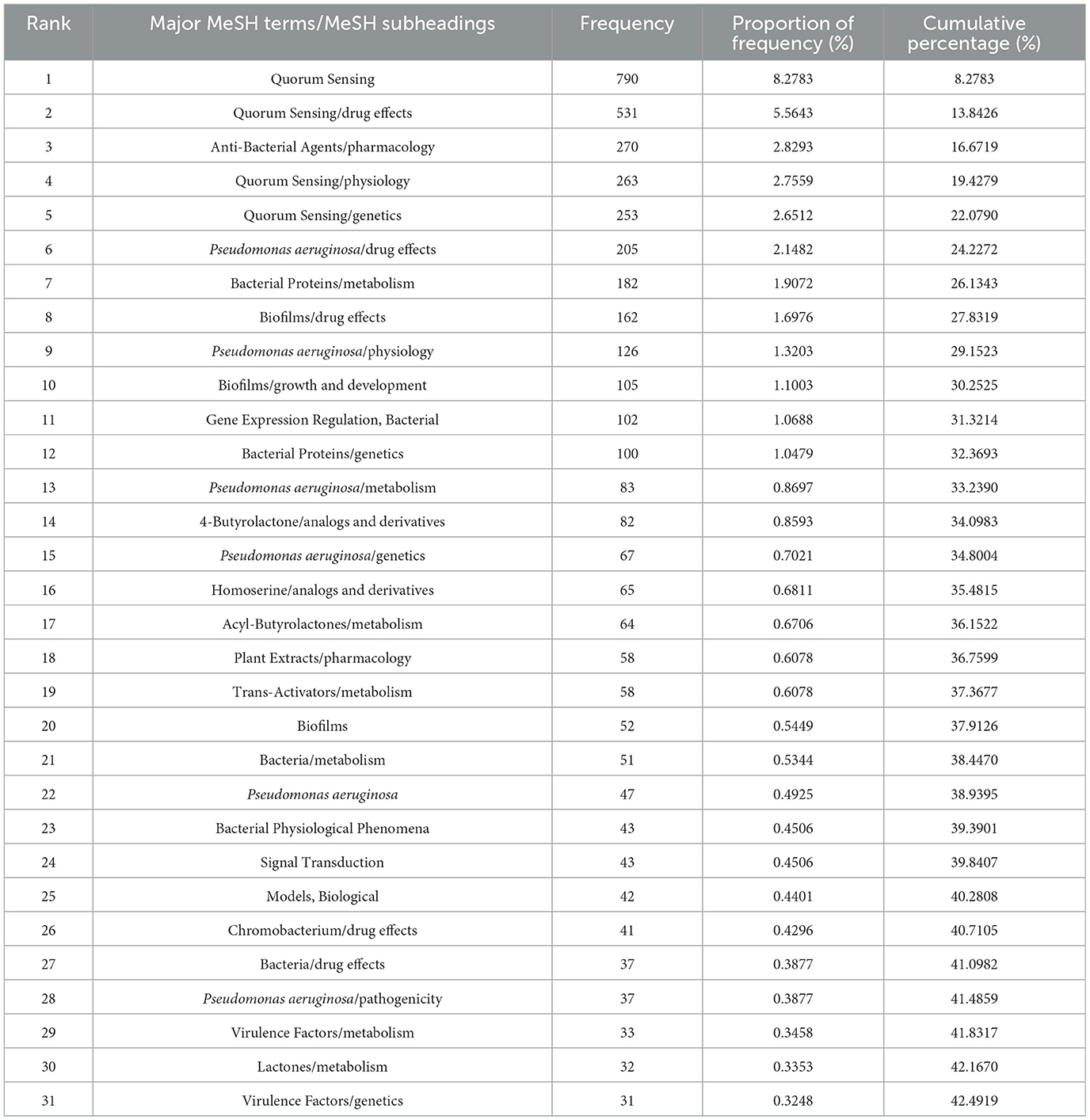
Table 5. Major MeSH terms/MeSH subheadings from the included publications on quorum sensing (2004–2023).
In order to visualize these prominent research themes, we conducted biclustering analysis using BICOMB and gCLUTO. BICOMB was utilized to create a co-word matrix, which was then imported into gCLUTO to generate matrix visualization (Figure 9) and mountain visualization (Figure 10). The matrix on the left aligns with the primary MeSH terms and MeSH subheading terms depicted on the right. The visual distinction in the matrix blocks indicates the frequency of term occurrences. The mountain graph revealed the presence of three distinct clusters within the research domain. The gap between these peaks signifies the level of correlation between clusters, with mountain height and volume reflecting their internal similarity and term coverage. Additionally, the transition in colors, from red to green atop these peaks, corresponds to standard deviation levels. The biclustering analysis of the publications led to the identification of three primary clusters: (I) Drug effects and pharmacology of quorum sensing; (II) Genetics and metabolism of quorum sensing; (III) Physiology of quorum sensing.
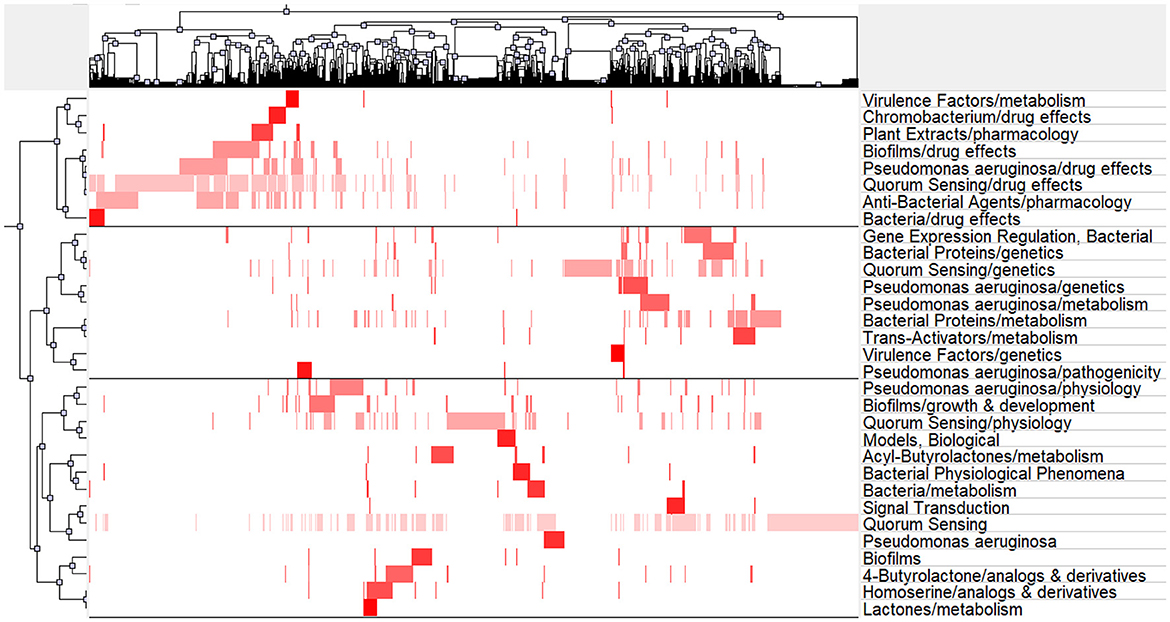
Figure 9. Matrix visualization of major MeSH terms/MeSH subheading terms of articles on quorum sensing.
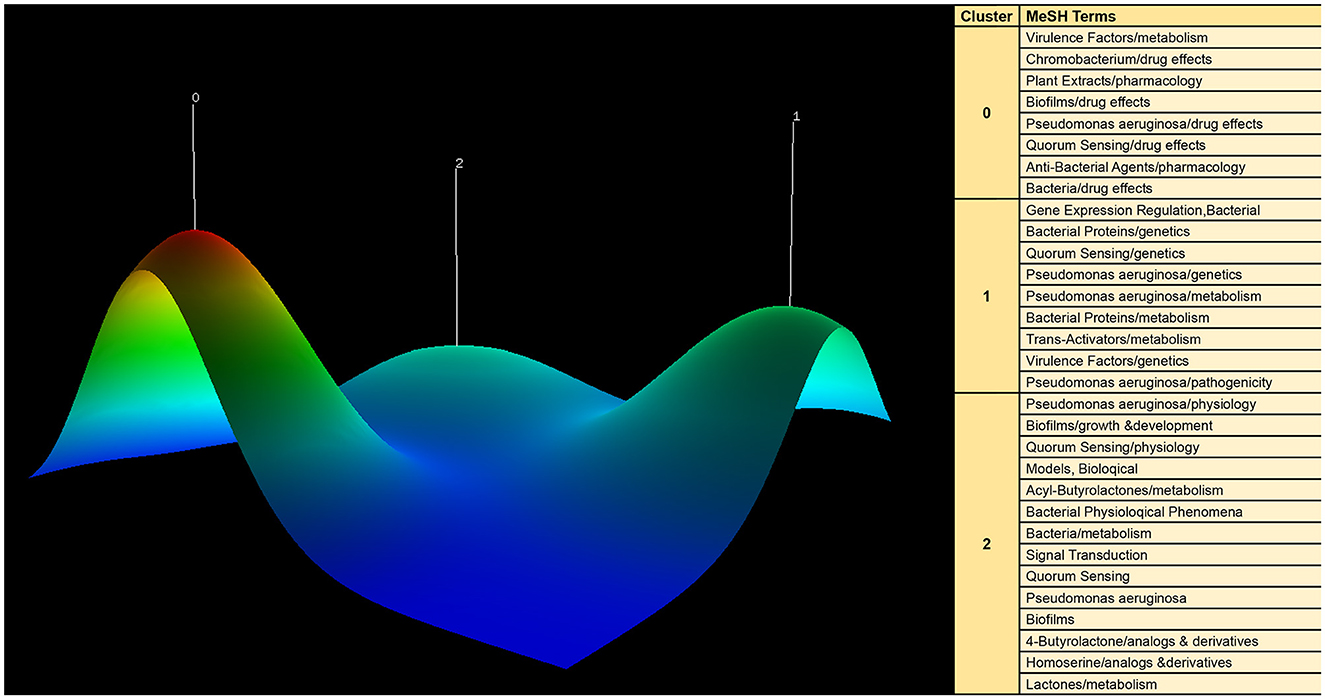
Figure 10. Mountain visualization of major MeSH terms/MeSH subheading terms of articles on quorum sensing.
Discussion
The findings of the bibliometric analysis reveal a substantial expansion in the literature concerning QS from 2004 to 2023, signifying the increasing complexity of staying updated with current research trends. To address this challenge, we conducted a comprehensive investigation employing both bibliometric and biclustering analysis methodologies. This extensive study encompassed the retrieval of relevant articles from the WOS and NCBI databases, spanning two decades period. The outcomes of this inquiry are anticipated to yield valuable insights into research focal points and provide guidance on future trends.
Overview of quorum sensing research
The exploration of QS mechanisms has unveiled a complex network of bacterial communication, surpassing the traditional boundaries of microbiological research and revealing its profound impact on microbiology, clinical medicine, and environmental science. For instance, the development of QS interference compounds could lead to significant advancements in preventing biofilm-associated infections. Moreover, the application of QS research in environmental engineering, especially in wastewater treatment, demonstrates tremendous potential, contributing to more efficient and environmentally friendly pollution control.
This study has evaluated national academic contributions and research quality within the QS domain, considering various factors such as article quantity, total citation count, centrality, and average citations per publication for each country or region. Notably, the results indicate that USA holds the most significant influence in QS research, accumulating a total of 1,292 article counts. In close pursuit, the China and India garnered 1,048 and 514, respectively. It's noteworthy that research on QS in the United States has exhibited a declining trend, while China has consistently increased its QS publications, surpassing the United States in 2018. China leads in influence (centrality = 0.32), ranking second among the top 10 countries. However, there is room for improvement in the quality of QS publications from China, as the average citations per study are relatively low (21.03), placing it last among the top 10 countries. This phenomenon may be associated with the impetuous atmosphere prevalent in the academic community, where there is a one-sided pursuit of the quantity of publications. The thermal world map highlights regions making substantial investments in QS research. The findings suggest that specific regions, such as Africa, Central Asia, and Eastern Europe, have limited participation in QS research and could enhance their contributions to this field through international collaboration and support.
The results reveal that nearly half of the top 10 institutions making substantial contributions to the field of QS are affiliated with the United States (n = 4), highlighting its significant impact. We utilized a visually engaging density visualization technique to represent distinct institution clusters, facilitating an intuitive evaluation of their collaborative networks. Institutions that collaborate closely are depicted within color-coded clusters, where the size of each cluster area signifies its importance in the field. Furthermore, the size of each institution's label corresponds to the extent of its collaboration with other research institutions. Prominent contributors to QS research include University of Nottingham (n = 129), Princeton University (n = 112), University of Wisconsin Madison (n = 90), Ghent University (n = 85).
The leading journals within the QS research domain, including Frontiers in Microbiology, Journal of Bacteriology, and PLoS ONE, have collectively made a substantial contribution, accounting for over 9% of the research output. Among these publications, Frontiers in Microbiology stands as the prominent leader, evident from its significantly higher article counts number of QS-related articles compared to its closest counterpart. Additionally, this journal boasts the high H-index, further underscoring its pivotal role in advancing knowledge within the QS field. These findings highlight the influential contributions of Frontiers in Microbiology in shaping the discourse and progression of QS research.
The examination of prominent terms in this field indicated a significant shift in the primary research focus between 2012 and 2018. Previously, emphasis centered primarily on the molecular mechanisms of QS, with a particular interest in bioactive compounds and their impact on bacterial cooperation, biofilm formation, and antibiotic resistance. In the contemporary landscape, the scope of research has expanded significantly to include investigations into novel QS inhibitors, antibacterial activities, and the use of advanced techniques like transcriptomics and synthetic biology to dissect the intricate workings of QS systems. This transformation underscores the dynamic nature of scientific exploration, as researchers adapt their inquiries to address pressing issues related to antimicrobial resistance, biofilm control, and the broader applications of QS research.
Three clustering hotspots of quorum sensing research
Drug effects and pharmacology of quorum sensing
Cluster 0 presents a correlation with drug effects and pharmacology of QS. The research of QS has ushered in a new era of controlling bacterial behavior and physiological processes, highlighting the potential of drug effects and pharmacological interventions. Biofilms, complex communities of bacteria encased in a protective extracellular matrix, are notorious for their resistance to traditional antibiotics (Dufour et al., 2010). However, the development of anti-bacterial agents specifically targeting QS mechanisms has shown great promise in disrupting biofilm formation and rendering bacteria more susceptible to treatment (Bhardwaj et al., 2013; Zhao et al., 2013). Furthermore, QS inhibitors play a crucial role in mitigating toxin production. Many pathogens rely on QS to regulate the synthesis of virulence factors (Rutherford and Bassler, 2012). Inhibitors can significantly reduce the release of harmful substances by blocking these QS signals, thereby weakening the pathological effects caused by bacterial infections (Ni et al., 2009; El-Mowafy et al., 2014). QS inhibitors may play a key role in inhibiting the spread of antibiotic resistance genes by interfering with bacterial gene transfer mechanisms (Zhang et al., 2018). Additionally, they affect bacterial motility and iron acquisition, which are essential for bacterial colonization and the progression of infection (Sethupathy et al., 2016; Bahari et al., 2017). Currently, in the field of drug discovery, a variety of compounds, including furanone derivatives (Chang et al., 2019), benzimidazole derivatives (El-Gohary and Shaaban, 2017), Benzothiazole derivatives (Chu et al., 2023), Pyranone derivatives (Chen et al., 2019), Quinolines derivatives (Beus et al., 2020), Pyrrole derivatives (Hassan et al., 2016), Indole derivatives (Odularu et al., 2022), and others, have exhibited QS inhibitory properties.
It is noteworthy that various animal models are crucial for elucidating the therapeutic potential and mechanisms of these compounds. In addition to rats or mice, the use of Caenorhabditis elegans has provided a foundational understanding of the effects of QS inhibitors on host-pathogen interactions (Husain et al., 2016; Liu et al., 2020). Similarly, the zebrafish model, owing to its transparent embryonic development, has become a valuable tool for the visualization of microbial infections in vivo (Papaioannou et al., 2013; Nogaret et al., 2021). These models collectively contribute to the advancement of research on QS inhibitors. Thus, it is essential to emphasize the importance of these animal models in propelling the research on QS inhibitors forward. And antibodies designed to quench QS signaling represent a biotechnological approach that has gained traction in recent years. By specifically targeting QS molecules, these antibodies disrupt bacterial communication pathways and interfere with the expression of virulence factors and biofilm formation (Ahmed et al., 2019; Munir et al., 2020; Zhou et al., 2020; Gunaratnam et al., 2021). Moreover, the utilization of biotic polymers and polymeric materials in QS inhibition is a promising approach (Lu et al., 2022; Tajani et al., 2022). These materials are engineered to deter bacterial adhesion and biofilm development on surfaces, making them invaluable for reducing the risk of infections associated with medical devices and industrial settings.
In summary, in seeking innovative antibacterial strategies, QS inhibitors differ from traditional antibiotics in their bactericidal or bacteriostatic effects, reducing the selective pressure on bacteria, which, in theory, could lower bacterial resistance. Moreover, QS inhibitors exemplify a targeted antimicrobial strategy that can minimize harm to beneficial microbial communities, thus potentially reducing adverse effects associated with antibiotic treatment (Delago et al., 2016; Kang et al., 2019). Integrating QS inhibitors into treatment regimens could enhance pathogens' sensitivity to existing drugs, thereby improving the efficacy of antibiotics (Brackman et al., 2011; Sun et al., 2019). However, the complexity of translating laboratory findings into clinical applications must be acknowledged. Despite the proven effectiveness of various QS inhibitors in vitro, their transition to clinical application faces several challenges. Firstly, bacterial QS systems are diverse and complex, with different species using different signaling molecules and receptors, making the development of broad-spectrum QS inhibitors or those targeting specific bacterial QS systems difficult and limited. Secondly, in the complex environment of the human body, factors like pH and enzyme activity can affect their functionality, potentially impacting the efficiency and stability of QS inhibitors. Most importantly, the safety and specificity of these inhibitors require rigorous toxicity assessments and multi-phase clinical trials. Therefore, although QS inhibitors are theoretically a promising antimicrobial strategy, overcoming these challenges and successfully applying them to clinical practice still requires further research and development.
Genetics and metabolism of quorum sensing
Cluster 1 indicates the correlations with genetics and metabolism of QS. QS plays a pivotal role in orchestrating cooperative gene expression and metabolic adaptations within bacterial populations. This intricate genetic and metabolic regulation enables bacteria to synchronize their behavior, fostering cooperation within the population while enhancing competitiveness among different bacterial communities (Ng and Bassler, 2009; Schluter et al., 2016; Abisado et al., 2018). When the QS pathway is upregulated in pathogens, the burden on pathogen metabolism primarily manifests in the allocation of energy and resources. To respond to QS signaling molecules, pathogens need to invest substantial metabolic resources, which include but are not limited to ATP and precursor molecules (Vendeville et al., 2005; Goo et al., 2015). This reallocation of resources may result in a weakening of other physiological functions in the pathogen, such as a potential slowdown in growth rate (Albuquerque et al., 2014). Although the upregulation of the QS pathway can increase the pathogenicity of the pathogen, it also places stress on its metabolism.
One of the key features of QS is its ability to coordinate gene expression across multiple bacterial cells, leading to the emergence of collective group behaviors (Antonioli et al., 2019; Striednig and Hilbi, 2022). These behaviors encompass a wide range of vital processes, such as biofilm formation, virulence factor expression, and swarming motility (Castillo et al., 2014; Li et al., 2018). Such coordinated alterations in gene expression are central to understanding the physiological and ecological significance of QS in bacterial communities. Autoinducer-dependent gene expression regulation is a hallmark of QS, where signaling molecules, such as AHL, mediate the communication between bacteria (Czajkowski and Jafra, 2009; LaRock et al., 2013; Calatrava-Morales et al., 2018). AHL-mediated QS is intricately linked to substance metabolism and energy utilization. For instance, in the opportunistic pathogen Pseudomonas aeruginosa, AHL serves as the principal signaling molecule controlling the expression of ~300 genes involved in various cellular functions, including pathogenesis (Brindhadevi et al., 2020). This molecular dialogue, facilitated by AHL, underscores the profound impact of QS on bacterial metabolism, influencing the utilization of resources and the expression of virulence factors essential for host colonization and infection (Haque et al., 2018; Warrier et al., 2021; Zhu et al., 2023).
In some species, there are multiple QS pathways, aimed at providing more complex and nuanced behavioral regulation. This intricate regulatory system allows bacteria to respond to a variety of internal and external signals under different environmental conditions, implementing more flexible and efficient survival strategies. Each QS system can specifically sense different signal molecules and trigger specific gene expression patterns, enabling bacteria to adjust their metabolic pathways, production of virulence factors, and biofilm formation in response to changes in environmental conditions and population density (Miller and Bassler, 2001). Moreover, cross-communication between multiple QS pathways is very common, which can either promote or inhibit each other. Furthermore, QS's role extends beyond mere bacterial communication, acting as a pivotal driver in the evolutionary and adaptive processes of microbial life. Its integration with mobile genetic elements, such as conjugative elements (Qixin et al., 2022), fosters horizontal gene transfer among diverse bacterial species (Brameyer and Heermann, 2015; Hoover et al., 2015). Through this mechanism, bacteria are able to integrate information from different signals, allowing them to coordinate their behavior in specific environments and enhance their adaptability.
By delving into the genetic and metabolic frameworks underpinning QS, researchers unravel the intricate mechanisms of bacterial behaviors. This knowledge not only sheds light on the molecular underpinnings of microbial consortia but also reveals the profound influence of QS on microbial evolution, community structuring, and ecological function. Therefore, exploring the depths of QS systems offers valuable insights into microbial ecology, promising advancements in biotechnological applications and novel approaches in combating bacterial pathogenicity.
Physiology of quorum sensing
Cluster 2 presents the correlations with physiology of QS. In these complex ecosystems, various bacterial species utilize specific QS signal molecules to establish communication, enabling them to engage in mutually beneficial symbiotic relationships or to compete for essential resources (Hoover et al., 2015; Qixin et al., 2022). This intricate web of interspecies interactions is facilitated by a diverse array of QS signal molecules, including AHLs (Brameyer and Heermann, 2015), autoinducer-2 (AI-2; Hooshangi and Bentley, 2008; Zhao et al., 2018), extracellular death factor (EDF; León-Félix and Villicaña, 2021), γ-butyrolactone (GBL; Decho et al., 2010), diffusible signal factor (DSF; He et al., 2023), and α-hydroxyketones (AHKs; Mangwani et al., 2016). Notably, AHKs and DSF have been shown to mediate signal communication not only between bacterial species but also between fungi and even between eukaryotic host cells (Ahn et al., 2022; Fan et al., 2022). In addition, nitric oxide is involved in a variety of physiological processes, including antimicrobial activities in immune responses (MacMicking et al., 1997). At the same time, the presence of nitric oxide can also be sensed by bacteria through their QS system, thereby regulating their metabolic behavior in response to these signals. For example, nitric oxide (NO) in influencing the autophosphorylation of partner histidine kinases integrated in the QS pathway of Vibrio (Heckler and Boon, 2019). The existence of these signals reveals the complex interactions between hosts and microbes.
Advancements in QS research have revealed the specificity of signal transduction mechanisms across different species. Particularly, the LuxI-LuxR pathway plays a crucial role in the regulation of virulence, biofilm formation, and antibiotic resistance in various Gram-negative bacteria. For instance, P. aeruginosa regulates virulence genes through QS circuits, including the LasI/LasR and RhlI/RhlR systems. These systems control the production of virulence factors essential for the bacterium's infection capability and survival under diverse environmental conditions (Banerjee and Ray, 2016). On the other hand, Gram-positive bacteria primarily utilize autoinducing peptides (AIPs). The system in S. pneumoniae and B. subtilis exemplifies a QS pathway where a competence stimulating peptide activates receptor kinase to trigger DNA uptake, showcasing QS's role in competence (Miller and Bassler, 2001; Wolf et al., 2016). Similarly, the accessory gene regulator (Agr) system in S. aureus and C. perfringens underlines QS's importance in virulence factor regulation (Wang and Muir, 2016), with the Agr locus orchestrating a phosphorylation relay that upregulates Agr genes and virulence factors like δ-toxin (Bhatt, 2018).
Limitation
The utilization of bibliometric analysis in research is not without limitations. Among these constraints is the potential omission of high-quality research from the dataset due to inadequate citation frequency. This situation may arise because recently published articles might not have had ample time to accumulate citations, which constitutes a significant basis for bibliometric assessment (Wallin, 2005). Additionally, the biclustering analysis employed for categorizing and identifying pertinent keywords may not encompass all relevant subjects within a research field, such as this study primarily focusing on the biomedical field. Furthermore, data derived from the Web of Science database might not encompass the most recent publications owing to update delays. Nonetheless, it is imperative to acknowledge that bibliometric analysis retains its value as a tool for achieving a comprehensive and meaningful comprehension of the research landscape within a specific domain.
Conclusions
In conclusion, this bibliometric analysis has illuminated significant aspects of QS research, shedding light on its evolving trajectory and its growing importance within the scientific community. Our examination of QS literature has revealed a consistent upward trend in research output, reflecting the expanding interest and recognition of QS as a pivotal mechanism in microbial communication and its implications across various disciplines. By identifying key contributors, prolific journals, and prominent geographical regions, this study provides critical insights into the landscape of QS research. Moreover, the diversified applications of QS in biotechnology, medicine, and ecology underscore its multifaceted significance. Therefore, collaboration among microbiologists, medical scientists, ecologists, computer scientists, and others is encouraged. Interdisciplinary research can facilitate breakthroughs in understanding the mechanisms of QS and its applications in biotechnology, medicine, and environmental science. As we delve deeper into the intricate web of QS, it becomes evident that this field's potential remains largely untapped, with ample opportunities for future exploration and innovation. The physiology, pharmacology, genetics, and metabolism of QS are research hotspots in the biomedical field. Moreover, the importance of researching novel QS inhibitors is increasingly emphasized. Through a comprehensive analysis of QS research hotspots, we have identified the tremendous potential of QS research for developing new therapeutic strategies and eco-friendly biotechnological applications. Furthermore, we discuss the importance of transforming research outcomes of QS inhibitors into practical applications. These findings not only consolidate the current state of QS research but also provide a valuable foundation for guiding future endeavors in unraveling the complexities of microbial communication and its wide-ranging impacts. Anticipatedly, this study aims to offer valuable support and direction to scholars and practitioners engaged in this particular domain.
Data availability statement
The original contributions presented in the study are included in the article/supplementary material, further inquiries can be directed to the corresponding authors.
Author contributions
XC: Conceptualization, Formal analysis, Methodology, Software, Supervision, Writing – original draft, Visualization. JL: Conceptualization, Formal analysis, Software, Writing – original draft, Visualization. RL: Data curation, Formal analysis, Software, Writing – original draft, Supervision. XS: Data curation, Writing – original draft, Software. YX: Data curation, Software, Writing – original draft. XX: Writing – original draft, Conceptualization, Supervision. HX: Conceptualization, Funding acquisition, Writing – review & editing, Supervision. DX: Conceptualization, Funding acquisition, Project administration, Visualization, Writing – review & editing.
Funding
The author(s) declare financial support was received for the research, authorship, and/or publication of this article. We are grateful to the funds for supporting this study, including National Natural Science Foundation of China (82002289), Natural Science Foundation of Sichuan Province (2022NSFSC0685), Medical Research Project Plan of Sichuan Province (S20012), Science and Technology Support program of Sichuan Province (2023YFS0065), Science and Technology Foundation of Sichuan Province (No. 23ZDYF2471), Soft Science Research Project of Science and Technology Department of Sichuan Province (2023JDR0209), and College-City Cooperation Project of Nanchong City (22SXJCQN0002, 20SXQT0335, 20SXQT0162, and 20SXQT0106).
Conflict of interest
The authors declare that the research was conducted in the absence of any commercial or financial relationships that could be construed as a potential conflict of interest.
Publisher's note
All claims expressed in this article are solely those of the authors and do not necessarily represent those of their affiliated organizations, or those of the publisher, the editors and the reviewers. Any product that may be evaluated in this article, or claim that may be made by its manufacturer, is not guaranteed or endorsed by the publisher.
References
Abisado, R. G., Benomar, S., Klaus, J. R., Dandekar, A. A., and Chandler, J. R. (2018). Bacterial quorum sensing and microbial community interactions. MBio 9:17. doi: 10.1128/mBio.02331-17
Ahmed, S. A., Rudden, M., Smyth, T. J., Dooley, J. S., Marchant, R., Banat, I. M., et al. (2019). Natural quorum sensing inhibitors effectively downregulate gene expression of Pseudomonas aeruginosa virulence factors. Appl. Microbiol. Biotechnol. 103, 3521–3535. doi: 10.1007/s00253-019-09618-0
Ahn, S. H., Karlsson, A. J., Bentley, W. E., and Raghavan, S. R. (2022). Capsules with bacteria and fungi in distinct compartments: a platform for studying microbes from different kingdoms and their cross-communication. PLoS ONE 17:277132. doi: 10.1371/journal.pone.0277132
Albuquerque, P., Nicola, A. M., Nieves, E., Paes, H. C., Williamson, P. R., Silva-Pereira, I., et al. (2014). Quorum sensing-mediated, cell density-dependent regulation of growth and virulence in Cryptococcus neoformans. MBio 5:13. doi: 10.1128/mBio.00986-13
Alibi, S., Selma, W. B., Ramos-Vivas, J., Smach, M. A., Touati, R., Boukadida, J., et al. (2020). Anti-oxidant, antibacterial, anti-biofilm, and anti-quorum sensing activities of four essential oils against multidrug-resistant bacterial clinical isolates. Curr. Res. Transl. Med. 68, 59–66. doi: 10.1016/j.retram.2020.01.001
Annous, B. A., Fratamico, P. M., and Smith, J. L. (2009). Scientific status summary: quorum sensing in biofilms: why bacteria behave the way they do. J. Food Sci. 74, R24–R37. doi: 10.1111/j.1750-3841.2008.01022.x
Antonioli, L., Blandizzi, C., Pacher, P., Guilliams, M., and Haskó, G. (2019). Rethinking communication in the immune system: the quorum sensing concept. Trends Immunol. 40, 88–97. doi: 10.1016/j.it.2018.12.002
Arnold, J., Singley, C. T., and Williams-Arnold, L. D. (1972). Embryonic development and post-hatching survival of the sepiolid squid Euprymna scolopes under laboratory conditions. Veliger 14, 361–364.
Bahari, S., Zeighami, H., Mirshahabi, H., Roudashti, S., and Haghi, F. (2017). Inhibition of Pseudomonas aeruginosa quorum sensing by subinhibitory concentrations of curcumin with gentamicin and azithromycin. J. Glob. Antimicrob. Resist. 10, 21–28. doi: 10.1016/j.jgar.2017.03.006
Banerjee, G., and Ray, A. K. (2016). The talking language in some major Gram-negative bacteria. Archiv. Microbiol. 198, 489–499. doi: 10.1007/s00203-016-1220-x
Berg, G. (2009). Plant-microbe interactions promoting plant growth and health: perspectives for controlled use of microorganisms in agriculture. Appl. Microbiol. Biotechnol. 84, 11–18. doi: 10.1007/s00253-009-2092-7
Beus, M., Savijoki, K., Patel, J. Z., Yli-Kauhaluoma, J., Fallarero, A., Zorc, B., et al. (2020). Chloroquine fumardiamides as novel quorum sensing inhibitors. Bioorg. Med. Chem. Lett. 30:127336. doi: 10.1016/j.bmcl.2020.127336
Bhardwaj, A. K., Vinothkumar, K., and Rajpara, N. (2013). Bacterial quorum sensing inhibitors: attractive alternatives for control of infectious pathogens showing multiple drug resistance. Recent Pat. Anti-infective Drug Discov. 8, 68–83. doi: 10.2174/1574891X11308010012
Bhatt, V. S. (2018). Quorum sensing mechanisms in gram positive bacteria. Implicat. Quor. Sens. Syst. Biofilm Form. Virul. 20, 297–311. doi: 10.1007/978-981-13-2429-1_20
Brackman, G., Cos, P., Maes, L., Nelis, H. J., and Coenye, T. (2011). Quorum sensing inhibitors increase the susceptibility of bacterial biofilms to antibiotics in vitro and in vivo. Antimicrob. Agents Chemother. 55, 2655–2661. doi: 10.1128/AAC.00045-11
Brameyer, S., and Heermann, R. (2015). Specificity of signal-binding via non-AHL LuxR-type receptors. PLoS ONE 10:124093. doi: 10.1371/journal.pone.0124093
Brindhadevi, K., LewisOscar, F., Mylonakis, E., Shanmugam, S., Verma, T. N., and Pugazhendhi, A. (2020). Biofilm and Quorum sensing mediated pathogenicity in Pseudomonas aeruginosa. Process Biochem. 96, 49–57. doi: 10.1016/j.procbio.2020.06.001
Calatrava-Morales, N., McIntosh, M., and Soto, M. J. (2018). Regulation mediated by N-acyl homoserine lactone quorum sensing signals in the rhizobium-legume symbiosis. Genes 9:263. doi: 10.3390/genes9050263
Castillo, S., Heredia, N., Arechiga-Carvajal, E., and García, S. (2014). Citrus extracts as inhibitors of quorum sensing, biofilm formation and motility of Campylobacter jejuni. Food Biotechnol. 28, 106–122. doi: 10.1080/08905436.2014.895947
Chang, Y., Wang, P. C., Ma, H. M., Chen, S. Y., Fu, Y. H., Liu, Y. Y., et al. (2019). Design, synthesis and evaluation of halogenated furanone derivatives as quorum sensing inhibitors in Pseudomonas aeruginosa. Eur. J. Pharmaceut. Sci. 140:105058. doi: 10.1016/j.ejps.2019.105058
Chen, J., Wang, B., Lu, Y., Guo, Y., Sun, J., Wei, B., et al. (2019). Quorum sensing inhibitors from marine microorganisms and their synthetic derivatives. Mar. Drugs 17:80. doi: 10.3390/md17020080
Chen, X., Shi, X., Xiao, H., Xiao, D., and Xu, X. (2023a). Research hotspot and trend of chronic wounds: a bibliometric analysis from 2013 to 2022. Wound Repair Regener. 2023:13117. doi: 10.1111/wrr.13117
Chen, X., Xiao, H., Shi, X., Zhao, Q., Xu, X., Fan, P., et al. (2023b). Bibliometric analysis and visualization of transdermal drug delivery research in the last decade: global research trends and hotspots. Front. Pharmacol. 14:1173251. doi: 10.3389/fphar.2023.1173251
Chen, X., Xiao, H., Zhao, Q., Xu, X., Cen, Y., Xiao, D., et al. (2022). Research hotspot and trend of microneedles in biomedical field: a bibliometric analysis from 2011 to 2020. Burns 48, 959–972. doi: 10.1016/j.burns.2022.04.004
Chu, P. L., Feng, Y. M., Long, Z. Q., Xiao, W. L., Ji, J., Zhou, X., et al. (2023). Novel benzothiazole derivatives as potential anti-quorum sensing agents for managing plant bacterial diseases: synthesis, antibacterial activity assessment, and SAR study. J. Agri. Food Chem. 71, 6525–6540. doi: 10.1021/acs.jafc.2c07810
Cornforth, D. M., Popat, R., McNally, L., Gurney, J., Scott-Phillips, T. C., Ivens, A., et al. (2014). Combinatorial quorum sensing allows bacteria to resolve their social and physical environment. Proc. Natl. Acad. Sci. U. S. A. 111, 4280–4284. doi: 10.1073/pnas.1319175111
Czajkowski, R., and Jafra, S. (2009). Quenching of acyl-homoserine lactone-dependent quorum sensing by enzymatic disruption of signal molecules. Acta Biochim. Polon. 56:2512. doi: 10.18388/abp.2009_2512
Decho, A. W., Norman, R. S., and Visscher, P. T. (2010). Quorum sensing in natural environments: emerging views from microbial mats. Trends Microbiol. 18, 73–80. doi: 10.1016/j.tim.2009.12.008
Delago, A., Mandabi, A., and Meijler, M. M. (2016). Natural quorum sensing inhibitors-small molecules, big messages. Israel J. Chem. 56, 310–320. doi: 10.1002/ijch.201500052
Dufour, D., Leung, V., and Lévesque, C. M. (2010). Bacterial biofilm: structure, function, and antimicrobial resistance. Endodont. Top. 22, 2–16. doi: 10.1111/j.1601-1546.2012.00277.x
Eickhoff, M. J., and Bassler, B. L. (2018). SnapShot: bacterial quorum sensing. Cell 174:1328.e1. doi: 10.1016/j.cell.2018.08.003
El-Gohary, N., and Shaaban, M. (2017). Synthesis and biological evaluation of a new series of benzimidazole derivatives as antimicrobial, antiquorum-sensing and antitumor agents. Eur. J. Med. Chem. 131, 255–262. doi: 10.1016/j.ejmech.2017.03.018
El-Mowafy, S. A., Abd El Galil, K. H., El-Messery, S. M., and Shaaban, M. I. (2014). Aspirin is an efficient inhibitor of quorum sensing, virulence and toxins in Pseudomonas aeruginosa. Microb. Pathog. 74, 25–32. doi: 10.1016/j.micpath.2014.07.008
Fan, Q., Wang, H., Mao, C., Li, J., Zhang, X., Grenier, D., et al. (2022). Structure and signal regulation mechanism of interspecies and Interkingdom quorum sensing system receptors. J. Agri. Food Chem. 70, 429–445. doi: 10.1021/acs.jafc.1c04751
Fisher, R. A., Gollan, B., and Helaine, S. (2017). Persistent bacterial infections and persister cells. Nat. Rev. Microbiol. 15, 453–464. doi: 10.1038/nrmicro.2017.42
Goo, E., An, J. H., Kang, Y., and Hwang, I. (2015). Control of bacterial metabolism by quorum sensing. Trends Microbiol. 23, 567–576. doi: 10.1016/j.tim.2015.05.007
Grandclément, C., Tannières, M., Moréra, S., Dessaux, Y., and Faure, D. (2016). Quorum quenching: role in nature and applied developments. FEMS Microbiol. Rev. 40, 86–116. doi: 10.1093/femsre/fuv038
Gunaratnam, S., Millette, M., McFarland, L. V., DuPont, H. L., and Lacroix, M. (2021). Potential role of probiotics in reducing Clostridioides difficile virulence: interference with quorum sensing systems. Microb. Pathog. 153:104798. doi: 10.1016/j.micpath.2021.104798
Haque, S., Ahmad, F., Dar, S. A., Jawed, A., Mandal, R. K., Wahid, M., et al. (2018). Developments in strategies for Quorum Sensing virulence factor inhibition to combat bacterial drug resistance. Microb. Pathog. 121, 293–302. doi: 10.1016/j.micpath.2018.05.046
Hassan, R., Shaaban, M. I., Abdel Bar, F. M., El-Mahdy, A. M., and Shokralla, S. (2016). Quorum sensing inhibiting activity of Streptomyces coelicoflavus isolated from soil. Front. Microbiol. 7:659. doi: 10.3389/fmicb.2016.00659
He, Y. W., Deng, Y., Miao, Y., Chatterjee, S., Tran, T. M., Tian, J., et al. (2023). DSF-family quorum sensing signal-mediated intraspecies, interspecies, and inter-kingdom communication. Trends Microbiol. 31, 36–50. doi: 10.1016/j.tim.2022.07.006
Heckler, I., and Boon, E. M. (2019). Insights into nitric oxide modulated quorum sensing pathways. Front. Microbiol. 10:2174. doi: 10.3389/fmicb.2019.02174
Hooshangi, S., and Bentley, W. E. (2008). From unicellular properties to multicellular behavior: bacteria quorum sensing circuitry and applications. Curr. Opin. Biotechnol. 19, 550–555. doi: 10.1016/j.copbio.2008.10.007
Hoover, S. E., Perez, A. J., Tsui, H. C. T., Sinha, D., Smiley, D. L., DiMarchi, R. D., et al. (2015). A new quorum-sensing system (TprA/PhrA) for Streptococcus pneumoniae D 39 that regulates a lantibiotic biosynthesis gene cluster. Mol. Microbiol. 97, 229–243. doi: 10.1111/mmi.13029
Huang, J., Shi, Y., Zeng, G., Gu, Y., Chen, G., Shi, L., et al. (2016). Acyl-homoserine lactone-based quorum sensing and quorum quenching hold promise to determine the performance of biological wastewater treatments: an overview. Chemosphere 157, 137–151. doi: 10.1016/j.chemosphere.2016.05.032
Husain, F. M., Ahmad, I., Baig, M. H., Khan, M. S., Khan, M. S., Hassan, I., et al. (2016). Broad-spectrum inhibition of AHL-regulated virulence factors and biofilms by sub-inhibitory concentrations of ceftazidime. RSC Adv/ 6, 27952–27962. doi: 10.1039/C6RA02704K
Irie, Y., and Parsek, M. R. (2008). Quorum sensing and microbial biofilms. Bacterial Biofilms 4, 67–84. doi: 10.1007/978-3-540-75418-3_4
Kang, M., Kim, S., Kim, H., Song, Y., Jung, D., Kang, S., et al. (2019). Calcium-binding polymer-coated poly (lactide-co-glycolide) microparticles for sustained release of quorum sensing inhibitors to prevent biofilm formation on hydroxyapatite surfaces. ACS Appl. Mater. Interf. 11, 7686–7694. doi: 10.1021/acsami.8b18301
Kim, H. S. J., Wahid, M., Choi, C., Das, P., Jung, S., Khosa, F., et al. (2020). Bibliometric analysis of manuscript characteristics that influence citations: a comparison of ten major dermatology journals. Burns 46, 1686–1692. doi: 10.1016/j.burns.2020.05.002
Kiran, S., Sharma, P., Harjai, K., and Capalash, N. (2011). Enzymatic quorum quenching increases antibiotic susceptibility of multidrug resistant Pseudomonas aeruginosa. Iran. J. Microbiol. 3:1.
LaRock, C. N., Yu, J., Horswill, A. R., Parsek, M. R., and Minion, F. C. (2013). Transcriptome analysis of acetyl-homoserine lactone-based quorum sensing regulation in Yersinia pestis. PLoS ONE 8:61. doi: 10.1371/annotation/3f0e8e82-1db8-45fe-a700-c64ede3e3c61
León-Félix, J., and Villicaña, C. (2021). The impact of quorum sensing on the modulation of phage-host interactions. J. Bacteriol. 203:20. doi: 10.1128/JB.00687-20
Li, L., Yu, T., Yuan, L., Doulgeraki, A. I., and Iseppi, R. (2022). Biofilm formation and quorum sensing of foodborne microorganism. Front. Microbiol. 13:1107603. doi: 10.3389/fmicb.2022.1107603
Li, T., Wang, D., Liu, N., Ma, Y., Ding, T., Mei, Y., et al. (2018). Inhibition of quorum sensing-controlled virulence factors and biofilm formation in Pseudomonas fluorescens by cinnamaldehyde. Int. J. Food Microbiol. 269, 98–106. doi: 10.1016/j.ijfoodmicro.2018.01.023
Liu, W., Lu, H., Chu, X., Lou, T., Zhang, N., Zhang, B., et al. (2020). Tea polyphenols inhibits biofilm formation, attenuates the quorum sensing-controlled virulence and enhances resistance to Klebsiella pneumoniae infection in Caenorhabditis elegans model. Microb. Pathog. 147:104266. doi: 10.1016/j.micpath.2020.104266
Lu, L., Li, M., Yi, G., Liao, L., Cheng, Q., Zhu, J., et al. (2022). Screening strategies for quorum sensing inhibitors in combating bacterial infections. J. Pharmaceut. Anal. 12, 1–14. doi: 10.1016/j.jpha.2021.03.009
MacMicking, J., Xie, Q. W., and Nathan, C. (1997). Nitric oxide and macrophage function. Ann. Rev. Immunol. 15, 323–350. doi: 10.1146/annurev.immunol.15.1.323
Mangwani, N., Kumari, S., and Das, S. (2016). Bacterial biofilms and quorum sensing: fidelity in bioremediation technology. Biotechnol. Genetic Eng. Rev. 32, 43–73. doi: 10.1080/02648725.2016.1196554
Miller, M. B., and Bassler, B. L. (2001). Quorum sensing in bacteria. Ann. Rev. Microbiol. 55, 165–199. doi: 10.1146/annurev.micro.55.1.165
Monzon, O., Yang, Y., Li, Q., and Alvarez, P. J. (2016). Quorum sensing autoinducers enhance biofilm formation and power production in a hypersaline microbial fuel cell. Biochem. Eng. J. 109, 222–227. doi: 10.1016/j.bej.2016.01.023
Munir, S., Shah, A. A., Shahid, M., Manzoor, I., Aslam, B., Rasool, M. H., et al. (2020). Quorum sensing interfering strategies and their implications in the management of biofilm-associated bacterial infections. Brazil. Archiv. Biol. Technol. 63:e20190555. doi: 10.1590/1678-4324-2020190555
Ng, W. L., and Bassler, B. L. (2009). Bacterial quorum-sensing network architectures. Ann. Rev. Genet. 43, 197–222. doi: 10.1146/annurev-genet-102108-134304
Ni, N., Li, M., Wang, J., and Wang, B. (2009). Inhibitors and antagonists of bacterial quorum sensing. Med. Res. Rev/ 29, 65–124. doi: 10.1002/med.20145
Nogaret, P., El Garah, F., and Blanc-Potard, A. B. (2021). A novel infection protocol in zebrafish embryo to assess Pseudomonas aeruginosa virulence and validate efficacy of a quorum sensing inhibitor in vivo. Pathogens 10:401. doi: 10.3390/pathogens10040401
Odularu, A. T., Afolayan, A. J., Sadimenko, A. P., Ajibade, P. A., and Mbese, J. Z. (2022). Multidrug-resistant biofilm, quorum sensing, quorum quenching, and antibacterial activities of indole derivatives as potential eradication approaches. BioMed Res. Int. 2022:9048245. doi: 10.1155/2022/9048245
Papaioannou, E., Utari, P. D., and Quax, W. J. (2013). Choosing an appropriate infection model to study quorum sensing inhibition in Pseudomonas infections. Int. J. Mol. Sci. 14, 19309–19340. doi: 10.3390/ijms140919309
Popat, R., Cornforth, D., McNally, L., and Brown, S. (2015). Collective sensing and collective responses in quorum-sensing bacteria. J. Royal Soc. Interf. 12:20140882. doi: 10.1098/rsif.2014.0882
Prescott, R. D., and Decho, A. W. (2020). Flexibility and adaptability of quorum sensing in nature. Trends Microbiol. 28, 436–444. doi: 10.1016/j.tim.2019.12.004
Qixin, L., Xuan, F., Zhiya, S., Wenxin, S., Shuo, W., Ji, L., et al. (2022). Enhanced wastewater treatment performance by understanding the interaction between algae and bacteria based on quorum sensing. Bioresour. Technol. 354:127161. doi: 10.1016/j.biortech.2022.127161
Rutherford, S. T., and Bassler, B. L. (2012). Bacterial quorum sensing: its role in virulence and possibilities for its control. Cold Spring Harbor Perspect. Med. 2:a012427. doi: 10.1101/cshperspect.a012427
Schluter, J., Schoech, A. P., Foster, K. R., and Mitri, S. (2016). The evolution of quorum sensing as a mechanism to infer kinship. PLoS Comput. Biol. 12:e1004848. doi: 10.1371/journal.pcbi.1004848
Scutera, S., Zucca, M., and Savoia, D. (2014). Novel approaches for the design and discovery of quorum-sensing inhibitors. Expert Opin. Drug Discov. 9, 353–366. doi: 10.1517/17460441.2014.894974
Sethupathy, S., Prasath, K. G., Ananthi, S., Mahalingam, S., Balan, S. Y., Pandian, S. K., et al. (2016). Proteomic analysis reveals modulation of iron homeostasis and oxidative stress response in Pseudomonas aeruginosa PAO1 by curcumin inhibiting quorum sensing regulated virulence factors and biofilm production. J. Proteom. 145, 112–126. doi: 10.1016/j.jprot.2016.04.019
Sharma, D., Misba, L., and Khan, A. U. (2019). Antibiotics versus biofilm: an emerging battleground in microbial communities. Antimicrob. Resist. Infect. Contr. 8, 1–10. doi: 10.1186/s13756-019-0533-3
Sharma, R., and Jangid, K. (2014). Fungal quorum sensing inhibitors, Quorum sensing vs. quorum quenching: a battle with no end in sight. Springer 20, 237–257. doi: 10.1007/978-81-322-1982-8_20
Shrout, J. D., and Nerenberg, R. (2012). Monitoring bacterial twitter: does quorum sensing determine the behavior of water and wastewater treatment biofilms? Environ. Sci. Technol. 46, 1995–2005. doi: 10.1021/es203933h
Siddiqui, M. F., Rzechowicz, M., Harvey, W., Zularisam, A. W., and Anthony, G. F. (2015). Quorum sensing based membrane biofouling control for water treatment: a review. J. Water Process Eng. 7, 112–122. doi: 10.1016/j.jwpe.2015.06.003
Sivasankar, P., Poongodi, S., Seedevi, P., Sivakumar, M., Murugan, T., Loganathan, S., et al. (2019). Bioremediation of wastewater through a quorum sensing triggered MFC: a sustainable measure for waste to energy concept. J. Environ. Manag. 237, 84–93. doi: 10.1016/j.jenvman.2019.01.075
Skandamis, P. N., and Nychas, G. J. E. (2012). Quorum sensing in the context of food microbiology. Appl. Environ. Microbiol. 78, 5473–5482. doi: 10.1128/AEM.00468-12
Steinberg, N., and Kolodkin-Gal, I. (2015). The matrix reloaded: how sensing the extracellular matrix synchronizes bacterial communities. J. Bacteriol. 197, 2092–2103. doi: 10.1128/JB.02516-14
Striednig, B., and Hilbi, H. (2022). Bacterial quorum sensing and phenotypic heterogeneity: how the collective shapes the individual. Trends Microbiol. doi: 10.1016/j.tim.2021.09.001
Subramani, R., and Jayaprakashvel, M. (2019). Bacterial quorum sensing: biofilm formation, survival behaviour and antibiotic resistance. Implicat. Quor. Sens. Biofilm Format. Med. Agri. Food Industry 3, 21–37. doi: 10.1007/978-981-32-9409-7_3
Sun, Y., Qin, H., Yan, Z., Zhao, C., Ren, J., Qu, X., et al. (2019). Combating biofilm associated infection in vivo: integration of quorum sensing inhibition and photodynamic treatment based on multidrug delivered hollow carbon nitride sphere. Adv. Funct. Mater. 29:1808222. doi: 10.1002/adfm.201808222
Tajani, A. S., Soheili, V., Moosavi, F., Ghodsi, R., Alizadeh, T., Bazzaz, B. S. F., et al. (2022). Ultra selective and high-capacity dummy template molecular imprinted polymer to control quorum sensing and biofilm formation of Pseudomonas aeruginosa. Analyt. Chim. Acta 1199:339574. doi: 10.1016/j.aca.2022.339574
Tay, S. B., and Yew, W. S. (2013). Development of quorum-based anti-virulence therapeutics targeting Gram-negative bacterial pathogens. Int. J. Mol. Sci. 14, 16570–16599. doi: 10.3390/ijms140816570
Vendeville, A., Winzer, K., Heurlier, K., Tang, C. M., and Hardie, K. R. (2005). Making'sense'of metabolism: autoinducer-2, LuxS and pathogenic bacteria. Nat. Rev. Microbiol. 3, 383–396. doi: 10.1038/nrmicro1146
Wallin, J. A. (2005). Bibliometric methods: pitfalls and possibilities. Basic Clin. Pharmacol. Toxicol. 97, 261–275. doi: 10.1111/j.1742-7843.2005.pto_139.x
Wang, B., and Muir, T. W. (2016). Regulation of virulence in Staphylococcus aureus: molecular mechanisms and remaining puzzles. Cell Chem. Biol. 23, 214–224. doi: 10.1016/j.chembiol.2016.01.004
Warrier, A., Satyamoorthy, K., and Murali, T. S. (2021). Quorum-sensing regulation of virulence factors in bacterial biofilm. Fut. Microbiol. 16, 1003–1021. doi: 10.2217/fmb-2020-0301
Wolf, D., Rippa, V., Mobarec, J. C., Sauer, P., Adlung, L., Kolb, P., et al. (2016). The quorum-sensing regulator ComA from Bacillus subtilis activates transcription using topologically distinct DNA motifs. Nucl. Acids Res. 44, 2160–2172. doi: 10.1093/nar/gkv1242
Wu, S., Feng, J., Liu, C., Wu, H., Qiu, Z., Ge, J., et al. (2022). Machine learning aided construction of the quorum sensing communication network for human gut microbiota. Nat. Commun. 13:3079. doi: 10.1038/s41467-022-30741-6
Zhang, Y., Ma, Q., Su, B., Chen, R., Lin, J., Lin, Z., et al. (2018). A study on the role that quorum sensing play in antibiotic-resistant plasmid conjugative transfer in Escherichia coli. Ecotoxicology 27, 209–216. doi: 10.1007/s10646-017-1886-0
Zhao, G., Usui, M. L., Lippman, S. I., James, G. A., Stewart, P. S., Fleckman, P., et al. (2013). Biofilms and inflammation in chronic wounds. Adv. Wound Care 2, 389–399. doi: 10.1089/wound.2012.0381
Zhao, J., Quan, C., Jin, L., and Chen, M. (2018). Production, detection and application perspectives of quorum sensing autoinducer-2 in bacteria. J. Biotechnol. 268, 53–60. doi: 10.1016/j.jbiotec.2018.01.009
Zhou, L., Zhang, Y., Ge, Y., Zhu, X., and Pan, J. (2020). Regulatory mechanisms and promising applications of quorum sensing-inhibiting agents in control of bacterial biofilm formation. Front. Microbiol. 11:589640. doi: 10.3389/fmicb.2020.589640
Keywords: quorum sensing, research trends, bibliometric analysis, biclustering analysis, visualization
Citation: Chen X, Li J, Liao R, Shi X, Xing Y, Xu X, Xiao H and Xiao D (2024) Bibliometric analysis and visualization of quorum sensing research over the last two decade. Front. Microbiol. 15:1366760. doi: 10.3389/fmicb.2024.1366760
Received: 07 January 2024; Accepted: 25 March 2024;
Published: 05 April 2024.
Edited by:
Robert Czajkowski, University of Gdansk, PolandReviewed by:
Anju V. T., Pondicherry University, IndiaVinothkannan Ravichandran, Amity University, Mumbai, India
Sahana Vasudevan, Institute for Stem Cell Science and Regenerative Medicine (InStem), India
Copyright © 2024 Chen, Li, Liao, Shi, Xing, Xu, Xiao and Xiao. This is an open-access article distributed under the terms of the Creative Commons Attribution License (CC BY). The use, distribution or reproduction in other forums is permitted, provided the original author(s) and the copyright owner(s) are credited and that the original publication in this journal is cited, in accordance with accepted academic practice. No use, distribution or reproduction is permitted which does not comply with these terms.
*Correspondence: Haitao Xiao, aHh5eXhodCYjeDAwMDQwOzE2My5jb20=; Dongqin Xiao, eGlhb2RvbmdxaW4mI3gwMDA0MDtuc21jLmVkdS5jbg==
†These authors have contributed equally to this work