- 1College of Agronomy, Yanbian University, Yanji, China
- 2College of Integration Science, Yanbian University, Yanji, China
- 3College of Agronomy and Biotechnology, China Agricultural University, Beijing, China
- 4College of Life Science, Northeast Forestry University, Harbin, China
Low temperature is one of the limiting factors for anaerobic digestion in cold regions. To improve the efficiency of anaerobic digestion for methane production in stationary reactors under low-temperature conditions, and to improve the structure of the microbial community for anaerobic digestion at low temperatures. We investigated the effects of different concentrations of exogenous Methanomicrobium (10, 20, 30%) and different volumes of carbon fiber carriers (0, 10, 20%) on gas production and microbial communities to improve the performance of low-temperature anaerobic digestion systems. The results show that the addition of 30% exogenous microorganisms and a 10% volume of carbon fiber carrier led to the highest daily (128.15 mL/g VS) and cumulative (576.62 mL/g VS) methane production. This treatment effectively reduced the concentrations of COD and organic acid, in addition to stabilizing the pH of the system. High-throughput sequencing analysis revealed that the dominant bacteria under these conditions were Acidobacteria and Firmicutes and the dominant archaea were Candidatus_Udaeobacter and Methanobacterium. While the abundance of microorganisms that metabolize organic acids was reduced, the functional abundance of hydrogenophilic methanogenic microorganisms was increased. Therefore, the synergistic effect of Methanomicrobium bioaugmentation with carbon fiber carriers can significantly improve the performance and efficiency of low-temperature anaerobic fermentation systems.
1 Introduction
Low-temperature anaerobic fermentation utilizes microorganisms to break down organic matter and produce methane at low temperatures (<30°C) (Quispe-Cardenas and Rogers, 2021; Jaimes-Estévez et al., 2023). Traditional anaerobic digestion is typically conducted at moderate-to-high temperatures (30–60°C) (Rusín et al., 2021). In comparison with the high-temperature anaerobic process, low-temperature anaerobic fermentation does not require additional reactor heating, which can significantly reduce energy consumption and operational costs (Rajagopal et al., 2017). In a typical anaerobic digestion process, organic acid metabolic methanogenesis is different significantly from hydrogen metabolic methanogenesis in terms of mechanism and environmental tolerance (Liu et al., 2022). Organic acid metabolic pathways account for 72% of the total methane production, while H2 and CO2 metabolic pathways contribute only 28%. Organic acid metabolism accounts for a higher percentage, but its reaction process requires more microorganisms to participate, so the microbial activity will be significantly reduced at low temperatures, thus affecting the efficiency of gas production (Hupfauf et al., 2018; Nie et al., 2021). Another factor limiting the efficiency of low-temperature gas production is the excessive accumulation of toxic substances (Lu et al., 2023). This is because the low-temperature tolerance of methanogenic microorganisms is weak compared with that of organic acid-producing microorganisms, which leads to the fact that organic acids cannot be decomposed into methane promptly (Holohan et al., 2022; Liu et al., 2023), and this also causes a negative feedback effect in the digestive system (Kotsyurbenko, 2005). So how improving the low-temperature tolerance of methanogenic microorganisms is one of the effective ways to solve the problem of low-temperature anaerobic digestion.
Many previous studies have used bioaugmentation to improve the efficiency of anaerobic digestion (Jang et al., 2018; Lovato et al., 2021). Bioaugmentation is a method for improving degradation reactions by adding specific microorganisms or microbial communities (Li et al., 2018). Application of bioaugmentation to anaerobic fermentation can effectively enhance the degradation of organic matter and increase methane production (Xu et al., 2022; Yan et al., 2023), Therefore, we expect to improve the limitations of low-temperature anaerobic digestion systems using bioaugmentation. Previous studies have shown that Methanomicrobium occurs in relatively high abundance in low-temperature anaerobic digestion reactions and shows a strong correlation with methane production (Lv et al., 2019; Yue et al., 2021). Our earlier experimental results showed that Methanomicrobium exhibited significant enrichment on carbon fiber carriers (Zhao et al., 2013), which usually have a developed porous structure and a large surface area that can provide abundant attachment points for microorganisms (Abbas et al., 2021). This not only directly increases the number of microorganisms in the reactor, but also provides a comfortable and stable living environment for the microorganisms, which is conducive to the formation of Methanomicrobium (Yang et al., 2017; Zhang et al., 2017). Meanwhile, Methanomicrobium exhibits high biological activity on carbon fiber carriers, therefore, we consider combining the Methanomicrobium bioaugmentation with the carbon fiber carriers to construct an anaerobic digestive system that has a stronger adaptive ability to low-temperature environments.
To investigate whether biological augmentation with Methanomicrobium and the addition of carbon fiber carriers can enhance the performance of low-temperature anaerobic digestion systems, we conducted preliminary work involving the isolation and purification of Methanomicrobium for use as an exogenous microbial enhancer. Simultaneously, different quantities of carbon fiber carriers were introduced to improve the performance of low-temperature anaerobic digestion and increase methane production. We evaluated the impact of different microbial concentrations and numbers of carbon fiber carriers on the reaction efficiency to determine the optimal dosages. Furthermore, we examined changes in the microbial community within the reaction system to understand the structure and functional characteristics. Our results provide a theoretical basis for optimization strategies in low-temperature anaerobic digestion.
2 Materials and methods
2.1 Experimental setup and procedure
The experiment utilized a fixed-bed reactor made of synthetic glass (10 cm in outer diameter, 18 cm in height, and an effective volume of 1 L). The reactor was operated under anaerobic conditions with a one-time feed setup.
To regulate the operating temperature, the bioreactor was placed inside a biochemical constant-temperature chamber (Model MIR 254, Sanyo, Japan) maintained at 20°C.
Gas volume was measured using the drainage method, and regular samples were taken from the reactor outlet to determine the concentrations of volatile fatty acids (VFAs), chemical oxygen demand (COD), pH, and methane.
In the reactor, cylindrical carbon fiber fabric was added as a biofilm carrier (outer diameter of 3 cm and a height of 5 cm, supplied by the Japanese Carbon Company in Tokyo, Japan). The height of the carbon fiber fabric and the liquid level were kept consistent. Each cylindrical carbon fiber carrier occupied 10% of the effective volume of the reactor. Three gradients were set: 0, 10, and 20% carbon fiber carriers, labeled cv0, cv10, and cv20, respectively.
2.2 Reactants, inoculants, and bioaugmentation strains
The reaction substrate was an artificially synthesized glucose wastewater composed of water, syrup (homogeneity: 70%, solid content: 45%), and industrial feed (mainly protein, fat, and crude fiber). The COD: N:P ratio was maintained at 300–500 5:1 (Zhang et al., 2012).
The initial inoculum was obtained from laboratory-cultured activated sludge that had been long-term acclimated at 20°C. It was passed through a 1 mm sieve to remove larger solids. The resulting inoculum had a total solid (TS) content of 48.82% and a volatile solid (VS) content of 4.56%, sludge inoculum was controlled at 250 g for all treatment groups.
The biological augmentation strain was laboratory-purified L2, which was isolated and purified from the sludge of wetlands in Yanbian Prefecture, Jilin Province, China (latitude 43°45′22″ N, longitude 128°44′10″ E). This strain is Gram-negative, rod-shaped, strictly anaerobic, and belongs to the Methanomicrobium, which can produce methane via both H2 and CO2 metabolism and organic acid metabolism (Liu and Whitman, 2008).
Three different microbial liquid concentration gradients were established, namely 10, 20, and 30%, corresponding to 30 mL, 60 mL, and 90 mL added microbial liquid, respectively. The total volume of microbial liquid added to the culture medium was controlled at 90 mL. The control group used a sterile culture medium without microbial liquid. Finally, artificial glucose wastewater was used to finalize the volume to 600 mL. Each experiment was repeated three times.
2.3 Analytical methods
VFAs were determined using high-performance liquid chromatography (LC-MS2020, Japan), COD was measured using a water quality monitoring instrument (Lovi-bond 99731COD, Germany), pH was determined using a compact pH meter from Horiba, and CH4 levels were measured using a biogas analyzer (Model ADG, Landtec, United States).
2.4 Microbial analysis
Sludge samples were taken from the reactor at predetermined time points, sample DNA was extracted using the MN NucleoSpin 96 Soil DNA extraction kit. Specific amplification of the bacterial 16S V3 + V4 variable region was carried out using the primers 338F (5′-ACTCCTACGGGAGGCAGCA-3′) and 806R (5′-GGACTACHVGGGTWTCTAAT-3′). For archaea, the 16S V3 + V4 variable region was specifically amplified using the primers Arch349F (5′-GYGCASCAGKCGMGAAW-3′) and Arch806R (5′-GGACTACVSGGGTATCTAAT-3′). PCR products were quantitated by gel electrophoresis (ImageJ software), and samples were mixed at a 1:1 ratio based on their mass. DNA was then purified using the OMEGA DNA nucleic acid purification kit. After purification, a library was constructed for high-throughput sequencing, which was provided by Beijing BioMighty Biotech Co., Ltd.
A bioinformatics analysis was performed using the BioMighty cloud platform. Beta diversity distances were calculated using QIIME (version 1.9.1) and alpha diversity analysis was performed using Mothur v. 1.30. The igraph, Hmisc, pheatmap, and vegan packages were used to organize and initially map the data in the R 4.2.1 environment.
3 Results and discussion
3.1 Performance of low-temperature fixed-bed digesters with different microbial liquid concentrations and carrier volumes
Figure 1 compares the performance of low-temperature anaerobic digestion in fixed-bed reactors at different microbial liquid concentrations (control, 10, 20, and 30%) and effective volumes of carbon fiber carriers (0, 10, and 20%). Over a 28 days period, the daily methane production in all treatment groups was higher than that in the control group, and methane production in each treatment group reached its peak on day 21.
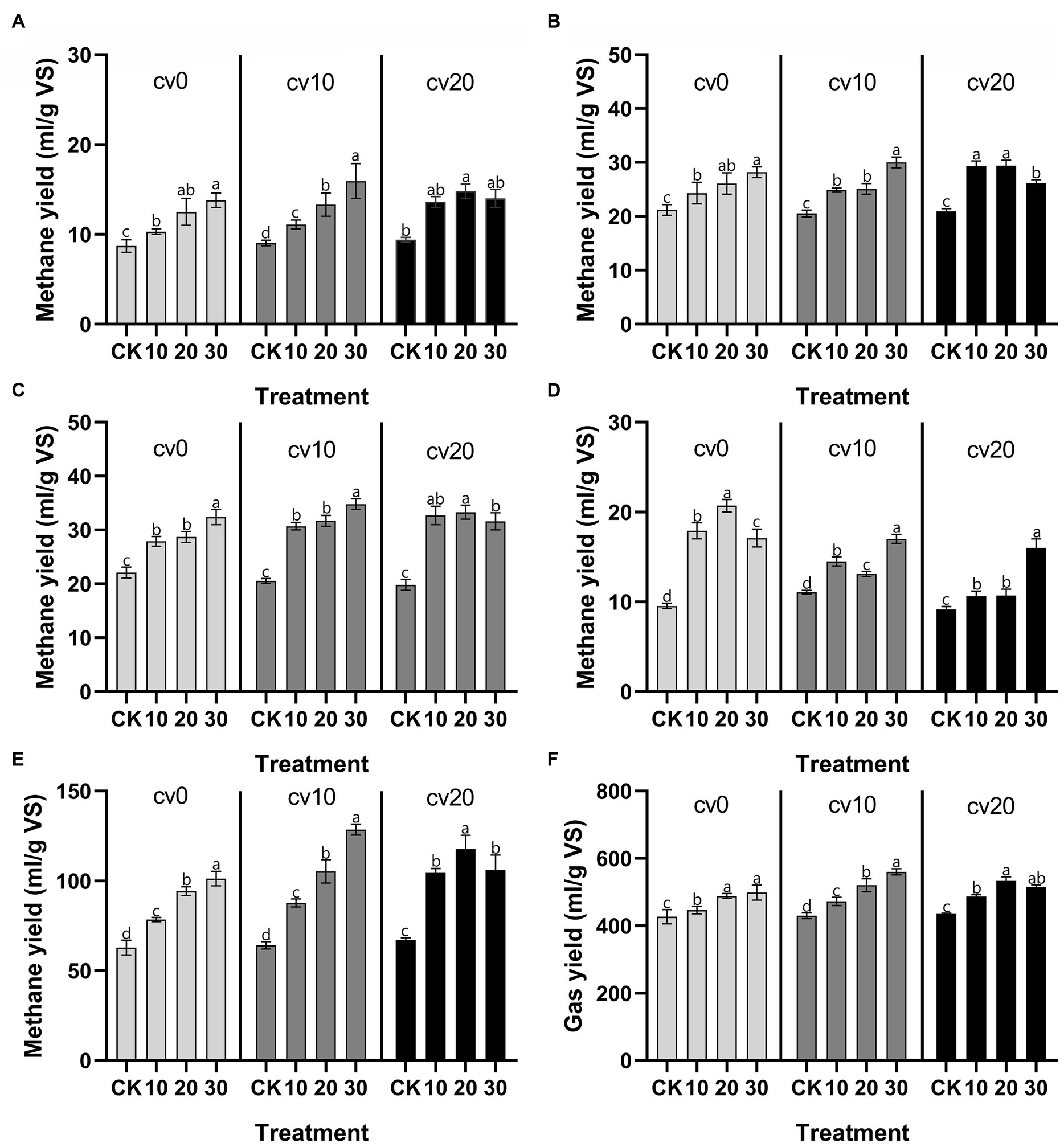
Figure 1. Changes in methane gas production. Daily methane production at 7 days (A), 14 days (B), 21 days (C), and 28 days (D). Cumulative methane production throughout the experiment (E). Cumulative total gas production throughout the experiment (F). cv0 represents the treatment without fiber carriers, cv10 represents a carrier volume of 10%, and cv20 represents a carrier volume of 20%.
As the microbial liquid concentration increased, daily methane production also increased. After the addition of carbon fiber carriers, the daily methane production in the low-concentration microbial liquid treatment group reached a higher level, especially in the first 21 days of the experimental period. In anaerobic digestion processes, low temperatures generally limit bacterial metabolism and reaction kinetics (Zhou et al., 2018); however, the low energy consumption advantage of low-temperature anaerobic digestion makes it valuable for research related to anaerobic digestion in cold regions (Martí-Herrero et al., 2022). Previous studies have shown that the addition of a reasonable amount of exogenous microorganisms through bioaugmentation can effectively optimize the efficiency of anaerobic digestion systems (Martin-Ryals et al., 2015; Li et al., 2022; Linsong et al., 2022). Our results demonstrate that carbon fiber carriers can to some extent reduce the cost of bioaugmentation, implying that carbon fiber carriers can lower the concentration requirements for microbial liquid in fixed reactors while achieving high methane production.
In addition, the cumulative methane production data indicate that bioaugmentation with Methanomicrobiaceae effectively enhances the total cumulative methane production in the system and that the cumulative methane production is directly proportional to the microbial liquid concentration. In the treatment group with a 20% carrier volume, the methane production at the highest microbial liquid concentration was relatively reduced; however, in the treatment group with a 10% carrier volume, 30% microbial liquid resulted in the highest methane and total gas production, suggesting that the excessive addition of carbon fiber carriers inhibits the gas production efficiency of the system.
Furthermore, as shown in Figure 2, the COD removal efficiency in all treatment groups was higher than that in the control group at all time points. The COD removal efficiency was directly proportional to the microbial liquid concentration. In the treatment group with a 10% carbon fiber carrier volume and 30% microbial liquid, the maximum COD removal efficiency was 93.48%. In the treatment group with a 20% carbon fiber carrier volume, the COD removal efficiency remained relatively consistent during the latter stages of the experiment. Regarding the anaerobic fermentation process, the pH value in all treatment groups fluctuated between 7.0 and 8.0, which is within the suitable pH range for anaerobic digestion (Zhang et al., 2010). Additionally, the pH value in the treatment groups was lower than that in the control group, indicating that bioaugmentation microorganisms and carrier addition effectively improved the environmental conditions in the reaction system.
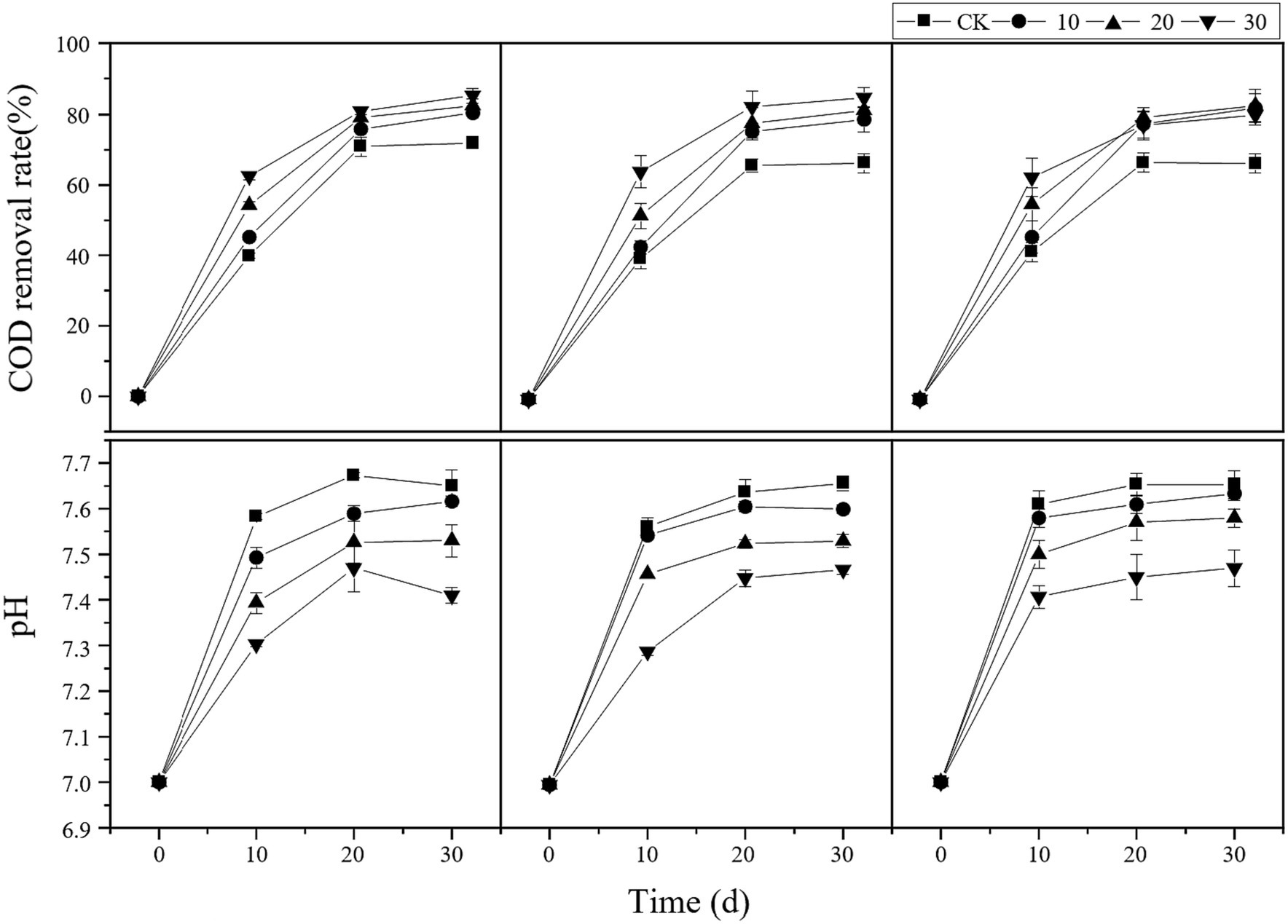
Figure 2. Changes in reactor COD and pH. cv0 represents treatment without fiber carriers, cv10 represents a carrier volume of 10%, cv20 represents a carrier volume of 20%, and different shapes represent different microbial liquid concentrations.
As shown in Figure 3, the organic acid concentration in each treatment group was significantly higher than that in the control group at the beginning of the reaction. As digestion progressed, the concentrations of formic acid and acetic acid significantly decreased, indicating that the reaction system effectively inhibited the generation of organic acids and maintained them at a relatively stable level.
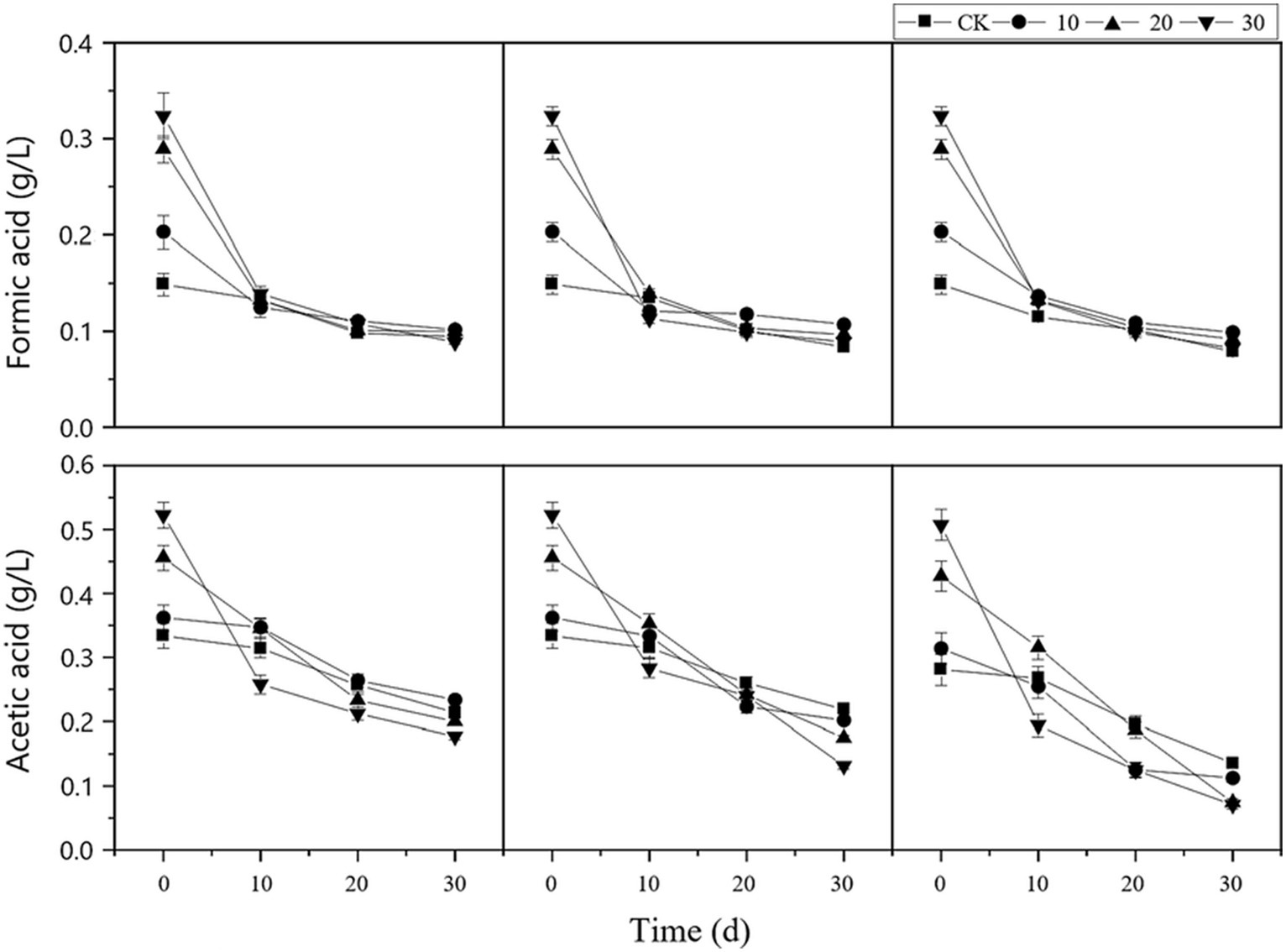
Figure 3. Changes in organic acid concentration in the reactor. cv0 represents treatment without fiber carriers, cv10 represents a carrier volume of 10%, cv20 represents a carrier volume of 20%, and different shapes represent different microbial liquid concentrations.
3.2 Bacterial and archaeal community structure in different treatment groups
Figure 4A illustrates the top 10 phyla in terms of abundance in each treatment group. At 0% carrier volume, the abundance of Acidobacteria, Proteobacteria, and Actinobacteria increased with increasing microbial concentration. By contrast, the abundance of Firmicutes, Bacteroidetes, and Caldiserica decreased with increasing microbial concentration. The addition of carbon fiber carriers reduced the abundance of Acidobacteria and Firmicutes while significantly increasing the abundance of Proteobacteria.
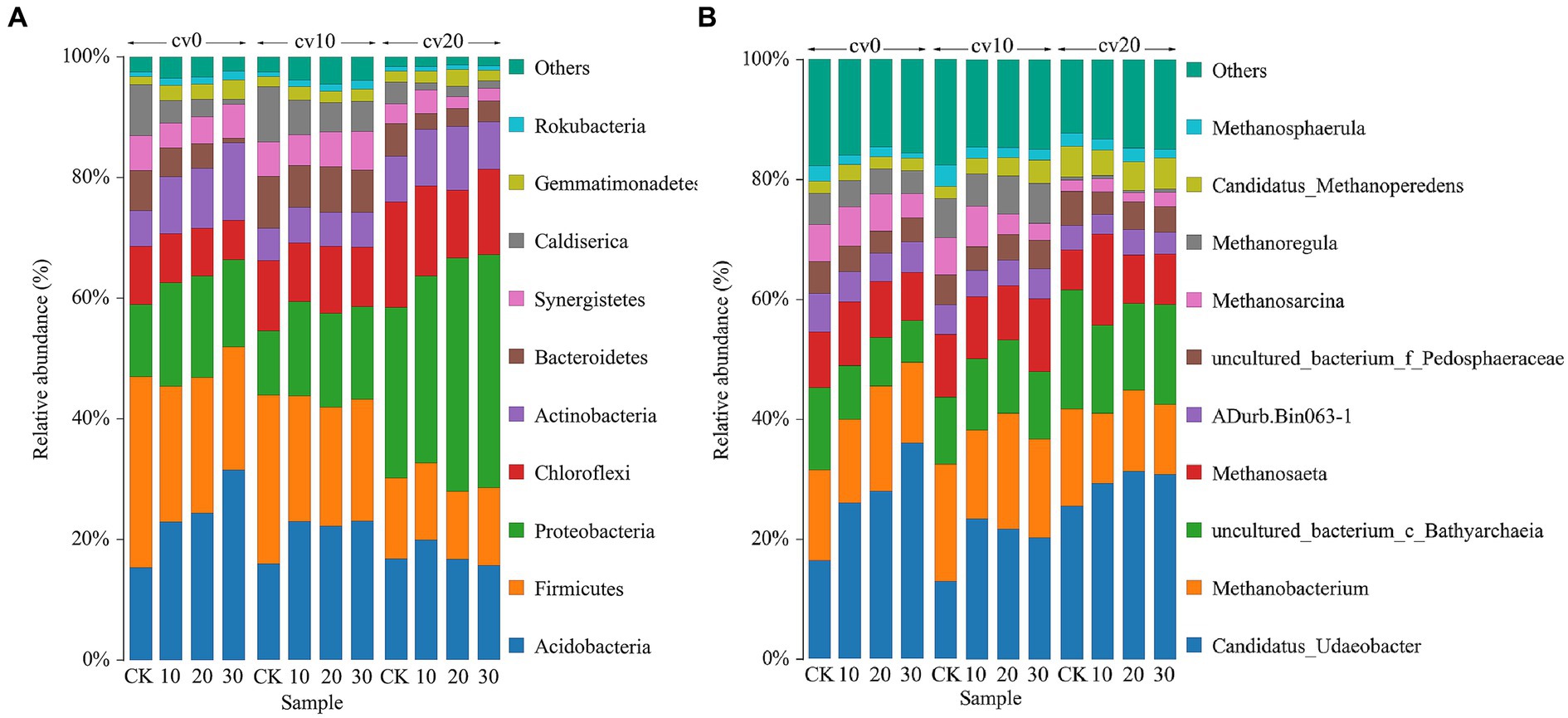
Figure 4. The bacterial (A) and archaeal (B) community structures in different treatment groups. Different colors represent different species classifications, and species with less than 5% abundance were combined with others.
Figure 4B displays the archaeal community structure in each treatment group. At cv0, the abundance of Candidatus_Udaeobacter increased with the concentration of microbial agents, while the abundance of Bathyarchaeia decreased. Previous reports suggest that Candidatus_Udaeobacter contains highly affinitive hydrogenases that can metabolize H2 to provide energy for the respiratory chain under nutrient-limited conditions (Greening et al., 2015; Willms et al., 2020). On the other hand, Bathyarchaeia are known for their autotrophic acetogenic metabolism capabilities (Xie et al., 2022; Hou et al., 2023). This indicates that following the addition of Methanomicrobiaceae, which produce methane through H2 and CO2 metabolism pathways, the microbial community in the reaction system gradually shifts toward these pathways, while the proportion of microorganisms utilizing organic acids to produce methane decreases. These findings are by the organic acid measurements, further demonstrating that bioaugmentation with Methanomicrobiaceae effectively reduces the biological production of organic acids and enhances the efficiency of methane production via H2 and CO2 pathways. At cv20, the abundance of Bathyarchaeia was relatively higher in comparison with that at cv10, suggesting that an excessive amount of fiber carriers can alter the community composition and reduce methane production.
3.3 Different treatments exhibit significantly different species
LEFse was used to assess the phylogenetic tree and LDA scores of bacteria and archaea in different carbon fiber carrier ratio treatments, as shown in Figure 5. The significantly different bacteria in the treatment without carbon fiber carriers included Acidobacteria and Clostridiaceae_1, which have the highest LDA scores. At 10% effective carrier volume, the significantly different bacteria were Synergistetes and Bacteroidetes, with Bacteroidetes having the highest LDA score. At 20% effective carrier volume, the different bacteria were Chloroflexota and Proteobacteria, with Proteobacteria having the highest LDA score.
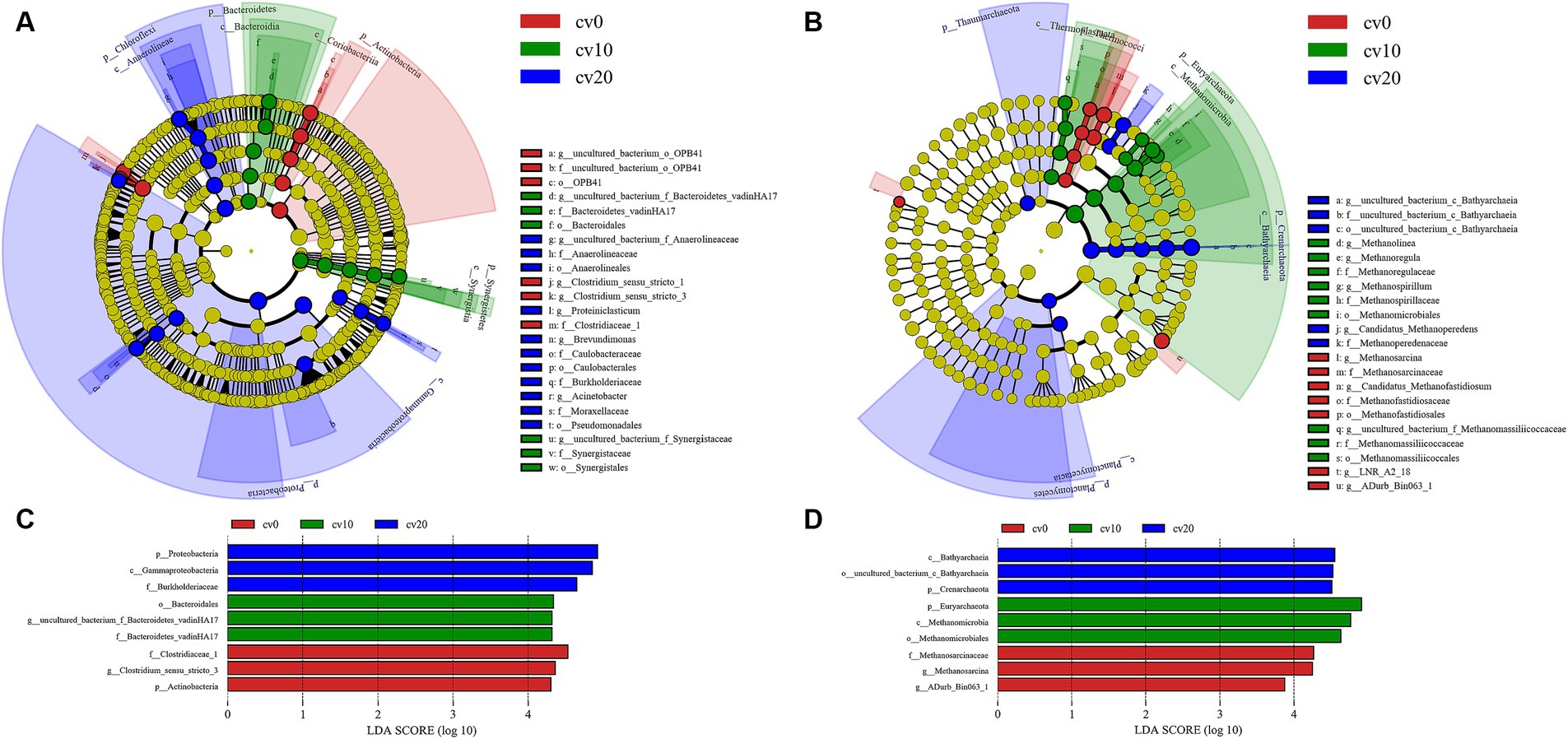
Figure 5. LEFse analysis of bacteria and archaea in different treatment groups. LEFse dendrogram for (A) bacteria and (B) archaea. LDA scores for (C) bacteria and (D) archaea.
The phylogenetic tree of archaea in different treatments is displayed in Figure 5. In all treatments, Methanobacterium-related species consistently exhibited a significantly dominant position. This phenomenon was more pronounced at 10% effective carrier volume; while at 20% effective carrier volume, Bathyarchaeia was present in more marked abundance. This further validates the earlier results showing differences in acetate and methane production.
3.4 FAPROTAX functional gene prediction
FAPROTAX functional gene prediction can annotate and predict microbial community functions based on the taxonomic classification of 16S sequences. In Figure 6, the horizontal axis represents the samples, and the vertical axis represents the functional groups of the top 10 dominant bacteria in the microbial community. Among these groups, The abundance of hydrogenotrophic_methanogenesis increased to different degrees in all treatment groups, implying that the addition of Methanomicrobium strains significantly improved the methanogenic functioning of the anaerobic digestive system, with 30% exogenous microorganisms and a 10% volume of carbon fiber carrier had the highest functional abundance of hydrogenotrophic_methanogenesis, thus suggesting that the specific biofortification with carbon fiber carrier can maintain stable methanogenic efficiency at low temperatures.
4 Conclusion
The addition of 30% methane microbial inoculum and 10% by volume carbon fiber carriers significantly increased the daily and cumulative methane production during anaerobic digestion in fixed-bed reactors at low temperatures. This method effectively reduced COD and organic acids in the system and stabilized the pH. Microbial community analysis and functional prediction indicate that the addition of Methanomicrobiaceae inoculum enhanced the abundance of methane-metabolizing microorganisms in the system, reduced the abundance of acetate-metabolizing microorganisms, and increased the proportion of hydrogenotrophic metabolism. These modifications led to higher methane production under low-temperature conditions.
Data availability statement
The original contributions presented in the study are included in the article/supplementary material, further inquiries can be directed to the corresponding author.
Author contributions
YW: Conceptualization, Data curation, Formal analysis, Methodology, Software, Validation, Visualization, Writing – original draft, Writing – review & editing. XA: Data curation, Formal analysis, Investigation, Methodology, Supervision, Validation, Writing – review & editing. JW: Data curation, Formal analysis, Investigation, Methodology, Validation, Writing – review & editing. XJ: Data curation, Investigation, Supervision, Validation, Writing – review & editing. XL: Data curation, Formal analysis, Investigation, Methodology, Writing – review & editing. JY: Investigation, Methodology, Supervision, Validation, Writing – review & editing. WW: Project administration, Supervision, Validation, Writing – review & editing. JP: Conceptualization, Methodology, Project administration, Supervision, Writing – review & editing. HZ: Conceptualization, Data curation, Funding acquisition, Methodology, Project administration, Resources, Supervision, Validation, Writing – review & editing. ZC: Project administration, Resources, Supervision, Validation, Writing – review & editing.
Funding
The author(s) declare financial support was received for the research, authorship, and/or publication of this article. This research was supported by the National Science Foundation of China (Nos. 51741809 and 81960686), the Special Fund for Agro-Scientific Research in the Public Interest (No. 201503137), the Department of Natural Science Fund Project of Jilin Province (No. YDZJ202201ZYTS578), and the Jilin Provincial Education Department Project (No. JJKH20240688HT).
Conflict of interest
The authors declare that the research was conducted in the absence of any commercial or financial relationships that could be construed as a potential conflict of interest.
Publisher’s note
All claims expressed in this article are solely those of the authors and do not necessarily represent those of their affiliated organizations, or those of the publisher, the editors and the reviewers. Any product that may be evaluated in this article, or claim that may be made by its manufacturer, is not guaranteed or endorsed by the publisher.
References
Abbas, Y., Yun, S., Wang, Z., Zhang, Y., Zhang, X., and Wang, K. (2021). Recent advances in bio-based carbon materials for anaerobic digestion: a review. Renew. Sust. Energy Rev. 135:110378. doi: 10.1016/j.rser.2020.110378
Greening, C., Constant, P., Hards, K., Morales, S. E., Oakeshott, J. G., Russell, R. J., et al. (2015). Atmospheric hydrogen scavenging: from enzymes to ecosystems. Appl. Environ. Microbiol. 81, 1190–1199. doi: 10.1128/AEM.03364-14
Holohan, B. C., Duarte, M. S., Szabo-Corbacho, M. A., Cavaleiro, A. J., Salvador, A. F., Pereira, M. A., et al. (2022). Principles, advances, and perspectives of anaerobic digestion of lipids. Environ. Sci. Technol. 56, 4749–4775. doi: 10.1021/acs.est.1c08722
Hou, J. L., Wang, Y. Z., Zhu, P. F., Yang, N., Liang, L. W., Yu, T. T., et al. (2023). Taxonomic and carbon metabolic diversification of Bathyarchaeia during its coevolution history with early Earth surface environment. Sci. Adv. 9:eadf5069. doi: 10.1126/sciadv.adf5069
Hupfauf, S., Plattner, P., Wagner, A. O., Kaufmann, R., Insam, H., and Podmirseg, S. M. (2018). Temperature shapes the microbiota in anaerobic digestion and drives efficiency to a maximum at 45°C. Bioresour. Technol. 269, 309–318. doi: 10.1016/j.biortech.2018.08.106
Jaimes-Estévez, J., Martí-Herrero, J., Poggio, D., Zafra, G., Gómez, K., Escalante, H., et al. (2023). The role of biochar in the psychrophilic anaerobic digestion: effects on kinetics, acids metabolism, and microbial population. Bioresour. Technol. Rep. 23:101566. doi: 10.1016/j.biteb.2023.101566
Jang, H. M., Choi, Y.-K., and Kan, E. (2018). Effects of dairy manure-derived biochar on psychrophilic, mesophilic and thermophilic anaerobic digestions of dairy manure. Bioresour. Technol. 250, 927–931. doi: 10.1016/j.biortech.2017.11.074
Kotsyurbenko, O. R. (2005). Trophic interactions in the methanogenic microbial community of low-temperature terrestrial ecosystems. FEMS Microbiol. Ecol. 53, 3–13. doi: 10.1016/j.femsec.2004.12.009
Li, Y., Li, L., Sun, Y., and Yuan, Z. (2018). Bioaugmentation strategy for enhancing anaerobic digestion of high C/N ratio feedstock with methanogenic enrichment culture. Bioresour. Technol. 261, 188–195. doi: 10.1016/j.biortech.2018.02.069
Li, M.-T., Rao, L., Wang, L., Gou, M., Sun, Z.-Y., Xia, Z.-Y., et al. (2022). Bioaugmentation with syntrophic volatile fatty acids-oxidizing consortia to alleviate the ammonia inhibition in continuously anaerobic digestion of municipal sludge. Chemosphere 288:132389. doi: 10.1016/j.chemosphere.2021.132389
Linsong, H., Lianhua, L., Ying, L., Changrui, W., and Yongming, S. (2022). Bioaugmentation with methanogenic culture to improve methane production from chicken manure in batch anaerobic digestion. Chemosphere 303:135127. doi: 10.1016/j.chemosphere.2022.135127
Liu, X., Lu, Q., Du, M., Xu, Q., and Wang, D. (2022). Hormesis-like effects of Tetrabromobisphenol A on anaerobic digestion: responses of metabolic activity and microbial community. Environ. Sci. Technol. 56, 11277–11287. doi: 10.1021/acs.est.2c00062
Liu, Y.-C., Ramiro-Garcia, J., Paulo, L. M., Braguglia, C. M., Gagliano, M. C., and O'Flaherty, V. (2023). Psychrophilic and mesophilic anaerobic treatment of synthetic dairy wastewater with long chain fatty acids: process performances and microbial community dynamics. Bioresour. Technol. 380:129124. doi: 10.1016/j.biortech.2023.129124
Liu, Y., and Whitman, Y. (2008). Metabolic, phylogenetic, and ecological diversity of the methanogenic archaea. Ann. N. Y. Acad. Sci 1125, 171–189. doi: 10.1196/annals.1419.019
Lovato, G., Kovalovszki, A., Alvarado-Morales, M., Arjuna Jéglot, A. T., Rodrigues, J. A. D., and Angelidaki, I. (2021). Modelling bioaugmentation: engineering intervention in anaerobic digestion. Renew. Energy 175, 1080–1087. doi: 10.1016/j.renene.2021.04.096
Lu, Q., He, D., Liu, X., Du, M., Xu, Q., and Wang, D. (2023). 1-butyl-3-methylimidazolium chloride affects anaerobic digestion through altering organics transformation, cell viability, and microbial community. Environ. Sci. Technol. 57, 3145–3155. doi: 10.1021/acs.est.2c08004
Lv, Z., Wu, X., Zhou, B., Wang, Y., Sun, Y., Wang, Y., et al. (2019). Effect of one step temperature increment from mesophilic to thermophilic anaerobic digestion on the linked pattern between bacterial and methanogenic communities. Bioresour. Technol. 292:121968. doi: 10.1016/j.biortech.2019.121968
Martí-Herrero, J., Castro, L., Jaimes-Estévez, J., Grijalva, M., Gualatoña, M., Aldás, M. B., et al. (2022). Biomethane potential test applied to psychrophilic conditions: three issues about inoculum temperature adaptation. Bioresour. Technol. Rep. 20:101279. doi: 10.1016/j.biteb.2022.101279
Martin-Ryals, A., Schideman, L., Li, P., Wilkinson, H., and Wagner, R. (2015). Improving anaerobic digestion of a cellulosic waste via routine bioaugmentation with cellulolytic microorganisms. Bioresour. Technol. 189, 62–70. doi: 10.1016/j.biortech.2015.03.069
Nie, E., He, P., Zhang, H., Hao, L., Shao, L., and Lü, F. (2021). How does temperature regulate anaerobic digestion? Renew. Sust. Energy Rev. 150:111453. doi: 10.1016/j.rser.2021.111453
Quispe-Cardenas, E., and Rogers, S. (2021). Microbial adaptation and response to high ammonia concentrations and precipitates during anaerobic digestion under psychrophilic and mesophilic conditions. Water Res. 204:117596. doi: 10.1016/j.watres.2021.117596
Rajagopal, R., Bellavance, D., and Rahaman, M. S. (2017). Psychrophilic anaerobic digestion of semi-dry mixed municipal food waste: for north American context. Process Saf. Environ. Prot. 105, 101–108. doi: 10.1016/j.psep.2016.10.014
Rusín, J., Chamrádová, K., and Basinas, P. (2021). Two-stage psychrophilic anaerobic digestion of food waste: comparison to conventional single-stage mesophilic process. Waste Manag. 119, 172–182. doi: 10.1016/j.wasman.2020.09.039
Willms, I. M., Rudolph, A. Y., Göschel, I., Bolz, S. H., Schneider, D., Penone, C., et al. (2020). Globally abundant “Candidatus udaeobacter” benefits from release of antibiotics in soil and potentially performs trace gas scavenging. mSphere 5:e00186. doi: 10.1128/msphere.00186-20
Xie, Z., Cao, Q., Chen, Y., Luo, Y., Liu, X., and Li, D. (2022). The biological and abiotic effects of powdered activated carbon on the anaerobic digestion performance of cornstalk. Bioresour. Technol. 343:126072. doi: 10.1016/j.biortech.2021.126072
Xu, X., Sun, Y., Sun, Y., and Li, Y. (2022). Bioaugmentation improves batch psychrophilic anaerobic co-digestion of cattle manure and corn straw. Bioresour. Technol. 343:126118. doi: 10.1016/j.biortech.2021.126118
Yan, M., Wang, C., Li, Y., Tian, H., and Sun, Y. (2023). Effect of bioaugmentation on psychrotrophic anaerobic digestion: bioreactor performance, microbial community, and cellular metabolic response. Chem. Eng. J. 455:140173. doi: 10.1016/j.cej.2022.140173
Yang, Y., Zhang, Y., Li, Z., Zhao, Z., Quan, X., and Zhao, Z. (2017). Adding granular activated carbon into anaerobic sludge digestion to promote methane production and sludge decomposition. J. Clean. Prod. 149, 1101–1108. doi: 10.1016/j.jclepro.2017.02.156
Yue, Y., Wang, J., Wu, X., Zhang, J., Chen, Z., Kang, X., et al. (2021). The fate of anaerobic syntrophy in anaerobic digestion facing propionate and acetate accumulation. Waste Manag. 124, 128–135. doi: 10.1016/j.wasman.2021.01.038
Zhang, P., Chen, Y., Zhou, Q., Zheng, X., Zhu, X., and Zhao, Y. (2010). Understanding short-chain fatty acids accumulation enhanced in waste activated sludge alkaline fermentation: kinetics and microbiology. Environ. Sci. Technol. 44, 9343–9348. doi: 10.1021/es102878m
Zhang, J., Mao, L., Zhang, L., Loh, K.-C., Dai, Y., and Tong, Y. W. (2017). Metagenomic insight into the microbial networks and metabolic mechanism in anaerobic digesters for food waste by incorporating activated carbon. Sci. Rep. 7:11293. doi: 10.1038/s41598-017-11826-5
Zhang, D., Zhu, W., Tang, C., Suo, Y., Gao, L., Yuan, X., et al. (2012). Bioreactor performance and methanogenic population dynamics in a low-temperature (5–18°C) anaerobic fixed-bed reactor. Bioresour. Technol. 104, 136–143. doi: 10.1016/j.biortech.2011.10.086
Zhao, H., Li, J., Li, J., Yuan, X., Piao, R., Zhu, W., et al. (2013). Organic loading rate shock impact on operation and microbial communities in different anaerobic fixed-bed reactors. Bioresour. Technol. 140, 211–219. doi: 10.1016/j.biortech.2013.04.027
Keywords: bioaugmentation, anaerobic digestion, carbon carrier, methane production, microbial community
Citation: Wang Y, An X, Wang J, Jiang X, Li X, Yin J, Wang W, Piao J, Zhao H and Cui Z (2024) Effect of bioaugmentation on gas production and microbial community during anaerobic digestion in a low-temperature fixed-bed reactor. Front. Microbiol. 15:1365289. doi: 10.3389/fmicb.2024.1365289
Edited by:
Xiaowu Huang, Guangdong Technion-Israel Institute of Technology (GTIIT), ChinaReviewed by:
Xuran Liu, Hunan University, ChinaSeung Gu Shin, Gyeongsang National University, Republic of Korea
Jianliang Xue, Shandong University of Science and Technology, China
Copyright © 2024 Wang, An, Wang, Jiang, Li, Yin, Wang, Piao, Zhao and Cui. This is an open-access article distributed under the terms of the Creative Commons Attribution License (CC BY). The use, distribution or reproduction in other forums is permitted, provided the original author(s) and the copyright owner(s) are credited and that the original publication in this journal is cited, in accordance with accepted academic practice. No use, distribution or reproduction is permitted which does not comply with these terms.
*Correspondence: Hongyan Zhao, emh5QHlidS5lZHUuY24=; Jin Piao, cGlhb2ppbkB5YnUuZWR1LmNu
†These authors share first authorship