- 1Division of Plant Pathology, ICAR-Indian Agricultural Research Institute, New Delhi, India
- 2Division of Genetics, ICAR-Indian Agricultural Research Institute, New Delhi, India
Spot blotch disease incited by Bipolaris sorokiniana severely affects the cultivation of barley. The resistance to B. sorokiniana is quantitative in nature and its interaction with the host is highly complex which necessitates in-depth molecular analysis. Thus, the study aimed to conduct the transcriptome analysis to decipher the mechanisms and pathways involved in interactions between barley and B. sorokiniana in both the resistant (EC0328964) and susceptible (EC0578292) genotypes using the RNA Seq approach. In the resistant genotype, 6,283 genes of Hordeum vulgare were differentially expressed out of which 5,567 genes were upregulated and 716 genes were downregulated. 1,158 genes of Hordeum vulgare were differentially expressed in the susceptible genotype, out of which 654 genes were upregulated and 504 genes were downregulated. Several defense-related genes like resistant gene analogs (RGAs), disease resistance protein RPM1, pathogenesis-related protein PRB1-2-like, pathogenesis-related protein 1, thaumatin-like protein PWIR2 and defensin Tm-AMP-D1.2 were highly expressed exclusively in resistant genotype only. The pathways involved in the metabolism and biosynthesis of secondary metabolites were the most prominently represented pathways in both the resistant and susceptible genotypes. However, pathways involved in MAPK signaling, plant-pathogen interaction, and plant hormone signal transduction were highly enriched in resistant genotype. Further, a higher number of pathogenicity genes of B. sorokiniana was found in response to the susceptible genotype. The pathways encoding for metabolism, biosynthesis of secondary metabolites, ABC transporters, and ubiquitin-mediated proteolysis were highly expressed in susceptible genotype in response to the pathogen. 14 and 11 genes of B. sorokiniana were identified as candidate effectors from susceptible and resistant host backgrounds, respectively. This investigation will offer valuable insights in unraveling the complex mechanisms involved in barley- B. sorokiniana interaction.
1 Introduction
Spot blotch incited by Bipolaris sorokiniana (Teleomorph: Cochliobolus sativus) is a significant disease affecting barley production. Barley in grown worldwide in an area of 47.14 million hectares with an annual production of 154.88 million tonnes (FAO, 2022). In India, barley occupies an area of 0.45 million hectares with an annual production of 1.37 million tonnes (FAO, 2022). The disease is considered a serious threat in the hot and humid South-Asian regions (Chaurasia et al., 2000; Kumar et al., 2007; Chand et al., 2008; Vaish et al., 2011). A yield loss of 25–45% has been reported in barley due to the pathogen, which has the potential to escalate even more under conducive environments (Iftikhar et al., 2009). In Indian context, yield losses can extent to 53% (Vaish et al., 2011). Changes in agricultural methods and the quick substitution of locally adapted varieties with high-yielding cultivars have resulted in the emergence of spot blotch (Bala and Kaur, 2008). This disease poses a threat to barley cultivation in the future and can cause devastating losses under the climate change scenario.
Bipolaris sorokiniana is a hemi-biotrophic pathogen causing spot blotch on foliar parts, black point on grains, and root rot symptoms. The symptoms are characterized by necrotic lesions accompanied by chlorosis, severe cases lead to the complete drying of leaves. The pathogen is seed, soil-borne and can spread by air-borne conidia making it one of the most destructive pathogens (Gupta et al., 2018). It produces thick-walled conidia and has a wide host range attacking various other cereals and weed hosts. This pathogen shows high genetic variability which can be attributed to parasexuality and heterokaryosis (Glass et al., 2000). The pathogenicity determinants of B. sorokiniana include factors like higher melanin content, cell wall degrading enzymes, and the presence of toxic compounds (Jahani et al., 2006; Chand et al., 2014; Aich et al., 2017). The genome size of Bipolaris sorokiniana (ND90Pr) is 34.42 Mb (Condon et al., 2013). Being a hemibiotrophic pathogen, it consists of an early biotrophic phase where effector proteins are employed to counteract host defense responses (Kaladhar et al., 2023). In the wheat pathosystem, effectors like BsToxA, CsSp1and BsCE66 have been found to govern virulence in B. sorokiniana (Friesen et al., 2018; Zhang et al., 2022; Kaladhar et al., 2023). Few studies have also reported the VHv1 gene to be responsible for governing virulence in barley (Zhong et al., 2002).
This disease can be managed by the use of chemical fungicides belonging to the triazole group. However, as chemicals cause a negative impact on the environment, the use of resistant cultivars remains the most feasible option. Few genes like Rcs 5, Rcs 6, and a few QTLs have been identified in governing resistance to spot blotch in barley. Some reports suggested that mlo gene-based resistance to powdery mildew caused by Blumeria graminis results in susceptibility to B. sorokiniana in barley (Kumar et al., 2001; Leng et al., 2020). However, the presence of complex factors and the quantitative nature of spot blotch resistance makes breeding programs challenging and time-consuming. Additionally, the classical gene for gene model is not the principal system operating in the H. vulgare-B. sorokiniana pathosystem (Ghazvini and Tekauz, 2008). Thus, there is a need for in-depth elucidation of genetic factors responsible for governing resistance. In addition, the elucidation of pathogen factors responsible for virulence will provide greater insights into the molecular basis of B. sorokiniana-Hordeum vulgare interaction.
Keeping in view, we investigated the transcriptome of resistant and susceptible genotypes of barley with challenge inoculation of B. sorokiniana to investigate the mechanisms associated and identified a few defense-related genes. In addition, differentially expressed genes (DEGs), pathways involved in defense response, and pathogenicity factors involved in virulence were identified. The present investigation will offer valuable understanding in unraveling the complex pathogenic mechanisms involved in barley- B. sorokiniana interaction.
2 Materials and methods
2.1 Fungal material
A virulent isolate (BS 52, NCBI accession number OR262940) of B. sorokiniana inciting spot blotch of barley was established in the Fungal Molecular Biology Laboratory, Division of Plant Pathology, ICAR-Indian Agricultural Research Institute, New Delhi. B. sorokiniana was maintained in test tubes containing Potato dextrose agar media in an incubator at 25°C. Inoculum was multiplied by inoculation of B. sorokiniana in sorghum grains for 15–20 days at 25°C followed by preparation of spore suspension (104 conidia/ml). The in-vitro transcriptome of B. sorokiniana was assessed and compared to the in-planta transcriptome. For pathogen mycelia, B. sorokiniana was inoculated in potato dextrose broth media and kept at 25°C in a shaker incubator (Kuhner Lab-Therm). The fungal mycelia were extracted after 10 days of incubation and kept in a deep freezer (−80°C) until further study.
2.2 Experimental genotypes and growth conditions
The resistant (EC0328964) and susceptible (EC0578292) genotypes (ICAR-NBPGR, New Delhi) were taken for RNA-seq analysis. The genotypes were sown in 4-inch pots containing an equal proportion of sand, soil and FYM under net house conditions. Two replications for each treatment (control and inoculated) were maintained. After 30 days, the plants were inoculated with B. sorokiniana (BS 52) spore solution (104 conidia/ml) and kept in a moisture chamber (100% humidity). The control plants were inoculated with sterile distilled water. After inoculation, leaves were sampled at 0 h, 12 h, 24 h, and 36 h and kept in aluminum foil, and immediately frozen in liquid nitrogen. Then the inoculated leaves were kept in a deep freezer (−80°C) for further study.
2.3 RNA isolation, library preparation, and RNA sequencing
The transcriptome analysis was performed for resistant and susceptible genotypes of barley with challenge inoculation of the pathogen. Two biological replicates for each treatment were considered for the RNA sequencing. Total RNA was extracted from the leaves and the B. sorokiniana mycelia using the Trizol method with slight modifications. The double-strand cDNA quality was checked using the Qubit dsDNA estimation and agarose gel. Pooling of the samples (different time points) for each treatment was done. The libraries for all the samples were prepared using the NEB Next UltraII DNA library preparation kit for the Illumina sequencing. The DNA underwent fragmentation to achieve an insert size of approximately 300–400 base pairs per sample. Subsequently, the fragmented DNA was repaired at the ends, followed by A-tailing, and ligation of indexed adapters. The resulting products were purified, and PCR amplification was conducted to produce the final library. The quantification of the library was carried out using the Qubit Fluorometer (Invitrogen, Life Technologies, Grand Island, NY, USA). The distribution of fragments within the library was checked on the HSDNA kit using the Tape station (Agilent Technologies, USA). The DNA libraries, now tagged, were combined in equal proportions, and loaded onto the c-bot automated system for the cluster generation. The sequencing was conducted in the S4 flow cell of the Illumina NOVASEQ 6000 platform by 150 bp paired-end. Post-sequencing, the samples were demultiplexed, and the sequences associated with indexed adapters were removed using the CASAVA v1.8.2 software (Illumina Inc.).
2.4 Read quality check and adapter trimming
The FastQC software was used to perform a quality assessment of the reads. The parameters like base quality score distribution, sequence quality score distribution, average base content per read and GC distribution in the reads were examined. The Universal Illumina Adapters (AGATCGGAAGAGC) were eliminated using the trim galore (version 0.6.2), and sequences shorter than 20 base pairs were excluded. Apart from removing adapters, low-quality ends of the reads were trimmed, maintaining a phred score of 20.
2.5 Differential gene expression, functional annotation, and effector prediction
The reference genomes of Hordeum vulgare (Morex; GenBank assembly: GCA_904849725; Mascher et al., 2021) and B. sorokiniana (ND90Pr; GenBank accession: AEIN00000000; Condon et al., 2013) were used. Indexing of these reference genomes were conducted through BWA (version 0.7.5). The adapter-trimmed sequences were aligned to the Hordeum vulgare reference genome using the bwa mem algorithm with default parameters (version 0.7.5). The mapped and unmapped sequences were separated using the SAMTOOLS (version 0.1.19). The unmapped sequences were subsequently aligned to the reference genome of B. sorokiniana using the SAMTOOLS. The count of reads mapped to genes was determined using the SAMTOOLS (version 0.1.19). For differential gene expression analysis, all possible combinations were assessed using the DESeq (version 1), an R package. Transcripts exhibiting a minimum two-fold difference in the gene expression and a p-value of ≤0.05 were selected for further analysis. The gene annotation was performed using the Blast2GO1 (Gotz et al., 2008) and the pathways were identified using the KEGG database2 (Kanehisa and Goto, 2000). For the prediction of effectors from the B. sorokiniana transcripts, the following prediction pipeline is used sequentially (Zhang et al., 2020). The transcripts were checked for the presence of N-terminal signal peptide using the Signal P5.0 server.3 The selected sequences were then checked for subcellular localization using the Target P2.0 server4 followed by TMHMM 2.0 server5 to check for the presence of transmembrane domain. The filtered sequences were checked with the EffectorP 3.0 server6 for the prediction of effectors.
3 Results
3.1 Data statistics and mapping of reads generated from RNA-sequencing
To understand the mechanisms and key genes involved during B. sorokiniana infection in barley, transcriptome analysis was performed using the RNA Seq approach. A total of 333.40 million reads were generated from Illumina sequencing. The raw reads were deposited to the NCBI SRA database (Accession no: PRJNA996376). The reads were mapped to the reference genome of barley (Morex) and the unmapped reads were then mapped to the reference genome of B. sorokiniana (ND90Pr). The reads ranged from 13.6 million to 77.7 million across samples. The mapping percentage ranged from 72.79 to 98.55% having a read length of 159 bp. The GC content ranged from 48–54% (Table 1).
3.2 Analysis of genes of Hordeum vulgare
3.2.1 Differential gene expression analysis (DEGs)
DEGs were assessed in both the combinations of resistant control-resistant inoculated (RC_RI) and susceptible control-susceptible inoculated (SC_SI). In the resistant genotype (EC0328964), 6,283 genes were differentially expressed with a p-value of ≤0.05 and log2 fold, out of which 5,567 genes were upregulated and 716 genes were downregulated. In the susceptible genotype (EC0578292), 1,158 genes were differentially expressed with a p-value of≤0.05 and log2 fold, out of which 654 genes were upregulated, and 504 genes were downregulated (Figure 1). Between resistant and susceptible combinations, 350 genes were commonly expressed, 5,933 genes were exclusively expressed in resistant genotype and 808 genes were exclusively expressed in the susceptible genotype (Figure 2) (Supplementary Table S1).
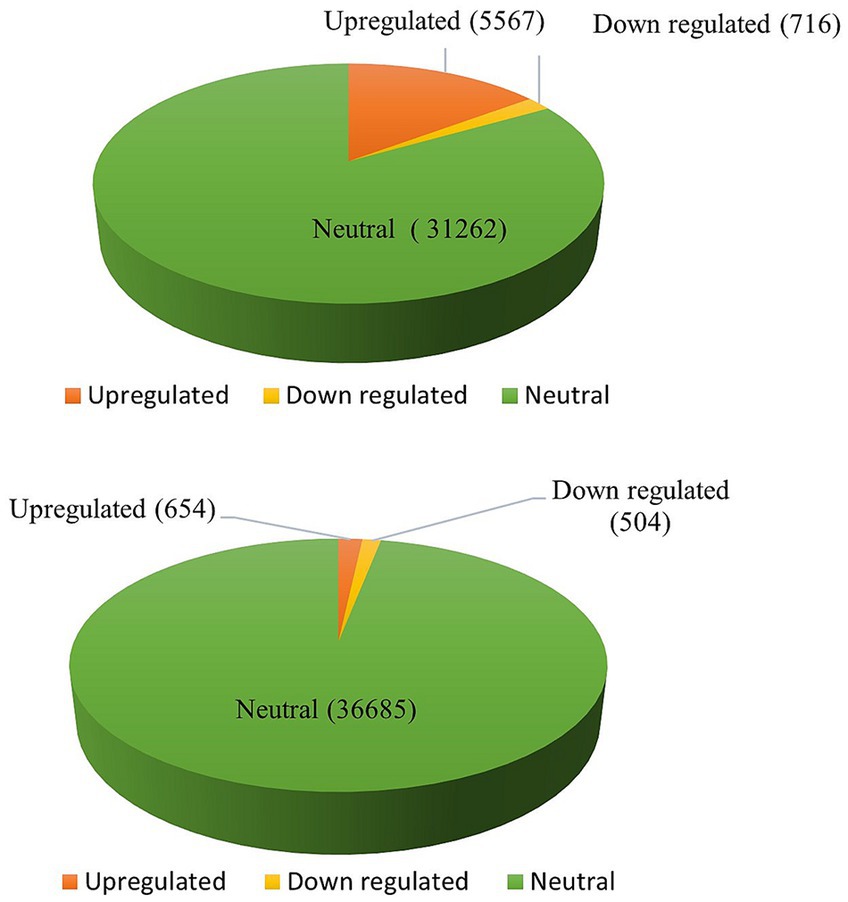
Figure 1. Differential gene expression analysis (top) resistant genotype (EC0328964) and (bottom) susceptible genotype (EC0578292) of barley upon infection of B. sorokiniana.
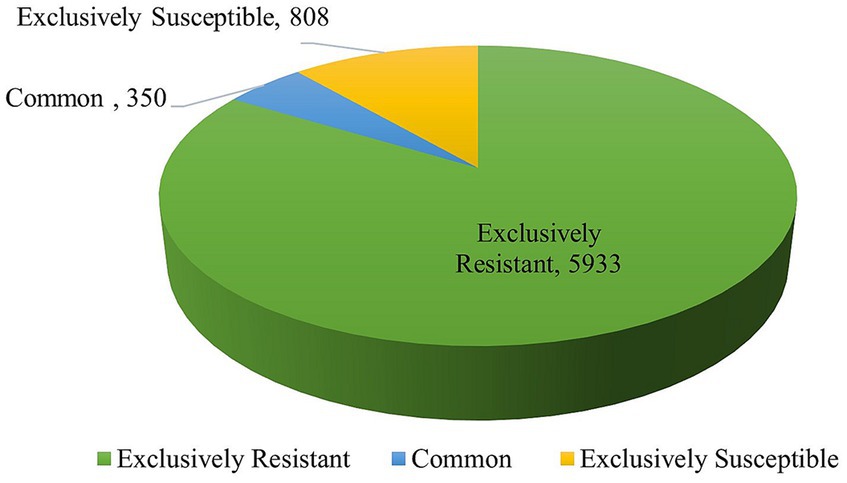
Figure 2. Common and unique genes between resistant and susceptible genotypes of barley upon infection of B. sorokiniana.
3.2.2 Gene ontology and functional annotation
The gene ontology analysis was conducted to determine the functions of the potential differentially expressed genes (DEGs). In the resistant genotype (EC0328964) upon B. sorokiniana inoculation, 10,740 DEGs were attributed to biological process, 9,637 DEGs to the cellular component, and 7,591 DEG’s to molecular functions. A high proportion of DEGs were attributed to biological process in resistant genotype. In the biological process, most DEGs were attributed to the organic substance metabolic process (1,445), primary metabolic process (1,393), and cellular metabolic process (1,344), apart from that a high number of DEGs were attributed to response to stress and biotic stimulus (Figure 3). In the cellular component category, most of the DEGs were attributed to cell part (1,314), intracellular (1,125), intracellular part (1,122), and membrane part (1,048). In the molecular function category, most of the DEGs were attributed to organic cyclic compound binding (1,458), heterocyclic compound binding (1,458), ion binding (1,104), and transferase activity (675).
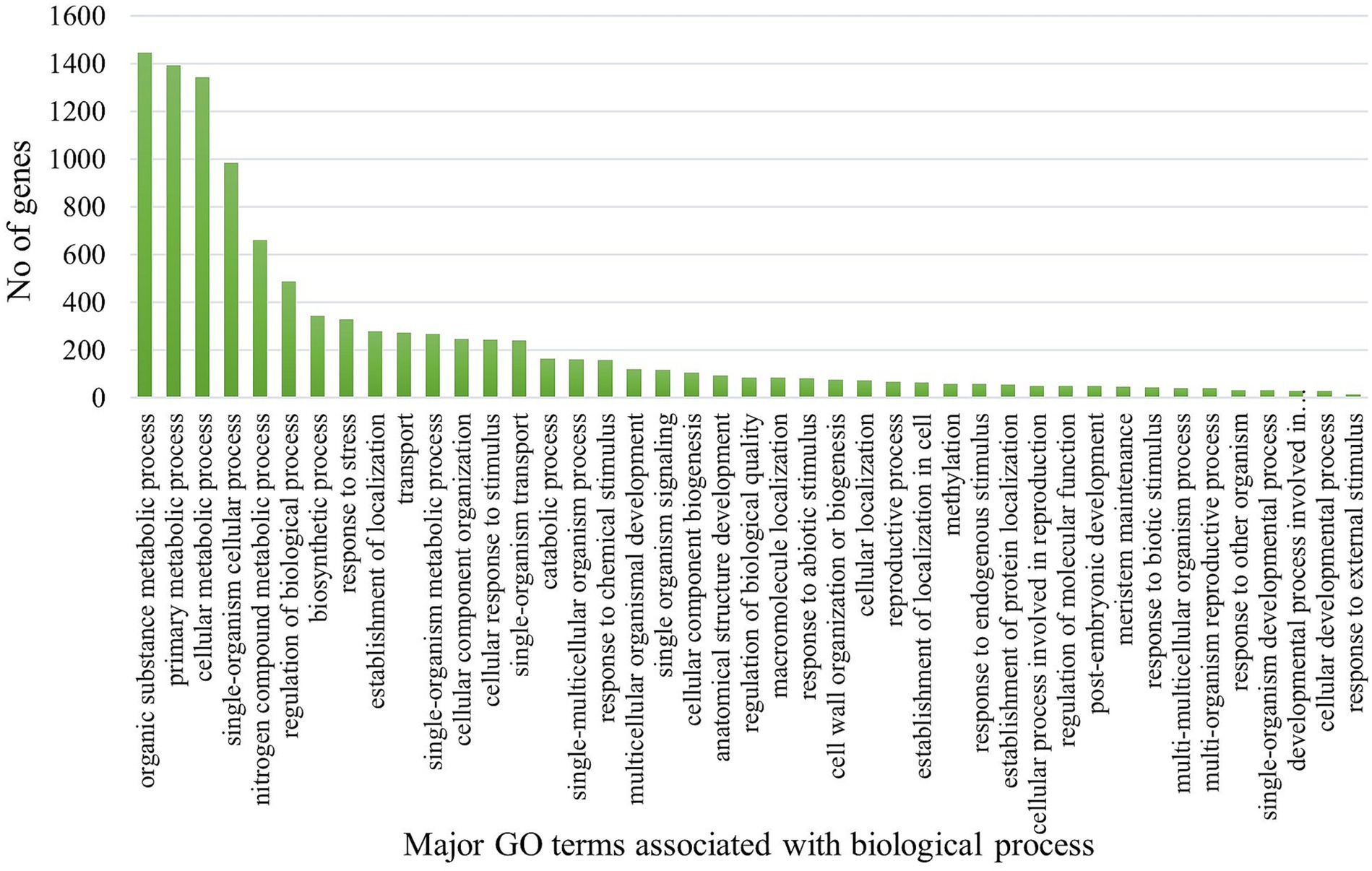
Figure 3. Distribution of genes across GO categories (biological process) in resistant genotype (EC0328964) upon infection of B. sorokiniana.
In the susceptible genotype (EC0578292), 2,618 DEGs were attributed to biological process, 2,704 DEGs were attributed to cellular component and 1,768 DEGs were attributed to molecular functions. The highest number of DEG’s were found in cellular components but almost an equal proportion to biological process in the susceptible genotype (Figure 4). In the biological process category, most DEGs were attributed to the organic substance metabolic process (326), cellular metabolic process (316), and primary metabolic process (299). In the cellular component category, most of the DEGs were attributed to cell part (392), intracellular (338), intracellular part (337), and intracellular organelle (270). In the molecular functions category, most of the DEGs were attributed to organic cyclic compound binding (280), heterocyclic compound binding (280), ion binding (272), and transferase activity (166). It indicated that a higher number of DEGs were present in the resistant genotype compared to the susceptible genotype pointing toward the role of genetic factors in governing resistance to spot blotch disease. In KEGG pathway analysis, metabolic pathways and biosynthesis of secondary metabolites were the most overrepresented in both the resistant and susceptible genotypes. However, the number of DEGs for both pathways (metabolic and biosynthesis of secondary metabolites) was higher in resistant than susceptible genotype (Supplementary Table S2). In addition, a higher number of DEGs in plant-pathogen interaction, plant hormone signal transduction, and MAPK signaling pathways were found in the resistant genotype (Figure 5).
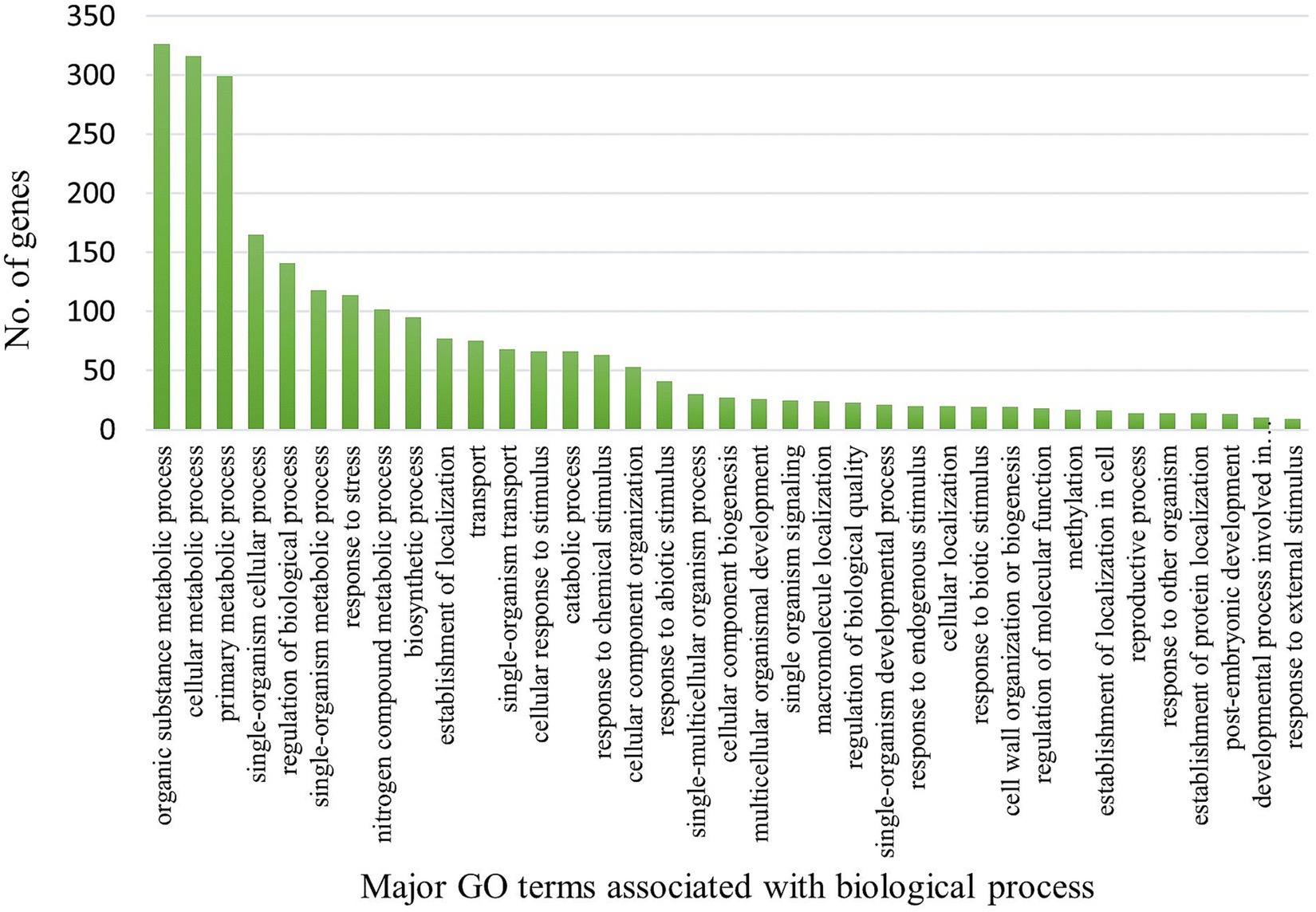
Figure 4. Distribution of genes across GO categories (biological process) in susceptible genotype (EC0578292) upon infection of B. sorokiniana.
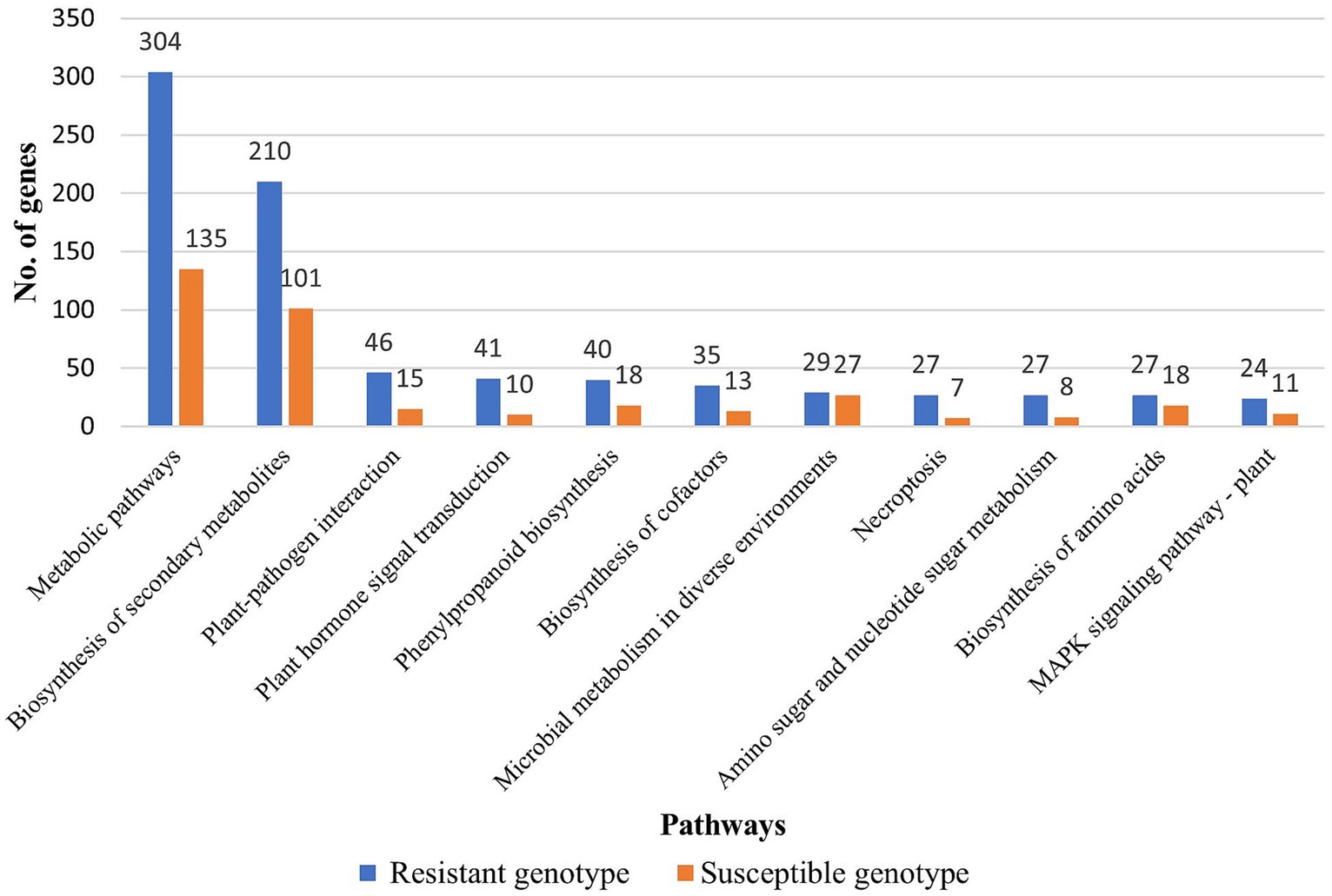
Figure 5. Major KEGG pathways identified in resistant and susceptible genotype of barley upon pathogen inoculation.
3.2.3 Defense-related genes in resistant genotype
The top up and down-regulated genes in the resistant genotype (EC0328964) were found based on log2 fold analysis. The highly up-regulated genes exhibited the functions viz. cation/H (+) antiporter-like, hypothetical protein, predicted protein, ribulose bisphosphate carboxylase, and hypothetical protein. The highly down-regulated genes function like Histone H3.2, low molecular mass early light-inducible protein HV90, ergosterol biosynthetic protein 28-like, histone H4, and high molecular mass early light-inducible protein HV58 (Table 2). The genes coding for defense responses in resistant genotype were identified based on GO terms like response to biotic stimulus, defense response, and response to stress in biological process (Table 3). Genes coding for RGAs (Resistance gene analogs) were highly upregulated in resistance genotypes like disease resistance protein RGA2-like (7-fold), disease resistance protein RGA5-like isoform X1 (6-fold), putative disease resistance protein RGA3 (6-fold), disease resistance protein RGA5-like isoform X1 (6-fold) and putative disease resistance protein RGA4 (6-fold). Other upregulated disease resistance protein were At1g50180 (7-fold), RPM1 (6-fold), RPH8A (6-fold), Pik-2-like (6-fold), PIK6-NP-like (6-fold), PIK6-NP (6-fold), NBS-LRR protein (4-fold), and Pyricularia oryzae resistance 21 protein (4-fold). Various PR proteins were also found to be upregulated like thaumatin-like protein PWIR2 (6-fold), pathogenesis-related protein 1(5-fold), pathogenesis-related protein PRB1-2-like (5-fold), and defensin Tm-AMP-D1.2-like (3-fold). Other genes like diacylglycerol kinase 4-like (5-fold), proline-rich protein HaeIII subfamily 1-like (5-fold), protein SRC2-like (4-fold), MLO protein homolog 1(4-fold) and transcription factor TCP14-like (3-fold) were found to be upregulated.
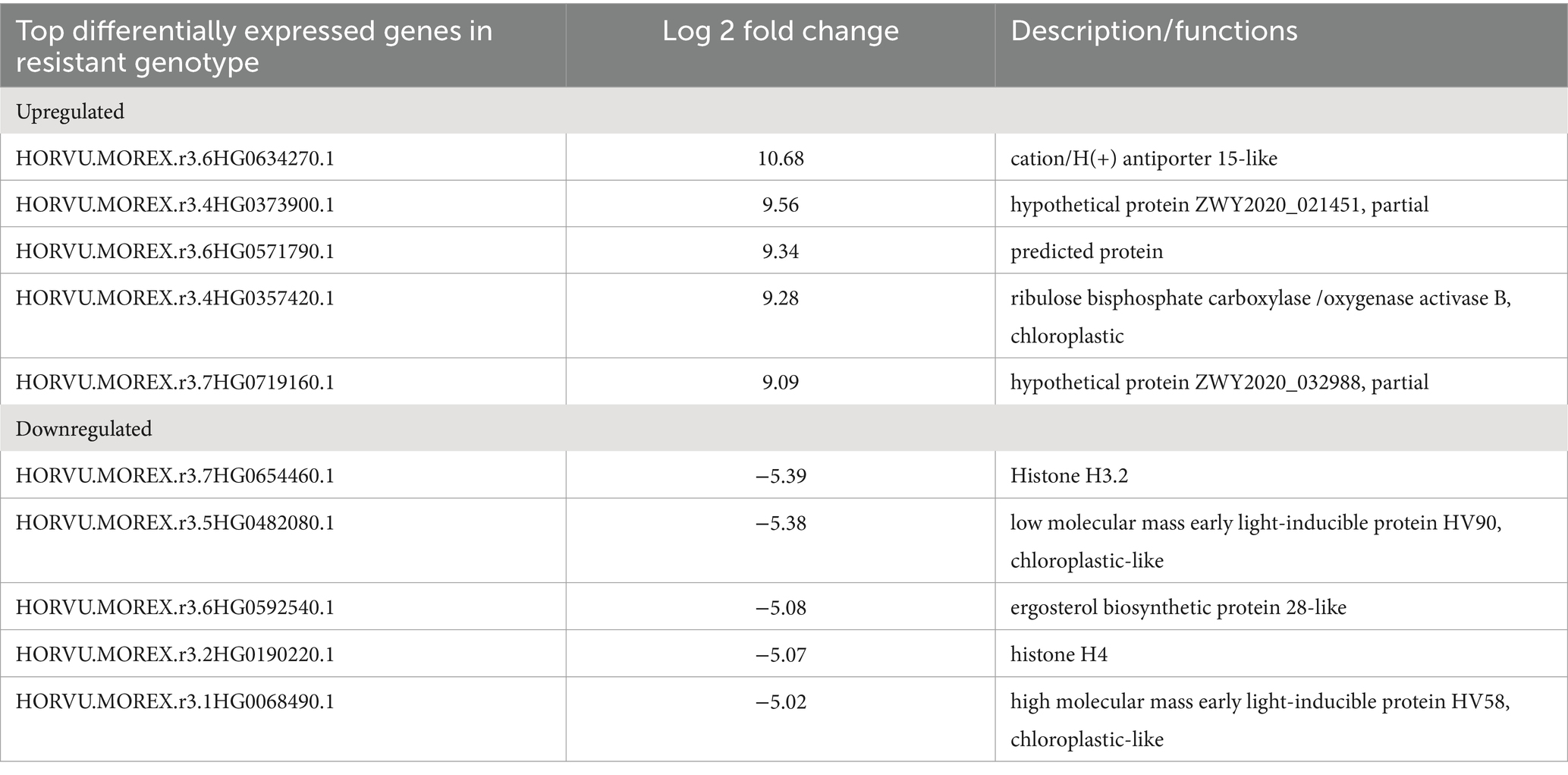
Table 2. Top differentially expressed genes based on log2 fold change in resistant genotype (EC0328964).
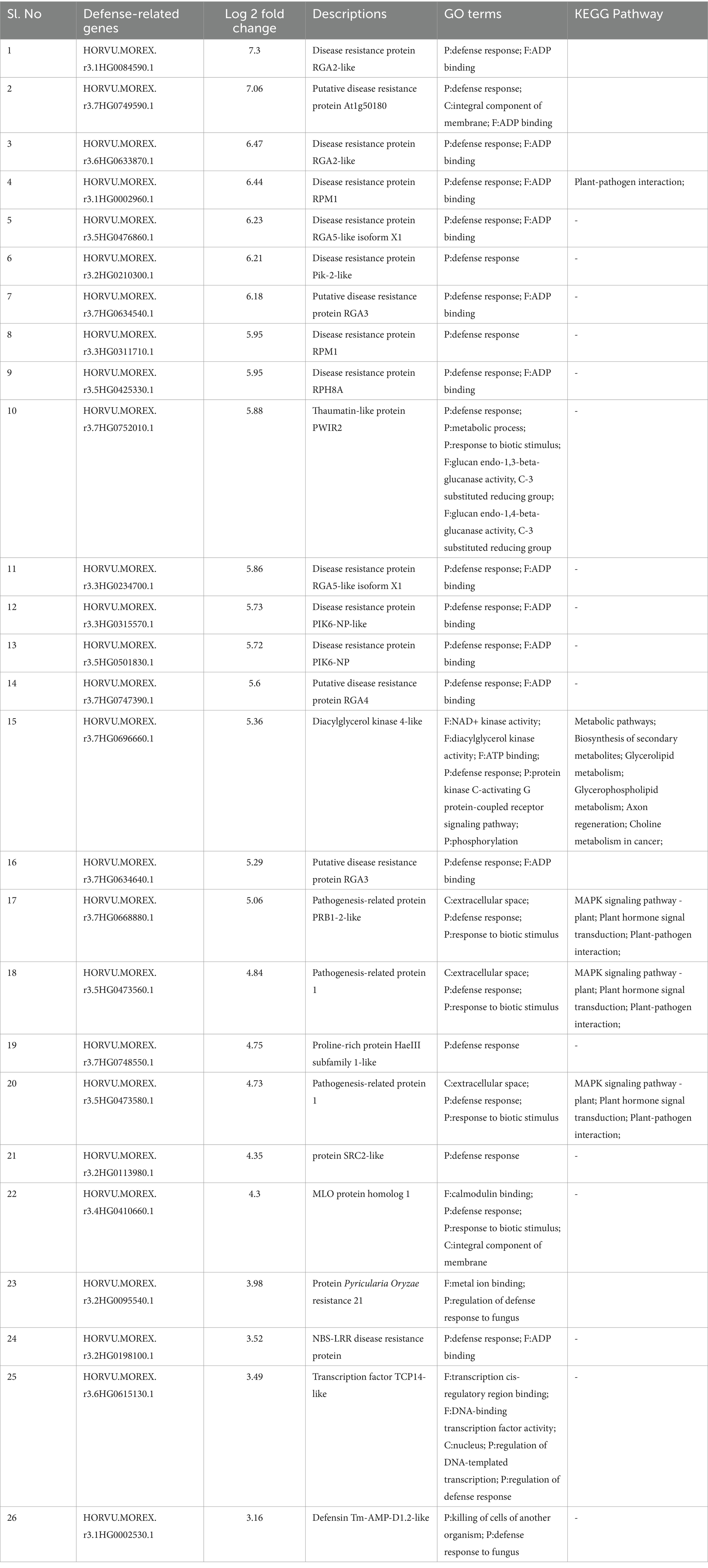
Table 3. Significantly expressed defense-related genes exclusively in resistant (EC0328964) genotype.
3.3 Analysis of virulence genes of Bipolaris sorokiniana
3.3.1 Differential gene expression analysis, gene ontology and functional annotation
The differentially expressed genes of B. sorokiniana were analyzed in both the combinations of B. sorokiniana grown in vitro-resistant inoculated (BS_RI) and B. sorokiniana grown in vitro-susceptible inoculated (BS_SI). In the resistant genotype (EC0328964) background, 128 genes were differentially expressed. Out of the 128 genes, 9 genes were upregulated, and 119 genes were downregulated (Figure 6). In the susceptible genotype (EC0578292) background, 205 genes were differentially expressed with a p-value of ≤0.05. Out of 205 genes, 10 genes were upregulated, and 195 genes were downregulated. In the two combinations, 97 genes were commonly expressed, 31 genes were exclusively expressed in resistant genotype and 108 genes were exclusively expressed in susceptible genotype (Figure 7) (Supplementary Table S3).
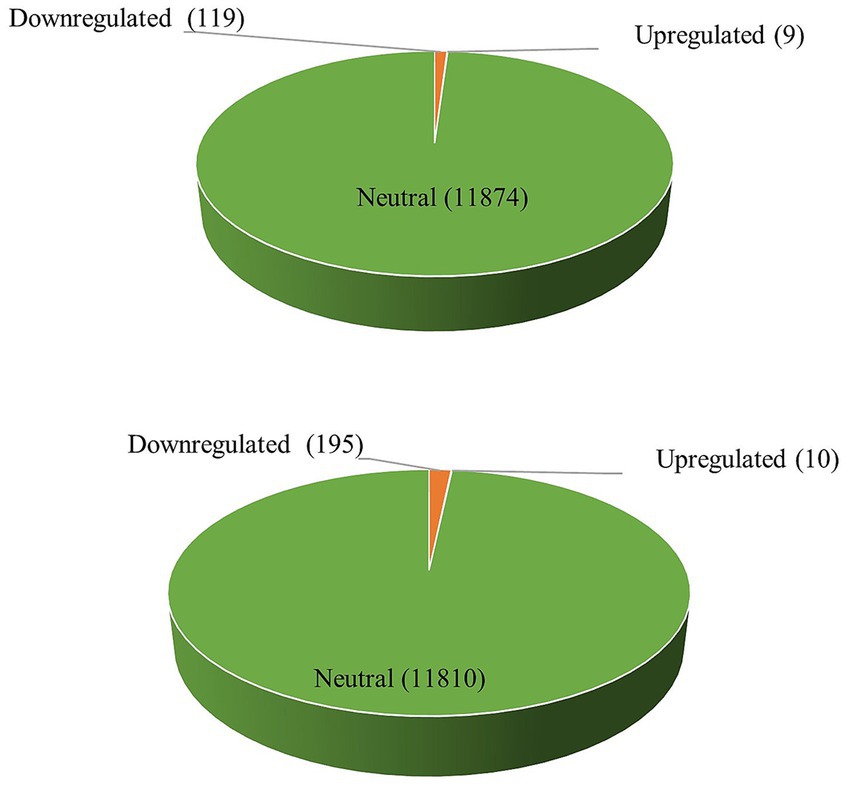
Figure 6. Differential gene expression analysis of Bipolaris sorokiniana inoculated in (top) resistant host genotype background (EC0328964) and (bottom) susceptible host genotype background (EC0578292).
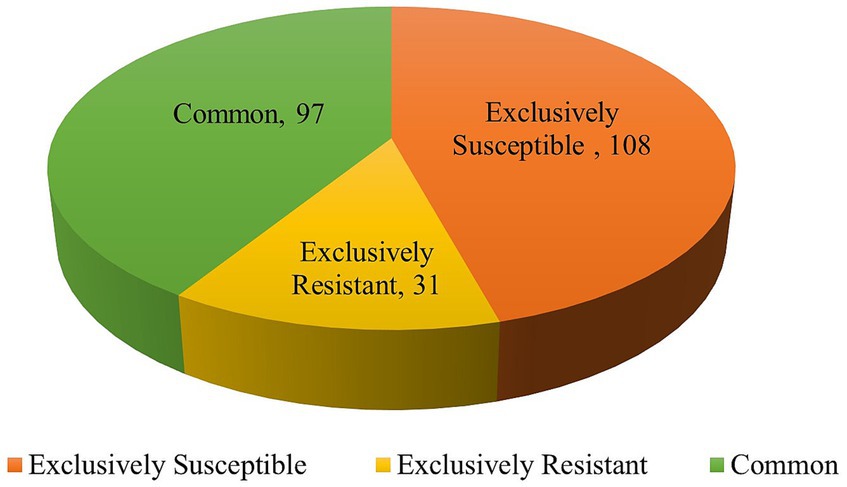
Figure 7. Common and unique genes of Bipolaris sorokiniana identified upon inoculation in two different host genotype backgrounds.
In the resistant genotype (EC0328964) background, 137 DEGs were attributed to biological process, 123 DEGs to cellular component and 78 DEGs to molecular functions. In the biological process, most DEGs were attributed to the organic substance metabolic process (19), primary metabolic process (18), and single organism cellular process (17). In the cellular component category, most of the DEGs were attributed to cell part (18), intracellular (17), intracellular part (17), and intracellular organelles (17). In the molecular function category, most of the DEGs were attributed to oxidoreductase activity (16), transferase activity (13), heterocyclic compound binding (11), and organic cyclic compound binding (11) (Figure 8). In the susceptible genotype (EC0578292) background, 374 DEGs were attributed to cellular component, 368 DEGs were attributed to biological process and 298 DEGs were attributed to molecular functions. In the cellular component category, most of the DEGs were attributed to the membrane part (62), intrinsic to membrane (61), cell part (36), and intracellular (34). In the biological process category, most DEGs were attributed to the organic substance metabolic process (48), primary metabolic process (45), and cellular metabolic process (43). In the molecular functions category, most of the DEGs were attributed to organic cyclic compound binding (49), heterocyclic compound binding (49), ion binding (45), and oxidoreductase activity (24) (Figure 9) (Supplementary Table S4). Higher number of putative virulence genes were differentially expressed in the susceptible genotype. Upon KEGG pathway analysis, numerous pathways were enriched in the susceptible host. Metabolic pathways and biosynthesis of secondary metabolites were the most overrepresented pathways in both the resistant and susceptible host backgrounds (Figure 10). The genes encoding for ABC transporters and Ubiquitin mediated proteolysis pathways were found to be more in susceptible host indicating its probable role in the infection process.
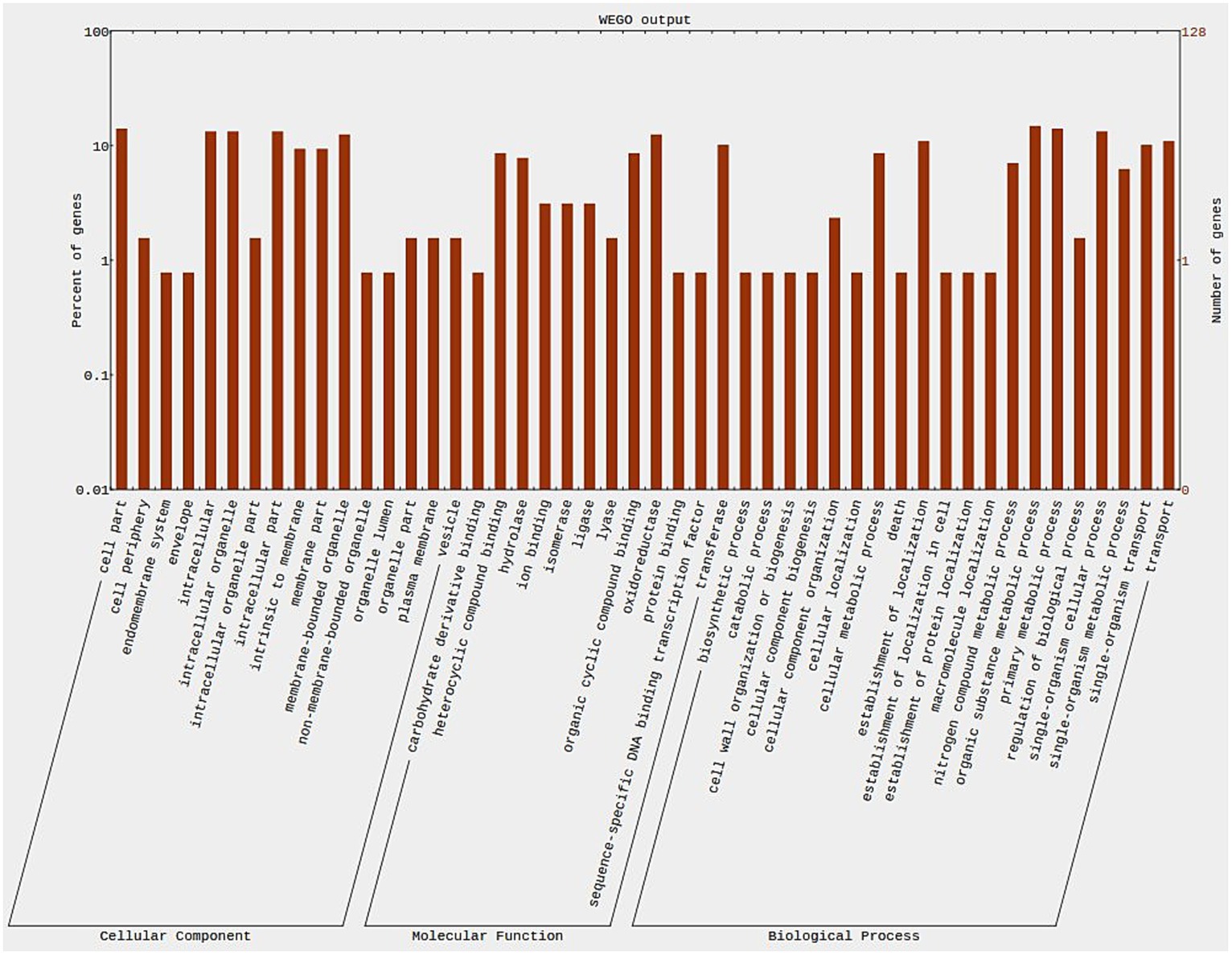
Figure 8. Wego plot showing distribution of Bipolaris sorokiniana genes in resistant host genotype background across three categories.
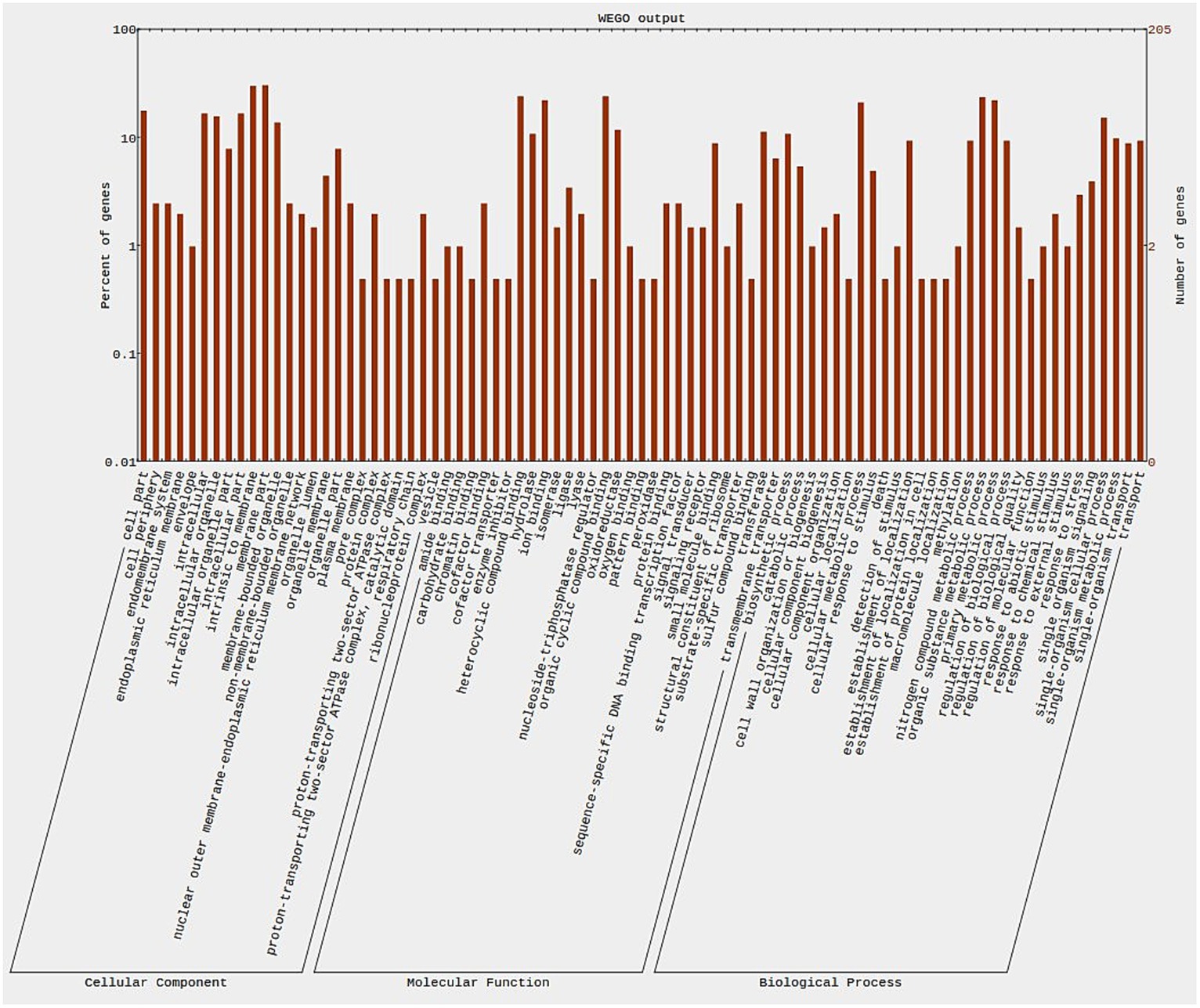
Figure 9. Wego plot showing distribution of Bipolaris sorokiniana genes in Susceptible host genotype background across three categories.
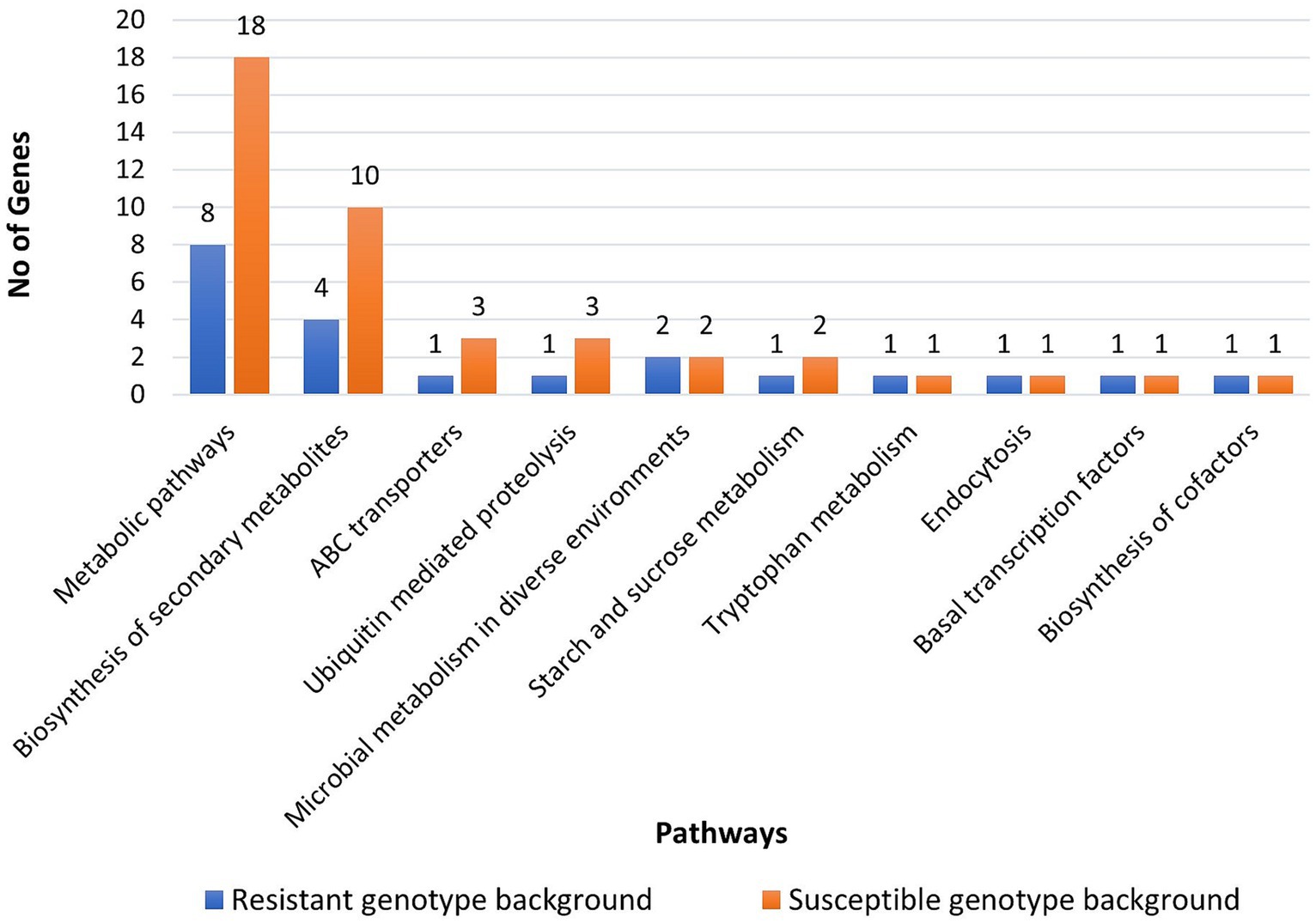
Figure 10. Major KEGG pathways identified in Bipolaris sorokiniana upon inoculation in resistant and susceptible host genotype.
3.3.2 Genes with probable role in pathogenesis and possible candidate effectors
A higher number of genes were found downregulated than upregulated in both resistant and susceptible genotype backgrounds. The top 10 differentially expressed genes were analyzed for both the host backgrounds (Table 4). The gene encoding for HET domain-containing protein was highly upregulated (8.8–8.5-fold) irrespective of the host genetic background suggesting its involvement in pathogen functionality. CENPB Domain containing protein, which is involved in DNA binding activity was found specifically upregulated (8.4-fold) in susceptible host background. The P-type cation-transporting protein-encoding gene having hydrolase activity was specifically upregulated (8.2-fold) in resistant host background. The specificity of genes being regulated implies altered pathogen functions when inoculated in two contrasting host genetic backgrounds. Pyruvate decarboxylase and hexose transporter HXT13 were among the highly downregulated genes in both the host genetic backgrounds.
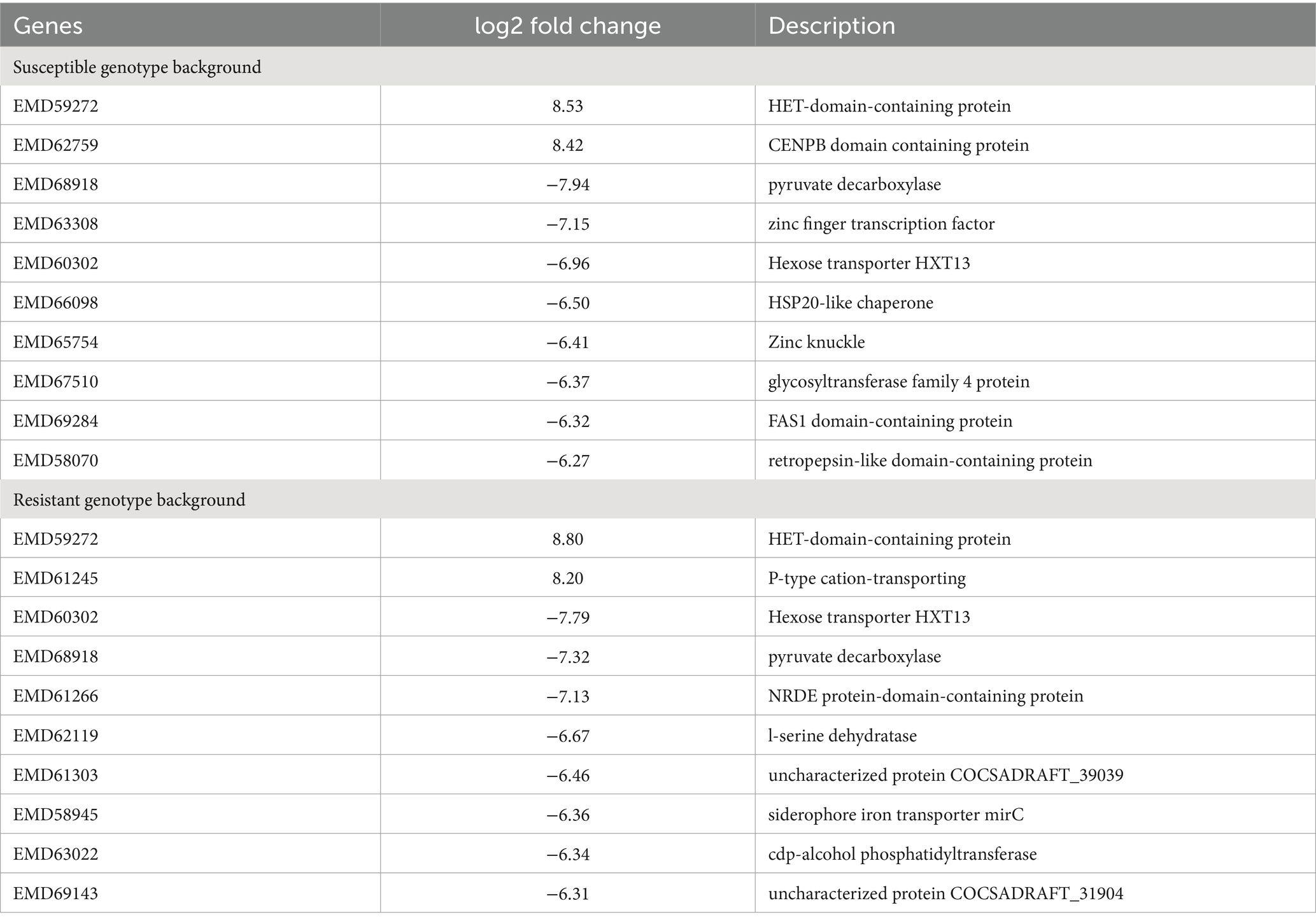
Table 4. Top up and down-regulated genes of B. sorokiniana upon inoculation in two different host genotype backgrounds.
205 differentially expressed genes of B. sorokiniana in the susceptible background were analyzed for the presence of N-terminal signal peptide. 42 gene sequences were predicted as signal peptides. 41 sequences were predicted as signal peptides with no subcellular localization. To check for the presence of transmembrane domain, 41 sequences were subjected to TMHMM 2.0 out of which 33 were predicted as non-transmembrane secreted proteins. The 33 sequences were then further analyzed using the Effector P 3.0 which predicted 14 gene sequences (11 apoplastic and 3 cytoplasmic) as effectors (Table 5). The identified sequences belonged to families viz. hydrolases, GPI anchored serine–threonine rich protein, FAS1 domain-containing protein, etc. Similarly, the presence of signal peptides was analyzed from 128 differentially expressed genes of B. sorokiniana in the resistant host. Sequentially, 29, 28, 22 and 11 sequences were identified by Signal P 5.0, Target P 2.0, TMHMM 2.0, and EffectorP 3.0, respectively. Out of which, 11 sequences were identified as effectors, 2 were denoted as cytoplasmic effectors and 9 were apoplastic effectors (Figure 11) (Supplementary Table S5). All the identified effectors except one (nad mitochondrial precursor) were also identified in the susceptible host.
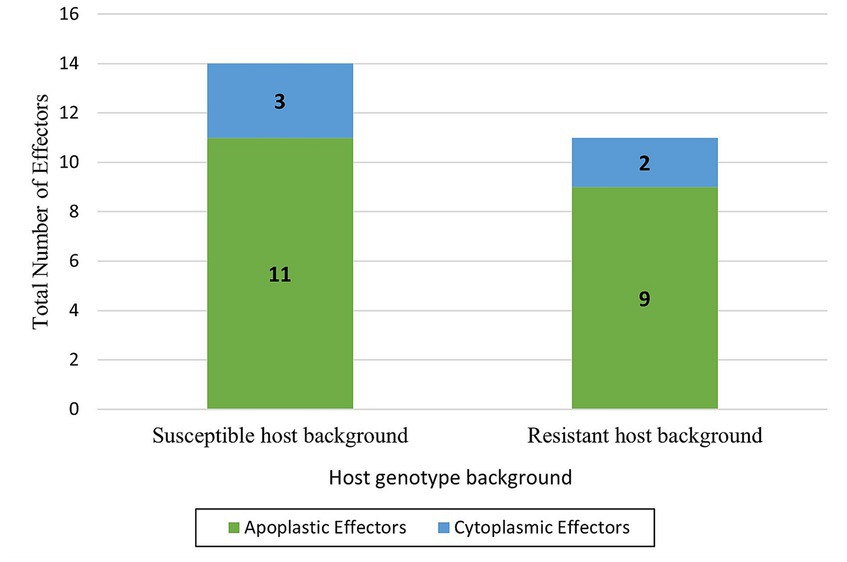
Figure 11. Candidate effectors (Apoplastic and Cytoplasmic) identified in Bipolaris sorokiniana upon inoculation in two different host genotype backgrounds.
4 Discussion
Plant–pathogen interaction is an intricate process that initiates numerous molecular reactions at various stages of infection (Peyraud et al., 2017). Analysis of transcriptome is a useful technique to decipher complex underlying processes governing plant-pathogen interaction. B. sorokiniana is a devastating pathogen causing spot blotch of wheat and barley. Untill now, no information is available on the interaction between B. sorokiniana and H. vulgare. Keeping this in view, an RNA sequencing approach was employed to analyse the transcriptome of the barley-B. sorokiniana interaction in both the resistant (EC0328964) and susceptible (EC0578292) genotypes. This investigation was carried out to gain insights into the resistance mechanisms in the host and the pathogen factors contributing to virulence.
Plants defend themselves by activating the defense response, which is triggered by the recognition of molecules of pathogen origin (De Wit et al., 2009). In the present study, several disease-resistant proteins of Hordeum vulgare were identified in the resistant genotype (EC0328964). The genes encoding for RGAs (resistant gene analogs) like disease resistance protein RGA2-like (7-fold), disease resistance protein RGA5-like isoform X1 (6-fold), putative disease resistance protein RGA3 (6-fold), and putative disease resistance protein RGA4 (6-fold) were found to be highly upregulated and present exclusively in resistant genotype in response to B. sorokiniana. Earlier studies revealed that these RGAs shared sequence similarities to the known R genes and were present abundantly in the genome of many plants (McDowell and Simon, 2006). Later, RGAs were involved in the resistance mechanisms against different pathogens (Zhang et al., 2013; Islam et al., 2020). The present investigation indicated an important role of RGAs in governing resistance in barley against B. sorokiniana. Pathogenesis-related proteins, which are involved in defense responses in the host, were also highly upregulated in the resistance genotype. The genes like pathogenesis-related protein PRB1-2-like (5-fold) pathogenesis-related protein 1(5-fold), thaumatin-like protein PWIR2 (6-fold), and defensin Tm-AMP-D1.2-like protein (3-fold) were highly expressed in resistant genotype only. Other PR proteins like chitinase 8 like were found in both the resistant and susceptible genotypes but were highly expressed in the resistant as compared to the susceptible genotype. In a recent study, through transcriptome analysis, the PR proteins like PR1, Chitinase 11, and defensin were reported slightly upregulated in the susceptible genotype than highly susceptible genotype of wheat in response to B. sorokiniana pathogen (Li et al., 2021). Thaumatin-like proteins (PR-5 family) have been earlier reported to be upregulated during plant-pathogen interaction (Singh et al., 2013). RPM1 is a peripheral membrane protein, which governs resistance to the pathogen Pseudomonas syringae (Boyes et al., 1998). In the present study, RPM1 protein (6-fold) was found to be upregulated in resistant genotype. The gene governing resistance to powdery mildew mlo (recessive) renders the plant susceptible to B. sorokiniana and other pathogens like Ramularia collo-cygni, Magnaporthe grisea, and Fusarium graminearum (Jarosch et al., 1999; Kumar et al., 2001; Jansen et al., 2005; McGrann et al., 2014). Thus, the presence of MLO (dominant/wild type) gene contributes to the defense responses against B. sorokiniana. MLO protein (4-fold) was upregulated and exclusively present in resistant genotypes in our study. Similarly, RNA-Seq analysis of barley- Ramularia collo-cygni interaction, Lemcke et al. (2021) revealed that MLO proteins have a role in resistance. Diacylglycerol kinase (DGK) is a pivotal enzyme within plant lipid signaling, holds significance in a plant’s metabolic framework, and influences its reaction to diverse external stresses (Carther et al., 2020). DGK proteins have been increased upon pathogen infection in crops like tomato and rice (Young et al., 1996; Van Der Luit et al., 2000). In our study, diacyl-glycerol kinase protein (5-fold) was upregulated in the resistant genotype. The disease-resistant PIK protein is known to play a role in interaction with the effector protein in the rice-Magnaporthe oryzae pathosystem (Zhai et al., 2011). In this study, genes similar to disease resistance PIK protein (6-fold) were expressed in resistant host. Based on GWAS in barley-B. sorokiniana reported that most QTLs mapped to genes coding NBS-LRR proteins (Visioni et al., 2020). Yazawa et al. (2013) conducted transcriptome analysis in B. sorghicola -sorghum pathosystem and found LRR family receptors to be involved in defense mechanisms. We also found NBS-LRR protein (4-fold) upregulated in the resistant genotype. TCP transcription factors serve as a cellular hub in plant defense signaling and stimulate the biosynthetic pathways like brassinosteroid (BR), jasmonic acid (JA), and flavonoids (Kim et al., 2014; Li, 2015). TCP-14-like transcription factor (4-fold) was found to be upregulated in resistant genotype in our study. Overall, the genetic basis of resistance of H. vulgare toward spot blotch might be due to a variety of genes having defense-related activities.
In the present investigation, gene ontology analysis conducted revealed several GO term representations in both the susceptible and resistant genotypes. In the biological process category, most of the DEGs were found to be involved in the metabolic process. Previous studies reported that the transcriptome of wheat-Tilletia indica pathosystem had a high number of DEGs attributed to metabolic process (Gurjar et al., 2022). In the molecular function and cellular component category, most of the DEGs were involved in binding and cell part, respectively. Similar results have been obtained in earlier reports (Gurjar et al., 2022). In our study, KEGG pathway analysis revealed predominantly metabolic pathways, with biosynthesis of secondary metabolites present in both the resistant and susceptible genotypes. Earlier, transcriptomes of Arabidopsis in response to different pathogens (Pseudomonas syringae pv. maculicola, Hyaloperonospora arabidopsidis, Fusarium oxysporum, Pseudomonas syringae, Cabbage leaf curl virus, Botrytis cinerea, Pseudomonas syringae) revealed metabolic pathways and biosynthesis of secondary metabolites as the most overrepresented pathways (Biniaz et al., 2022). The role of metabolic plant responses and secondary metabolites during plant-pathogen interaction have been reported (Bednarek, 2012). In our study, the MAPK signaling pathway, plant-pathogen interaction, and plant hormone signal transduction were more enriched in resistant (EC0328964) than susceptible genotype (EC0578292) during barley-B. sorokiniana interaction. Earlier studies on other host-pathogen in barley-Blumeria graminis pathosystem have shown that mitogen-activated protein kinase (MAPK) signaling and plant hormone signaling pathways are upregulated in resistant genotype (Li et al., 2019). In our opinion, the DEGs associated with the MAPK signaling pathway were more in the resistant genotype than the susceptible genotype indicating an important role of this pathway in spot blotch resistance mechanism in barley. Further, MAPK signaling pathway genes will be characterized using the functional genomics approach.
Plant pathogens have several strategies for the production of cell wall degrading enzymes, secondary metabolites, and secreted proteins to counteract plant defense responses (De Wit et al., 2009; Kubicek et al., 2014). The intricate mechanisms underlying the interaction between B. sorokiniana and Hordeum vulgare is poorly understood and it is confined to Triticum aestivum-B. sorokiniana pathosystem. In this study, we identified a few genes involved in the pathogenicity of B. sorokiniana. In both the host genetic backgrounds, most of the pathogen genes were downregulated rather than upregulated. The functional annotation revealed that in the susceptible host genotype background the pathways governing metabolism, biosynthesis of secondary metabolites, ABC transporters, and Ubiquitin mediated proteolysis were highly enriched in comparison to the resistant host genotype background. This indicates that the susceptible host promotes the activities of the pathogen and provides a suitable environment for successful colonization. In earlier reports, secondary metabolites aid the pathogens to successfully evade plant defense responses (Keller et al., 2005). ABC transporters too play an important role in safeguarding pathogens against plant defense compounds (Yazaki, 2006). In the present study, upregulation of gene encoding for HET domain-containing was observed in both the genotypes. The HET domain contains proteins highly abundant in fungal genomes and attributed to governing incompatibility systems (Paoletti and Clave, 2007). With emerging studies, these proteins might have other functions as well and likely play a role in fungal immunity (Daskalov, 2023). However, most of the HET domain proteins remain unannotated and their probable roles during in-planta interactions are yet to be deciphered. In the present study, the gene encoding CENPB domain-containing protein was noticed to be exclusively upregulated in susceptible genotype background. In earlier reports, CENPB proteins were responsible for centromere formation in mammals (Masumoto et al., 1989; Fachinetti et al., 2015). Homologs for CENPB protein have been reported in Neurospora crassa but its function remains unknown (Smith et al., 2012). Enzymes responsible for hydrolase, oxidoreductase, and transferase activity were found to be downregulated in our study. These enzymes play an important role in various reaction cycles. Glycoside hydrolase represents the largest family of hydrolase enzymes, which is responsible for enzymatic activity in degrading plant cell walls. In earlier reports, glycoside hydrolase proteins were downregulated in the biotrophic phase of Cladosporium fulvum and upregulated during necrotrophic phase (Okmen et al., 2019). Effectors are traditionally classified as small cysteine-rich, transmembrane domains lacking proteins along with signal peptide domains (Zhang et al., 2020). In the present study, 14 sequences were identified as candidate effectors in the susceptible host and 11 effectors in the resistant host background, which belonged to families like hydrolase, fascilin domain protein, and other uncharacterized proteins. Earlier, Fasciclin/FAS1 family proteins have been reported as cell adhesion molecules in fungi and other organisms (Miyazaki et al., 2007). Liu et al., 2009 identified a fasciclin-like protein (MoFLP1) involved in conidiation, conidia adhesion, and pathogenicity in Magnaporthe oryzae. The glycoside hydrolase family 16 protein (GH16) was also identified as apoplastic effectors in both the barley genotypes. Plant pathogens deploy an arsenal of effectors which are expressed in a spatio-temporal manner, thereby making it essential to identify its expression across various tissues and time points (Toruno et al., 2016).
5 Conclusion
Transcriptomic analysis of barley genotypes with challenge inoculation of B. sorokiniana was performed using the RNA Seq approach and defense-related genes and pathogenicity determinants involved during Hordeum vulgare-B. sorokiniana interaction were identified. The defense-related genes viz., RGA2-like (7-fold), disease resistance protein RGA5-like isoform X1 (6-fold), putative disease resistance protein RGA3 (6-fold) and putative disease resistance protein RGA4 (6-fold) were highly expressed in resistant genotype only. 14 effectors of B. sorokiniana were identified viz. 3 cytoplasmic and 11 apoplastic effectors in resistant host background. The pathways encoding for metabolism, biosynthesis of secondary metabolites, ABC transporters, and Ubiquitin mediated proteolysis were higher in susceptible genotype. This is the first report elucidating the transcriptome of barley-B. sorokiniana interaction which might provide valuable information on genetic factors involved in the spot blotch resistance of barley.
Data availability statement
The datasets presented in this study are deposited in the NCBI database under accession number PRJNA996376 (SRR25379257, SRR25379256, SRR25379255, SRR25379254, SRR25379253, SRR25379252, SRR25379251, SRR25379250, SRR25379249, SRR25379248).
Author contributions
PB: Data curation, Formal analysis, Investigation, Methodology, Software, Writing – original draft. MG: Conceptualization, Funding acquisition, Supervision, Writing – original draft, Writing – review & editing. TK: Data curation, Methodology, Writing – review & editing. NK: Data curation, Formal analysis, Writing – original draft. DS: Data curation, Methodology, Writing – review & editing. SJ: Formal analysis, Methodology, Writing – review & editing. MS: Methodology, Project administration, Writing – review & editing.
Funding
The author(s) declare that financial support was received for the research, authorship, and/or publication of this article. The financial support received from the ICAR-Consortium Research Platform on Genomics-Pathogenomics (ICARG/CRP-Genomics/2015-2720/IARI-12-151) for this present investigation.
Acknowledgments
We are highly thankful to Director, Joint Director (Research), ICAR-Indian Agricultural Research Institute, New Delhi, for guiding us and providing the resources needed to complete this research.
Conflict of interest
The authors declare that the research was conducted in the absence of any commercial or financial relationships that could be construed as a potential conflict of interest.
Publisher’s note
All claims expressed in this article are solely those of the authors and do not necessarily represent those of their affiliated organizations, or those of the publisher, the editors and the reviewers. Any product that may be evaluated in this article, or claim that may be made by its manufacturer, is not guaranteed or endorsed by the publisher.
Supplementary material
The Supplementary material for this article can be found online at: https://www.frontiersin.org/articles/10.3389/fmicb.2024.1360571/full#supplementary-material
Footnotes
2. ^https://www.genome.jp/kegg/kaas/
3. ^https://services.healthtech.dtu.dk/services/SignalP-5.0/
4. ^https://services.healthtech.dtu.dk/services/TargetP-2.0/
References
Aich, S., Singh, R. K., Kundu, P., Pandey, S. P., and Datta, S. (2017). Genome-wide characterization of cellulases from the hemi-biotrophic plant pathogen, Bipolaris sorokiniana, reveals the presence of a highly stable GH7 endoglucanase. Biotechnol. Biofuels 10:135. doi: 10.1186/s13068-017-0822-0
Bala, A., and Kaur, S. (2008). Cross infectivity of Bipolaris sorokiniana among wheat, barley, triticale, rye and Phalaris minor. Plant Dis. Res. 23, 7–12.
Bednarek, P. (2012). Chemical warfare or modulators of defence responses – the function of secondary metabolites in plant immunity. Curr. Opin. Plant Biol. 15, 407–414. doi: 10.1016/j.pbi.2012.03.002
Biniaz, Y., Tahmasebi, A., Tahmasebi, A., Albrectsen, B. R., Poczai, P., and Afsharifar, A. (2022). Transcriptome meta-analysis identifies candidate hub genes and pathways of pathogen stress responses in Arabidopsis thaliana. Biology 11:1155. doi: 10.3390/biology11081155
Boyes, D. C., Nam, J., and Dangl, J. L. (1998). The Arabidopsis thaliana RPM1 disease resistance gene product is a peripheral plasma membrane protein that is degraded coincident with the hypersensitive response. Proc. Natl. Acad. Sci. USA 95, 15849–15854. doi: 10.1073/pnas.95.26.15849
Carther, K. F. I., Ketehouli, T., Zhou, Y., Li, X., Wang, F., and Li, H. (2020). The emerging roles of diacylglycerol kinase (DGK) in plant stress tolerance, growth, and development. Agronomy 10:1375. doi: 10.3390/agronomy10091375
Chand, R., Kumar, M., Kushwaha, C., Shah, K., and Joshi, A. K. (2014). Role of melanin in release of extracellular enzymes and selection of aggressive isolates of Bipolaris sorokiniana in barley. Curr. Microbiol. 69, 202–211. doi: 10.1007/s00284-014-0559-y
Chand, R., Sen, D., Prasad, K. D., Singh, A. K., Bashyal, B. M., Prasad, L. C., et al. (2008). Screening for disease resistance in barley cultivars against Bipolaris sorokiniana using callus culture method. Indian J. Exp. Biol. 46, 249–253.
Chaurasia, S., Chand, R., and Joshi, A. K. (2000). Relative dominance of Alternaria triticina Pras. Et Prab. & Bipolaris sorokiniana (Sacc.) shoemaker, in different growth stages of wheat (T. aestivum L.). J. Plant Dis. Prot. 107, 176–181.
Condon, B. J., Leng, Y., Wu, D., Bushley, K. E., Ohm, R. A., et al. (2013). Comparative genome structure, secondary metabolite, and effector coding capacity across Cochliobolus pathogens. PLoS Genet. 9:e1003233. doi: 10.1371/journal.pgen.1003233
Daskalov, A. (2023). Emergence of the fungal immune system. iScience 26:106793. doi: 10.1016/j.isci.2023.106793
De Wit, P., Mehrabi, R., Van Den Burg, H., and Stergiopoulos, I. (2009). Fungal effector proteins: past, present and future. Mol. Plant Pathol. 10, 735–747. doi: 10.1111/j.1364-3703.2009.00591.x
Fachinetti, D., Han, J. S., McMahon, M. A., Ly, P., Abdullah, A., Wong, A. J., et al. (2015). DNA sequence-specific binding of CENP-B enhances the fidelity of human centromere function. Dev. Cell 33, 314–327. doi: 10.1016/j.devcel.2015.03.020
FAO. (2022). Food and agriculture organization of the United Nations. FAOSTAT statistics database. Rome
Friesen, T. L., Holmes, D. J., Bowden, R. L., and Faris, J. D. (2018). ToxA is present in the U.S. Bipolaris sorokiniana population and is a significant virulence factor on wheat harboring Tsn1. Plant Dis. 102, 2446–2452. doi: 10.1094/PDIS-03-18-0521-RE
Ghazvini, H., and Tekauz, A. (2008). Host-pathogen interactions among barley genotypes and Bipolaris sorokiniana isolates. Plant Dis. 92, 225–233. doi: 10.1094/PDIS-92-2-0225
Glass, N. L., Jacobson, D. J., and Shiu, P. K. (2000). The genetics of hyphal fusion and vegetative incompatibility in filamentous ascomycete fungi. Annu. Rev. Genet. 34, 165–186. doi: 10.1146/annurev.genet.34.1.165
Gotz, S., GarcÃa-Gómez, J. M., Terol, J., Williams, T. D., Nagaraj, S. H., Nueda, M. J., et al. (2008). High-throughput functional annotation and data mining with the blast 2GO suite. Nucleic Acids Res. 36, 3420–3435. doi: 10.1093/nar/gkn176
Gupta, P. K., Chand, R., Vasistha, N. K., Pandey, S. P., Kumar, U., Mishra, V. K., et al. (2018). Spot blotch disease of wheat: the current status of research on genetics and breeding. Plant Pathol. 67, 508–531. doi: 10.1111/ppa.12781
Gurjar, M., Jain, S., Aggarwal, R., Saharan, M., Kumer, T., and Kharbikar, L. (2022). Transcriptome analysis of wheat–Tilletia indica interaction provides defense and pathogenesis-related genes. Plan. Theory 11:3061. doi: 10.3390/plants11223061
Iftikhar, S., Shahzad, A., Munir, A., Sultan, A., and Iftikhar, A. (2009). Hosts of Bipolaris sorokiniana, the major pathogen of spot blotch of wheat in Pakistan. Pak. J. Bot. 41, 1433–1436.
Islam, M. R., Hossain, M. R., Jesse, D. M. I., Jung, H. J., Kim, H. T., Park, J. I., et al. (2020). Characterization, identification and expression profiling of genome-wide R-genes in melon and their putative roles in bacterial fruit blotch resistance. BMC Genet. 21:80. doi: 10.1186/s12863-020-00885-9
Jahani, M., Aggarwal, R., Dureja, P., and Srivastava, K. D. (2006). Toxin production by Bipolaris sorokiniana and its role in determining resistance in wheat genotypes. Indian Phytopathol. 59, 340–344.
Jansen, C., Wettstein, D. V., Schafer, W., Kogel, K. H., Felk, A., and Maier, F. J. (2005). Infection patterns in barley and wheat spikes inoculated with wild-type and trichodiene synthase gene disrupted fusarium graminearum. Proc. Natl. Acad. Sci. USA 102, 16892–16897. doi: 10.1073/pnas.0508467102
Jarosch, B., Kogel, K. H., and Schaffrath, U. (1999). The ambivalence of the barley Mlo locus: mutations conferring resistance against powdery mildew (Blumeria graminis f. sp, hordei) enhance susceptibility to the rice blast fungus Magnaporthe grisea. Mol. Plant-Microbe Interact. 12, 508–514. doi: 10.1094/MPMI.1999.12.6.508
Kaladhar, V. C., Singh, Y., Nair, A. M., Kumar, K., Singh, A. K., and Verma, P. K. (2023). A small cysteine-rich fungal effector, BsCE66 is essential for the virulence of Bipolaris sorokiniana on wheat plants. Fungal Genet. Biol. 166:103798. doi: 10.1016/j.fgb.2023.103798
Kanehisa, M., and Goto, S. (2000). KEGG: Kyoto encyclopedia of genes and genomes. Nucleic Acids Res. 28, 27–30. doi: 10.1093/nar/28.1.27
Keller, N. P., Turner, G., and Bennett, J. W. (2005). Fungal secondary metabolism from biochemistry to genomics. Nat. Rev. Microbiol. 3, 937–947. doi: 10.1038/nrmicro1286
Kim, S. H., Son, G. H., Bhattacharjee, S., Kim, H. J., Nam, J. C., Nguyen, P. D., et al. (2014). The Arabidopsis immune adaptor SRFR1 interacts with TCP transcription factors that redundantly contribute to effector-triggered immunity. Plant J. 78, 978–989. doi: 10.1111/tpj.12527
Kubicek, C. P., Starr, T. L., and Glass, N. L. (2014). Plant cell wall-degrading enzymes and their secretion in plant-pathogenic fungi. Annu. Rev. Phytopathol. 52, 427–451. doi: 10.1146/annurev-phyto-102313-045831
Kumar, D., Chand, R., Prasad, L. C., and Joshi, A. K. (2007). A new technique for monoconidial culture of the most aggressive isolate in a given population of Bipolaris sorokiniana, cause of foliar spot blotch in wheat and barley. World J. Microbiol. Biotechnol. 23, 1647–1651. doi: 10.1007/s11274-007-9410-y
Kumar, J., Hückelhoven, R., Beckhove, U., Nagarajan, S., and Kogel, K. H. (2001). A compromised Mlo pathway affects the response of barley to the necrotrophic fungus Bipolaris sorokiniana (teleomorph: Cochliobolus sativus). Phytopathology 91, 127–133. doi: 10.1094/PHYTO.2001.91.2.127
Lemcke, R., Sjokvist, E., Visentin, S., Kamble, M., James, E. K., Hjortshoj, R. L., et al. (2021). Deciphering molecular host-pathogen interactions during Ramularia Collo-Cygni infection on barley. Front. Plant Sci. 12:747661. doi: 10.3389/fpls.2021.747661
Leng, Y., Zhao, M., Fiedler, J. D., Dreiseitl, A., Chao, S., Li, X., et al. (2020). Molecular mapping of loci conferring susceptibility to spot blotch and resistance to powdery mildew in barley using the sequencing-based genotyping approach. Phytopathology 110, 440–446. doi: 10.1094/PHYTO-08-19-0292-R
Li, S. (2015). The Arabidopsis thaliana TCP transcription factors: a broadening horizon beyond development. Plant Signal. Behav. 10:e1044192. doi: 10.1080/15592324.2015.1044192
Li, Q., Gao, C., Xu, K., Jiang, Y., Niu, J., Guo, Y., et al. (2021). Transcriptome-based analysis of resistance mechanism to black point caused by Bipolaris sorokiniana in wheat. Sci. Rep. 11:6911. doi: 10.1038/s41598-021-86303-1
Li, Y., Guo, G., Zhou, L., Chen, Y., Zong, Y., Huang, J., et al. (2019). Transcriptome analysis identifies candidate genes and functional pathways controlling the response of two contrasting barley varieties to powdery mildew infection. Int. J. Mol. Sci. 21:151. doi: 10.3390/ijms21010151
Liu, T. B., Chen, G. Q., Min, H., and Lin, F. C. (2009). MoFLP1, encoding a novel fungal fasciclin-like protein, is involved in conidiation and pathogenicity in Magnaporthe oryzae. J Zhejiang Univ Sci B 10, 434–444. doi: 10.1631/jzus.B0920017
Mascher, M., Wicker, T., Jenkins, J., Plott, C., Lux, T., Koh, C. S., et al. (2021). Long-read sequence assembly: a technical evaluation in barley. Plant Cell 33, 1888–1906. doi: 10.1093/plcell/koab077
Masumoto, H., Masukata, H., Muro, Y., Nozaki, N., and Okazaki, T. (1989). A human centromere antigen (CENP-B) interacts with a short specific sequence in alphoid DNA, a human centromeric satellite. J. Cell Biol. 109, 1963–1973. doi: 10.1083/jcb.109.5.1963
McDowell, J. M., and Simon, S. A. (2006). Recent insights into R gene evolution. Mol. Plant Pathol. 7, 437–448. doi: 10.1111/j.1364-3703.2006.00342.x
McGrann, G. R. D., Stavrinides, A., Russell, J., Corbitt, M. M., Booth, A., and Chartrain, L. (2014). A trade-off between MLO resistance to powdery mildew and increased susceptibility of barley to a newly important disease, Ramularia leaf spot. J. Exp. Bot. 65, 1025–1037. doi: 10.1093/jxb/ert452
Miyazaki, Y., Kaneko, S., Sunagawa, M., Shishido, K., Yamazaki, T., Nakamura, M., et al. (2007). The fruiting-specific Le. flp1 gene, encoding a novel fungal fasciclin-like protein, of the basidiomycetous mushroom Lentinula edodes. Curr. Genet. 51, 367–375. doi: 10.1007/s00294-007-0133-2
Okmen, B., Bachmann, D., and De Wit, P. (2019). A conserved GH17 glycosyl hydrolase from plant pathogenic Dothideomycetes releases a DAMP causing cell death in tomato. Mol. Plant Pathol. 20, 1710–1721. doi: 10.1111/mpp.12872
Paoletti, M., and Clave, C. (2007). The fungus-specific HET domain mediates programmed cell death in Podospora anserina. Eukaryot. Cell 6, 2001–2008. doi: 10.1128/EC.00129-07
Peyraud, R., Dubiella, U., Barbacci, A., Genin, S., Raffaele, S., and Roby, D. (2017). Advances on plant-pathogen interactions from molecular toward systems biology perspectives. Plant J. 90, 720–737. doi: 10.1111/tpj.13429
Singh, N. K., Kumar, K. R., Kumar, D., Shukla, P., and Kirti, P. B. (2013). Characterization of a pathogen induced thaumatin-like protein gene AdTLP from Arachis diogoi, a wild peanut. PLoS One 8:e83963. doi: 10.1371/journal.pone.0083963
Smith, K. M., Galazka, J. M., Phatale, P. A., Connolly, L. R., and Freitag, M. (2012). Centromeres of filamentous fungi. Chromosom. Res. 20, 635–656. doi: 10.1007/s10577-012-9290-3
Toruno, T. Y., Stergiopoulos, I., and Coaker, G. (2016). Plant-pathogen effectors: cellular probes interfering with plant defenses in spatial and temporal manners. Annu. Rev. Phytopathol. 54, 419–441. doi: 10.1146/annurev-phyto-080615-100204
Vaish, S. S., Ahmed, S. B., and Prakash, K. (2011). First documentation on status of barley diseases from the high altitude cold arid trans-Himalayan Ladakh region of India. Crop Prot. 30, 1129–1137. doi: 10.1016/j.cropro.2011.04.015
Van Der Luit, A. H., Piatti, T., Van Doorn, A., Musgrave, A., Felix, G., Boller, T., et al. (2000). Elicitation of suspension-cultured tomato cells triggers the formation of phosphatidic acid and diacylglycerol pyrophosphate. Plant Physiol. 123, 1507–1516. doi: 10.1104/pp.123.4.1507
Visioni, A., Rehman, S. U., Viash, S. S., Singh, S., Vishwakarma, R. A., Gyawali, S., et al. (2020). Genome wide association mapping of spot blotch resistance at seedling and adult plant stages in barley. Front. Plant Sci. 11:642. doi: 10.3389/fpls.2020.00642
Yazaki, K. (2006). ABC transporters involved in the transport of plant secondary metabolites. FEBS Lett. 580, 1183–1191. doi: 10.1016/j.febslet.2005.12.009
Yazawa, T., Kawahigashi, H., Matsumoto, T., and Mizuno, H. (2013). Simultaneous transcriptome analysis of sorghum and Bipolaris sorghicola by using RNA-seq in combination with de novo transcriptome assembly. PLoS One 8:e62460. doi: 10.1371/journal.pone.0062460
Young, S. A., Wang, X., and Leach, J. E. (1996). Changes in the plasma membrane distribution of rice phospholipase D during resistant interactions with Xanthomonas oryzae pv oryzae. Plant Cell 8, 1079–1090. doi: 10.2307/3870217
Zhai, C., Lin, F., Dong, Z., He, X., Yuan, B., Zeng, X., et al. (2011). The isolation and characterization of Pik, a rice blast resistance gene which emerged after rice domestication. New Phytol. 189, 321–334. doi: 10.1111/j.1469-8137.2010.03462.x
Zhang, Y. L., Jia, Q. L., Li, D. W., Wang, J. E., Yin, Y. X., and Gong, Z. H. (2013). Characteristic of the pepper CARGA2 gene in defense responses against Phytophthora capsici Leonian. Int. J. Mol. Sci. 14, 8985–9004. doi: 10.3390/ijms14058985
Zhang, W., Li, H., Wang, L., Xie, S., Zhang, Y., Kang, R., et al. (2022). A novel effector, CsSp1, from Bipolaris sorokiniana, is essential for colonization in wheat and is also involved in triggering host immunity. Mol. Plant Pathol. 23, 218–236. doi: 10.1111/mpp.13155
Zhang, Y., Wei, J., Qi, Y., Li, J., Amin, R., Yang, W., et al. (2020). Predicating the effector proteins secreted by Puccinia triticina through transcriptomic analysis and multiple prediction approaches. Front. Microbiol. 11:538032. doi: 10.3389/fmicb.2020.538032
Keywords: Hordeum vulgare (barley), transcriptome, differentially expressed genes, resistant gene analogs, metabolism, effector
Citation: Basak P, Gurjar MS, Kumar TPJ, Kashyap N, Singh D, Jha SK and Saharan MS (2024) Transcriptome analysis of Bipolaris sorokiniana - Hordeum vulgare provides insights into mechanisms of host-pathogen interaction. Front. Microbiol. 15:1360571. doi: 10.3389/fmicb.2024.1360571
Edited by:
Anukool Vaishnav, GLA University, IndiaReviewed by:
R. P. Ghasolia, Sri Karan Narendra Agriculture University, IndiaSachin Gupta, Sher-e-Kashmir University of Agricultural Sciences and Technology, India
Rakesh Kumar Verma, Mody University of Science and Technology, India
Copyright © 2024 Basak, Gurjar, Kumar, Kashyap, Singh, Jha and Saharan. This is an open-access article distributed under the terms of the Creative Commons Attribution License (CC BY). The use, distribution or reproduction in other forums is permitted, provided the original author(s) and the copyright owner(s) are credited and that the original publication in this journal is cited, in accordance with accepted academic practice. No use, distribution or reproduction is permitted which does not comply with these terms.
*Correspondence: Malkhan Singh Gurjar, malkhan_iari@yahoo.com