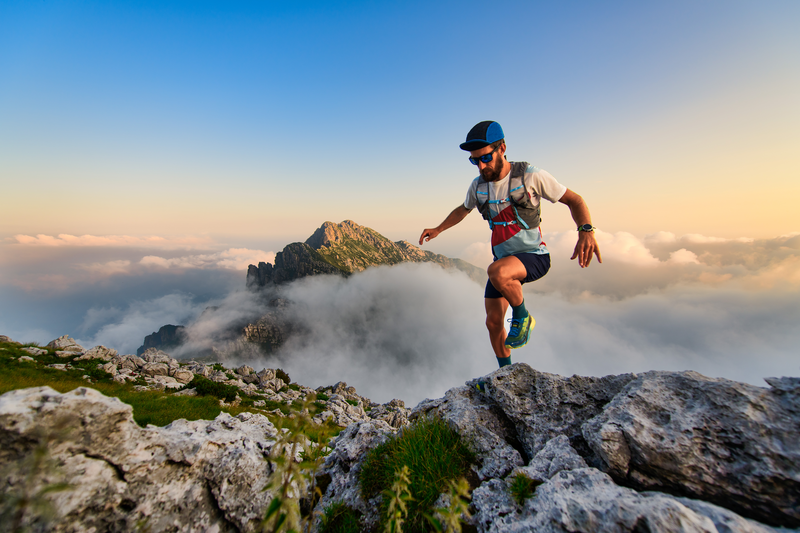
95% of researchers rate our articles as excellent or good
Learn more about the work of our research integrity team to safeguard the quality of each article we publish.
Find out more
ORIGINAL RESEARCH article
Front. Microbiol. , 25 April 2024
Sec. Microorganisms in Vertebrate Digestive Systems
Volume 15 - 2024 | https://doi.org/10.3389/fmicb.2024.1360505
This article is part of the Research Topic The Role of Nutritional Strategies in the Regulation of Gut Microbiota and Host Immune System View all 27 articles
Introduction: Utilizing roughage resources is an effective approach to alleviate the shortage of corn-soybean feed and reducing the costs in the swine industry. Hezuo pig is one group of plateau type local Tibetan pig with strong tolerance to crude feeding. Nevertheless, current research on the roughage tolerance in Hezuo pigs and the microbiological mechanisms behind it is still minimally.This study explored the impact of various ratios of whole-plant silage (WPS) maize on the pH, cellulase activity, short-chain fatty acids (SCFAs), and intestinal microbiota in Hezuo pigs.
Methods: Thirty-two Hezuo pigs were randomly divided into four groups (n = 8). The control group received a basal diet, while experimental groups I, II, and III were given diets with incremental additions of 5%, 10%, and 15% air-dried WPS maize, respectively, for 120 days.
Results: The findings revealed that compared with the control group, in Group II, the pH of cecum and colon were notably decreased (p < 0.05), while acid detergent fiberdigestibility, the concentration of propionic and isobutyric acid in the cecum, and the concentration of isobutyric acid in the colon were significantly increased (p < 0.05). Also, carboxymethyl cellulase activity in the cecum in group II of Hezuo pigs was significantly higher than that in the other three groups (p < 0.05). Furthermore, the cecum microbiota showed a higher diversity in the group II of Hezuo pigs than that in the control group, as shown by the Simpson and Shannon indices. Specifically, 15 and 24 bacterial species showed a significant difference in relative abundance at the family and genus levels, respectively. Correlation analyses revealed significant associations between bacterial genera and SCFAs concentrations in the cecum. The abundance of Bacteroides and NK4A214_group was positively correlated with amounts of valeric and isovaleric acid but negatively with propionic acid (p < 0.05). The abundance of UCG-010 was positively linked with acetic acid and negatively correlated with butyric acid (p < 0.05). Actinobacillus abundance was positively associated with butyric acid levels (p < 0.05).
Discussion: In conclusion, a 10% WPS maize diet improved crude fiber digestibility by lowering cecal and colonic chyme pH, enhancing intestinal cellulase activity, improving SCFA production, and increasing intestinal microbiota diversity.
With the improving living standards, consumers’ demand for meat, eggs, milk, and other nutritious foods has grown, leading to rapid developments in livestock and poultry farming. This also significantly increased the demand for animal feed, highlighting issues such as the shortage of feed resources and rising costs. China, a major player in hog farming and pork consumption, produced over 49 million tons of pork in 2021, accounting for 60% of the total meat consumption in the country. Thus, it is critical to efficiently develop and utilize roughage resources for reducing the costs and increasing the economical benefits of pig production.
Whole-plant silage (WPS) maize, which is nutritionally rich and high in crude fiber compared to ordinary corn forage, is a quality roughage. It has been increasingly used in grass-fed livestock production but is not yet widely adopted in pigs and other forage-feeding animals. Dietary fiber at appropriate levels can enhance intestinal microorganism fermentation in animals, leading to significant short-chain fatty acid (SCFAs) production and improving host metabolic processes (Gill et al., 2021). Studies have shown that green juicy feeds and high-quality fiber can regulate nutrient absorption and enhance immune function in pigs (De Lange et al., 2010; Knudsn et al., 2012). Wang et al. (2023) found that adding 10% WPS maize to pig diets improves feed intake and daily weight gain, and reduces pollutants and harmful gasses. Ren et al. (2021) observed that adding 80% WPS maize in Bamei pigs’ could significantly decreased the feeding costs.
Chinese local pig breeds, which have been historically grazed or fed low-nutrient-level diets with green roughage supplemented by concentrates, have developed better roughage tolerance over foreign breeds (Zhao et al., 2019). Chinese local breeds have a distinct gut flora composition and structure from imported lean-type pig breeds, which is beneficial for their stronger fiber utilization ability (Tan et al., 2020). In pigs, crude fiber digestion mainly occurs through anaerobic fermentation by microbial fiber enzymes in the large intestine (cecum and colon). Here, fiber-degrading bacteria secrete cellulase complexes, using the fiber as a fermentation substrate and producing SCFAs that are efficiently absorbed by the host (Cheng and Yan, 2008; Zhu, 2020).
The state has recently emphasized protecting and innovatively using local germplasm resources, promoting several excellent local pig breeds for industrial development. The Hezuo pig, a plateau-type Tibetan pig breed raised semi-grazing in Gansu Province’s Gannan Tibetan Autonomous Prefecture, exhibits excellent traits like good meat quality and adversity resistance. However, it has slow growth and unique nutritional requirements compared to lean pigs. It effectively digests high-fiber unconventional feeds, providing a significant advantage in utilizing China’s rural feed resources. Despite these traits, the traditional feeding method of the Hezuo pig faces challenges due to new national regulations on ecosystem protection, necessitating exploration into housed-feeding alternatives. Furthermore, there is a lack of data supporting the roughage tolerance in Hezuo pigs and the microbiological mechanisms behind it.
Our previous study found that adding different proportions of WPS maize can significantly improves growth performance, meat quality, and blood biochemical indices in Hezuo pigs (Yin et al., 2024). Based on this foundation, in this study, we further investigates the effects of WPS maize on fiber digestibility, cecum and colon pH values, fiber-digesting enzyme activity, SCFAs, and intestinal bacterial flora in Hezuo pigs. Our findings provide a reference for applying WPS maize in Hezuo pig feeding to revitalize pig farming in Tibetan area villages.
Thirty-two healthy Hezuo pigs, aged 60 days, were selected after a 7-day pre-feeding period on a basal diet. These pigs had similar body weights, averaging 7.88 ± 0.81 kg. They were randomly divided into four groups (n = 8), maintaining an equal gender balance in each group. The control group received a basal diet, while experimental groups I, II, and III were given 5, 10, and 15% air-dried WPS maize diets, respectively, for 120 days.
The WPS maize was provided by a dairy plant in Yongdong Farm (118°72′ E, 35°33’ N, Gansu Province, China). Its nutrient content levels are listed in Table 1. Whole-plant silage maize was dried and crushed to a particle size of 1.65 mm and mixed according to the feed formula. The test rations were configured according to the Pig Feeding Standard (NY/T 65–2004) and the details are listed in Table 2.
The experiment was conducted at Gansu Bailu Tourism Farm from June to October 2022. Pigs were fed thrice daily (08:00, 13:00, and 19:00). Feeding was regulated to ensure a slight surplus in the trough after satiation, with water available ad libitum. All groups were maintained under identical feeding and management conditions.
Three days before the end of the experiment, fresh feces were collected from each group in the morning, midday, and evening. Daily collections from each pig were mixed and stored at −20°C. After the collection period, these samples were thawed, mixed, and sampled at 10% of the total weight. To every 100 g sample, 10 mL of 10% sulfuric acid was added. The dried feces were then crushed and stored at −20°C for fiber digestibility analysis.
At the end of the experiment, six pigs per group were randomly selected for slaughter. Their intestines were dissected to collect cecum and colon chyme in 15 mL centrifuge tubes for pH analysis. The remaining samples were stored in 5 mL sterilized EP tubes, flash-frozen in liquid nitrogen, and preserved at −80°C for analyzing fiber digestive enzyme activity, SCFAs concentration, and intestinal microbiota.
The apparent digestibility of neutral detergent fiber, acid detergent fiber, and crude fiber was determined and calculated according to Feed Analysis and Feed Quality Testing Techniques (Zhang, 2007).
T: The apparent digestibility of a nutrient; A: Nutrient content of the consumed diet; B: Content of the nutrient in manure.
The pH of the cecum and colonic chyme was measured using a PHSI-3F type (Leici Instrument, Shanghai, China) pH meter.
The 0.5 g of cecum and colonic chyme was combined with pre-cooled 0.9% sodium chloride solution (V:W, 9:1), homogenized for 2 min in an ice-water bath, and centrifuged at 3500 rpm for 10 min at 4°C. The obtained supernatant was stored at −20°C for testing. The ELISA kits for carboxymethyl cellulase, fibrous di-glucosidase, microcrystalline cellulase, and cellulase activity assay were obtained from Jiangsu Meimian Industrial Co., Ltd., China.
The SCFAs content in the cecum and colon was determined by gas chromatography (GC). Briefly, the contents were thawed and 0.5 g of the cecum was weighed in a centrifuge tube. It was added with ultrapure water (W:V, 1.0:2.5), and the tube was vortexed for 3–5 min, and then centrifuged at 5,000 rpm for 10 min. Then, 0.2 mL of metaphosphoric acid deproteinizing solution with 2-ethylbutyric acid (2-EB) was added to 1 mL of the obtained supernatant. The mixture was vortexed and then centrifuged for 30 min at 10,000 rpm and 4°C. Finally, the SCFAs content was determined using an Agilent 7,890 N gas chromatograph. The column length was 30 m, the inner diameter was 0.32 mm, the membrane thickness was 0.5 μm, and the injector and detector temperatures were 260°C and 280°C, respectively. The carrier gas was nitrogen at a flow rate of 2.5 mL/min.
Total bacterial DNA was extracted using the TianGen magnetic bead method DNA extraction kit (TianGen, China), following the manufacturer’s instructions. The purity and concentration of the extracted total DNA were assessed using 1% agarose gel electrophoresis. An appropriate volume of sample DNA was placed in a centrifuge tube and diluted to 1 ng/μL with sterile water. The diluted bacterial genomic DNA served as a template for amplifying the V4 hypervariable region of the 16S rRNA gene using primers (515F, 5´-GTGCCAGCMGCCGCGGTAA-3′ and 806R, 5´-GGACTACHVGGGTWTCTAAT-3′). Library construction was performed using the NEB Next® Ultra™ II FS DNA PCR-free Library Prep Kit (New England Biolabs, China). The library was then quantified by Qubit and Q-PCR. The qualified library was sequenced (PE 250) using the NovaSeq 6,000 platform. Following Reads splicing and filtering, OTUs (Operational Taxonomic Units) clustering, and species annotation, valid data were subjected to species annotation and abundance analysis.
One-way analysis of variance (one-way ANOVA) was conducted on the experimental data using SPSS 26.0. Multiple comparisons were made using the LSD method, and results are presented as mean ± standard deviation (Mean ± SEM).
Alpha diversity of the cecum microflora was analyzed using Qiime2 (Qiime2-2020.6). PCoA analysis was conducted with R software (Version 4.0.3). Significant species differences between groups were analyzed using LEfSe or R software. LEfSe analysis was conducted with LEfSe software with a default LDA score threshold of 4. R software’s T-test method was employed for analyzing significant species differences at each classification level. Spearman’s correlation analysis was used to examine the relationship between the cecal abundance of bacterial genera and SCFAs concentration in test pigs, using Origin 2022. Data with p < 0.05 denoted a significant difference, and those with p < 0.01 were considered highly significant differences.
The effects of different additive ratios of WPS maize on the fiber digestibility of Hezuo pigs are presented in Figure 1. The differences in neutral detergent fiber digestibility among groups were not significant (p > 0.05). However, acid detergent fiber digestibility in group II and group III was significantly higher than in the control group and group I (p < 0.05).
Figure 1. Fiber digestibility in different groups of Hezuo pigs with different additive proportions of whole plant silage maize diet. (A) NDF digestibility; (B) ADF digestibility). The same letter indicates a non-significant difference (p > 0.05), and different letters indicate a significant difference (p < 0.05); the same applies to other figures.
Data in Figure 2 displays the impact of varying WPS maize ratios on the pH of cecum and colonic chowders in Hezuo pigs. The pH levels in the cecum and colonic chowders of the test groups were lower compared to the control group. Notably, the colonic pH in group II was significantly lower than in the control group (p < 0.05). However, pH differences among other groups were not significant (p > 0.05). The cecum pH in group II was also significantly lower than in the other three groups (p < 0.05). This suggests that adding WPS maize to the diet may enhance fermentation in the cecum and colon, with a 10% addition (group II) leading to the highest fermentation level, potentially improving nutrient absorption.
Figure 2. Appendix (A) and colonic chyme pH (B) in different groups of Hezuo pigs with different additive proportions of whole plant silage maize diet.
Data in Figure 3 presents the effects of various WPS maize ratios on fiber digestive enzyme activities in the cecum and colon of Hezuo pigs. In the cecum, carboxymethyl cellulase activity in group II pigs was significantly higher than in other groups (p < 0.05). Cellobiase activity was highest in group II, significantly exceeding groups I and III (p < 0.05). However, groups I and III had significantly lower cellobiase activity compared to the control group (p < 0.05). Microcrystalline cellulase activity was significantly higher in the control and group II than in group III (p < 0.05), with group II showing the highest activity, though not significantly different from the control group (p > 0.05). Xylanase activity ranked from highest to lowest as group II, control group, group I, and group III, but without significant differences (p > 0.05). This indicates that a 10% addition of WPS maize enhanced fiber digestive enzyme activities in the cecum.
Figure 3. Effect of cellulase activity in the cecum (A–D) and colon (E–H) in different groups of Hezuo pigs with different additive proportions of whole plant silage maize diet. CMCase, carboxymethyl cellulase; MCCase, microcrystalline cellulase.
In the colon, group I had the highest cellobiase activity, significantly differing from groups II and III, and the control group (p < 0.05). The activity in group III was significantly lower than in group II (p < 0.05). Activities of carboxymethyl cellulase, microcrystalline cellulase, and xylanase did not show significant differences among groups (p > 0.05). This suggests that a 5% addition (group I) of WPS maize may boost fibrous disaccharidase activity in the colon of Hezuo pigs.
The results of the effect of different addition ratios of WPS corn on the concentration of SCFAs in the cecum and colonic chowders of Hezuo pigs are listed in Table 3. We found the highest concentration of acetic acid and total SCFAs in the cecum of group II Hezuo pigs, which was significantly higher than in group III (p < 0.05); the difference between the control group, group I, and group III was not significant (p > 0.05). The highest concentration of propionic acid in cecal chyme occurred in group II, exceeding that of the control group and group III (p < 0.05); it was also significantly higher in group I than in group III (p < 0.05). The isobutyric acid concentration in the cecum was highest in group II and significantly higher in groups I and III compared to the control group (p < 0.05). Concentrations of butyric acid, isovaleric, and valeric acids in the cecum were not significantly different among all groups (p > 0.05).
Table 3. Effect of different additive ratios of whole plant silage maize on volatile fatty acids in Hezuo pigs (mmol/L).
In the colonic chyme, only the isovaleric acid concentration in group II was significantly higher than in the other groups (p < 0.05). The differences among the control group, group I, and group III were not significant (p > 0.05). Concentrations of the other five SCFAs and the total SCFA did not differ significantly among the groups (p > 0.05). The above results indicate that the cecum is the main site of digestion and utilization of fibrous material from WPS maize by Hezuo pigs. Adding 10% WPS maize results in the highest concentration of SCFAs in the cecum of Hezuo pigs.
Based on these results, 16S rDNA sequencing was conducted on the cecum contents of Hezuo pigs in the control group and those supplemented with 10% WPS maize (group II). This was aimed to analyze the effect of the silage on the cecal microbiota.
The diversity analysis of the cecal microflora in group II Hezuo pigs revealed that the alpha diversity abundance indices, Chao1 and Observed-species, did not show significant differences from the control group (p > 0.05; Figures 4A,B). However, the diversity indices, Simpson and Shannon, were significantly higher in the silage-supplemented group (p < 0.05; Figures 4C,D). The β-diversity index did not differ significantly between the two groups (p > 0.05; Figure 4E). The principal coordinate analysis (PCoA) analysis, based on Unweighted Unifrac distance at the OUT level, indicated two principal components, PC1 and PC2, with contribution values of 34.46 and 23.40%, respectively. The plot showed a clear distinction between the cecal flora of the control and silage-supplemented groups, suggesting significant changes in the overall structure and diversity of microbial flora (Figure 4F).
Figure 4. Box plots showing differences between groups in α-diversity Chao1 index (A). Observed-species index (B). Simpson’s index (C). Shannon’s index (D). β-diversity (E), and PCoA analysis based on Unweighted UniFrac distances (F) (* denotes p < 0.05).
The top 10 species of Hezuo porcine cecum microflora at phylum and genus levels are presented in Figures 5A,B, respectively. The major bacterial phyla in the cecal microbiota of Hezuo pigs were Firmicutes, Bacteroidota, and Spirochaetota (Figure 5A), with their relative abundance comprising over 95% of the cecal microbiota in both the control and WPS corn addition groups. Streptococcus and Muribaculaceae were identified as the main dominant genera in the control group, with mean abundance values of 9.72 and 7.33% (Figure 5B). In the WPS corn addition group, p-251-o5 and Rikenellaceae_RC9_gut_group were the predominant genera, with mean abundances of 8.46 and 7.28%, respectively. Relative mean abundance values of p-251-o5, Clostridium_sensu_stricto_1, Rikenellaceae_RC9_gut_group, Alloprevotella, UCG-005, and Terrisporobacter in the WPS maize addition group increased by 38.19, 16.77, 24.56, 30.21, 42.66, and 44.27% respectively, compared to the control group.
Figure 5. Mean relative abundance of major bacteria at the phylum (A) and genus (B) levels in the cecal colony control and 10% silage maize addition groups.
Figure 6 shows a significant difference analysis of bacterial groups annotated to the family and genus levels. At the family level, compared to the control group, the WPS corn additive group, comprising Oscillospiraceae, Eptostreptococcaceae, Eubacterium_coprostanoligenes_group, Bacteroidaceae, RF39 etc. showed a significant increase in relative abundance (p < 0.05); Lachnospiraceae, Streptococcaceae, and Lactobacillaceae decreased significantly in relative abundance (p < 0.05). At the genus level, UCG-005, Terrisporobacter, Bacteroides, RF39, Parabacteroides etc. exhibited significant increases in relative abundance (p < 0.05); Streptococcus, Lactobacillus, Prevotellaceae_NK3B31_group, Eubacterium_xylanophilum_group, and Frisingicoccus showed a significant decrease in relative abundance (p < 0.05).
Figure 6. Bar graph of t-test showing differences in cecum flora between control and experimental group of pigs.
According to the LEfSe analysis results (Figure 7), the main bacterial taxa enriched in the cecum of control Hezuo pigs were Lactobacillaceae, Lactobacillus, and Lachnospiraceae. In contrast, the cecum of WPS corn-supplemented Hezuo pigs showed enrichment in Oscillospirales, Oscillospiraceae, and Peptostreptococcaceae_Tissierellales (LDA Score > 4).
Spearman’s correlation analysis of SCFAs between bacterial genus levels in the cecum of Hezuo pigs in the WPS maize addition group and the control group revealed that the relative abundance of Bacteroides and NK4A214_group was significantly positively correlated with the contents of valeric and isovaleric acid (p < 0.05), and was significantly negatively correlated with propionic acid (Figure 8). The relative abundance of UCG-010 was significantly positively correlated with acetate (p < 0.05), and negatively correlated with butyrate (p < 0.05). The relative abundance of Actinobacillus was significantly positively correlated with butyrate (p < 0.05).
Figure 8. Correlation analysis between cecum intestinal microbiota and SCFAs in Hezuo pigs (* indicates p < 0.05) (ACE, acetate; PRO, propionate; ISOB, isobutyrate; BUTY, butyrate; ISOV, isovalerate; VAL, valerate).
Dietary fiber, the feed component with indigestible plant cell wall components, cannot be digested by innate animal digestive enzymes (Peng, 1999). In pigs, the small intestine is the primary site for nutrient digestion, where most proteins, lipids, and carbohydrates are digested and absorbed. Substances that remain undigested in the small intestine, particularly fibrous materials, pass into the large intestine. Here, they are utilized by caecum and colon microorganisms (Burkey et al., 2009). Chinese local pig breeds, with their high fiber digestibility, exhibit a high tolerance to roughage (Tan et al., 2020). He et al. (2022) discovered that high-fiber diets significantly decreased the average daily feed intake in Xinhuai pigs. However, this did not negatively impact their average daily weight gain, feed-to-weight ratio, or energy utilization in the hindgut. Additionally, the diets did not adversely affect the apparent digestibility of crude proteins and neutral detergent fibers, and they moderately enhanced the apparent digestibility of crude fibers (He et al., 2022). Tan et al. (2022) observed that Tibetan pigs, under grazing conditions, demonstrate a superior ability to digest fiber, their fibrous substance digestibility exceeds that of confined-fed pigs by over 10%. Wang et al. (2023) noted that introducing 7% bran into the diet of Meishan pigs notably reduced the apparent digestibility of protein and energy, however, this did not significantly alter the digestibility of neutral detergent fiber or growth performance (Huan et al., 2020). These studies collectively indicate that local pig breeds inherently possess robust fiber digestion capabilities, and incorporating a certain amount of fiber does not detrimentally affect their growth and digestive processes. In this study, we analyzed fiber digestibility in Hezuo pigs fed WPS maize rations with varying additive ratios. We found that adding 10% WPS maize substantially increased the digestibility of acid detergent fiber by 4.92%, respectively, compared to the control group. Changes in the pH of the gastrointestinal tract directly affect the digestive and absorptive capacity of the animal intestine. An appropriate intestinal pH ensures the activity of digestive enzymes and provides a suitable environment for intestinal probiotic bacteria. This also improves the intestinal microecological balance (Luckstadt et al., 2004; Kim et al., 2005). Our results showed that the dietary addition of 10% WPS maize significantly reduced the pH of the cecum and colon of Hezuo pigs. This reduction may be because the pH of WPS maize is generally in the range of 3.9–4.2. The prolonged intake of this feed by Hezuo pigs stimulates gastric acid secretion, thus reducing the cecum pH.
Microorganisms in the digestive tract of animals produce cellulase enzymes that catalyze the breakdown of cellulose, hemicellulose, and some related polysaccharides (Li, 2019). Carboxymethyl cellulase and cellobiase aid in the digestion of cellulose, while xylanase aids in the digestion of hemicellulose (Jing et al., 2013). The level of cellulase activity is often related to the level of dietary fiber that provides the intestinal microorganisms with a fermentation substrate. Measuring the activities of microcrystalline cellulase, carboxymethyl cellulase, cellobiase, and xylanase often reflects the dietary fiber degradation ability of the animal. Varel et al. (1987) showed that increasing alfalfa fiber content (40%) in the diet of sows increased the degradation rate of xylan and cellulose, increased the number and activity of cellulolytic bacteria, and increased the number of cellulase and xylanase enzymes in pigs. This study showed that the addition of 10% WPS maize significantly increased the cecum fibrous disaccharidase activity, carboxymethyl cellulase activity, and microcrystalline cellulase activity in Hezuo pigs. It indicates that the WPS maize diet increased the relative bacterial population of certain fiber-degrading bacteria in the cecum. This increases enhanced cellulase activity and promotes the digestion and utilization of fiber in Hezuo pigs, but the mechanism still needs further research.
Most of the carbohydrates in the hindgut of monogastric animals and the rumen of ruminants are fermented by microorganisms to produce a large number of monocarboxylic acids (organic acids containing 2–4 carbon atoms and 1 carbonyl end) for the body to utilize, such as short-chain fatty acids, β-hydroxybutyric acid, acetoacetic acid, and lactic acid, etc. (Moschen et al., 2012). Among them, short-chain fatty acids, as important energy substances, can provide 15 to 20% of energy for monogastric animals and 75% of energy for ruminants (Bergman, 1990). In addition, short-chain fatty acids play an important role in maintaining the morphology and function of intestinal epithelial cells, intestinal microecological balance and intestinal immune response (Liu et al., 2018). Among them, acetic acid is converted into acetate, which improves the protective function of the intestinal epithelium (Chang et al., 2021). Propionic acid inhibits the occurrence of intestinal inflammation (Dupraz et al., 2021). Butyric acid is converted into butyrate, which stimulates mucin secretion in the intestinal tract and directly supplies energy to the intestinal mucosa (Gaudier et al., 2009). Hung et al. (2019) found that high levels of fiber in the diet promoted the production of SCFAs by intestinal bacteria. Carneiro et al. (2008) demonstrated that wheat fiber significantly lowered the pH of the piglet cecum and increased the cecal content of acetic and butyric acids. Pu et al. (2020) showed that feeding high-fiber diets to Suhuai pigs promoted the metabolism of SCFAs in the cecum, increasing the amount of acetic acid. Acetic acid primarily comes from cellulose and hemicellulose in plant cell walls, which are degraded by rumen’s fibrolytic bacteria (Iwamoto et al., 2001). In this study, the addition of 10% WPS maize significantly increased the cecum content of propionic acid, isobutyric acid, and total SCFAs in Hezuo pigs. It also increased the colon content of isovaleric acid, suggesting that the plant fiber of WPS maize is easily digested and utilized by the cecum of Hezuo pigs.
The intestinal flora composition is the Primary factor that influences the level of SCFAs (Dalile et al., 2019). Certain intestinal bacteria have been shown to ferment indigestible fibers into SCFAs, such as propionic acid and butyric acid. These processes mediate host energy homeostasis, immunomodulation, and mucosal barrier function (Kelly et al., 2015; Makki et al., 2018; Mendes et al., 2022). In this study, the overall structure and diversity of pig cecum flora in the WPS group changed significantly. These changes were notable compared to the control group, and the microflora alpha diversity indices, Simpson and Shannon, were significantly higher. This suggests that feeding WPS maize may promote gut health in Hezuo pigs. It does this by increasing the abundance and diversity of their gut flora and responding to changes in dietary fiber levels. Further analysis showed that at the phylum level, the major cecal bacteria of Hezuo pigs were Firmicutes, Bacteroidota, and Spirochaetota. Both Shoaie et al. (2013) and Greenhill (2015) showed that the intestinal flora of fattening pigs mainly consists of representatives of thick-walled Firmicutes and Bacteroidota. These findings are consistent with our results; the abundance of Bacteroidota increased and the abundance of Firmicutes decreased in the colons of Hezuo pigs fed WPS maize. Firmicutes and Bacteroidota are widely present in the intestines of herbivores. Firmicutes aid in the fermentation of polysaccharides in the intestines. Together with Bacteroidota, Firmicutes help hosts digest food that they cannot digest themselves. Firmicutes are major cellulose decomposers, whereas Bacteroidetes are the primary polysaccharide-degrading and utilizing bacteria. Together, they increase the utilization rate of carbohydrates, proteins, and other substances in the host. The stable balance of these two phyla plays a vital role in maintaining the micro-ecology and health of the host gut (Hooper et al., 2001; Hooper, 2004; Ley et al., 2008; Ruiz et al., 2013). At the genus level, the relative mean abundance of Alloprevotella and UCG-005 in the porcine cecum was elevated by 30.21 and 42.66%, respectively, in the WPS maize addition group compared to the control group. UCG-005 has fiber-degrading activity, and its abundance in the cecum increased significantly with higher levels of fiber in the diet. Alloprevotella is closely related to the digestion of dietary fiber and is abundant in the cecum of the Sujang pig and Jinhua pig (Ren et al., 2020; Zhang et al., 2020). It is the dominant genus, with relative abundance increasing with higher levels of dietary fiber. Consumption of high-insoluble fiber diets during the second trimester of gestation increases the relative abundance of Alloprevotella in the feces of sows (Liu, 2019). Alloprevotella levels were higher in the feces of grazing Tibetan pigs than in housed Tibetan pigs and Duroc×Landrace×Yorkshire pigs, indicating their higher fiber degradation capacity. Overall, Alloprevotella and UCG-005 may be closely related to crude fiber digestion in Hezuo pigs.
Our correlation analysis of differential genera with SCFAs revealed that the relative abundance of Bacteroides and NK4A214_group was significantly positively correlated with the amounts of valeric acid and isovaleric acid. They were significantly negatively correlated with propionic acid. Additionally, the relative abundance of Actinobacillus was significantly positively correlated with butyric acid. This indicates that feeding WPS maize can influence SCFAs production. This, in turn, affects fiber digestion.
Adding 10% WPS maize improves fiber digestibility, lowers cecal and colonic chow pH, and increases intestinal cellulase activity and SCFA production in Hezuo pigs. It also enhances intestinal microbiota abundance. These findings provide valuable guidance for the characterization of roughage tolerance in Hezuo pigs.
The datasets presented in this study can be found in online repositories. The names of the repository/repositories and accession number(s) can be found in the article/supplementary material.
The animal study was approved by Animal Protection and Use Committee of Gansu Agricultural University. The study was conducted in accordance with the local legislation and institutional requirements.
LW: Data curation, Validation, Visualization, Writing – original draft, Writing – review & editing. PW: Funding acquisition, Project administration, Software, Writing – review & editing. ZY: Conceptualization, Resources, Supervision, Writing – review & editing. PZ: Software, Visualization, Writing – review & editing. XY: Writing – review & editing. RJ: Investigation, Writing – review & editing. YL: Writing – review & editing. JY: Writing – review & editing. SG: Funding acquisition, Project administration, Resources, Writing – review & editing. QY: Conceptualization, Formal analysis, Methodology, Project administration, Resources, Writing – review & editing.
The author(s) declare financial support was received for the research, authorship, and/or publication of this article. This work was supported by the Natural Science Foundation of Gansu Province (23JRRA1422) and the Animal Husbandry Pig Industry Technology Innovation Team Project of Gansu Agricultural University (GAU-XKTD-2022-25).
The authors declare that the research was conducted in the absence of any commercial or financial relationships that could be construed as a potential conflict of interest.
All claims expressed in this article are solely those of the authors and do not necessarily represent those of their affiliated organizations, or those of the publisher, the editors and the reviewers. Any product that may be evaluated in this article, or claim that may be made by its manufacturer, is not guaranteed or endorsed by the publisher.
Bergman, E. N. (1990). Energy contributions of volatile fatty acids from the gastrointestinal tract in various species. Physiol. Rev. 70, 567–590. doi: 10.1152/physrev.1990.70.2.567
Burkey, T. E., Skjolass, K. A., and Minton, J. E. (2009). Board-invited review: porcine mucosal immunity of the gastrointestinal tract. J. Anim. Sci. 87, 1493–1501. doi: 10.2527/jas.2008-1330
Carneiro, M. S. C., Lordelo, M. M., Cunha, L. F., and Freire, J. P. B. (2008). Effects of dietary fibre source and enzyme supplementation on faecal apparent digestibility, short chain fatty acid production and activity of bacterial enzymes in the gut of piglets. Anim. Feed Sci. Tech. Animal. 146, 124–136. doi: 10.1016/j.anifeedsci.2007.12.001
Chang, Y. H., Jeong, C. H., Cheng, W. N., Choi, Y., Shin, D. M., Lee, S., et al. (2021). Quality characteristics of yogurts fermented with short-chain fatty acid-producing probiotics and their effects on mucin production and probiotic adhesion onto human colon epithelial cells. J. Dairy Sci. 104, 7415–7425. doi: 10.3168/jds.2020-19820
Cheng, J. L., and Yan, J. C. (2008). Application and study of dietary fiber in swine production. Anim. Agric. 6, 50–53. doi: 10.3969/j.issn.1006-6314-B.2008.06.016
Dalile, B., Van Oudenhove, L., Vervliet, B., and Verbeke, K. (2019). The role of short-chain fatty acids in microbiota-gut-brain communication. Nat. Rev. Gastro. Hepat. 16, 461–478. doi: 10.1038/s41575-019-0157-3
De Lange, C. F. M., Pluske, J., Gong, J., and Nyachoti, C. M. (2010). Strategic use of feed ingredients and feed additives to stimulate gut health and development in young pigs. Livestock Sci. 134, 124–134. doi: 10.1016/j.livsci.2010.06.117
Dupraz, L., Magniez, A., Rolhion, N., Richard, M. L., Da Costa, G., Touch, S., et al. (2021). Gut microbiota-derived short-chain fatty acids regulate IL-17 production by mouse and human intestinal γδ T cells. Cell Rep. 36:109332. doi: 10.1016/j.celrep.2021.109332.9
Gaudier, E., Rival, M., Buisine, M. P., Robineau, I., and Hoebler, C. (2009). Butyrate enemas upregulate MUC genes expression but decrease adherent mucus thickness in mice colon. Physiol. Res. 58, 111–119. doi: 10.33549/physiolres.931271
Gill, S. K., Rossi, M., Bajka, B., and Whelan, K. (2021). Dietary fibre in gastrointestinal health and disease. Nat. Rev. Gastro. Hepat. 18, 101–116. doi: 10.1038/s41575-020-00375-4
Greenhill, C. (2015). Firmicutes and Bacteroidetes involved in insulin resistance by mediating levels of glucagon-like peptide 1. Nat. Rev. Endocrinol. 11:254. doi: 10.1038/nrendo.2015.40
He, X. F., Du, T. R., Pu, G., Zhang, Y. Q., Hao, S. S., Li, Q., et al. (2022). Effects of high dietary fiber levels on the growth performance, serum biochemical indexes, gut relative weight and apparent digestibility of nutrients in Xinhuai pigs. Anim. Husb. Vet. Med. 54, 31–35.
Hooper, L. V. (2004). Bacterial contributions to mammalian gut development. Trends Microbiol. 12, 129–134. doi: 10.1016/j.tim.2004.01.001
Hooper, L. V., Wong, M. H., Thelin, A., Hansson, L., Falk, P. G., and Gordon, J. I. (2001). Molecular analysis of commensal host-microbial relationships in the intestine. Science 291, 881–884. doi: 10.1126/science.291.5505.881
Huan, W., Yang, M., Pu, G., Fan, L. J., Gao, C., Niu, P. P., et al. (2020). Effects of fiber levels in diet on rectal nitrogen and phosphorus contents and colonic bacteria number in Suhuai pigs. Anim. Husb. Vet. Med. 52, 45–49.
Hung, D. Y., Cheng, Y. H., Chen, W. J., Hua, K. F., Pietruszka, A., Dybus, A., et al. (2019). Bacillus licheniformis-fermented products reduce diarrhea incidence and alter the fecal microbiota community in weaning piglets. Animals 9:1145. doi: 10.3390/ani9121145
Iwamoto, M., Asanma, N., and Hino, T. (2001). Effects of pH and electron donors on nitrate and nitrite reduction in ruminal microbiota. J. Anim. Sci. 72, 117–125.
Jing, C. L., Li, F. C., Wang, X. P., and Wang, C. Y. (2013). Effects of dietary ADF levels on growth performance, nitrogen metabolism, cellulase activity and caecum fermentation of rex rabbits from weaning to 3-month-old. Acta Vet. Zootech. Sinica 44, 1417–1424.
Kelly, C. J., Zheng, L., Campbell, E. L., Saeedi, B., Scholz, C. C., Bayless, A. J., et al. (2015). Crosstalk between microbiota-derived short-chain fatty acids and intestinal epithelial HIF augments tissue barrier function. Cell Host Microbe 17, 662–671. doi: 10.1016/j.chom.2015.03.005
Kim, Y. Y., Kil, D. Y., Oh, H. K., and Han, I. K. (2005). Acidifier as an alternative material to antibiotics in animal feed. Asian Austral. J. Anim. 18, 1048–1060. doi: 10.5713/ajas.2005.1048
Knudsn, K. E. B., Hedemann, M. S., and Laerke, H. N. (2012). The role of carbohydrates in intestinal health of pigs. Anim. Feed Sci. Technolo. 173, 41–53. doi: 10.1016/j.anifeedsci.2011.12.020
Ley, R. E., Hamady, M., Lozupone, C., Turnbaugh, P. J., Ramey, R. R., Bircher, J. S., et al. (2008). Evolution of mammals and their gut microbes. Science 320, 1647–1651. doi: 10.1126/science.1155725
Li, Z. J.. (2019). Isolation and degradation characteristics of cellulose degrading bacteria from rumen of black goats. [master’s thesis]. China, Hunan: Hunan Agricultural University.
Liu, Y. (2019). Effects of dietary soluble or insoluble fiber intake in late gestation on reproductive performance, immune function and intestinal microbiota of sows around parturition. [master’s thesis]. China (Ya’an): Sichuan Agricultural University.
Liu, B. S., Wang, W. J., Zhu, X. Y., Sun, X., Xiao, J. N., Li, J. F., et al. (2018). Response of gut microbiota to dietary fiber and metabolic interaction with SCFAs in piglets. Front. Microbiol. 9:2344. doi: 10.3389/fmicb.2018.02344
Luckstadt, C., Senkoylu, N., Akyurekh, H., and Agma, A.. (2004). Acidifier-a modern alternative for antibiotic free feeding in livestock production, with special focus on broiler production. Vet. Zootech. 27, 91–93.
Makki, K., Deehan, E. C., Walter, J., and Bäckhed, F. (2018). The impact of dietary fiber on gut microbiota in host health and disease. Cell Host Microbe 23, 705–715. doi: 10.1016/j.chom.2018.05.012
Mendes, N. P., Ribeiro, P. V. M., and Alfenas, R. C. G. (2022). Does dietary fat affect advanced glycation end products and their receptors? A systematic review of clinical trials. Nutr. Rev. 80, 598–612. doi: 10.1093/nutrit/nuab095
Moschen, I., Bröer, A., Galić, S., Lang, F., and Bröer, S. (2012). Significance of short chain fatty acid transport by members of the monocarboxylate transporter family (MCT). Neurochem. Res. 37, 2562–2568. doi: 10.1007/s11064-012-0857-3
Peng, J. (1999). Dietary Fiber: definition, composition, analytical methods and processing implications. Anim. Sci. Abroad 4, 8–11.
Pu, G., Li, P., Du, T., Niu, Q., Fan, L., Wang, H., et al. (2020). Adding appropriate fiber in diet increases diversity and metabolic capacity of distal gut microbiota without altering fiber digestibility and growth rate of finishing pig. Front. Microbiol. 11:533. doi: 10.3389/fmicb.2020.00533
Ren, M. M., Yang, H., Xiang, Y., Zhang, X. J., Xu, E., Shen, L. L., et al. (2020). Effects of dietary fiber levels on growth performance, microbial community structure and short-chain fatty acid content in cecum of Jinhua pigs. Chin. J. Anim. Nutr. 32, 2575–2585. doi: 10.3969/j.issn.1006-267x.2020.06.017
Ren, J. S., Zhang, J., Zhang, M. L., Sun, G. H., and Wang, F. B. (2021). Effects of different supplementation ratio of whole corn silage on growth performance, blood biochemical indices and feed cost of Bamei pigs. China Feed. 13, 34–38. doi: 10.15906/j.cnki.cn11-2975/s.20211307
Ruiz, L., Margolles, A., and Sánchez, B. (2013). Bile resistance mechanisms in lactobacillus and Bifidobacterium. Front. Microbiol. 4:396. doi: 10.3389/fmicb.2013.00396
Shoaie, S., Karlsson, F., Mardinoglu, A., Nookaew, I., Bordel, S., and Nielsen, J. (2013). Understanding the interactions between bacteria in the human gut through metabolic modeling. Sci Rep. 3:2532. doi: 10.1038/srep02532
Tan, Z. K., Shang, Z. D., Chu, G. Y., Cai, C. J., Zhu, Y. B., Deng, W., et al. (2022). Study on dietary fibre digestion and its correlation with intestinal microbiome of Tibetan pigs. Acta Vet. Zootech. Sinica 53, 3063–3078. doi: 10.11843/j.issn.0366-6964.2022.09.022
Tan, B. E., Wu, S. S., He, J. H., and He, J. H.. (2020). Metabolic mechanism of coarse feeding tolerance and quality traits formation based on microbial metabolism in local pigs. Chin. J. Anim. Nutr. 32, 2941–2946. doi: 10.3969/j.issn.1006-267x.2020.07.001
Varel, V. H., Robinson, I. M., and Jung, H. J. (1987). Influence of dietary fiber on xylanolytic and cellulolytic bacteria of adult pigs. Appl. Environ. Microbiol. 53, 22–26. doi: 10.1128/aem.53.1.22-26.1987
Wang, Y. P., Zhao, X. Y., Wang, H. Z., Zhang, G. P., Jinag, F. G., Wang, Y., et al. (2023). Effects of different supplementation level of whole plant silage corn on growth performance, pollutant emission and fecal microbial flora of fattening pigs. J. Dom. Anim. Ecol. 44, 40–46. doi: 10.3969/j.issn.1673-1182.2023.05.007
Yin, X. T., Wang, P. F., Yan, Z. Q., Yang, Q. L., Huang, X. Y., and Gun, S. B. (2024). Effects of whole-plant corn silage on growth performance, serum biochemical indices, and fecal microorganisms in Hezuo pigs. Animals 14:662. doi: 10.3390/ani14050662
Zhang, L. Y.. (2007). Feed analysis and feed quality testing techniques. Beijing: China Agricultural University Press. 49–80.
Zhang, J. S., Xu, P., Tao, Y., Ni, L. G., Zhou, C. B., Cai, J. Y., et al. (2020). Analysis on the difference of intestinal microflora of Sujing piglets with different growth performance. Microbiol. China 47, 4240–4249. doi: 10.13344/j.microbiol.china.200130
Zhao, Y., Lu, X., Cheng, Z., Tian, M., Qiangba, Y., Fu Q, R., et al. (2019). Comparative proteomic analysis of Tibetan pig spermatozoa at high and low altitudes. BMC Genom. 20, 569–568. doi: 10.1186/s12864-019-5873-0
Keywords: Hezuo pigs, pH, whole plant silage corn, cellulase, short-chain fatty acids, intestinal microbiota
Citation: Wang L, Wang P, Yan Z, Zhang P, Yin X, Jia R, Li Y, Yang J, Gun S and Yang Q (2024) Whole-plant silage maize to improve fiber digestive characteristics and intestinal microbiota of Hezuo pigs. Front. Microbiol. 15:1360505. doi: 10.3389/fmicb.2024.1360505
Received: 23 December 2023; Accepted: 11 April 2024;
Published: 25 April 2024.
Edited by:
Caihong Hu, Zhejiang University, ChinaReviewed by:
Nazir Ahmad Khan, University of Agriculture, Peshawar, PakistanCopyright © 2024 Wang, Wang, Yan, Zhang, Yin, Jia, Li, Yang, Gun and Yang. This is an open-access article distributed under the terms of the Creative Commons Attribution License (CC BY). The use, distribution or reproduction in other forums is permitted, provided the original author(s) and the copyright owner(s) are credited and that the original publication in this journal is cited, in accordance with accepted academic practice. No use, distribution or reproduction is permitted which does not comply with these terms.
*Correspondence: Qiaoli Yang, yangql0112@163.com
Disclaimer: All claims expressed in this article are solely those of the authors and do not necessarily represent those of their affiliated organizations, or those of the publisher, the editors and the reviewers. Any product that may be evaluated in this article or claim that may be made by its manufacturer is not guaranteed or endorsed by the publisher.
Research integrity at Frontiers
Learn more about the work of our research integrity team to safeguard the quality of each article we publish.