- 1College of Basic Medicine, Chengde Medical University, Chengde, China
- 2Chengde Center for Disease Control and Prevention, Chengde, China
Rodents have been confirmed as hosts of various vector-borne zoonotic pathogens and are important for the maintenance of these microbes in nature. However, surveillance for zoonotic pathogens is limited for many wild rodent species in China, so our knowledge of pathogen ecology, genetic diversity, and the risk of cross-species transmission to humans is limited. In this study, 165 spleen samples of Daurian ground squirrels (Spermophilus dauricus) were collected from Weichang Manchu and the Mongolian Autonomous County of Hebei Province, China, and Rickettsia, Bartonella, and Anaplasma were identified by DNA detection using polymerase chain reaction (PCR). Sequence analysis identified eight bacterial pathogens: R. raoultii, R. sibirica, Candidatus R. longicornii, B. washoensis, B. grahamii, B. jaculi, A. capra, and Candidatus Anaplasma cinensis. Co-infection of B. grahamii and R. raoultii in one sample was observed. Our results demonstrated the genetic diversity of bacteria in Daurian ground squirrels and contributed to the distribution of these pathogens. Six species, A. capra, R. raoultii, R. sibirica, Candidatus R. longicornii, B. washoensis, and B. grahamii, are known to be pathogenic to humans, indicating a potential public health risk to the local human population, especially to herders who frequently have close contact with Daurian ground squirrels and are thus exposed to their ectoparasites.
Introduction
Rodents are the most diverse taxa of mammals (Huchon et al., 2002; Wilson and Reeder, 2005) and serve as reservoirs of many important zoonotic pathogens (Meerburg et al., 2009; Han et al., 2015). These pathogens consist of bacteria, viruses, and parasites. They comprise 66 identified zoonotic pathogens harbored by a minimum of 217 rodent species as reservoirs. Additionally, at least 79 rodent species can host between 2 and 11 zoonotic causative agents (Han et al., 2015). In recent years, an increasing number of viruses have been discovered by next-generation sequencing technology in rodents, some of which possess zoonotic potential (Chen et al., 2023). Furthermore, most of these zoonotic pathogens are indirectly transmitted from rodents to humans via arthropod vectors (Meerburg et al., 2009).
Rickettsia and Anaplasma (Order Rickettsiales) are obligate intracellular bacteria that are transmitted by ticks (Darby et al., 2007). Some Rickettsia species have been detected in rodents, although the role that rodents play in their life cycle is unclear (Schex et al., 2011; Kuo et al., 2015; Lu et al., 2019). Unlike Rickettsia, Anaplasma cannot be transmitted transovarially in ticks; therefore, vertebrate mammals such as rodents are required to complete their life cycle (Battilani et al., 2017). Bacteria belonging to the genus Bartonella include several zoonotic species that can be transmitted from animals to humans through the bites of infected bloodsucking arthropods (Deng et al., 2012), as well as through the scratch of an infected cat or by contact with the infected feces of a vector (Krügel et al., 2022). Although Bartonella DNA has been detected in ticks, the role of ticks as vectors in the transmission of Bartonella spp. remains unclear (Telford and Wormser, 2010). As the natural hosts, more than half of all Bartonella species, including human pathogens, have been identified in rodents to date (Jian et al., 2022).
The Daurian ground squirrel (Spermophilus dauricus) may be a potential reservoir of tick-borne pathogens. In China, a tentative Ehrlichia species was identified from this ground squirrel in Inner Mongolia (Li et al., 2023a). In addition, B. rochalimae and B. washoensis were detected in Inner Mongolia (Li et al., 2023b). Except for the three above-mentioned bacterial species and Y. pestis, no other vector-borne intracellular bacteria have been found in Daurian ground squirrels. In our preliminary studies, herders parasitized by ticks were found in the Bashang area of Weichang Manchu and the Mongolian Autonomous County of Hebei Province, China. Therefore, when herders enter the habitat of Daurian ground squirrels, they may come into contact with the rodents and be exposed to their ectoparasites. A previous study showed that intracellular bacterial pathogens, including R. raoultii and Candidatus R. tarasevichiae, were identified in 26 ticks collected from humans (Xue et al., 2023) in Weichang County, as well as A. ovis with zoonotic potential. Therefore, to better understand the genetic diversity of intracellular bacterial pathogens, Daurian ground squirrels were collected from the Bashang area to screen for the presence of neglected vector-borne Anaplasma, Bartonella, and Rickettsia. The results will help to assess the potential transmission risk of these pathogens to humans.
Materials and methods
Sample collection of rodents and DNA extraction
From April to October 2021, rodents were captured alive using baited cages with a treadle release mechanism from one sampling site (approximately 3 km2) located in the Bashang area of Weichang Manchu and Mongolian Autonomous County, Hebei Province, China (Figure 1). The cages were set at night and then retrieved the following day. Two hundred cages were used in each trapping session, and two or three trapping sessions were conducted each month. Based on the standard taxonomic characteristics (Wang, 2003), the rodents were first identified morphologically at the species level. After species identification, the sampled rodents were euthanized with an isoflurane overdose before surgery to minimize suffering. Spleen tissue samples were aseptically collected from each individual and stored on dry ice. A volume of 200 μL of spleen tissue suspension was prepared by homogenizing a 30 mg sample in 200 μL of TL buffer. DNA was isolated from the spleen suspension of each rodent using a Tissue DNA Kit (Omega, Norcross, GA, United States) according to the manufacturer’s protocol. The extracted DNA was eluted in 80 μL of elution buffer and stored at −20°C before pathogen detection and confirmation of rodent species by molecular methods. All samples tested negative for Yersinia pestis by detecting immunoglobulin (IgG) antibodies against the F1 antigen and caf1 and pla genes before screening for other pathogens (Zhao et al., 2017). The rodent species was confirmed by sequence analysis of the mitochondrial cytochrome b (mt-cyt b) gene (Guo et al., 2013). This study was approved by the Scientific Ethics Committee of the Chengde Medical University (No. 202004).
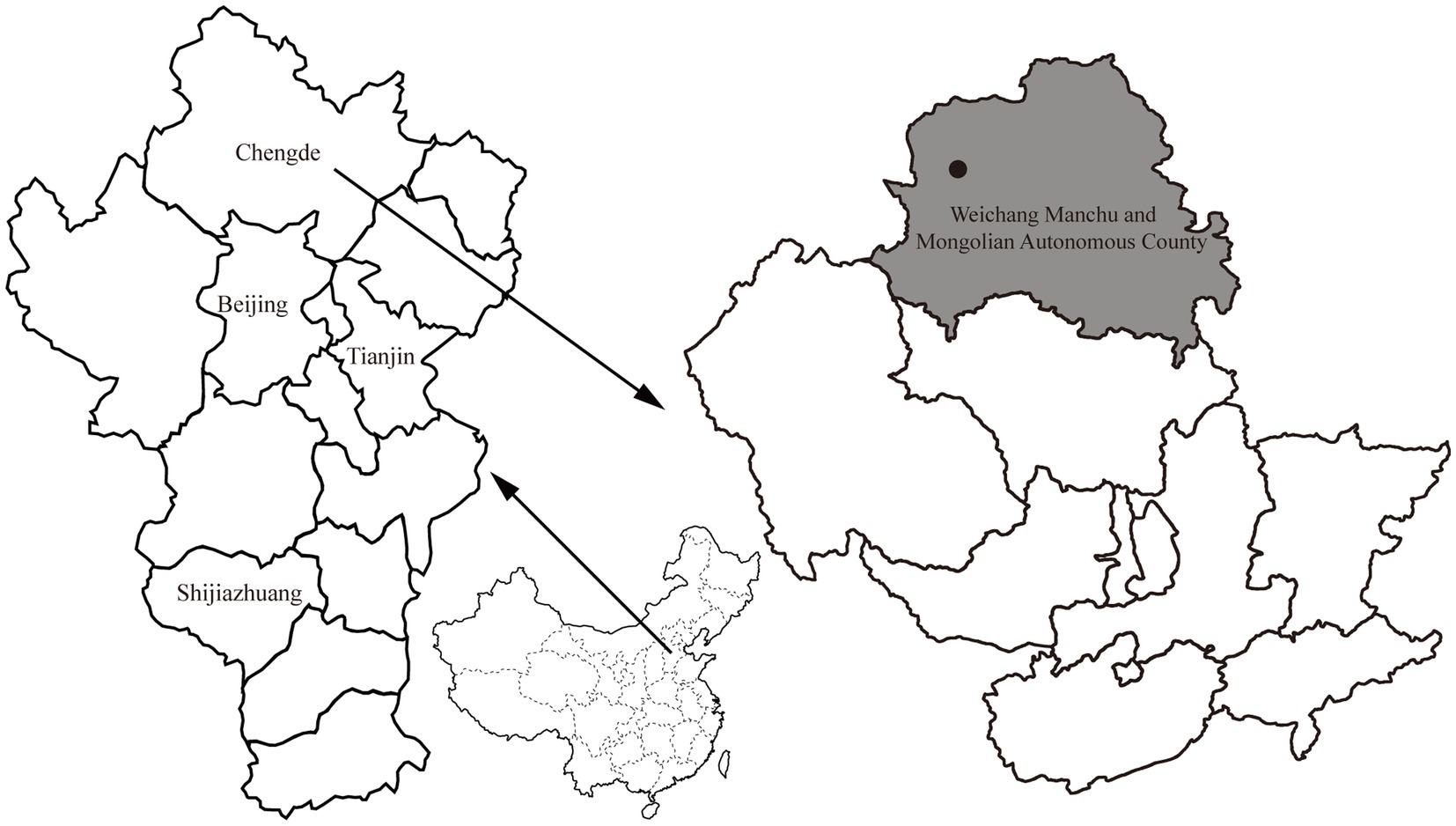
Figure 1. Map with the location of the sampling site (black circle) in Weichang Manchu and Mongolian Autonomous County (shown in gray), Hebei, China.
Molecular detection of Rickettsia, Anaplasma, and Bartonella
Rickettsia, Anaplasma, and Bartonella were detected for the presence of DNA using polymerase chain reaction (PCR). Rickettsia was screened using semi-nested PCR targeting a conserved region of the outer membrane protein (ompA) (Ishikura et al., 2003). The DNA of Bartonella was detected by amplifying a fragment of the citrate synthase (gltA) gene (Jian et al., 2022). Anaplasma was detected by semi-nested PCR using the primer pairs fD1/Eh-out2 and fD1/Eh-gs1 for primary and secondary rounds, targeting the 16S rRNA (Weisburg et al., 1991; Wen et al., 2002). In addition, the DNA of Candidatus Anaplasma cinensis was detected to confirm its presence by amplifying the partial gltA gene using primers designed for this study. The partial groEL gene of Candidatus Anaplasma cinensis was amplified to better characterize its genetic characteristics. The primers used in the present study are listed in Table 1. For Candidatus A. cinensis, the PCR for the primary round of semi-nested PCR was performed in a 20 μL reaction volume containing 10 μL of Premix Taq (Takara, Dalian, China), 1.6 μL of DNA, 1.0 μL of each primer (10 pmol), and 5.4 μL of water. The secondary round consisted of a final volume of 50 μL that contained 25 μL of PCR mixture Premix Taq (Takara, Dalian, China), 3 μL of the first round PCR products, 2 μL of each primer (10 pmol), and 18 μL of water. The same thermal cycling conditions were used for both rounds and were as follows: pre-denaturation at 94°C for 5 min; 35 cycles of denaturation at 94°C for 40 s; annealing at 56°C for 40 s; elongation at 72°C for 1 min; and a final extension at 72°C for 7 min. Double-distilled water was used as a negative control. In addition, positive controls were included in the reactions using DNA deposited in our laboratory.
The PCR products were separated by electrophoresis using 1% agarose gels, and the bands were observed after staining. The PCR products of the expected size were purified using a MiniBEST Agarose Gel DNA Extraction Kit Ver.4.0 (TaKaRa, Dalian, China). Due to the sequences being less than 700 bp, the amplicons were bidirectionally sequenced using the primers for the secondary round of the PCR after purification. If double peaks were visible on the sequencing chromatograms, the amplicons were cloned into pMD19-T vectors (Takara, Dalian, China) for further sequencing with the universal primers RV-M and M13-47 (Sangon, Beijing, China).
Nucleotide sequence identity and phylogenetic analyses
All the newly generated sequences were searched against the GenBank database using the Basic Local Alignment Search Tool (BLAST). The nucleotide sequence identities between the sequences in this study and reference sequences were determined using the MegAlign program in Lasergene (Burland, 2000). A maximum likelihood (ML) tree used to identify the pathogens by species was reconstructed using PhyML 3.0 (Guindon et al., 2010) based on the optimal nucleotide substitution model (GTR + Γ + I) estimated by MEGA 6.0.6 (Tamura et al., 2013). The reliability of the tree was evaluated by bootstrap analysis with 1,000 replicates.
Statistical analysis
The differences between positive rates of Anaplasma, Bartonella, and Rickettsia infections in Daurian ground squirrels were compared using a χ2 test with a p-value of 0.05 as the threshold.
Results
Rodent sample collection
A total of 165 rodents were captured in Weichang Manchu and Mongolian Autonomous County, Hebei Province, China. Based on both morphological and molecular methods, all of the rodents were identified as Daurian ground squirrels, belonging to the family Sciuridae. The cytb gene sequences shared 99.3–100% nucleotide identity with each other and displayed 98.5–99.7% nucleotide identity with known corresponding sequences from Daurian ground squirrels in the GenBank database.
Molecular detection of pathogens
After electrophoresis, 35 PCR products of expected size were subjected to sequencing, and the results were further subjected to BLAST searches. In total, 34 rodent samples tested positive for at least one causative agent, and one co-infection of two pathogens was found. Furthermore, eight pathogens were identified. Specifically, 13 rodent samples tested positive for the genus Rickettsia, with a positive rate of 7.9%, including 7 R. raoultii, 3 R. sibirica, and 3 Candidatus R. longicornii. Fifteen rodent samples tested positive for the genus Bartonella, with a positive rate of 9.1%, including 10 B. washoensis, 3 B. grahamii, and 2 B. jaculi. Co-infection of B. grahamii and R. raoultii was observed in one sample. Six samples tested positive for Candidatus A. cinensis, with a positive rate of 3.6%. When using the primer pairs fD1/Eh-out2 and fD1/Eh-gs1 targeting the 16S rRNA of the genus Anaplasma, none of the six samples mentioned above tested positive. Instead, an additional sample yielded a positive result. After sequencing, BLASTn analysis based on this partial 16S rRNA sequence indicated that this agent could be identified as A. capra, with a low positive rate of 0.6%. Unfortunately, we failed to get other genes. Rickettsia and Bartonella infections in Daurian ground squirrels had no significant difference (χ2 = 0.16, p = 0.69). The positive rate of Bartonella infection in Daurian ground squirrels was higher than that of Anaplasma (χ2 = 4.12, p = 0.04). However, no significant difference was observed between Rickettsia and Anaplasma infections (χ2 = 2.74, p = 0.10).
Molecular characterization of identified vector-borne intracellular bacterial pathogens
For the genus Bartonella, 10 partial gltA gene sequences of B. washoensis in this study shared 99.3–100% nucleotide identity with each other and the highest nucleotide identity of 99.7–100% with Bartonella sp. DR 1–1, also in Daurian ground squirrels from China (Inoue et al., 2009). Specifically, the gltA gene sequences recovered in this study shared 97.1–97.9% nucleotide identity with those of strain 085-0475 isolated from humans. In addition, the gltA gene sequences in this study shared 97.0–99.7% nucleotide identity with known ones of B. washoensis from ground squirrels and 96.4–98.8% nucleotide identity with known B. washoensis variants from other reservoirs. In the phylogenetic tree, all B. washoensis variants in this study clustered with the reference B. washoensis strain 085-0475, and then with B. washoensis isolate human_1487_18, also from humans (Figure 2). However, these newly generated sequences only shared 91.1–92.0% nucleotide identity with the gltA gene sequence of isolate human_1487_18. In addition, another three partial gltA gene sequences of B. grahamii in this study shared 99.1–99.8% nucleotide identity with each other, and 96.6–98.9% with known ones of B. grahamii. In the phylogenetic tree, all three newly generated gltA gene sequences clustered with those of B. grahamii (Figure 2). Two partial gltA gene sequences of B. jaculi recovered in this study shared 95.7% nucleotide identity with each other and 96.3–99.7% nucleotide identity with known ones of B. jaculi. In the phylogenetic tree, these two sequences clustered with that of a known B. jaculi isolate (Figure 2).
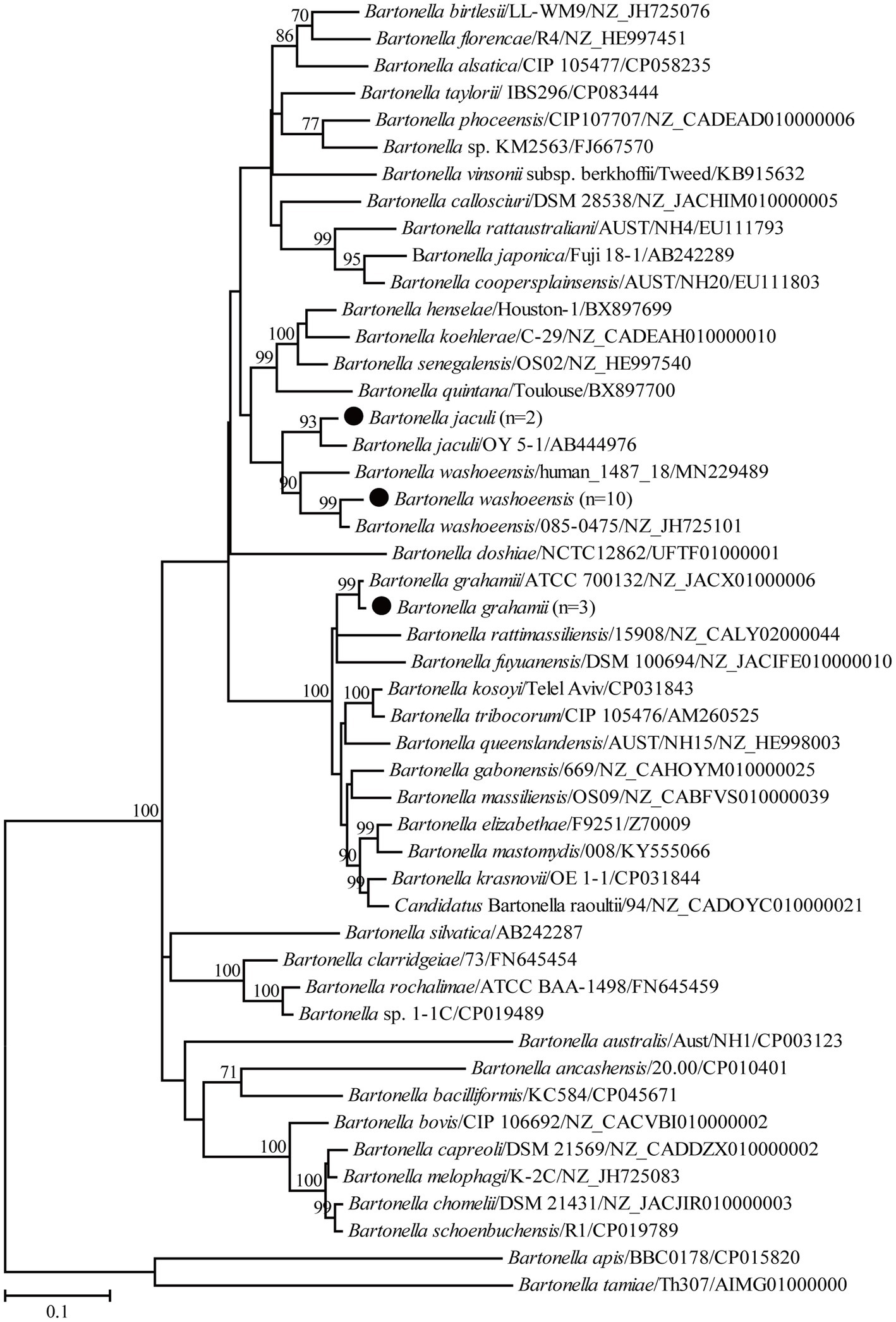
Figure 2. Phylogenetic tree based on the partial gltA gene sequences of the genus Bartonella. Numbers at each node indicate bootstrap values. The tree was mid-point rooted for clarity, and the scale bar represents the number of nucleotide substitutions per site. The representative sequence obtained in this study was used to reconstruct the tree and is marked with circles.
The partial 16S rRNA gene sequence in this study presented a 100% similarity with some sequences of A. capra variants, suggesting a possible A. capra infection in Daurian ground squirrels. Using the primers designed in this study, Candidatus A. cinensis was detected by amplifying its gltA gene in six samples. All six of these partial gltA gene sequences in this study shared 99.0–99.7% nucleotide identity and shared 98.7–99.7% nucleotide identity with known ones of Candidatus A. cinensis. In addition, a partial groEL gene sequence was obtained and showed the highest nucleotide identity of 98.4% with that of known Candidatus A. cinensis variants. Moreover, the groEL and gltA gene sequences herein shared 82.8% and 74.0–74.7% nucleotide identity, respectively, with those of the A. platys reference strain S3. Consistently, these variants in the present study clustered with other known Candidatus A. cinensis rather than specific A. platys. Specifically, the Candidatus A. cinensis variants herein clustered within lineages 2 and 4 of Guo et al. (2019) for the gltA and groEL genes, respectively (Figure 3).
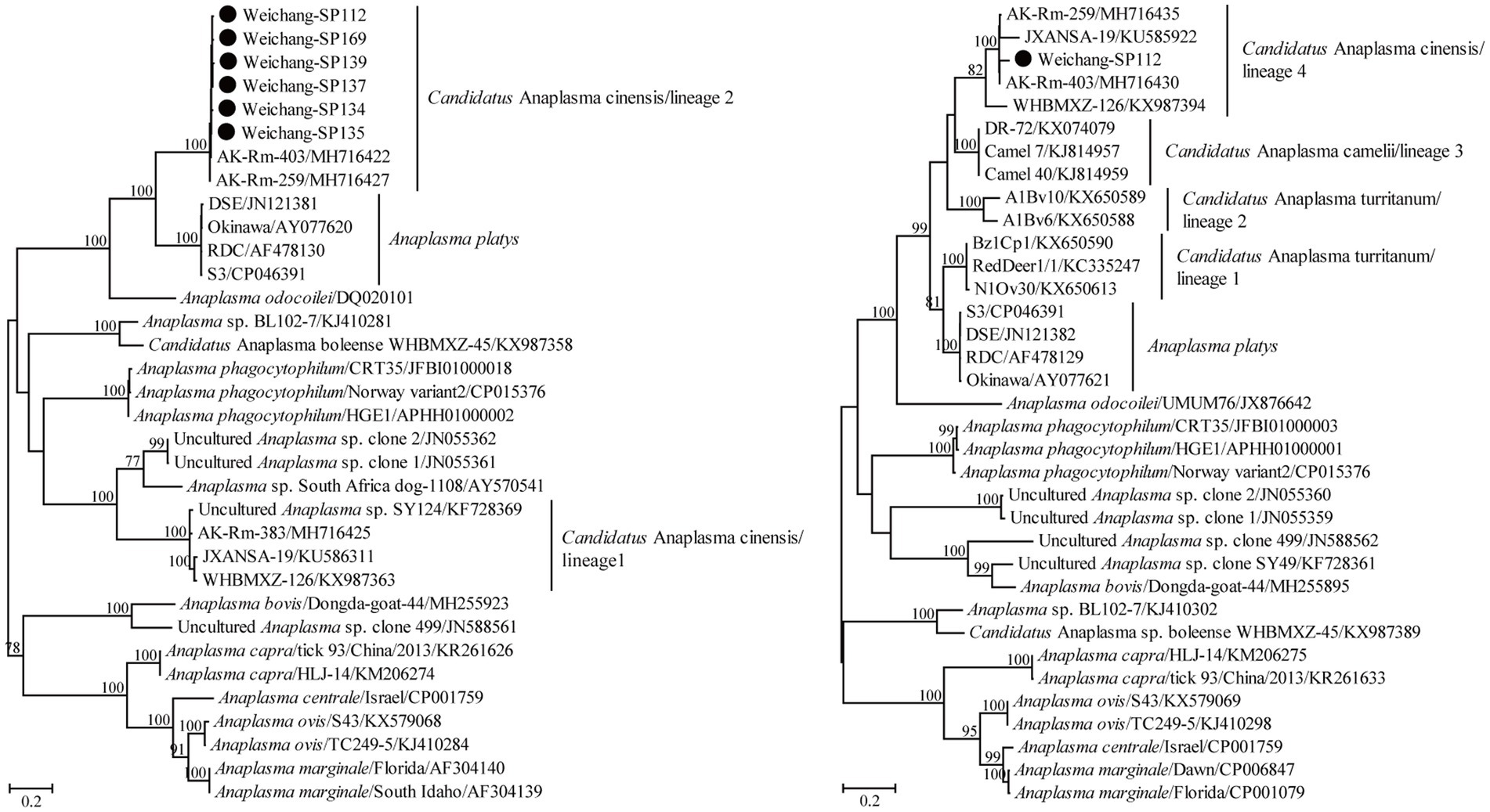
Figure 3. Phylogenetic tree based on the partial gltA (left) and groEL (right) gene sequences of the genus Anaplasma. Numbers at each node indicate bootstrap values. The tree was mid-point rooted for clarity, and the scale bar represents the number of nucleotide substitutions per site. The representative sequence obtained in this study was used to reconstruct the tree and is marked with circles.
For the genus Rickettsia, seven partial ompA gene sequences of R. raoultii in this study shared 99.2–100% nucleotide identity with each other and 97.7–99.4% with known R. raoultii variants. In addition, three partial ompA gene sequences of R. sibirica in this study shared 98.1–100% nucleotide identity with the known ones of R. sibirica variants. Another three partial ompA gene sequences of Candidatus R. longicornii in this study shared 98.8–100% nucleotide identity with each other and 96.6–100% nucleotide identity with known Candidatus R. longicornii variants. Interestingly, the ompA gene sequence of isolate Weichang-Sd-87 had an insertion of three bases (GAC) compared to the other four isolates, the same as isolates CCBF8, GXS12, and GXS34 identified from rodents in Guangxi, China. In the phylogenetic tree, 19 ompA gene sequences were classified into three groups, corresponding to R. raoultii, R. sibirica, and Candidatus R. longicornii (Figure 4).
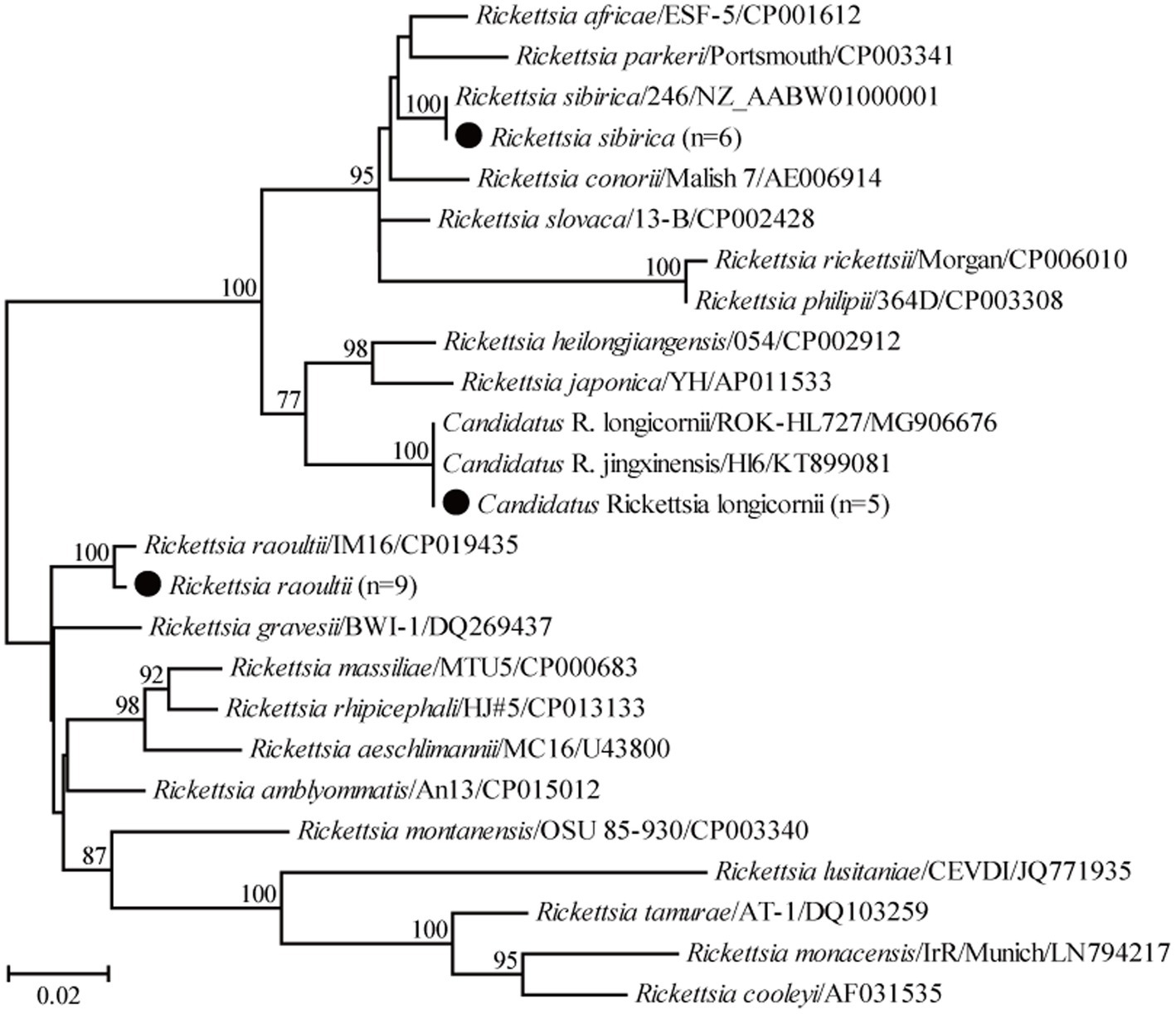
Figure 4. Phylogenetic tree based on the partial ompA gene sequences of the genus Rickettsia. Numbers at each node indicate bootstrap values. The tree was mid-point rooted for clarity, and the scale bar represents the number of nucleotide substitutions per site. The representative sequence obtained in this study was used to reconstruct the tree and is marked with circles.
Discussion
Rodents are the main hosts of many human pathogens and can indirectly transmit vector-borne intracellular bacteria to humans, such as Rickettsiales and Bartonella (Han et al., 2015). The Daurian ground squirrel is a wild rodent species mainly distributed in northern China, Mongolia, and Russia and is a host of Yersinia pestis (Zhou et al., 2004a,b; Tian, 2018). Daurian ground squirrels frequently come into contact with herders, which can increase the risk of plague transmission in humans. To date, the diversity of vector-borne intracellular bacterial pathogens carried by Daurian ground squirrels has been unclear, although the ground squirrels are known to be hosts of ticks. In this study, eight intracellular bacterial species were discovered, namely two Anaplasma species, three Rickettsia species, and three Bartonella species. Specifically, these eight species were A. capra, Candidatus A. cinensis, R. raoultii, R. sibirica, Candidatus R. longicornii, B. washoensis, B. grahamii, and B. jaculi. Importantly, A. capra, R. raoultii, R. sibirica, Candidatus R. longicornii, B. washoensis, and B. grahamii are pathogenic to humans, suggesting potential threats to public health. In this study, a low rate of co-infection between B. grahamii and R. raoultii was observed. In a previous study, co-infection of Rickettsia and Bartonella was found to be prevalent in rodents in Vietnam (Anh et al., 2021), although the bacterial species within Rickettsia and Bartonella were not identified. All these results imply that the co-infection of Rickettsia and Bartonella in rodents does not interfere with each other. Our results give important insights into the distribution of these vector-borne pathogens and the genetic diversity of vector-borne pathogens in Daurian ground squirrels in the local area.
To date, Rickettsia has been found in many rodent species, even in single rodent species collected from a single location (Schex et al., 2011; Kuo et al., 2015; Lu et al., 2019). In previous studies, R. heilongjiangensis, R. japonica, and an unidentified Rickettsia species were detected in Apodemus agrarius in China (Lu et al., 2019); R. felis and R. helvetica were found in A. flavicollis in Germany (Schex et al., 2011); and seven Rickettsia species were identified in Rattus losea in Taiwan (Kuo et al., 2015). Similar to these results, three Rickettsia species, namely R. raoultii, R. sibirica, and Candidatus R. longicornii, were identified in Daurian ground squirrels in the present study, indicating that there is genetic diversity in Rickettsia hosted by a rodent species in a single location. In our previous studies, two uncultured Rickettsia species, Candidatus R. tarasevichiae, and Candidatus R. principis, were detected in ticks from the same county (Xue et al., 2023). However, neither was recovered in this study, although Candidatus R. tarasevichiae was detected in R. norvegicus and Clethrionomys rufocanus in Dashigou around Mudanjiang City, Heilongjiang Province, Northeast China (Yuan et al., 2021). This may be due to differences in sampling sites or because the Daurian ground squirrel is not the primary host. Our previous study also showed that at least three tick species, Ixodes persulcatus, Dermacentor silvarum, and Haemaphysalis concinna, were present in local areas, and R. raoultii has been identified in those ticks (Xue et al., 2023). However, R. sibirica and Candidatus R. longicornii were not identified, although R. sibirica has been detected in the same tick species from other parts of China (Zhao et al., 2021). Hence, ticks should be collected to determine their circulation and prevent further threats to human health.
As the main hosts, rodents are infected by many species within the genus Bartonella. Among the rodent-associated Bartonella species, eight detected in Daurian ground squirrels, including B. rochalimae and B. washoensis, are pathogenic to humans (Jian et al., 2022). In addition to B. washoensis, which was identified in Daurian ground squirrels in a previous study (Inoue et al., 2009; Li et al., 2023b), B. grahamii and B. jaculi were detected in Daurian ground squirrels in the present study. Combining the data from previous and our studies, four Bartonella species have been recovered in Daurian ground squirrels, indicating that this rodent species is an important host of Bartonella. Bartonella jaculi was first identified in Jaculus orientalis from Egypt (Sato et al., 2013) and then only detected in Allactaga sibirica from the Qaidam Basin of China (Rao et al., 2021). These results demonstrated the worldwide geographical distribution of B. jaculi, and it has a wide range of rodent hosts. In this study, B. washoensis was the predominant Bartonella species, followed by B. grahamii. Considering their pathogenicity to humans, the ectoparasites (including fleas, mites, lice, and ticks) of Daurian ground squirrels should be collected to determine those that can act as potential vectors of both B. washoensis and B. grahamii in future studies.
Anaplasma capra was first confirmed to be a human pathogen in 2015 (Li et al., 2015) and has been mainly identified in livestock based on sequences deposited in GenBank and previous studies (Guo et al., 2018; Amer et al., 2019; He et al., 2021; Remesar et al., 2022; Sahin et al., 2022). In this study, only one partial 16S rRNA sequence was obtained, and we conjectured that this may have resulted from a low bacterial load or DNA degradation by some enzymes in the host. Alternatively, rodents may not be a competent reservoir of A. capra. Anaplasma platys is considered a potential human pathogen because its DNA was detected in two female patients from Venezuela (Arraga-Alvarado et al., 2014). Unlike other Anaplasma species, A. platys showed a high level of great genetic diversity, manifested by the 16S rRNA gene forming a monophyletic group and the gltA and groEL genes forming polyphyletic groups (Ben Said et al., 2017; Guo et al., 2019; Nguyen et al., 2020; Zobba et al., 2022). In China, A. platys-like variants formed a lineage in the groEL tree, while they were classified into two lineages in the gltA tree (Guo et al., 2019). In Thailand, similar A. platys-like variants were also identified, and they clustered together with the above-mentioned variants in China in the groEL tree (Nguyen et al., 2020). At present, this lineage is named Candidatus A. cinensis. In addition, another two genetically related lineages of A. platys-like in ruminants from Tunisia were named Candidatus A. turritanum (Ben Said et al., 2017). Moreover, Candidatus A. camelii fell into the genetic diversity of A. platys and A. platys-like (Guo et al., 2019). In this study, Candidatus A. cinensis was identified in Daurian ground squirrels, and this is the first molecular evidence of its infection in rodents. In detail, the gltA gene sequences presented the closest genetic relationship with known sequences belonging to lineage 2 rather than those of lineage 1 from Guo et al. (2019), while the groEL gene sequences clustered together and formed one lineage. The data from this and previous studies suggest that Candidatus A. cinensis is widely distributed and infects a greater variety of potential hosts than suggested by previous studies (Ben Said et al., 2017; Guo et al., 2019; Nguyen et al., 2020). Therefore, its pathogenicity should be given more attention.
There were several limitations to this study. First, we did not check for the presence of blood-sucking vectors on the bodies of the ground squirrels in this study. Therefore, the real risks of the pathogens identified in this study to the local population and the association between the presence of vectors and infected Daurian ground squirrels could not be determined. As such, human cases should be identified in future studies to reveal the risks to the local population. Second, we did not record the age or gender of the captured rodents or the rodent numbers in each month because the aim of this study was to better understand the genetic diversity of vector-borne Anaplasma, Rickettsia, and Bartonella. Therefore, the assessment of the prevalence of each pathogen over time or across age groups or sexes of Daurian ground squirrels could not be determined. Moreover, rodent samples were collected from one sampling site for this study; therefore, potential spatial differences could not be determined, resulting in an incomplete picture of the intracellular bacterial pathogens in Daurian ground squirrels.
In summary, eight vector-borne bacterial species, including six that are pathogenic to humans, were molecularly identified in Daurian ground squirrels. Our results contributed to the characterization of the genetic diversity of bacterial species associated with Daurian ground squirrels, such as Rickettsia, Anaplasma, and Bartonella. Our results also provided evidence that Daurian ground squirrels were potential bacterial hosts. In addition, the results suggested that the pathogens could pose threats to public health in the local population. Considering that there could be an indirect transmission of the above-mentioned bacteria from Daurian ground squirrels to humans, potential vectors should be identified in future studies.
Data availability statement
The datasets presented in this study can be found in online repositories. The nucleotide sequences recovered in this study were deposited in GenBank (https://www.ncbi.nlm.nih.gov/genbank) under the accession numbers OR976065 and OR988094–OR988128.
Ethics statement
The animal study was approved by the ethical committee of Chengde Medical University. The study was conducted in accordance with the local legislation and institutional requirements.
Author contributions
JX: Writing – original draft, Data curation, Formal analysis, Investigation. S-SC: Data curation, Investigation, Methodology, Writing – review & editing. Z-YX: Investigation, Resources, Writing – review & editing. F-NW: Investigation, Writing – review & editing. JW: Funding acquisition, Resources, Writing – review & editing. DD: Investigation, Resources, Writing – review & editing. LD: Supervision, Validation, Writing – review & editing. G-CX: Writing – review & editing. W-PG: Conceptualization, Funding acquisition, Methodology, Project administration, Supervision, Writing – original draft, Writing – review & editing.
Funding
The author(s) declare that financial support was received for the research, authorship, and/or publication of this article. This study was supported by the Hebei Natural Science Foundation (No. C2022406003), the Young Talent Program of Higher School in Hebei Province (No. BJ2020024), the Scientific Research Foundation for High-level Talents of Chengde Medical University (No. 202001), and the Hebei Medical Science Research Project (No. 20210234).
Conflict of interest
The authors declare that the research was conducted in the absence of any commercial or financial relationships that could be construed as a potential conflict of interest.
Publisher’s note
All claims expressed in this article are solely those of the authors and do not necessarily represent those of their affiliated organizations, or those of the publisher, the editors and the reviewers. Any product that may be evaluated in this article, or claim that may be made by its manufacturer, is not guaranteed or endorsed by the publisher.
Supplementary material
The Supplementary material for this article can be found online at: https://www.frontiersin.org/articles/10.3389/fmicb.2024.1359797/full#supplementary-material
References
Amer, S., Kim, S., Yun, Y., and Na, K. J. (2019). Novel variants of the newly emerged Anaplasma capra from Korean water deer (Hydropotes inermis argyropus) in South Korea. Parasit. Vectors 12:365. doi: 10.1186/s13071-019-3622-5
Anh, L. T. L., Balakirev, A. E., and Chau, N. V. (2021). Investigation of multiple infections with zoonotic pathogens of rodents in northern Vietnam. J. Vector Borne Dis. 58, 47–53. doi: 10.4103/0972-9062.321750
Arraga-Alvarado, C. M., Qurollo, B. A., Parra, O. C., Berrueta, M. A., Hegarty, B. C., and Breitschwerdt, E. B. (2014). Case report: molecular evidence of Anaplasma platys infection in two women from Venezuela. Am J Trop Med Hyg 91, 1161–1165. doi: 10.4269/ajtmh.14-0372
Battilani, M., De Arcangeli, S., Balboni, A., and Dondi, F. (2017). Genetic diversity and molecular epidemiology of Anaplasma. Infect. Genet. Evol. 49, 195–211. doi: 10.1016/j.meegid.2017.01.021
Ben Said, M., Belkahia, H., El Mabrouk, N., Saidani, M., Alberti, A., Zobba, R., et al. (2017). Anaplasma platys-like strains in ruminants from Tunisia. Infect. Genet. Evol. 49, 226–233. doi: 10.1016/j.meegid.2017.01.023
Burland, T. G. (2000). DNASTAR's Lasergene sequence analysis software. Methods Mol. Biol. 132, 71–91. doi: 10.1385/1-59259-192-2:71
Chen, Y. M., Hu, S. J., Lin, X. D., Tian, J. H., Lv, J. X., Wang, M. R., et al. (2023). Host traits shape virome composition and virus transmission in wild small mammals. Cell 186, 4662–4675.e12. doi: 10.1016/j.cell.2023.08.029
Darby, A. C., Cho, N. H., Fuxelius, H. H., Westberg, J., and Andersson, S. G. (2007). Intracellular pathogens go extreme: genome evolution in the Rickettsiales. Trends Genet. 23, 511–520. doi: 10.1016/j.tig.2007.08.002
Deng, H., Le Rhun, D., Buffet, J. P., Cotté, V., Read, A., Birtles, R. J., et al. (2012). Strategies of exploitation of mammalian reservoirs by Bartonella species. Vet. Res. 43:15. doi: 10.1186/1297-9716-43-15
Guindon, S., Dufayard, J. F., Lefort, V., Anisimova, M., Hordijk, W., and Gascuel, O. (2010). New algorithms and methods to estimate maximum-likelihood phylogenies: assessing the performance of PhyML 3.0. Syst. Biol. 59, 307–321. doi: 10.1093/sysbio/syq010
Guo, W. P., Huang, B., Zhao, Q., Xu, G., Liu, B., Wang, Y. H., et al. (2018). Human-pathogenic Anaplasma spp., and Rickettsia spp. in animals in Xi'an, China. PLoS Negl. Trop. Dis. 12:e0006916. doi: 10.1371/journal.pntd.0006916
Guo, W. P., Lin, X. D., Wang, W., Tian, J. H., Cong, M. L., Zhang, H. L., et al. (2013). Phylogeny and origins of hantaviruses harbored by bats, insectivores, and rodents. PLoS Pathog. 9:e1003159. doi: 10.1371/journal.ppat.1003159
Guo, W. P., Tie, W. F., Meng, S., Li, D., Wang, J. L., Du, L. Y., et al. (2020). Extensive genetic diversity of Anaplasma bovis in ruminants in Xi'an. China. Ticks Tick Borne Dis 11:101477. doi: 10.1016/j.ttbdis.2020.101477
Guo, W. P., Zhang, B., Wang, Y. H., Xu, G., Wang, X., Ni, X., et al. (2019). Molecular identification and characterization of Anaplasma capra and Anaplasma platys-like in Rhipicephalus microplus in Ankang. Northwest China. BMC Infect Dis 19:434. doi: 10.1186/s12879-019-4075-3
Han, B. A., Schmidt, J. P., Bowden, S. E., and Drake, J. M. (2015). Rodent reservoirs of future zoonotic diseases. Proc. Natl. Acad. Sci. USA 112, 7039–7044. doi: 10.1073/pnas.1501598112
He, Y., Chen, W., Ma, P., Wei, Y., Li, R., Chen, Z., et al. (2021). Molecular detection of Anaplasma spp., Babesia spp. and Theileria spp. in yaks (Bos grunniens) and Tibetan sheep (Ovis aries) on the Qinghai-Tibetan plateau, China. Parasit. Vectors 14:613. doi: 10.1186/s13071-021-05109-2
Huchon, D., Madsen, O., Sibbald, M. J., Ament, K., Stanhope, M. J., Catzeflis, F., et al. (2002). Rodent phylogeny and a timescale for the evolution of Glires: evidence from an extensive taxon sampling using three nuclear genes. Mol. Biol. Evol. 19, 1053–1065. doi: 10.1093/oxfordjournals.molbev.a004164
Inoue, K., Maruyama, S., Kabeya, H., Hagiya, K., Izumi, Y., Une, Y., et al. (2009). Exotic small mammals as potential reservoirs of zoonotic Bartonella spp. Emerg. Infect. Dis. 15, 526–532. doi: 10.3201/eid1504.081223
Ishikura, M., Ando, S., Shinagawa, Y., Matsuura, K., Hasegawa, S., Nakayama, T., et al. (2003). Phylogenetic analysis of spotted fever group rickettsiae based on gltA, 17-kDa, and rOmpA genes amplified by nested PCR from ticks in Japan. Microbiol. Immunol. 47, 823–832. doi: 10.1111/j.1348-0421.2003.tb03448.x
Jian, R., Ren, Q., Xue, J., Xie, G. C., Wang, J., Chen, G. Q., et al. (2022). Genetic diversity of Bartonella infection in residential and field rodents in Hebei. China. Front Microbiol 13:1039665. doi: 10.3389/fmicb.2022.1039665
Kuo, C. C., Shu, P. Y., Mu, J. J., and Wang, H. C. (2015). High prevalence of Rickettsia spp. infections in small mammals in Taiwan. Vector Borne Zoonotic Dis. 15, 13–20. doi: 10.1089/vbz.2014.1584
Krügel, M., Król, N., Kempf, V. A. J., Pfeffer, M., and Obiegala, A. (2022). Emerging rodent-associated Bartonella: A threat for human health? Parasit. Vectors 15:113. doi: 10.1186/s13071-022-05162-5
Li, J., Zhang, C., Lu, M., Wang, Y., Liu, F., Wang, W., et al. (2023a). Infection by a previously uncharacterized Ehrlichia species in rodents from Inner Mongolia. Northern China. Ticks Tick Borne Dis 14:102116. doi: 10.1016/j.ttbdis.2022.102116
Li, J., Zhang, C., Lu, M., Wang, Y., Wang, W., Liu, F., et al. (2023b). The diverse genetic genotypes of Bartonella species circulating in rodents from Inner Mongolia. Northern China. PLoS Negl Trop Dis 17:e0011462. doi: 10.1371/journal.pntd.0011462
Li, H., Zheng, Y. C., Ma, L., Jia, N., Jiang, B. G., Jiang, R. R., et al. (2015). Human infection with a novel tick-borne Anaplasma species in China: a surveillance study. Lancet Infect. Dis. 15, 663–670. doi: 10.1016/S1473-3099(15)70051-4
Lu, M., Li, F., Liao, Y., Shen, J. J., Xu, J. M., Chen, Y. Z., et al. (2019). Epidemiology and diversity of Rickettsiales Bacteria in humans and animals in Jiangsu and Jiangxi provinces. China. Sci Rep 9:13176. doi: 10.1038/s41598-019-49059-3
Meerburg, B. G., Singleton, G. R., and Kijlstra, A. (2009). Rodent-borne diseases and their risks for public health. Crit. Rev. Microbiol. 35, 221–270. doi: 10.1080/10408410902989837
Nguyen, A. H. L., Tiawsirisup, S., and Kaewthamasorn, M. (2020). Molecular detection and genetic characterization of Anaplasma marginale and Anaplasma platys-like (Rickettsiales: Anaplasmataceae) in water buffalo from eight provinces of Thailand. BMC Vet. Res. 16:380. doi: 10.1186/s12917-020-02585-z
Rao, H., Li, S., Lu, L., Wang, R., Song, X., Sun, K., et al. (2021). Genetic diversity of Bartonella species in small mammals in the Qaidam Basin, western China. Sci. Rep. 11:1735. doi: 10.1038/s41598-021-81508-w
Remesar, S., Prieto, A., García-Dios, D., López-Lorenzo, G., Martínez-Calabuig, N., Díaz-Cao, J. M., et al. (2022). Diversity of Anaplasma species and importance of mixed infections in roe deer from Spain. Transbound. Emerg. Dis. 69, e374–e385. doi: 10.1111/tbed.14319
Sahin, O. F., Erol, U., and Altay, K. (2022). Buffaloes as new hosts for Anaplasma capra: molecular prevalence and phylogeny based on gtlA, groEL, and 16S rRNA genes. Res. Vet. Sci. 152, 458–464. doi: 10.1016/j.rvsc.2022.09.008
Sato, S., Kabeya, H., Fujinaga, Y., Inoue, K., Une, Y., Yoshikawa, Y., et al. (2013). Bartonella jaculi sp. nov., Bartonella callosciuri sp. nov., Bartonella pachyuromydis sp. nov. and Bartonella acomydis sp. nov., isolated from wild Rodentia. Int. J. Syst. Evol. Microbiol. 63, 1734–1740. doi: 10.1099/ijs.0.041939-0
Schex, S., Dobler, G., Riehm, J., Müller, J., and Essbauer, S. (2011). Rickettsia spp. in wild small mammals in lower Bavaria, south-eastern Germany. Vector Borne Zoonotic Dis. 11, 493–502. doi: 10.1089/vbz.2010.0060
Tamura, K., Stecher, G., Peterson, D., Filipski, A., and Kumar, S. (2013). MEGA6: molecular evolutionary genetics analysis version 6.0. Mol. Biol. Evol. 30, 2725–2729. doi: 10.1093/molbev/mst197
Telford, S. R., and Wormser, G. P. (2010). Bartonella spp. transmission by ticks not established. Emerg. Infect. Dis. 16, 379–384. doi: 10.3201/eid1603.090443
Tian, L. (2018). Relationship between environmental factors and the spatial distribution of Spermophilus dauricus during 2000-2015 in China. Int. J. Biometeorol. 62, 1781–1789. doi: 10.1007/s00484-018-1580-9
Wang, Y.X. (2003) A complete checklist of mammal species and subspecies in China - a taxonomic and geographic reference. Beijing: China Forestry Publishing House
Weisburg, W. G., Barns, S. M., Pelletier, D. A., and Lane, D. J. (1991). 16S ribosomal DNA amplification for phylogenetic study. J. Bacteriol. 173, 697–703. doi: 10.1128/jb.173.2.697-703.1991
Wen, B., Jian, R., Zhang, Y., and Chen, R. (2002). Simultaneous detection of Anaplasma marginale and a new Ehrlichia species closely related to Ehrlichia chaffeensis by sequence analyses of 16S ribosomal DNA in Boophilus microplus ticks from Tibet. J. Clin. Microbiol. 40, 3286–3290. doi: 10.1128/JCM.40.9.3286-3290.2002
Wilson, D.E., and Reeder, D.M. (2005) Mammal species of the world. A taxonomic and geographic reference. 3rd ed. Johns Hopkins University Press; Baltimore, Maryland
Xue, J., Ren, Q., Yang, X. L., Wang, J., Xie, G., Du, L., et al. (2023). Human pathogens in ticks removed from humans in Hebei. China. Heliyon 9:e13859. doi: 10.1016/j.heliyon.2023.e13859
Yuan, T. T., Ma, L., Jiang, B. G., Fu, W. M., Sun, Y., Jia, N., et al. (2021). First confirmed infection of Candidatus Rickettsia Tarasevichiae in rodents collected from northeastern China. Vector Borne Zoonotic Dis. 20, 88–92. doi: 10.1089/vbz.2019.2443
Zhao, S. S., Pulati, Y., Yin, X. P., Li, W., Wang, B. J., Yang, K., et al. (2017). Wildlife plague surveillance near the China-Kazakhstan border: 2012–2015. Transbound. Emerg. Dis. 64, e48–e51. doi: 10.1111/tbed.12603
Zhao, G. P., Wang, Y. X., Fan, Z. W., Ji, Y., Liu, M. J., Zhang, W. H., et al. (2021). Mapping ticks and tick-borne pathogens in China. Nat. Commun. 12:1075. doi: 10.1038/s41467-021-21375-1
Zhou, D., Han, Y., Song, Y., Huang, P., and Yang, R. (2004b). Comparative and evolutionary genomics of Yersinia pestis. Microbes Infect. 6, 1226–1234. doi: 10.1016/j.micinf.2004.08.002
Zhou, D., Han, Y., Song, Y., Tong, Z., Wang, J., Guo, Z., et al. (2004a). DNA microarray analysis of genome dynamics in Yersinia pestis: insights into bacterial genome microevolution and niche adaptation. J. Bacteriol. 186, 5138–5146. doi: 10.1128/JB.186.15.5138-5146.2004
Keywords: Daurian ground squirrel, Anaplasma, Bartonella, Rickettsia, genetic diversity
Citation: Xue J, Chen S-S, Xu Z-Y, Wang F-N, Wang J, Diao D, Du L, Xie G-C and Guo W-P (2024) Anaplasma, Bartonella, and Rickettsia infections in Daurian ground squirrels (Spermophilus dauricus), Hebei, China. Front. Microbiol. 15:1359797. doi: 10.3389/fmicb.2024.1359797
Edited by:
Kevin S. W. Tan, National University of Singapore, SingaporeReviewed by:
Clifton McKee, Johns Hopkins University, United StatesMiriam Maas, National Institute for Public Health and the Environment (Netherlands), Netherlands
Copyright © 2024 Xue, Chen, Xu, Wang, Wang, Diao, Du, Xie and Guo. This is an open-access article distributed under the terms of the Creative Commons Attribution License (CC BY). The use, distribution or reproduction in other forums is permitted, provided the original author(s) and the copyright owner(s) are credited and that the original publication in this journal is cited, in accordance with accepted academic practice. No use, distribution or reproduction is permitted which does not comply with these terms.
*Correspondence: Wen-Ping Guo, Z3Vvd2VucGluZ0Bud3N1YWYuZWR1LmNu