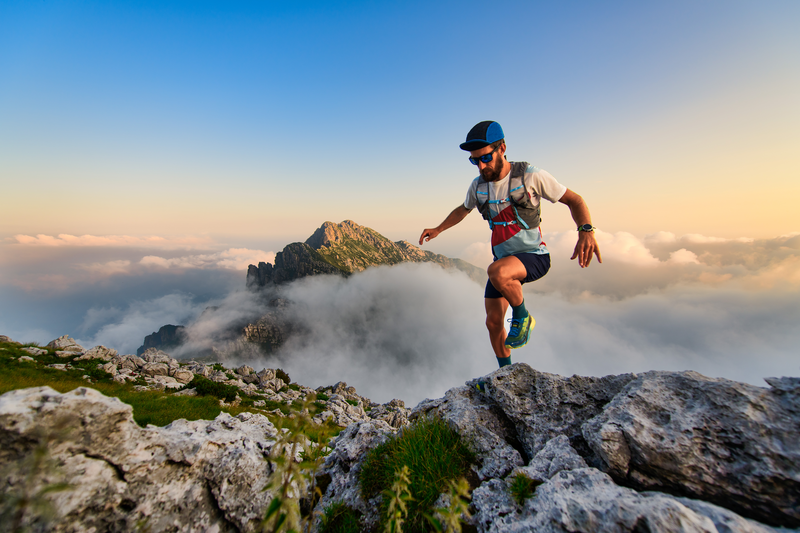
95% of researchers rate our articles as excellent or good
Learn more about the work of our research integrity team to safeguard the quality of each article we publish.
Find out more
ORIGINAL RESEARCH article
Front. Microbiol. , 19 February 2024
Sec. Microorganisms in Vertebrate Digestive Systems
Volume 15 - 2024 | https://doi.org/10.3389/fmicb.2024.1356462
This article is part of the Research Topic Breastfeeding's Role in Shaping Infant Gut Microbiota for Long-Term Health View all 6 articles
Introduction: The short-chain fatty acids (SCFAs) contained in breast milk play a key role in infant growth, affecting metabolism and enhancing intestinal immunity by regulating inflammation.
Methods: In order to examine the associations between the microbiota and SCFA levels in breast milk, and explore the roles of SCFAs in regulating the infant gut microbiota, we enrolled 50 paired mothers and infants and collected both breast milk and infant fecal samples. Breast milk SCFA contents were determined by UPLC-MS, and whole genome shotgun sequencing was applied to determine the microbial composition of breast milk and infant feces. The SCFA levels in breast milk were grouped into tertiles as high, medium, or low, and the differences of intestinal microbiota and KEGG pathways were compared among groups.
Results: The results demonstrated that breast milk butyric acid (C4) is significantly associated with Clostridium leptum richness in breastmilk. Additionally, the specific Bifidobacterium may have an interactive symbiosis with the main species of C4-producing bacteria in human milk. Women with a low breast milk C4 tertile are associated with a high abundance of Salmonella and Salmonella enterica in their infants' feces. KEGG pathway analysis further showed that the content of C4 in breast milk is significantly correlated with the infants' metabolic pathways of lysine and arginine biosynthesis.
Discussion: This study suggests that interactive symbiosis of the microbiota exists in breast milk. Certain breast milk microbes could be beneficial by producing C4 and further influence the abundance of certain gut microbes in infants, playing an important role in early immune and metabolic development.
Breast milk is rich in nutrients and is considered as the most ideal natural food for infants aged 0–6 months (Gates et al., 2023; Szugye et al., 2023). Not only it can ensure infants' growth; certain breast milk components also play essential roles in regulating intestinal microbiota colonization and metabolism; serve as anti-inflammatory and anti-infectious agents; and perform many other functions (Fougère et al., 2023; Ouyang et al., 2023; Ozen et al., 2023).
Fat is the second most abundant solid nutrient in breast milk and can provide around 50% of the energy needs of newborns (George et al., 2021). In addition to its richness in medium- and long-chain fatty acid content, breast milk also contains small amounts of free short-chain fatty acids (SCFAs, including acetic acid C2, propionic acid C3, butyric acid C4, isobutyric acid iC4, 2-Methylbutyric acid mC4, valeric acid C5, and isovaleric acid iC5) (Stinson et al., 2020; Stinson and Geddes, 2022). The level of SCFAs in breast milk varies greatly among individuals. Different studies have found that the total content of SCFAs in breast milk ranges from 13 to 4,300 μmol/L (Prentice et al., 2019; Stinson et al., 2020). However, the source of breast milk SCFAs is still not clear. Some studies indicate that breast milk SCFAs may be produced by the maternal gut microbiota and distributed to the breast through circulation; however, it is also suggested that they could be produced in breast milk by its own microbiota (Stinson et al., 2020; Stinson and Geddes, 2022). Currently, increasing evidence demonstrates that SCFAs play key roles in infant growth and development (Nogal et al., 2023). In vitro and in vivo studies have found that the breast milk SCFAs could help to decrease inflammation, increase immunity, and reduce intestinal inflammation (Gao et al., 2021; Xu et al., 2023). In addition, breast milk SCFAs could affect in infant metabolism by regulating inflammation. One study showed that breast milk SCFAs, especially C4, can directly or indirectly affect fat formation and fat cell metabolism through anti-inflammatory mechanisms in infants (Prentice et al., 2019). Furthermore, C4 is reported primarily associated with the growth of intestinal epithelial cells and could benefit for the development of immunity (Meijer et al., 2010; Brahe et al., 2013). However, the previous studies mainly conducted in vitro cell tests and animal experiments, and the number of population-based study is limited. One population-based cohort study revealed that breast milk SCFAs, including C2, C3 and C4, as the essential energy sources could protect essential fatty acids from oxidation, which could regulate infant growth parameters and bring a protective effect against overweight in early life (Prentice et al., 2019). This finding suggested specific breast milk SCFAs may have certain functions in early life. However, the research on specific breast milk SCFAs and their impact on infants is quite limited, with the exploration of potential regulatory mechanisms being rarely undertaken.
It is well known that certain microbials can produce SCFAs in the gut (Shi et al., 2023). Likewise, it has been suggested that one of the functions of SCFAs is to regulate the gut microbiome (Gotoh and Shibata, 2023). A previous study found that SCFAs, in particular C4, can inhibit the growth of Escherichia coli or Salmonella in vitro. By nourishing intestinal cells and inhibiting the spread of Salmonella in the intestine, butyrate could significantly enhance colon epithelial barrier function (Homann et al., 2023). However, to our knowledge, there are no data reported on the associations between breast milk SCFAs and the infant microbiota. Therefore, whether breast milk SCFAs could affect infant health in the same way as those produced by gut microbes should be examined, and whether the health functions of SCFAs rely on its regulating roles in the infant gut microbiota is worth exploring.
Using paired mother-infant samples, this pilot study aims to (1) examine the associations between the microbiota and SCFA levels in breast milk, and (2) explore the roles of breast milk SCFAs in regulating the infant gut microbiota and its related metabolic functions.
A total of 50 paired mothers and their infants were recruited from three Chinese cities (Xuchang, Cangzhou, and Chenzhou), and breast milk and infant fecal samples were collected. The current study design included mothers who were at 1–3 months postpartum with (1) a healthy singleton birth, (2) a full-term delivery (37–42 weeks), and (3) exclusive breastfeeding. Their paired infants should have (1) no disability or disease diagnosed at birth and (2) an Apgar score ≥8. Mothers with gastric and intestinal illnesses and nipple or lacteal gland diseases were excluded. Infants who had received any prebiotics, probiotics, or antibiotics in the last 4 weeks prior to sample collection were excluded from the current analysis. All participants provided written informed consent. The protocol was approved by the Research Center for Public Health, of Tsinghua University (No. THUSM/PHREC/2021-003).
The study was conducted by trained health professionals using interview questionnaires to collect relevant information about the subjects, including sociodemographic characteristics, lifestyle, pregnancy information, and birth history. The height and weight of lactating women were measured by trained professionals at clinics.
Breast milk was sampled using a standardized method for all subjects to avoid the influence of related factors on the composition of breast milk. The protocol was as follows: nursing mothers were instructed to feed their infants, empty their breasts between 6 and 7 a.m., and collect breast milk between 9 and 11 a.m. Whole milk (fore and hind milk) was collected from one breast using a sterile breast pump. The breast milk was gently mixed and then immediately frozen at −80°C. Around 3 g of infant feces was collected using a sterile collection tube. Samples were stored immediately at −80°C.
SCFAs were extracted from human milk using acetonitrile and derivatized using 3-nitrophenylhdyrazones. SCFAs were analyzed on a Jasper HPLC coupled to a Sciex 4500 MD system. Individual SCFAs were separated on a Phenomenex Kinetex C18 column (100 × 2.1 mm, 2.6 μm) using 0.1% formic acid in water as mobile phase A and 0.1% formic acid in acetonitrile as mobile phase B. Octanoic acid-1-13C1 purchased from Sigma-Aldrich and Butyric-2,2-d2 from CDN Isotopes were used as internal standards for quantitation.
A QIAamp DNA Stool Mini Kit (QIAGEN, Hilden, Germany) was used to extract DNA from samples. The extracted DNA was tested for its concentration (≥12.5 ug/ul), integrity (the main peak of the electrophoresis glue map > 20 kb) and purity (no protein, RNA/salt ion contamination). Samples meeting the above conditions entered the library construction process. Library preparation and subsequent metagenomic sequencing were carried out on the Illumina's novaseq PE150, and the sequencing technology was whole genome shotgun sequencing.
The raw fastq files were demultiplexed based on the indices. The raw data were trimmed and quality controlled using trimmomatic (Version 0.35) and cutadapt (Version 1.16) software. The host sequences were removed by using bowtie2 (Version 2.2.5) aligned with GRCh38/hg38 sequence. The clean data were assembled and predicted ORF by software Megahit (Version 1.2.9) and Prodigal (Version 2.6.3). The predicted genes were clustered and a non-redundant gene catalog was constructed with the CD-HIT (Version 4.8.1) (parameters: identity = 95%, coverage = 90%). The abundance of genes was estimated by the genomeCoverageBed in Bedtools (Version 2.25.0). Then the gene abundance was normalized to 1 MB. The representative sequences for each ORF were assignment for taxonomy against NCBI nr database with a cut-off E-value of 1e-5 by DIAMOND (Version 0.9.27). The taxon was generated into the kingdom, phylum, class, order, family, and genera levels. The ORF were assignment against the KEGG database using the DIAMOND (Version 0.9.27) with a cut-off E-value of 1e-5. For the alpha-diversity analysis, Shannon, simpson, Chao1, Abundance-based coverage estimator (ACE) index was calculated by vegan (Version 2.5-6) in R (Version 3.6.3). The Chao and ACE indices are used to measure community abundance. The larger the Chao and ACE, the higher the community richness. LEfSe analysis was used to identify taxa differences among groups, using the default parameters.
Breast milk was divided into tertiles as high, medium, or low according to the SCFA content, and the differences of intestinal microbiota in alpha diversity, composition and KEGG pathways were compared among the groups used Kruskal-Wallis test.
Continuous variables are described statistically by mean ± standard deviation (SD) or median (P25, P75), and categorical variables are described by proportion or composition ratio. The Shapiro–Wilk method was used to investigate whether the distribution of data variables obeyed normality. Spearman's correlation was used to analyze the correlation between the content of SCFAs in breast milk and the microbiota in breast milk, and the correlation between microbiota in breast milk and infant intestinal microbiota. R 3.6.2 (R Development Core Team, Vienna, Austria) was used to calculate the strength of the symbiotic relationship between the microbiota, and Gephi was used for visualization. Significant differences between groups were analyzed by the KW rank sum test. Differences were considered statistically significant at p < 0.05.
The data for 50 pairs of mothers and infants are shown in Table 1. The average post-natal age was 69.3 ± 40.5 days. Among them, 62.0% of mothers had given birth under the age of 30. The average birth weight of the infants was 3.4 ± 0.7 kg. Among the 50 nursing mothers, there were 26 in the normal weight range (BMI 18.5–23.9), accounting for 52%, and 24 in the overweight and obese group (BMI > 24.0). In addition, there were 9 people with a household monthly income (RMB: yuan) between 5,000 and 19,999, accounting for 18%; 29 people with a household monthly income between 20,000 and 49,999, accounting for 58%; and 12 people with an income >50,000.
We determined seven kinds of SCFAs in human milk with UPLC-MS, including C2, C3, C4, iC4, mC4, C5, and iC5, with an average content of 12.83 ± 36.67, 4.32 ± 2.43, 46.93 ± 38.86, 0.36 ± 3.29, 2.38 ± 1.59, 0.59 ± 0.49, and 0.98 ± 3.53 pmol/g, respectively, Table 2). The total SCFAs were 69.39 ± 86.83 pmol/g.
According to cluster tree analysis of the breast milk microbiota at the genus level (Supplementary Figure 1), the breast milk microbiota was mainly composed of four clusters, namely Streptococcus (27.19%), Lactobacillus (22.70%), Staphylococcus (20.38%), and Enterococcus (10.31%). The breast milk microbiota was mainly composed of Lactobacillus gasseri, Enterococcus faecalis, Staphylococcus epidermidis, and Streptococcus salivarius at the species level.
First, we explored the correlation between the top 20 most-abundant breast milk microbiota and infant gut microbiota based on spearman analysis (Supplementary Figure 2), and found that there was a significant positive correlation between Lactobacillus, Leuconostoc and Gardnerella in breast milk and Lactobacillus in infant gut microbiota. Enterococcus and Escherichia in breast milk showed a significant positive correlation with Enterobacter in infant intestinal microbiota, while Bifidobacterium in breast milk showed a significant positive correlation with Enterococcus in infant intestinal microbiota.
We further explored the correlation of breast milk SCFA contents with the 25 most abundant breast milk microbes (Figure 1A). The results showed that the C2 content in breast milk was positively correlated with the abundance of Streptococcus agalactiae and Enterococcus faecalis. The contents of C3 and mC4 were negatively correlated with the abundance of Streptococcus spp. and positively correlated with that of Staphylococcus epidermidis and Staphylococcus aureus. Meanwhile, the C4 content in breast milk was also positively correlated with the abundance of Lactobacillus oris and negatively correlated with the abundance of Lactobacillus gasseri. The mC4 content of breast milk was positively correlated with the abundance of Lactobacillus lactis. Breastmilk C5 was also found to be correlated negatively with the abundance of Lactobacillus gasseri and positively with that of Streptococcus salivarius and Streptococcus vestibularis.
Figure 1. Correlation heatmap of breast milk SCFAs with the breast milk microbiota at the species level based on Spearman analysis. (A) Represents correlation heatmap of breast milk SCFAs with the top 25 most-abundant breast milk microbes at the species level. (B) Represents the correlation heatmap of SCFAs with Bifidobacterium, Lactobacillus, and Clostridium in breast milk. The horizontal axis represents the SCFAs detected in breast milk, and the vertical axis represents the microbiota at the species level in breast milk. The color represents the r-value of the correlation coefficient (unadjusted), red represents a positive correlation, blue represents a negative correlation, and the darker means a higher r-value, *p < 0.05, **p < 0.01.
The correlations between SCFAs and the breast milk microbiota that have the potential ability to produce SCFAs were also examined, including Bifidobacterium, Lactobacillus, and Clostridium (Figure 1B). The results showed that the C2 content of breast milk was positively correlated with the abundance of Bifidobacterium myosotis and Lactobacillus farraginis in breast milk. The C3 content of breast milk was negatively correlated with the abundance of Clostridium tyrobutyricum in breast milk. The C4 content was correlated positively with the abundance of Bifidobacterium pseudocatenulatum, Bifidobacterium tissieri, Bifidobacterium scaligerum, Bifidobacterium myosotis, and Lactobacillus antri and negatively with that of Lactobacillus crispatus and Lactobacillus hominis. The contents of C3, mC4, and C5 were negatively correlated with the abundance of Lactobacillus crispatus, Lactobacillus jensenii, Lactobacillus delbrueckii, Lactobacillus ruminis, and Lactobacillus iners in breast milk. The abundances of C4 and C5 were positively correlated with that of Clostridium leptum in breast milk.
Based on the above results, we found that C4 was significantly related to the level of various Bifidobacterium spp. in human milk. We hypothesized that the main species of C4-producing bacteria may have an interactive symbiosis with specific Bifidobacterium spp. in human milk. Therefore, we further conducted the above microbiota analysis through a network symbiosis relationship diagram to explore its symbiosis inference. According to the analysis of the Spearman symbiotic relationship (Figure 2A), Clostridium leptum in breast milk had a direct symbiotic relationship with Bifidobacterium pseudocatenulatum and Clostridium innocuum in human milk (Figure 2B). Moreover, Clostridium leptum had an indirect symbiotic relationship with most Bifidobacterium spp. in breast milk, such as Bifidobacterium reuteri, Bifidobacterium lemurum, Bifidobacterium biavatii, Bifidobacterium mongolinse, and Bifidobacterium dentium, through Clostridium innocuum (Figure 2C), which suggest that the main species of C4-producing bacteria have an interactive symbiosis with specific Bifidobacterium spp. in human milk.
Figure 2. Symbiotic network diagram of breast milk microorganisms based on the Spearman relationship. (A) Symbiotic network diagram of Bifidobacterium, Lactobacillus, and Clostridium in breast milk. (B, C) Respectively represent the symbiotic relationship between Clostridium leptum in human milk and different Bifidobacterium spp. at the species level. Red represents a positive correlation, blue represents a negative correlation, and the thickness of the lines represents the strength of the correlation.
We categorized the SCFA levels in breast milk into high, medium, and low according to their content. Then, we further analyzed the differences in the diversity and composition of infants' intestinal microbiota among different breast milk SCFA groups. For the alpha diversity index, there were no significant differences between the C2, C4, iC4, mC4, C5, and iC5 groups in the Chao, ACE, or Shannon and Simpson indexes (Supplementary Figure 3). The Chao and ACE indexes of the high C3 group were significantly higher than those of the low and medium groups.
PLS-DA analysis showed that the contents of different SCFAs in breast milk, including C2, C3, C4, iC4, mC4, C5, and iC5, were significantly associated with the overall composition of the infant intestinal microbiota (Supplementary Figure 4).
To further analyze the impact of milk SCFAs on the specific composition of the infant intestinal microbiota, we analyzed the above association at the genus and species levels, respectively (Supplementary Figure 5). The results showed that the infant intestinal microbiota was mainly composed of Bifidobacteria, Escherichia, Lactobacillus, and Bacteroides at the genus level, and the content of SCFAs in human milk was mainly related to the composition of Bifidobacterium, Lactobacillus, and Lacticaseibacillus in infants' microbiota.
LEfSe analyses of the infants' intestinal microbiota were conducted to determine significant biomarkers of different breast milk SCFA levels. It was found that infants in the low C4 tertile (6.48–20.57 pmol/g) had a relatively high abundance of Salmonella and Salmonella enterica; the medium C4 tertile (21.81–45.11 pmol/g) had a relatively high abundance of Microcystis and Eubacterium, and the high C4 tertile (47.05–140.11 pmol/g) had a relatively high abundance of Ruminococcus gnavus and Clostridiales bacterium (Figure 3A). A significantly different microbial biomarker was also found for C5, in which the medium tertile had a relatively high abundance of Lactobacillus paracasei, Streptococcus agalactiae, and Staphylococcus sp. and the high C5 tertile (0.64- 2.35 pmol/g) had a relatively high abundance of Clostridiaceae and Ruminiclostridium papyrosolvens (Figure 3B). Biomarkers of other SCFAs were not significantly different, and the results are not shown.
Figure 3. Biomarkers of differences between different SCFA content groups in human milk. (A, B) Represent LEfSe analysis of C4 and C5 groups, respectively. Taxonomic cladogram obtained from LEfSe and linear discriminant analysis (LDA) of these groups. Biomarker taxa are highlighted with colored circles and shaded areas. Each circle's diameter reflects the abundance of those taxa in the community, using a cutoff value of ≥2.0. C4: low (6.48–20.57 pmol/g), medium (21.81–45.11 pmol/g) and high content (47.05–140.11 pmol/g); C5: low (0.10–0.29 pmol/g), medium (0.33–0.63 pmol/g) and high content (0.64–2.35 pmol/g).
Based on the above analysis of significant biomarkers of the intestinal microbiota at the species level, we screened the key SCFAs (C4 and C5) in breast milk for their effects on metabolic pathways by conducting a functional correlation study. We classified the metabolic pathways at the functional level using KEGG pathway analysis. The 50 infants' microbiota corresponded to 6 first-level, 45 second-level, and 422 third-level metabolic pathways. Focusing on the studied population, we attempted to analyze 200 out of the 422 tertiary metabolic pathways under 17 KEGG secondary metabolic pathway branches (including carbohydrate metabolism, amino acid metabolism, cofactors and vitamins, lipid metabolism, circulatory system, digestive system, endocrine system, excretory system, immune system, etc.), which are strongly related to early health, growth, and development.
The results showed significant differences in arginine and lysine biosynthesis among different breast milk C4 levels, in which the high and medium groups had a higher abundance than the low C4 content groups (Figure 4A). There were also significant differences in carotenoid biosynthesis among C5 groups, in which the high tertile had a relatively high abundance (Figure 4B).
Figure 4. Analysis of specific SCFAs and KEGG tertiary metabolic pathway abundance levels in human milk with different SCFA contents. (A, B) Represent the KEGG tertiary enrichment and differential pathways of breast milk C4 and C5, respectively, in different content groups (orange, green, and blue indicate low, medium, and high content, respectively). A Kruskal–Wallis rank sum test was used to compare the KEGG functional levels of different SCFA groups in breast milk (orange, green, and blue indicate low, medium, and high content, respectively). The horizontal coordinate represents the sample information detected. Yellow to red indicates the abundance distribution of different samples in tertiary metabolic pathways from low to high. The lower bar represents the tertiary metabolic pathways with significant differences. Differences were considered significant at p < 0.05. C4: low (6.48–20.57 pmol/g), medium (21.81–45.11 pmol/g) and high content (47.05–140.11 pmol/g); C5: low (0.10–0.29 pmol/g), medium (0.33–0.63 pmol/g) and high content (0.64–2.35 pmol/g).
We further searched the KO (Kyoto Encyclopedia of Genes and Genomes Orthology) corresponding to the above-mentioned differential metabolic pathways in the KEGG database. For C4, the most significant corresponding signal pathways were arginine biosynthesis and lysine biosynthesis, which involved 61 and 112 related KO reported in the KO database, respectively. When these KO were compared to the total of 10,016 KO annotated with the microbiota data from this study, it was found that 49 KO were under the arginine biosynthesis pathway, and 88 were under the lysine biosynthesis pathway. A KW rank sum test was used to compare the relative KO abundances between C4 content groups. The functional relative abundances of K01915 (in the arginine biosynthesis pathway), which corresponds to glutamine synthetase [EC:6.3.1.2], were significantly higher in the medium and high C4 groups (Figure 5A). K00620 (in lysine biosynthesis), which corresponds to amino acid N-acetyltransferase [EC:2.3.1.35 2.3.1.1], was significantly higher in the medium and high C4 group. K01915 (in lysine biosynthesis), which corresponds to glutamine synthetase [EC:6.3.1.2], was significantly higher in the medium and high C4 groups (Figure 5B). There was no significant difference in the KO involved in different signal pathways between C5 content groups.
Figure 5. Kyoto Encyclopedia of Genes and Genomes Orthology (KO) analysis of significant differences for certain breast milk SCFAs. (A, B) Represent the KO analysis of breast milk C4 of the arginine and lysine biosynthesis pathways, and the results are shown in the box diagram (orange, green, and blue indicate low, medium, and high content, respectively). The horizontal coordinate represents the different SCFAs groups, and the vertical coordinate represents the abundance of the corresponding differential KO, in which K01915 corresponds to glutamine synthetase [EC:6.3.1.2], and K00620 corresponds to amino-acid N-acetyltransferase [EC:2.3.1.35 2.3.1.1]. *p < 0.05. C4: low (6.48–20.57 pmol/g), medium (21.81–45.11 pmol/g) and high content (47.05–140.11 pmol/g); C5: low (0.10–0.29 pmol/g), medium (0.33–0.63 pmol/g) and high content (0.64–2.35 pmol/g). **p < 0.01.
In this study, the associations between the breast milk microbiota and SCFAs in milk were investigated by correlation analysis, and the potential effect of different contents of breast milk SCFAs on infants' intestinal microbiota were further explored. To the best of our knowledge, we are the first to report that the specific Bifidobacterium spp. in human milk may have an interactive symbiosis with main species of C4-producing bacteria and help to produce C4 in breast milk. We also suggested that certain breast milk SCFAs that might be involved in inhibiting infant gut pathogens, such as C4, were found to be significantly associated with lower prominence of certain microbial species in the infants' gut, including Salmonella, Salmonella enterica, and Eubacterium. Moreover, based on KEGG pathway analysis, the changes of microbiota associated with SCFAs related to the key metabolic pathways for infants.
Current research demonstrates that SCFAs in breast milk may be produced by the mother's gut bacteria and transported to the mammary gland through the circulatory system (Stinson et al., 2020; Stinson and Geddes, 2022). Scientists also theoretically suggested that milk SCFAs could be metabolized by bacteria in breast milk (Stinson and Geddes, 2022); however, there is no experimental evidence to support this hypothesis, and the real-world data on the association between SCFAs and the microbiota in human breast milk are limited. Based on the current results, we did not find significant associations of C2, C3, C5, and iC5 in breast milk with Bifidobacterium and Lactobacillus in breast milk, which were considered as the main SCFA-producing microbials (Yang et al., 2022). The above results indirectly supports the idea that these milk SCFAs came from the maternal gut.
Surprisingly, we also noticed that the levels of C4 and C5 in breast milk were significantly positively correlated with the abundance of Clostridium leptum in breast milk. Clostridium leptum is the most abundant butyrate-producing bacteria. Moreover, according to the symbiotic network relationship analysis, breast milk C4-producing bacteria, such as Clostridium leptum and Clostridium innocuum, were observed to be significantly correlated with Bifidobacterium at the species level in breast milk. This phenomenon was also observed in in vitro experiments and the human gut (Prentice et al., 2019; Stinson et al., 2020). It could be explained by the fact that most butyrate-producing bacteria cannot directly ferment prebiotics, while some lactic acid bacteria (e.g., Bifidobacterium) can partially hydrolyze prebiotics that are difficult to digest and produce intermediate metabolites, such as short oligosaccharides and monosaccharides. In addition, lactic acid bacteria can also completely hydrolyze certain prebiotics to produce metabolic end products, such as C2 and lactic acid, and then allow the butyrate-producing bacteria to further ferment these products as secondary substrates to produce C4. Therefore, although the symbiotic network was first observed in human breast milk, we could reasonably speculate that breast milk C4 could be produced by C4-producing bacteria in breast milk with the help of lactic acid-producing bacteria.
This study further revealed that the composition of the intestinal microbiota in infants is significantly associated with maternal SCFAs. According to the results of diversity analysis, we observed several SCFAs associated with the overall composition and distribution of infants' intestinal microbiota.
SCFAs induce a wide range of biological effects through interactions with G protein-coupled receptors and inhibition of histone deacetylase, including antibacterial and anti-inflammatory activities and promoting the integrity of the intestinal epithelial barrier (Chen et al., 2023). However, most previous findings based on in vitro studies or investigated on SCFAs exhibited in the gut rarely reported SCFA in breast milk and their functions. Our study extended the previous findings by illustrating that the breast milk SCFAs could also perform certain functions in infants. We observed significant differences in gut microbial composition between different breast milk SCFA levels according to LEfSe analysis. The results showed that the content of Salmonella in the low C4 group was relatively high, while that of Eubacterium and Clostridiales bacterium in the medium and high C4 groups were relatively high, which might indicate that consuming breast milk with a high C4 could help to inhibit the growth of intestinal pathogenic bacteria. A previous animal study, which showed that butyrate could inhibit the spread of Salmonella in the intestine and further enhance the barrier function of the gut mucosa, supported our findings (Homann et al., 2023).
Considering the potential function of the abovementioned different infant intestinal microbes regulated by SCFAs, we further conducted KEGG pathway analysis to explore the potential gene expression differences and metabolic pathways among different C4 levels. The results revealed that C4 is associated with high expression of K00620 in the lysine biosynthesis pathway, corresponding to amino-acid N-acetyltransferase [EC:2.3.1.35 2.3.1.1]. Amino acid acetyltransferase can transfer the acetyl group in acetyl-CoA to the amino group in the amino acid molecule and is a key enzyme in lysine bio-anabolism (Liu et al., 2022; Ghasemi et al., 2023). As an essential amino acid for infants, lysine plays an important role in regulating the physiological activity of intestinal epithelial cells and inhibiting the colonization of harmful bacteria (Wang et al., 2023). Gebeyew et al. (2021) reported that dietary lysine supplementation improved intestinal barrier function and down-regulated mRNA expression of intestinal inflammatory factors such as IL-1β, TNF-α, interferon-γ, and toll-like receptor-4. Lysine-derived Maillard reaction products inhibit the growth of Salmonella enterica (Wong et al., 2023). The mechanism of their inhibition of Salmonella may be closely related to lysine acetylation, which can inhibit protein activity and DNA binding ability in pathogenic bacteria (Koo et al., 2020). Meanwhile, C4 is closely related to modification by acetylation (Kang et al., 2023).
Similarly, we also identified that K01915 [glutamine synthetase (EC:6.3.1.2)] was highly expressed in medium and high C4 breast milk groups, which response to the arginine biosynthesis metabolism (Xie et al., 2023). Arginine plays an important role in regulating intestinal epithelial cells and inhibiting the expression of inflammatory factors (Ge et al., 2022). At present, the relationship between the C4 content in breast milk and arginine metabolism has not been reported. Although our study revealed that C4 levels in breast milk may be related to infant arginine metabolism, this finding should be validated in future studies. In summary, according to KEGG analysis, we can further demonstrate that breast milk C4 may inhibit the abundance of harmful bacteria by regulating amino acid metabolism and intestinal mucosal barrier function.
The strength of this study lies in its use of metagenome sequencing, which can identify specific intestinal bacteria at the species level that could be affected by SCFAs. We also adopted the method of maternal–infant pairs to explore the role of breast milk in infant health. Combined with KEGG analysis, the data could further clarify the function of breast milk SCFAs and provide a more detailed explanation for the mechanisms involved in their function.
Four limitations should be addressed in our study. Firstly, as it was a pilot study, the sample size was limited. Secondly, this study was a cross-sectional study, and causation cannot be fully determined. Thirdly, although several confounders were taken into consideration in this study, there are plenty confounding factors in breast milk that could affect the results, such as other fatty acid and prebiotic-like ingredients. Future prospective studies with a large sample size are required to confirm the results of this study and further examine the long-term effects of breast milk SCFAs on infant's intestinal microbiota and development. Fourthly, in this study, SCFA contents in infant feces were not detected due to the limited amount of infant stool samples collected. Account the SCFA contents analysis in future study could provide additional insights into how SCFAs from breast milk influence the gut microbiota of infants.
Based on whole genome shotgun sequencing and UPLC-MS analyses, the current results suggest that in human milk the specific Bifidobacterium may have an interactive symbiosis with main species of C4-producing bacteria and help to produce C4 in breast milk. Certain breast milk SCFAs, such as C4, might be involved in inhibiting infant gut pathogens. The current study reveals that C4 might correlate with increased abundance or presence of specific enzymes, which corresponds to the potential regulatory function of amino acid metabolism. These findings may illustrate the potential bond between maternal and infant health via breast milk SCFAs. In the future, large-scale prospective studies can be conducted to further explore the long-term effects and mechanisms of breast milk SCFAs on the gut microbiota and development of early life.
The datasets presented in this study can be found in online repositories. The names of the repository/repositories and accession number(s) can be found in the article/Supplementary material.
The protocol was approved by the Research Center for Public Health, of Tsinghua University (No. THUSM/PHREC/2021-003). The studies were conducted in accordance with the local legislation and institutional requirements. Written informed consent for participation in this study was provided by the participants' legal guardians/next of kin.
MX: Conceptualization, Data curation, Formal analysis, Investigation, Methodology, Writing – original draft. YY: Data curation, Formal analysis, Investigation, Writing – original draft. SD: Data curation, Formal analysis, Investigation, Writing – original draft. TL: Data curation, Formal analysis, Investigation, Writing – original draft. IS: Data curation, Formal analysis, Investigation, Writing – original draft. AZ: Project administration, Supervision, Writing – review & editing.
The author(s) declare financial support was received for the research, authorship, and/or publication of this article. This work was supported by National Center of Technology Innovation for Dairy and Inner Mongolia Dairy Technology Research Institute (Hohhot Science & Technology Plan, 2022-Ke Yan Gong Guan-No. 4 Project), School-enterprise Cooperation Project of Inner Mongolia Yili Industrial Co. Ltd. (Project No.: 20212001788), and General Program of National Natural Science Foundation of China (Project No.: 82273619).
We are sincerely grateful to the participating mothers and local health professionals for their contribution and support in this study.
YY, SD, TL, and IS were employed by Inner Mongolia Yili Industrial Group Co. Ltd. and Inner Mongolia Dairy Technology Research Institute Co. Ltd.
The remaining authors declare that the research was conducted in the absence of any commercial or financial relationships that could be construed as a potential conflict of interest.
All claims expressed in this article are solely those of the authors and do not necessarily represent those of their affiliated organizations, or those of the publisher, the editors and the reviewers. Any product that may be evaluated in this article, or claim that may be made by its manufacturer, is not guaranteed or endorsed by the publisher.
The Supplementary Material for this article can be found online at: https://www.frontiersin.org/articles/10.3389/fmicb.2024.1356462/full#supplementary-material
Brahe, L. K., Astrup, A., and Larsen, L. H. (2013). Is butyrate the link between diet, intestinal microbiota and obesity-related metabolic diseases? Obes. Rev. 14, 950–959. doi: 10.1111/obr.12068
Chen, M., Peng, L., Zhang, C., Liu, Q., Long, T., and Xie, Q. (2023). Gut microbiota might mediate the benefits of high-fiber/acetate diet to cardiac hypertrophy mice. J. Physiol. Biochem. 79, 745–756. doi: 10.1007/s13105-023-00971-3
Fougère, H., Greffard, K., Guillot, M., Rudkowska, I., Pronovost, E., Simonyan, D., et al. (2023). Docosahexaenoic acid-rich algae oil supplementation in mothers of preterm infants is associated with a modification in breast milk oxylipins profile. Lipids Health Dis. 22, 103. doi: 10.1186/s12944-023-01870-8
Gao, Y., Davis, B., Zhu, W., Zheng, N., Meng, D., and Walker, W. A. (2021). Short-chain fatty acid butyrate, a breast milk metabolite, enhances immature intestinal barrier function genes in response to inflammation in vitro and in vivo. Am. J. Physiol. Gastrointest. Liver Physiol. 320, G521–G530. doi: 10.1152/ajpgi.00279.2020
Gates, A., Hair, A. B., Salas, A. A., Thompson, A. B., and Stansfield, B. K. (2023). Nutrient composition of donor human milk and comparisons to preterm human milk. J. Nutr. 153, 2622–2630. doi: 10.1016/j.tjnut.2023.07.012
Ge, Y., Li, F., He, Y., Cao, Y., Guo, W., Hu, G., et al. (2022). L-arginine stimulates the proliferation of mouse mammary epithelial cells and the development of mammary gland in pubertal mice by activating the GPRC6A/PI3K/AKT/mTOR signalling pathway. J. Anim. Physiol. Anim. Nutr. 106, 1383–1395. doi: 10.1111/jpn.13730
Gebeyew, K., Yang, C., He, Z., and Tan, Z. (2021). Low-protein diets supplemented with methionine and lysine alter the gut microbiota composition and improve the immune status of growing lambs. Appl. Microbiol. Biotechnol. 105, 8393–8410. doi: 10.1007/s00253-021-11620-4
George, A. D., Gay, M. C. L., Wlodek, M. E., and Geddes, D. T. (2021). The importance of infants' lipid intake in human milk research. Nutr. Rev. 79, 1353–1361. doi: 10.1093/nutrit/nuaa141
Ghasemi, A., Farazmand, A., Hassanzadeh, V., Poursani, S., Soltani, S., Akhtari, M., et al. (2023). Upregulation of KAT2B and ESCO2 gene expression level in patients with rheumatoid arthritis. JCR 42, 253–259. doi: 10.1007/s10067-022-06351-4
Gotoh, K., and Shibata, H. (2023). Association between the gut microbiome and the renin-angiotensin-aldosterone system: a possible link via the activation of the immune system. Hypertens. Res. 46, 2315–2317. doi: 10.1038/s41440-023-01384-x
Homann, C., Eckey, I., Chuppava, B., Teich, K., Buch, J., Zimmermann, A., et al. (2023). Rye and rye bran as components of diets in piglet production-effects on Salmonella prevalence. Animals 13:2262. doi: 10.3390/ani13142262
Kang, X., Li, C., Liu, S., Baldwin, R., Liu, G. E., and Li, C. J. (2023). Genome-wide acetylation modification of H3K27ac in bovine rumen cell following butyrate exposure. Biomolecules 13:1137. doi: 10.3390/biom13071137
Koo, H., Park, S., Kwak, M. K., and Lee, J. S. (2020). Regulation of gene expression by protein lysine acetylation in Salmonella. J. Microbiol. 58, 979–987. doi: 10.1007/s12275-020-0483-8
Liu, H. Y., Liu, Y. Y., Zhang, Y. L., Ning, Y., Zhang, F. L., and Li, D. Q. (2022). Poly(ADP-ribosyl)ation of acetyltransferase NAT10 by PARP1 is required for its nucleoplasmic translocation and function in response to DNA damage. CCS 20:127. doi: 10.1186/s12964-022-00932-1
Meijer, K., de Vos, P., and Priebe, M. G. (2010). Butyrate and other short-chain fatty acids as modulators of immunity: what relevance for health? Curr. Opin. Clin. Nutr. Metab. Care. 13, 715–721. doi: 10.1097/MCO.0b013e32833eebe5
Nogal, A., Asnicar, F., Vijay, A., Kouraki, A., Visconti, A., Louca, P., et al. (2023). Genetic and gut microbiome determinants of SCFA circulating and fecal levels, postprandial responses and links to chronic and acute inflammation. Gut Microbes 15:2240050. doi: 10.1080/19490976.2023.2240050
Ouyang, R., Zheng, S., Wang, X., Li, Q., Ding, J., Ma, X., et al. (2023). Crosstalk between breast milk N-acetylneuraminic acid and infant growth in a gut microbiota-dependent manner. Metabolites 13:846. doi: 10.3390/metabo13070846
Ozen, M., Piloquet, H. S., and Chaubeck, M. (2023). Limosilactobacillus fermentum CECT5716: clinical potential of a probiotic strain isolated from human milk. Nutrients 15:2207. doi: 10.3390/nu15092207
Prentice, P. M., Schoemaker, M. H., Vervoort, J., Hettinga, K., Lambers, T. T., Acerini, C. L., et al. (2019). Human milk short-chain fatty acid composition is associated with adiposity outcomes in infants. J. Nutr. 149, 716–722. doi: 10.1093/jn/nxy320
Shi, J., Ma, D., Gao, S., Long, F., Wang, X., Pu, X., et al. (2023). Probiotic Escherichia coli Nissle 1917-derived outer membrane vesicles modulate the intestinal microbiome and host gut-liver metabolome in obese and diabetic mice. Front. Microbiol. 14:1219763. doi: 10.3389/fmicb.2023.1219763
Stinson, L. F., Gay, M. C. L., Koleva, P. T., Eggesb, M., Johnson, C. C., Wegienka, G., et al. (2020). Human milk from atopic mothers has lower levels of short chain fatty acids. Front. Immunol. 11:1427. doi: 10.3389/fimmu.2020.01427
Stinson, L. F., and Geddes, D. T. (2022). Microbial metabolites: the next frontier in human milk. Trends Microbiol. 30, 408–410. doi: 10.1016/j.tim.2022.02.007
Szugye, H., Murra, A., and Lam, S. K. (2023). A new policy update on breastfeeding: what all clinicians need to know. Cleveland Clin. J. Med. 90, 469–473. doi: 10.3949/ccjm.90a.22099
Wang, Y., Sun, W., Yan, S., Meng, Z., Jia, M., Tian, S., et al. (2023). A new strategy to alleviate the obesity induced by endocrine disruptors-A unique lysine metabolic pathway of nanoselenium Siraitia grosvenorii to repair gut microbiota and resist obesity. FCT 175:113737. doi: 10.1016/j.fct.2023.113737
Wong, C. W. Y., Mu, K., Kitts, D. D., and Wang, S. (2023). Lysine-derived maillard reaction products inhibit the growth of salmonella enterica serotype typhimurium. Pathogens. 12:215. doi: 10.3390/pathogens12020215
Xie, Y., Lv, Y., Jia, L., Zheng, L., Li, Y., Zhu, M., et al. (2023). Plastid-localized amino acid metabolism coordinates rice ammonium tolerance and nitrogen use efficiency. Nat. Plants 9, 1514–1529. doi: 10.1038/s41477-023-01494-x
Xu, W., Ishii, Y., Rini, D. M., Yamamoto, Y., and Suzuki, T. (2023). Microbial metabolite n-butyrate upregulates intestinal claudin-23 expression through SP1 and AMPK pathways in mouse colon and human intestinal Caco-2 cells. Life Sci. 329:121952. doi: 10.1016/j.lfs.2023.121952
Keywords: short-chain fatty acids, breast milk, whole genome shotgun sequencing, infant microbiota, KEGG
Citation: Xi M, Yan Y, Duan S, Li T, Szeto IM-Y and Zhao A (2024) Short-chain fatty acids in breast milk and their relationship with the infant gut microbiota. Front. Microbiol. 15:1356462. doi: 10.3389/fmicb.2024.1356462
Received: 15 December 2023; Accepted: 29 January 2024;
Published: 19 February 2024.
Edited by:
Lorenzo Nissen, University of Bologna, ItalyReviewed by:
Yu Pi, Chinese Academy of Agricultural Sciences, ChinaCopyright © 2024 Xi, Yan, Duan, Li, Szeto and Zhao. This is an open-access article distributed under the terms of the Creative Commons Attribution License (CC BY). The use, distribution or reproduction in other forums is permitted, provided the original author(s) and the copyright owner(s) are credited and that the original publication in this journal is cited, in accordance with accepted academic practice. No use, distribution or reproduction is permitted which does not comply with these terms.
*Correspondence: Ai Zhao, YWl6aGFvMThAdHNpbmdodWEuZWR1LmNu
Disclaimer: All claims expressed in this article are solely those of the authors and do not necessarily represent those of their affiliated organizations, or those of the publisher, the editors and the reviewers. Any product that may be evaluated in this article or claim that may be made by its manufacturer is not guaranteed or endorsed by the publisher.
Research integrity at Frontiers
Learn more about the work of our research integrity team to safeguard the quality of each article we publish.