- 1College of Forestry, Gansu Agricultural University, Lanzhou, China
- 2Department of Soil and Environmental Sciences, University of Poonch Rawalakot, Rawalakot, Pakistan
- 3College of Grassland Science, Gansu Agricultural University, Lanzhou, China
- 4Biology Department, College of Science, Tabuk University, Tabuk, Saudi Arabia
- 5Department of Biological Sciences, Faculty of Science and Humanities, Shaqra University, Ad-Dawadimi, Saudi Arabia
- 6Biology Department, Faculty of Science, Al-Baha University, Alaqiq, Saudi Arabia
- 7Department of Biological Science, College of Science & Humanities, Shaqra University, Riyadh, Saudi Arabia
- 8Seeds Development Department, El-Nada Misr Scientific Research and Development Projects, Turrell, Mansoura, Egypt
- 9Maryout Research Station, Genetic Resources Department, Desert Research Center, Cairo, Egypt
- 10College of Horticulture and the Fujian provincial Key Laboratory of Plant Functional Biology, Fujian Agricultural and Forestry University, Fuzhou, China
- 11Department of Agronomy, University of Poonch Rawalakot, Rawalakot, Pakistan
- 12Department of Biology, College of Sciences and Arts-Rafha, Northern Border University, Arar, Saudi Arabia
Climate change is one of the main challenges, and it poses a tough challenge to the agriculture industry globally. Additionally, greenhouse gas (GHG) emissions are the main contributor to climate change; however, croplands are a prominent source of GHG emissions. Yet this complex challenge can be mitigated through climate-smart agricultural practices. Conservation tillage is commonly known to preserve soil and mitigate environmental change by reducing GHG emissions. Nonetheless, there is still a paucity of information on the influences of conservation tillage on wheat yield, soil properties, and GHG flux, particularly in the semi-arid Dingxi belt. Hence, in order to fill this gap, different tillage systems, namely conventional tillage (CT) control, straw incorporation with conventional tillage (CTS), no-tillage (NT), and stubble return with no-tillage (NTS), were laid at Dingxi, Gansu province of China, under a randomized complete block design with three replications to examine their impacts on yield, soil properties, and GHG fluxes. Results depicted that different conservative tillage systems (CTS, NTS, and NT) significantly (p < 0.05) increased the plant height, number of spikes per plant, seed number per meter square, root yield, aboveground biomass yield, thousand-grain weight, grain yield, and dry matter yield compared with CT. Moreover, these conservation tillage systems notably improved the soil properties (soil gravimetric water content, water-filled pore space, water storage, porosity, aggregates, saturated hydraulic conductivity, organic carbon, light fraction organic carbon, carbon storage, microbial biomass carbon, total nitrogen, available nitrogen storage, microbial biomass nitrogen, total phosphorous, available phosphorous, total potassium, available potassium, microbial counts, urease, alkaline phosphatase, invertase, cellulase, and catalase) while decreasing the soil temperature and bulk density over CT. However, CTS, NTS, and NT had non-significant effects on ECe, pH, and stoichiometric properties (C:N ratio, C:P ratio, and N:P ratio). Additionally, conservation-based tillage regimes NTS, NT, and CTS significantly (p < 0.05) reduced the emission and net global warming potential of greenhouse gases (carbon dioxide, methane, and nitrous oxide) by 23.44, 19.57, and 16.54%, respectively, and decreased the greenhouse gas intensity by 23.20, 29.96, and 18.72%, respectively, over CT. We conclude that NTS is the best approach to increasing yield, soil and water conservation, resilience, and mitigation of agroecosystem capacity.
1 Introduction
Climate change is one of the main challenges, and it poses a tough challenge to the agriculture industry globally (Feng et al., 2023). Additionally, greenhouse gas (GHG) emissions are the main contributor to environmental change, and croplands are potential sources of major GHG emissions, for instance, carbon dioxide (CO2), methane (CH4), and nitrous oxide (N2O), that lead to increased global warming (Pu et al., 2022; Wu et al., 2022; Li et al., 2023). The agricultural sector, particularly farmlands, mainly contributes almost 12% of GHG emissions in the atmosphere (Raihan and Tuspekova, 2022). The average worldwide temperature will rise by 3.8°C by the end of the 21st century if the GHG concentrations continue to rise to the existing level (IPCC, 2021). Consequently, for achieving carbon reduction or carbon neutrality goals, the decline of emissions of major GHG is very significant to alleviate greenhouse effects in the agroecosystem. In addition, croplands have very low soil organic matter (SOM) due to environmental limitations that limit primary crop productivity (Yuan et al., 2023a). One approach to increasing soil and water conservation and crop yields while reducing greenhouse gas emissions in crop cultivation systems is to adopt climate-smart farming practices known as conservation agriculture (Ma et al., 2021; Roy et al., 2022; Li et al., 2023).
Wheat is lately one of the vigorous cereal crops with universal importance, and it has a massive influence on global food security (Huang et al., 2003). Approximately 24 million hectares’ area in China is under cultivation (Li et al., 2019). Climate change or environmental variability seriously affects crop production in the agriculture sector in China, especially in the Northwestern Loess Plateau (Alhassan et al., 2021). Furthermore, traditional or conventional tillage is the dominant crop cultivation practice in wheat cropping, generally plowing two times a year with the removal of crop straw in China, particularly in the Dingxi belt. Nevertheless, this CT practice can cause variations in soil properties (physical, chemical, biochemical, and biological) (Jat et al., 2020; Sadiq et al., 2021a; Yuan et al., 2023a), crop yield (Alhassan et al., 2021; Sadiq et al., 2021b), and greenhouse gas fluxes (Alhassan et al., 2021; Yuan et al., 2022; Feng et al., 2023) and enhance the risk of soil degradation by erosion (Liu et al., 2015; Gao et al., 2019) and global warming by greenhouse gas emissions (Alhassan et al., 2021; Feng et al., 2023). To decrease land, crop, and environmental degradation under traditional tillage measures, substantial consideration has been paid lately to soil conservation tillage as a maintainable approach for cropland ecosystems (Wulanningtyas et al., 2021; Kristine et al., 2022; Yuan et al., 2022). Soil conservation tillage is an innovative mode of modern farming that might effectively mitigate and solve the negative influences and problems of intensive tillage (Lv et al., 2023).
Conservation tillage system broadly refers to techniques employing no or less soil inversion and a minimum number of tillage operations lacking of any soil inversion and leaving at least 30% stubbles on the surface of the soil, which enhances soil and water conservation. It could be roughly divided into straw incorporation into the field, no-tillage, mulch tillage, or straw-retention with no-tillage system, minimum tillage, ridge tillage, and strip tillage (Yuan et al., 2023a). It is a well-established approach to improving soil physicochemical, microbial, and crop yields, reducing greenhouse gas emissions, mitigating negative influences of conventional tillage and climate change through soil carbon sequestering in agricultural systems, and improving agricultural and environmental sustainability globally (Jat et al., 2021; Yuan et al., 2023b). The worldwide population is exerting a noteworthy burden on land resources due to intensive land cultivation strategies that destroy the soil and environmental quality. Consequently, soil quality must be sustained to certify crop yield and soil and environmental sustainability (Lal, 2005; Pu et al., 2022).
Sustainable crop production in the agricultural industry is highly dependent on the sustainability of the soil system and restricted by soil properties (physical, chemical, and biological) (Wang et al., 2008; Wozniak and Gos, 2014; Indoria et al., 2016). Land management strategies that would fulfill the food demand globally and preserve as well as conserve the previously stressed environmental conditions (Lal, 2005) are significant to sustainable crop production. Conservation tillage practices, for instance, straw-retention or residue mulch and no-tillage systems, are commonly advocated to preserve the soil (Wulanningtyas et al., 2021; Krauss et al., 2022). No-tillage with a crop stubble integration is a more operative approach for enhancing the properties of soil (Yuan et al., 2023a,b), crop yield (Sadiq et al., 2021b), and reduction of global warming (Alhassan et al., 2021), as well as preserving soil health by increasing the quality of soil (Sadiq et al., 2021a). Nevertheless, the influence of conservation tillage practices on soil properties, crop yield, and global warming mitigation has been intensively discussed due to the extensive contradiction in distinct field research (Zheng et al., 2014). Previous studies have depicted that conservation tillage significantly reduced soil properties (Zhao et al., 2014; Khan et al., 2017), crop yield (Taa et al., 2004), and greenhouse gas emissions (Pu et al., 2022; Salamanca-Fresno et al., 2022; Li et al., 2023). On the contrary, conservation tillage pointedly increased soil properties (Jat et al., 2020; Sadiq et al., 2021a), crop yield (Sadiq et al., 2021b; Yuan et al., 2023a), and greenhouse gas emissions (Dencso et al., 2020). Researchers have also demonstrated insignificant change regarding soil properties (Bayer et al., 2015), yield (Lampurlanes et al., 2002), and greenhouse gas fluxes (Bayer et al., 2015) under conservation agriculture and conventional tillage practice. Consequently, more study on the influence of soil tillage on soil properties, crop yield, and global warming is needed.
The accumulation of GHG in the atmosphere alters the earth’s energy balance and participates in the boosted “greenhouse effect.” Additionally, GHG emissions have also been implicated in environmental chemistry given their contribution to the depletion of stratospheric ozone (IPCC, 2013). Global warming is chiefly attributed to the raised GHG concentrations in the atmosphere by anthropogenic activities (O’Neill et al., 2021; Wu et al., 2022). Soil management activities and climatic situations determine the croplands’ capacity to yield, transport, and consume GHG and accordingly determine the direction and intensity of GHG fluxes in farmlands. Conservation tillage practices have been proposed as a substitute land management technique for CT that can mitigate the agricultural sector’s environmental influence through a reduction of GHG emissions (Li et al., 2023). Higher CO2 emission was stated by Yeboah et al. (2016) and Dencso et al. (2020) under conservation tillage, while Salamanca-Fresno et al. (2022) reported an almost 50% reduction in CO2 flux in conservation tillage treatment compared with CT. In agroecosystems, CH4 is also a significant greenhouse gas and acts as a sink or source (Maucieri et al., 2021). The CH4 uptake improved under conservation tillage in comparison with CT (Alhassan et al., 2021; Pu et al., 2022); however, Maucieri et al. (2021) did not find any significant difference between conservation and conventional agricultural practices. Regarding N2O flux, Yuan et al. (2022) found a reduction in its emission under conservative tillage systems, whereas Pu et al. (2022) reported the maximum emission of N2O under conservation agriculture over CT. Moreover, soil physical, chemical, and biological quality indicators greatly influenced GHG fluxes (Alskaf et al., 2021; Hu et al., 2022). A significant correlation between positive and negative GHG fluxes and soil properties was found by many scholars globally (Yeboah et al., 2016; Feng et al., 2018; Huang et al., 2018; Mei et al., 2018; Alhassan et al., 2021; Shakoor et al., 2021; Li et al., 2023). Greater inconsistency in emissions of major GHG from croplands under divergent land management practices has required further investigation under site-specific and soil conditions.
Agroecosystems’ response to diverse soil tillage management systems strongly depends on the local environmental and socioeconomic conditions (Schwilch et al., 2015; Smart SOIL, 2015; Sanz et al., 2017). In this context, broadening the spectrum of scientific research is very essential to cover various environmental (i.e., soil type and climate) and socioeconomic (i.e., funding, market price variations, and type of crop) conditions. In this background, despite the fact that there are numerous studies available worldwide, there is a lack of studies exploring the effectiveness of different conservation tillage practices toward the improvement of soil physical, chemical, and biological properties and crop yield while reducing greenhouse gas emissions and global warming. Too, few studies exist on some selected soil properties and one or two (carbon and nitrous oxide) major greenhouse gas emissions. Studies on greenhouse gas (carbon, methane, and nitrous oxide) emissions, wheat yield, and soil properties (physical, chemical, and biological) responses to different conservation tillage systems on the Loess Plateau, particularly at the Dingxi Belt, are scarce in spring wheat mono-cropping conditions and abundant.
This research tested the hypothesis that conservation tillage practices CTS, NT, and NTS in spring wheat agroecosystems provide better soil physical, chemical, and biological quality indicators and crop yield and yield-attributing traits and reduce the global warming potential of carbon dioxide, methane, and nitrous oxide compared with conventional tillage system. The overall objective of this research was the exploration of soil and environmental quality-based management practices and parameter identification that are sensitive to disturbance of soil. The specific objectives were (i) to evaluate the influence of conservation tillage systems: stubble incorporation with conventional tillage (CTS), no-tillage (NT) and straw-retention with no-tillage (NTS) in the improvement of numerous soil quality indicators, thereby increasing physical, chemical and biological properties; (ii) to assess the effect of the conservation tillage strategies on spring wheat yield and yield-attributing traits; and (iii) to quantify the impact of the conservation tillage techniques on greenhouse gas emissions, namely carbon dioxide, methane, and nitrous oxide emission and also the response of greenhouse gas fluxes to variations in environmental variables because of different tillage systems.
2 Materials and methods
2.1 Field site description and history of experiment region
The field experiment, installed in 2016, is situated on a Calcaric Cambisol at the Anjiapo catchment of Loess Plateau in Dingxi under the Department of Soil and Water Conservation Administration, Gansu Province, Northwestern China (35°34′53′′ N, 104°38′30′′ E), as shown in Figure 1. The study site relief is gently sloped, and the altitude is 2,000 m above sea level. The research county has semi-arid climatic conditions. The type of soil in the study region is a Huangmian sandy loam texture (sand: 60.5%, silt: 24.3%, and clay: 15.2%) according to WRB (IUSS Working Group, 2006), having low soil organic carbon with a slightly alkaline pH (Chinese Soil Taxonomy Cooperative Research Group, 1995).
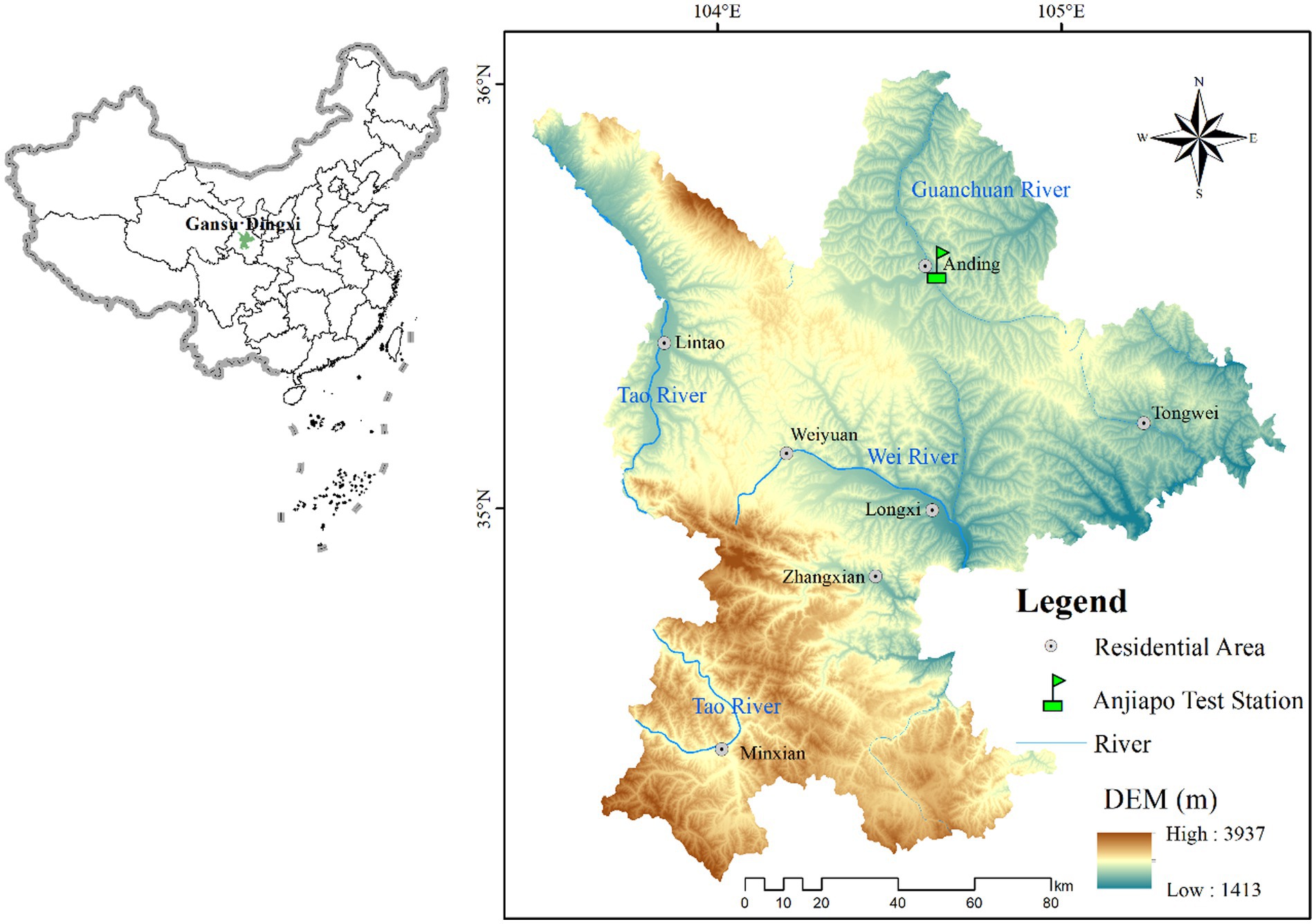
Figure 1. The geographical map of the experimental research site in Dingxi County, Gansu Province, China. ArcGIS 10.2 software was applied for production. The basic geographic information data come from the resource and environmental science and data center (http://www.resdc.cn/).
The average temperatures in this area are −22°C and 35°C in the coolest and warmest months, respectively, and regular frosts in the winter (Xingchen et al., 2019). We have 50 years of continuous climatic data from 1971 to 2020 for this research. The 50-year average annual rainfall from 1971 to 2020 was 400 mm per year, with an irregular distribution. The 50-year average annual radiation is 5,930 MJ m−2 with 2,480 h of sunshine per year. This area has 140 days of frost-free period, an average annual evaporation of 1,531 mm, and an annual temperature of 6.9°C from 1971 to 2020. The monthly average of rainfall and temperature for the study period in 2021 is presented in Figure 2.
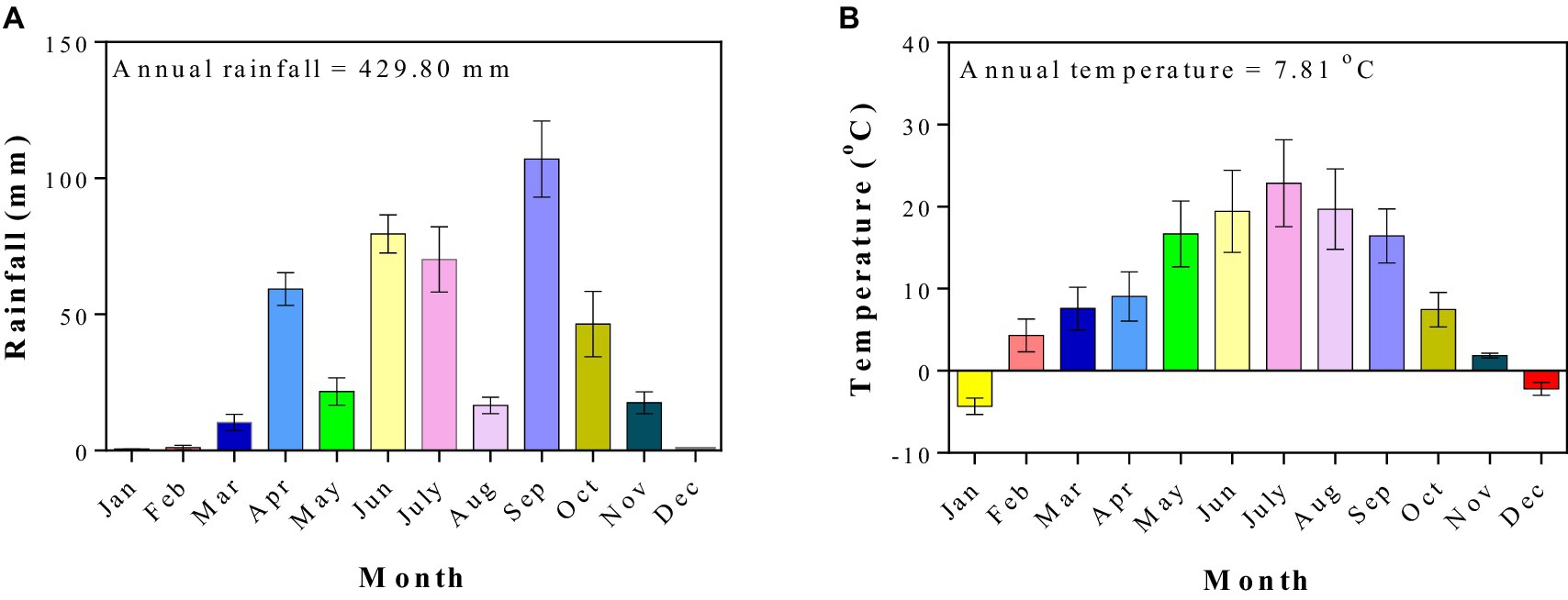
Figure 2. Climatic conditions of the study region in 2021. (A) The monthly average rainfall and (B) the monthly average temperature.
Before the experiment in 2016, the research field area was bare, which was cut and cleared for spring wheat cultivation. Then, spring wheat was introduced and cultivated at different sowing times (early, normal, and late) under tillage systems. The tillage practices were the same for all spring wheat sowing dates. The Dingxi in Gansu, China, is a research hub and has an extensive wheat cultivation history, and wheat straws were frequently removed prior to the subsequent crop cycle. A comprehensive experiment field site description has been provided in previous studies (Niu et al., 2016; Xu et al., 2020; Sadiq et al., 2021a).
2.2 Experimental design
This experimental study was conducted in 2021, from March to August. The research comprised four treatments, including one conventional tillage (CT) control and three different conservation tillage regimes, specifically stubble incorporation with conventional tillage (CTS), no-tillage system (NT), and straw-return with no-tillage (NTS). The conservation tillage regimes were compared with conventional tillage control. The experimental treatments were replicated three times in an 8 m × 3 m plot size under randomized complete block design (RCBD), giving a total of 12 individual plots with a total area of 24 m2. These conservation tillage regimes have been practiced since 2016, with 5 years of non-stop spring wheat cultivation. At the beginning of the research, soil samples contained an average bulk density (BD) of 1.41 ± 0.02 g cm−3, soil porosity (P) 47.05 ± 3.31%, total nitrogen (TN) 0.59 ± 0.02 g kg−1, total phosphorous (TP) 0.43 ± 0.03 g kg−1, total potassium (TK) 18.48 ± 0.01 g kg−1, soil organic carbon (SOC) 5.85 ± 0.34 g kg−1, and pH 8.34 ± 0.05 in 0–15 cm depth (Table 1).
2.3 Tillage systems and crop management practices
The soil tillage management practices compared in this trial were conventional tillage (CT), stubble incorporation with conventionally tilled soil (CTS), no-tillage (NT), and no-till with straw-retention (NTS). Descriptions of these tillage treatments are presented in Table 2. For the CT system, land cultivation was performed three times with moldboard plow in a year at 20 cm, 10 cm, and 5 cm, respectively, and harrowed two times, followed by planting in the absence of stubble. In order to manage CTS, after harvesting the wheat crop, the fields were plowed with moldboard plow, harrowed, and followed by planting exactly as for the CT practice (three plow passes and harrows two times), but stubble incorporation was done at the time of first plowing. After threshing, all the wheat-straw from the earlier spring wheat crop was returned to the original plot instantly and then incorporated into the field. The spring wheat was planted exactly as in the CT system. In the NT-treated plots, after harvesting the spring wheat, all stubbles were removed and crop planting was performed with a no-tillage crop planter; nevertheless, in the NTS practice, after harvesting the wheat, all stubble was returned to the field and crop sowing was done with a no-tillage crop planter. The wheat-stubble chemical composition is shown in Table 3. The wheat-straw nitrogen, phosphorous, potassium, and carbon were 0.79 ± 0.2%, 0.08 ± 0.01%, 0.49 ± 0.04%, and 39.24 ± 2.8%, respectively. Nitrogen at the rate of (146 kg ha−1) and phosphorous at the rate of (63 kg ha−1) were applied as basal doses in all treatments, counting control in the form of diammonium phosphate and urea. Semi-arid Dingxi in the Loess Plateau zone of China has a suitable concentration of soil potassium (Li et al., 2014), which was satisfactory for encouraging wheat growth and germination; therefore, potassium was not applied in basal fertilization. The spring wheat (cultivar Dingxi 42) crop was sown by hand in mid-March (seed rate was 187.5 kg seeds ha−1) and allowed to grow until late-August (Table 3). The wheat crop was sown using 20 cm row-to-row spacing and a plant density of 400 plant m−2. In order to control the weeds, the red sun herbicide with glyphosate (30%) was used according to the manufacturer’s instructions, and when weeding was required during the growing season, it was done manually.
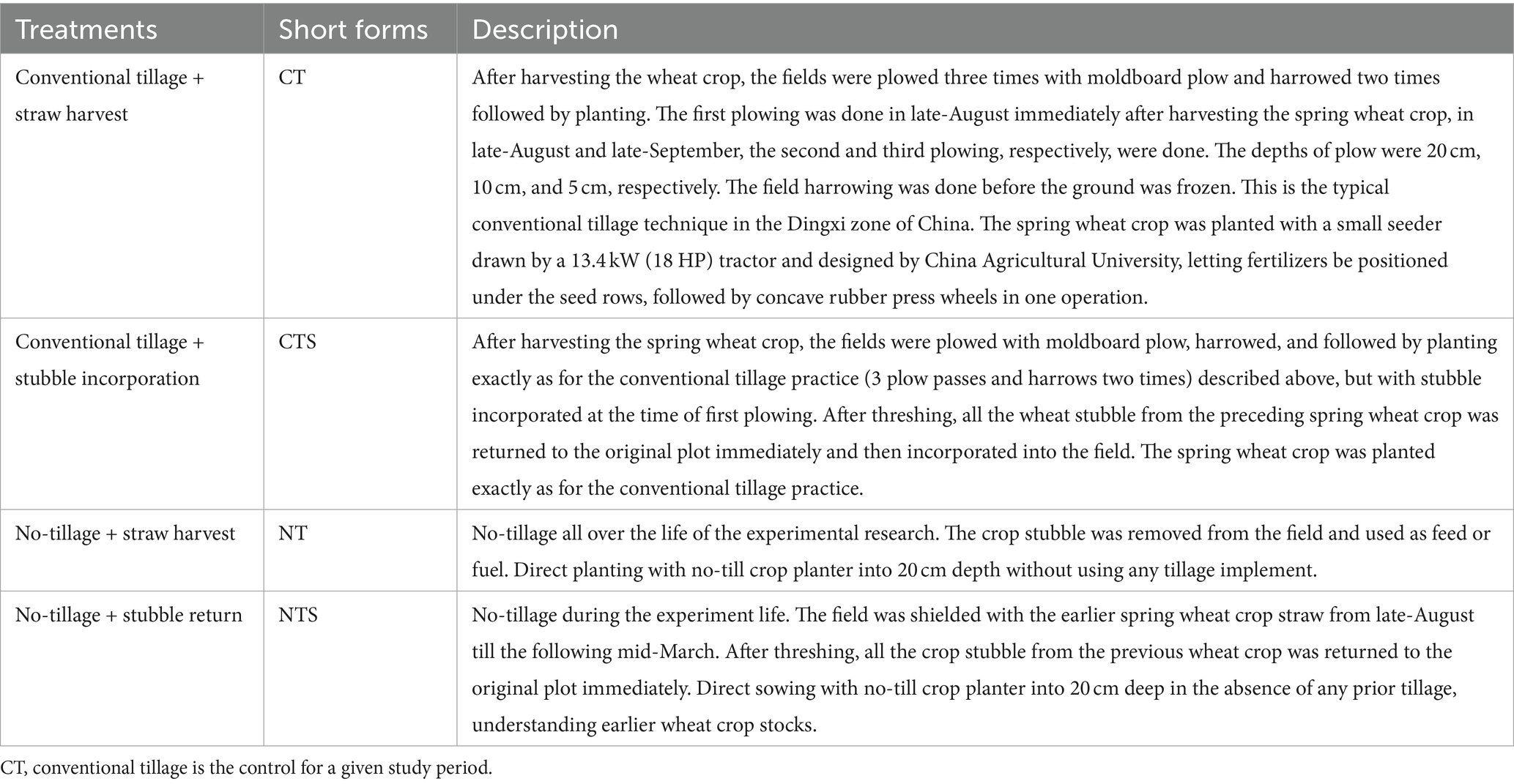
Table 2. Treatment details of tillage regimes and stubble application for spring wheat cultivation tested during the course of this study.
2.4 Agronomic traits
The agronomic attributes of plant height, spike number per plant, seed m2, root yield, aboveground biomass yield, grain yield, 1,000-grain weights, dry matter yield, and harvest index were determined. The wheat plant height was measured using the procedure described by Dokuyucu et al. (2002) and Demuner-Molina et al. (2014).
2.5 Soil measurements
At the spring wheat harvest stage in 2021, five disturbed and undisturbed soil samples were collected with an auger having a diameter of 4 cm from different experimental treatments (CT, CTS, NT, and NTS), including three replications for the determination of soil physicochemical, biochemical, and biological properties. All soil quality indicators were observed at a soil layer of 0–10 cm depth.
The oven-dry method was used for the determination of gravimetric soil water content (SWC) (O’Kelly, 2004), water-filled pore space (WFPS) in percent, and soil water storage (SWS), which was calculated as described by O’Kelly (2004). Soil temperature (ST) was measured using a geothermometer (Lu, 1999). The procedure described by Campbell (1994) was used for soil bulk density (BD) determination with the core sampler method. and soil pore space (P) percent was calculated in accordance with the procedure described by Campbell (1994). The wet-sieved method was used for measurements of soil aggregates (Yang et al., 2018). Auger-hole method using the Guelph Permeameter was used for the determination of saturated soil hydraulic conductivity (Ks) (Reynolds et al., 2008).
The soil organic carbon (SOC) was determined by the standard method of Walkley-Black dichromate oxidation (Nelson and Sommers, 1983), while the density fractionation approach was used for the isolation of light fraction organic carbon (Gregorich and Ellert, 1993), and C and N analyzer (Elementar Vario MACRO cube) was used for its determination. The calculation of soil organic carbon storage (t hm−2) was done using the procedure described by Wu et al. (2021). The estimation of microbial biomass carbon (MBC) and microbial biomass nitrogen (MBN) was done by the fumigation-extraction method (Vance et al., 1987).
Soil total nitrogen (TN), total phosphorous (TP), total potassium (TK), available nitrogen (AN), available phosphorous (AP), available potassium (AK), electrical conductivity (ECe), and pH were determined by using standard procedures (Lu, 1999). Nitrogen storage was calculated, followed by the equation of Wu et al. (2021). Soil microbial propagules (colony forming units, CFUs) were determined using the enumeration of luminescent colonies on agar media (Li et al., 1996). Soil urease activity was estimated following the procedures by Dick and Burns (2011), soil alkaline phosphates were estimated as described by Zhao and Jiang (1986), soil invertase activity was determined by the method of Frankeberger and Johanson (1983), soil cellulase activity was estimated following the method of Guan (1986), and soil catalase activity was determined as described by Yan (1988).
2.6 Gas sampling and flux measurement
The procedure of greenhouse gas sampling, such as CO2, CH4, and N2O, was conducted during different crop growth stages of spring wheat in 2021. On the basis of the static dark chamber and gas chromatography method described by Yuesi and Yinghong (2003), gas sampling and flux measurements were done. A stainless-steel base with a collar (50 × 50 × 10 cm) was installed to support sampling chamber placement (50 × 50 × 50 cm) for greenhouse gas sampling in each plot (a total of 12 plots). The samples of air were drawn from the chambers simultaneously, including three replications of each treatment. At five different times (0, 9, 18, 27, and 36 min), respectively, by using 150 mL gas-tight polypropylene syringes, the air samples were drawn and released into 100 mL aluminum foil sampling bags (Shanghai Sunrise Instrument Co. Ltd., Shanghai). Then the samples of gas were analyzed in the laboratory with a GC system (Echrom GC A90, China) equipped with a flame ionization detector for methane and carbon dioxide and electron capture detector for nitrous oxide analysis. At 250°C temperature and 35 cm3 min−1 H2 flow rate, the flame ionization detector operates. Methane, carbon dioxide, and nitrous oxide peak areas were analyzed in the Echrom-ChemLab software. Calibrations were done with standard gas obtained from Shanghai Jiliang Standard Reference Gases Co., Ltd., China, before the sample gas analyses. Standard gas concentrations were 2.00 ppmv, 456.00 ppmv, and 0.355 ppmv for methane, carbon dioxide, and nitrous oxide, respectively. In order to obtain the concentration change over sampling time, the concentrations of the sample gas obtained for the five sampling times were plotted against time. Emissions of carbon dioxide in terms of ecosystem respiration, methane, and nitrous oxide fluxes were calculated as described by Wei et al. (2014), followed by equation (1):
In order to estimate global warming potential (GWP) of greenhouse gases, the cumulative flux of CO2 in µmol m−2s−1 was converted to mg CO2–C and typically taken as the reference gas; therefore, methane and nitrous oxide emissions are converted into “CO2 equivalents” (CO2-e). The methane GWP is 34 (based on a 100-year time horizon), the carbon dioxide GWP is 1, and the nitrous oxide GWP is 298 (IPCC, 2013). Net global warming potential (GWP) in kg CO2-eq ha−1 was determined by using equation (3):
Greenhouse gas intensity (GHGI) was determined by using equation (4):
The sign convention adopted is that positive (+) means emission, whereas negative (−) means absorption.
2.7 Statistical analysis
The experimental data were processed using Excel 2016 (Microsoft Corp., United States). The procedure used to analyze the data obtained from the experiment was one-way factor interaction analysis of variance (ANOVA), suitable for randomized complete block design (RCBD). The linear model procedure of the appropriate computer software program Statistical Package for Social Science (SPSS) window version 25.0 (IBM Corp., Chicago, IL, USA) was used for all statistical analysis. The significant differences among different treatments were separated by Duncan’s test at 5% significance level (p < 0.05). Data obtained from treatments are displayed as the mean of three replications with standard deviation and computer software Origin 2021 was used for drawing figures. Additionally, in order to explore the multivariate variability introduced by the various tillage practices for soil properties, crop yield, and greenhouse gases, the principle component analysis (PCA) was performed. The Pearson heatmap correlation analysis was used to describe the relationship among the factors.
3 Results and discussion
3.1 Effect of conservation tillage on wheat agronomic traits
Conservation tillage management had an influence on spring wheat agronomic features, including growth, yield, and yield-attributing traits. The NTS practice generated maximum 95.26 ± 3.08 cm plant height, 44.66 ± 5.12 number of spikes per plant, seeds number per meter square 9321.97 ± 623, root yield (RY) 532.88 ± 24 kg ha−1, aboveground biomass yield (ABY) of 5225.31 ± 327 kg ha−1, thousand-grain weight (TSW) 50.95 ± 2.02 g, grain productivity (GY) of 2543.88 ± 275 kg ha−1 and 2681.43 ± 257 kg ha−1 dry matter yield (DMY) while lowest values of all investigated agronomic characters were associated with CT technique. The agronomic parameters of wheat followed the trend of CT > NT > CTS > NTS, except for plant height and spike number per plant, where no-till depicted better performance over CTS. However, CT produced pointedly highest 48.76 ± 1.33% harvest index (HI) compared with other tested conservative tillage treatments (Table 4). This research verified our hypothesis that a conservation tillage technique can improve productivity; it would be a sustainable and reliable agronomical practice in dry regions.
Soil inversion elimination and crop stubble implementation are the most significant practices in the agriculture production system. The data reveal that different conservation tillage systems are suitable for producing spring wheat in the semi-arid Dingxi belt. The significant maximum agronomic trait values of conservative tillage systems indicate the competent use of stubbles (Zhao et al., 2019; Jat et al., 2021; Li et al., 2023), water, and nutrients. The better wheat agronomic performance was due to straw-retention because crop stubble has the potential to retain essential soil nutrients, which led to increased crop agronomic parameters (Han et al., 2020). This is consistent with previous results (Sadiq et al., 2021b), in which they recorded increased crop yield under conservation agricultural practices and associated it with residue retention. The higher wheat yield and yield-attributing traits under NTS, CTS, and NT systems than CT can further be ascribed to increased soil organic matter, which improves soil quality and leads to increased crop agronomic parameters (Sadiq et al., 2021a). Maximum crop production under straw-return and residue incorporation and the NT system over CT might also be due to better hydrothermal soil characteristics (Huang et al., 2012; Wang et al., 2017). Our current study results are in agreement with the findings of Alhassan et al. (2021), as they verified that stubble return and residue incorporation and no-till systems enhanced wheat yield compared with CT in a dry region farming system.
3.2 Soil properties as influenced by conservation tillage management practices
Conservation tillage systems have noteworthy impact on soil properties, for instance, soil water content (SWC), water-filled pore space (WFPS), soil water storage (SWS), soil temperature (ST), soil bulk density (BD), soil porosity (P), aggregates, soil saturated hydraulic conductivity (Ks), soil organic carbon (SOC), light fraction organic carbon (LFOC), carbon storage (CS), microbial biomass carbon (MBC), total nitrogen (TN), available nitrogen (AN), nitrogen storage (NS), microbial biomass nitrogen (MBN), total phosphorous (TP), available phosphorous (AP), total potassium (TK), available potassium (AK), microbial counts (MC), urease, alkaline phosphatase, invertase, cellulase, and catalase at a soil depth of 0–10 cm (Figure 3 and Table 5). The concentration of SWC (p < 0.05) was maximum (10.35 ± 0.64%) in the NTS treatment variant with two other CTS and NT conservative tillage treatments, while CT had a minimum (8.70 ± 0.40%) SWC value. The conservation tillage practices NTS, CTS, and NT increased SWC by 9.35, 9.13, and 9.05%, respectively, over the CT system. The WFPS was highest (20.80 ± 0.81%) under the NT system, which was followed by NTS and CTS techniques, while the lowest WFPS was associated with CT practice. Additionally, the same trend was observed in the case of SWS, with the highest value in NT and the lowest in CT. The rise in SWC, WFPS, and SWS under CTS and NTS measures may be owing to crop straw-retention, thus decreasing evaporation losses, improving water availability and infiltration rate, and conserving soil water (Yadav et al., 2018; Zhang et al., 2018). Additionally, crop stubble addition to soil increases SOM and thus improves soil water retention capacity. The increase in SWC, WFPS, and SWS under NT practice over the CT system might be due to the earth flip inherent to CT practice, resulting in a big water loss due to evaporation. This water loss is avoided in NT, which lacks the requirement for stubble addition (Liebhard et al., 2022). Parallel findings were found by Yuan et al. (2023a).
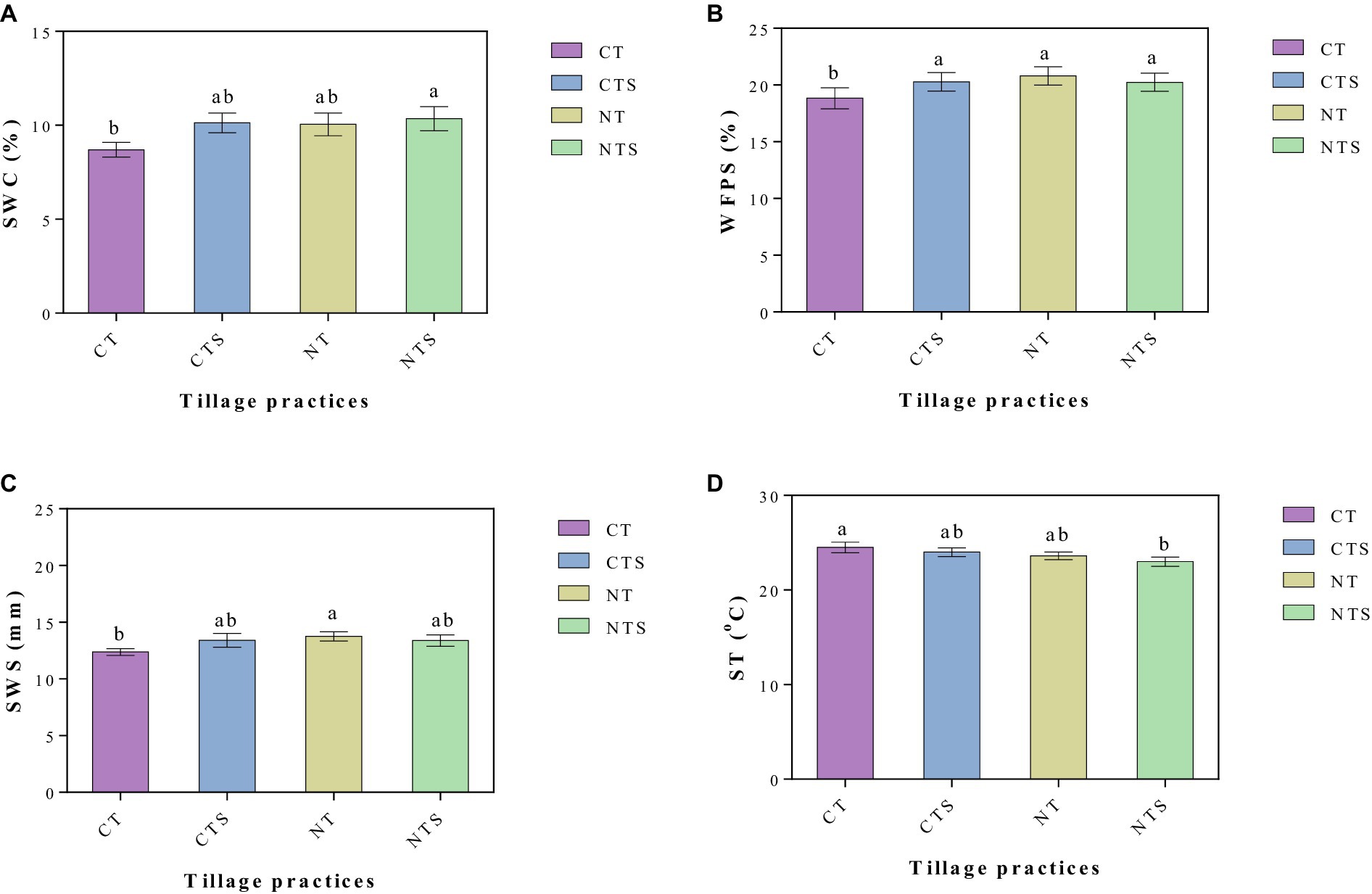
Figure 3. Properties of soil under conservative tillage systems in 2021 at 0–10 cm soil depth. (A) The soil gravimetric water content influenced by conservation tillage technique; (B) the water-filled pore spaces under conservation tillage; (C) the soil water storage affected by tillage practices; and (D) the soil temperature affected by tillage measures. Vertical error bars denote the corresponding standard error of mean values; n = 3. Significant differences were determined by a Duncan’s test.
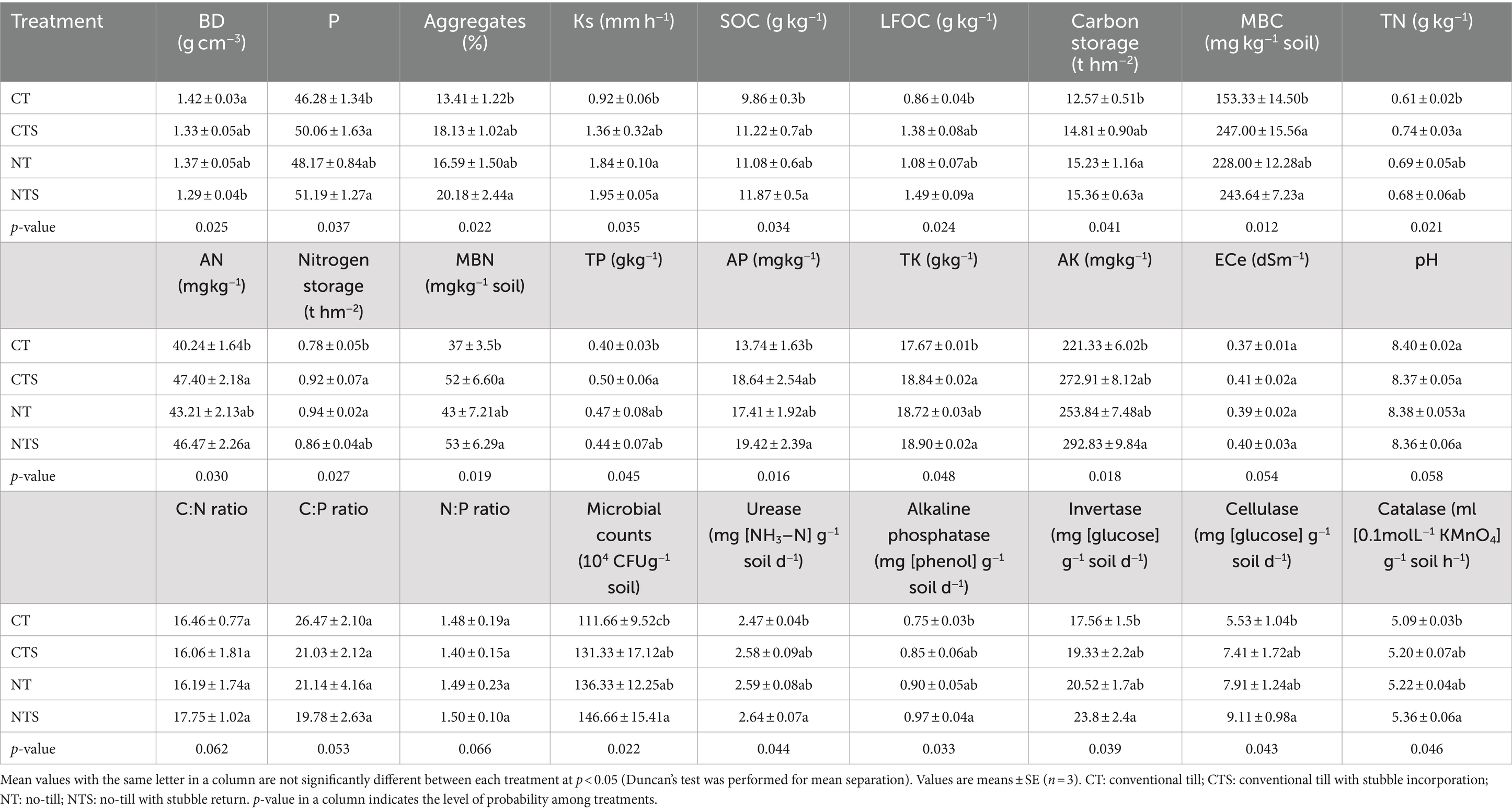
Table 5. Changes in soil properties under sustainable conservation tillage in 2021 at 0–10 cm soil depth.
The CT had the highest ST, whereas the NTS had the minimum ST values. The temperature moderation influence was observed in conservative tillage treatments as decreased ST by NTS, NT, and CTS systems (Figure 3). This is due to the accumulation of crop straws. The surface of the soil is abstemiously shielded by stubble remnants from prior crops in conservative tillage systems, encouraging the soil to absorb minimum solar radiation (Wang et al., 2009). Furthermore, the CT practice makes the soil more porous, and therefore, the soil under CT possibly has minor thermal conductivity (Sarkar and Singh, 2007). In addition, conservative tillage treatments increased the infiltration rate, which helped water movement toward the bottom (Liebhard et al., 2022) and reduced ST (Yuan et al., 2023a). Similar observations were noted by Sadiq et al. (2021a).
On average, conservation tillage systems NTS, CTS, and NT reduced BD by 29, 33, and 37%, respectively, over CT. The maximum soil BD under CT was connected to the soil disturbance by tillage implementation, which resulted in soil compactness (Gathala et al., 2011). In addition, due to traffic physical compaction by direct heavy machinery (Jat et al., 2018). In addition, as BD values are connected with soil porosity values, these conservative tillage systems improved the p-values, with the highest under NTS and the lowest recorded in CT. The NTS, CTS, and NT increased the soil porosity because of the organic matter accumulation under conservation tillage, which led to decreased BD and improved p-values (Ordoñez-Morales et al., 2019). Similar results were noted by Khorami et al. (2018), who recorded that conservation tillage decreased the BD and increased pore space over CT.
Almost similar to SWC, NTS attained the highest soil aggregates, followed by CTS, which was statistically on par with NT, while CT linked with the lowest soil aggregates. The CT strongly disturbs the soil due to plowing, which can diminish the aggregate degree and aggregate stability (Yeboah et al., 2018). Higher aggregates in conservation tillage might be due to residue retention (Niu et al., 2016). Similar results are reported in accordance with (Yuan et al., 2023a). Additionally, conservation tillage practices increased the Ks over CT; the highest value of Ks was reported in NTS, which was statistically at par with NT, followed by CTS, while the lowest Ks value was associated with CT. The minimum soil Ks in CT and CTS might be ascribed to the destruction of soil aggregation and reduction of macro-porosity (Singh et al., 2002). In arid and semi-arid cropping systems, the soil property variations under conservation tillage influences are habitually slow to arise, attributable to the limited production of plant biomass (Mikha et al., 2006).
The SOC, LFOC, CS, and MBC differed significantly (p < 0.05) in all tested tillage systems, whereas the non-significant (p < 0.05) differences were noted among CTS and NT for SOC and LFOC as well as NT and NTS had also non-significant differences for carbon storage, and in case of MBC, the NTS and CTS are statistically at par (Table 5). The NTS system had the highest values of SOC, LFOC, and carbon storage except for MBC, where CTS was related to higher values, while the CT system had the lowest values. The SOC, LFOC, carbon storage, and MBC contents were low in the CT system because of extensive tillage operations, breakdown of aggregates, and exposure of the soil carbon contents. More values of carbon-associated parameters in CTS, NTS, and NT systems soil were attributable to residue retention and no soil physical disturbance (Chen et al., 2009). In conservative tillage systems, the SOC solid stratification, which was significantly attributable to surface residue retention, is identical to that in other studies (Blanco-Canqui and Lal, 2008; Yuan et al., 2023b). Soil carbon storage is the retention of carbon in the ecosystem and a chief index to measure the gauge and quantity of ecosystem primary productivity. A study by Yeboah et al. (2018) revealed that NTS can significantly increase the soil organic carbon content so as to progress soil carbon storage. This study also found that compared with CT, conservation tillage systems were more helpful in improving soil carbon storage. The higher values of MBC appear because of high microbial biomass and soil organic matter contents. Organic stubbles are used as carbon source inputs for the microorganisms’ activities, activation with the support of microbial biomass, conservation tillage systems enrich the soil carbon contents (Liu et al., 2017). The role of straw-retention and residue incorporation in increasing carbon elements was also stated by numerous scholars (Zhao et al., 2019; Sadiq et al., 2021a; Yuan et al., 2023b). The conservation tillage system is a straw addition or plowless tillage with a minimum number of tillage operations, and its beneficial impact on soil functions and quality has been extensively identified (Han et al., 2020; Wulanningtyas et al., 2021; Sadiq et al., 2021b).
Conservation tillage practices (NTS, CTS, and NT) significantly improved total and available soil nutrients, namely TN, AN, TP, AP, TK, and AK, compared to CT. In addition, NC and MBN were notably increased under these investigated conservative tillage systems over CT. Higher TN, AN nitrogen storage, and TP were related to CTS but the NTS system had dominant MBN, AP, TK, and AK values, while CT had the lowest values. Beneficial influence of NTS, CTS, and NT on nutrient accumulation and storage might be due to multiple reasons:
i. Higher total and available soil nutrients, for instance, nitrogen, phosphorous, and potassium in CTS and NTS, might be because of straw-retention because straw has the potential to add essential nutrients to the soil system (Zhao et al., 2019).
ii. Maximum nitrogen accumulation under straw-treated treatments may be attributed to straw microbial biomass and nitrogen immobilization (Huang et al., 2012).
iii. Stubble retention and incorporation can decrease leaching and volatilization losses of nitrogen by diminishing the soil temperature and lead to increased nitrogen accumulation (Sadiq et al., 2021a).
iv. Highest nitrogen accumulation under conservative tillage treatments (CTS, NT, and NTS) may also be due to better biological activity (Wang et al., 2017).
v. Straw can increase organic matter and lead to improved phosphorous solubilization and fixed potassium availability by reducing phosphorous adsorption to mineral surfaces (Celik et al., 2011) and potassium adsorption to clay mineral surfaces (Celik et al., 2011).
Our findings concur with those of Zhao et al. (2019), Han et al. (2020), Sadiq et al. (2021a), Wulanningtyas et al. (2021), Lv et al. (2023), who found that nutrient elements and their storages were higher under conservation tillage than plowed ones.
The ECe, pH, C:N ratio, C:P ratio, and N:P ratio under different conservation tillage measures were inconsistent and non-significant. However, CTS, NT, and NTS showed a trend of increased ECe and decreased pH compared with CT. The dropping of pH under conservative tillage was previously described by numerous scholars (Jat et al., 2018; Sinha et al., 2019). Soil pH reduction in CTS, NT, and NTS conservation-based tillage regimes may be owed to organic acid production during retained stubble decomposition (Sinha et al., 2019). The C:N ratio was lower in CTS and NT than in CT, which might be due to slower decomposition under these systems. Our results are in line with those (Jat et al., 2018), which pointed out the minor values of C:N ratio under conservative tillage over CT. In general, the soil C:P and N:P ratios were highest under CT and NTS treatment, respectively. These results are in agreement with Feng et al. (2014), who stated that NT practice coupled with straw increases the soil N:P ratio.
Conservation tillage system had a great influence on soil microbial count and enzymatic activities. The highest values of soil microbial count, urease, alkaline phosphatase, invertase, cellulase, and catalase were obtained under NTS treatment, followed by CTS and NT, while the CT system had the lowest values of all these parameters. The conservative tillage systems NTS, CTS, and NT increased microbial count by 31.34, 17.61, and 21.19%, respectively; urease activity by 6.88, 4.45, and 4.85%, respectively; activity of alkaline phosphatase by 29.33, 13.35, and 20%, respectively; invertase activity by 35.53, 10.07, and 16.85%, respectively; cellulase activity by 64.73, 33.99, and 43.03%, respectively; and catalase activity by 5.30, 2.16, and 2.55%, respectively, over CT. The microbial count and enzyme activity depicted the trend of CT < CTS < NT < NTS. The soil microorganisms are a significant portion of the cropland ecosystem and are involved in the decomposition of SOM, nutrient circulation in the soil system, humus formation, and soil fertility (Yu, 2015). Soil microbes’ stability is a vital indicator of soil quality, health, and fertility (Yang et al., 2023). Land cultivation methods and cropland management practices affect the soil microbial community. Conservation tillage has the potential to improve the soil microbial community and the activities of soil enzymes (Rakesh et al., 2021). This study depicts that NTS, CTS, and NT raised the microbial community and activities of urease, alkaline phosphatase, catalase, invertase, and cellulase in the soil system to a greater extent than the CT system. This shows that conservative tillage mechanically makes the soil more porous (Yang et al., 2018) and increases the SOM distribution while facilitating the activity of enzymes (Balota et al., 2004). Hence, stubbles coupled with no-tillage (NTS) improved the soil microbial count, urease, alkaline phosphatase, invertase, cellulase, and catalase, which improved crop germination, growth, and yield. Optimal soil physicochemical properties could increase enzyme activity (Wei et al., 2013). In this context, NTS, CTS, and NT treatments were more effective than CT control treatment. Measurements of soil characteristics and variations are crucial for evaluating the influence of soil management techniques (Jat et al., 2021). The improved enzyme activities may be because of augmented carbon sequestration and immobilization of carbon as well as nitrogen during the decomposition of residues, as numerous studies have revealed that the activities of soil enzymes can be influenced by SOC sequestration (He et al., 2020; Pu et al., 2020). Our results are in accordance with Bhattacharya et al. (2020).
Furthermore, the principal component analysis (PCA) in accordance with the Jolliffe cutoff value permits isolating five principal components. In PCA analysis, the observation point made by contact between PC1 and PC2 illustrates the general variance defined by the five chief components. The PCA analysis of 39 variables (namely SWC, WFPS, SWS, ST, BD, P, soil aggregates, Ks, SOC, LFOC, CS, MBC, TN, AN, NS, MBN, TP, AP, TK, AK, ECe, pH, C:N ratio, C:P ratio, N:P ratio, MC, urease, alkaline phosphatase, invertase, cellulase, catalase, plant height, root yield, number of spikes per plant, number of seeds per meter square, grain yield, aboveground biomass yield, dry matter yield, harvest index), PC1 and PC2 were extracted with eigenvalues >1 and explained 65.7% of the total variance. Nevertheless, PC3, PC4, and PC5 do not allow the addition of additional information; that is why they are not involved. The highest PC1 loadings encompassed 50.4% of the total variance, and in PC2, the maximum loadings of 15.3% of the total variance were detected (Figure 4). According to our expectations, the correlation between wheat seed productivity and yield-attributing traits was significantly positive. Moreover, a significant positive correlation was noted between soil hydraulic properties, soil nutrients, and enzymatic activities and the seed yield of spring wheat (Figure 5). This concurs with the results reported by Yuan et al. (2023a).
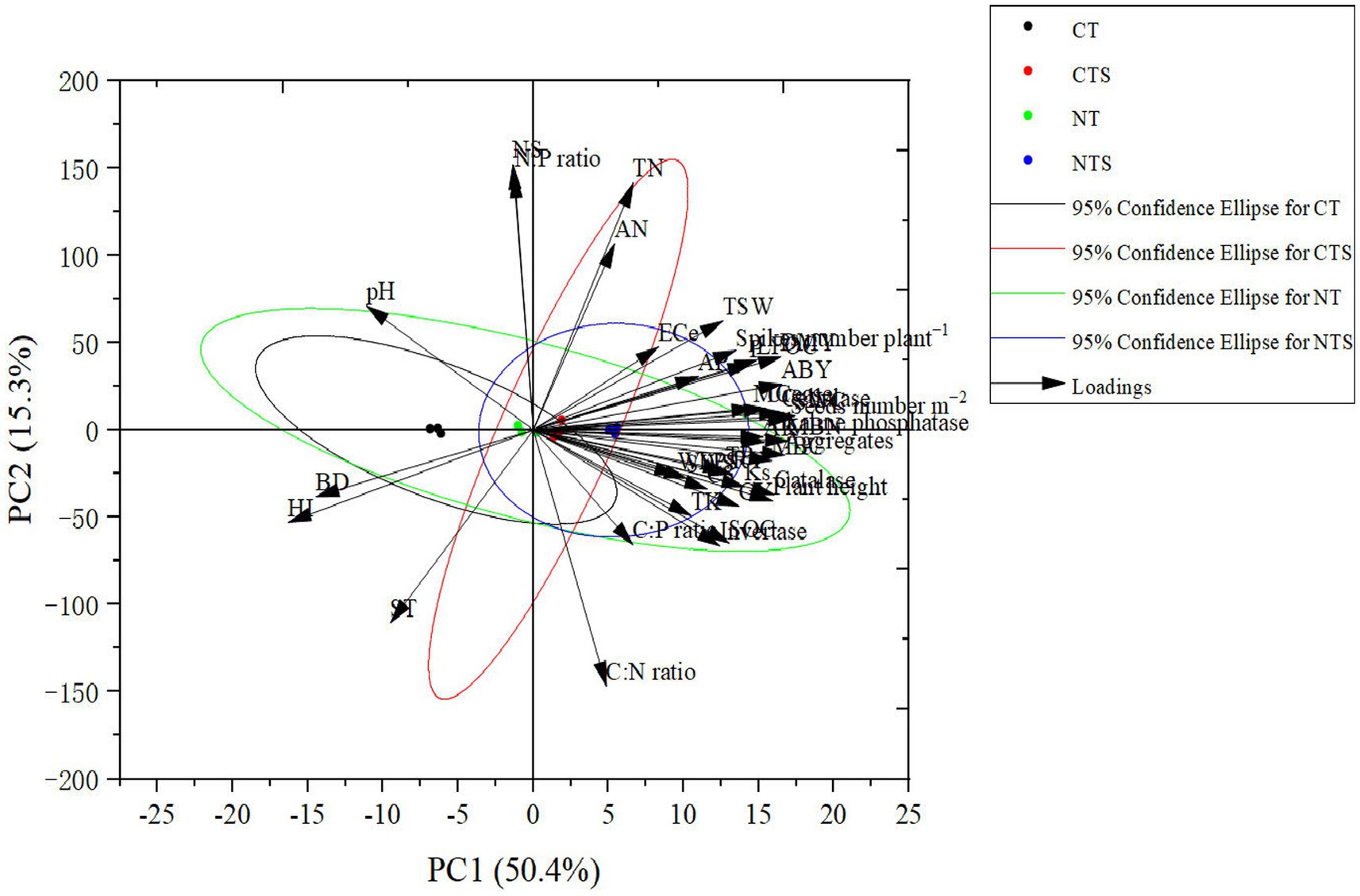
Figure 4. Principal component analysis of spring wheat agronomic attributes and soil properties (physical, chemical, and biological).
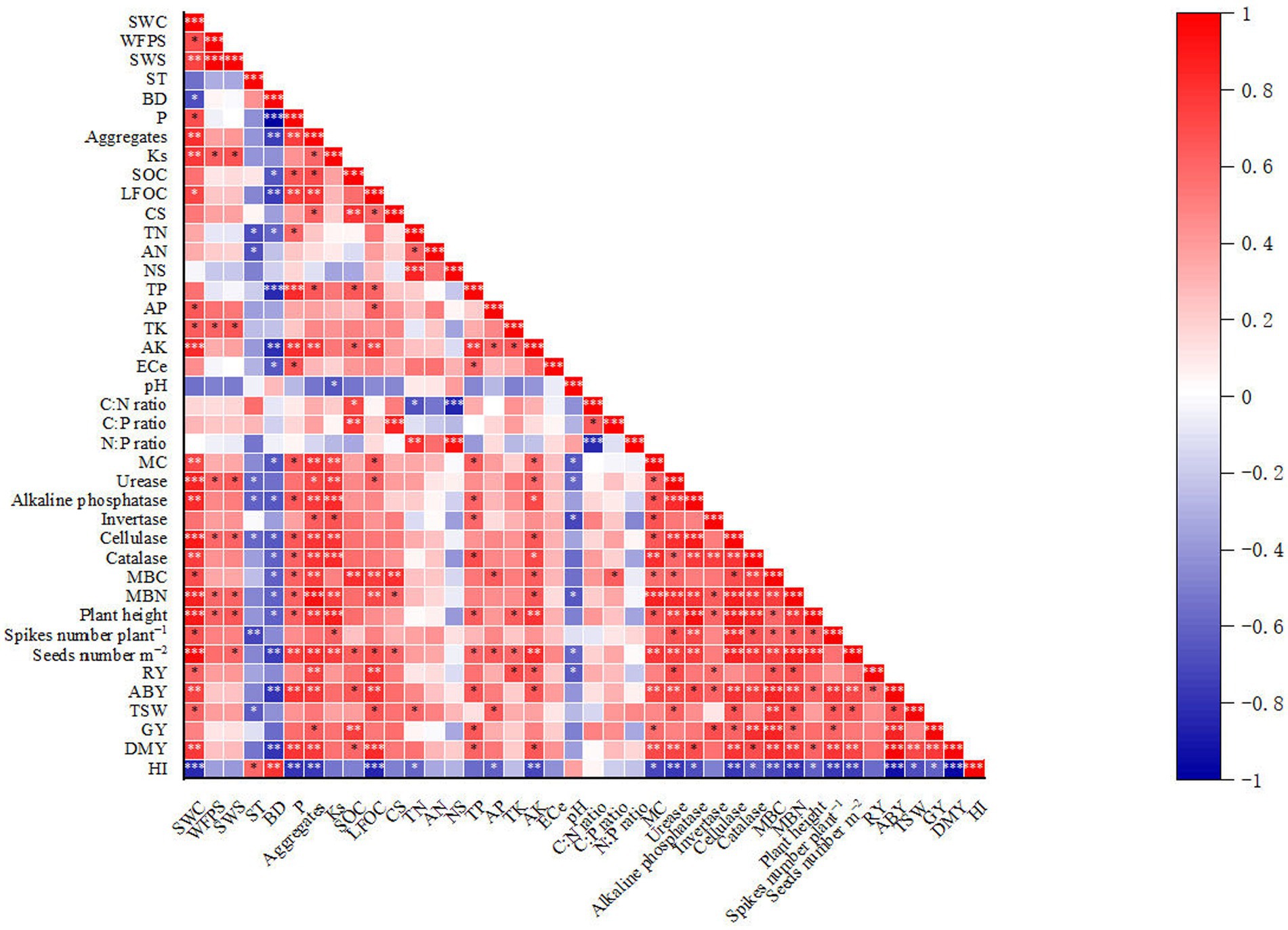
Figure 5. Heat map correlation study of wheat agronomic traits and soil physical, chemical, and biological properties. Indicates significance at: *p < 0.05, **p < 0.010, and ***p < 0.0010. Note: the abbreviated words stand for SWC = soil gravimetric water content; WFPS = water-filled pore space; SWS = soil water storage; ST = soil temperature; BD = soil bulk density; P = soil porosity; Ks = soil saturated hydraulic conductivity; SOC = soil organic carbon; LFOC = light fraction organic carbon; SC = carbon storage; TN = total nitrogen; AN = available nitrogen; NS = nitrogen storage; TP = total phosphorous; AP = available phosphorous; TK = total potassium; AK = available potassium; ECe = soil electrical conductivity; pH = soil pH; MC = soil microbial counts; MBC = microbial biomass carbon; MBN = microbial biomass nitrogen; RY = wheat root yield; ABY = wheat aboveground biomass yield; TSW = thousand seed weight; GY = wheat grain yield; DMY = dry matter yield; HI = harvest index.
3.3 Greenhouse gas fluxes and drivers of GHG emissions
Significant variation in GHG (CO2, CH4, and N2O) was observed under different tillage regimes (Figure 6). The efflux rates of CO2 (representing ecosystem respiration) under conservation tillage depicted apparent seasonal variations (Figure 6A). The maximum emissions arising during the summer months peak in the CT system in July, at the grouting spring wheat crop growth stage. The CT and CTS treatments showed the highest ecosystem respiration rates, including all investigated crop growth stages, while the NTS and NT treatments had the lowest CO2 emission rates, but a significant difference between the different treatments was observed in bloom, grouting, maturity, and harvest stages. The highest CO2 efflux rates during the summer months correspond with the maximum ST and dry matter accumulation, hence augmented soil respiration (Ussiri et al., 2009). Wu et al. (2011) clarified that an increase in soil temperature from 5°C to 15°C led to a noteworthy increase in the emission of carbon dioxide because of high soil organic matter mineralization and improved soil microbial activity. Lowering of soil CO2 efflux rates during the growing seasons under conservation tillage was previously reported by many researchers (Alhassan et al., 2021; Li et al., 2023). Al-Kaisi and Yin (2005) stated a huge 80% reduction in CO2 efflux rates under the NT compared to the CT system. Average CO2 efflux rates were higher in tilled soils compared with non-tilled soils. The average CO2 efflux rates were 180 ± 14.2, 171 ± 25.8, 245 ± 8.8 and 260 ± 15.3 mg Cm−2 h−1 in NTS, NT, CTS and CT respectively (Figure 4B). A significant positive correlation of CO2 emission among soil moisture and soil temperature and a negative correlation of CO2 emission with nitrogen and carbon contents and hydraulic conductivity were observed during the current study (Table 6). The CO2 efflux rates are habitually controlled by plenty of factors, including carbon dioxide concentration gradient in the environment and the wind speed, soil water, soil temperature, soil medium, and soil physicochemical properties (Raich and Schlesinger, 1992). The tillage effect on these parameters would affect carbon dioxide emissions as well. The CT causes soil disturbance, which increases rates of decomposition because of improved soil microbe activities (Al-Kaisi and Yin, 2005), leading to maximum emissions of CO2. Quite the reverse, under NTS and NT treatments, decomposition is slower because of no soil disturbance (Curtin et al., 2000). Conservation tillage practices (CTS, NT, and NTS) might correspondingly improve soil properties, which in turn can decrease soil CO2 emissions. Maximum SWC under CTS treatment, combined with maximum ST, produced higher cumulative soil CO2 emissions compared with NT and NTS. The SWC and ST habitually exert a collaborative effect on CO2 emissions (Bond-Lamberty et al., 2016).
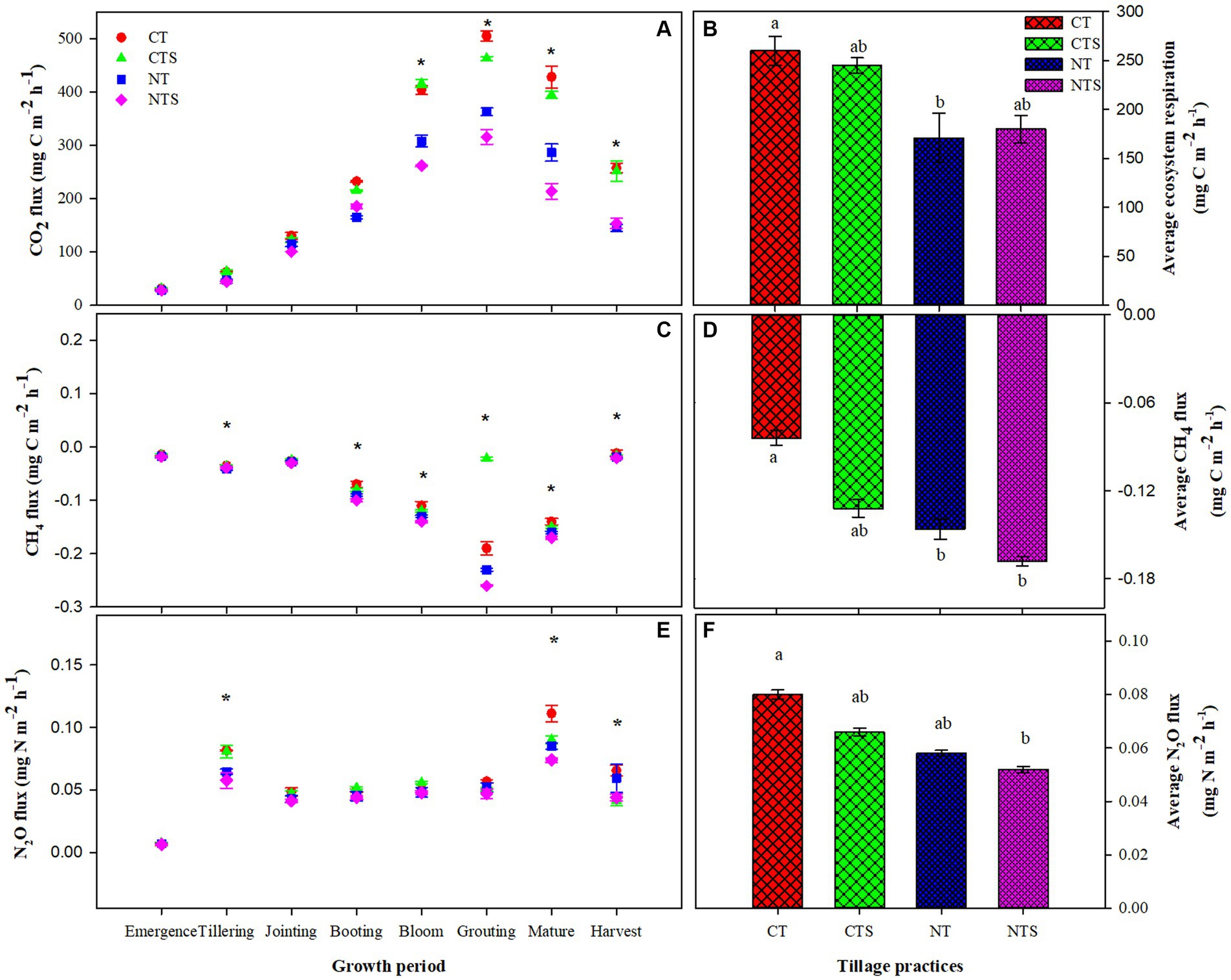
Figure 6. Seasonal and average greenhouse gas emissions under tillage systems in spring-wheat agroecosystem in 2021. Error bars represent the corresponding standard error of mean values; n = 3. Different lower-case letters and ‘*’ indicate significant differences amongst different treatments at p < 0.05 (Duncan’s test performed for mean separation). Note: (A,B) is the seasonal and average ecosystem respiration as affected by the tillage treatments; (C,D) is the seasonal and average CH4 flux under conservation tillage; (E,F) is the seasonal and average N2O flux under tillage practices.
All the tested tillage systems acted as CH4 sinks during the study period (Figure 6C). Peak absorption arose during the summer months at booting and bloom crop growth stages under the NT system. Seasonal changes were recorded in the sink capacities of different tillage systems. The CT had minimum CH4 absorption rates during the investigated crop growth stages. A significant difference between different treatments was observed except for emergence and jointing of spring wheat crop growth stages. The maximum CH4 emissions during the summer months were because of the high SWC status and ST, which may perhaps have boosted the activity of CH4-oxidizing bacteria. The analysis of variance showed that all the investigated tillage techniques, namely CT, CTS, NT, and NTS, had a significant influence on average methane emission. In general, our results showed the average absorption rate of CH4 followed the trend of CT < CTS < NT < NTS (Figure 4D). Another study done by Yeboah et al. (2016) had comparable consequences. Shen et al. (2018) showed that semi-arid agroecosystems habitually act as sinks of CH4 as a result of soil aerobic conditions (Schaufler et al., 2010). Similar to the emission of CO2, the absorption has a significant positive correlation with ST and SWC and a negative correlation with Ks and carbon and nitrogen elements (Table 6). The results of the current study are consistent with the results recorded by Yeboah et al. (2016) and Wang et al. (2022), who stated that improved SWC status can lead to high CH4 uptake owing to the anaerobiosis occurrence and methanogenesis increment. Singh et al. (2010) observed maximum CH4 emission rates under 13% soil water content in sandy loamy soils, and a parallel situation was detected in this study. Our study depicted that the methane flux was constantly negative, signifying CH4 uptake by all the tillage systems. The NTS had the highest CH4 uptake in this study. Under NTS treatment, minor ST might have played a substantial role in high CH4 uptake. During high ST, the dominant methanogen (Methanosarcinaceae) employs H2/CO2 and acetate as precursors of CH4 producing and produces far maximum CH4 over the methanogen at lesser temperatures (Methanosaetaceae), which uses solitary acetate as a precursor of CH4 producing (Ding et al., 2003). Our results are consistent with other appraisals in the Loess Plateau of China, where NT with straw-return was found to be a net sink for atmospheric CH4 (Alhassan et al., 2021). The greater methane uptake under the NTS system might be because of good soil aeration and less degradation of the soil, which improved the activity of methanotrophs. McLain and Martens (2006) recorded that the activity of methanotrophs was heightened under tolerable gas diffusion and from the microbial activity sites. The NTS system inclines to increase soil organic carbon and decrease the bulk density of soil, which might lead to lesser CH4 emissions risk. Yeboah et al. (2016) stated that the degradation of soil can diminish the soil’s ability to oxidize or consume atmospheric methane by as much as 30–90%. However, we proved that the most noteworthy CH4 uptake control was SWC at the surface soil depth, with ST and Ks brought about by enhanced soil condition or quality and improved SOC. Soil carbon and nitrogen contents are also negatively correlated with CH4 emission. This obscures the fact that improved soil carbon and nitrogen levels condensed the emission of CH4.
Our study data showed that peak N2O emission occurred in August at the final crop maturity growth stage, while the lowest N2O flux was observed in the spring months. This trend is consistent with the findings stated by Cai et al. (2013) at the identical research site. During most tested crop growth stages, the CT and CTS emit more N2O compared with the NT and NTS treatments, while at the harvest crop growth stage, NT served as a slight emitter and dominated the CTS system. A significant difference between different treatments was observed only at tillering, maturity, and harvest crop growth stages (Figure 6E). The N2O flux pattern depicted that when SWC and ST appeared higher during the summer season, the N2O emissions were higher. These consequences are similar to earlier studies (Bhattacharyya et al., 2023; Bozal-Leorri et al., 2023; Li et al., 2023). The ST is a significant factor influencing seasonal variations of N2O flux. Taghizadeh-Toosi et al. (2022) observed that the variation in N2O flux rate is almost harmonized with the surface temperature of the soil. Zhang et al. (2022) noted that ST increasement can diminish the nitrification contribution to N2O and increase the N2O amount produced during the denitrification process. The highest N2O emissions noted during the rainiest months under all tested tillage systems might be credited to the fact that the spell’s rainfall levels might be higher than normal on that date, making all tillage systems very wet, so that denitrification situations were not dissimilar among them.
Significantly higher N2O fluxes were observed under CT treatment compared with NTS, NT, and CTS treatments. The N2O flux rates followed the trend of NTS < NT < CTS < CT (Figure 4F). On average, all investigated tillage systems served as slight N2O emitters. These results are in accordance with those described by Pokharel and Chang (2021). Quite the reverse, Alhassan et al. (2021) observed maximum N2O fluxes under the NT system over the CT technique. In this study, different conservation tillage practices, for instance, CTS, NT, and NTS, decreased the emission of N2O by increasing soil structure, as revealed by bulk density of soil, pore space, soil aggregates, and soil hydraulic conductivity. Higher N2O emissions under the CT system have been credited to the condensed diffusivity of gas and air-filled pore space (Rabot et al., 2015). Additionally, N2O flux rates recorded in this experimental research were in the range of N2O flux rates noted by Yeboah et al. (2016).
A significant positive correlation was noted between ST and N2O emissions, which is attributed to the microbial respiration augmentation after heating origins oxygen dearth in the soil profile, which generates anaerobic conditions for denitrifying microbes’ activity (Castaldi, 2000; Mehnaz et al., 2018), and all together, warming also rises soil denitrification activity (Braker et al., 2010), which led to a rise in N2O emissions. Additionally, our results also showed positive correlations among SWC and N2O emissions. This is due to the improvement in SWC that limits the soil oxygen concentration, chiefly the anaerobic soil environment formation, pointedly falling nitrification and increasing denitrification, which in turn led to dominant denitrification to produce N2O emissions (Pokharel and Chang, 2021). Our results are identical with other scientific reports by Taghizadeh-Toosi et al. (2022), demonstrating the influence of ST and SWC on the emission of soil N2O.
The correlation study in Table 6 showed a significant negative relationship between N2O emission and carbon and nitrogen contents, supporting the (He et al., 2023) that enhancing carbon stocks and nitrogen soil status can decrease the emission of soil N2O. Consequently, these findings are consistent with earlier results reported by Zhao et al. (2020). High soil bulk density related to the degradation of soil by tillage application can have consequences for soil aeration reduction. Soil degradation reduction, whereas surface soil preservation covered by crop straws, might lead to minor denitrification as well as N2O emissions risk. Quite the reverse, Li et al. (2005) reported a positive correlation between soil N2O emissions and soil carbon contents. This is due to the increase in soil carbon contents delivering sufficient sources of nitrogen and a suggestive rise in the heterotrophic microorganism’s respiration. All at once, carbon availability in the soil offers electron donors for denitrifying microbes, which endorses the denitrification incidence, in that way increasing emissions of N2O. Soil nitrogen contents were positively correlated with N2O emission. Su et al. (2021) stated that improved N2O production might be a consequence of augmented nitrogen contents and bacterial activities; meanwhile, the activities of microbes are controlled by soil carbon contents. This obscures that soil carbon contents improve the N2O-producing microbe’s activities. This also verifies the results of Guenet et al. (2021), who showed that high carbon contents mostly raise the emission of N2O. Changes in CO2, N2O, and CH4 emission seemed to be described by variations in soil temperature, moisture content, and saturated hydraulic conductivity in the surface soil layer of 0–10 cm soil depth, with 95% of the data variance described by these environmental variables.
3.4 Global warming potential under conservation tillage system
The GWP of CO2, CH4 and N2O and net global warming potential (GWP) under all investigated tillage practices are shown in (Figure 7). The highest cumulative CO2 flux was recorded under CT, whereas conservation tillage measures NTS, NT, and CTS pointedly reduced the cumulative CO2 flux, as the least cumulative CO2 flux was noted under NT, which was followed by NTS. The global warming potential due to the emissions of CH4 was significantly lower under NTS, which was followed by NT over CT during the study period. The global warming potential due to the emissions of N2O was significantly less under NT, which was followed by NTS over CT. The net global warming potential followed the trend of CT > CTS > NT > NTS (Figure 7D). The NTS, NT, and CTS decreased net-GWP by 23.44, 19.57, and 16.54%, respectively, over the CT system. The effect of different tillage practices on the atmospheric radiative forcing and henceforth changing climate can be evaluated by global warming potential determination from the biosphere–atmosphere exchange of numerous greenhouse gases. The noted global warming potential for the current study ranged between 1000 and 1400 kg CO2-e ha−1 which is in the range of those stated by Ma et al. (2013) but superior to those stated by Yeboah et al. (2016). The net-GWP was positively correlated with ST and SWC and negatively correlated with carbon and nitrogen elements and Ks (Table 6). In PCA analysis, we found that soil moisture, soil temperature, bulk density, hydraulic conductivity, carbon, and nitrogen elements explained 96.2, 69.6, 67.8, and 77% of the variations in soil CO2, CH4, and N2O fluxes and GWP, respectively (Figures 8A–D). Compared with CH4 and N2O fluxes and GWP, soil CO2 had a strong relationship with environmental variables, as shown in Figure 8A. Conservation agriculture has the potential to contribute to environmental conservation, though its influence differs depending on management strategies. The higher greenhouse gas intensity (GHGI) was associated with the CT system, while the minimum GHGI value was observed with the NT system, which was followed by the NTS system. The GHGI followed the trend of CT > CTS > NTS > NT. The conservation tillage systems NT, NTS, and CTS decreased GHGI by 29.96, 23.20, and 18.72%, respectively, compared with CT practice (Figure 7E). The current noted global warming intensity values were higher compared with other studies (Qin et al., 2010; Ma et al., 2013), which is attributable to the overall lower grain yield in this experimental research. Global warming intensity is a crop yield function, and it is directly affected by low grain yield. A significant reduction was observed under NTS and NT practices over CTS and CT systems in case of global warming potential and global warming intensity values. These findings are in line with Alhassan et al. (2021) and Li et al. (2023), who stated that different conservation tillage systems decreased the global warming potential and global warming intensity compared with the CT technique.
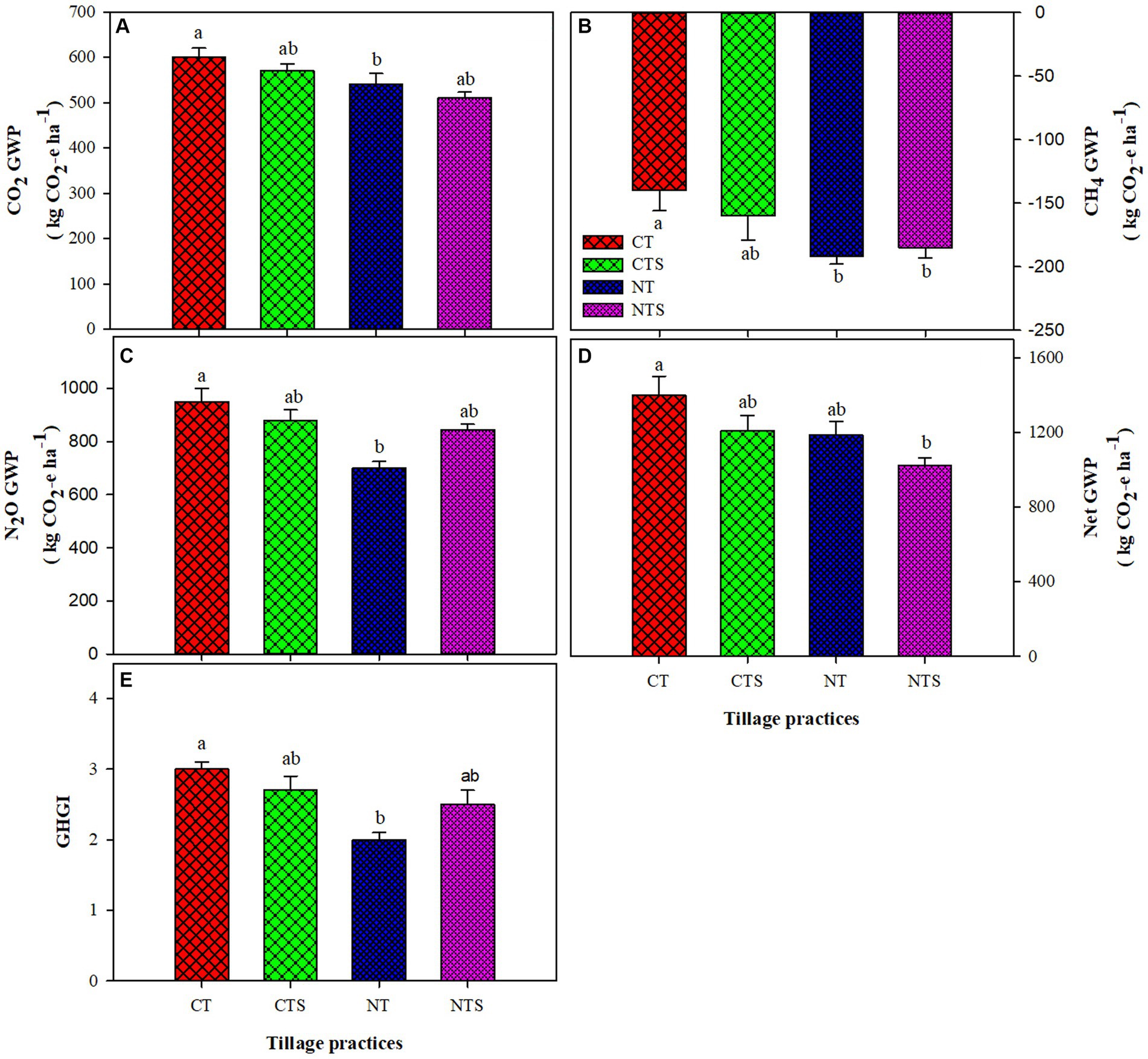
Figure 7. Global warming potential (GWP) of CO2, CH4 and N2O and greenhouse gas intensity (GHGI) under tillage practices in spring-wheat agroecosystem in 2021. Error bars represent the corresponding standard error of mean values; n = 3. Different lower-case letters and ‘*’ indicate significant differences amongst different treatments at p < 0.05 (Duncan’s test performed for mean separation). Note: (A) is the GWP of carbon dioxide as affected by the tillage treatments; (B) is the GWP of CH4 as influenced by the tillage system; (C) is the GWP of N2O as affected by the different tillage techniques; (D) is the net-GWP under tillage measures and (E) is the GHGI under tillage practices.
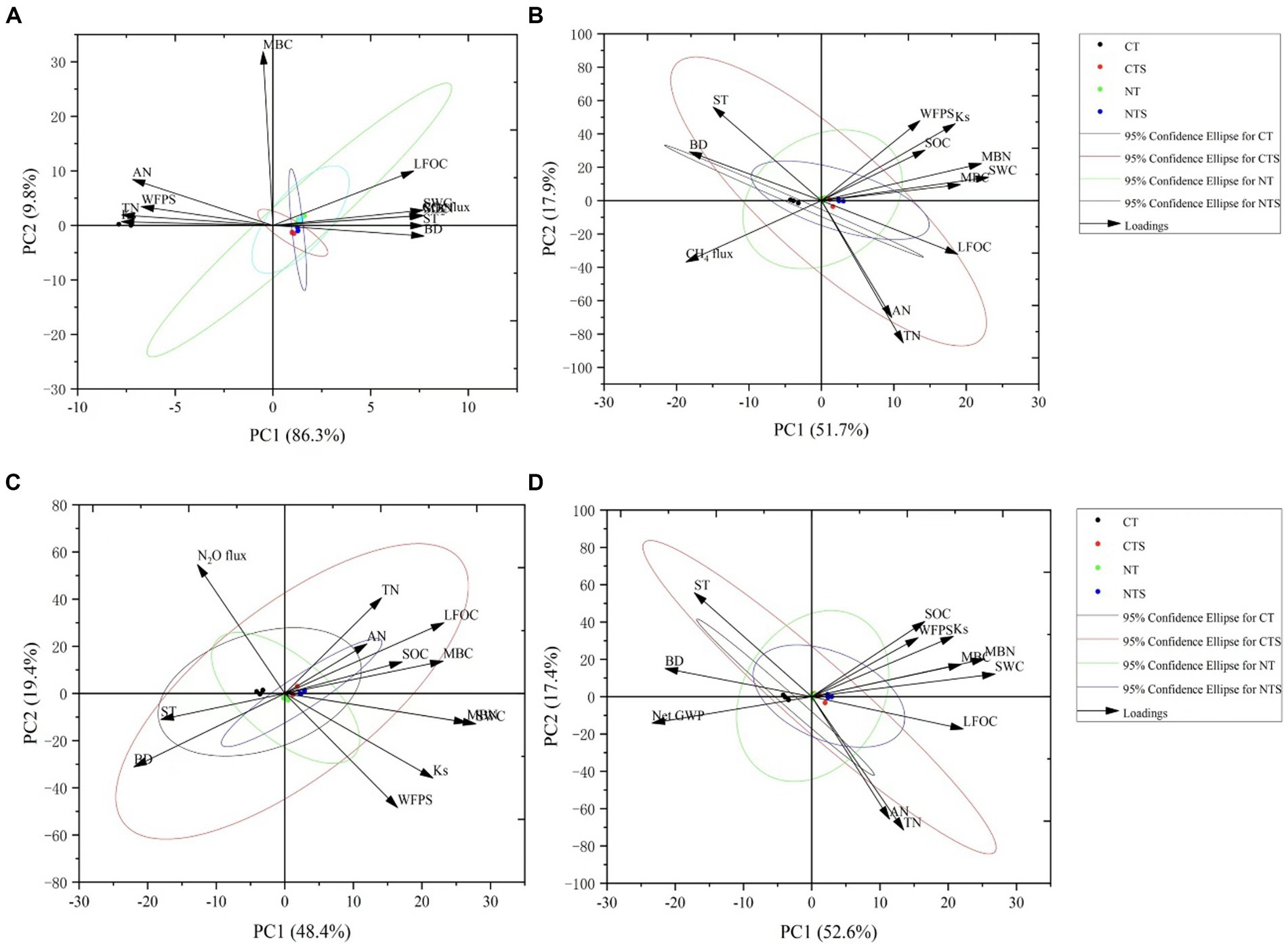
Figure 8. Principal component analysis for evaluating the influence of environmental variables on greenhouse gas emissions under conservation tillage practices. (A) The PCA analysis of environmental variables with carbon dioxide emission; (B) the PCA analysis of environmental variables with methane emission; (C) the PCA analysis of environmental variables with nitrous oxide emission; and (D) the PCA analysis of environmental variables with net global warming under different tillage systems.
4 Conclusion
Conservation tillage is a significant climate-smart approach to increasing soil and water conservation, soil carbon sequestration, crop production and reducing greenhouse gas emissions. It is argued to decrease global warming potential in relation to changing climate mitigation. Our study suggests that conservation tillage practices CTS, NT, and NTS have the potential to increase wheat agronomic traits (plant height, number of spikes per plant, seeds number per meter square, root yield, aboveground biomass yield, thousand-grain weight, grain yield and dry matter yield), soil physical properties (gravimetric soil water content, water-filled pore space, water storage, pore space, aggregates, and hydraulic conductivity), chemical properties (soil organic carbon, light fraction organic carbon, carbon storage, total nitrogen, available nitrogen, nitrogen storage, total phosphorous, available phosphorous, total potassium, available potassium), biological properties (soil microbial count, urease, alkaline phosphatase, invertase, cellulase, catalase, microbial biomasses carbon and nitrogen) and decrease carbon, methane and nitrous oxide emissions in semi-arid sandy loam cropland of Dingxi China in comparison with plowing conventional tillage system. Moreover, NTS and NT significantly reduced the global warming potential of carbon dioxide, methane, and nitrous oxide and their yield-scale global warming potential. The correlation analyses show that crop yield is significantly positively correlated with yield-attributing traits, soil physicochemical properties, and biological properties. The global warming potential due to CO2, CH4, and N2O greenhouse gases had a positive correlation with soil and environmental variables, and the dominant controlled factors were soil moisture and soil temperature. Accordingly, straw-retention with no tilled soil and stubble incorporation with conventionally tilled soil should be recommended and promoted among the smallholder farmer systems in semi-arid zones to raise soil and water conservation, soil and environmental quality, and sustainability. More comprehensive long-term research and different tillage operations are needed regarding carbon sequestration and stocks and genes involved in nitrogen cycling and global warming to produce more powerful data for climate-smart agriculture.
Data availability statement
The raw data supporting the conclusions of this article will be made available by the authors, without undue reservation.
Ethics statement
The studies involving humans were approved by College of Forestry, Gansu Agricultural University, Lanzhou 730,070, China; a2hhbm1haHJhbjQyMEBnbWFpbC5jb20= (MS). The studies were conducted in accordance with the local legislation and institutional requirements. The ethics committee/institutional review board waived the requirement of written informed consent for participation from the participants or the participants’ legal guardians/next of kin because College of Forestry, Gansu Agricultural University, Lanzhou 730,070, China; a2hhbm1haHJhbjQyMEBnbWFpbC5jb20= (MS).
Author contributions
MS: Conceptualization, Data curation, Formal analysis, Methodology, Resources, Software, Validation, Writing – original draft, Writing – review & editing. NR: Writing – review & editing, Writing – original draft. MT: Writing – review & editing, Writing – original draft. ARA: Writing – review & editing, Conceptualization, Methodology, Writing – original draft. MMA: Writing – review & editing, Conceptualization, Data curation, Methodology, Writing – original draft. ALA: Writing – review & editing, Conceptualization, Formal analysis, Methodology, Validation, Writing – original draft. KZG: Funding acquisition, Resources, Writing – review & editing, Data curation, Formal analysis, Investigation, Visualization, Writing – original draft. MAA: Writing – review & editing, Data curation, Investigation, Software. MA: Writing – review & editing, Conceptualization, Formal analysis, Methodology. NM: Writing – review & editing, Conceptualization, Data curation, Writing – original draft. JY: Writing – review & editing. AS: Writing – review & editing. MS: Writing – review & editing, Writing – original draft. MHE: Funding acquisition, Writing – original draft, Data curation, Formal analysis, Writing – review & editing. GC: Writing – review & editing. GL: Formal analysis, Funding acquisition, Project administration, Resources, Supervision, Visualization, Data curation, Methodology, Writing – original draft, Writing – review & editing.
Funding
The author(s) declare financial support was received for the research, authorship, and/or publication of this article. This research was honored to be supported by the National Natural Science Foundation Project (32360438) and the Key R&D Projects in Gansu Province (22YF7FA116) in China.
Acknowledgments
The authors extend their appreciation to the Deanship of Scientific Research at Northern Border University, Arar, KSA, for funding this research work through the project number “NBU–FFR–2024–337–03”.
Conflict of interest
The authors declare that the research was conducted in the absence of any commercial or financial relationships that could be construed as a potential conflict of interest.
Publisher’s note
All claims expressed in this article are solely those of the authors and do not necessarily represent those of their affiliated organizations, or those of the publisher, the editors and the reviewers. Any product that may be evaluated in this article, or claim that may be made by its manufacturer, is not guaranteed or endorsed by the publisher.
References
Alhassan, A.-R. M., Yang, C., Ma, W., and Li, G. (2021). Influence of conservation tillage on greenhouse gas fluxes and crop productivity in spring-wheat agroecosystems on the loess plateau of China. PeerJ 9:e11064. doi: 10.7717/peerj.11064
Al-Kaisi, M. M., and Yin, X. (2005). Tillage and crop residue effects on soil carbon and carbon dioxide emission in corn-soybean rotations. J. Environ. Qual. 34, 437–445. doi: 10.2134/jeq2005.0437
Alskaf, K., Mooney, S. J., Sparkes, D. L., Wilson, P., and Sjogersten, S. (2021). Short-term impacts of different tillage practices and plant residue retention on soil physical properties and greenhouse gas emissions. Soil Tillage Res. 206:104803. doi: 10.1016/j.still.2020.104803
Balota, E. L., Colozzi Filho, A., Andrade, D. S., and Dick, R. P. (2004). Long-term tillage and crop rotation effects on microbial biomass and c and n mineralization in a Brazilian oxisol. Soil Tillage Res. 77, 137–145. doi: 10.1016/j.still.2003.12.003
Bayer, C., Zschornack, T., Pedroso, G. M., da Rosa, C. M., Camargo, E. S., Boeni, M., et al. (2015). A seven-year study on the effects of fall soil tillage on yield-scaled greenhouse gas emission from flood irrigated rice in a humid subtropical climate. Soil Tillage Res. 145, 118–125. doi: 10.1016/j.still.2014.09.001
Bhattacharya, P., Pramanik Maity, P., Mowrer, J., Maity, A., Rao, M., Das, S., et al. (2020). Assessment of soil health parameters and application of the sustainability index to fields under conservation agriculture for 3, 6, and 9 years in India. Heliyon 6:e05640. doi: 10.1016/j.heliyon.2020.e05640
Bhattacharyya, S. S., Leite, F. G. D., France, C. L., Adekoya, A. O., and Ros, G. H. (2023). Soil carbon sequestration, greenhouse gas emissions, and water pollution under different tillage practices. Sci. Total Environ. 826:154161.
Blanco-Canqui, H., and Lal, R. (2008). No-tillage and soil-profile carbon sequestration: an on-farm assessment. Soil Sci. Soc. Am. J. 72, 693–701. doi: 10.2136/sssaj2007.0233
Bond-Lamberty, B., Smith, A. P., and Bailey, V. (2016). Temperature and moisture effects on greenhouse gas emissions from deep active-layer boreal soils. Biogeosciences 13, 6669–6681. doi: 10.5194/bg-13-6669-2016
Bouyoucos, G. J. (1927). The hydrometer as a new method for the mechanical analysis of soils. Soil Sci. 23, 343–354. doi: 10.1097/00010694-192705000-00002
Bozal-Leorri, A., Corrochano-Monsalve, M., Arregui, L. M., Aparicio-Tejo, P. M., and González-Murua, C. (2023). Evaluation of a crop rotation with biological inhibition potential to avoid N2O emissions in comparison with synthetic nitrification inhibition. J. Environ. Sci. 127, 222–233. doi: 10.1016/j.jes.2022.04.035
Braker, G., Schwarz, J., and Conrad, R. (2010). Influence of temperature on the composition and activity of denitrifying soil communities. FEMS Microbiol. Ecol. 73, 134–148. doi: 10.1111/j.1574-6941.2010.00884.x
Cai, L., Wang, J., Luo, Z., Wu, J., and Zhang, R. (2013). Greenhouse gas emissions in double sequence pea-wheat rotation fields under different tillage conditions. Zhongguo Shengtai Nongye Xuebao/Chinese Journal of Eco-Agriculture 21, 921–930. doi: 10.3724/SP.J.1011.2013.00921
Campbell, D. (1994). Determination and use of soil bulk density in relation to soil compaction. In Developments in Agricultural Engineering. Elsevier: Amsterdam, The Netherlands, 11, 113–139.
Castaldi, S. (2000). Responses of nitrous oxide, dinitrogen and carbon dioxide production and oxygen consumption to temperature in forest and agricultural light-textured soils determined by model experiment. Biol. Fertil. Soils 32, 67–72. doi: 10.1007/s003740000218
Celik, I., Barut, Z., Ortas, I., Gok, M., Demirbas, A., Tulun, Y., et al. (2011). Impacts of different tillage practices on some soil microbiological properties and crop yield under semi-arid Mediterranean conditions. Int. J. Plant Prod. 5, 237–254,
Chen, H. Q., Hou, R. X., Gong, Y. S., Li, H. W., Fan, M. S., and Kuzyakov, Y. (2009). Effects of 11 years of conservation tillage on soil organic matter fractions in wheat monoculture in loess plateau of China. Soil Tillage Res. 106, 85–94. doi: 10.1016/j.still.2009.09.009
Chinese Soil Taxonomy Cooperative Research Group (1995). Chinese soil taxonomy (revised proposal); Institute of Soil Science. Beijing, China: Agricultural Science Technology Press.
Curtin, D., Wang, H., Selles, F., McConkey, B., and Campbell, C. (2000). Tillage effects on carbon fluxes in continuous wheat and fallow–wheat rotations. Soil Sci. Soc. Am. J. 64, 2080–2086. doi: 10.2136/sssaj2000.6462080x
Demuner-Molina, G., Cadena-Zapata, M., and Campos-Magaña, S. G. (2014). Efecto de labranza y mejoradores de suelo en humedad y desarrollo radicular. Tecnol. Y Cienc. Del Agua 5, 123–130,
Dencso, M., Horel, A., Bogunovic, I., and Toth, E. (2020). Effects of environmental drivers and agricultural management on soil CO2 and N2O emissions. Agronomy. 11:54. doi: 10.3390/agronomy11010054
Dick, R. P., and Burns, R. G. (2011). A brief history of soil enzymology research. Methods Soil Enzymol. 9, 1–34. doi: 10.2136/sssabookser9.c1
Ding, Y., Wang, H., Shen, H., Li, Z., Geng, J., Han, H., et al. (2003). The clinical pathology of severe acute respiratory syndrome (SARS): a report from China. J. Pathol. Soc. Great Brit. Ireland 200, 282–289. doi: 10.1002/path.1440
Dokuyucu, T., Akkaya, A., and Akcura, M. (2002). The effects of intraplot competition on yield-related traits in wheat plots. Turk. J. Field Crops 7, 40–46,
Feng, J., Li, F., Zhou, X., Xu, C., Ji, L., Chen, Z., et al. (2018). Impact of agronomy practices on the effects of reduced tillage systems on CH4 and N2O emissions from agricultural fields: a global meta-analysis. PLoS One 13:e0196703. doi: 10.1371/journal.pone.0196703
Feng, Y., Ning, T., Li, Z., Han, B., Han, H., Li, Y., et al. (2014). Zhang, X. Effects of tillage practices and rate of nitrogen fertilization on crop yield and soil carbon and nitrogen. Plant Soil Environ. 60, 100–104. doi: 10.17221/820/2013-PSE
Feng, X., Tian, H., Cong, J., and Zhao, C. (2023). A method review of the climate change impact on crop yield. Front. For. Glob. Change 6:1198186. doi: 10.3389/ffgc.2023.1198186
Frankeberger, W., and Johanson, J. (1983). Method of measuring invertase activity in soils. Plant Soil 74, 301–311. doi: 10.1007/BF02181348
Gao, L., Bisheng, W., Shengping, L., Huijun, W., Xueping, W., Guopeng, L., et al. (2019). Soil wet aggregate distribution and pore size distribution under different tillage systems after 16 years in the loess plateau of China. Catena 173, 38–47. doi: 10.1016/j.catena.2018.09.043
Gathala, M. K., Ladha, J., Saharawat, Y. S., Kumar, V., Kumar, V., and Sharma, P. K. (2011). Effect of tillage and crop establishment methods on physical properties of a medium-textured soil under a seven-year rice-wheat rotation. Soil Sci. Soc. Am. J. 75, 1851–1862. doi: 10.2136/sssaj2010.0362
Gregorich, E., and Ellert, B. (1993). Light fraction and macroorganic. Soil Sampl. Methods Anal. 397, 397–407,
Guenet, B., Gabrielle, B., Chenu, C., Arrouays, D., Balesdent, J., Bernoux, M., et al. (2021). Can N2O emissions offset the benefits from soil organic carbon storage? Glob. Chang. Biol. 27, 237–256. doi: 10.1111/gcb.15342
Han, Y., Ma, W., Zhou, B., Yang, X., Salah, A., Li, C., et al. (2020). Effects of straw-return method for the maize–Rice rotation system on soil properties and crop yields. Agronomy 10:461. doi: 10.3390/agronomy10040461
He, Y., Mao, H., Makinia, J., Drewnowski, J., Wu, B., Xu, J., et al. (2023). Impact of soluble organic matter and particulate organic matter on anammox system: performance, microbial community and N2O production. J. Environ. Sci. 124, 146–155. doi: 10.1016/j.jes.2021.11.007
He, M., Wang, Y. C., Wang, L. G., Cheng, Q., Wang, L. M., Li, Y. H., et al. (2020). Effects of subsoiling combined with fertilization on the fractions of soil active organic carbon and soil active nitrogen, and enzyme activities in black soil in Northeast China. Acta Pedol. Sin. 57, 446–456. doi: 10.11766/trxb201810180282
Hu, X., Zhou, Y., Zhou, L., Zhang, Y., Wu, L., Xu, H., et al. (2022). Urban and agricultural land use regulates the molecular composition and bio-lability of fluvial dissolved organic matter in human-impacted southeastern China. Carbon Res. 1:19. doi: 10.1007/s44246-022-00020-6
Huang, M., Dang, T., Gallichand, J., and Goulet, M. (2003). Effect of increased fertilizer applications to wheat crop on soil-water depletion in the loess plateau China. Agric. Water Manag. 58, 267–278. doi: 10.1016/S0378-3774(02)00086-0
Huang, G., Luo, Z., Li, L., Zhang, R., Li, G., Cai, L., et al. (2012). Effects of stubble management on soil fertility and crop yield of rainfed area in Western loess plateau. China. Appl. Environ. Soil Sci. 2012:9. doi: 10.1155/2012/256312
Huang, Y., Ren, W., Wang, L., Hui, D., Grove, J. H., Yang, X., et al. (2018). Greenhouse gas emissions and crop yield in no-tillage systems: a meta-analysis. Agric. Ecosyst. Environ. 268, 144–153. doi: 10.1016/j.agee.2018.09.002
Indoria, A. K., Sharma, K. L., Reddy, K. S., and Rao, C. S. (2016). Role of soil physical properties in soil health management and crop productivity in rainfed systems II. Management technologies and crop productivity. Curr. Sci. 110, 320–328. doi: 10.18520/cs/v110/i3/320-328
IPCC (2013). Climate change: The physical science basis. Contribution of working group I to the fifth assessment report of the intergovernmental panel on climate change. Cambridge, United Kingdom: Cambridge University Press.
IPCC (2021). Climate change 2021: The physical science basis. Contribution of working group I to the sixth assessment report of the intergovernmental panel on climate change. Cambridge: Cambridge University Press.
Jat, H. S., Choudhary, M., Datta, A., Yadav, A. K., Meena, M. D., Devi, R., et al. (2020). Temporal changes in soil microbial properties and nutrient dynamics under climate smart agriculture practices. Soil Tillage Res. 199:104595. doi: 10.1016/j.still.2020.104595
Jat, H. S., Datta, A., Choudhary, M., Sharma, P. C., Dixit, B., and Jat, M. L. (2021). Soil enzymes activity: effect of climate smart agriculture on rhizosphere and bulk soil under cereal based systems of north-West India. Eur. J. Soil Biol. 103:103292. doi: 10.1016/j.ejsobi.2021.103292
Jat, H., Datta, A., Sharma, P., Kumar, V., Yadav, A., et al. (2018). Assessing soil properties and nutrient availability under conservation agriculture practices in a reclaimed sodic soil in cereal-based systems of north-West India. Arch. Agron. Soil Sci. 64, 531–545. doi: 10.1080/03650340.2017.1359415
Khan, S., Aqeel, S., Muhammad, N., and Mohsin, K. (2017). Impact of different tillage practices on soil physical properties, nitrate leaching and yield attributes of maize (Zea mays L.). J. Soil Sci. Plant Nutr. 1, 240–252. doi: 10.4067/S0718-95162017005000019
Khorami, S. S., Kazemeini, S. A., Afzalinia, S., and Gathala, M. K. (2018). Changes in soil properties and productivity under different tillage practices and wheat genotypes: a short-term study in Iran. Sustain. For. 10:3273. doi: 10.3390/su10093273
Krauss, M., Wiesmeier, M., Don, A., Cuperus, F., Gattinger, A., Gruber, S., et al. (2022). Reduced tillage in organic farming affects soil organic carbon stocks in temperate Europe. Soil and Tillage Research. 216:105262.
Kristine, V., Jovita, P.-U., Olga, S., Sindija, L., et al. (2022). Soil tillage and agricultural crops affect greenhouse gas emissions from Cambic Calcisol in a temperate climate. Acta Agric. Scand. Sect. Plant Sci. 72, 835–846. doi: 10.1080/09064710.2022.2097123
Lal, R. (2005). “Climate change, soil carbon dynamics, and global food security” in Climate change and global food security (Boca Raton: Taylor and Francis), 113–143.
Lampurlanes, J., Angas, P., and Cantero-Martinez, C. (2002). Tillage effects on water storage during fallow, and on barley root growth and yield in two contrasting soils of the semi-arid Segarra region in Spain. Soil Tillage Res. 65, 207–220. doi: 10.1016/S0167-1987(01)00285-9
Li, C., Frolking, S., and Butterbach-Bahl, K. (2005). Carbon sequestration in arable soils is likely to increase nitrous oxide emissions, offsetting reductions in climate radiative forcing. Clim. Chang. 72, 321–338. doi: 10.1007/s10584-005-6791-5
Li, F. D., Yu, Z. N., and He, S. J. (1996). Experimental technique of agricultural microbiology. Beijing, China: China Agriculture Press.
Li, Z., Zhang, Q., Li, Z., Qiao, Y., Du, K., Yue, Z., et al. (2023). Different responses of agroecosystem greenhouse gas emissions to tillage practices in a Chinese wheat–maize cropping system. Carbon Res. 2:7. doi: 10.1007/s44246-023-00042-8
Li, L., Zhang, R., Luo, Z., Liang, W., Xie, J., Cai, L., et al. (2014). Evolution of soil and water conservation in rain-fed areas of China. Int. Soil Water Conserv. Res. 2, 78–90. doi: 10.1016/S2095-6339(15)30015-0
Li, H., Zhou, Y., Xin, W., Wei, Y., Zhang, J., and Guo, L. (2019). Wheat breeding in northern China: achievements and technical advances. Crop J. 7, 718–729. doi: 10.1016/j.cj.2019.09.003
Liebhard, G., Klik, A., Neugschwandtner, R. W., and Nolz, R. (2022). Effects of tillage systems on soil water distribution, crop development and evaporation and transpiration rates of soybean. Agric. Water Manag. 269:107719. doi: 10.1016/j.agwat.2022.107719
Liu, E., Chen, B., Yan, C., Zhang, Y., Mei, X., and Wang, J. (2015). Seasonal changes and vertical distributions of soil organic carbon pools under conventional and no-till practices on loess plateau in China. Soil Sci. Soc. Am. J. 79, 517–526. doi: 10.2136/sssaj2014.02.0069
Liu, J., Jing, F., Jiang, G. Y., and Liu, J. G. (2017). Effects of straw incorporation on soil organic carbon density and the carbon Pool management index under long-term continuous cotton. Commun. Soil Sci. Plant Anal. doi: 10.1080/00103624.2016.1269799
Lu, R. (1999). Analytical Methods of Soil Agrochemistry. Beijing, China: China Agricultural Science and Technology Press.
Lv, L., Zhoubing, G., Kaihua, L., Qing, Z., and Junjun, Z. (2023). Impact of conservation tillage on the distribution of soil nutrients with depth. Soil Tillage Res. 225. doi: 10.1016/j.still.2022.105527
Ma, L., Kong, F., Lv, X., Wang, Z., Zhou, Z., and Meng, Y. (2021). Responses of greenhouse gas emissions to different straw management methods with the same amount of carbon input in cotton field. Soil Tillage Res. 213:105126. doi: 10.1016/j.still.2021.105126
Ma, Y., Kong, X., Yang, B., Zhang, X., Yan, X., Yang, J., et al. (2013). Net global warming potential and greenhouse gas intensity of annual rice-wheat rotations with integrated soil–crop system management. Agric. Ecosyst. Environ. 164, 209–219. doi: 10.1016/j.agee.2012.11.003
Maucieri, C., Tolomio, M., McDaniel, M. D., Zhang, Y., Robatjazi, J., and Borin, M. (2021). No-tillage effects on soil CH4 fluxes: a meta-analysis. Soil Tillage Res. 212:105042. doi: 10.1016/j.still.2021.105042
McLain, J. E., and Martens, D. A. (2006). Moisture controls on trace gas fluxes in semiarid riparian soils. Soil Sci. Soc. Am. J. 70, 367–377. doi: 10.2136/sssaj2005.0105
Mehnaz, K. R., Keitel, C., and Dijkstra, F. A. (2018). Effects of carbon and phosphorus addition on microbial respiration, N2O emission, and gross nitrogen mineralization in a phosphorus-limited grassland soil. Biol. Fertil. Soils 54, 481–493. doi: 10.1007/s00374-018-1274-9
Mei, K., Wang, Z., Huang, H., Zhang, C., Shang, X., Dahlgren, R. A., et al. (2018). Stimulation of N2O emission by conservation tillage management in agricultural lands: a meta-analysis. Soil Tillage Res. 182, 86–93. doi: 10.1016/j.still.2018.05.006
Mikha, M., Vigil, M., Liebig, M., Bowman, R., McConkey, B., Deibert, E., et al. (2006). Cropping system influences on soil chemical properties and soil quality in the Great Plains. Renew. Agric. Food Syst. 21, 26–35. doi: 10.1079/RAFS2005123
Nelson, D. A., and Sommers, L. (1983). Total carbon, organic carbon, and organic matter. Methods soil anal. Part 2 Chem. Microbiol. Prop. 9, 539–579,
Niu, Y., Zhang, R., Luo, Z., Li, L., Cai, L., Li, G., et al. (2016). Contributions of long-term tillage systems on crop production and soil properties in the semi-arid loess plateau of China. J. Sci. Food Agric. 96, 2650–2659. doi: 10.1002/jsfa.7382
O’Kelly, B. C. (2004). Accurate determination of moisture content of organic soils using the oven drying method. Dry. Technol. 22, 1767–1776. doi: 10.1081/DRT-200025642
O’Neill, M., Lanigan, G. J., Forristal, P. D., and Osborne, B. A. (2021). Greenhouse gas emissions and crop yields from winter oilseed rape cropping systems are unaffected by management practices. Front. Environ. Sci. 9:636. doi: 10.3389/fenvs.2021.716636
Ordoñez-Morales, K. D., Cadena-Zapata, M., Zermeño-González, A., and Campos-Magaña, S. (2019). Effect of tillage systems on physical properties of a clay loam soil under oats. Agriculture 9:62. doi: 10.3390/agriculture9030062
Pokharel, P., and Chang, S. X. (2021). Biochar decreases the efficacy of the nitrification inhibitor nitrapyrin in mitigating nitrous oxide emissions at different soil moisture levels. J. Environ. Manag. 295:113080. doi: 10.1016/j.jenvman.2021.113080
Pu, C., Chen, J. S., Wang, H. D., Virk, A. L., Zhao, X., and Zhang, H. L. (2022). Greenhouse gas emissions from the wheat-maize cropping system under different tillage and crop residue management practices in the North China plain. Sci. Total Environ. 819:153089. doi: 10.1016/j.scitotenv.2022.153089
Pu, Q. M., Yang, P., Deng, Y. C., Xiang, C. Y., Lin, B. M., Liu, L. S., et al. (2020). Effects of different fertilization methods on soil enzyme activity, soil nutrients and quality of spring cabbage. J. Agric. Sci. Technol. 22, 130–139,
Qin, Y., Liu, S., Guo, Y., Liu, Q., and Zou, J. (2010). Methane and nitrous oxide emissions from organic and conventional rice cropping systems in Southeast China. Biol. Fertil. Soils 46, 825–834. doi: 10.1007/s00374-010-0493-5
Rabot, E., Cousin, I., and Henault, C. A. (2015). Modeling approach of the relationship between nitrous oxide fluxes from soils and the water-filled pore space. Biogeochemistry 122, 395–408. doi: 10.1007/s10533-014-0048-1
Raich, J. W., and Schlesinger, W. H. (1992). The global carbon dioxide flux in soil respiration and its relationship to vegetation and climate. Tellus B. 44, 81–99. doi: 10.3402/tellusb.v44i2.15428
Raihan, A., and Tuspekova, A. (2022). Dynamic impacts of economic growth, renewable energy use, urbanization, industrialization, tourism, agriculture, and forests on carbon emissions in Turkey. Carbon Res. 1:20. doi: 10.1007/s44246-022-00019-z
Rakesh, S., Sarkar, D., Sinha, A. K., Danish, S., Bhattacharya, P. M., Mukhopadhyay, P., et al. (2021). Soil organic carbon and labile and recalcitrant carbon fractions attributed by contrasting tillage and cropping systems in old and recent alluvial soils of subtropical eastern India. PLoS One 16:e0259645. doi: 10.1371/journal.pone.0259645
Reynolds, W., Carter, M., and Gregorich, E. (2008). Saturated hydraulic properties: Well permeameter. In Soil Sampling Methods of Analysis, 2nd ed. Boca Raton, FL, USA: CRC Press. 1025–1042.
Roy, D., Datta, A., Jat, H., Choudhary, M., Sharma, P., Singh, P., et al. (2022). Impact of long-term conservation agriculture on soil quality under cereal based systems of north West India. Geoderma 405:115391. doi: 10.1016/j.geoderma.2021.115391
Sadiq, M., Li, G., Rahim, N., and Tahir, M. M. (2021a). Sustainable conservation tillage technique for improving soil health by enhancing soil physicochemical quality indicators under wheat mono-cropping system conditions. Sustain. For. 13:8177. doi: 10.3390/su13158177
Sadiq, M., Li, G., Rahim, N., and Tahir, M. M. (2021b). Effect of conservation tillage on yield of spring wheat (Triticum aestivum L.) and soil mineral nitrogen and carbon content. Int. Agrophys. 35, 83–95. doi: 10.31545/intagr/132363
Salamanca-Fresno, C., Soriano, M. A., Testi, L., and Gomez-Macpherson, H. (2022). Effects of conservation tillage, controlled traffic and regulated deficit irrigation on soil CO2 emissions in a maize-based system in Mediterranean conditions. Sci. Total Environ. 813:152454. doi: 10.1016/j.scitotenv.2021.152454
Sanz, M. J., de Vente, J., Chotte, J. L., Bernoux, M., et al. (2017). Sustainable land management contribution to successful land-based climate change adaptation and mitigation. A report of the science-policy Interface. Bonn, Germany: United Nations convention to combat desertification (UNCCD).
Sarkar, S., and Singh, S. (2007). Interactive effect of tillage depth and mulch on soil temperature, productivity and water use pattern of rainfed barley (Hordium vulgare L.). Soil Tillage Res. 92, 79–86. doi: 10.1016/j.still.2006.01.014
Schaufler, G., Kitzler, B., Schindlbacher, A., Skiba, U., Sutton, M., and Zechmeister-Boltenstern, S. (2010). Greenhouse gas emissions from European soils under different land use: effects of soil moisture and temperature. Eur. J. Soil Sci. 61, 683–696. doi: 10.1111/j.1365-2389.2010.01277.x
Schwilch, G., Laouina, A., Chaker, M., Machouri, N., Sfa, K., and Stroosnijder, L. (2015). Challenging conservation agriculture on marginal slopes in Sehoul. Morocco. Renew. Agr. Food Syst. 30, 233–251. doi: 10.1017/S1742170513000446
Shakoor, A., Shahbaz, M., Farooq, T. H., Sahar, N. E., Shahzad, S. M., Altaf, M. M., et al. (2021). A global meta-analysis of greenhouse gases emission and crop yield under no-tillage as compared to conventional tillage. Sci. Total Environ. 750:142299. doi: 10.1016/j.scitotenv.2020.142299
Shen, Y., Sui, P., Huang, J., Wang, D., Whalen, J. K., and Chen, Y. (2018). Greenhouse gas emissions from soil under maize–soybean intercrop in the North China plain. Nutr. Cycl. Agroecosyst. 110, 451–465. doi: 10.1007/s10705-018-9908-8
Singh, B. K., Bardgett, R. D., Smith, P., and Reay, D. S. (2010). Microorganisms and climate change: terrestrial feedbacks and mitigation options. Nat. Rev. Microbiol. 8, 779–790. doi: 10.1038/nrmicro2439
Singh, Y., Bhardwaj, A., Singh, S., Singh, R., Chaudhary, D., et al. (2002). Effect of rice (Oryza sativa)-establishment methods, tillage practices in wheat (Triticum aestivum) and fertilization on soil physical properties and rice-wheat system productivity on a silty day Mollisol of Uttaranchal. Indian J. Agric. Sci. 72, 200–205,
Sinha, A. K., Ghosh, A., Dhar, T., Bhattacharya, P. M., Mitra, B., Rakesh, S., et al. (2019). Trends in key soil parameters under conservation agriculture-based sustainable intensification farming practices in the eastern ganga Alluvial Plains. Soil Res. 57, 883–893. doi: 10.1071/SR19162
Smart SOIL (2015). Fact sheets providing technical and economic information on five practices that promote good soil carbon management: crop rotation, residue management, adding manure or compost, cover and catch crops, and conservation agriculture. EUFP7 smart SOIL project–289694, Available at: https://projects.au.dk/fileadmin/SmartSOIL_factsheet_crop-rotations.pdf.
Su, X., Wen, T., Wang, Y., Xu, J., Cui, L., Zhang, J., et al. (2021). Stimulation of N2O emission via bacterial denitrification driven by acidification in estuarine sediments. Glob. Chang. Biol. 27, 5564–5579. doi: 10.1111/gcb.15863
Taa, A., Tanner, D., and Bennie, A. T. P. (2004). Effects of stubble management, tillage and cropping sequence on wheat production in the south-eastern highlands of Ethiopia. Soil Tillage Res. 76, 69–82. doi: 10.1016/j.still.2003.08.002
Taghizadeh-Toosi, A., Hansen, E. M., Olesen, J. E., Baral, K. R., and Petersen, S. O. (2022). Interactive effects of straw management, tillage, and a cover crop on nitrous oxide emissions and nitrate leaching from a sandy loam soil. Sci. Total Environ. 828:154316. doi: 10.1016/j.scitotenv.2022.154316
Ussiri, D. A., Lal, R., and Jarecki, M. K. (2009). Nitrous oxide and methane emissions from long-term tillage under a continuous corn cropping system in Ohio. Soil Tillage Res. 104, 247–255. doi: 10.1016/j.still.2009.03.001
Vance, F., Brookes, P., and Jenkinson, D. (1987). Microbial biomass measurements in forest soil: the use of the chloroform fumigation incubation method in strongly acid soils. Soil Biol. Biochem. 19, 697–702.
Wang, Q., Bai, Y., Gao, H., He, J., Chen, H., Chesney, R. C., et al. (2008). Soil chemical properties and microbial biomass after 16 years of no-tillage farming on the loess plateau, China. Geoderma 144, 502–508. doi: 10.1016/j.geoderma.2008.01.003
Wang, Y., Chen, S., Sun, H., and Zhang, X. (2009). Effects of different cultivation practices on soil temperature and wheat spike differentiation. Cereal Res. Commun. 37, 575–584. doi: 10.1556/CRC.37.2009.4.12
Wang, W., Sardans, J., Wang, C., Pan, T., Zeng, C., Lai, D., et al. (2017). Straw application strategy to optimize nutrient release in a southeastern China rice cropland. Agronomy 7:84. doi: 10.3390/agronomy7040084
Wang, W., Zhang, H., Mo, F., Liao, Y., and Wen, X. (2022). Reducing greenhouse gas emissions and improving net ecosystem economic benefit through long-term conservation tillage in a wheat-maize multiple cropping system in the loess plateau, China. Euro. J. Agron. 141:126619. doi: 10.1016/j.eja.2022.126619
Wei, D., Liu, Y., Wang, Y., and Wang, Y. (2014). Three-year study of CO2 efflux and CH4/N2O fluxes at an alpine steppe site on the central Tibetan plateau and their responses to simulated N deposition. Geoderma 232-234, 88–96. doi: 10.1016/j.geoderma.2014.05.002
Wei, Z., Wu, S., Zhou, S., and Lin, C. (2013). Installation of impervious surface in urban areas affects microbial biomass, activity (potential c mineralisation), and functional diversity of the fine earth. Soil Res. 51, 59–67. doi: 10.1071/SR12089
Wozniak, A., and Gos, M. (2014). Yield and quality of spring wheat and soil properties as affected by tillage system. Plant Soil Environ 60, 141–145. doi: 10.17221/7330-PSE
Wu, Z., Huang, N. E., Wallace, J. M., Smoliak, B. V., and Chen, X. (2011). On the time-varying trend in global-mean surface temperature. Clim. Dyn. 37, 759–773. doi: 10.1007/s00382-011-1128-8
Wu, F., Li, F., Zhao, X., Bolan, N. S., Fu, P., Lam, S. S., et al. (2022). Meet the challenges in the “carbon age”. Carbon Res. 1:1. doi: 10.1007/s44246-022-00001-9
Wu, X., Niu, Y. B., Xun, M. Y., Jin, J. Y., Tang, Y. K., and Chen, Y. M. (2021). Soil carbon, nitrogen, and phosphorus storages and their stoichiometry due to mixed afforestation with Hippophae rhamnoides in the loess hilly region, China. Forests 12:1718. doi: 10.3390/f12121718
Wulanningtyas, H. S., Gong, Y., Li, P., Sakagami, N., Nishiwaki, J., and Komatsuzaki, M. (2021). A cover crop and no-tillage system for enhancing soil health by increasing soil organic matter in soybean cultivation. Soil Tillage Res. 205:104749. doi: 10.1016/j.still.2020.104749
Xingchen, D., Zhang, J., Huizhen, Q., Zhang, H., Chaoyue, L., Delei, D., et al. (2019). Chronic nitrogen fertilization modulates competitive interactions among microbial ammonia oxidizers in a loess soil. Pedosphere 29, 24–33. doi: 10.1016/S1002-0160(18)60055-4
Xu, A., Li, L., Coulter, J. A., Xie, J., Gopalakrishnan, S., Zhang, R., et al. (2020). Long-term nitrogen fertilization impacts on soil bacteria, grain yield and nitrogen use efficiency of wheat in semiarid loess plateau, China. Agronomy 10:1175. doi: 10.3390/agronomy10081175
Yadav, G. S., Das, A., Lal, R., Babu, S., et al. (2018). Conservation tillage and mulching effects on the adaptive capacity of direct-seeded upland rice (Oryza sativa L.) to alleviate weed and moisture stresses in the north eastern Himalayan region of India. Arch. Agron. Soil Sci. 64, 1254–1267. doi: 10.1080/03650340.2018.1423555
Yang, Y., Liu, H., Wu, J., Zhang, S., Gao, C., Zhang, S., et al. (2023). Soil enzyme activities, soil physical properties, photosynthetic physical characteristics and water use of winter wheat after long-term straw mulch and organic fertilizer application. Front. Plant Sci. 14:1186376. doi: 10.3389/fpls.2023.1186376
Yang, Y. H., Wu, J. C., Zhao, S. W., Han, Q. Y., Pan, X. Y., He, F., et al. (2018). Assessment of the responses of soil pore properties to combined soil structure amendments using X-ray computed tomography. Sci. Rep. 8:695. doi: 10.1038/s41598-017-18997-1
Yeboah, S., Lamptey, S., and Zhang, R. (2018). Effects of different tillage and straw management systems on soil aggregation and crop yield in Rainfed loess plateau. Adv. Agric. Sci. :6,
Yeboah, S., Zhang, R., Cai, L., Song, M., Li, L., Xie, J., et al. (2016). Greenhouse gas emissions in a spring wheat–field pea sequence under different tillage practices in semi-arid Northwest China. Nutr. Cycl. Agroecosyst. 106, 77–91. doi: 10.1007/s10705-016-9790-1
Yu, H. (2015). Study on the regulation effect of straw returning on soil microorganisms and maize. Jilin: Jilin Agricultural University.
Yuan, J., Liang, Y., Zhuo, M., Sadiq, M., Liu, L., Wu, J., et al. (2023b). Soil nitrogen and carbon storages and carbon pool management index under sustainable conservation tillage strategy. Front. Ecol. Evol. 10, 2296–701X. doi: 10.3389/fevo.2022.1082624
Yuan, J., Sadiq, M., Rahim, N., Tahir, M. M., Liang, Y., Zhuo, M., et al. (2023a). Changes in soil properties and crop yield under sustainable conservation tillage Systems in Spring Wheat Agroecosystems. Land 12:1253. doi: 10.3390/land12061253
Yuan, J., Yan, L., Li, G., Sadiq, M., Rahim, N., Wu, J., et al. (2022). Effects of conservation tillage strategies on soil physicochemical indicators and N2O emission under spring wheat monocropping system conditions. Sci. Rep. 12:7066. doi: 10.1038/s41598-022-11391-6
Yuesi, W., and Yinghong, W. (2003). Quick measurement of CH4, CO2 and N2O emissions from a short-plant ecosystem. Adv. Atmos. Sci. 20, 842–844. doi: 10.1007/BF02915410
Zhang, S., Wang, Y., and Shen, Q. (2018). Influence of straw amendment on soil physicochemical properties and crop yield on a consecutive mollisol slope in Northeastern China. Water 10:559. doi: 10.3390/w10050559
Zhang, J., Zhao, S., Liu, Y., Liang, H., Wang, T., Zhao, Y., et al. (2022). Differences in methane and nitrous oxide emissions and soil bacteria communities between straw return methods in Central China. Environ. Sci. Poll. Res., 1–9,
Zhao, Z., Cao, L., Deng, J., Sha, Z., Chu, C., Zhou, D., et al. (2020). Modeling CH4 and N2O emission patterns and mitigation potential from paddy fields in Shanghai, China with the DNDC model. Agric. Syst. 178:102743. doi: 10.1016/j.agsy.2019.102743
Zhao, L., and Jiang, Y. (1986). Discussion on measurements of soil phosphates. Chin. J. Soil Sci. 17, 138–141,
Zhao, Y. G., Pang, H. C., Wang, J., Huo, L., and Li, Y. Y. (2014). Effects of straw mulch and buried straw on soil moisture and salinity in relation to sunflower growth and yield. Field Crop Res. 161, 16–25. doi: 10.1016/j.fcr.2014.02.006
Zhao, X., Yuan, G., Wang, H., Lu, D., Chen, X., and Zhou, J. (2019). Effects of full straw incorporation on soil fertility and crop yield in rice-wheat rotation for silty clay loamy cropland. Agronomy 9:133. doi: 10.3390/agronomy9030133
Keywords: climate-smart agriculture, carbon sequestration, greenhouse gases, global warming, nutrients, sustainable conservation tillage, yield
Citation: Sadiq M, Rahim N, Tahir MM, Alasmari A, Alqahtani MM, Albogami A, Ghanem KZ, Abdein MA, Ali M, Mehmood N, Yuan J, Shaheen A, Shehzad M, El-Sayed MH, Chen G and Li G (2024) Conservation tillage: a way to improve yield and soil properties and decrease global warming potential in spring wheat agroecosystems. Front. Microbiol. 15:1356426. doi: 10.3389/fmicb.2024.1356426
Edited by:
Upendra Kumar, National Rice Research Institute (ICAR), IndiaReviewed by:
D. R. Bhardwaj, Dr. Yashwant Singh Parmar University of Horticulture and Forestry, IndiaBiswaranjan Behera, Indian Institute of Water Management (ICAR), India
Copyright © 2024 Sadiq, Rahim, Tahir, Alasmari, Alqahtani, Albogami, Ghanem, Abdein, Ali, Mehmood, Yuan, Shaheen, Shehzad, El-Sayed, Chen and Li. This is an open-access article distributed under the terms of the Creative Commons Attribution License (CC BY). The use, distribution or reproduction in other forums is permitted, provided the original author(s) and the copyright owner(s) are credited and that the original publication in this journal is cited, in accordance with accepted academic practice. No use, distribution or reproduction is permitted which does not comply with these terms.
*Correspondence: Guang Li, bGlnQGdzYXUuZWR1LmNu