- 1College of Life Sciences, Shandong Normal University, Jinan, China
- 2State Key Laboratory of Mycology, Institute of Microbiology, Chinese Academy of Sciences, Beijing, China
- 3State Key Laboratory of Efficient Production of Forest Resources, School of Ecology and Nature Conservation, Beijing Forestry University, Beijing, China
The fungus Rhizopus arrhizus (=R. oryzae) is commonly saprotrophic, exhibiting a nature of decomposing organic matter. Additionally, it serves as a crucial starter in food fermentation and can act as a pathogen causing mucormycosis in humans and animals. In this study, two distinct endofungal bacteria (EFBs), associated with individual strains of R. arrhizus, were identified using live/dead staining, fluorescence in situ hybridization, transmission electron microscopy, and 16S rDNA sequencing. The roles of these bacteria were elucidated through antibiotic treatment, pure cultivation, and comparative genomics. The bacterial endosymbionts, Pandoraea sputorum EFB03792 and Mycetohabitans endofungorum EFB03829, were purified from the host fungal strains R. arrhizus XY03792 and XY03829, respectively. Notably, this study marks the first report of Pandoraea as an EFB genus. Compared to its free-living counterparts, P. sputorum EFB03792 exhibited 28 specific virulence factor-related genes, six specific CE10 family genes, and 74 genes associated with type III secretion system (T3SS), emphasizing its pivotal role in invasion and colonization. Furthermore, this study introduces R. arrhizus as a new host for EFB M. endofungorum, with EFB contributing to host sporulation. Despite a visibly reduced genome, M. endofungorum EFB03829 displayed a substantial number of virulence factor-related genes, CE10 family genes, T3SS genes, mobile elements, and significant gene rearrangement. While EFBs have been previously identified in R. arrhizus, their toxin-producing potential in food fermentation has not been explored until this study. The discovery of these two new EFBs highlights their potential for toxin production within R. arrhizus, laying the groundwork for identifying suitable R. arrhizus strains for fermentation processes.
Introduction
Bacteria residing within the vegetative or reproductive structures of fungi are referred to as endofungal or endohyphal bacteria (EFBs or EHBs), representing one of the most intricate relationships between bacteria and fungi (Deveau et al., 2018; Pawlowska et al., 2018). The presence of EFBs was initially reported by Mosse (1970) through electron microscopy in the cytoplasm of Endogone spores. In the following decades, researchers discovered EFBs in various species of arbuscular mycorrhizal fungi (AMF), distinguishing two shapes (rod-shaped and irregularly coccoid; MacDonald and Chandler, 1981; Sward, 1981; Scannerini and Bonfante-Fasolo, 1991; Schüßler et al., 1994). EFBs were later identified using bacteria-specific dyes, fluorescence in situ hybridization (FISH) with bacteria-specific probes, and pyrosequencing (Sun et al., 2019). They were categorized into facultative and obligate based on their in vitro cultivability (Mondo et al., 2012; Bonfante and Desirò, 2017; Uehling et al., 2017, 2023). The symbiotic relationship between EFBs and host fungi involves mutualistic benefits and occasional antagonism (Lastovetsky et al., 2020; Venkatesh et al., 2022), impacting asexual and sexual reproduction of the host fungi (Partida-Martinez et al., 2007c; Mondo et al., 2017). This symbiosis extends to form a tripartite interaction with plants or animals, contributing to plant or animal health and performance (Desirò et al., 2015; Guo and Narisawa, 2018; Büttner et al., 2023; Cappelli et al., 2023). The EFB-fungi interaction has gained attention due to its relevance to agriculture and industry.
Many EFBs are associated with the fungal phylum Mucoromycota, and belong to Betaproteobacteria (Burkholderia-related endobacteria, BREs) and Mollicutes (Mycoplasma-related endobacteria, MREs) (Pawlowska et al., 2018; Okrasińska et al., 2021; Richter et al., 2022; Uehling et al., 2023). The BRE Mycetohabitans rhizoxinica, highly dependent on its host R. microsporus, was protected by transcription activator-like (TAL) effectors, while produced toxins rhizoxin and rhizonin with implications in causing rice seedling blight disease and hepatotoxicity (Partida-Martinez and Hertweck, 2005; Partida-Martinez et al., 2007a; Richter et al., 2023). More EFBs of Mucoromycota demonstrate significant potential in biosynthesizing secondary metabolites and activating fungal genes related to toxin synthesis and pathogenicity (Muszewska et al., 2021; Cheng et al., 2022; Niehs et al., 2022; Ghasemi et al., 2023). However, the chemical signals involved in these interactions remain poorly understood, posing potential risks to third parties beyond bacteria and host fungi (Zhou et al., 2022).
The genus Rhizopus, characterized by abundant rhizoids on hyphae and stolons, encompasses 12 species widely distributed in soil and air, playing key roles in industrial, agricultural, and medical applications (Zheng et al., 2007; Liu et al., 2019; Zhao et al., 2023). Notably, R. arrhizus and R. microsporus are crucial in food fermentation and mucormycosis (Cheng et al., 2017; Yao et al., 2018; Rudramurthy et al., 2023), presenting concerns about the role of EFBs in mucormycosis infection, especially in the context of COVID-19 complications (Dogra et al., 2022). Recent studies have screened EFB-free strains of R. arrhizus to ensure food safety during fermentation (Hamza and Gunyar, 2022). The impact of EFB Ralstonia pickettii in R. microsporus on phagocyte evasion and opportunistic virulence has been reported (Itabangi et al., 2022). The presence of EFB Mycetohabitans rhizoxinica in a cancer patient further emphasizes the role of endosymbionts in the virulence of their host fungus R. microsporus (Yang et al., 2022). While mucormycosis is usually caused by co-infection of R. arrhizus and R. microsporus, the contribution of EFB to the pathogenesis of R. arrhizus remains to be confirmed.
To date, five EFB species have been detected in Rhizopus arrhizus, including two unnamed BREs and three named Gammaproteobacteria (Serratia marcescens, Pseudomonas fluorescens, and Klebsiella pneumoniae; Ibrahim et al., 2008; Itabangi et al., 2019; Birol and Gunyar, 2021). In this study, we confirmed the presence of two Burkholderiaceae EFBs in different parts of R. arrhizus through 16S rDNA sequencing and microscopic observation. To assess their potential impact on food safety and toxin production, we conducted a comparative analysis of their genetic background through whole-genome resequencing.
Materials and methods
Strains
This study utilized two strains, XY03792 and XY03829, of Rhizopus arrhizus. The XY03792, sourced from soy sauce in Malaysia, is a fermentative strain. The XY03829 is a wild strain obtained from animal dung in Pakistan. Both strains demonstrated the capability to ferment, resulting in the production of various compounds such as maltose, glucose, ethanol, lactic acid, fumaric acid, malic acid, glycerol, among others (Yao et al., 2018; Liu et al., 2022). These strains were preserved at Shandong Normal University under −20°C with 15% glycerine.
Manipulation and cultivation
To prevent bacterial contamination, sporangiospores underwent a meticulous two-step surface sterilization process following the method outlined by Becard and Fortin (1988). In the initial step, sporangiospores were immersed in a 0.05% Tween 20 solution for two minutes, followed by a 10 min soak in a 2% chloramine T solution. Subsequently, they were thoroughly rinsed three times with sterile distilled water. This soak and wash procedure was repeated once more, after which the sporangiospores were preserved in a sterile solution containing 200 mg/L streptomycin and 100 mg/L gentamicin at 4°C. Moving to the second step, the stored sporangiospores underwent another round of soaking in a 2% chloramine T solution and were washed with sterile distilled water immediately before inoculation. The surface-sterilized sporangiospores, treated through this two-step process, were then cultured on potato dextrose agar (PDA: 200 g/L potato, 20 g/L glucose, 20 g/L agar, and 1,000 mL distilled water) at 30°C.
DNA extraction, PCR, and sanger sequencing
To avoid bacterial contamination during incubation, the mycelia cultivated on PDA for 5 days underwent surface sterilization using 30% hydrogen peroxide, following the procedure outlined by Izumi et al. (2006). Metagenomic DNAs of Rhizopus arrhizus and its EFBs were extracted using the GOMag Rapid Plant DNA Kit (GO-GPLF-400, GeneOn BioTech, China). According to the manufacturer’s instructions, approximately 30 mg of thalli were successively lysed, adsorbed, washed, and eluted for metagenome extraction. An empty centrifuge tube served as a negative control. The 16S rDNA was amplified using primers 27F (5′-AGA GTT TGA TCC TGG CTC AG-3′) and 1541R (5′-AAG GAG GTG ATC CAG CC-3′). The PCR mixture (25.0 μL) included 1.0 μL of template DNA (10.0 ng/μL), 1.0 μL of the two primers each (10.0 μM), 12.5 μL of 2 × Taq PCR Master Mix (Biomed Diagnostics Pte Ltd., Singapore), and 9.5 μL of sterile deionized water (Caporaso et al., 2012). PCR amplification involved an initial step at 94°C for 5 min, followed by 35 cycles of 94°C for 30 s, 55°C for 30 s, and 72°C for 1 min, with a final extension at 72°C for 10 min. Sanger sequencing was carried out using the same primers (27F and 1541R) as used in PCR. Phylogenetic reconstruction employed the maximum likelihood (ML) and Bayesian inference (BI) methods through RAxML version 8.1.5 and MrBayes 3.2.7a, respectively (Ronquist et al., 2012; Stamatakis, 2014). Bootstrap supports (BS) for branches were obtained through 1,000 replicates (Estrada-De Los Santos et al., 2018). The resulting tree was edited online using the interactive Tree of Life platform (iTOL, https://itol.embl.de/itol.cgi; Letunic and Bork, 2019).
Visualizing EFB by microscopic observation
Live/dead staining
The Live/dead BacLight Bacterial Viability Kit (catalogue number L7012, Invitrogen, United States) was employed for the initial detection of EFBs following the method outlined by Arendt et al. (2016) and Takashima et al. (2018). Fresh hyphae and sporangiospores, obtained by scraping from the fungal colony on PDA, were deposited onto a glass slide along with 15.00 μL of a 1:1:200 mixed stain solution (SYTO9: propidium iodide: sterile 0.85% NaCl). Subsequently, cover slips were mounted onto the slide, and the preparation was incubated at room temperature in darkness for a few minutes. The stained hyphae and sporangiospores were then examined using an inverted fluorescence microscope (Axio observer Z1, Zeiss, Germany).
Fluorescence in situ hybridization
A probe (5′-CTT CCG GTA CCG TCA TCC CCC CGA GG-3′) labeled with Invitrogen Cyanine3 (Cy3) dye was designed for fluorescence in situ hybridization (FISH), targeting the 16S rDNA sequences specific to Pandoraea sputorum. FISH procedures were conducted following the method outlined by Hoffman and Arnold (2010). The general steps were as follows: Mycelia cultivated on PDA for 3 days were fixed at 4°C for 3 h using a 3:1 mixed fix solution of formalin (10%) and phosphate-buffered saline (PBS). The fixed mycelia were washed twice with PBS buffer and subsequently dehydrated with 50, 70, and 95% ethanol. The mycelia were then incubated with 8 μL of a 40% formamide hybridization stringency solution (800 μL formamide, 800 μL diethyl pyrocarbonate water, 500 μL 5 M EDTA) and 2 μL of the probe (10 μM) at 46°C for 1.5 h. Each sample underwent rinsing with 100 μL of wash buffer (460 μL 5 M NaCl, 1,000 μL 1 M Tris, 50 μL 10% SDS, made up to 50 mL with diethyl pyrocarbonate water) at 46°C. Fungal DNA was stained with 10 μL of 4,6-diamidine-2-phenylindole dihydrochloride (DAPI, Sigma) for 10 min and subsequently removed by washing with distilled water. Fluorescence images were captured using an inverted fluorescence microscope (Axio observer Z1, Zeiss, Germany). For the Cy3-labelled probe, the excitation and emission wavelengths were 550 nm and 580 nm, respectively (Naumann et al., 2010). For DAPI staining, the excitation and emission wavelengths were 358 nm and 461 nm, respectively (Guo et al., 2017).
Transmission electron microscopy
To precisely determine their specific location within the mycelium, EFB were visualized using transmission electron microscopy (TEM). A small mycelial pellet from a 3 days-old culture of Rhizopus arrhizus XY03829 was fixed with 0.1% glutaraldehyde/4% paraformaldehyde in 1× Phosphate Buffered Saline (PBS, pH 7.0) for 1 h at 25°C and subsequently overnight at 4°C. The pellets were then embedded in a drop of water agar and subjected to five washes with 1 × PBS. Further fixation was performed with a 1% (w/v) osmium tetraoxide (OsO4) solution for one hour. After three rinses with 1 × PBS, the samples underwent sequential dehydration in an ethanol series and were then immersed three times in 100% acetone. For infiltration, the samples were treated with a 3:1 acetone-resin mixture for 0.5 h, 1:1 for 1 h, and 1:3 for 1.5 h. Subsequently, the fungal samples were embedded in fresh Spurr resin and polymerized for 12 h at 70°C. Ultrathin sections were cut using an ultramicrotome (EM FC7, LEICA) and stained with uranyl acetate and lead citrate. The grids were examined using a JEM-1400Plus transmission electron microscope with an EM-14830RUBY2 charge-coupled device (CCD) camera (JEOL, Tokyo, Japan) at an acceleration voltage of 100 kV.
Isolation and identification of EFB
To isolate endosymbiotic bacteria, the host fungi were cultivated on PDA at 28°C for 3 days. A pellet of thalli, sterilized glass beads, and 1 mL lysogeny broth (LB: 10 g/L tryptone, 5 g/L yeast extract, 10 g/L NaCl) were added to a 2 mL centrifuge tube. The mixture was homogenized using a high-throughput tissue grinder (SCIENTZ-48) at 45 Hz for 30 s. The homogenized tissue fluid was then filtered through a 5 μM membrane and spread on lysogeny agar (LA: 10 g/L tryptone, 5 g/L yeast extract, 10 g/L NaCl, agar 30 g/L). Finally, it was incubated at 30°C for 7 days, and a single colony was transferred to another LA plate.
EFB genome sequencing and comparative genomic analysis
A single colony of EFBs grown on LA plates was inoculated into 15 mL of LB medium and shaken at 180 rpm at 37°C for 18 h. Bacterial cells were collected by centrifugation, and genomic DNAs were extracted using the Wizard Genomic DNA Purification Kit (A1120) following the manufacturer’s instructions. DNA integrity was verified on an agarose gel. Whole-genome resequencing was performed on the BENAGEN platform using Nanopore and Illumina NovaSeq PE150 platforms.
Raw data were assessed using FastQC 0.11.8 (Andrews, 2010) and Trimmomatic 0.39 (Bolger et al., 2014) for the filtration of low-quality reads, resulting in clean reads. The clean reads were assembled using MaSuRCA 3.4.3b (Zimin et al., 2013) and SPAdes 3.14 (Bankevich et al., 2012). Gene-coding models were predicted with Prokka (Seemann, 2014). For gene functional annotation, the predicted models were compared to various databases, including UniProt,1 NR,2 Pfam,3 KEGG,4 GO,5 CAZy,6 COG,7 CARD,8 and VFDB.9 Prophages, insertional sequences, and gene islands were predicted using PHASTER,10 ISFinder,11 and IslandViewer12, respectively.
Transposase and integrase sequences were sourced from the NCBI protein database, followed by clustering and classification using transposon and integron annotation databases, respectively. Initially, the Diamond blast+ software (version 0.9.31; Buchfink et al., 2015) was employed to compare genome and protein sequences against the Uniprot database. The outcomes of this comparison were integrated with the pre-constructed transposon and integron annotation databases to facilitate the prediction of transposons and integrons. Genomic collinearity analysis was conducted using the MAUVE (Darling et al., 2004). Subsequently, based on the results obtained from the Comprehensive Antibiotic Resistance Database (CARD) and the Virulence Factor Database (VFDB), a Venn diagram illustrating differences in gene numbers was generated using the Venny website.13
Two free-living Pandoraea sputorum strains, NCTC13161 (BioProject ID: PRJEB6403) and DSM21091 (BioProject ID: PRJNA262705), were downloaded from the NCBI database as references for P. sputorum EFB03792. The genome of EFB Mycetohabitans endofungorum HKI456 (BioProject ID: PRJNA370785) was used as the reference for M. endofungorum EFB03829.
Curing fungal strains and co-culturing with free-living EFB
The strains, preserved at −20°C with 15% glycerine, were inoculated on PDA plates supplemented with 100 μg/mL ampicillin, 50 μg/mL kanamycin, 10 μg/mL tetracycline, and 40 μg/mL ciprofloxacin. The plates were then incubated at 28°C for 36 h. Subcultures were performed under the same conditions for 30 generations. In each generation, the strain’s morphology was documented through photography and verified using live/dead staining. The cured fungal strain and free-living EFB isolated from the corresponding wild-type strain were simultaneously inoculated at the same position on lysogeny agar (LA) plates to observe whether the cured fungi resumed sporulation.
Results
Molecular detection and identification of EFBs
The 16S rDNA was successfully amplified from the metagenome of the two strains of the fungus Rhizopus arrhizus, resulting in a target fragment with a length of approximately 1.5 kb. These sequences were deposited in GenBank under the accession numbers OL413494 and OL413496. Identical 16S rDNA sequences were also annotated from the metagenome of the corresponding fungal strains. The maximum likelihood phylogenetic tree of EFBs based on 16S rDNA sequences is presented in Figure 1. In this phylogram, the two EFBs individually residing in the R. arrhizus strains XY03792 and XY03829 were grouped into the clades Pandoraea sputorum and Mycetohabitans endofungorum, respectively. Specifically, P. sputorum exhibited a close relationship with P. apista and P. norimbergensis, while M. endofungorum was closely related to M. rhizoxinica.
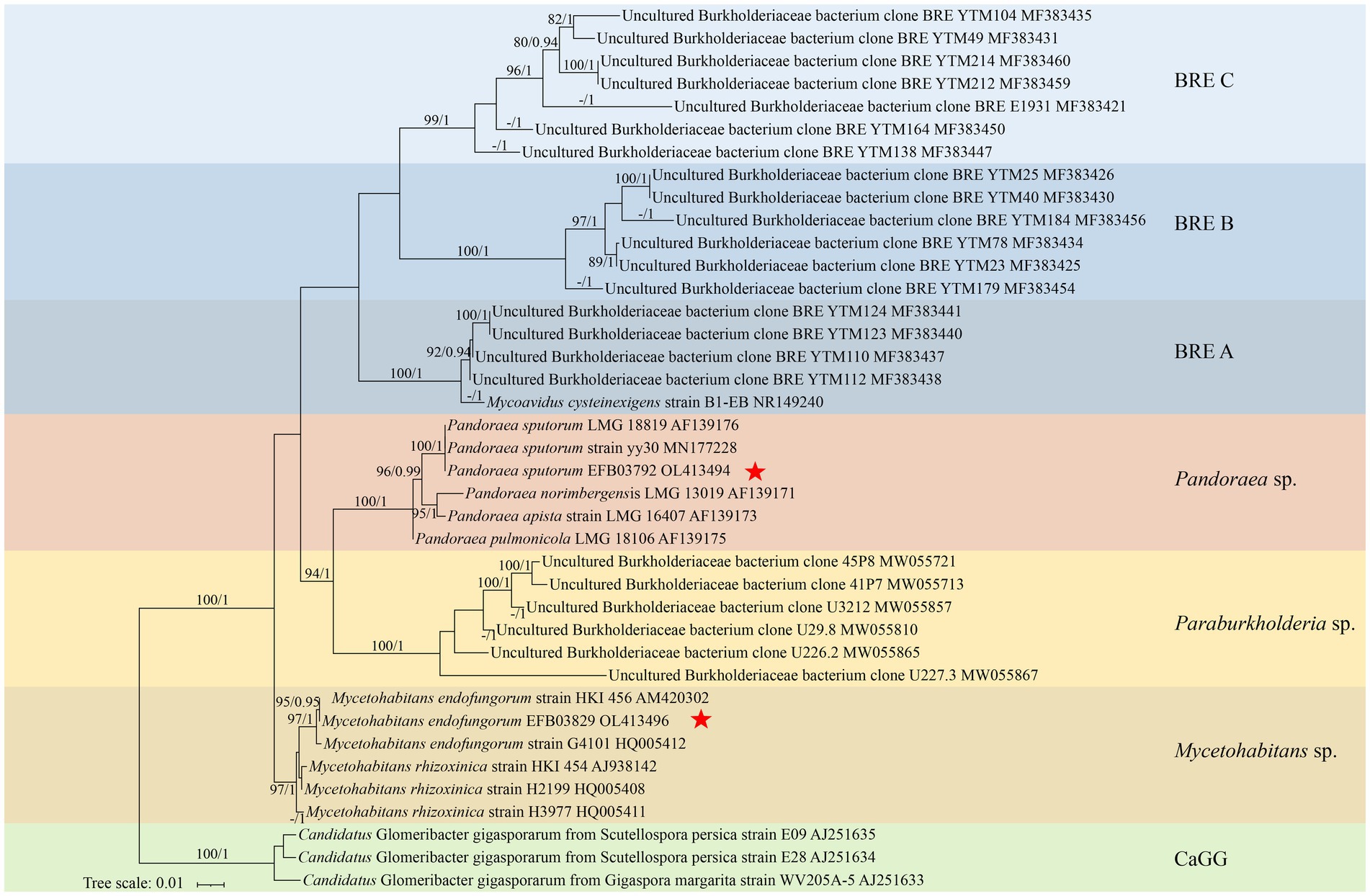
Figure 1. A maximum likelihood consensus phylogenetic tree of Burkholderia-related endobacteria (BRE) showing the placement of Pandoraea sputorum EFB03792 and Mycetohabitans endofungorum EFB03829. The Candidatus Glomeribacter gigasporarum was used as outgroup. All nodes with maximum likelihood bootstrap values (MLBV) and Bayesian inference posterior probabilities (BIPP) >80% and >0.90 are successively labelled and separated by a slash “/.” Sequences obtained herein are marked with a red star “*.” GenBank accession numbers are shown after the species name. Background colours indicate groups [blue, BRE (A–C); red, Mycetohabitans spp.; yellow, Paraburkholderia spp.; brown, Pandoraea spp.; green, Candidatus Glomeribacter gigasporarum]. The lower left bar represents 0.01 expected substitutions per site.
In situ detection of EFBs
Following live/dead staining, EFBs with green fluorescence were observed within the hyphae, columellae, and sporangiospores of both fungal strains (Figures 2A–I). Fluorescence in situ hybridization (FISH) revealed red fluorescence in the hyphae of Rhizopus arrhizus XY03792 (Figures 2K–N), while green fluorescence was observed in the hyphae of R. arrhizus XY03829 (data not shown), confirming the presence of specific EFBs. Transmission electron microscopy (TEM) images showed clear transverse sections of bacterial rods (Figure 2J), indicating the localization of EFBs within the cytoplasm of fungal mycelia rather than in vacuoles. The bacteria within the cytosol were distinguishable from fungal organelles due to their visible cell walls, nucleoids, reserve materials, and morphology. The cross-section size of these cells ranged from 0.6 to 0.8 μm in diameter.
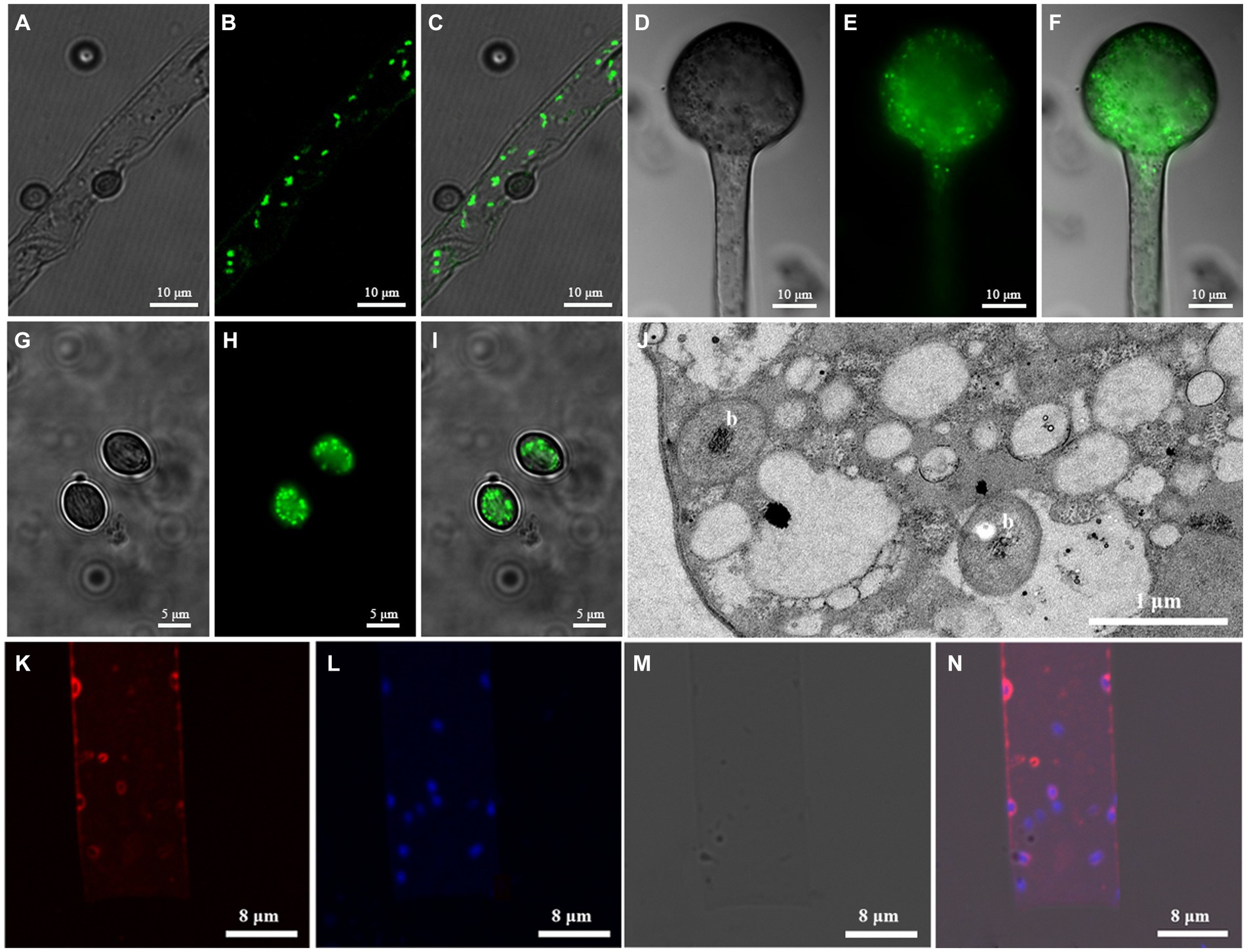
Figure 2. Microscopic images showing EFB living in Rhizopus arrhizus XY03792 and XY03829. (A–I) Live/dead staining images showing EFB living in XY03829. (A-C) Hyphae; (D-F) Columellae; (G-I) Sporangiospores; A/D/G, SYTO-9; B/E/H, DIC (Differential interference contrast); (C/F/I), Mixed image; (J) Transmission electron microscopy (TEM) images of EFB (marked with letter b) living in the mycelia of XY03829; (K–N) Fluorescence in situ hybridization images showing EFB living in XY03792. (K) Cy3; (L) DAPI; (M) DIC; (N) Mixed image.
General genome features of EFBs
The genome of Pandorea sputorum EFB03792 comprised approximately 1.00 Gb of clean data from the Nanopore sequencing platform, encompassing 22,138 reads with an average sequence read length of 45,173 bp, achieving full coverage (100%) and an average sequencing depth of around 171×. The Illumina-filtered clean data amounted to approximately 1.19 Gb, encompassing 7,962,964 reads with an average sequence read length of 150 bp, achieving full coverage (100%) and an average sequencing depth of approximately 203×. The assembled genome contained one circular chromosome spanning 5,845,363 bp with a GC content of 62.63%. A total of 5,215 gene models were encoded, including 5,099 coding sequences (CDS), 75 transfer RNA (tRNA), 12 ribosomal RNA (rRNA), and two transfer-messenger RNA (tmRNA) (Figure 3A and Table 1).
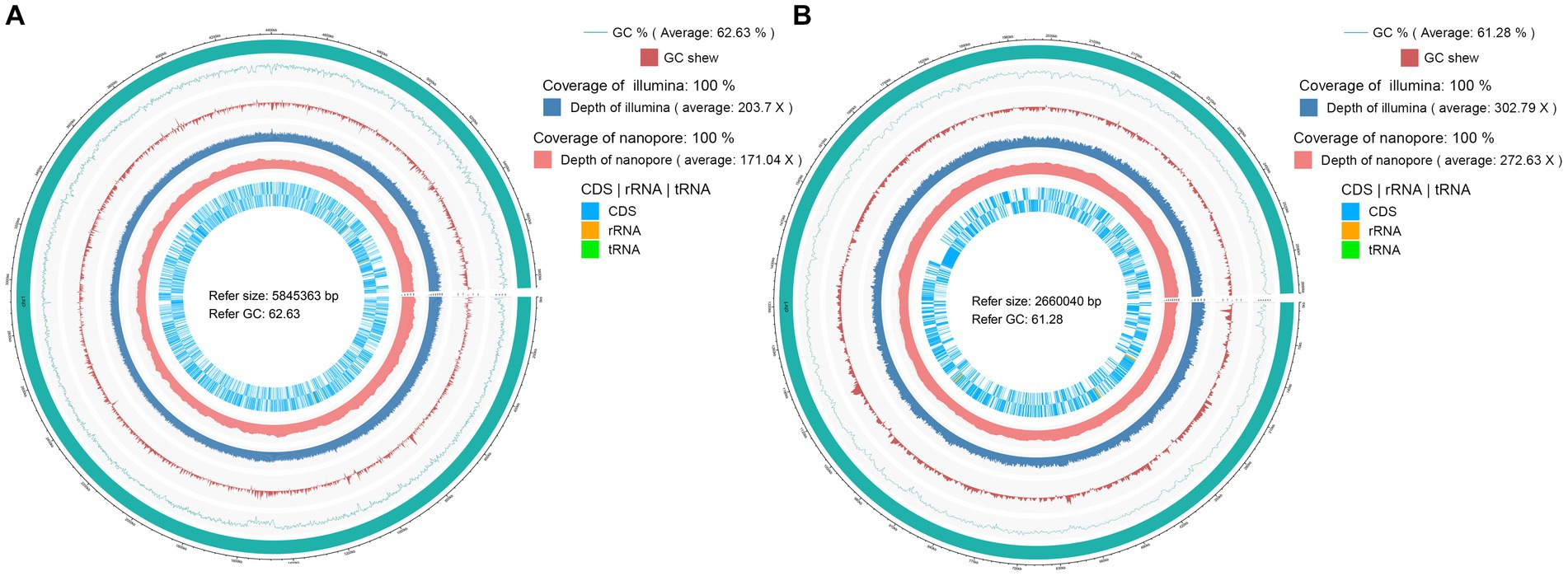
Figure 3. Circular maps of the complete genomes of two EFB associated with Rhizopus arrhizus. (A) Pandoraea sputorum EFB03792; (B) Mycetohabitans endofungorum EFB03829.
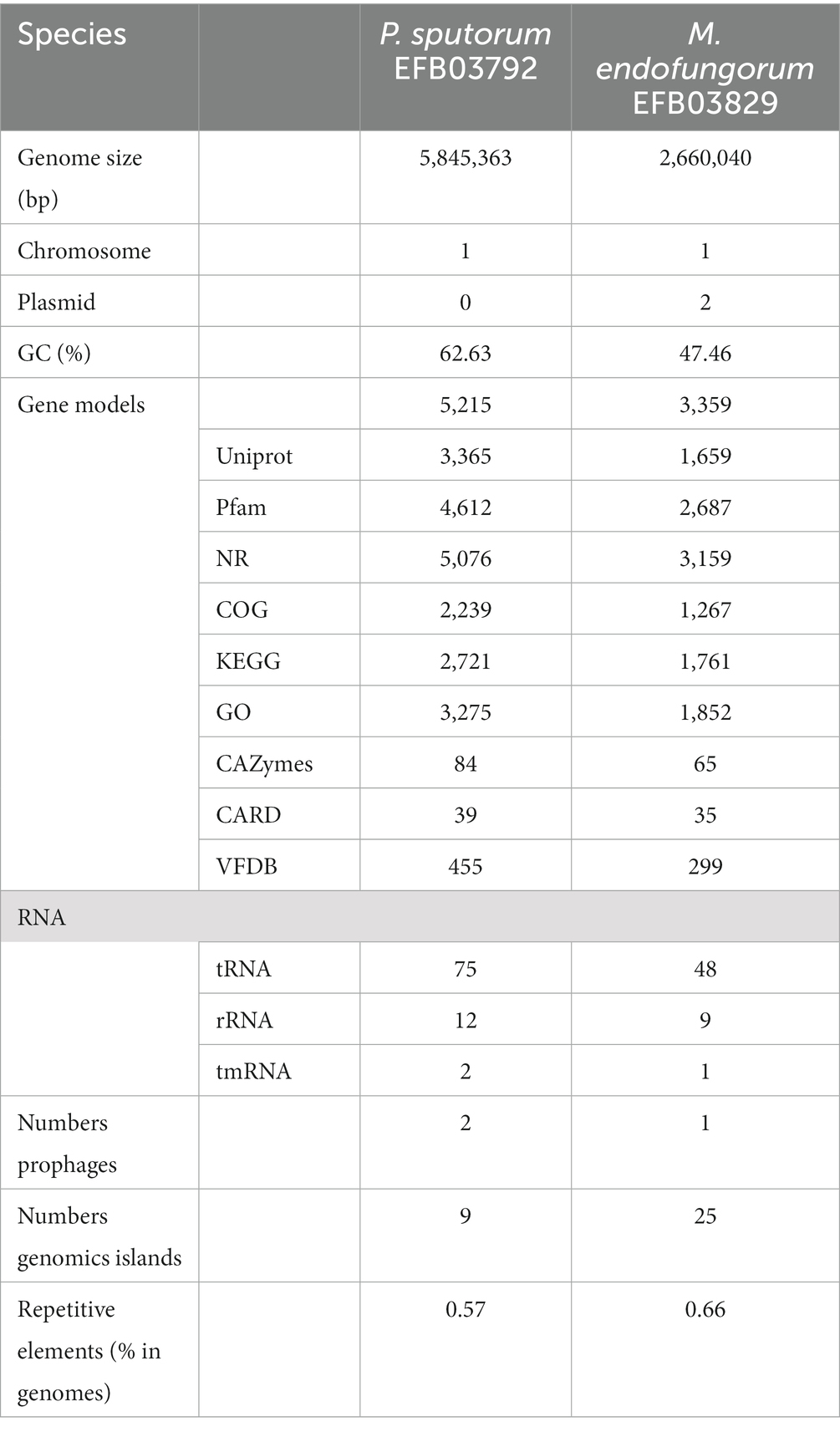
Table 1. Genomic features of Pandoraea sputorum and Mycetohabitans endofungorum sequenced and de novo assembled in this study.
The genome of Mycetohabitans endofungorum EFB03829 consisted of approximately 1.00 Gb of clean data from the Nanopore sequencing platform, encompassing 30,159 reads with an average sequence read length of 33,158 bp, achieving full coverage (100%) and an average sequencing depth of around 273×. The Illumina-filtered clean data amounted to 1.14 Gb, encompassing 7,598,064 reads with an average sequence read length of 150 bp, achieving full coverage (100%) and an average sequencing depth of approximately 303×. The assembled genome comprised one circular chromosome spanning 2,660,040 bp with a GC content of 61.28%. A total of 3,359 gene models were encoded, including 3,268 CDS, 48 tRNA, nine rRNA, and one tmRNA (Figure 3B and Table 1). Additionally, two plasmids were assembled, with Plasmid 1 measuring 800,149 bp long and exhibiting a GC content of 59.61%, and Plasmid 2 measuring 181,953 bp long with a GC content of 57.63%.
Functional annotations of genomes
Among the 5,215 gene models of the strain EFB03792 of Pandorea sputorum, 3,365, 4,612, 5,076, 2,239, 2,721, 3,275, 84, 39, and 455 genes were annotated with the UniProt, Pfam, NR, COG, KEGG, GO, CAZy, CARD, and VFDB databases, respectively (Table 1 and Supplementary File S1). In the case of Mycetohabitans endofungorum EFB03829, out of 3,359 gene models, 1,659, 2,687, 3,159, 1,267, 1,761, 1,852, 65, 35, and 299 genes were annotated with the UniProt, Pfam, NR, COG, KEGG, GO, CAZy, CARD, and VFDB databases, respectively (Table 1 and Supplementary File S2).
The COG annotation results (Supplementary Figure S1) indicated that M. endofungorum EFB03829 had fewer genes in all groups compared to P. sputorum EFB03792, except for mobile genes, which were more in EFB03792 than in EFB03829 (35 vs. 12, Supplementary Figure S1).
In terms of CAZy annotation, P. sputorum EFB03792 possessed 12 auxiliary activity genes (AAs), five carbohydrate-binding module genes (CBMs), 13 carbohydrate esterase genes (CEs), 19 glycoside hydrolase genes (GHs), 34 glycosyl transferase genes (GTs), and one polysaccharide lyase gene (PL; Supplementary File S3). M. endofungorum EFB03829 completely lost CBM and PL genes but had four AAs, five CEs, 18 GHs, and 38 GTs (Supplementary File S3).
CARD annotation revealed that P. sputorum EFB03792 possessed a unique drug resistance gene, specifically the FAD-containing monooxygenase EthA, which confers resistance to ethionamide through antibiotic target alteration. There were no differences in drug resistance genes between M. endofungorum EFB03829 and HKI45.
VFDB annotation results showed that P. sputorum EFB03792 and M. endofungorum EFB03829 had 656 and 309 virulence factor-related genes, respectively (Supplementary Files S4, S5). Among these genes, 28 were specific in P. sputorum EFB03792 and 25 were specific in M. endofungorum EFB03829 (Figures 4C,D and Supplementary Table S1).
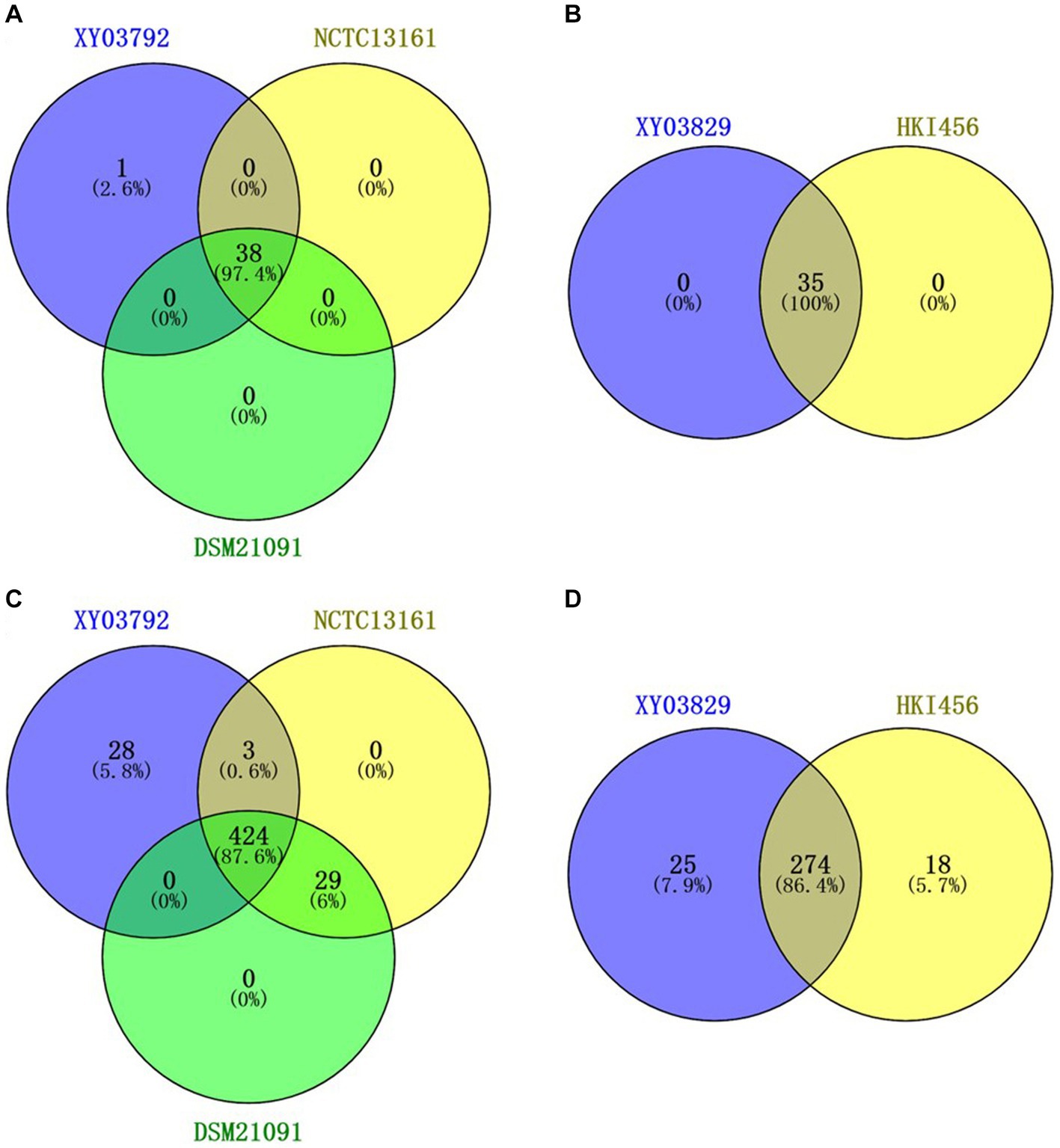
Figure 4. Venn diagram of different genes based on CARD (A,B) and VFDB (C,D) annotation. (A,C) Pandoraea sputorum strains EFB03792, NCTC13161, and DSM21091; (B,D) Mycetohabitans endofungorum strains EFB03892 and HKI456.
Additionally, two prophages were predicted in P. sputorum EFB03792, and one was predicted in M. endofungorum EFB03829 (Table 1). Nine and 25 genomic islands were annotated in P. sputorum EFB03792 and M. endofungorum EFB03829, respectively (Table 1).
Gene structure of EFBs
For Pandorea sputorum, compared with the free-living strains NCTC13161 and DSM21091, the endosymbiotic strain EFB03792 exhibited an inversion in the structure for more than half of its genes (Figure 5A) and contained a higher number of mobile elements (111 vs. 60; Table 1).
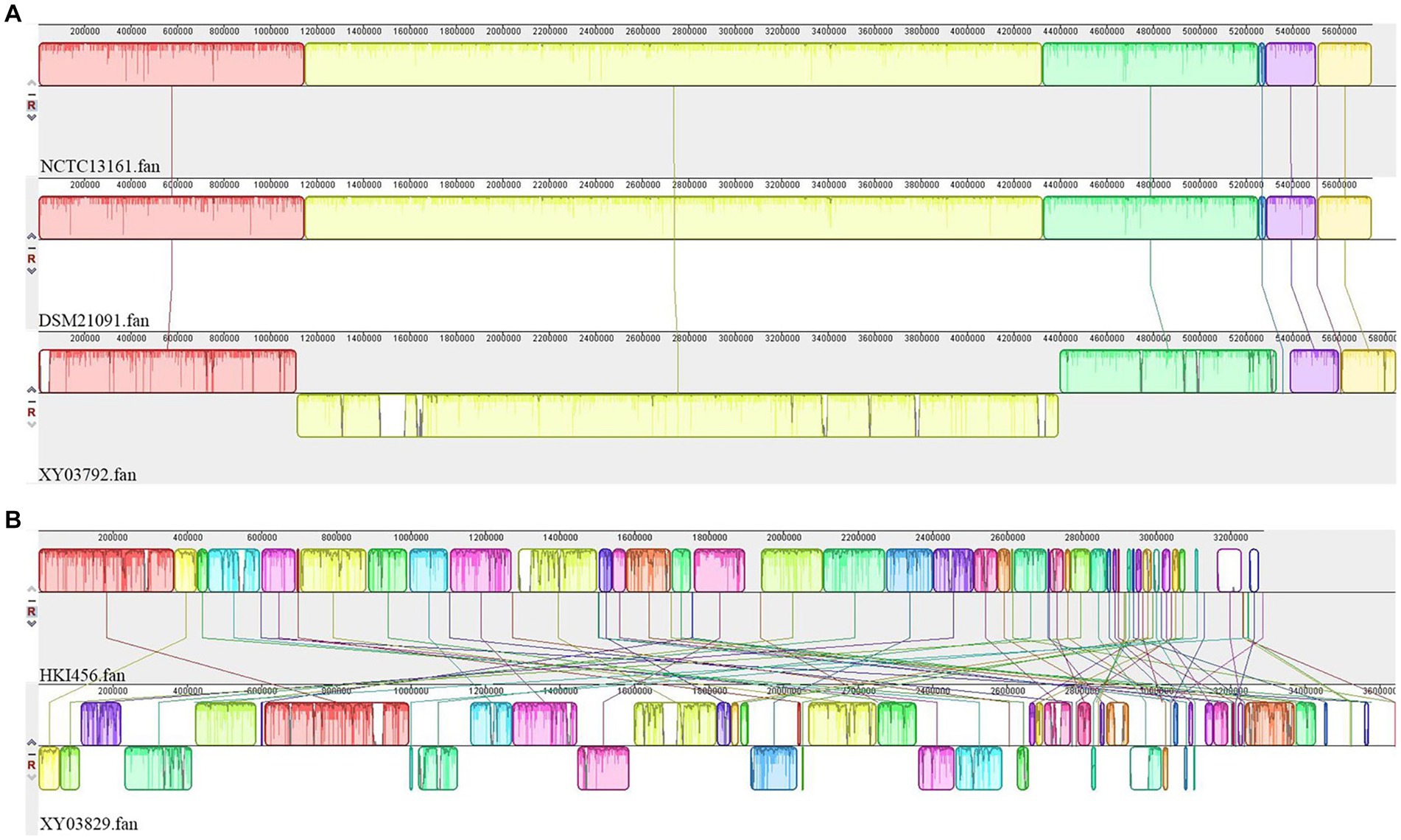
Figure 5. Collinearity analyses of EFB genomes. (A) Pandoraea sputorum; (B) Mycetohabitans endofungorum.
Concerning Mycetohabitans endofungorum, in comparison with the obligate endosymbiotic strain HKI456, the facultative endosymbiotic strain EFB03829 displayed a significant number of genes involved in inversion and/or translocation (Figure 5B) and contained a much larger number of mobile elements (1,517 vs. 392; Table 2).
CAZy analyses in EFBs
In this study, CAZy annotation was performed on five strains, namely Mycetohabitans endofungorum EFB03829, HKI456, Pandoraea sputorum EFB03792, DSM21091, and NCTC13161. The newly assembled genomes of M. endofungorum EFB03829 and P. sputorum EFB03792 were predicted with three and six CE10 family genes, respectively, while the other three genomes lacked (Figure 6). The CE10 family genes encoded some enzymes that catalyzed the hydrolysis of carboxylic ester bonds, such as acetyl-hydrolase, monoterpene epsilon-lactone hydrolase, acetyl esterase/lipase, and carboxylesterase.
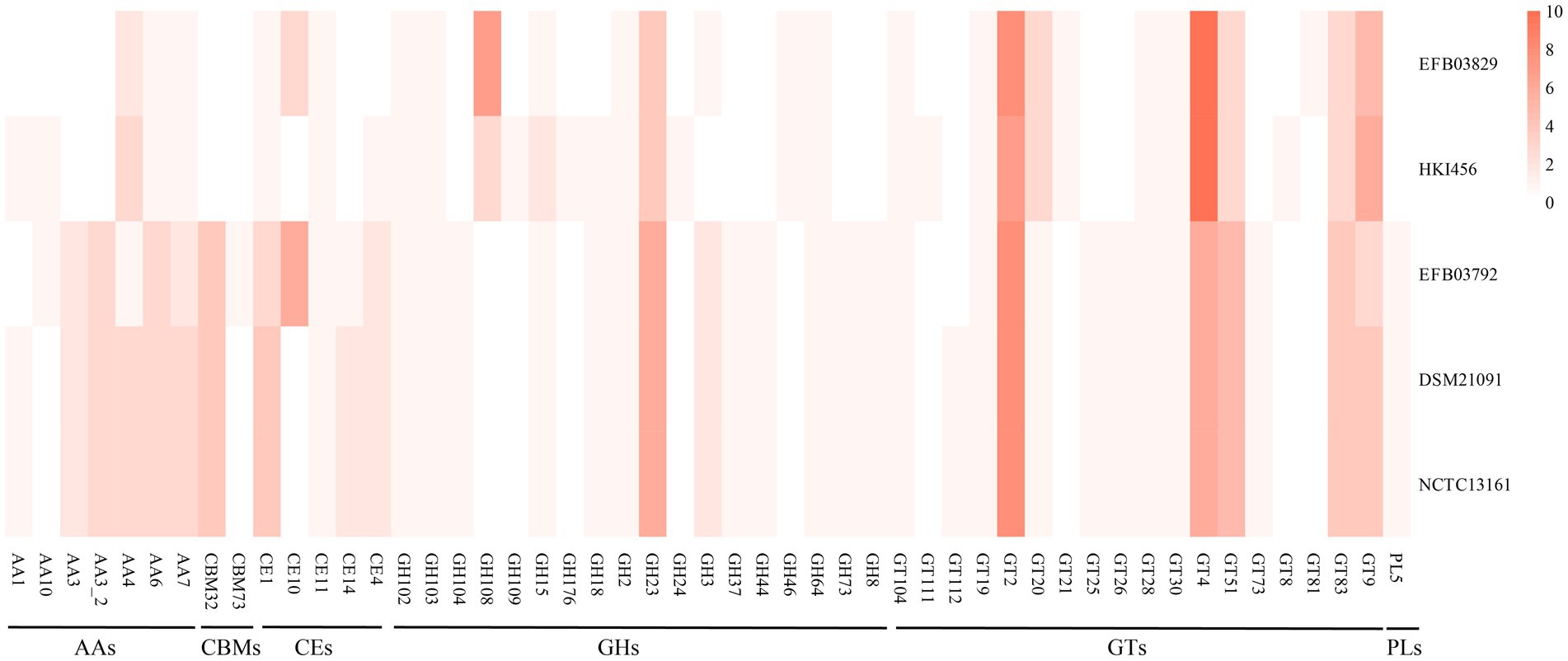
Figure 6. The genes number of CAZymes form Mycetohabitans endofungorum (EFB03829 and HKI456) and Pandoraea sputorum (EFB03792, DSM21091, and NCTC13161).
Fewer CAZy genes were identified in M. endofungorum (65–67) than in P. sputorum (84). Mycetohabitans endofungorum exhibited a complete loss of CBM (CBM32 and CBM73) and PL5 family genes compared to P. sputorum (Figure 6 and Supplementary File S3). The CBM32, CBM73, and PL5 families encoded beta-galactosidase, chitin binding, and alginate lyase, respectively. All strains possessed a rich abundance of CEs, GHs, and GTs (e.g., CE1, GH23, GT4, GT83, and GT9 family) genes (Figure 6). GH108 family genes were enriched in M. endofungorum (seven in EFB03829 and three in HKI456), but none in P. sputorum. These GH108 family genes were presumed to have a putative peptidoglycan binding domain and a predicted peptidoglycan domain.
Type III secretion system predicted in EFBs
In this study, 24 and 74 genes related to the type III secretion system (T3SS) were predicted from Mycetohabitans endofungorum EFB03829 and Pandorea sputorum EFB03792, respectively (Supplementary File S6), and M. endofungorum EFB03829 and P. sputorum EFB03792 have completely T3SS. The T3SS of M. endofungorum EFB03829 have five ATPase complexes, two basal bodies, four cytoplasmic rings, three export apparatuses, eight regulators, and two invasion protein genes. The T3SS of P. sputorum EFB03792 exhibited five ATPase complexes, eight basal bodies, six cytoplasmic rings, six export apparatuses, 45 regulators, and five invasion protein genes.
Morphological changes of cured fungal strains
Rhizopus arrhizus underwent continuous sub-culturing on a PDA plate containing antibiotics. Throughout the subculturing process, the sporangiophores of the strain XY03829 exhibited increased bending, and the production of sporangiospores gradually decreased. Starting from the 22nd generation, no sporangiospores were formed, and the strain could not recover to produce any sporangiospores (Figure 7). In contrast, the mycelial morphology of R. arrhizus XY03792 remained unchanged during the subculturing process.
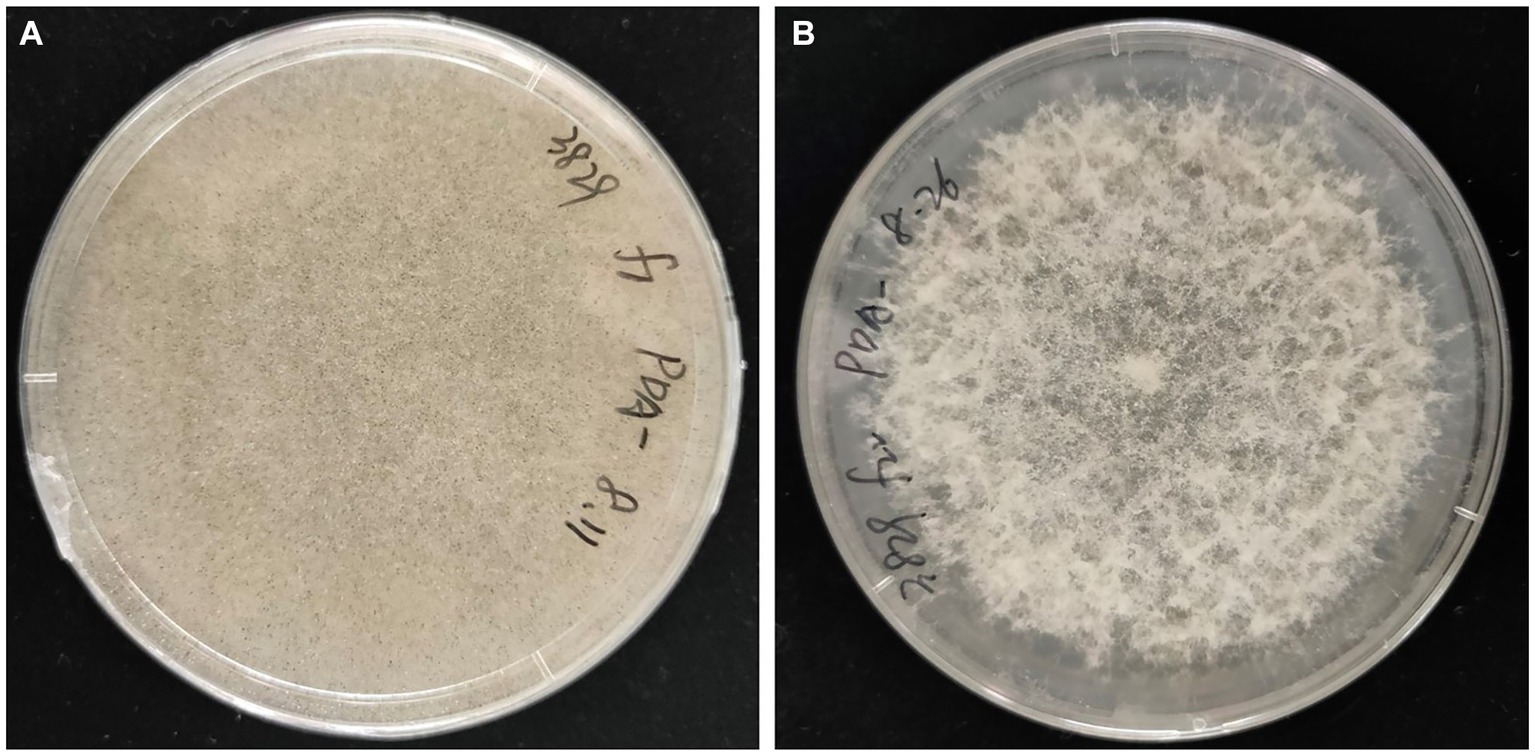
Figure 7. Mycelial morphologies of Rhizopus arrhizus XY03829. (A) Without antibiotic treatments; (B) Undergoing antibiotic treatments.
Discussion
New endofungal bacteria in Rhizopus
With the progress in exploring EFBs, an increasing number of Burkholderia-related endobacteria (BRE) and Mycoplasma-related endobacteria (MRE) have been identified in fungi, especially within the phylum Mucoromycota (Bianciotto et al., 2003; Partida-Martinez et al., 2007b; Sato et al., 2010; Okrasińska et al., 2021; Uehling et al., 2023). However, Pandoraea sputorum, a member of the family Burkholderiaceae, has never been previously detected within fungal hosts.
Pandoraea sputorum represents an emerging human pathogen known for inducing a pro-inflammatory response that can lead to lung dysfunction in individuals with cystic fibrosis (Xiao et al., 2019). This pathogenic microorganism has been exclusively isolated from respiratory tract sources (Pimentel and Macleod, 2008; Martínez-Lamas et al., 2011; Fernández-Olmos et al., 2012; Pugès et al., 2015; Kwizera et al., 2017) and blood samples (Xiao et al., 2019). Alongside this species, the pathogenic genus Pandoraea includes ten other species (Xiao et al., 2019). While these species have been identified in various specimens, such as sputum, blood, urine, lung tissue, and wounds, they have not been observed within fungi. Therefore, this study marks the initial proposal of the pathogenic bacterium Pandoraea as an EFB genus, particularly thriving within a potential pathogenic fungus of Rhizopus arrhizus. This underscores the heightened relevance of this genus in the field of medicine.
It has been reported that the EFB Mycetohabitans rhizoxinica plays a role in enhancing sporulation in the host fungus Rhizopus microsporus (Partida-Martinez et al., 2007c). In this study, we detected EFB M. endofungorum in the host fungus R. arrhizus, a species closely related to R. microsporus (Liu et al., 2008). The cured R. arrhizus exhibited impaired growth and an inability to produce sporangiospores, underscoring the essential role of EFB M. endofungorum in the growth and reproduction of R. arrhizus. Attempts to restore sporulation through co-culturing on LB plates were unsuccessful, likely attributed to the limited infectivity of EFB03829 on the host. Laser-mediated microinjection (Partida-Martinez et al., 2007c) emerges as a potential superior method for the reintroduction of M. endofungorum into its host R. arrhizus.
Gene structure and specific genes of the two EFBs
Based on the complete assembly genome sequences available in the NCBI database14 for the Burkholderia genus, it is observed that their genome sizes span a range from 5.23 Mb to 10.63 Mb. Notably, the genome of Mycetohabitans endofungorum EFB03829 is markedly reduced, measuring only 3.64 Mb (composed of a 2,660,040 bp of chromosome, 800,149 bp of plasmid 1, and 181,953 bp of plasmid 2). This represents a significant reduction when compared to its Burkholderia spp. counterparts. While the genome of Pandoraea sputorum EFB03792 (5.85 Mb) does not exhibit notable streamlining when compared to other strains of the same species of P. sputorum, which range from 5.74 Mb to 6.45 Mb (see footnote 14). The prevailing consensus is that obligate endosymbionts undergo genome reduction as an adaptation to their reliance on host-derived resources (Uehling et al., 2023). In contrast, facultative endosymbiotic bacteria are generally not subject to genome reduction (Baltrus et al., 2017). Both M. endofungorum and P. sputorum are facultative endosymbiotic bacteria, demonstrating the ability to thrive not only within fungal mycelia but also on artificial media. Surprisingly, M. endofungorum EFB03829 exhibited a remarkable genome reduction.
According to Salvioli et al. (2017), the genome of Mycetohabitans rhizoxinica includes toxin-antitoxin modules (TAs), which involve in modulating growth under stress conditions and promoting survival in host cells. M. endofungorum also harbors TAs in its genome, potentially influencing the regulation of fungal endobacteria life (Lackner et al., 2011b; Salvioli et al., 2017). The genomes of both M. endofungorum and Pandorea sputorum strains encompass genes associated with virulence factors. And M. endofungorum has demonstrated the ability to produce the toxin rhizonin, exhibiting significant nonspecific hepatotoxicity (Partida-Martinez et al., 2007a). Previous research has indicated that the two R. arrhizus strains under investigation can undergo fermentation to generate glucose and lactic acid for food fermentation purposes (Liu et al., 2022). Consequently, the potential EFBs may pose a risk of toxin production in fermented foods.
The analyses of collinearity indicated a more pronounced change in the gene structure of M. endofungorum EFB03829 compared to P. sputorum EFB03792 (Figure 5). Furthermore, M. endofungorum EFB03829 possesses a significantly higher number of mobile elements (1,517) than M. endofungorum HKI456 (392) and P. sputorum (60–111; Table 1). The repeated insertion and loss of mobile elements, including prophages, can contribute to genome reduction and alterations in gene structure (Vale et al., 2022). Thus, the substantial presence of mobile genetic elements in M. endofungorum EFB03829 is implicated in its genome reduction and structural changes.
CAZymes, or Carbohydrate-Active Enzymes, play a key role in metabolism, involved in the synthesis, modification, and degradation of carbohydrates, including polysaccharides, glycoproteins, and glycolipids (Drula et al., 2022). Our results suggested that the newly sequenced genomes were annotated several CE10 family genes, suggesting their involvement in the metabolism of various compounds in the host R. arrhizus, including drugs, pesticides, and lipids.
The type III secretion system (T3SS) plays a vital role in maintaining symbiosis and is highly conserved in the genomes of Gram-negative pathogenic or symbiotic bacteria (Deng et al., 2017), such as endosymbiont Candidatus Glomeribacter gigasporarum associated with the arbuscular mycorrhizal fungus (AMF) Gigaspora margaritain (Ghignone et al., 2012) and Burkholderia rhizoxinica in the zygomycetous fungus Rhizopus microsporus (Lackner et al., 2011a). In our study, we predicted 74 and 24 genes related to T3SS in P. sputorum EFB03792 and M. endofungorum EFB03829, respectively, indicating their role as symbiotic bacteria with Rhizopus arrhizus. The identification of these specific genes in our study contributes to a better understanding of the mechanisms underlying the actions of symbiotic bacteria in fungi during invasion and colonization stages.
Conclusion
This study presents a comprehensive investigation of two bacterial species, Pandoraea sputorum EFB03792 and Mycetohabitans endofungorum EFB03829, in association with Rhizopus arrhizus strains based on live/dead staining, FISH, TEM, and 16S rDNA sequencing. The wild-type R. arrhizus strains underwent more than 22 sub-cultures on a medium containing antibiotics. The results showed that M. endofungorum could control the sporulation of R. arrhizus, while P. sputorum had no significant effect on the morphology of R. arrhizus. The genome sequencing results indicate that M. endofungorum EFB03829 underwent genome reduction, resulting in a smaller genome size compared to P. sputorum EFB03792. Despite its reduced genome, EFB03829 contains more mobile genetic elements. Gene annotation revealed the presence of toxin genes in both EFBs. This raises potential safety concerns for food fermentation involving R. arrhizus, as the presence of toxin genes in these endofungal bacteria may pose risks during the fermentation process. The study provides valuable insights into the interactions between EFBs and R. arrhizus, highlighting the need for careful consideration of safety aspects in food fermentation processes involving these microorganisms.
Data availability statement
The datasets presented in this study can be found in online repositories. The names of the repository/repositories and accession number(s) can be found at: https://www.ncbi.nlm.nih.gov/genbank/, PRJNA1046224.
Author contributions
X-LL: Conceptualization, Data curation, Methodology, Writing – original draft, Writing – review & editing. HZ: Conceptualization, Data curation, Investigation, Methodology, Software, Writing – review & editing. Y-XW: Formal analysis, Investigation, Writing – review & editing. X-YeL: Formal analysis, Investigation, Writing – review & editing. YJ: Formal analysis, Investigation, Writing – review & editing. M-FT: Formal analysis, Investigation, Writing – original draft, Writing – review & editing. X-YoL: Funding acquisition, Methodology, Project administration, Writing – review & editing.
Funding
The author(s) declare financial support was received for the research, authorship, and/or publication of this article. This study was supported by the National Natural Science Foundation of China (Grant Nos. 31970009 and 32170012).
Acknowledgments
The authors thank the peer reviewers for their helpful comments on the manuscript.
Conflict of interest
The authors declare that the research was conducted in the absence of any commercial or financial relationships that could be construed as a potential conflict of interest.
Publisher’s note
All claims expressed in this article are solely those of the authors and do not necessarily represent those of their affiliated organizations, or those of the publisher, the editors and the reviewers. Any product that may be evaluated in this article, or claim that may be made by its manufacturer, is not guaranteed or endorsed by the publisher.
Supplementary material
The Supplementary material for this article can be found online at: https://www.frontiersin.org/articles/10.3389/fmicb.2024.1346252/full#supplementary-material
Footnotes
2. ^https://www.ncbi.nlm.nih.gov/protein/
7. ^https://www.ncbi.nlm.nih.gov/research/cog
11. ^https://www-is.biotoul.fr/
12. ^https://www.pathogenomics.sfu.ca/islandviewer/
References
Arendt, K. R., Hockett, K. L., Araldi-Brondolo, S. J., Baltrus, D. A., and Arnold, A. E. (2016). Isolation of endohyphal bacteria from foliar ascomycota and in vitro establishment of their symbiotic associations. Appl. Environ. Microbiol. 82, 2943–2949. doi: 10.1128/AEM.00452-16
Baltrus, D. A., Dougherty, K., Arendt, K. R., Huntemann, M., Clum, A., Pillay, M., et al. (2017). Absence of genome reduction in diverse, facultative endohyphal bacteria. Microb. Genom. 3:e000101. doi: 10.1099/mgen.0.000101
Bankevich, A., Nurk, S., Antipov, D., Gurevich, A. A., Dvorkin, M., Kulikov, A. S., et al. (2012). SPAdes: a new genome assembly algorithm and its applications to single-cell sequencing. J. Comput. Biol. 19, 455–477. doi: 10.1089/cmb.2012.0021
Becard, G., and Fortin, J. (1988). Early events of vesicular-arbuscular mycorrhiza formation on Ri T-DNA transformed roots. New Phytol. 108, 211–218. doi: 10.1111/j.1469-8137.1988.tb03698.x
Bianciotto, V., Lumini, E., Bonfante, P., and Vandamme, P. (2003). Candidatus glomeribacter gigasporarum gen. Nov., sp. nov., an endosymbiont of arbuscular mycorrhizal fungi. Int. J. Syst. Evol. Microbiol. 53, 121–124. doi: 10.1099/ijs.0.02382-0
Birol, D., and Gunyar, O. A. (2021). Investigation of presence of endofungal bacteria in Rhizopus spp. isolated from the different food samples. Arch. Microbiol. 203, 2269–2277. doi: 10.1007/s00203-021-02251-4
Bolger, A. M., Lohse, M., and Usadel, B. (2014). Trimmomatic: a flexible trimmer for illumina sequence data. Bioinformatics 30, 2114–2120. doi: 10.1093/bioinformatics/btu170
Bonfante, P., and Desirò, A. (2017). Who lives in a fungus? The diversity, origins and functions of fungal endobacteria living in mucoromycota. ISME J. 11, 1727–1735. doi: 10.1038/ismej.2017.21
Buchfink, B., Xie, C., and Huson, D. H. (2015). Fast and sensitive protein alignment using DIAMOND. Nat. Methods 12, 59–60. doi: 10.1038/nmeth.3176
Büttner, H., Pidot, S. J., Scherlach, K., and Hertweck, C. (2023). Endofungal bacteria boost anthelminthic host protection with the biosurfactant symbiosin. Chem. Sci. 14, 103–112. doi: 10.1039/d2sc04167g
Caporaso, J. G., Lauber, C. L., Walters, W. A., Berg-Lyons, D., Huntley, J., Fierer, N., et al. (2012). Ultra-high-throughput microbial community analysis on the Illumina hiseq and miseq platforms. ISME J. 6, 1621–1624. doi: 10.1038/ismej.2012.8
Cappelli, A., Damiani, C., Capone, A., Bozic, J., Mensah, P., Clementi, E., et al. (2023). Tripartite interactions comprising yeast-endobacteria systems in the gut of vector mosquitoes. Front. Microbiol. 14:1157299. doi: 10.3389/fmicb.2023.1157299
Cheng, Y., Gao, Y., Liu, X. Y., Wang, G. Y., Zhang, G. Q., and Gao, S. Q. (2017). Rhinocerebral mucormycosis caused by Rhizopus arrhizus var. tonkinensis. J. Mycol. Médicale 27, 586–588. doi: 10.1016/j.mycmed.2017.10.001
Cheng, S., Jiang, J. W., Tan, L. T., Deng, J. X., Liang, P. Y., Su, H., et al. (2022). Plant growth-promoting ability of mycorrhizal Fusarium strain kb-3 enhanced by its IAA producing endohyphal bacterium, Klebsiella aerogenes. Front. Microbiol. 13:855399. doi: 10.3389/fmicb.2022.855399
Darling, A. C. E., Mau, B., Blattner, F. R., and Perna, N. T. (2004). Mauve: multiple alignment of conserved genomic sequence with rearrangements. Genome Res. 14, 1394–1403. doi: 10.1101/gr.2289704
Deng, W., Marshall, N. C., Rowland, J. L., McCoy, J. M., Worrall, L. J., Santos, A. S., et al. (2017). Assembly, structure, function and regulation of type III secretion systems. Nat. Rev. Microbiol. 15, 323–337. doi: 10.1038/nrmicro.2017.20
Desirò, A., Faccio, A., Kaech, A., Bidartondo, M. I., and Bonfante, P. (2015). Endogone, one of the oldest plant-associated fungi, host unique mollicutes-related endobacteria. New Phytol. 205, 1464–1472. doi: 10.1111/nph.13136
Deveau, A., Bonito, G., Uehling, J., Paoletti, M., Becker, M., Bindschedler, S., et al. (2018). Bacterial–fungal interactions: ecology, mechanisms and challenges. FEMS Microbiol. Rev. 42, 335–352. doi: 10.1093/femsre/fuy008
Dogra, S., Arora, A., Aggarwal, A., Passi, G., Sharma, A., Singh, G., et al. (2022). Mucormycosis amid covid-19 crisis: pathogenesis, diagnosis, and novel treatment strategies to combat the spread. Front. Microbiol. 12:794176. doi: 10.3389/fmicb.2021.794176
Drula, E., Garron, M., Dogan, S., Lombard, V., Henrissat, B., and Terrapon, N. (2022). The carbohydrate-active enzyme database: functions and literature. Nucleic Acids Res. 50, D571–D577. doi: 10.1093/nar/gkab1045
Estrada-De Los Santos, P., Palmer, M., Chavez-Ramirez, B., Beukes, C., Steenkamp, E. T., Briscoe, L., et al. (2018). Whole genome analyses suggests that Burkholderia sensu lato contains two additional novel genera (Mycetohabitans gen. Nov., and Trinickia gen. Nov.): implications for the evolution of diazotrophy and nodulation in the Burkholderiaceae. Genes. 9:389. doi: 10.3390/genes9080389
Fernández-Olmos, A., Morosini, M. I., Lamas, A., García-Castillo, M., García-García, L., Cantón, R., et al. (2012). Clinical and microbiological features of a cystic fibrosis patient chronically colonized with Pandoraea sputorum identified by combining 16S rRNA sequencing and matrix-assisted laser desorption ionization-time of flight mass spectrometry. J. Clin. Microbiol. 50, 1096–1098. doi: 10.1128/JCM.05730-11
Ghasemi, S., Harighi, B., and Ashengroph, M. (2023). Biosynthesis of silver nanoparticles using Pseudomonas canadensis, and its antivirulence effects against Pseudomonas tolaasii, mushroom brown blotch agent. Sci. Rep. 13:3668. doi: 10.1038/s41598-023-30863-x
Ghignone, S., Salvioli, A., Anca, I., Lumini, E., Ortu, G., Petiti, L., et al. (2012). The genome of the obligate endobacterium of an AM fungus reveals an interphylum network of nutritional interactions. ISME J. 6, 136–145. doi: 10.1038/ismej.2011.110
Guo, H. J., Glaeser, S. P., Alabid, I., Imani, J., Haghighi, H., Kampfer, P., et al. (2017). The abundance of endofungal bacterium Rhizobium radiobacter (syn. Agrobacterium tumefaciens) increases in its fungal host Piriformospora indica during the tripartite sebacinalean symbiosis with higher plants. Front. Microbiol. 8:629. doi: 10.3389/fmicb.2017.00629
Guo, Y., and Narisawa, K. (2018). Fungus-bacterium symbionts promote plant health and performance. Microbes Environ. 33, 239–241. doi: 10.1264/jsme2.ME3303rh
Hamza, A. A., and Gunyar, O. A. (2022). Functional properties of Rhizopus oryzae strains isolated from agricultural soils as a potential probiotic for broiler feed fermentation. World J. Microbiol. Biotechnol. 38:41. doi: 10.1007/s11274-021-03225-w
Hoffman, M. T., and Arnold, A. E. (2010). Diverse bacteria inhabit living hyphae of phylogenetically diverse fungal endophytes. Appl. Environ. Microbiol. 76, 4063–4075. doi: 10.1128/AEM.02928-09
Ibrahim, A. S., Gebremariam, T., Liu, M., Chamilos, G., Kontoyiannis, D., Mink, R., et al. (2008). Bacterial endosymbiosis is widely present among zygomycetes but does not contribute to the pathogenesis of mucormycosis. J. Infect. Dis. 198, 1083–1090. doi: 10.1086/591461
Itabangi, H., Sephton-Clark, P. C. S., Tamayo, D. P., Zhou, X., Starling, G. P., Mahamoud, Z., et al. (2022). A bacterial endosymbiont of the fungus Rhizopus microsporus drives phagocyte evasion and opportunistic virulence. Curr. Biol. 32, 1115–1130. doi: 10.1016/j.cub.2022.01.028
Itabangi, H., Sephton-Clark, P. C. S., Zhou, X., Insua, I., Probert, M., Correia, J., et al. (2019). A bacterial endosymbiont enables fungal immune evasion during fatal mucormycete infection. bioRxiv 101:584607. doi: 10.1101/584607
Izumi, H., Anderson, I. C., Alexander, I. J., Killham, K. S., and Moore, E. (2006). Endobacteria in some ectomycorrhiza of scots pine (Pinus sylvestris). FEMS Microbiol. Ecol. 56, 34–43. doi: 10.1111/j.1574-6941.2005.00048.x
Kwizera, R., Parkes-Ratanshi, R., Page, I. D., Sekaggya-Wiltshire, C., Musaazi, J., Fehr, J., et al. (2017). Elevated aspergillus-specific antibody levels among HIV infected ugandans with pulmonary tuberculosis. BMC Pulm. Med. 17:149. doi: 10.1186/s12890-017-0500-9
Lackner, G., Moebius, N., and Hertweck, C. (2011a). Endofungal bacterium controls its host by an hrp type III secretion system. ISME J. 5, 252–261. doi: 10.1038/ismej.2010.126
Lackner, G., Moebius, N., Partida-Martinez, L. P., Boland, S., and Hertweck, C. (2011b). Evolution of an endofungal lifestyle: deductions from the Burkholderia rhizoxinica genome. BMC Genomics 12:210. doi: 10.1186/1471-2164-12-210
Lastovetsky, O. A., Krasnovsky, L. D., Qin, X., Gaspar, M. L., Gryganskyi, A. P., Huntemann, M., et al. (2020). Molecular dialogues between early divergent fungi and bacteria in an antagonism versus a mutualism. MBio 11:e02088. doi: 10.1128/mbio.02088-20
Letunic, I., and Bork, P. (2019). Interactive tree of life (iTOL) v4: recent updates and new developments. Nucleic Acids Res. 47, W256–W259. doi: 10.1093/nar/gkz239
Liu, X. Y., Huang, H., and Zheng, R. Y. (2008). Delimitation of Rhizopus varieties based on IGS rDNA sequences. Sydowia 60, 93–112. doi: 10.1074/jbc.271.14.8126
Liu, X. L., Ju, X., Jia, B. S., Qiao, T. Y., and Liu, X. Y. (2022). The correlation of fermentation metabolites with sporulation capability and variety in Rhizopus arrhizus. Acta Microbiol Sin. 62, 1131–1149. doi: 10.13343/j.cnki.wsxb.20210409
Liu, Z., Sun, X., Liu, X. L., Jia, B. S., and Liu, X. Y. (2019). Research progress on endofungal bacteria. Mycosystema 38, 1581–1599. doi: 10.13346/j.mycosystema.190286
MacDonald, R., and Chandler, M. R. (1981). Bacterium-like organelles in the vesicular-arbuscular mycorrhizal fungus glomus caledonius. New Phytol. 89, 241–246. doi: 10.1111/j.1469-8137.1981.tb07486.x
Martínez-Lamas, L., Rabade Castedo, C., Martín Romero Domínguez, M., Barbeito Castiñeiras, G., Palacios Bartolomé, A., and Pérez Del Molino Bernal, M. L. (2011). Colonización por Pandoraea sputorum en un paciente con fibrosis quística. Arch. Bronconeumol. 47, 571–574. doi: 10.1016/j.arbres.2011.06.015
Mondo, S. J., Lastovetsky, O. A., Gaspar, M. L., Schwardt, N. H., Barber, C. C., Riley, R., et al. (2017). Bacterial endosymbionts influence host sexuality and reveal reproductive genes of early divergent fungi. Nat. Commun. 8:1843. doi: 10.1038/s41467-017-02052-8
Mondo, S. J., Toomer, K. H., Morton, J. B., Lekberg, Y., and Pawlowska, T. E. (2012). Evolutionary stability in a 400-million-year-old heritable facultative mutualism. Evolution 66, 2564–2576. doi: 10.1111/j.1558-5646.2012.01611.x
Mosse, B. (1970). Honey-coloured, sessile endogone spores: II. Changes in fine structure during spore development. Arch. Microbiol. 74, 129–145. doi: 10.1007/BF00446901
Muszewska, A., Okrasinska, A., Steczkiewicz, K., Drgas, O., Orlowska, M., Perlinska-Lenart, U., et al. (2021). Metabolic potential, ecology and presence of associated bacteria is reflected in genomic diversity of mucoromycotina. Front. Microbiol. 12:636986. doi: 10.3389/fmicb.2021.636986
Naumann, M., Schussler, A., and Bonfante, P. (2010). The obligate endobacteria of arbuscular mycorrhizal fungi are ancient heritable components related to the Mollicutes. ISME J. 4, 862–871. doi: 10.1038/ismej.2010.21
Niehs, S. P., Scherlach, K., Dose, B., Uzum, Z., Stinear, T. P., Pidot, S. J., et al. (2022). A highly conserved gene locus in endofungal bacteria codes for the biosynthesis of symbiosis-specific cyclopeptides. PNAS Nexus. 1, 1–8. doi: 10.1093/pnasnexus/pgac152
Okrasińska, A., Bokus, A., Duk, K., Gęsiorska, A., Sokołowska, B., Miłobędzka, A., et al. (2021). New endohyphal relationships between mucoromycota and burkholderiaceae representatives. Appl. Environ. Microbiol. 87, e02707–e02720. doi: 10.1128/AEM.02707-20
Partida-Martinez, L. P., De Looss, C. F., Ishida, K., Ishida, M., Roth, M., Buder, K., et al. (2007a). Rhizonin, the first mycotoxin isolated from the Zygomycota, is not a fungal metabolite but is produced by bacterial endosymbionts. Appl. Environ. Microbiol. 73, 793–797. doi: 10.1128/AEM.01784-06
Partida-Martinez, L. P., Groth, I., Schmitt, I., Richter, W., Roth, M., and Hertweck, C. (2007b). Burkholderia rhizoxinica sp. nov. and Burkholderia endofungorum sp. nov., bacterial endosymbionts of the plant-pathogenic fungus Rhizopus microsporus. Int. J. Syst. Evol. Microbiol. 57, 2583–2590. doi: 10.1099/ijs.0.64660-0
Partida-Martinez, L. P., and Hertweck, C. (2005). Pathogenic fungus harbours endosymbiotic bacteria for toxin production. Nature 437, 884–888. doi: 10.1038/nature03997
Partida-Martinez, L. P., Monajembashi, S., Greulich, K. O., and Hertweck, C. (2007c). Endosymbiont-dependent host reproduction maintains bacterial-fungal mutualism. Curr. Biol. 17, 773–777. doi: 10.1016/j.cub.2007.03.039
Pawlowska, T. E., Gaspar, M. L., Lastovetsky, O. A., Mondo, S. J., Real-Ramirez, I., Shakya, E., et al. (2018). Biology of fungi and their bacterial endosymbionts. Annu. Rev. Phytopathol. 56, 289–309. doi: 10.1146/annurev-phyto-080417-045914
Pimentel, J. D., and Macleod, C. (2008). Misidentification of Pandoraea sputorum isolated from sputum of a patient with cystic fibrosis and review of Pandoraea species infections in transplant patients. J. Clin. Microbiol. 46, 3165–3168. doi: 10.1128/JCM.00855-08
Pugès, M., Debelleix, S., Fayon, M., Mégraud, F., and Lehours, P. (2015). Persistent infection because of Pandoraea sputorum in a young cystic fibrosis patient resistant to antimicrobial treatment. Pediatr. Infect. Dis. J. 34, 1135–1137. doi: 10.1097/INF.0000000000000843
Richter, I., Radosa, S., Cseresnyés, Z., Ferling, I., Büttner, H., Niehs, S. P., et al. (2022). Toxin-producing endosymbionts shield pathogenic fungus against micropredators. MBio 13, e01440–e01422. doi: 10.1128/mbio.01440-22
Richter, I., Wein, P., Uzum, Z., Stanley, C. E., Krabbe, J., Molloy, E. M., et al. (2023). Transcription activator-like effector protects bacterial endosymbionts from entrapment within fungal hyphae. Curr. Biol. 33, 2646–2656. doi: 10.1016/j.cub.2023.05.028
Ronquist, F., Teslenko, M., and Van Der Mark, P. (2012). Mrbayes 3.2: efficient Bayesian phylogenetic inference and model choice across a large model space. Syst. Biol. 61, 539–542. doi: 10.1093/sysbio/sys029
Rudramurthy, S. M., Singh, S., Kanaujia, R., Chaudhary, H., Muthu, V., Panda, N., et al. (2023). Clinical and mycologic characteristics of emerging mucormycosis agent Rhizopus homothallicus. Emerging Infect. Dis. 29, 1313–1322. doi: 10.3201/eid2907.221491
Salvioli, F. A., Lipuma, J., Venice, F., Dupont, L., and Bonfanteet, P. (2017). The endobacterium of an arbuscular mycorrhizal fungus modulates the expression of its toxin-antitoxin systems during the life cycle of its host. ISME J. 2017, 2394–2398. doi: 10.1038/ismej.2017.84
Sato, Y., Narisawa, K., Tsuruta, K., Umezu, M., Nishizawa, T., Tanaka, K., et al. (2010). Detection of betaproteobacteria inside the mycelium of the fungus Mortierella elongata. Microbes Environ. 25, 321–324. doi: 10.1264/jsme2.ME10134
Scannerini, S., and Bonfante-Fasolo, P. (1991). Bacteria and bacteria-like objects in endomycorrhizal fungi (Glomaceae). L Margulis. and R. Fester, (Eds.) Symbiosis as a source of evolutionary innovation: Speciation and morphogenesis. Cham: The MIT Press, 273–287.
Schüßler, A., Mollenhauer, D., Schnepf, E., and Kluge, M. (1994). Geosiphon pyriforme, an endosymbiotic association of fungus and cyanobacteria: the spore structure resembles that of arbuscular mycorrhizal (AM) fungi. Botanica Acta 107, 36–45. doi: 10.1111/j.1438-8677.1994.tb00406.x
Seemann, T. (2014). Prokka: rapid prokaryotic genome annotation. Bioinformatics 30, 2068–2069. doi: 10.1093/bioinformatics/btu153
Stamatakis, A. (2014). RAxML version 8: a tool for phylogenetic analysis and post-analysis of large phylogenies. Bioinformatics 30, 1312–1313. doi: 10.1093/bioinformatics/btu033
Sun, X., Chen, W., Ivanov, S., Maclean, A. M., Wight, H., Ramaraj, T., et al. (2019). Genome and evolution of the arbuscular mycorrhizal fungus Diversispora epigaea (formerly glomus versiforme) and its bacterial endosymbionts. New Phytol. 221, 1556–1573. doi: 10.1111/nph.15472
Sward, R. (1981). The structure of the spores of Gigaspora margarita the dormant spore. New Phytol. 87, 761–768. doi: 10.1111/j.1469-8137.1981.tb01712.x
Takashima, Y., Seto, K., Degawa, Y., Guo, Y., Nishizawa, T., Ohta, H., et al. (2018). Prevalence and intra-family phylogenetic divergence of Burkholderiaceae-related endobacteria associated with species of Mortierella. Microbes Environ. 33, 417–427. doi: 10.1264/jsme2.ME18081
Uehling, J., Gryganskyi, A., Hameed, K., Tschaplinski, T., Misztal, P., Wu, S., et al. (2017). Comparative genomics of Mortierella elongata and its bacterial endosymbiont Mycoavidus cysteinexigens. Environ. Microbiol. 19, 2964–2983. doi: 10.1111/1462-2920.13669
Uehling, J. K., Salvioli, A., Amses, K. R., Partida-Martínez, L. P., Bonito, G., and Bonfante, P. (2023). Bacterial endosymbionts of Mucoromycota fungi: diversity and function of their interactions, S. Pöggeler and T James. (Eds.) Evolution of fungi and fungal-like organisms. Cham: Springer International Publishing, 177–205.
Vale, F. F., Lehours, P., and Yamaoka, Y. (2022). Editorial: the role of mobile genetic elements in bacterial evolution and their adaptability. Front. Microbiol. 13:849667. doi: 10.3389/fmicb.2022.849667
Venkatesh, N., Greco, C., Drott, M. T., Koss, M. J., Ludwikoski, I., Keller, N. M., et al. (2022). Bacterial hitchhikers derive benefits from fungal housing. Curr. Biol. 32, 1523–1533. doi: 10.1016/j.cub.2022.02.017
Xiao, X., Tian, H., Cheng, X., Li, G., Zhou, J., Peng, Z., et al. (2019). Pandoraea sputorum bacteremia in a patient who had undergone allogeneic liver transplantation plus immunosuppressive therapy: a case report. Infect. Drug Resist. 12, 3359–3364. doi: 10.2147/IDR.S227643
Yang, S., Anikst, V., and Adamson, P. C. (2022). Endofungal Mycetohabitans rhizoxinica bacteremia associated with Rhizopus microsporus respiratory tract infection. Emerging Infect. Dis. 28, 2091–2095. doi: 10.3201/eid2810.220507
Yao, L. D., Ju, X., James, T. Y., Qiu, J. Z., and Liu, X. Y. (2018). Relationship between saccharifying capacity and isolation sources for strains of the Rhizopus arrhizus complex. Mycoscience 59, 409–414. doi: 10.1016/j.myc.2018.02.011
Zhao, H., Nie, Y., Zong, T. K., Wang, K., Lv, M. L., Cui, Y. J., et al. (2023). Species diversity, updated classification and divergence times of the phylum Mucoromycota. Fungal Divers. 123, 49–157. doi: 10.1007/s13225-023-00525-4
Zheng, R. Y., Chen, G. Q., Huang, H., and Liu, X. Y. (2007). A monograph of Rhizopus. Sydowia 59, 273–372.
Zhou, Y., Wang, H., Xu, S., Liu, K., Qi, H., Wang, M., et al. (2022). Bacterial-fungal interactions under agricultural settings: from physical to chemical interactions. Stress Biology. 2:22. doi: 10.1007/s44154-022-00046-1
Keywords: Rhizopus oryzae, novel endosymbiont, endohyphal bacterium, Pandoraea sputorum, comparative genomics
Citation: Liu X-L, Zhao H, Wang Y-X, Liu X-Y, Jiang Y, Tao M-F and Liu X-Y (2024) Detecting and characterizing new endofungal bacteria in new hosts: Pandoraea sputorum and Mycetohabitans endofungorum in Rhizopus arrhizus. Front. Microbiol. 15:1346252. doi: 10.3389/fmicb.2024.1346252
Edited by:
Robert Czajkowski, University of Gdansk, PolandReviewed by:
Sakineh Abbasi, Institut National de recherche pour l’agriculture, l’alimentation et l’environnement (INRAE), FrancePaola Bonfante, University of Turin, Italy
Copyright © 2024 Liu, Zhao, Wang, Liu, Jiang, Tao and Liu. This is an open-access article distributed under the terms of the Creative Commons Attribution License (CC BY). The use, distribution or reproduction in other forums is permitted, provided the original author(s) and the copyright owner(s) are credited and that the original publication in this journal is cited, in accordance with accepted academic practice. No use, distribution or reproduction is permitted which does not comply with these terms.
*Correspondence: Xiao-Yong Liu, bGl1eHlAc2RudS5lZHUuY24=
†These authors have contributed equally to this work