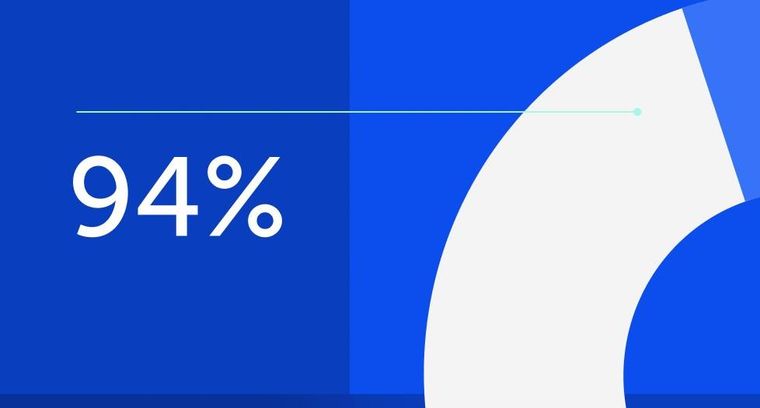
94% of researchers rate our articles as excellent or good
Learn more about the work of our research integrity team to safeguard the quality of each article we publish.
Find out more
ORIGINAL RESEARCH article
Front. Microbiol., 24 January 2024
Sec. Food Microbiology
Volume 15 - 2024 | https://doi.org/10.3389/fmicb.2024.1345772
This article is part of the Research TopicMicrobial Communities in Fermented Products: Current Knowledge and Future ProspectsView all 10 articles
This study investigated the microbial community in three-color sauce-flavor Daqu (black, yellow, and white) throughout their maturation processes, together with their physicochemical factors, culturable microbes, flavor components, and fermenting vitalities. Results from high-throughput sequencing revealed distinct microbial diversity, with more pronounced variations in bacterial community than in fungal community. Firmicutes and Ascomycota emerged as the most dominant bacterial and fungal phyla, respectively, during maturation. Genus-level analysis identified Kroppenstedia, Virgibacillus, and Bacillus as dominant bacteria in black Daqu, yellow Daqu, and white Daqu, severally, while Thermoascus was shared as the core dominant fungi for these Daqu. Physicochemical factors, particularly acidity, were found to exert a significant impact on microbial community. Kroppenstedtia was the key bacteria influencing the color formation of these Daqu. Furthermore, correlations between dominant microbes and flavor compounds highlighted their role in Daqu quality. Molds (Aspergillus, Rhizomucor, and Rhizopus), excepting Bacillus, played a crucial role in the formation of pyrazine compounds. Consequently, this study offers innovative insights into the microbial perspectives on color and pyrazine formation, establishing a groundwork for future mechanized Daqu production and quality control of sauce-flavor baijiu.
Baijiu, one of the six globally recognized distilled spirits, has gained a lot of attention owing to its profound historical roots and cultural significance in China (Yan et al., 2021). Recent data from National Bureau of Statistics revealed the impressive scale of this industry, with the year 2022 witnessing a baijiu production of 6.71 billion liters, considerably contributing to national economy (National Statistics Bureau, 2023). Remarkably, sauce-flavor baijiu, is the leader among the three major categories of baijiu flavor, including light-flavor, strong-flavor, and sauce-flavor. The increasing interest among national consumers in sauce-flavor baijiu is largely driven by its exceptional quality and pleasurable drinking experience. The intricate and traditional craftsmanship involved in brewing sauce-flavor baijiu plays a pivotal role in producing high-quality baijiu. The intricate brewing processes encompass several essential stages, consisting of Daqu production, solid-state fermentation, distillation, storage and aging, and blending (Jin et al., 2017; Niu et al., 2022; Yang et al., 2023). As the adage goes, “Daqu is the backbone of baijiu brewing.” emphasizing the crucial role of Daqu—a fermented agent initiating the solid-state fermentation of baijiu by introducing a diverse array of microbes, enzymes, and flavor precursors, ultimately determining the final quality of base liquor (De Melo et al., 2019; Xia et al., 2022; Li H. et al., 2023; Yang et al., 2024).
In the context of sauce-flavor baijiu brewing, the Daqu employed is known as high-temperature Daqu, signifying its production under elevated temperature conditions (above 60°C) (Deng et al., 2020; Sakandar et al., 2020; Pan et al., 2023). The essential processes involved in the production of this Daqu are illustrated in Figure 1. Wheat is crushed, mixed with 34–40% water and 5–8% mother-Daqu powder, and then molded into Daqu bricks. These bricks undergo a 50-day high-temperature fermentation in a dedicated chamber, followed by a 6-month maturation period in a storage room (Niu et al., 2022). After maturation, the Daqu is crushed and used in the production of sauce-flavor baijiu, highlighting the critical role of fermentation and maturation in determining Daqu quality. Notably, the production of Daqu involves spontaneous processes with minimal human intervention, leading to the natural formation of three distinct Daqu color: black, yellow, and white (Cai et al., 2021; Shi W. et al., 2022). Previous studies have delved into the difference in microbial community of these three kinds of Daqu during fermentation process. Zhu’s study uncovered specific insight in the microbial community succession during Daqu fermentation (Zhu C. et al., 2022). High temperature emerged as the most significant factor driving core functional community of Daqu, correlating with flavor formation throughout fermentation (Zhu Q. et al., 2022; Wu et al., 2023). Others studies compared the differences in microbial community and flavor between artificial and mechanical Daqu during fermentation (Huang et al., 2023). Deng’s research shed light on the discrepancies in microbial composition among colored Daqu (Deng et al., 2020). In addition, several studies unveiled the potential factors contributing to color formation in the three types of Daqu, highlighting the significant roles played by amino acid metabolism and the Maillard reaction (Zhang et al., 2022; Zhu Q. et al., 2023).
Despite the big differences in the properties of the three-color Daqu, they are used in combination with specifical proportions to ensure the stable brewing and producing of sauce-flavor baijiu. These Daqu types undergo spontaneous fermentation, presenting challenges in controlling color formation and hindering the targeted production of specific Daqu varieties, impacting future quality control efforts. While previous researches have primarily focused on the microbiota of these Daqu during the fermentation process and proposed potential reasons for color formation, insufficient attention has been given to the maturation process, specifically regarding microbial composition, flavor components, and vitalities. Additionally, the microbes associated with color formation remain unclear. This study is dedicated to exploring the maturation process of these three Daqu types, investigating their physicochemical properties, cultivable microbes, fermenting vitalities, flavor substances, and corresponding microbial community. Hence, a systematically comparing analysis was carried out to reveal the microbiota differences among these colored Daqu, uncovering the core microbes related to color formation and laying a foundation for targeted production of specific Daqu and enhanced quality control in the future.
The Daqu bricks were fermented in the Qu-producing shop of Jing Brand Maotai Town Liquor Co., Ltd., Renhuai, Guizhou province, China. Following fermentation, Daqu was categorized by color (black, yellow, and white) and moved to the storage room for maturation with a period of 180 days. Samples were collected at intervals of 0, 30, 60, 120, 150, and 180 days from each color of Daqu, resulting in 54 samples (three replicates per color) for subsequent analysis. Once the samples were gathered, the brick from each kind of Daqu was crushed to powder for the immediate assessment of physicochemical properties, cultivable microbes, and fermenting vitalities. The powder was stored at −80°C for subsequent flavor compounds analysis and amplicon sequencing.
The analysis of moisture, acidity, and reducing sugar content in three kinds of Daqu were conducted based on our previous study with minor revisions (Dong et al., 2022). The amino acid nitrogen content of Daqu was detected following the methodology outlined in Huang’s study (Huang et al., 2021). Specifically, moisture content was measured by taking 4 g of Daqu powder in an oven for desiccation (overnight) until a constant weight, and calculating the ratio of weight loss. Before the acidity assessing, 10 g of Daqu powder was added into 200 mL of ddH2O with 30 min of static for acids extraction. Subsequently, the supernatant was used for acid–base titration to determine the acidity. The content of reducing sugar in Daqu was evaluated via Fehling’s test in reliance on the standard reducing sugar solution. As for the amino acid nitrogen, it was evaluated based on the amphoteric behavior of amino acids. Firstly, the amino acid nitrogen was extracted form Daqu by mixing 20 g of samples into 60 mL of ddH2O together with vibration at 120 rpm for 30 min, and the filtrate was collected and used for detecting. Subsequently, the pH value of filtrate was adjusted to 8.2 with 50 mM NaOH, together with 10 mL of methanal being added to fix the amino base. Finally, the titration with standard NaOH solution was performed to determine the end-point (pH 9.2) via a pH meter, and thus calculating the amino acid nitrogen content by the consuming volume of standard NaOH solution.
The cultivable microbes in Daqu were quantified using traditional dilution and plating method, following our previous protocols with minor adjustments (Dong et al., 2022). Specifically, Bacillus, lactic acid bacteria (LABs), yeasts, and molds were targeted for detection. Ten grams of Daqu powder were combined with 90 mL of sterile H2O and shaken at 160 rpm for 30 min. Subsequently, a gradient dilution was performed, and 100 μL of the diluted suspension was plated on respective agar plates to cultivate cultivable microbes. LB agar, YPD agar (with ampicillin), MRS agar (with nystatin), and PDA (with ampicillin) were employed to support the growth of Bacillus, LABs, yeasts, and molds, respectively, under appropriate conditions. Following the designated incubation period, the number of visible colonies on the respective plates was enumerated.
The fermenting vitalities of Daqu was monitored by detecting neutral protease activity, α-amylase activity, glucoamylase activity, and fermentation ability. Thereinto, the neutral protease activity was determined via colorimetry at 680 nm using Folin–Ciocalteu method based on our previous study (Dong et al., 2020). The α-amylase activity was detected based on iodine-starch colorimetric assay, and the decrease in absorbance at 620 nm was indicative of α-amylase activity. Glucoamylase activity was evaluated by determining the glucose produced from starch degradation via DNS (3,5-dinitrosalicylic acid) method. Additionally, fermentation ability was assessed by quantifying the weight of CO2 produced during sorghum juice fermentation inoculated with Daqu powder.
The flavor substances in Daqu were detected by a head-space solid-phase microextraction and gas chromatography–mass spectrometry (HS-SPMEGC–MS) according to our previous study (Dong et al., 2022). In this process, 2.0 g of Daqu powder and 3.0 g NaCl were combined in 10 mL of 10% ethanol within a headspace bottle, then internal standard substances (2-octanol and 2-ethyl hexanol) were added. After that, the flavor substances in mixture were extracted by an automatic headspace sampling system (Multipurpose Sample MPS 2XL) at 50°C for 45 min. Subsequently, the SPME fiber was introduced into the injection port, set at 250°C, for a duration of 5 min. Compounds separation was achieved using an Agilent HP-5 column (30 m × 0.25 mm; 0.25 μm film thickness) and a DB-FFAP column (60 m × 0.25 mm; 0.25 μm film thickness), followed by analysis via GC–MS analysis employing the Agilent 7890B GC system and the 5977C mass selective detector. Compounds identification was carried out by comparing mass spectral profiles with a match quality of ≥80 in the NIST14 database.
The third-generation amplicon sequencing was employed to analyze the composition, structure, and succession of microbial community in three types of Daqu during maturation, specifically on day 0, 30, 90, and 180. Initially, total DNA was extracted from 0.2 grams of Daqu powder using the E.Z.N.A.® Soil DNA Kit (Omega Bio-Tek, United States) as per the provided instructions. After the concentration and quality assessment of total DNA were conducted, it served as the template for library construction. For the full-length 16S rDNA library of bacteria, amplification was performed using Q5 high-fidelity DNA polymerase (NEB, United States) with primers 27F (AGRGTTTGATYNTGGCTCAG) and 1492R (TASGGHTACCTTGTTASGACTT) (Callahan et al., 2019). The amplification parameters were as follows: 98°C for 5 min, 98°C for 30 s, 55°C for 30 s, and 72°C for 45 s for 30 cycles, and final at 72°C for 5 min. Simultaneously, the full-length ITS library of fungi was constructed by Q5 high-fidelity DNA polymerase using primers ITS1-F (CTTGGTCATTTAGAGGAAGTAA) and ITS4 (TCCTCCGCTTATTGATATGC) (Kuang et al., 2021). The amplification parameters for this library were: 98°C for 5 min, 98°C for 30 s, 53°C for 30 s, and 72°C for 20 s for 30 cycles, with a final step at 72°C for 5 min. Subsequently, the purified PCR products of these two libraries were subjected to high-throughput sequencing (HTS) using the PacBio Sequel II platform. The post-sequencing data were analyzed bioinformatically using QIIME2, with the main analytical workflow referenced from Yang’s study (Yang et al., 2024).
The raw data generated from HTS has been securely archived in NCBI under the BioProject accession number PRJNA 1034761 and PRJNA 1034762. Statistical analyses in this study involved data processing with Excel (version 2019), and the determination of significance was conducted using Origin (version 9.0), with a significance threshold set at 0.05.
The physicochemical properties of the three types of Daqu during the maturation process were shown in Figure 2, emphasizing key parameters such as moisture content, acidity, reducing sugar, and amino acid nitrogen levels. A gradual reduction in moisture content was observed among the three types of Daqu, with levels decreasing from 8.09 to 7.08% for black Daqu, 8.43 to 7.20% for yellow Daqu, and 7.68 to 6.89% for white Daqu (Figure 2A). Notably, all three types reached a final moisture content below 9%, adhering to the standard for qualified Daqu, preserving enzymatic and microbial activities (Paredes-López et al., 1988; Xia et al., 2022). While the dropping trend of acidity during maturation was similar across the three colored Daqu, black Daqu exhibited the highest acidity, white Daqu the lowest, and yellow Daqu intermediate, consistent with findings in Zhang’s study (Zhang et al., 2022). At the end of the maturation period, the acidity values for these three types of Daqu were 1.03, 0.56, and 0.35 mmol/10 g, respectively (Figure 2B), which were lower than those in previous studies (Deng et al., 2020; Zhang et al., 2022). In terms of reducing sugar, initial content varied significantly among the Daqu types but showcased an overall dropping trend, stabilizing at a low level of approximately 1.40% by the end of maturation (Figure 2C). This low reducing sugar content is conducive to microbial stabilization but insufficient for microbial growth in Daqu. Amino acid nitrogen content showed an initial increase followed by a decrease, with black Daqu having the lowest final content (4.11 g/kg), white Daqu next (4.28 g/kg), and yellow Daqu having the highest content (4.78 g/kg) (Figure 2D).
Figure 2. The physicochemical factors of three kinds Daqu during maturation, including moisture (A), acidity (B), reducing sugar (C), and amino acid nitrogen (D).
The counts of cultivable microbes in the three-color Daqu manifested an overall raising trend followed by dwindling during the maturation process (Table 1), aligning with the previously noted decrease in moisture content (Figure 2A). In cases of Bacillus, the number ranged from 6.33 × 105 to 9.03 × 108 cfu/g, with white Daqu exhibiting the highest count, followed by yellow Daqu, and black Daqu (Table 1). This Bacillus count falls within a reasonable range reported in previous studies, where the maximal order of magnitudes for Bacillus is around 108 in Daqu (Sakandar et al., 2020). The prevalence of Bacillus in cultivable microbes may be attributed to its ability to survive in harsh conditions (Liu et al., 2019; Sakandar et al., 2020). The number of LABs were lower (6.30 × 105 to 6.21 × 107 cfu/g) than those of Bacillus in the three Daqu and exhibited an opposite trend, with black Daqu having the highest LAB count, followed by yellow Daqu and white Daqu (Table 1). This finding aligns with previous research indicating that LABs contribute to substrate acidity, inhibiting the growth of Bacillus (Zou et al., 2018). The quantity of cultivable fungi was significantly lower than that of bacteria, with yeast counts ranging from 3.62 × 102 to 3.32 × 105 cfu/g and mold counts varying from 7.66 × 102 to 3.53 × 106 cfu/g (Table 1). Among these, white Daqu had the highest counts of yeast and mold, while black Daqu had the lowest counts, suggesting a synergistic effect of yeast and mold during the maturation process.
The fermenting vitalities of Daqu play a crucial role in the production of sauce-flavor baijiu, influencing the initiation of baijiu fermentation (Wang B. et al., 2018; Li H. et al., 2023). In this study, we conducted a comprehensive analysis of neutral protease, α-amylase, glucoamylase, and fermentation ability in the three types of Daqu to evaluate their fermenting vitalities. Neutral protease activity displayed an initial increase followed by a decrease in all three types of Daqu (Figure 3A). White Daqu exhibited the final activity at 80.61 U/g, followed by yellow Daqu at 48.51 U/g, and black Daqu at 9.98 U/g on day 180 of maturation (Figure 3A). This result is consistent with the changes in the counts of Bacillus mentioned earlier (Table 1), as Bacillus is recognized for its superior protease production (Contesini et al., 2017). The activities of α-amylase, glucoamylase, and fermentation vitalities all exhibited similar trends, with initial increases followed by a decline and subsequent stabilization (Figures 3B–D). Overall, white Daqu demonstrated the highest activity, followed by yellow Daqu, and black Daqu had the lowest (Figures 3B–D). These results align with the counts of yeast and mold in cultivable microbes (Table 1), indicating that a higher count of mold and yeast promotes the production of α-amylase and glucoamylase (Wang X. D. et al., 2018), ultimately resulting in stronger fermentation activity (Yang et al., 2022). In summary, the analysis of the fermenting vitalities of the three types of Daqu suggests that white Daqu has the highest quality and provides stronger fermentation capabilities, contributing to the initiation of sauce-flavor baijiu brewing.
Figure 3. The fermenting vitalities of three kinds Daqu during maturation, including neutral protease (A), α-amylase (B), glucoamylase activity (C), and fermenting ability (D).
The flavor substances in Daqu serve as the precursors to determine the final flavor and characteristics of baijiu (Li H. et al., 2023). A total of 160 different chemicals were detected, and the fluctuations in flavor substances during the maturation of the three types of Daqu are intricate. Here, we focused on analyzing alcohols, organic acids, esters, pyrazines, aldehydes and ketones (Figure 4). The content of alcohols in the colored Daqu all exhibited an overall decreasing trend throughout the maturation process (Figure 4A). Yellow Daqu initially had the highest alcohols content (79.79%) on day 0, dropping to 30.36% on day 180, while black Daqu started with 44.82%, decreasing to 24.33% at maturity (Figure 4A). In contrast, white Daqu showed the lowest initial alcohols content of 1.97%, declining to 0.68% on day 180 (Figure 4A). Organic acids content also decreased during maturation in these three types of Daqu, albeit with a milder decline than the variation observed in alcohols, ranging from 1.60 to 3.75% (Figure 4B). Ultimately, black Daqu had the highest organic acids content (1.85%), followed by yellow Daqu (0.73%), and white Daqu (0.54%) at the end of maturation (Figure 4B), in line with changes in acidity mentioned earlier (Figure 2B). Esters content varied significantly among the three types of Daqu, with white Daqu having the highest overall content, fluctuating throughout maturation, while black Daqu and yellow Daqu showed a similar upward trend (Figure 4C). Thus, the decline in alcohols and organic acids observed here can be attributed to their substantial consumption in the formation of esters. Pyrazine substances, crucial flavor compounds in sauce-flavor baijiu, contribute to pleasant aromas of roasted nuts and cocoa (Mortzfeld et al., 2020; Shi X. et al., 2022). They were considered one type of the characteristic flavor components that differentiate sauce-flavor baijiu from other types of baijiu (Zhang et al., 2013; Niu et al., 2022). The content of pyrazines in the three-color Daqu showed big differences (Figure 4D). White Daqu and black Daqu maintained high levels of pyrazines (>10%), with white Daqu reaching 19.18% and black Daqu at 12.37% on day 180 (Figure 4D). In contrast, the content of pyrazines in yellow Daqu remained at low level, reaching 4.53% on day 180 (Figure 4D). Aldehydes and ketones exhibited an increasing trend in both black Daqu and yellow Daqu, reaching 9.17 and 10.96% at the end of maturation, respectively, whereas white Daqu experienced a sharp increase to 13.04% during day 60–120, followed by a rapid decrease to 4.45% in the end (Figure 4E). Other flavor substances exhibited varying changes among the three types of Daqu (Figure 4F). In summary, the diverse changes in flavor substances during the maturation process of the three types of Daqu indicate each type’s unique characteristics, suggesting potential combinations based on differences in flavor substances for sauce-flavor baijiu brewing.
Figure 4. The variation of main flavor compounds in three kinds Daqu during maturation. Here, the alcohols (A), acids (B), esters (C), pyrazines (D), aldehydes and ketones (E), and the others (F) were presented.
Through third-generation HTS, we analyzed the microbial community of the three-color Daqu during maturation. The results indicated that the sequencing coverage exceeded 0.991 (Table 2), demonstrating the depth and reliability of the amplicon sequencing. Regarding bacterial α diversity, the Chao1 and Shannon indices for the three types of Daqu generally exhibited a trend of initial decrease followed by an increase as they mature (Table 2). Overall, the Chao1 richness was highest in black Daqu, followed by white Daqu, and lowest in yellow Daqu. The Shannon index was higher in black and white Daqu compared to yellow Daqu. For fungal α diversity, the Chao1 richness decreased for all three types of Daqu, indicating a decline in fungal richness during Daqu maturation. This decrease may be associated with the decline in moisture content during Daqu maturation since the lower moisture levels, below 9%, can reduce free water in microbial cells, hampering their growth and reproduction. In contrast, the fungal Shannon index remained relatively stable, with white Daqu exhibiting the highest value, suggesting a more even distribution of fungi during maturation. In summary, the primary diversity differences among the three types of Daqu are reflected in the Chao1 richness, with less variation in the Shannon index (Table 2).
To further assess the structure of microbial community during Daqu maturation, a Principal Component Analysis (PCA) based on Bray-Curtis distances was conducted. The β diversity results revealed that the structural differences in bacterial community among the three types of Daqu were more pronounced than for fungal community. In the bacterial β diversity plot, samples representing the three types of Daqu were more scattered, indicating greater differences (Supplementary Figure S1A). In contrast, in the fungal β diversity plot, samples from all three Daqu types clustered closely and were not easily distinguishable (Supplementary Figure S1B). This implies that the differences in microbial diversity mainly exist in the bacterial community rather than the fungal community.
To investigate the composition of microbial community in Daqu, differences in taxa at the phylum and genus levels were analyzed during the maturation of the three-color Daqu. For bacterial composition, Firmicutes, Actinobacteriota, Bacteroidota, Proteobacteria, Fusobacteriota, and Verrucomicrobiota constituted the predominant phyla (Figure 5A). Among them, Firmicutes emerged as the most abundant phylum, which was congruent with Gan’s study (Gan et al., 2019). However, the abundance of Firmicutes varied among three kinds of Daqu. In black Daqu, there was an initial increase followed by decrease (49.59 to 88.80 to 32.17%) (Figure 5A). Yellow Daqu exhibited a minor decline from 97.61 to 91.07%, while Firmicutes remained relatively stable in white Daqu, with abundances consistently above 80.52% (Figure 5A). As for fungal composition, the predominant phyla included Ascomycota, Mucoromycota, Basidiomycota, Mortierellomycota, Chytridiomycota, and Rozellomycota (Figure 5B). Ascomycota was the most dominant phylum, collaborating with previous studies (Deng et al., 2020; Zhang et al., 2022). The abundance of Ascomycota in black Daqu maintained above 94.29%, yellow Daqu exhibited fluctuations ranging from 80.51 to 99.77%, and white Daqu displayed an initial increase followed by a decrease (80.03 to 97.43 to 74.23%) (Figure 5B).
Figure 5. The microbial community of three kinds of Daqu during maturation, including bacterial phylum level (A), fungal phylum level (B), bacterial genus level (C), and fungal genus level (D).
At the genus level, the composition of microbial community in the three-color Daqu showed even greater differences (Figures 5C,D). For bacterial, Virgibacillus, Kroppenstedtia, Bacillus, Scopulibacillus, Candidatus Sulcia, Oceanobacillus, Enterobacter, Saccharopolyspora, Pediococcus, and Staphylococcus were the top 10 abundant genera (Figure 5C). However, their relative abundances in the microbial community changed significantly during Daqu maturation. In black Daqu, Kroppenstedtia (31.58%), Candidatus Sulcia (17.49%), and Virgibacillus (10.27%) constituted the top 3 dominant bacterial genera (Figure 5C). Kroppenstedtia, in particular, was the most dominated genera in black Daqu, and its relative abundance rapidly increased from 6.48% (day 0) to 68.20% (day 30), followed by a continue decrease to 3.11% on day 180 (Figure 5C). Previous studies have unveiled that Kroppenstedtia is the core microbe in connection with the biosynthesis of organic acids (lactic acid and short chain fatty acids) (Zhu C. et al., 2023; Zhang et al., 2024), explaining the highest acidity observed in black Daqu during maturation. In yellow Daqu, Virgibacillus (54.66%), Kroppenstedtia (21.47%), and Oceanobacillus (8.57%) made up the top 3 dominant bacterial genera (Figure 5C). Virgibacillus was the main dominant genus in yellow Daqu, with its relative abundance quickly rosing from 54.83% (day 0) to 71.45% (day 30) and then gradually dropping to 33.67% (day 180) (Figure 5C). This result differs from previous studies where Virgibacillus was a dominant bacterium but not the most dominant in Daqu (Zhu C. et al., 2023; Zhu Q. et al., 2023). The roles and functions of Virgibacillus in Daqu production and baijiu brewing are not clear and needs further study. In white Daqu, Bacillus (35.53%), Virgibacillus (22.13%), and Scopulibacillus (10.01%) were the top three dominant bacterial genera (Figure 5C). Bacillus was the most dominant genus, and its relative abundance gradually decreased during maturation (from 49.44 to 25.86%) (Figure 5C). Despite the decreasing trend, the abundance of Bacillus remained above 25%, suggesting that it could still function effectively. Bacillus is known for secreting amylase and proteases and playing essential roles in liquefaction and saccharification (Ding et al., 2013; Wang et al., 2014, 2020), contributing to the higher fermenting vitalities observed in white Daqu (Figure 3). In addition, the average abundance of Kroppenstedtia in three kinds of Daqu followed the order: black Daqu, yellow Daqu, and white Daqu. Kroppenstedtia was found to be positively correlated with the production of various amino acids in Daqu (Zhu C. et al., 2023), which might promote the Maillard reaction with reducing sugar, leading to the formation of the yellow-brown color and contributed to the color difference in these three-color Daqu. Therefore, Kroppenstedtia, Virgibacillus, and Bacillus were identified as the three core bacteria in these colored Daqu, with Kroppenstedtia being the potential key microbe related to color formation.
For fungal genera, differences were smaller than those of bacteria among the three types of Daqu. The top 10 abundant fungal genera included Thermoascus, Thermomyces, Aspergillus, Rasamsonia, Rhizomucor, Pichia, Candida, Mortierella, Gelasinospora, and Monascus (Figure 5D). Among them, Thermoascus was the most dominant genera shared in all three types of Daqu, which was similar to Zhu’s study (Zhu C. et al., 2022). The relative abundance of Thermoascus changed in the same manner, initially decreasing, then increasing, and finally decreasing again (Figure 5D). In black Daqu, Thermoascus (57.38%), Aspergillus (17.18%), and Thermomyces (12.48%) constituted the top 3 dominant fungal genera, and the relative abundance of Thermoascus ranged from 35.92 to 92.58% (Figure 5D). In yellow Daqu, the top 3 dominant fungal genera belonged to Thermoascus (59.84%), Rasamsonia (6.85%), and Thermomyces (6.08%), whereas the abundance of Thermoascus varied from 36.51 to 73.91% (Figure 5D). In white Daqu, Thermoascus (39.35%), Thermomyces (25.01%), and Rhizomucor (7.50%) comprised the top 3 fungal genera, while the abundance of Thermoascus fluctuated from 6.44 to 65.88% (Figure 5D). Here, Thermoascus is known to produce various enzymes such as catalase, endoglucanase, glucosidase, keratinase, and chitinase, which are able to degrade starch, cellulose or protein from raw materials, providing basic nutrition for other microbes’ metabolism that contributed to flavor formation (Jain et al., 2014; Cai et al., 2021). Thus, Thermoascus was identified as the most important fungal genera during Daqu maturation.
Physicochemical factors play a crucial role in shaping and influencing the composition and succession of microbial community during spontaneously fermentation processes (Guan et al., 2020). Therefore, canonical correlation analysis (CCA) was employed to examine the impact of physicochemical factors on the microbial community of the three types of Daqu. The results, as shown in Figure 6, indicated that acidity had the most substantial impact on microbial community, as the arrow length representing acidity was the longest among the four physicochemical factors. Regarding bacterial communities, the influence of the four physicochemical factors was as follows: acidity > reducing sugar > moisture > amino acid nitrogen (Figure 6A). Specifically, the dominant bacterium Kroppenstedia in black Daqu showed a positive correlation with acidity, reducing sugar, and moisture, while it had a negative correlation with amino acid nitrogen (Figure 6A). Kroppenstedtia is known to correlate with the biosynthesis of organic acids, evidencing its positive relationship with acidity (Zhang et al., 2024). Its negative correlation with amino acid nitrogen contradicted a previous study that found Kroppenstedtia to be positively connected to the production of various amino acids in Daqu (Zhu C. et al., 2023). This discrepancy might be caused by the color formation in Daqu, where amino acids were consumed via the Maillard reaction to form the dark color. In yellow Daqu, the dominant bacterium Virgibacillus displayed a positive correlation with reducing sugar, moisture, and amino acid nitrogen, but a negative correlation with acidity (Figure 6A). In white Daqu, the dominant bacterium Bacillus exhibited a positive correlation with amino acid nitrogen but a negative correlation with the other three physicochemical properties (Figure 6A). Here, Bacillus is the typical microbe for producing protease (Wang et al., 2014; Contesini et al., 2017), thus hydrolyzing protein to amino acids or small peptides, interpretating this positive correlation. When considering fungal communities, the influence of these four physicochemical factors was as follows: acidity > amino acid nitrogen > moisture > reducing sugar (Figure 6B). The dominant fungus Thermoascus in all three types of Daqu shared a positive correlation with all four physicochemical factors (Figure 6B), indicating Thermoascus’ ability to adapt to the maturation process of Daqu. Therefore, acidity was the most critical factor affecting the microbial community of these three kinds of Daqu during maturation.
Figure 6. Canonical correlation analysis between microbial community and physicochemical parameters based on the top 20 dominant microbes at genus levels. The different color of dots represented different groups of samples. The blue arrows expressed physicochemical factors, and red arrows stood for core microbes. The length of arrows determines the degree of importance. The correlations of bacterial community (A) and fungal community (B) to physicochemical parameters.
During the maturation of Daqu, microbes utilize substrates such as starch, protein, and others, producing a plethora of flavor compounds and various enzymes. These, in turn, have a profound impact on the subsequent production of baijiu, making microbes pivotal in the maturation of Daqu and significantly influencing the quality of the final product. To explore these connections, we employed Spearman correlation analysis to unravel the relationships between dominant microbes and flavor compounds, as well as fermenting vitalities. The results, presented in Figure 7, delineated the influence of dominant bacteria and fungi on flavor compounds and fermenting vitalities. In black Daqu, the dominant bacterium Kroppenstedia exhibited a substantial positive correlation with acids and pyrazines, along with a significant negative correlation with amylase, fermenting vitality, and esters (Figure 7A). These correlations may be attributed to the unique characteristics of Kroppenstedia, as reported previously. Kroppenstedtia was identified as a core microbe related to the production of organic acids (lactic acid and short chain fatty acids) (Zhu C. et al., 2023; Zhang et al., 2024), and positively correlated with the formation of certain pyrazines such as tetramethylpyrazine and 2,3,5-trimethyl pyrazine (Zhang et al., 2021). Within yellow Daqu, the dominant bacterium Virgibacillus demonstrated a positive correlation with neutral protease and alcohols, while displaying a negative correlation with pyrazines (Figure 7A). However, the functions of Virgibacillus in fermented food is not clear and warrant further investigation. In white Daqu, the dominant bacterium Bacillus displayed a positive correlation with all enzymes, fermenting vitality, and pyrazines, while holding a negative correlation with alcohols (Figure 7A). Bacillus is known to produce various hydrolytic enzymes, including amylase, protease, and lipase, to degrade macromolecules and hence promoting the formation of flavor compounds during baijiu brewing (Jiang et al., 2021; Li Z. et al., 2023). Especially, the pyrazine compounds, providing sauce-flavor baijiu with its unique flavor characteristic, were closely linked to Bacillus (He et al., 2019). Considering fungi, the dominant fungus Thermoascus across all three types of Daqu showed a positive correlation with amylase, glucoamylase, alcohols, and esters (Figure 7B), hinting that Thermoascus could promote the formation of flavor by producing various enzymes and collaborating with others functional microbes (Jain et al., 2014; Cai et al., 2021). Notably, the formation of pyrazine substances, characteristic of sauce-flavor baijiu, exhibited a significant positive correlation with Aspergillus, Rhizomucor and Rhizopus (Figure 7B), implying that these fungi also played a crucial role in the formation of pyrazines. This conclusion complements the prevailing belief that Bacillus is the core microbe responsible for pyrazine formation, indicating the substantial contributions of molds to this process.
Figure 7. The heatmap of relationships between flavor compounds & activity and dominant microbes. Here, their relationships to bacteria were presented as in (A) part, and their relationships to fungi were presented as in (B) part. The dominant microbes at genus level (top 20) were selected to analyze the correlations to flavor compounds using Spearman correlation coefficient (*p < 0.05 and **p < 0.01). R value was set at 0.1.
In this study, the physicochemical properties exhibited similar trends during the maturation of the three types of Daqu, except for acidity, which showed significant differences. A comprehensive analysis of the fermenting capabilities revealed that white Daqu attained the highest quality, followed by yellow Daqu, while black Daqu exhibited the lowest quality. Moreover, the differences in microbial community during maturation were more pronounced for bacteria than for fungi based on the third-generation HTS. Kroppenstedia, Virgibacillus, and Bacillus were the most dominant bacteria in black, yellow, and white Daqu, respectively, whereas their dominant fungi all belonged to the Thermoascus. Acidity acted as the most notable factor influencing the microbial community. Kroppenstedtia was the potential core bacterium affecting the color formation in Daqu via Maillard reaction. Furthermore, molds played a pivotal role in pyrazine compounds formation. Therefore, this study provides some novel explanations of color and pyrazine formation from a microbial perspective, laying a foundation for the mechanized Daqu production and quality control in the future.
The datasets presented in this study can be found in online repositories. The names of the repository/repositories and accession number(s) can be found below: https://www.ncbi.nlm.nih.gov/, PRJNA 1034761 https://www.ncbi.nlm.nih.gov/, PRJNA 1034762.
WD: Conceptualization, Data curation, Formal analysis, Funding acquisition, Writing – original draft. XY: Data curation, Formal analysis, Writing – review & editing. LW: Methodology, Software, Writing – review & editing. MZ: Investigation, Methodology, Writing – review & editing. JM: Data curation, Software, Writing – review & editing. JL: Conceptualization, Data curation, Writing – review & editing. YF: Investigation, Resources, Writing – review & editing. SZ: Conceptualization, Resources, Writing – review & editing. QY: Resources, Supervision, Writing – review & editing. YH: Funding acquisition, Supervision, Writing – review & editing. SC: Conceptualization, Funding acquisition, Supervision, Writing – review & editing.
The author(s) declare financial support was received for the research, authorship, and/or publication of this article. This research was supported by the Hubei Key Laboratory of Edible Wild Plants Conservation and Utilization (EWPL202306), the Excellent Young and Middle-Aged Science and Technology Innovation Team Plan Project of University in Hubei Province (T2022028), the Hubei Provincial Key Research and Development Program (2023BBB004), and the Hubei Natural Science Foundation (2022CFB137).
WD, QY, and SC were employed by Jing Brand Co., Ltd.
The remaining authors declare that the research was conducted in the absence of any commercial or financial relationships that could be construed as a potential conflict of interest.
All claims expressed in this article are solely those of the authors and do not necessarily represent those of their affiliated organizations, or those of the publisher, the editors and the reviewers. Any product that may be evaluated in this article, or claim that may be made by its manufacturer, is not guaranteed or endorsed by the publisher.
The Supplementary material for this article can be found online at: https://www.frontiersin.org/articles/10.3389/fmicb.2024.1345772/full#supplementary-material
Cai, W., Xue, Y. A., Wang, Y., Wang, W., Shu, N., Zhao, H., et al. (2021). The fungal communities and flavor profiles in different types of high-temperature Daqu as revealed by high-throughput sequencing and electronic senses. Front. Microbiol. 12:784651. doi: 10.3389/fmicb.2021.784651
Callahan, B. J., Wong, J., Heiner, C., Oh, S., Theriot, C. M., Gulati, A. S., et al. (2019). High-throughput amplicon sequencing of the full-length 16S rRNA gene with single-nucleotide resolution. Nucleic Acids Res. 47:e103. doi: 10.1093/nar/gkz569
Contesini, F. J., Melo, R. R. D., and Sato, H. H. (2017). An overview of Bacillus proteases: from production to application. Crit. Rev. Biotechnol. 38, 321–334. doi: 10.1080/07388551.2017.1354354
De Melo, V., Pereira, G., De Carvalho Neto, D. P., Junqueira, A. C. D. O., Karp, S. G., Letti, L. A. J., et al. (2019). A review of selection criteria for starter culture development in the food fermentation industry. Food Rev. Intl. 36, 135–167. doi: 10.1080/87559129.2019.1630636
Deng, L., Mao, X., Liu, D., Ning, X. Q., Shen, Y., Chen, B., et al. (2020). Comparative analysis of physicochemical properties and microbial composition in high-temperature Daqu with different colors. Front. Microbiol. 11:588117. doi: 10.3389/fmicb.2020.588117
Ding, X. F., Wu, C. D., Zhang, L. Q., Zheng, J., and Zhou, R. Q. (2013). Characterization of eubacterial and archaeal community diversity in the pit mud of Chinese Luzhou-flavor liquor by nested PCR–DGGE. World J. Microbiol. Biotechnol. 30, 605–612. doi: 10.1007/s11274-013-1472-4
Dong, W., Shen, H., Liu, H., Song, F., Li, P., Peng, N., et al. (2022). Unraveling the microbial community and succession during zha-chili fermentation and their relationships with flavor formation. Food Res. Int. 157:111239. doi: 10.1016/j.foodres.2022.111239
Dong, W., Yang, Q., Liao, Y., Liu, Y., Hu, Y., Peng, N., et al. (2020). Characterisation and comparison of the microflora of traditional and pure culture xiaoqu during the baijiu liquor brewing process. J. Inst. Brew. 126, 213–220. doi: 10.1002/jib.597
Gan, S. H., Yang, F., Sahu, S. K., Luo, R. Y., Liao, S. L., Wang, H. Y., et al. (2019). Deciphering the composition and functional profile of the microbial communities in Chinese Moutai liquor starters. Front. Microbiol. 10:1540. doi: 10.3389/fmicb.2019.01540
Guan, T., Lin, Y., Chen, K., Ou, M., and Zhang, J. (2020). Physicochemical factors affecting microbiota dynamics during traditional solid-state fermentation of Chinese strong-flavor baijiu. Front. Microbiol. 11:2090. doi: 10.3389/fmicb.2020.02090
He, G., Huang, J., Zhou, R., Wu, C., and Jin, Y. (2019). Effect of fortified Daqu on the microbial community and flavor in Chinese strong-flavor liquor brewing process. Front. Microbiol. 10:56. doi: 10.3389/fmicb.2019.00056
Huang, H., Hu, X., Tian, J., Jiang, X., Luo, H., and Huang, D. (2021). Rapid detection of the reducing sugar and amino acid nitrogen contents of Daqu based on hyperspectral imaging. J. Food Compos. Anal. 101:103970. doi: 10.1016/j.jfca.2021.103970
Huang, P., Jin, Y., Liu, M., Peng, L., Yang, G., Luo, Z., et al. (2023). Exploring the successions in microbial community and flavor of Daqu during fermentation produced by different pressing patterns. Foods 12:2603. doi: 10.3390/foods12132603
Jain, K. K., Bhanja Dey, T., Kumar, S., and Kuhad, R. C. (2014). Production of thermostable hydrolases (cellulases and xylanase) from Thermoascus aurantiacus RCKK: a potential fungus. Bioprocess Biosyst. Eng. 38, 787–796. doi: 10.1007/s00449-014-1320-4
Jiang, Q., Wu, X., Xu, Y., Zhang, Y., Wang, Z., Shen, L., et al. (2021). Microbial composition and dynamic succession during the Daqu production process of northern Jiang-flavored liquor in China. 3 Biotech. 11:224. doi: 10.1007/s13205-021-02779-8
Jin, G., Zhu, Y., and Xu, Y. (2017). Mystery behind Chinese liquor fermentation. Trends Food Sci. Technol. 63, 18–28. doi: 10.1016/j.tifs.2017.02.016
Kuang, J., Han, S., Chen, Y., Bates, C. T., Wang, P., and Shu, W. (2021). Root-associated fungal community reflects host spatial co-occurrence patterns in a subtropical forest. ISME Commun. 1:65. doi: 10.1038/s43705-021-00072-6
Li, H., Liu, S., Liu, Y., Hui, M., and Pan, C. (2023). Functional microorganisms in baijiu Daqu: research progress and fortification strategy for application. Front. Microbiol. 14:1119675. doi: 10.3389/fmicb.2023.1119675
Li, Z., Zheng, M., Zheng, J., and Gänzle, M. G. (2023). Bacillus species in food fermentations: an underappreciated group of organisms for safe use in food fermentations. Curr. Opin. Food Sci. 50:101007. doi: 10.1016/j.cofs.2023.101007
Liu, J., Cui, X., Liu, Z., Guo, Z., Yu, Z., Yao, Q., et al. (2019). The diversity and geographic distribution of cultivable Bacillus-like Bacteria across black soils of Northeast China. Front. Microbiol. 10:1424. doi: 10.3389/fmicb.2019.01424
Mortzfeld, F. B., Hashem, C., Vranková, K., Winkler, M., and Rudroff, F. (2020). Pyrazines: synthesis and industrial application of these valuable flavor and fragrance compounds. Biotechnol. J. 15:2000064. doi: 10.1002/biot.202000064
National Statistics Bureau. (2023). Baijiu industry analysis in China by acquiring data via searching “baijiu” in National Statistics Bureau from Jan to Dec of 2022 (data acquisition in Oct 2023). Available at: https://data.stats.gov.cn.
Niu, J., Yang, S., Shen, Y., Cheng, W., Li, H., Sun, J., et al. (2022). What are the Main factors that affect the flavor of sauce-aroma baijiu. Foods 11:3534. doi: 10.3390/foods11213534
Pan, F., Qiu, S., Lv, Y., and Li, D. (2023). Exploring the controllability of the baijiu fermentation process with microbiota orientation. Food Res. Int. 173:113249. doi: 10.1016/j.foodres.2023.113249
Paredes-López, O., Harry, G. I., and Murray, E. D. (1988). Food biotechnology review: traditional solid-state fermentations of plant raw materials — application, nutritional significance, and future prospects. Crit. Rev. Food Sci. Nutr. 27, 159–187. doi: 10.1080/10408398809527483
Sakandar, H. A., Hussain, R., Farid Khan, Q., and Zhang, H. (2020). Functional microbiota in Chinese traditional baijiu and Mijiu Qu (starters): a review. Food Res. Int. 138:109830. doi: 10.1016/j.foodres.2020.109830
Shi, W., Chai, L. J., Fang, G. Y., Mei, J. L., Lu, Z. M., Zhang, X. J., et al. (2022). Spatial heterogeneity of the microbiome and metabolome profiles of high-temperature Daqu in the same workshop. Food Res. Int. 156:111298. doi: 10.1016/j.foodres.2022.111298
Shi, X., Zhao, S., Chen, S., Han, X., Yang, Q., Zhang, L., et al. (2022). Tetramethylpyrazine in Chinese baijiu: presence, analysis, formation, and regulation. Front. Nutr. 9:1004435. doi: 10.3389/fnut.2022.1004435
Wang, X. D., Ban, S. D., and Qiu, S. Y. (2018). Analysis of the mould microbiome and exogenous enzyme production in Moutai-flavor Daqu. J. Inst. Brew. 124, 91–99. doi: 10.1002/jib.467
Wang, C. D., Chen, Q., Wang, Q., Li, C. H., Leng, Y. Y., Li, S. G., et al. (2014). Long-term batch brewing accumulates adaptive microbes, which comprehensively produce more flavorful Chinese liquors. Food Res. Int. 62, 894–901. doi: 10.1016/j.foodres.2014.05.017
Wang, Z. M., Wang, C. T., Shen, C. H., Wang, S. T., Mao, J. Q., Li, Z., et al. (2020). Microbiota stratification and succession of amylase-producing Bacillus in traditional Chinese Jiuqu (fermentation starters). J. Sci. Food Agric. 100, 3544–3553. doi: 10.1002/jsfa.10405
Wang, B., Wu, Q., Xu, Y., and Sun, B. (2018). Specific volumetric weight-driven shift in microbiota compositions with Saccharifying activity change in starter for Chinese baijiu fermentation. Front. Microbiol. 9:2394. doi: 10.3389/fmicb.2018.02349
Wu, S., Du, H., and Xu, Y. (2023). Daqu microbiota adaptability to altered temperature determines the formation of characteristic compounds. Int. J. Food Microbiol. 385:109995. doi: 10.1016/j.ijfoodmicro.2022.109995
Xia, Y., Luo, H., Wu, Z., and Zhang, W. (2022). Microbial diversity in jiuqu and its fermentation features: saccharification, alcohol fermentation and flavors generation. Appl. Microbiol. Biotechnol. 107, 25–41. doi: 10.1007/s00253-022-12291-5
Yan, Q., Zhang, K., Zou, W., and Hou, Y. (2021). Three main flavour types of Chinese baijiu: characteristics, research, and perspectives. J. Inst. Brew. 127, 317–326. doi: 10.1002/jib.669
Yang, L., Fan, W., and Xu, Y. (2024). Qu-omics elucidates the formation and spatio-temporal differentiation mechanism underlying the microecology of high temperature Daqu. Food Chem. 438:137988. doi: 10.1016/j.foodchem.2023.137988
Yang, L., Xian, C., Li, P., Wang, X., Song, D., Zhao, L., et al. (2023). The spatio-temporal diversity and succession of microbial community and its environment driving factors during stacking fermentation of Maotai-flavor baijiu. Food Res. Int. 169:112892. doi: 10.1016/j.foodres.2023.112892
Yang, Q., Yao, H., Liu, S., and Mao, J. (2022). Interaction and application of molds and yeasts in Chinese fermented foods. Front. Microbiol. 12:664850. doi: 10.3389/fmicb.2021.664850
Zhang, J., Du, R., Niu, J., Ban, S., Zhang, Y., Xu, L., et al. (2024). Daqu and environmental microbiota regulate fatty acid biosynthesis via driving the core microbiota in soy sauce aroma type liquor fermentation. Int. J. Food Microbiol. 408:110423. doi: 10.1016/j.ijfoodmicro.2023.110423
Zhang, Y., Shen, Y., Cheng, W., Wang, X., Xue, Y., Chen, X., et al. (2021). Understanding the shifts of microbial community and metabolite profile from wheat to mature Daqu. Front. Microbiol. 12:714726. doi: 10.3389/fmicb.2021.714726
Zhang, Y., Shen, Y., Niu, J., Ding, F., Ren, Y., Chen, X., et al. (2022). Bacteria-induced amino acid metabolism involved in appearance characteristics of high-temperature Daqu. J. Sci. Food Agric. 103, 243–254. doi: 10.1002/jsfa.12136
Zhang, R., Wu, Q., and Xu, Y. (2013). Aroma characteristics of Moutai-flavour liquor produced with Bacillus licheniformis by solid-state fermentation. Lett. Appl. Microbiol. 57, 11–18. doi: 10.1111/lam.12087
Zhu, Q., Chen, L., Peng, Z., Zhang, Q., Huang, W., Yang, F., et al. (2022). Analysis of environmental driving factors on Core functional community during Daqu fermentation. Food Res. Int. 157:111286. doi: 10.1016/j.foodres.2022.111286
Zhu, Q., Chen, L., Pu, X., Du, G., Yang, F., Lu, J., et al. (2023). The differences in the composition of Maillard components between three kinds of sauce-flavor Daqu. Fermentation 9:860. doi: 10.3390/fermentation9090860
Zhu, C., Cheng, Y., Shi, Q., Ge, X., Yang, Y., and Huang, Y. (2023). Metagenomic analyses reveal microbial communities and functional differences between Daqu from seven provinces. Food Res. Int. 172:113076. doi: 10.1016/j.foodres.2023.113076
Zhu, C., Cheng, Y., Zuo, Q., Huang, Y., and Wang, L. (2022). Exploring the impacts of traditional crafts on microbial community succession in Jiang-flavored Daqu. Food Res. Int. 158:111568. doi: 10.1016/j.foodres.2022.111568
Keywords: Daqu maturation, microbial community, Kroppenstedtia, color formation, pyrazines
Citation: Dong W, Yu X, Wang L, Zou M, Ma J, Liu J, Feng Y, Zhao S, Yang Q, Hu Y and Chen S (2024) Unveiling the microbiota of sauce-flavor Daqu and its relationships with flavors and color during maturation. Front. Microbiol. 15:1345772. doi: 10.3389/fmicb.2024.1345772
Received: 28 November 2023; Accepted: 11 January 2024;
Published: 24 January 2024.
Edited by:
Dimitrios Tsaltas, Cyprus University of Technology, CyprusCopyright © 2024 Dong, Yu, Wang, Zou, Ma, Liu, Feng, Zhao, Yang, Hu and Chen. This is an open-access article distributed under the terms of the Creative Commons Attribution License (CC BY). The use, distribution or reproduction in other forums is permitted, provided the original author(s) and the copyright owner(s) are credited and that the original publication in this journal is cited, in accordance with accepted academic practice. No use, distribution or reproduction is permitted which does not comply with these terms.
*Correspondence: Yuanliang Hu, eWxodUBoYm51LmVkdS5jbg==; Shenxi Chen, Y2hlbnNoZW54aTIwMDZAMTYzLmNvbQ==
†These authors have contributed equally to this work
Disclaimer: All claims expressed in this article are solely those of the authors and do not necessarily represent those of their affiliated organizations, or those of the publisher, the editors and the reviewers. Any product that may be evaluated in this article or claim that may be made by its manufacturer is not guaranteed or endorsed by the publisher.
Research integrity at Frontiers
Learn more about the work of our research integrity team to safeguard the quality of each article we publish.