Erratum: Genetic diversity of endosymbiotic bacteria Wolbachia infecting two mosquito species of the genus Eretmapodites occurring in sympatry in the Comoros archipelago
- 1Université de La Réunion, UMR PIMIT (Processus Infectieux en Milieu Insulaire Tropical) CNRS 9192, INSERM 1187, IRD 249, Saint-Denis, île de La Réunion, France
- 2Service de lutte antivectorielle, ARS Mayotte, Kawéni, France
- 3National Malaria Control Program, Moroni, Comoros
- 4UMR MIVEGEC (Maladies Infectieuses et Vecteurs: Écologie, Génétique, Évolution et Contrôle), IRD, CNRS, Université de Montpellier, Montpellier, France
Introduction: The influence of Wolbachia on mosquito reproduction and vector competence has led to renewed interest in studying the genetic diversity of these bacteria and the phenotypes they induced in mosquito vectors. In this study, we focused on two species of Eretmapodites, namely Eretmapodites quinquevittatus and Eretmapodites subsimplicipes, from three islands in the Comoros archipelago (in the Southwestern Indian Ocean).
Methods: Using the COI gene, we examined the mitochondrial genetic diversity of 879 Eretmapodites individuals from 54 sites. Additionally, we investigated the presence and genetic diversity of Wolbachia using the wsp marker and the diversity of five housekeeping genes commonly used for genotyping through Multiple Locus Sequence Typing (MLST).
Results and discussion: Overall, Er. quinquevittatus was the most abundant species in the three surveyed islands and both mosquito species occurred in sympatry in most of the investigated sites. We detected a higher mitochondrial genetic diversity in Er. quinquevittatus with 35 reported haplotypes (N = 615 specimens, Hd = 0.481 and π = 0.002) while 13 haplotypes were found in Er. subsimplicipes (N = 205 specimens, Hd = 0.338 and π = 0.001), this difference is likely due to the bias in sampling size between the two species. We report for the first time the presence of Wolbachia in these two Eretmapodites species. The prevalence of Wolbachia infection varied significantly between species, with a low prevalence recorded in Er. quinquevittatus (0.8%, N = 5/627) while infection was close to fixation in Er. subsimplicipes (87.7%, N = 221/252). Both male and female individuals of the two mosquito species appeared to be infected. The analysis of MLST genes revealed the presence of two Wolbachia strains corresponding to two new strain types (STs) within the supergroups A and B, which have been named wEretA and wEretB. These strains were found as mono-infections and are closely related, phylogenetically, to Wolbachia strains previously reported in Drosophila species. Finally, we demonstrate that maternal transmission of Wolbachia is imperfect in Er. subsimplicipes, which could explain the presence of a minority of uninfected individuals in the field.
Introduction
Endosymbiotic bacteria are of increasing interest due to their impact on the biology of arthropods. Some of these bacteria are known to be essential for the evolution of their hosts enabling them to adapt to new ecological niches (Douglas, 1998). Other bacteria provide selective advantages depending on the ecological contexts by providing for example protection against predators (Tsuchida et al., 2010) or pathogens (Oliver et al., 2003; Scarborough et al., 2005; Hedges et al., 2008; Teixeira et al., 2008; Jaenike et al., 2010). In addition to these positive effects, endosymbiotic bacteria are also selfish elements that can manipulate the reproduction of their hosts to increase their own fitness (Duron et al., 2008). This is the case for the bacteria Wolbachia which are associated with various reproductive manipulation phenotypes in arthropods (Werren et al., 2008).
Wolbachia are maternally inherited alpha-proteobacteria commonly found in arthropods and filarial nematodes (Werren et al., 2008). These bacteria are estimated to be present in up to 66% of insect species (Hilgenboecker et al., 2008; Zug and Hammerstein, 2012; Weinert et al., 2015), thus probably representing the most abundant endosymbiont described to date. Wolbachia exibit high genetic diversity and have been classified into 17 phylogenetic groups or supergroups (A to Q) (Baldo et al., 2006; Paraskevopoulos et al., 2006; Bordenstein et al., 2009; Ros et al., 2009; Glowska et al., 2015). The widespread distribution of Wolbachia is primarily attributed to their impact on the reproductive biology of their hosts. In arthropods, Wolbachia manipulate host reproduction by biasing the sex ratio toward females (the transmitting sex), or by causing sterility through a phenomenon known as cytoplasmic incompatibility (CI) (Werren et al., 2008). Cytoplasmic incompatibility results from sperm-egg incompatibility occuring when Wolbachia-infected males mate with either uninfected females or females infected with an incompatible Wolbachia strain, resulting in high embryonic mortality reaching up to 100% in certain mosquito species (Laven, 1951; Werren et al., 2008; Atyame et al., 2014). This phenotype is commonly observed in arthropods (Shropshire et al., 2020; Turelli et al., 2022) including in mosquito vectors (Sicard et al., 2019).
Aside from the manipulation of reproduction, Wolbachia can also impact the vector competence of mosquitoes, which refers to their ability to become infected with and transmit a pathogen. Wolbachia have shown to provide protection against major mosquito-borne pathogens like Dengue virus (DENV), Chikungunya virus (CHIKV) or Plasmodium infections (Moreira et al., 2009; Bian et al., 2010, 2013; Hoffmann et al., 2011; Walker et al., 2011; Aliota et al., 2016; Dutra et al., 2016). However, Wolbachia can also be linked to increased pathogen transmission in some cases (Hughes et al., 2012; Dodson et al., 2014; Zélé et al., 2014). Because of Wolbachia's influence on both mosquito reproduction and vector competence, these bacteria are increasingly seen as promising tools for mosquito and mosquito-borne diseases control (Bourtzis et al., 2014). In recent years, there has a growing number of studies focusing on the genetic diversity of Wolbachia and their associated phenotypes in mosquito vectors (Sicard et al., 2019). Wolbachia have been well studied in various medically importance mosquito species of such as Culex pipiens pipiens and Culex pipiens quinquefasciatus (Duron et al., 2005; Atyame et al., 2011, 2014; Dumas et al., 2013), Aedes albopictus (Kambhampati et al., 1993; Armbruster et al., 2003; Tortosa et al., 2010; Zouache et al., 2011), as well as more recently in Anopheles species (Baldini et al., 2014; Ayala et al., 2019) and Aedes aegypti (Coon et al., 2016; Thongsripong et al., 2018). However, there have been limited studies on the presence of Wolbachia in mosquitoes of the Eretmapodites genus (Tokash-Peters et al., 2022; Osuna et al., 2023), despite their role in the arbovirus transmission (arthropod-borne viruses) (Bamou et al., 2021; Cêtre-Sossah et al., 2023).
Mosquitoes from the Eretmapodites genus (Theobald, 1901) (subfamily: Culicinae; tribe: Aedini) are exclusively Afrotropical species occurring in continental Africa (Harbach, 2007), Madagascar (Tantely et al., 2016), and in the islands of the Comoros archipelago (composed of four volcanic islands: Grande Comore, Mohéli, Anjouan and Mayotte) within the Southwestern Indian Ocean (Le Goff et al., 2014; Boussès et al., 2018). A total of 51 Eretmapodites species have been described so far (https://mosquito-taxonomic-inventory, accessed in November 2023) (Harbach, 2013), most (32 species) from Cameroon (Bamou et al., 2021), while only four species are known in Madagascar (Tantely et al., 2016), and two species are reported in the Comoros archipelago (Le Goff et al., 2014; Boussès et al., 2018). Eretmapodites species are mostly found in forested areas but some species are also adapted to rural and peri-urban environments (Le Goff et al., 2014; Boussès et al., 2018; Bamou et al., 2021). Along with their aggressive daytime biting behavior, these mosquitoes are known to bite both animals and humans (Musa et al., 2020); as a result they have the potential to serve as bridge vectors of pathogens between animals and humans. Different arboviruses such as Rift Valley fever virus (RVFV), Semliki Forest virus (SFV), or CHIKV have been detected and/or isolated from Eretmapodites mosquitoes [review in Bamou et al. (2021)]. In addition, some studies have described the ability of Eretmapodites mosquitoes to transmit arboviruses under laboratory conditions. For example, Bauer (1928) showed that Eretmopodites chrysogaster is able to transmit the Yellow Fever virus (YFV). The study of Mclntosh and coworkers (McIntosh et al., 1980) demonstrated the ability of Eretmapodites quinquevittatus to transmit the RVFV. Likewise, it has been recently shown that Eretmapodites subsimplicipes is a competent vector for the transmission of RVFV (Cêtre-Sossah et al., 2023). Despite the medical interest of Eretmapodites species, the biology, ecology and genetics of these mosquitoes remain poorly investigated.
In this study we focused on two Eretmapodites species, namely Er. quinquevittatus and Er. subsimplicipes, from three islands in the Comoros archipelago: Grande Comore, Mohéli and Mayotte. We used molecular identification to determine the abundance of each mosquito species on the surveyed islands. Then, we examined the presence of Wolbachia in both Eretmapodites species through the presence/absence of the Wolbachia surface protein gene wsp (Braig et al., 1998). The genetic diversity of the detected Wolbachia was further characterized by sequencing the wsp gene and the five housekeeping genes developed for the Wolbachia typing (MLST) (Baldo et al., 2006). Finally, we examined the vertical transmission of Wolbachia in a laboratory colony of Er. subsimplicipes. The role of Wolbachia in the evolution of Eretmapodites species is discussed.
Materials and methods
Mosquito sampling
Adult Eretmapodites specimens were collected in 2019 (March to May and November to December) from 54 natural breeding sites on three islands of the Comoros archipelago (in the Southwestern Indian Ocean): Grande Comore (18 sites), Mohéli (eight sites), and Mayotte (28 sites) (Figure 1; Supplementary Table 1). Larvae were also collected in the site Bambo Est on Mayotte in March 2019 and March 2022 to establish a laboratory colony for testing vertical transmission of Wolbachia (see below). Adult mosquitoes were captured using portable electric aspirators (BioQuip InsectaVac aspirator, Bioquip, CA). Collected adults were introduced in cages (16 × 16 × 16 cm) and brought to the laboratory where they were sorted by sex and individually stored in 1.5 ml tubes at −80°C (for samples from Mayotte) or in 70% ethanol (for samples from Grande Comore and Mohéli) until morphological identification and molecular analyses.
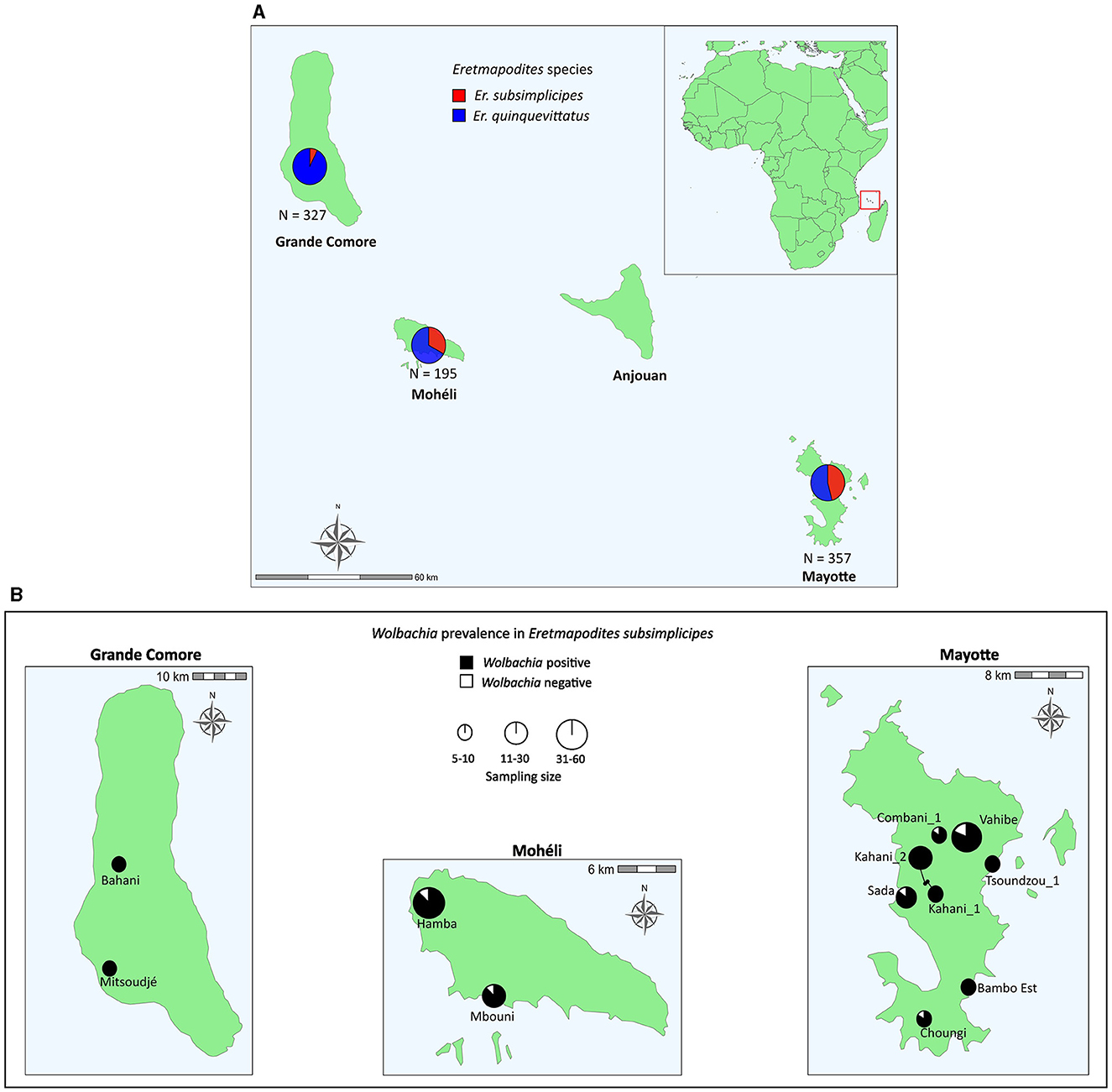
Figure 1. Maps showing the abundance of Eretmapodites quinquevittatus and Eretmapodites subsimplicipes (A) and the prevalence of Wolbachia in field populations of Er. subsimplicipes (B) in three islands of the Comoros archipelago (Grande Comore, Mohéli and Mayotte). Mosquitoes were sampled as adults and identified with the sequencing of the mitochondrial COI gene. The prevalence of Wolbachia is based on the presence/absence of the wsp gene. Only sites with more than five individuals are shown (see Supplementary Table 1 for more details).
Mosquito identification
The larvae and adults of Eretmapodites from the Bambo Est site (Mayotte) and collected in March 2019 and March 2022 were identified morphologically using taxonomic keys (Edwards, 1941; Hopkins, 1952; Service, 1990). The larvae of Er. quinquevittatus have a characteristic thick, heavily chitinized 3-VIII seta and, in adults, the scutum is decorated with five parallel bands of dark scales on a yellow-brown background. The larvae of Er. subsimplicipes are easily distinguished from those of Er. quinquevittatus by lateral setae of abdominal segments I-VI inserted on a sclerotized conical tubercle and, in adults, a more homogeneous scutum devoid of such dark bands. Molecular identification of species was realized on larvae and all collected adult specimens through PCR amplification and sequencing of a 658 bp fragment of the mitochondrial cytochrome c oxidase subunit 1 encoding gene (COI) (Folmer et al., 1994) (primers listed in Supplementary Table 2). DNA extraction, PCR, and sequencing were performed as described below.
Wolbachia genotyping
Wolbachia detection was performed in all sampled adults using PCR targeting the surface protein gene wsp (Braig et al., 1998) (Supplementary Table 2) which is more variable than the slowly evolving 16S rRNA gene (Zhou et al., 1998). For wsp-positive samples, Wolbachia were genotyped by sequencing the wsp gene and the five MLST loci: coxA, fbpA, ftsZ, gatB and hcpA (Baldo et al., 2006) (Supplementary Table 2). For all individuals in which Wolbachia DNA could not be amplified, the quality of the DNA template was checked by the amplification of the COI gene.
PCR amplification and sequencing
DNA was extracted from individual mosquitoes using the QIAcube HT robotic workstation and the associated Cador Pathogen 96 QIAcube HT Kit (Qiagen) following manufacturer's recommendations, eluted with 100 μl AVE buffer (Qiagen) and eventually stored at −20°C until molecular investigations. PCRs were performed with 0.5 ng of genomic DNA in a 25 μl final volume reaction containing 8.5 μl of water, 12.5 μl of GoTaq® G2 HotStart Green Master Mix (Promega), and 1 μl of each primer (10 μM) (Supplementary Table 2). All PCR programs included an initial denaturation step at 95°C for 5 min, followed by 36 cycles (30 cycles for the COI gene) at 94°C for 30 s, 52°C−59°C for 60 s and 72°C for 90 s, and a final elongation step at 72°C for 7 min. Amplified DNA fragments were ran on 1.5% agarose gel electrophoresis stained with 1X GelRedTM (Biotium Inc.) and visualized under ultraviolet light. PCR products were Sanger sequenced on both strands (Genoscreen, Lille, France). Only unique generated sequences were submitted to GenBank under the following accession numbers: OR282837-OR282884, OR296528-OR296530, OR296531-OR296533, OR296534-OR296536, OR296537-OR296539, OR296540-OR296543, and OR296544-OR296547 for COI, coxA, fbpA, ftsZ, gatB, hcpA, and wsp, respectively.
Sequences
All sequences were visually inspected and manually edited using Geneious Prime v.2022.2.2 (Kearse et al., 2012). For the COI gene, comparisons with public sequences were performed using basic local alignment search tool (BLAST) (www.ncbi.nlm.nih.gov/BLAST, accessed on 28 July 2023) from GenBank. The mitochondrial haplotype diversity (Hd) and nucleotide diversity (π) were calculated in the software DnaSP v6.12.03 (Rozas et al., 2017). For Wolbachia genes, the generated sequences were compared with data available in the Wolbachia MLST database (https://pubmlst.org/organisms/wolbachia-spp, accessed on 03 August 2023) (Jolley et al., 2018). For each MLST gene, a new allele was considered if there was at least one nucleotide difference with alleles already present in the pubMLST database. Thereafter, the combination of alleles allowed identifying the Sequence Types (STs) among those existing or to propose new STs.
Phylogenetic analysis
Phylogenetic relationships were evaluated for Eretmapodites COI and Wolbachia genes. Only unique mitochondrial haplotypes and bacterial alleles were included in the analyses. For Wolbachia, phylogenetic analyses were conducted for each of the six sequenced genes and on all five MLST concatenated genes (coxA, fbpA, ftsZ, gatB, and hcpA, in this order). The phylogenies were constructed with data from Baldo et al. (2006). For each data set, the best-fitting model of sequence evolution was determined using jMoldelTest v.2.1.4 (Darriba et al., 2012). Then, phylogenetic constructions were performed using MrBayes v.3.2.3 (Ronquist et al., 2012). For each phylogeny, the analysis corresponded to two independent runs of four incrementally heated Metropolis Coupled Markov Chain Monte Carlo (MCMCMC) starting from a random tree. The MCMCMC was run for 10 million generations with trees and associated model parameters sampled every 100 generations. The convergence level was accessed with an average standard deviation of split frequencies inferior to 0.05. The 10% initial trees for each run were discarded as burn-in and the phylogeny along with posterior probabilities were obtained from the remaining trees. The resulting Bayesian phylogeny trees were visualized and annotated with FigTree v.1.4.2 (Rambaut, 2014).
Vertical transmission of Wolbachia
Since only the Er. subsimplicipes species could be reared from larvae collected in the Bambo Est site (Mayotte) in March 2019, we used it to examine the vertical transmission of Wolbachia. Field larvae (F0 generation) were brought to the laboratory and kept alive in the insectary where they were identified morphologically and maintained under standard rearing conditions (27°C and 80% relative humidity with a 12h:12h photoperiod). Larvae were supplied every 2 days with yeast tablets and adults were fed with 10% sucrose solution. To get eggs and ensure the maintenance of mosquitoes, females were blood-fed using a Hemotek feeding system (Hemotek Limited, GreatHarwood, UK) with defibrinated cow blood. The eggs of the next generation (F1 generation) were collected and reared to adulthood. The amplification process was performed over four generations (F4 generation) to increase the number of females for the experiment. Then, the vertical transmission of Wolbachia was assessed using females and males from the established laboratory colony. Females of the F4 generation were allowed to mate in the laboratory with males from the same colony. After mating, the females were blood-fed and individually isolated to lay eggs. Then, the presence of Wolbachia was tested for each female by PCR using the wsp gene as described above. The offsprings from each Wolbachia-infected female were kept alive until adulthood and males and females were screened for the presence of Wolbachia.
Results
Eretmapodites quinquevittatus is more abundant than Eretmapodites subsimplicipes
Larvae and adults collected in the site Bambo Est (Mayotte) in March 2019 and March 2022 were morphologically identified as Er. subsimplicipes. COI sequencing of these morphologically identified specimens showed a closed match with the published sequence of Er. subsimplicipes from Mozambique (GenBank accession number: LC664011, 99.8%−100.0% percentage of identity based on 633 bp), thus confirming the identification of our specimens. We then sequenced a total of 879 mosquitoes (655 females and 224 males) from Grande Comore (N = 327), Mohéli (N = 195), and Mayotte (N = 357). The comparison of the obtained COI sequences with the GenBank database indicated the presence of two Eretmapodites species: Er. quinquevittatus (GenBank accession number: LC664009, 98.4%−100.0% percentage of identity based on 629 bp) and Er. subsimplicipes (GenBank accession number: LC664011, 99.7%−100.0% percentage of identity based on 633 bp). Among the sequences, 71.3% (N = 627/879) and 28.7% (N = 252/879) belonged to Er. quinquevittatus and Er. subsimplicipes, respectively (Supplementary Tables 1, 3). Eretmapodites quinquevittatus appeared more common than Er. subsimplicipes in all three investigated islands (Figure 1A). Both Eretmapodites species were found in sympatry in 31 out of 54 sampled sites (seven sites in Grande Comore, seven sites in Mohéli and 17 sites in Mayotte), while Er. quinquevittatus was found alone in 22 sites (11 sites in Grande Comore, one site in Mohéli and ten sites in Mayotte) and Er. subsimplicipes alone at one site (Bambo Est) on Mayotte (Supplementary Table 1).
Higher mtDNA polymorphism in Er. quinquevittatus
Among the 627 Er. quinquevittatus specimens, COI sequences with good qualities (i.e., 658 bp with no ambiguities) were obtained for 615 samples leading to 35 haplotypes (Figure 2A; Supplementary Table 4). Pairwise nucleotide identity between the haplotypes ranged from 98.2% to 99.9%. The overall haplotype diversity (Hd) and nucleotide diversity (π) values were 0.481 and 0.002, respectively. The most frequent haplotype [EQ_H01, found in 71.4% of sequences (N = 439/615)] was also the most widespread in all three islands (Figure 2A; Supplementary Table 4). The second most frequent haplotype (EQ_H27, scored in 43 specimens) was geographically restricted to Mayotte (Supplementary Table 4). Of the 35 haplotypes, four haplotypes were shared by all three islands (EQ_H01, EQ_H10, EQ_H11, and EQ_H14), two haplotypes (EQ_H02 and EQ_H07) were shared by Grande Comore and Mohéli, one haplotype (EQ_H16) was common to Grande Comore and Mayotte, and no common haplotype was detected between Mohéli and Mayotte (Supplementary Table 4). On Grande Comore, 18 haplotypes were found whereas the number of haplotypes was similar between Mohéli and Mayotte (14 haplotypes on each island). A total of 11, eight and nine haplotypes were unique on Grande Comore, Mohéli and Mayotte, respectively (Figure 2A; Supplementary Table 4).
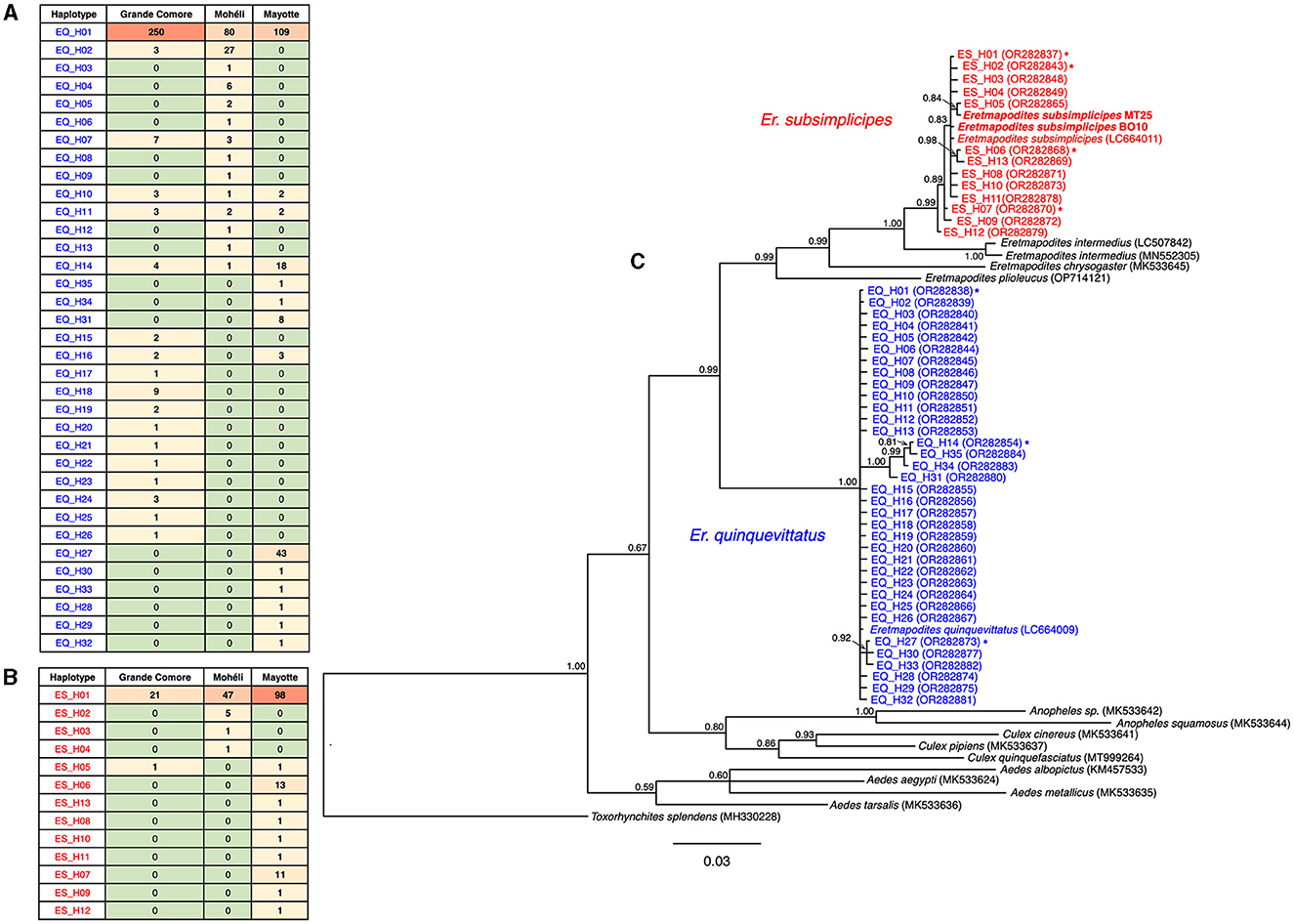
Figure 2. (A) Heatmap showing the distribution of COI haplotypes in Eretmapodites quinquevittatus from three islands of Comoros archipelago; (B) Heatmap showing the distribution of the COI haplotypes in Eretmapodites subsimplicipes from the three surveyed islands; (C) Bayesian phylogenetic tree based on COI gene (658 bp, 66 sequences) showing relationships between Er. subsimplicipes (in red) Er. quinquevittatus (in blue) mosquitoes. The phylogenetic tree was built using the substitution model: GTR+I+G. Only unique mtDNA haplotypes are shown: ES_H01 to ES_H13 for Er. subsimplicipes and EQ_H01 to EQ_H32 for Er. quinquevittatus. Asterisks indicate mitochondrial haplotypes detected in both Wolbachia infected and uninfected specimens. Names in bold indicate specimens morphologically identified as Er. subsimplicipes in this study. GenBank accession numbers are indicated in brackets. The tree was rooted on Toxorhynchites splendens and the numbers associated with nodes indicate the posterior probability. The scale bar is in units of substitutions/site.
COI good quality sequences were obtained for 205 of the 252 Er. subsimplicipes samples leading to 13 haplotypes (Figure 2B; Supplementary Table 5). Pairwise nucleotide identity between the haplotypes yielded values ranging from 99.4 to 99.9%. The overall Hd and π values were 0.338 and 0.001, respectively. The haplotype ES_H01 was the most frequently observed in the dataset, with 80.9% of specimens (N = 166/205) and the only one common to all three islands (Figure 2B; Supplementary Table 5). The number of haplotypes was higher in Mayotte (ten haplotypes), followed by Mohéli (four haplotypes), while the lowest diversity was observed in Grande Comore (two haplotypes). Unique haplotypes were only found in Mayotte (eight haplotypes) and Mohéli (three haplotypes) (Figure 2B; Supplementary Table 5).
We assessed phylogenetic relationships between the two Eretmapodites species by incorporating the COI haplotypes identified in the present study and those of other mosquito species retrieved from GenBank including sequences of Eremapodites mosquitoes: Er. quinquevittatus (GenBank: LC664009), Er. subsimplicipes (GenBank: LC664011), Eretmapodites intermedius (GenBank: LC507842 and MN552305), Eretmapodites chrysogaster (GenBank: MK533645), and Eretmapodites plioleucus (GenBank: OP714121). The phylogenetic tree revealed that Er. quinquevittatus and Er. subsimplicipes formed two well-supported clades (Figure 2C). Although higher haplotype diversity was found in Er. quinquevittatus, the genetic cluster formed by Er. subsimplicipes appears slightly more diverged than that of Er. quinquevittatus.
Lower prevalence of Wolbachia in Er. quinquevittatus than in Er. subsimplicipes
The 879 Eretmapodites mosquitoes were screened for Wolbachia infection based on the detection of the wsp gene by PCR. The overall prevalence of Wolbachia was 25.7% (N = 226/879), with a significant lower prevalence detected in Er. quinquevittatus (0.8%, N = 5/627) as compared to Er. subsimplicipes (87.7%, N = 221/252) (Table 1) (Fisher's exact test, P < 0.001). Wolbachia infections were detected in both males and females of both mosquito species (Table 1). In Er. quinquevittatus, two out of the five Wolbachia infected mosquitoes were females and three were males. In contrast, the majority of the Wolbachia infected Er. subsimplicipes mosquitoes were females, with 201 females and 3 males out of a total of 221 infected mosquitoes. For Er. quinquevittatus, the bacterial infection prevalence between sites ranged from 0.0% to 7.7% with the five Wolbachia-infected specimens detected from five sites: two sites in Grande Comore, one site in Mohéli and two sites in Mayotte (Supplementary Table 1). For Er. subsimplicipes, Wolbachia-positive specimens were detected in all but one site (the Iconi site on Grande Comore, N = 32 sites with Er. subsimplicipes specimens) and infection prevalence ranged from 50.0% to 100.0% including in sites with a large number of samples (Figure 1B; Supplementary Table 1). For both mosquito species, Wolbachia infection prevalence did not significantly vary according to the sampled islands (Fisher's exact tests, all P > 0.7) (Supplementary Table 1).
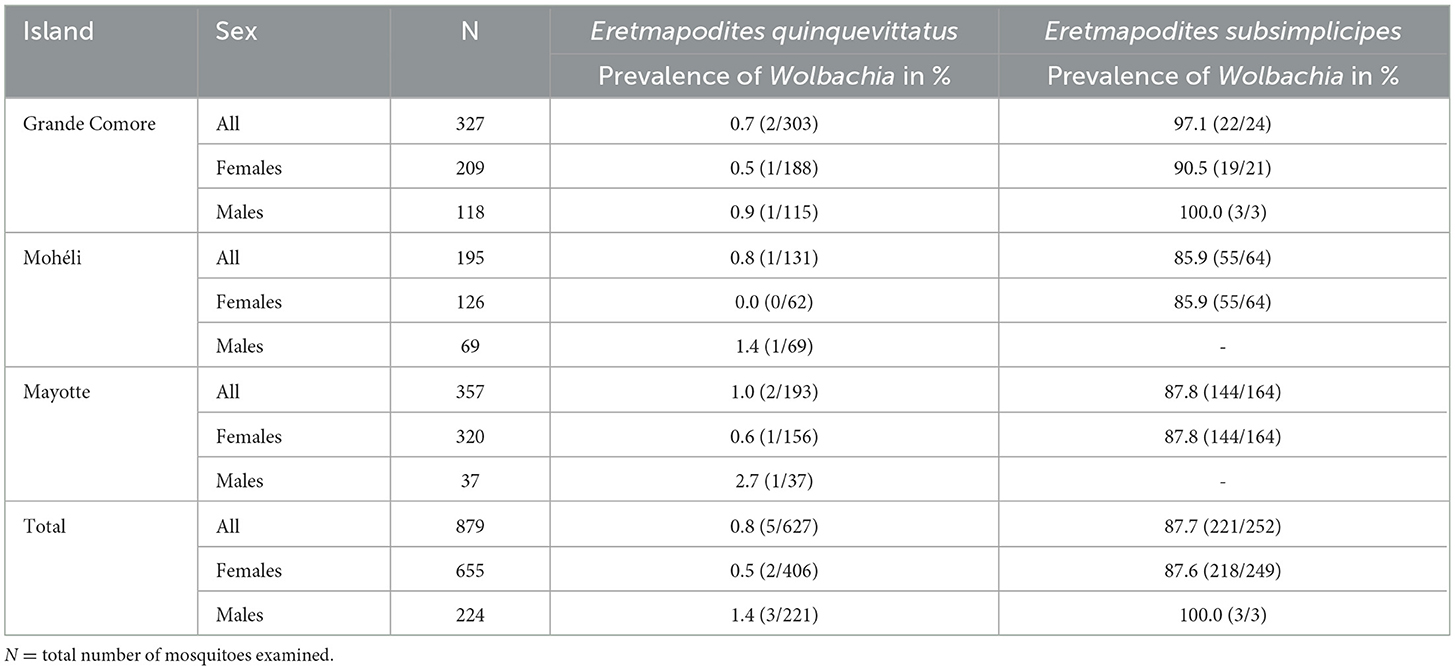
Table 1. Prevalence of Wolbachia in Eretmapodites quinquevittatus and Eretmapodites subsimplicipes in three islands of the Comoros archipelago, based on presence/absence of the wsp gene and according to the sex of mosquitoes.
Two Wolbachia A and B supergroups occurred in Er. quinquevittatus and Er. subsimplicipes
The sequencing of the wsp gene in Er. quinquevittatus and Er. subsimplicipes revealed the presence of two Wolbachia supergroups A and B in each Eretmapodites species (Figure 3). For Er. quinquevittatus, three samples out of the five Wolbachia-infected were successfully sequenced and one sample belonged to supergroup A while two samples belonged to supergroup B (Figure 3; Supplementary Table 3). Concerning Er. subsimplicipes, the sequencing of the wsp gene was succeful for 218 out of the 221 samples. Almost all of these samples (N = 217/218) belonged to supergroup A and one sample to supergroup B. When comparing the 218 wsp sequences of supergroup A (217 sequences for Er. subsimplicipes and one sequence for Er. quinquevittatus), no polymorphism was noted, a unique wsp allele shared by the two mosquito species was observed (Figure 3; Supplementary Table 3). The analysis of the three wsp sequences from supergroup B (two sequences for Er. quinquevittatus and one sequence for Er. subsimplicipes) also revealed one wsp allele shared by both Eretmapodites species.
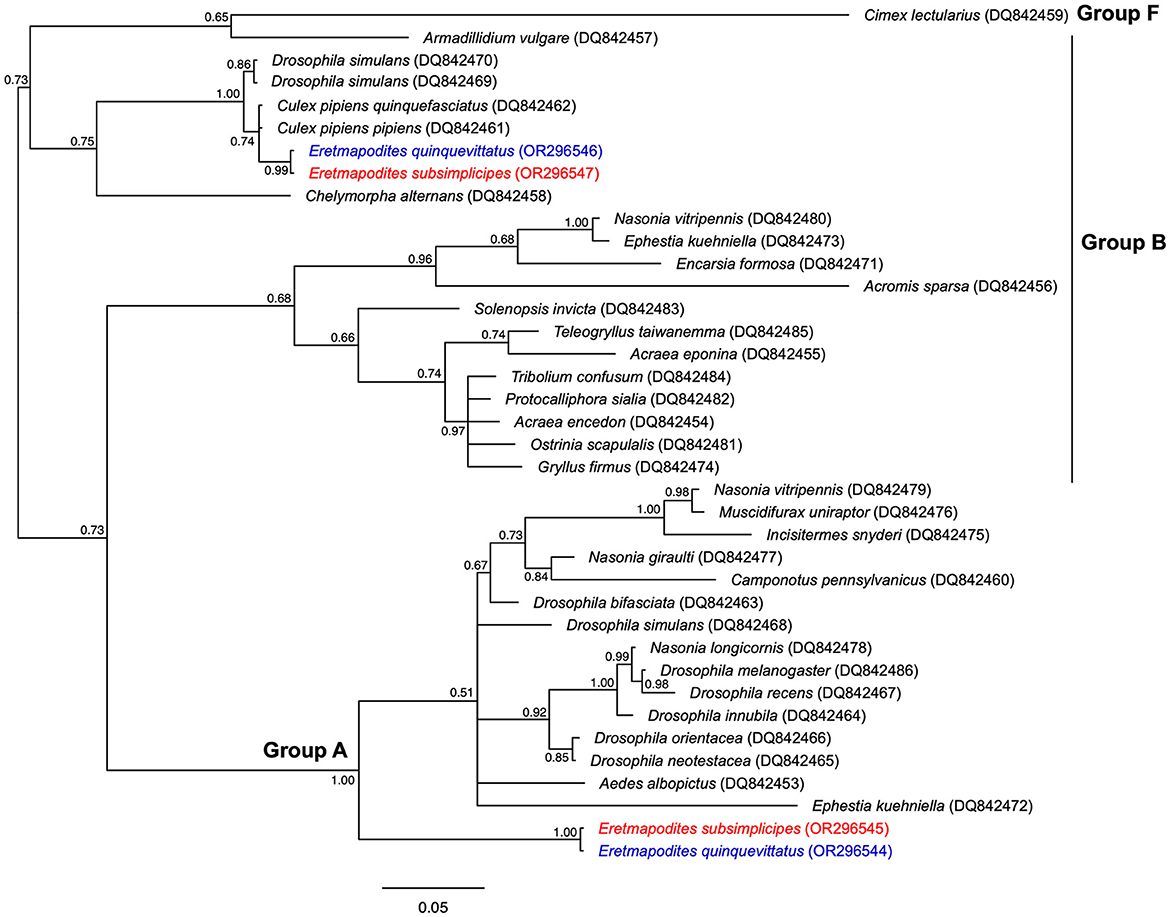
Figure 3. Bayesian phylogenic tree of Wolbachia strains based the wsp gene (513 bp, 38 sequences). The phylogenetic tree was built using the substitution model: GTR+I+G. Sequences in red and blue correspond to Wolbachia sequences detected in Er. subsimplicipes and Er. quinquevittatus, respectively. The accession numbers of Wolbachia strains identified in these mosquito species are also indicated. For each sequence the host species is indicated and the GenBank accession number is provided in brackets. The letters A, B and F correspond to Wolbachia supergroups. The tree is midpoint unrooted and the numbers associated with nodes correspond to posterior probability values. The scale bar is in units of substitutions/site.
As the wsp gene alone is not relevant for a reliable genotyping of Wolbachia strains due to recombination in Wolbachia genomes (Jiggins et al., 2001; Bordenstein and Wernegreen, 2004; Baldo et al., 2006; Atyame et al., 2011), we sequenced the five Wolbachia MLST genes coxA, fbpA, ftsZ, gatB and hcpA. The sequences of the five MLST genes were not obtained systematically for each of the 226 Wolbachia-infected Eretmapodites mosquitoes. Indeed, PCR amplifications have failed for some genes (particularly fbpA) in certain samples, possibly due to mutations in the targeted primers sites. Additionally, since we used universal degenerated primers (Baldo et al., 2006), it may have been possible to improve our protocols to increase amplification success for Eretmapodites. Ultimately, we obtained 214 sequences for coxA, 114 sequences for fbpA, 210 sequences for gatB, 177 sequences for hcpA and 214 sequences for fstZ. We confirmed the presence of Wolbachia strains belonging to supergroups A and B with each of the five MLST genes (Supplementary Figures 1–5; Supplementary Table 3). We found two alleles for four of the five genes (coxA, fbpA, ftsZ and gatB), one allele belonging to supergroup A and the other one to supergroup B (Supplementary Figures 1–4; Supplementary Table 3). The most polymorphic locus was hcpA with three alleles, two alleles for supergroup A and one allele for supergroup B (Supplementary Figure 5; Supplementary Table 3). The two hcpA alleles falling in the supergroup A were genetically close, with 99.8% pairwise identity based on 476 bp. Our data do not support co-infection by Wolbachia strains from supergroups A and B. None of the five MLST genes could be amplified in the single Er. quinquevittatus sample infected with a Wolbachia strain from supergroup A. Therefore, using the MLST genes, we detected supergroup A only in Er. subsimplicipes and supergroup B in both Er. subsimplicipes and Er. quinquevittatus (Supplementary Figures 1–5; Supplementary Table 3). As observed with the wsp gene, all MLST alleles were shared by the two mosquito species within supergroup B (Supplementary Figures 1–5).
Comparison of allelic polymorphism with pubMLST database revealed that within the supergroup A, alleles identified in the present study for coxA, fbpA, ftsZ, and hcpA are new with the exception of the gatB allele matching with allele #49 (Supplementary Table 6). The coxA allele showed a close match with allele #173, the fbpA allele with allele #60, the ftsZ allele with allele #52, and the two hcpA alleles were genetically closely related to allele #11 (Supplementary Table 6). The combination of the five alleles resulted in a new Wolbachia strain type, which we named “wEretA.” For supergroup B, all observed alleles for the five MLST genes are already present in the pubMLST database. Indeed, coxA, fbpA, ftsZ, gatB, and hcpA alleles matched with alleles #281, #453, #244, #283, and #309, respectively (Supplementary Table 6). However, no Wolbachia strain type was assigned to the combination of these five alleles in the pubMLST database. Hence, we considered this Wolbachia strain type as new and named it “wEretB.” The MLST allelic profiles of wEretA and wEretB appeared genetically different from those of a Wolbachia strain previously described in the species Eretmapodites chrysogaster from Cameroon for which coxA matched with #275, ftsZ matched with #106, and fbpA matched with #6 (Osuna et al., 2023). Using complete MLST profiles obtained for 84 mosquitoes (83 Er. subsimplicipes and one Er. quinquevittatus), we performed a phylogenetic analysis based on the 2,079 bp concatenated sequences of the five MLST genes. It appears that Wolbachia strains wEretA (infecting Er. subsimplicipes) and wEretB (infecting both Er. subsimplicipes and Er. quinquevittatus) form two robust monophyletic clades within A and B supergroups, respectively (Figure 4). wEretA is genetically closely related to wDori and wDneo infecting Drosophila orientacea and Drosophila neotestacea, respectively (Figure 4). wEretB is closely related to wMa infecting Drosophila simulans (Figure 4). In summary, MLST data revealed that (i) wEretA is restricted to Er. subsimplicipes (83 complete MLST allelic profiles) and (ii) wEretB infects both Er. subsimplicipes and Er. quinquevittatus (1 complete MLST allelic profile each) (Figure 4; Supplementary Table 3). Finally, we examined the evolution of the diversity of Wolbachia in their hosts by comparing the concatenated MLST phylogeny and the COI phylogeny from different host species including in Er. subsimplicipes and Er. quinquevittatus. No congruence between Wolbachia and COI phylogenies was shown (Figure 5), demonstrating that Wolbachia mainly use horizontal transfers to spread in their hosts.
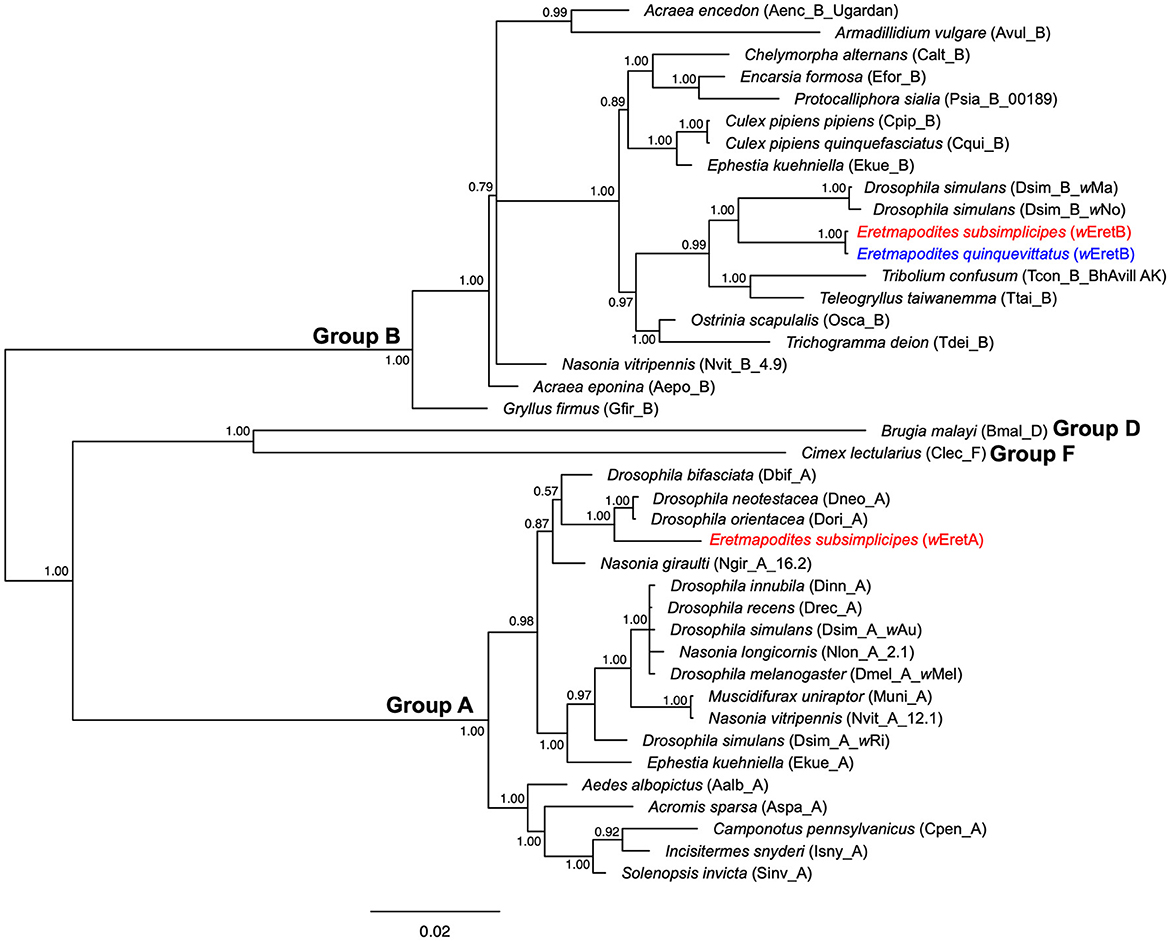
Figure 4. Bayesian phylogenic tree of Wolbachia strains obtained from concatenated data set of MLST genes (coxA, fbpA, ftsZ, gatB, and hcpA) (2,079 bp, 40 sequences). The phylogenetic tree was built using the substitution model: GTR+I+G. Sequences in red and blue correspond to Wolbachia sequences detected in Er. subsimplicipes and Er. quinquevittatus, respectively. The names of Wolbachia strains identified in these mosquito species (wEretA and wEretB) are indicated in brackets. The letters A, B, D, and F correspond to Wolbachia supergroups. The tree is midpoint unrooted and the numbers associated with nodes correspond to posterior probability values. The scale bar is in units of substitutions/site.
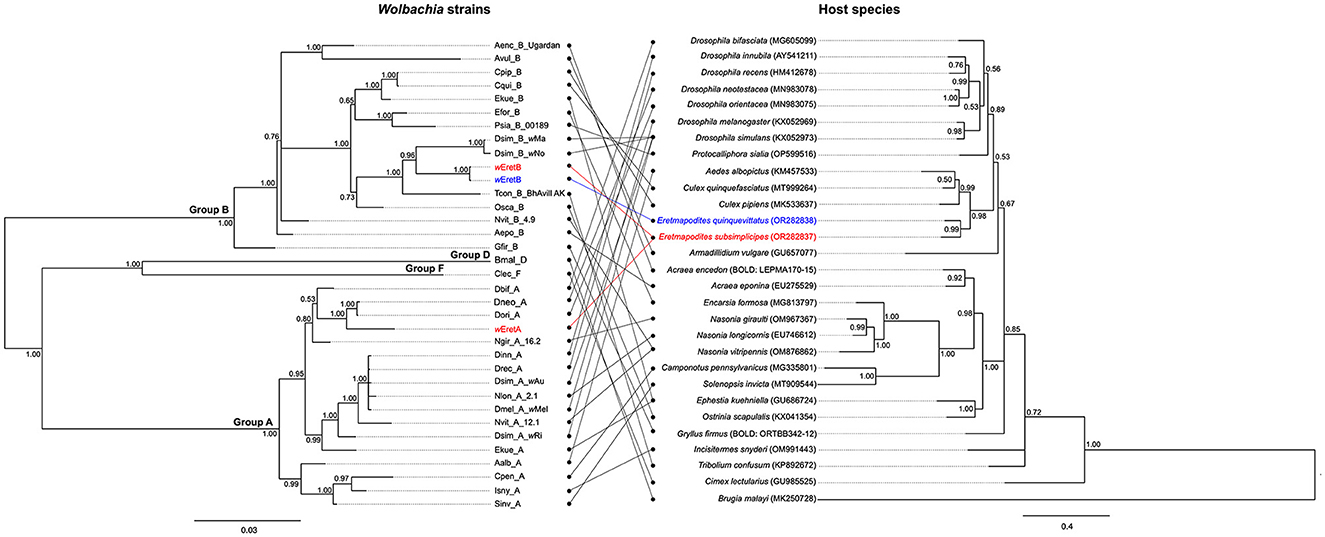
Figure 5. Comparisons between phylogeny of Wolbachia and phylogeny of arthropod hosts mitochondria (COI gene). The phylogeny of Wolbachia was constructed using concatenated sequences of the five MLST genes (coxA, fbpA, ftsZ, gatB, and hcpA) (2,079 bp, 35 sequences). The mitochondrial phylogeny was constructed using sequences of the COI gene (658 bp, 29 sequences). Only Wolbachia strains for which the COI sequences of the associated host species could be found in public database were used. The letters A, B, D and F correspond to Wolbachia supergroups. For COI phylogeny, the GenBank or BOLD accession numbers are indicated in brackets. The two phylogenies were built with Bayesian inference using the substitution model: GTR+I+G. The phylogenies were midpoint unrooted and the numbers associated with nodes correspond to posterior probability values. The scale bar is in units of substitutions/site.
Wolbachia is maternally inherited in Er. subsimplicipes
To assess maternal transmission of Wolbachia in Eretmapodites mosquitoes, we focused on the species Er. subsimplicipes as it is the only species for which we currently have a laboratory colony. We examined the progeny of 30 wEretA infected laboratory females (see above) based on the sequencing of the wsp gene. In general, the number of eggs per female ranging from 4 to 61 (mean number of 32 eggs per female) (Table 2). The hatching rate of the eggs ranged from 5% to 100%, with a mean rate of 74%. It seems that the number of adults produced by each female is limited, as the mean rates for eggs becoming larvae and larvae reaching the adult stage are only 15% and 26%, respectively (Table 2). A total of 131 offspring (74 males and 57 females) from the 30 investigated females were then screened for the presence of Wolbachia. Sixty per cent (N = 78/131) were found infected (Table 2), leading to a maternal transmission of Wolbachia ranging from 0% to 100%. Among the 30 females, four females did not transmit Wolbachia to their offspring, the transmission of Wolbachia was imperfect (between 6% and 88%) for ten females while perfect maternal transmission of Wolbachia (100%) was recorded for 16 females (Table 2).
Discussion
Using morphological and molecular methods, we confirmed the presence of two Eretmapodites species, Er. quinquevittatus and Er. subsimplicipes, in three islands of the Comoros archipelago (Grande Comore, Mohéli and Mayotte) (Le Goff et al., 2014; Boussès et al., 2018). The two species occurred in sympatry in the majority of investigated sites but Er. quinquevittatus was most commonly found in the three islands. The higher abundance of Er. quinquevittatus observed in this study may be due to sampling biases related to the type of samples collected and the method used for collection. In contrast to a previous study conducted between 2008 and 2012 in Mayotte, which found Er. subsimplicipes to be the most frequently encountered mosquito species on the island (Le Goff et al., 2014), our observations were based on adult mosquitoes. The difference between our findings and the previous study could be attributed to the fact that larvae were sampled in the study of Le Goff et al. (2014), whereas we focused on adult collection. It is possible that breeding sites of Er. quinquevittatus are less accessible compared to those of Er. subsimplicipes, which could result in sampling bias when working with adults that have the ability to fly far away from their breeding sites. However, it is also plausible that the distribution area of Er. quinquevittatus in Mayotte has increased over the last past 10 years. Additionally, the sampling method used in our study, which involved portable electric aspirators to collect resting adult mosquitoes in vegetation and flying adults around manipulators, may have better suited the collection of Er. quinquevittatus adults compared to Er. subsimplicipes. Since the biology and ecology of both species in the field are not well understood, it is possible that this methodological difference influenced our findings. It would be interesting in future investigations to compare the distribution area of both Eretmapodites species in Mayotte, but also in the other islands of the Comoros archipelago, using both larval and adult sampling.
The mtDNA polymorphism based on the COI gene revealed 13 and 35 haplotypes in Er. subsimplicipes and Er. quinquevittatus, respectively. In both species, we found unique haplotypes (i.e., encountered in only one island), suggesting different colonization events probably from Madagascar or the east coast of Africa, regions geographically close to the Comoros archipelago and where both Eretmapodites species have been also identified (Harbach, 2007; Tantely et al., 2016). Other mtDNA haplotypes were shared by different islands and could be the result of a single colonization event of Eretmapodites mosquitoes (from Madagascar or Africa) either to different islands, or to one island followed by a secondary dispersion event in a stepping stone mode. Such dispersion from a nearby island can be facilitated by frequent trade between the islands of the Comoros archipelago (Roger et al., 2014). For example, it is well known that the spread of the Asian tiger mosquito Ae. albopictus worldwide has been facilitated by the international trade of used tires (Reiter and Sprenger, 1987).
The mitochondrial haplotype diversity was higher in Er. quinquevittatus (35 haplotypes, Hd = 0.481 and π = 0.002, for N = 615 samples) than in Er. subsimplicipes (13 haplotypes, Hd = 0.338 and π = 0.001, for N = 205 samples). The difference between the two species can be explained by the sampling sizes as we found more Er. quinquevittatus specimens in our dataset. Alternatively, a higher mtDNA diversity in Er. quinquevittatus could result from a low prevalence of Wolbachia infection. Indeed, mitochondria and Wolbachia are in linkage disequilibrium, both cytoplasmic elements being linked through maternal cotransmission within egg cytoplasm's (Rasgon et al., 2006; Atyame et al., 2011; Dumas et al., 2013). Therefore, the spread of Wolbachia in host populations should result in an indirect selective sweep of the mtDNA leading to a reduction of mitochondrial diversity in Wolbachia infected host populations (Rasgon et al., 2006; Atyame et al., 2011; Dumas et al., 2013). In this study, we detected Wolbachia for the first time in both Er. quinquevittatus and Er. subsimplicipes, the lowest Wolbachia prevalence occurring in Er. quinquevittatus (0.8% vs. 87.7% in Er. subsimplicipes).
Wolbachia infection is not fixed in any of the Eretmapodites field populations, with Wolbachia-infected and uninfected specimens found within the same sampling sites. The presence of Wolbachia-infected and uninfected specimens is commonly observed in field populations of other arthropod species and can be associated with low phenotypic manipulation but also to imperfect maternal transmission (Werren et al., 2008). We have monitored maternal transmission of (the most frequent) wEretA using a laboratory colony of Er. subsimplicipes. It should be noted that it was challenging to rear Er. subsimplicipes species under insectary conditions and a reduced number of adult offspring was obtained for each female. Despite this challenge, our results show that maternal transmission of Wolbachia is imperfect or non-existent in some females, which could explain why Wolbachia infection is not fixed in Er. subsimplicipes field populations. COI sequencing data is also consistent with imperfect maternal transmission in Er. subsimplicipes since identical mtDNA haplotypes are shared by Wolbachia-infected and uninfected mosquitoes (Figure 2C).
The examined phylogenies of wsp and each of the five MLST genes showed that both Er. quinquevittatus and Er. subsimplicipes are infected with two Wolbachia supergroups A and B. Within each Wolbachia supergroup, the genetic diversity was low, only one allele being detected for almost all loci (except for hcpA). The concatenated phylogeny of the five MLST genes also confirmed the presence of two Wolbachia supergroups A and B strains (namely wEretA and wEretB, respectively) in our dataset. In Er. subsimplicipes, mosquitoes were infected with either wEretA or wEretB, although more higher infections by wEretA than wEretB were observed; while only wEretB was observed in Er. quinquevittatus. The presence of two divergent Wolbachia strains in Er. subsimplicipes can be explained by horizontal transfer events from other arhtropod species infected with genetically related Wolbachia such as Drosophila spp. which appeared to be infected with Wolbachia strains closely related to the strains wEretA and wEretB (Figure 4). Interestingly, the strain wEretB was shared by Er. subsimplicipes and Er. quinquevittatus. Several hypotheses can be proposed to explain this pattern. The wEretB strain might have been present in the common ancestor of both Eretmapodites species, and this Wolbachia strain was maintained in both species after their divergence, but the absence of nucleotide diversity between wEretB infecting both mosquito species does not support this assumption. For instance, some difference exists between the Wolbachia strains Dinn_A and Drec_A (within the supergroup A) infecting the genetically closely related Drosphila species D. innibula and D. recens (Figure 4). Another possibility would be horizontal transfers of Wolbachia between both mosquito species or from other host species. The widespread distribution of Wolbachia in arthropods is commonly associated with horizontal transfers occurring between closely related or genetically divergent host species (Heath et al., 1999; Ahmed et al., 2016; Tolley et al., 2019). These transfers would take place through mechanisms such as contamination, predation, or parasitism, particularly among species sharing the same ecological niches. Although evidence of horizontal transfers is rare, studies have shown that such transfers can occur in host-parasitoid associations (Huigens et al., 2004; Ahmed et al., 2015). The lack of congruence between Wolbachia and hosts phylogenies also support the possibility of horizontal transfers of Wolbachia between species (Tolley et al., 2019). In our study, we compared the phylogenies of concatenated MLST genes and COI, and no congruence was found (Figure 5), confirming the potential for horizontal transfers of Wolbachia between host species. Furthermore, the success of interspecific transfers of Wolbachia via embryonic microinjections (Sasaki and Ishikawa, 2000; McMeniman et al., 2009; Hughes and Rasgon, 2014) also supports the hypothesis of horizontal transfers of Wolbachia. Assuming a horizontal transfer of wEretB from Er. subsimplicipes to Er. quinquevittatus, the low prevalence of Wolbachia in Er. quinquevittatus could be explained either by a recent transfer of wEretB, or by differences in phenotypes induced by this Wolbachia strain when infecting each mosquito species. This change in phenotype expression of the same Wolbachia strain when infecting different host species has been previously described in Drosophila recens and Drosophila subquinaria (Jaenike, 2007). It would be interesting for future investigations to examine the phenotypes induced by the Wolbachia strains wEretA and wEretB in Er. subsimplicipes and Er. quinquevittatus to better understand the dynamics of these bacteria in the field. Lastly, a horizontal transfer of wEretB might have happened through introgression between both Eretmapodites species. Introgressions of Wolbachia have been observed in various subspecies of mosquitoes in the Culex pipiens complex, such as Culex pipiens pipiens and Culex pipiens quinquefasciatus, which hybridize in natural environments. This hybridization leads to subspecies sharing the same Wolbachia strains, as determined through the Wolbachia MLST genotyping method (Atyame et al., 2011; Dumas et al., 2013) (see also Figure 4). This hypothesis could be tested in the future by comparing the polymorphism in nuclear genomes of Er. subsimplicipes and Er. quinquevittatus mosquitoes.
Conclusion
In the present study, we characterized the mitochondrial genetic diversity of Er. quinquevittatus and Er. subsimplicipes occurring in sympatry in three islands of the Comoros archipelago. We also characterized the genetic diversity of Wolbachia infecting both mosquito species and identified two new Wolbachia strains, which have been named wEretA and wEretB. Experimental rearing of Er. subsimplicipes revealed imperfect maternal transmission of Wolbachia that might explain the infection patterns found in the field. Future studies will examine the phenotypes induced by these Wolbachia in Er. quinquevittatus and Er. subsimplicipes to better understand their dynamics in natura. As Eretmapodites mosquitoes are competent vectors for the transmission of arboviruses (Bamou et al., 2021; Cêtre-Sossah et al., 2023), future investigations should also consider the effects of wEretA and wEretB on vector competence.
Data availability statement
The datasets presented in this study can be found in online repositories. The names of the repository/repositories and accession number(s) can be found below: https://www.ncbi.nlm.nih.gov/genbank/, OR282837-OR282884, OR296528-OR296530, OR296531-OR296533, OR296534-OR296536, OR296537-OR296539, OR296540-OR296543, and OR296544-OR296547.
Ethics statement
The manuscript presents research on animals that do not require ethical approval for their study.
Author contributions
YG: Data curation, Formal analysis, Methodology, Writing—original draft, Writing—review & editing. SH: Conceptualization, Methodology, Writing—original draft, Writing—review & editing. CL: Investigation, Methodology, Writing—original draft. PR: Investigation, Resources, Writing—original draft. A-bI: Investigation, Resources, Writing—original draft. AY: Investigation, Resources, Writing—original draft. PB: Formal analysis, Methodology, Writing—original draft. PM: Conceptualization, Funding acquisition, Validation, Writing—review & editing. CA: Conceptualization, Investigation, Methodology, Supervision, Validation, Writing—original draft, Writing—review & editing.
Funding
The author(s) declare financial support was received for the research, authorship, and/or publication of this article. This work was supported by the European Regional Development Funds PO INTERREG trough the VECTOBIOMES project (number RE0009962) and the multi-years agreement of ARS-Mayotte/2022/n°75. SH was supported by a PhD degree scholarship from the Regional Council of Reunion Island (DIRED/20181182) and the University of Reunion Island.
Acknowledgments
We thank all collaborators from Union of Comoros for their help on the field and in the labs: Mohamed Salim Ben Said Hafi (Regional Director of Health, Anjouan), Djamaldine Mohamed Sambi (Regional Director of Health, Mohéli), Aboubacar Said Anli (Regional Director of Health, Moroni), Daanti Mouhtal Elhad and Idami Ousseni (PNLP, Anjouan), Yousra Ahamada Ali (PNLP, Mohéli), Youssouf Ismainla, and Amblat Ali Ahmed (PNLP, Moroni). We thank all collaborators from ARS Mayotte for their help on the field and in the labs: Ismael Nahouda, Mikidachi Said, Mourssalina Haraouna, Lassadi Hassani Ali, and Madi-Saindou Mouhamadi. We thank Louis Collet (Centre Hospitalier de Mayotte) for his help in the storage and the shipment of samples. We are thankful to Fiona Baudino (UMR PIMIT, Réunion) for her help in the lab. We are grateful to Pablo Tortosa (UMR PIMIT) for his proofreading and relevant remarks on the manuscript.
Conflict of interest
The authors declare that the research was conducted in the absence of any commercial or financial relationships that could be construed as a potential conflict of interest.
Publisher's note
All claims expressed in this article are solely those of the authors and do not necessarily represent those of their affiliated organizations, or those of the publisher, the editors and the reviewers. Any product that may be evaluated in this article, or claim that may be made by its manufacturer, is not guaranteed or endorsed by the publisher.
Supplementary material
The Supplementary Material for this article can be found online at: https://www.frontiersin.org/articles/10.3389/fmicb.2024.1343917/full#supplementary-material
References
Ahmed, M. Z., Breinholt, J. W., and Kawahara, A. Y. (2016). Evidence for common horizontal transmission of Wolbachia among butterflies and moths. BMC Evolut. Biol. 16:118. doi: 10.1186/s12862-016-0660-x
Ahmed, M. Z., Li, S.-J., Xue, X., Yin, X.-J., Ren, S.-X., Jiggins, F. M., et al. (2015). The intracellular bacterium Wolbachia uses parasitoid wasps as phoretic vectors for efficient horizontal transmission. PLoS Pathog. 10:e1004672. doi: 10.1371/journal.ppat.1004672
Aliota, M. T., Peinado, S. A., Velez, I. D., and Osorio, J. E. (2016). The wMel strain of Wolbachia reduces transmission of Zika virus by Aedes aegypti. Sci. Rep. 6:28792. doi: 10.1038/srep28792
Armbruster, P., Damsky, W. E. Jr., Giordano, R., Birungi, J., Munstermann, L. E., and Conn, J. E. (2003). Infection of new- and old-world Aedes albopictus (Diptera: Culicidae) by the intracellular parasite Wolbachia: implications for host mitochondrial DNA evolution. J. Med. Entomol. 40, 356–360. doi: 10.1603/0022-2585-40.3.356
Atyame, C. M., Delsuc, F., Pasteur, N., Weill, M., and Duron, O. (2011). Diversification of Wolbachia endosymbiont in the Culex pipiens mosquito. Molec. Biol. Evolut. 28, 2761–2772. doi: 10.1093/molbev/msr083
Atyame, C. M., Labbé, P., Dumas, E., Milesi, P., Charlat, S., Fort, P., et al. (2014). Wolbachia divergence and the evolution of cytoplasmic incompatibility in Culex pipiens. PLoS ONE 9:e87336. doi: 10.1371/journal.pone.0087336
Ayala, D., Akone-Ella, O., Rahola, N., Kengne, P., Ngangue, M. F., Mezeme, F., et al. (2019). Natural Wolbachia infections are common in the major malaria vectors in Central Africa. Evolut. Applic. 12, 1583–1594. doi: 10.1111/eva.12804
Baldini, F., Segata, N., Pompon, J., Marcenac, P., Robert Shaw, W., Dabiré, R. K., et al. (2014). Evidence of natural Wolbachia infections in field populations of Anopheles gambiae. Natu. Commun. 5:3985. doi: 10.1038/ncomms4985
Baldo, L., Dunning Hotopp, J. C., Jolley, K. A., Bordenstein, S. R., Biber, S. A., Choudhury, R. R., et al. (2006). Multilocus sequence typing system for the endosymbiont Wolbachia pipientis. Appl. Environ. Microbiol. 72, 7098–7110. doi: 10.1128/AEM.00731-06
Bamou, R., Mayi, M. P. A., Djiappi-Tchamen, B., Nana-Ndjangwo, S. M., Nchoutpouen, E., Cornel, A. J., et al. (2021). An update on the mosquito fauna and mosquito-borne diseases distribution in Cameroon. Paras. Vectors 14:527. doi: 10.1186/s13071-021-04950-9
Bauer, J. H. (1928). The transmission of yellow fever by mosquitoes other than Aedes aegypti. J. Am. Med. Assoc. 90, 2091–2092. doi: 10.1001/jama.1928.02690530019007
Bian, G., Joshi, D., Dong, Y., Lu, P., Zhou, G., Pan, X., et al. (2013). Wolbachia Invades Anopheles stephensi populations and induces refractoriness to Plasmodium infection. Science 340, 748–751. doi: 10.1126/science.1236192
Bian, G., Xu, Y., Lu, P., Xie, Y., and Xi, Z. (2010). The endosymbiotic bacterium Wolbachia induces resistance to dengue virus in Aedes aegypti. PLOS Pathog. 6:e1000833. doi: 10.1371/journal.ppat.1000833
Bordenstein, S. R., Paraskevopoulos, C., Dunning Hotopp, J. C., Sapountzis, P., Lo, N., Bandi, C., et al. (2009). Parasitism and mutualism in Wolbachia: what the phylogenomic trees can and cannot say. Molec. Biol. Evol. 26, 231–241. doi: 10.1093/molbev/msn243
Bordenstein, S. R., and Wernegreen, J. J. (2004). Bacteriophage flux in endosymbionts (Wolbachia): infection frequency, lateral transfer, and recombination rates. Molec. Biol. Evolut. 21, 1981–1991. doi: 10.1093/molbev/msh211
Bourtzis, K., Dobson, S. L., Xi, Z., Rasgon, J. L., Calvitti, M., Moreira, L. A., et al. (2014). Harnessing mosquito–Wolbachia symbiosis for vector and disease control. Acta Tropica 132, S150–S163. doi: 10.1016/j.actatropica.2013.11.004
Boussès, P., Le Goff, G., and Robert, V. (2018). Inventaire des moustiques (Diptera : Culicidae) des îles du sud-ouest de l'océan Indien, Madagascar excepté — Une revue critique. Ann. Soc. Entomol. France 54, 89–110. doi: 10.1080/00379271.2018.1429951
Braig, H. R., Zhou, W., Dobson, S. L., and O'Neill, S. L. (1998). Cloning and characterization of a gene encoding the major surface protein of the bacterial endosymbiont Wolbachia pipientis. J. Bacteriol. 180, 2373–2378. doi: 10.1128/JB.180.9.2373-2378.1998
Cêtre-Sossah, C., Lebon, C., Rabarison, P., Cardinale, E., Mavingui, P., and Atyame, C. (2023). Evidence of Eretmapodites subsimplicipes and Aedes albopictus as competent vectors for Rift Valley fever virus transmission in Mayotte. Acta Tropica 239:106835. doi: 10.1016/j.actatropica.2023.106835
Coon, K. L., Brown, M. R., and Strand, M. R. (2016). Mosquitoes host communities of bacteria that are essential for development but vary greatly between local habitats. Molec. Ecol. 25, 5806–5826. doi: 10.1111/mec.13877
Darriba, D., Taboada, G. L., Doallo, R., and Posada, D. (2012). jModelTest 2: more models, new heuristics and parallel computing. Nat. Methods 9, 772–772. doi: 10.1038/nmeth.2109
Dodson, B. L., Hughes, G. L., Paul, O., Matacchiero, A. C., Kramer, L. D., and Rasgon, J. L. (2014). Wolbachia enhances West Nile Virus (WNV) infection in the mosquito Culex tarsalis. PLoS Negl. Trop. Dis. 8:e2965. doi: 10.1371/journal.pntd.0002965
Douglas, A. E. (1998). Nutritional interactions in insect-microbial symbioses: aphids and their symbiotic bacteria Buchnera. Ann. Rev. Entomol. 43, 17–37. doi: 10.1146/annurev.ento.43.1.17
Dumas, E., Atyame, C. M., Milesi, P., Fonseca, D. M., Shaikevich, E. V., Unal, S., et al. (2013). Population structure of Wolbachia and cytoplasmic introgression in a complex of mosquito species. BMC Evolut. Biol. 13:181. doi: 10.1186/1471-2148-13-181
Duron, O., Bouchon, D., Boutin, S., Bellamy, L., Zhou, L., Engelstädter, J., et al. (2008). The diversity of reproductive parasites among arthropods: Wolbachia do not walk alone. BMC Biol. 6:27. doi: 10.1186/1741-7007-6-27
Duron, O., Fort, P., and Weill, M. (2005). Hypervariable prophage WO sequences describe an unexpected high number of Wolbachia variants in the mosquito Culex pipiens. Proc. R. Soc. B. 273, 495–502. doi: 10.1098/rspb.2005.3336
Dutra, H. L. C., Rocha, M. N., Dias, F. B. S., Mansur, S. B., Caragata, E. P., and Moreira, L. A. (2016). Wolbachia blocks currently circulating Zika Virus isolates in Brazilian Aedes aegypti mosquitoes. Cell Host Micr. 19, 771–774. doi: 10.1016/j.chom.2016.04.021
Edwards, F. W. (1941). Mosquitoes of the Ethiopian Region. III.-Culicine aults and pupae. Available online at: https://www.cabdirect.org/cabdirect/abstract/19411000211 (accessed November 2, 2023).
Folmer, O., Black, M., Hoeh, W., Lutz, R., and Vrijenhoek, R. (1994). DNA primers for amplification of mitochondrial cytochrome coxidase subunit-I from diverse metazoan invertebrates. Molec. Marine Biol. Biotechnol. 15, 294–299.
Glowska, E., Dragun-Damian, A., Dabert, M., and Gerth, M. (2015). New Wolbachia supergroups detected in quill mites (Acari: Syringophilidae). Infect. Genet. Evolut. 30, 140–146. doi: 10.1016/j.meegid.2014.12.019
Harbach, R. E. (2007). The Culicidae (Diptera): a review of taxonomy, classification and phylogeny. Zootaxa 1668, 591–638. doi: 10.11646/zootaxa.1668.1.28
Harbach, R. E. (2013). Mosquito taxonomic inventory. Available online at: https://mosquito-taxonomic-inventory.myspecies.info/how-reference-site (accessed November 3, 2023).
Heath, B. D., Butcher, R. D. J., Whitfield, W. G. F., and Hubbard, S. F. (1999). Horizontal transfer of Wolbachia between phylogenetically distant insect species by a naturally occurring mechanism. Curr. Biol. 9, 313–316. doi: 10.1016/S0960-9822(99)80139-0
Hedges, L. M., Brownlie, J. C., O'Neill, S. L., and Johnson, K. N. (2008). Wolbachia and virus protection in insects. Science 322, 702–702. doi: 10.1126/science.1162418
Hilgenboecker, K., Hammerstein, P., Schlattmann, P., Telschow, A., and Werren, J. H. (2008). How many species are infected with Wolbachia? – a statistical analysis of current data. FEMS Microbiol. Lett. 281, 215–220. doi: 10.1111/j.1574-6968.2008.01110.x
Hoffmann, A. A., Montgomery, B. L., Popovici, J., Iturbe-Ormaetxe, I., Johnson, P. H., Muzzi, F., et al. (2011). Successful establishment of Wolbachia in Aedes populations to suppress dengue transmission. Nature 476, 454–457. doi: 10.1038/nature10356
Hopkins, G. H. E. (1952). Mosquitoes of the Ethiopian Region. I. Larval bionomics of mosquitoes and taxonomy of Culicine larvae. Available online at: https://www.cabdirect.org/cabdirect/abstract/19532901687 (accessed November 2, 2023).
Hughes, G. L., and Rasgon, J. L. (2014). Transinfection: a method to investigate Wolbachia-host interactions and control arthropod-borne disease. Insect Molec. Biol. 23, 141–151. doi: 10.1111/imb.12066
Hughes, G. L., Vega-Rodriguez, J., Xue, P., and Rasgon, J. L. (2012). Wolbachia strain wAlbB enhances infection by the rodent malaria parasite Plasmodium berghei in Anopheles gambiae mosquitoes. Appl. Environ. Microbiol. 78, 1491–1495. doi: 10.1128/AEM.06751-11
Huigens, M. E., de Almeida, R. P., Boons, P.a,. H, Luck, R. F., and Stouthamer, R. (2004). Natural interspecific and intraspecific horizontal transfer of parthenogenesis-inducing Wolbachia in Trichogramma wasps. Proc. R. Soc. B. 271, 509–515. doi: 10.1098/rspb.2003.2640
Jaenike, J. (2007). Spontaneous emergence of a new Wolbachia phenotype. Evolution 61, 2244–2252. doi: 10.1111/j.1558-5646.2007.00180.x
Jaenike, J., Unckless, R., Cockburn, S. N., Boelio, L. M., and Perlman, S. J. (2010). Adaptation via symbiosis: recent spread of a Drosophila defensive symbiont. Science 329, 212–215. doi: 10.1126/science.1188235
Jiggins, F. M., Schulenburg, J. H. G., von der, Hurst, G. D. D., and Majerus, M. E. N. (2001). Recombination confounds interpretations of Wolbachia evolution. Proc. R. Soc. B. 268, 1423–1427. doi: 10.1098/rspb.2001.1656
Jolley, K. A., Bray, J. E., and Maiden, M. C. J. (2018). Open-access bacterial population genomics: BIGSdb software, the PubMLST.org website and their applications. Wellcome Open Res. 3:124. doi: 10.12688/wellcomeopenres.14826.1
Kambhampati, S., Rai, K. S., and Burgun, S. J. (1993). Unidirectional cytoplasmic incompatibility in the mosquito Aedes albopictus. Evolution 47, 673–677. doi: 10.2307/2410079
Kearse, M., Moir, R., Wilson, A., Stones-Havas, S., Cheung, M., Sturrock, S., et al. (2012). Geneious Basic: an integrated and extendable desktop software platform for the organization and analysis of sequence data. Bioinformatics 28, 1647–1649. doi: 10.1093/bioinformatics/bts199
Laven, H. (1951). Crossing experiments with Culex strains. Evolution 5, 370–375. doi: 10.2307/2405682
Le Goff, G., Goodman, S. M., Elguero, E., and Robert, V. (2014). Survey of the mosquitoes (Diptera: Culicidae) of Mayotte. PLoS ONE 9:e100696. doi: 10.1371/journal.pone.0100696
McIntosh, B. M., Jupp, P.G., Dos Santos, I., and Barnard, B.J.H. (1980). Vector studies on Rift Valley Fever Virus in South Africa. South African Med. J. 58, 127–132.
McMeniman, C. J., Lane, R. V., Cass, B. N., Fong, A. W. C., Sidhu, M., Wang, Y.-F., et al. (2009). Stable introduction of a life-shortening Wolbachia infection into the mosquito Aedes aegypti. Science 323, 141–144. doi: 10.1126/science.1165326
Moreira, L. A., Iturbe-Ormaetxe, I., Jeffery, J. A., Lu, G., Pyke, A. T., Hedges, L. M., et al. (2009). A Wolbachia symbiont in Aedes aegypti limits infection with Dengue, Chikungunya, and Plasmodium. Cell 139, 1268–1278. doi: 10.1016/j.cell.2009.11.042
Musa, A. A., Muturi, M. W., Musyoki, A. M., Ouso, D. O., Oundo, J. W., Makhulu, E. E., et al. (2020). Arboviruses and blood meal sources in zoophilic mosquitoes at human-wildlife interfaces in Kenya. Vector-Borne Zoonotic Dis. 20, 444–453. doi: 10.1089/vbz.2019.2563
Oliver, K. M., Russell, J. A., Moran, N. A., and Hunter, M. S. (2003). Facultative bacterial symbionts in aphids confer resistance to parasitic wasps. Proc. Natl. Acad. Sci. 100, 1803–1807. doi: 10.1073/pnas.0335320100
Osuna, A. M., Gidley, A., Mayi, M. P. A., Bamou, R., Dhokiya, V., Antonio-Nkondjio, C., et al. (2023). Diverse novel Wolbachia bacteria strains and genera-specific co-infections with Asaia bacteria in Culicine mosquitoes from ecologically diverse regions of Cameroon. Wellcome Open Res. 8:267. doi: 10.12688/wellcomeopenres.18580.2
Paraskevopoulos, C., Bordenstein, S. R., Wernegreen, J. J., Werren, J. H., and Bourtzis, K. (2006). Toward a Wolbachia multilocus sequence typing system: discrimination of Wolbachia strains present in Drosophila species. Curr.Microbiol. 53, 388–395. doi: 10.1007/s00284-006-0054-1
Rasgon, J. L., Cornel, A. J., and Scott, T. W. (2006). Evolutionary history of a mosquito endosymbiont revealed through mitochondrial hitchhiking. Proc. R. Soc. B. 273, 1603–1611. doi: 10.1098/rspb.2006.3493
Reiter, P., and Sprenger, D. (1987). The used tire trade: a mechanism for the worldwide dispersal of container breeding mosquitoes. J. Am. Mosquito Control Assoc. 3, 494–501.
Roger, M., Beral, M., Licciardi, S., Soulé, M., Faharoudine, A., Foray, C., et al. (2014). Evidence for circulation of the rift valley fever virus among livestock in the union of comoros. PLoS Negl. Trop. Dis. 8:e3045. doi: 10.1371/journal.pntd.0003045
Ronquist, F., Teslenko, M., van der Mark, P., Ayres, D. L., Darling, A., Höhna, S., et al. (2012). MrBayes 3.2: efficient bayesian phylogenetic inference and model choice across a large model space. System. Biol. 61, 539–542. doi: 10.1093/sysbio/sys029
Ros, V. I. D., Fleming, V. M., Feil, E. J., and Breeuwer, J. A. J. (2009). How diverse is the genus Wolbachia? Multiple-gene sequencing reveals a putatively new Wolbachia supergroup recovered from spider mites (Acari: Tetranychidae). Appl. Environ. Microbiol. 75, 1036–1043. doi: 10.1128/AEM.01109-08
Rozas, J., Ferrer-Mata, A., Sánchez-DelBarrio, J. C., Guirao-Rico, S., Librado, P., Ramos-Onsins, S. E., et al. (2017). DnaSP 6: DNA sequence polymorphism analysis of large data sets. Molec. Biol. Evol. 34, 3299–3302. doi: 10.1093/molbev/msx248
Sasaki, T., and Ishikawa, H. (2000). Transinfection of Wolbachia in the mediterranean flour moth, Ephestia kuehniella, by embryonic microinjection. Heredity 85, 130–135. doi: 10.1046/j.1365-2540.2000.00734.x
Scarborough, C. L., Ferrari, J., and Godfray, H. C. J. (2005). Aphid protected from pathogen by endosymbiont. Science 310, 1781–1781. doi: 10.1126/science.1120180
Service, M. W. (1990). Handbook to the Afrotropical toxorhynchitine and culicine mosquitoes, excepting Aedes and Culex. London: British Museum (Natural History) 1–207.
Shropshire, J. D., Leigh, B., and Bordenstein, S. R. (2020). Symbiont-mediated cytoplasmic incompatibility: what have we learned in 50 years? eLife 9:e61989. doi: 10.7554/eLife.61989
Sicard, M., Bonneau, M., and Weill, M. (2019). Wolbachia prevalence, diversity, and ability to induce cytoplasmic incompatibility in mosquitoes. Curr. Opin. Insect Sci. 34, 12–20. doi: 10.1016/j.cois.2019.02.005
Tantely, M. L., Goff, G. L., Boyer, S., and Fontenille, D. (2016). An updated checklist of mosquito species (Diptera: Culicidae) from Madagascar. Parasite 23:20. doi: 10.1051/parasite/2016018
Teixeira, L., Ferreira, Á., and Ashburner, M. (2008). The bacterial symbiont Wolbachia induces resistance to RNA viral infections in Drosophila melanogaster. PLoS Biol. 6:e1000002. doi: 10.1371/journal.pbio.1000002
Thongsripong, P., Chandler, J. A., Green, A. B., Kittayapong, P., Wilcox, B. A., Kapan, D. D., et al. (2018). Mosquito vector-associated microbiota: metabarcoding bacteria and eukaryotic symbionts across habitat types in Thailand endemic for dengue and other arthropod-borne diseases. Ecol. Evolut. 8, 1352–1368. doi: 10.1002/ece3.3676
Tokash-Peters, A. G., Niyonzima, J. D., Kayirangwa, M., Muhayimana, S., Tokash, I. W., Jabon, J. D., et al. (2022). Mosquito microbiomes of Rwanda: characterizing mosquito Host and microbial communities in the land of a thousand hills. bioRxiv 2022.08.03.502589. doi: 10.1101/2022.08.03.502589
Tolley, S. J. A., Nonacs, P., and Sapountzis, P. (2019). Wolbachia horizontal transmission events in ants: what do we know and what can we learn? Front. Microbiol. 10:296. doi: 10.3389/fmicb.2019.00296
Tortosa, P., Charlat, S., Labbé, P., Dehecq, J.-S., Barré, H., and Weill, M. (2010). Wolbachia age-sex-specific density in Aedes albopictus: a host evolutionary response to cytoplasmic incompatibility? PLoS ONE 5:e9700. doi: 10.1371/journal.pone.0009700
Tsuchida, T., Koga, R., Horikawa, M., Tsunoda, T., Maoka, T., Matsumoto, S., et al. (2010). Symbiotic bacterium modifies aphid body color. Science 330, 1102–1104. doi: 10.1126/science.1195463
Turelli, M., Katznelson, A., and Ginsberg, P. S. (2022). Why Wolbachia-induced cytoplasmic incompatibility is so common. Proc. Natl. Acad. Sci. 119:e2211637119. doi: 10.1073/pnas.2211637119
Walker, T., Johnson, P. H., Moreira, L. A., Iturbe-Ormaetxe, I., Frentiu, F. D., McMeniman, C. J., et al. (2011). The wMel Wolbachia strain blocks dengue and invades caged Aedes aegypti populations. Nature 476, 450–453. doi: 10.1038/nature10355
Weinert, L. A., Araujo-Jnr, E. V., Ahmed, M. Z., and Welch, J. J. (2015). The incidence of bacterial endosymbionts in terrestrial arthropods. Proc. R. Soc. B. 282:20150249. doi: 10.1098/rspb.2015.0249
Werren, J. H., Baldo, L., and Clark, M. E. (2008). Wolbachia: master manipulators of invertebrate biology. Nat. Rev. Microbiol. 6, 741–751. doi: 10.1038/nrmicro1969
Zélé, F., Nicot, A., Berthomieu, A., Weill, M., Duron, O., and Rivero, A. (2014). Wolbachia increases susceptibility to Plasmodium infection in a natural system. Proc. R. Soc. B. 281:20132837. doi: 10.1098/rspb.2013.2837
Zhou, W., Rousset, F., and O'Neil, S. (1998). Phylogeny and PCR-based classification of Wolbachia strains using wsp gene sequences. Proc. R. Soc. B. 265, 509–515. doi: 10.1098/rspb.1998.0324
Zouache, K., Raharimalala, F. N., Raquin, V., Tran-Van, V., Raveloson, L. H. R., Ravelonandro, P., et al. (2011). Bacterial diversity of field-caught mosquitoes, Aedes albopictus and Aedes aegypti, from different geographic regions of Madagascar. FEMS Microbiol. Ecol. 75, 377–389. doi: 10.1111/j.1574-6941.2010.01012.x
Keywords: Wolbachia, Eretmapodites quinquevittatus, Eretmapodites subsimplicipes, mitochondrial genetic diversity, Comoros archipelago
Citation: Gomard Y, Hafsia S, Lebon C, Rabarison P, Idaroussi A-b, Yssouf A, Boussès P, Mavingui P and Atyame C (2024) Genetic diversity of endosymbiotic bacteria Wolbachia infecting two mosquito species of the genus Eretmapodites occurring in sympatry in the Comoros archipelago. Front. Microbiol. 15:1343917. doi: 10.3389/fmicb.2024.1343917
Received: 24 November 2023; Accepted: 11 March 2024;
Published: 27 March 2024.
Edited by:
Sampath Kumar, Tata Institute for Genetics and Society, IndiaReviewed by:
Arunachalam Ramaiah, Georgia Department of Public Health, United StatesMarina S. Ascunce, Agricultural Research Service (USDA), United States
Copyright © 2024 Gomard, Hafsia, Lebon, Rabarison, Idaroussi, Yssouf, Boussès, Mavingui and Atyame. This is an open-access article distributed under the terms of the Creative Commons Attribution License (CC BY). The use, distribution or reproduction in other forums is permitted, provided the original author(s) and the copyright owner(s) are credited and that the original publication in this journal is cited, in accordance with accepted academic practice. No use, distribution or reproduction is permitted which does not comply with these terms.
*Correspondence: Célestine Atyame, Y2VsZXN0aW5lLmF0eWFtZS1udGVuJiN4MDAwNDA7dW5pdi1yZXVuaW9uLmZy; Patrick Mavingui, UGF0cmljay5NQVZJTkdVSSYjeDAwMDQwO2NucnMuZnI=
†These authors have contributed equally to this work and share first authorship