- 1Key Laboratory of Chinese Herbal Medicine Biology and Cultivation, Ministry of Agriculture and Rural Affairs, Institute of Chinese Herbal Medicine, Hubei Academy of Agricultural Science, Enshi, China
- 2Hubei Engineering Research Center of Good Agricultural Practices (GAP) Production for Chinese Herbal Medicines, Institute of Chinese Herbal Medicines, Hubei Academy of Agricultural Sciences, Enshi, China
Introduction: Southern blight, caused by Sclerotium rolfsii, poses a serious threat to the cultivation of Coptis chinensis, a plant with significant medicinal value. The overreliance on fungicides for controlling this pathogen has led to environmental concerns and resistance issues. There is an urgent need for alternative, sustainable disease management strategies.
Methods: In this study, Bacillus velezensis LT1 was isolated from the rhizosphere soil of diseased C. chinensis plants. Its biocontrol efficacy against S. rolfsii LC1 was evaluated through a confrontation assay. The antimicrobial lipopeptides in the fermentation liquid of B. velezensis LT1 were identified using Matrix-Assisted Laser Desorption/Ionization Time-of-Flight Mass Spectrometry (MALDI-TOF-MS). The effects of B. velezensis LT1 on the mycelial morphology of S. rolfsii LC1 were examined using scanning electron microscopy (SEM) and transmission electron microscopy (TEM).
Results: The confrontation assay indicated that B. velezensis LT1 significantly inhibited the growth of S. rolfsii LC1, with an inhibition efficiency of 78.41%. MALDI-TOF-MS analysis detected the presence of bacillomycin, surfactin, iturin, and fengycin in the fermentation liquid, all known for their antifungal properties. SEM and TEM observations revealed that the mycelial and cellular structures of S. rolfsii LC1 were markedly distorted when exposed to B. velezensis LT1.
Discussion: The findings demonstrate that B. velezensis LT1 has considerable potential as a biocontrol agent against S. rolfsii LC1. The identified lipopeptides likely contribute to the antifungal activity, and the morphological damage to S. rolfsii LC1 suggests a mechanism of action. This study underscores the importance of exploring microbial biocontrol agents as a sustainable alternative to chemical fungicides in the management of plant diseases. Further research into the genetic and functional aspects of B. velezensis LT1 could provide deeper insights into its biocontrol mechanisms and facilitate its application in agriculture.
1 Introduction
The rhizome of the perennial herb Coptis chinensis, also known as goldthread in Chinese medicine, has been commonly used alone or in combination with other herbs in many traditional Chinese medicine formulations (Wang et al., 2019). Due to its extensive antibacterial, antiviral, and antioxidant effects, it is often used to treat inflammatory diseases, diabetes, cardiovascular diseases, cancer, and neurodegenerative diseases (Zhen et al., 2011; Chou et al., 2017). Southern blight caused by Sclerotium rolfsii is one of the most serious diseases affecting C. chinensis, with a field incidence of approximately 10–20% and severe cases of up to 80%. Furthermore, southern blight infections have a considerable impact on the yield and quality of the herb and can even result in no harvest. Currently, the control of southern blight on C. chinensis mainly relies on the use of chemical fungicides. Only a few registered drugs, such as thifuramide and fluoxafen, are available for the prevention of southern blight (China Pesticide Information Network, http://www.chinapesticide.gov.cn). Excessive use of a single fungicide can lead to the rapid development of drug resistance, resulting in a decrease in the prevention and control effect (Mikaberidze et al., 2017). Furthermore, the excessive use of chemical agents can have an immeasurable impact on the quality of C. chinensis. Therefore, it is urgent to find a new green and efficient control method for southern blight on C. chinensis.
As public demand for eco-friendly and healthy food options surges, coupled with heightened environmental consciousness, biological control strategies are garnering increasing interest for their sustainable and eco-friendly attributes. Among a variety of pathogens, microbial antagonists have emerged as a focal point among a diverse array of pathogens due to their recognized safety, effectiveness, and environmentally benign characteristics (Li et al., 2022). These antagonists are emerging as trustworthy substitutes for traditional chemical agents. Over the past several decades, research has highlighted the pivotal role that microorganisms play in the plant rhizosphere soil in combatting plant pathogens, marking a critical advancement in agricultural sciences (Cakmakci et al., 2006; Mehta et al., 2014; Manuel et al., 2023). Plant growth-promoting rhizobacteria (PGPR) are beneficial bacteria found in the plant rhizosphere. They are capable of colonizing the root surface and interior, enhancing plant growth and suppressing disease occurrence (Oleńska et al., 2020). PGPR facilitate plant growth through a variety of mechanisms, such as the production of iron carriers, increased phosphorolysis, improved nutrient absorption, and the secretion of plant hormones (Backer et al., 2018). Moreover, many PGPRs can prevent the recurrence of diseases by inducing systemic resistance (ISR), engaging in interspecific competition, and emitting volatile organic compounds (VOCs) (Li et al., 2020). Soil-beneficial microorganisms are important sources of PGPRs, such as Bacillus, Streptomyces, Pseudomonas, Burkholderia, and Trichoderma, and all of them are isolated from rhizosphere soil, being widely used in disease control and soil improvement in agricultural production (Le et al., 2022; Liu et al., 2022; Venturi, 2022; Xue et al., 2022; Baard et al., 2023). Screening beneficial microorganisms from soil to combat pathogens is also a crucial strategy in plant disease management.
Bacillus velezensis was first identified by Ruiz-García et al. in 2005 and has been shown to produce multiple compounds that can antagonize plant pathogens (Ruiz-García et al., 2005). B. velezensis has been found to produce numerous active substances, such as surfactins, fengycins, bacillomycin-D, bacillaene, macrolactin, difficidin, bacillibactin, and bacilysin, which are involved in inhibiting the growth of plant pathogens (Gao et al., 2017; Rabbee et al., 2019). To date, several strains of B. velezensis have been reported to have the potential to be used for controlling plant diseases and promoting plant growth (Zhang et al., 2019; Kim et al., 2022; Sun et al., 2022). In this study, we aimed to identify rhizosphere growth-promoting bacteria that could inhibit southern blight of C. chinensis in rhizosphere soil. To achieve green prevention and control of southern blight on C. chinensis, we conducted the following work to screen for satisfactory biocontrol bacteria: (1) isolated and identified the bacterial community in the rhizosphere soil of C. chinensis and verified its inhibitory effect on S. rolfsii, (2) clarified the biological characteristics and control effect of B. velezensis LT1 against southern blight, and (3) clarified the types of lipopeptide antibiotics produced by B. velezensis LT1.
2 Materials and methods
2.1 Fungal and bacterial strains
All fungal and bacterial strains used in this study were isolated and preserved by the Institute of Chinese Herbal Medicines, Hubei Academy of Agricultural Sciences. The main strains, B. velezensis LT1 and S. rolfsii LC1, were stored in the China Center for Type Culture Collection Center with the numbers of CCTCC M 2020023 and CCTCC M 20231389, respectively.
2.2 Sample collection
Rhizosphere soil samples were collected from the C. chinensis GAP planting base (108°35′E, 30°22′N) located in Lichuan City, Enshi Prefecture, Hubei Province, in October 2019. The planting base had a history of perennial southern blight occurrence. The rhizosphere soil, which is located approximately 5–10 cm below the ground part of the C. chinensis plant, was collected using a JC-802 root soil sampler (Qingdao Juchuang Times Environmental Protection Technology Co., LTD, Qingdao, China). The rhizome samples obtained from five locations in the rhizosphere soil were uniformly mixed to form one sample.
2.3 Isolation and identification of bacterial strains
Bacteria in the rhizosphere soil of C. chinensis were isolated using the dilution coating method, as described by Li et al. (2023). The soil samples were prepared into suspensions with concentrations of approximately 10−1–10−6, and 50 μL of the soil dilutions was uniformly coated on the surface of NA medium, R2A medium, and brain-heart infusion liquid medium. The NA medium contained 10 g of tryptone, 3 g of beef powder, 5 g of NaCl, and 15 g of agar per liter. The R2A medium contained 0.5 g of yeast extract powder, 0.5 g tryptone, 0.5 g of casein hydrolysate, 0.5 g of glucose, 0.5 g soluble starch, 0.3 g of KH2PO4, 0.024 g of MgSO4, 0.3 g of CH3COCOONa, and 15 g of agar per liter. The brain-heart infusion liquid medium contained 5 g of tryptone, g of glucose, 5 g of NaCl, 2.5 g of Na2HPO4, 4 g of beef brain infusion, 16 g of casein peptone, and 15 g of agar per liter. The Petri dishes were then sealed and incubated at a constant temperature of 28°C. Colonies with distinct differences in morphology, size, and color were selected for purification. The colonies were purified three to four times using the scribing plate method until single colonies were observed. Single colonies were transferred to a bead-side culture medium test tube and stored in the refrigerator at 4°C for future use. Frozen stocks of all the purified colonies were prepared using 25% glycerol and stored at −80°C.
Bacterial genomic DNA was extracted using a Soil FastDNA SPIN Kit (MP Biomedicals (Shanghai) Co., Ltd., Shanghai, China). After assessing the quality of the genomic DNA extraction using a microspectrophotometer (NanoDrop One C, Thermo Fisher Scientific, Madison, United States), 16S rDNA was amplified using the primers 24F (5′-GTTTGATCCTGGCTCAG-3′) and 1494R (5′-ACGGCTACCTTGTTACGACTT-3′). The PCR products were purified and recovered by Wuhan Tsingke Biology Co., Ltd. for sequencing. The sequencing results were compared with the BLAST® sequence analysis tool at the US National Center for Biotechnology Information (NCBI) for preliminary identification of the isolated strains.
2.4 Screening of antagonistic strains
The plate confrontation method (Yue et al., 2023) was used to screen for antagonistic strains against S. rolfsii in C. chinensis. The activated S. rolfsii LC1 was inoculated with a mycelial plug using a hole punch of 5 mm diameter and placed in the center of the PDA medium with the mycelial side facing down. The isolated and purified bacteria were inoculated 2.5 cm away from the mycelial plug and cultured at 28°C for 4–7 days. The control group was not inoculated with the isolated strains, and each experiment was repeated three times. The colony diameter was measured using the crisscross method, and the inhibition rate was calculated using the following formula:
2.5 Morphological, biological characteristics, and sequence analysis of antagonistic bacteria LT1
The LT1 strain exhibited the strongest inhibitory effect on S. rolfsii LC1. Therefore, only the LT1 strain was subjected to morphological and molecular analyses. Morphological characteristics of the LT1 strain were observed using microscopy LSM 900 (Carl Zeiss Co. Ltd., Chengdu, China) and scanning electron microscopy (HITACHI Regulus 8,100) (Hitachi Production Co., LTD., Tokyo, Japan). Subsequently, the LT1 strain underwent Gram staining using the Gram Stain Kit HB8278 (Haibo Biotechnology Co. LTD., Qingdao, China). The gyrA gene was amplified using the primers gyrA-F (5’-CAGTCAGGAAATGCGTACGTCCTT-3′) and gyrA-R (5’-CAAGGTAATGCTCCAGGCATTGCT-3′). The PCR products were subjected to 1% (w/v) agarose gel electrophoresis, purified, and sequenced by Wuhan Aoke Biotechnology Co., Ltd. The sequencing results were compared using the BLAST® sequence analysis tool in NCBI1 and nucleic acid homology comparison with the data in GenBank. When constructing a phylogenetic tree using 16S rDNA and gyrA genes, bacteria with high homology to Bacillus were selected as ingroups and other bacteria were selected as outgroups. Clustal X was used to compare the sequences, and MEGA5.0 software (Mega Technology Co. LTD., Suzhou, China) was used to analyze the phylogeny of all bacteria. The two genes were combined to construct a phylogenetic tree, and the bootstrap value was tested 1,000 times. Finally, the optimum growth temperature and pH for the LT1 strain were determined.
2.6 Antagonistic activity of LT1 strain fermentation liquor
To obtain the fermentation liquor, 1 mL of the LT1 strain was inoculated into 1,000 mL of liquid LB and incubated for 48 h at 180 r/min at 28°C. The fermentation solution was centrifuged at 3000 r/min for 10 min at 4°C, and the upper fermentation solution was filtered using a bacterial filter (0.22 μm). The inhibition rate of the resulting fermentation liquor on the mycelial growth of S. rolfsii LC1 was determined using a solid plate method. The LT1 fermentation liquor was prepared in 20 mL PDA plates at proportions of 2, 6, and 10% (v/v). PDA plates without the fermentation filtrate were used as controls. The mycelial plug of activated S. rolfsii LC1 (φ = 5 mm) was inoculated in the center of the PDA plate and cultured at 28°C for 3 days. The diameter of each colony was measured using the criss-cross method with three replicates per treatment. The mycelial growth inhibition rate was calculated using the following formula:
Since the 10% fermentation liquor exhibited the best inhibitory effect against S. rolfsii LC1, it was selected to assess the effect of LT1 strain against other pathogens such as Rhizoctonia solani ZJSQK1 of Panax japonicus, Sclerotinia sclerotiorum BXHP1 of Pinellia ternata, Fusarium acuminatum MD1 of Ophiopogon japonicus, Fusarium oxysporum QK-4 of tobacco, Colletotrichum micotianae YCTJ1 of tobacco, Alternaria alternata YCCX1 of tobacco, and Botrytis cinerea ES8 of Paris polyphylla. All the aforementioned pathogens were obtained from the Institute of Chinese Herbal Medicines of the Hubei Academy of Agricultural Sciences and preserved.
2.7 Inhibitory effect of LT1 strain against southern blight on potted Coptis chinensis
First, the fermentation liquid and filtered liquid of the LT1 strain were directly sprayed onto potted C. chinensis, and then, healthy leaves were selected to inoculate the activated S. rolfsii LC1. Each Potted C. chinensis was inoculated with three leaves. Potted C. chinensis inoculated with LC1 was then sealed in a plastic bucket to maintain moisture, and the incidence of southern blight was observed. Untreated C. chinensis were inoculated with S. rolfsii LC1 as a control group, which were treated with tifuramide as a positive control group, and each experiment was repeated three times.
2.8 Effects of different extractants on inhibition activity of strain LT1 fermentation filtrate
From 150 mL of LT1 strain’s fermentation broth, 20 mL of acetic acid was incorporated to attain a pH of 3.0. The fermentation filtrate was then sequentially extracted with equal volumes (1:1 v/v) of ethyl acetate, dichloromethane, methanol, and acetone. The extracts were clarified by passing them through a 0.22 μm filter to remove any bacterial residues. Subsequently, these filtered extracts were incorporated into a PDA medium at a 1:10 (v/v) ratio to prepare agar plates. An actively growing mycelial plug of S. rolfsii LC1 (φ = 5 mm) was centrally inoculated onto each PDA plate. The plates were then incubated at 28°C for 3 days. As a control, an equal volume of each solvent was added to separate PDA plates. The experiment was conducted in triplicate for statistical robustness. The diameter of each treated colony was determined by the cross-cross method. The mycelial growth inhibition rate was calculated using the following formula:
2.9 Identification of Lipopeptide antimicrobial compounds produced by the LT1 strain
The fermentation filtrate was extracted with a 1:1 (v:v) acetone solution. In total, 1 μL of the acetone extract was placed on a target containing the same amount of mother liquor (CHCA) in the mass spectrometer and air-dried naturally. Matrix-assisted laser desorption/ionization time-of-flight mass spectrometry (MALDI-TOF-MS) (Waters Corporation, Milford, MA, United States) was used for mass spectrometry analysis by a Crylas FTS355-Q laser (CryLaS, Berlin, Germany) source (355 nm) as the ion analytical ionization source, with a frequency of 1,000 HZ, laser energy of 250, and sample test range of 0–2000 m/z.
2.10 Effect of LT1 strain on mycelial morphology of Sclerotium rolfsii LC1
The fermentation filtrate of LT1 was mixed with PDA medium at a ratio of 1:9 (v/v) to prepare a plate. An activated S. rolfsii LC1 mycelial plug (φ = 5 mm) was inoculated into the center of the PDA plate and cultured at 28°C for 3 days. The mycelium was excised and rapidly submerged into glutaraldehyde solution for electron microscopy fixation for 2 hours, then washed three times with 0.1M phosphate buffer (PB) at pH 7.4. The mycelial morphology was observed using a scanning electron microscope (HITACHI Regulus 8,100) (Hitachi Production Co., LTD., Tokyo, Japan) and a transmission electron microscope (HITACHI HT7800) (Hitachi Production Co., LTD., Tokyo, Japan).
2.11 Statistical analysis
The data were analyzed using analysis of variance and Duncan’s multiple comparison tests with SPSS 22.0 (International Business Machines Corporation, New York, United States). The mean ± standard error (SEM) was used to express the data. Significance levels were indicated as * p < 0.05 and ** p < 0.01 for two levels of significant differences. GraphPad Prism software (version 6.0, GraphPad Software, Calif., United States) was used for data visualization.
3 Results
3.1 Bacillus sp. LT1 isolated from the rhizosphere soil of Coptis chinensis significantly inhibited the growth of Sclerotium rolfsii LC1
A total of 46 bacterial strains with different morphologies were obtained by coating the diluted soil suspension onto R2A, BHI, and NA media to obtain single colonies. The 46 strains were preliminarily identified by 16S rDNA that belong to 4 phyla, 9 classes, 10 orders, 17 families, 24 genera, and 32 species (Supplementary Table S1). The sequencing results of 16S rDNA for all strains are shown in Supplementary Data S1. The inhibitory effect of LT1 strain on the mycelial growth of S. rolfsii LC1 was measured using the face-off culture method. Many strains, including LT1, LT2, LT4, and SLT26, could significantly inhibit the growth of S. rolfsii LC1. Among these, the LT1 strain showed the best inhibitory effect (78.41%) (Figures 1A,B).
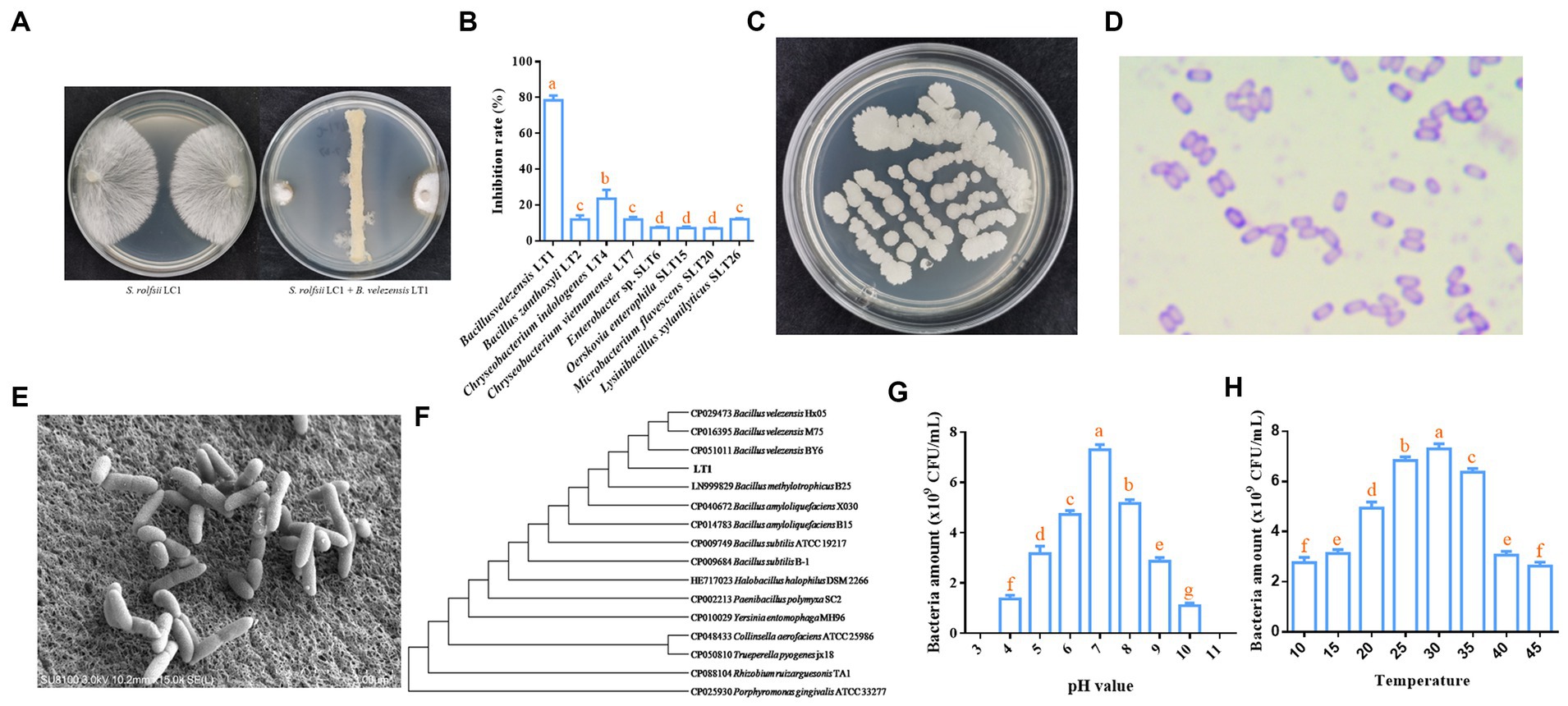
Figure 1. Biological and molecular biological characteristics of LT1 strain. (A) Morphology of the LT1 strain inhibiting the growth of S. rolfsii LC1; (B) Inhibitory effect of rhizosphere soil bacteria on S. rolfsii LC1 of C. chinensis; (C) Colony morphology of the LT1 strain on NA medium; (D) Morphology of Gram-stained LT1 strain; (E) Morphology of the LT1 strain observed under a scanning electron microscope; (F) Phylogenetic tree of LT1 based on 16S rDNA and gyrA genes; (G) Effect of pH on the growth of the LT1 strain; (H) Effect of temperature on the growth of the LT1 strain. Error bars represent mean ± standard deviation. One-way analysis of variance was performed using GraphPad Prism 6.0 software. Different letters represent significant differences between different treatment groups (p < 0.05).
3.2 LT1 Strain was identified as Bacillus velezensis LT1
Morphological and molecular biology methods were employed to identify the specific species of the LT1 strain with the best antagonistic effect. The single colony of LT1 on the NA medium was round, rough, and white (Figure 1C). The purple Gram staining indicated that the LT1 strain was a gram-positive bacterium (Figure 1D). Scanning electron microscopy showed that the bacteria were rod-shaped with obtuse ends and smooth cell walls, ranging in size from 0.3 μm x 0.39 μm to 0.75 μm x 1.53 μm (Figure 1E). The 16S rDNA sequence of the LT1 strain with gene number OL677384 was found to be 99.8% similar to that of B. velezensis Blz02 (number: MN961683) from NCBI. The gyrA sequence of the LT1 strain with gene number OL692738 was 100% similar to that of B. velezensis Blz02 (number: MT395302) from NCBI. Based on the 16S rDNA sequence and gyrA sequence, a joint evolutionary tree was constructed, and the LT1 strain was clustered with the B. velezensis BY6, M75, and Hx05 strains. It could be effectively distinguished from other bacteria, such as B. amyloliquefaciens and B. subtilis (Figure 1F). Based on the morphological characteristics and molecular sequences, the LT1 strain was identified as B. velezensis LT1. The optimum pH for the growth of B. velezensis LT1 was 7, and it could not grow when the pH was lower than 3 or greater than 11 (Figure 1G). The optimum growth temperature was between 25 and 30°C, and B. velezensis LT1 could grow at temperatures ranging from 10 to 45°C (Figure 1H).
3.3 Effectiveness of Bacillus velezensis LT1 fermentation liquor in controlling southern blight of Coptis chinensis
To assess the biocontrol potential of B. velezensis LT1 against southern blight of C. chinensis, we measured the inhibitory effect of the fermentation liquor without bacterial cells of B. velezensis LT1 on S. rolfsii LC1 in vitro and its control effect on potted C. chinensis. Three concentrations of B. velezensis LT1 fermentation filtrate showed inhibitory effects on the growth of S. rolfsii, with the inhibitory effects significantly varying among the concentrations. The inhibitory effect increased gradually as the fermentation filtrate concentration increased, reaching 90.43% when the fermentation filtrate concentration reached 10% (Figure 2A). We used a 10% fermentation filtrate to measure the inhibitory effects of B. velezensis LT1 on other pathogens. The results showed that B. velezensis LT1 had strong inhibitory effects on R. solani ZJSQK1 of P. japonicus, S. sclerotiorum BXHP1of P. ternata, F. acuminatum MD1 of O. japonicus, C. micotianae YCTJ1 of tobacco, A. alternata YCCX1 of tobacco, and B. cinerea ES8 of P. polyphylla (Figure 2B). These results demonstrate the high potential of B. velezensis LT1 for plant disease control. To further evaluate the control efficacy of B. velezensis LT1 against the southern blight of C. chinensis, we conducted pot experiments using different concentrations of fermentation liquid and filtrate. The control efficacy of 10% fermentation liquid and fermentation filtrate of B. velezensis LT1 against southern blight on potted C. chinensis reached 65%, whereas that of 1% fermentation liquid and fermentation filtrate of B. velezensis LT1 was approximately 40% (Figure 2C). The control efficacy of the 10% fermentation liquid was similar to that of the chemical fungicide tifuramide (Figure 2C). Notably, the same concentrations of fermentation liquid and filtrate without bacterial cells of B. velezensis LT1 showed equal control efficacy.
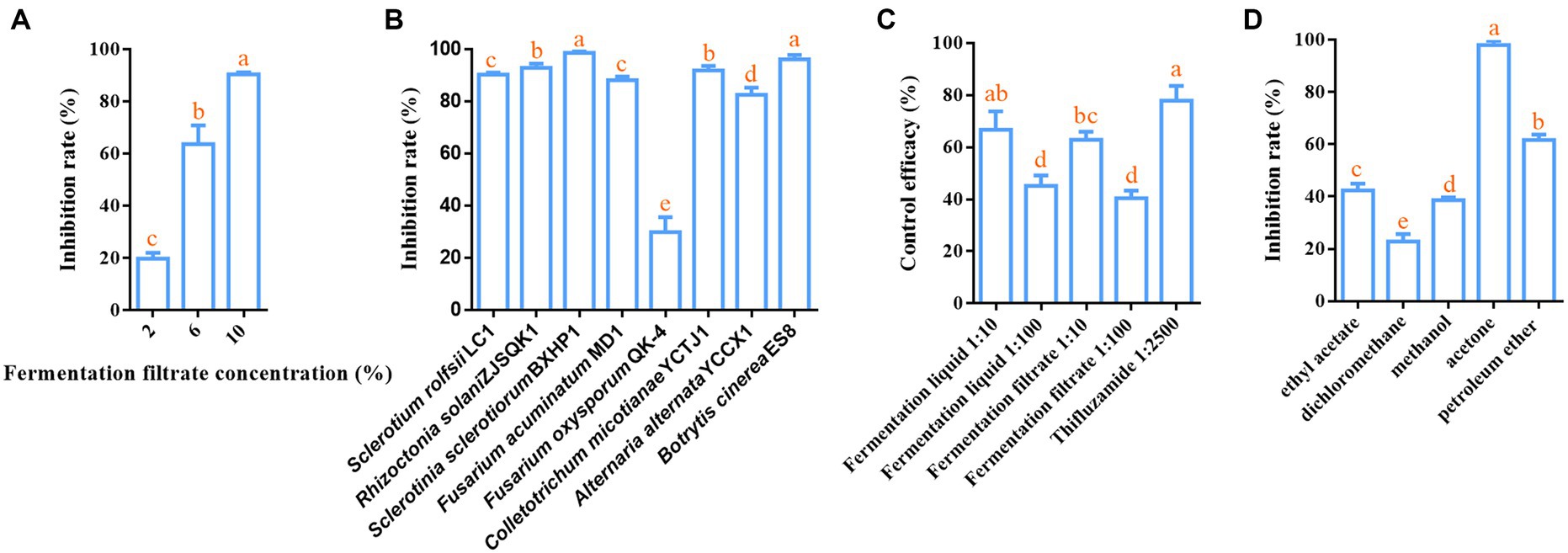
Figure 2. Inhibition effect of B. velezensis LT1 fermentation liquid on S. rolfsii LC1. (A) Inhibitory effect of fermentation liquid at different concentrations in PDA medium on S. rolfsii LC1; (B) Inhibitory effect of 10% fermentation liquid of B. velezensis LT1 on other pathogens; (C) Control efficacy of different concentrations of fermentation liquid and fermentation filtrate against southern blight in potted C. chinensis; (D) Inhibition effect of fermentation liquid of B. velezensis LT1 with different solvents extracted from S. rolfsii LC1. Error bars represent mean ± standard deviation. One-way analysis of variance was performed using GraphPad Prism 6.0 software. Different letters represent significant differences between different treatment groups (p < 0.05).
3.4 Bacillus velezensis LT1 Produces a variety of lipopeptide antimicrobial compounds to inhibit the growth of Sclerotium rolfsii LC1
The fermentation liquid without bacterial cells of B. velezensis LT1 was found to significantly inhibit the growth of S. rolfsii LC1. This led us to suspect that B. velezensis LT1 may have produced substances in the fermentation liquid that inhibit fungal growth. To identify the specific components of these substances, B. velezensis LT1 fermentation liquid was extracted using different solvents. Among the five extractants tested, the fermentation liquid extracted with acetone showed the best inhibitory effect with a mycelial inhibition rate of S. rolfsii LC1 of 99%, followed by petroleum ether extraction with an inhibition rate of 61.28%. The inhibition rates of the extraction products using the other three extractants were lower than 45% (Figure 2D). Therefore, the acetone extract of B. velezensis LT1 fermentation liquid was subjected to MALDI-TOF-MS (m/z 0–2000) analysis, and the resulting lipopeptide secondary metabolites (m/z 0–2000) are shown in Supplementary Data S2. B. velezensis LT1 was found to produce multiple antimicrobial compounds, including bacillomycin D, bacillomycin LC, iturin A, fengycin A, and surfactin (Table 1). Many of these antimicrobial compounds have been reported to inhibit the growth of fungal pathogens. These results suggest that the production of rich lipopeptide antimicrobial compounds by B. velezensis LT1 may be the primary mechanism by which it inhibits S. rolfsii LC1.
3.5 Bacillus velezensis LT1 significantly changed the mycelial morphology of Sclerotium rolfsii LC1
To further explore the effect of B. velezensis LT1 on S. rolfsii LC1, we observed the mycelial morphology of S. rolfsii LC1 under B. velezensis LT1 stress using scanning electron microscopy (SEM) and transmission electron microscopy (TEM). SEM observations showed that normal mycelia were arranged in an orderly manner with a smooth surface, uniform thickness, good extensibility, and a complete cell wall (Figure 3A). However, under the stress of B. velezensis LT1, the mycelia of S. rolfsii LC1 were folded with partial mycelial rupture, cytoplasmic leakage, and mycelial fragmentation (Figure 3B). Transmission electron microscopy showed that the control mycelia grew normally, with uniform and complete cell walls, clear boundaries, light cytoplasm, uniform distribution, clear nuclei, abundant and complete organelles evenly distributed in the cytoplasm, and a clear structure (Figure 3C). After treatment with B. velezensis LT1, the mycelial cell walls were significantly thickened, the cytoplasm was concentrated and dark in color, and a large number of white particles appeared. The nuclear structure was unclear, and no obvious nuclear membrane boundary was observed. Additionally, the number of mitochondria was significantly reduced (Figure 3D). These observations suggest that B. velezensis LT1 may have a destructive effect on the mycelial structure of S. rolfsii LC1.
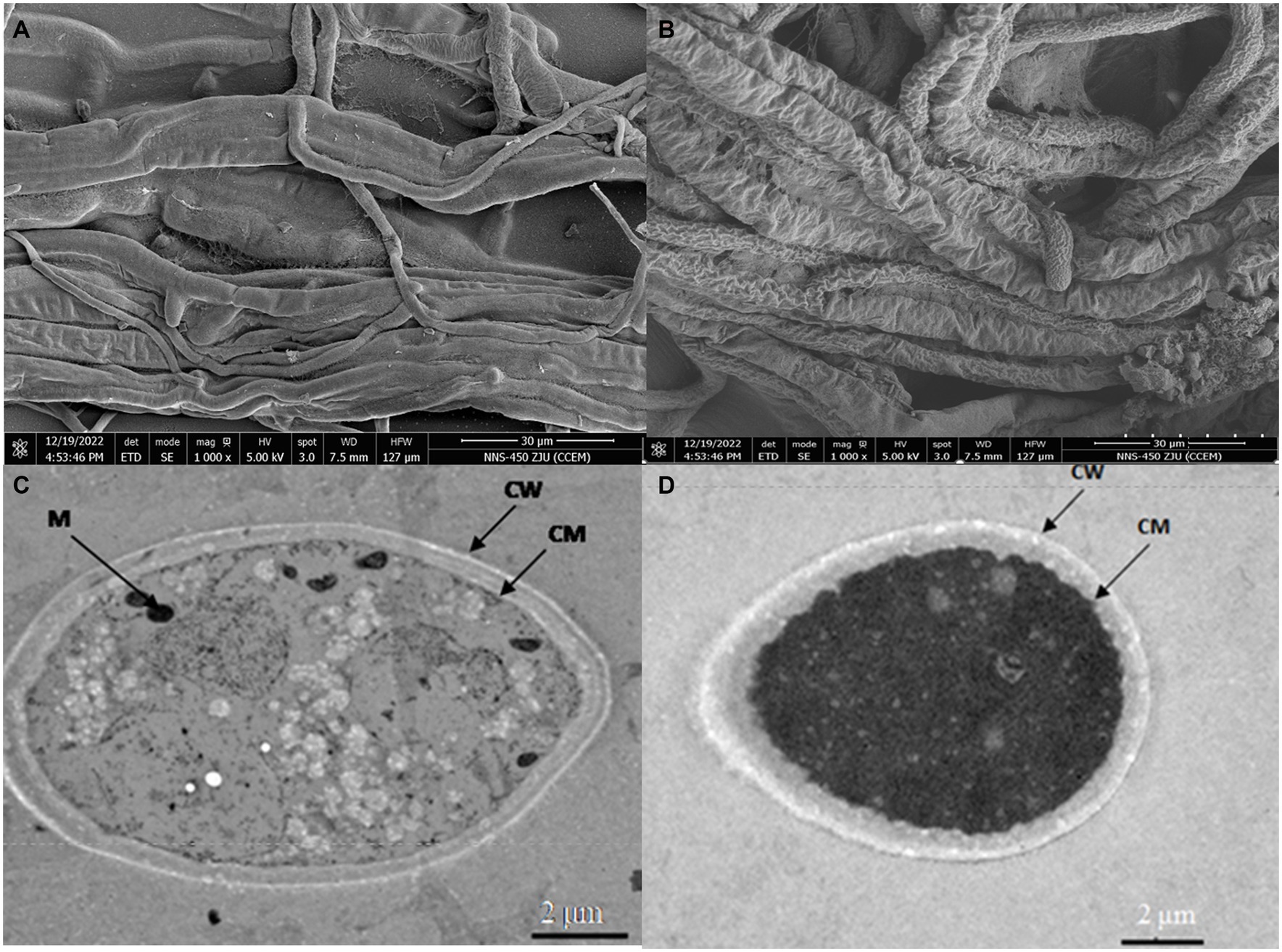
Figure 3. SEM and TEM images of mycelial morphology of S. rolfsii LC1. (A) SEM image of normal S. rolfsii LC1 mycelia; (B) SEM image of S. rolfsii LC1 mycelia under B. velezensis LT1 stress; (C) TEM image of normal S. rolfsii LC1 mycelia; (D) TEM image of S. rolfsii LC1 mycelia under B. velezensis LT1 stress. CM: cell membrane; CW: cell wall; M: mitochondria.
4 Discussion
The use of biological control agents (BCAs) is an important means to reduce the amount of chemical fungicides and protect the ecological environment (Bonaterra et al., 2022). Bacillus species are the most common soil-beneficial bacteria and one of the most studied and widely used BCAs (Kovács, 2019). In this study, B. velezensis LT1 was isolated from the rhizosphere soil where southern blight occurred severely. It can produce a variety of lipopeptide antagonistic fungal substances to inhibit the mycelial growth of S. rolfsii LC, a pathogen that causes southern blight. In pot assays, B. velezensis LT1 also showed beneficial control efficacy of southern blight. At the same time, B. velezensis LT1 had a strong inhibitory effect on the mycelial growth of various pathogens, showing great potential for biocontrol. This is the first time that B. velezensis has been reported to be used in the prevention and control of diseases in Chinese herbal medicines.
B. velezensis was first isolated, identified, and officially named by Ruiz et al. from the Velez River in 2005 (Ruiz-García et al., 2005). In 2007, the whole genome of B. velezensis FZB42 was sequenced, revealing the presence of nine gene clusters that encode antimicrobial compounds. These nine gene clusters, namely, fen, srf, bac, mln, bae, dfn, dhb, bmy, and nrs, encode synthase for the biosynthesis of antimicrobial compounds, such as fengycins, surfactin, bacillomycin, and others (Chen et al., 2007). Multiple studies have reported that B. velezensis produces various antibacterial compounds against plant pathogens. Vahidinasab et al. reported that B. velezensis UTB96 produced surfactin, fengycin, and iturin, which showed excellent inhibitory activity against the soybean fungal pathogen Diaporthe longicolla. Lysine and alanine could stimulate the production of these three lipopeptide antibacterial compounds (Vahidinasab et al., 2022). Kim et al. isolated B. velezensis TSA32-1 from the soil, which could inhibit the growth of F. graminearum, F. fujikuroi, A. alternatia, and Diaporthe actinidiae. The genome analysis of the strain TSA32-1 revealed the presence of a synthetic gene cluster of bacillaene and surfactant (Kim et al., 2022). In addition, B. velezensis CE100 secretes macrolactin A, which effectively controls the occurrence of bacterial diseases, such as bacterial soft rot of cucumber caused by Pectobacterium carotovorum (Htwe Maung et al., 2022). In this study, the lipopeptide antibiotics isolated from the fermentation filtrate of B. velezensis LT1 were found to be similar to those reported in previous studies. However, B. velezensis LT1 produced a relatively large number of antimicrobial compounds, including bacillomycin, surfactin, iturin, and fengycin, with different carbon chain lengths. Notably, the types of iturin and fengycin produced by B. velezensis LT1 were much higher than those reported in previous studies. These results suggest that B. velezensis LT1 may inhibit the growth of S. rolfsii LC1 by producing lipopeptide antibacterial compounds. However, this study did not differentiate the inhibitory effects of different lipopeptide antibacterial compounds on S. rolfsii LC1 or identify the main antibacterial compounds responsible for inhibiting its growth. Furthermore, the study did not explore the specific mechanism of action of lipopeptide antibacterial compounds on S. rolfsii LC1. Future research should focus on identifying the main antibacterial compounds responsible for inhibiting S. rolfsii LC1 and clarifying their molecular mechanisms of action. In addition, B. velezensis has been found to promote plant growth by secreting siderophores, indole-3-acetic acid (IAA), and volatile organic compounds (Raza et al., 2016; Myo et al., 2019; Wang et al., 2020). For example, B. velezensis HNH9 promoted the growth of upland cotton plants by upregulating the expression of growth-linked genes, such as EXP6, ARF1, ARF18, IAA9, CKX6, and GID1b (Hasan et al., 2022). Similarly, B. velezensis SX13 has been shown to promote the uptake and transport of elements by improving root structure and upregulating CsNRT1 expression, resulting in increased rates of element uptake and transport, which, in turn, promote photosynthesis and the activity of enzymes associated with carbon and nitrogen metabolism (Wang et al., 2022). Another study found that B. velezensis FH-1 promoted rice growth by regulating rhizosphere microbiome structure and nitrification function (Wang et al., 2023). In this study, the slow seed germination and growth of C. chinensis prevented determining whether B. velezensis LT1 could promote its growth. Nevertheless, the effect of B. velezensis LT1 on the germination of wheat seeds and rooting of onion was tested using hydroponics, and the results indicated that it could promote their growth (data not shown). This suggests that B. velezensis LT1 has the potential to promote plant growth. In future research, we will also investigate the promotion and mechanism of B. velezensis LT1 on the growth and accumulation of effective medicinal components of C. chinensis. In the past decade, researchers have discovered that complex microbial community assemblages in the rhizosphere, rather than single microbial strains, play an essential role in protecting plants from pathogens. Multiomics sequencing technologies have been widely used in the study of plant microbiome (Ritpitakphong et al., 2016). Therefore, the construction of synthetic communities (SynComs) based on beneficial microorganisms for plant disease control and growth promotion has gradually become a research focus. Schmitz et al. isolated the root core bacterial microflora of the desert plant Indigofera argentea and constructed a SynCom composed of 15 strains. This SynCom showed a strong growth-promoting effect on tomatoes under salt stress (Schmitz et al., 2022). Li et al. isolated bacterial strains from Astragalus mongholicus infected with F. oxysporum and constructed a SynCom that included Stenotrophomonas sp., Rhizobium sp., Ochrobactrum sp., and Advenella sp. The simplified SynCom effectively reduced the occurrence of root rot caused by F. oxysporum on A. mongholicus (Li et al., 2021). The use of cross-kingdom (fungal and bacterial) SynComs was more effective in suppressing Fusarium wilt disease (FWD) of tomato than fungal or bacterial SynComs alone (Zhou et al., 2022). The results demonstrate the great potential of SynComs in controlling crop diseases and promoting crop growth. In this study, more than 30 different strains of bacteria from 24 genera and 4 phyla were isolated from rhizosphere soil with severe rhizome rot of C. chinensis. Among them, eight strains could directly inhibit the growth of S. rolfsii LC1. Additionally, Pseudomonas sp., Streptomyces sp., Microbacterium sp., and Pseudarthrobacter sp. have often been reported as beneficial microorganisms in plants (Vurukonda et al., 2018; Zhao et al., 2019; Ham et al., 2022; Venturi, 2022). These beneficial bacteria can serve as an important foundation for constructing SynComs to control root rot in C. chinensis.
Many Bacillus species have been reported to act as biocontrol agents for plant diseases (Ribeiro et al., 2021; Nie et al., 2022; Baard et al., 2023; Hernández-Huerta et al., 2023; Kadiri et al., 2023). According to previous reports, lipopeptide antimicrobial compounds play a key role in Bacillus to inhibit of the growth and development of plant pathogens (Amaning Danquah et al., 2022; Kim et al., 2022). Similarly, B. velezensis LT1, isolated from the rhizosphere soil with severe rhizome rot of C. chinensis, can inhibit the growth of many plant pathogens by secreting lipopeptide antimicrobial compounds. The great biocontrol potential of B. velezensis LT1 deserves further detailed study. The potential of lipopeptide antimicrobial compounds identified in this study for plant disease control should also be further investigated. Bacterial isolates that stimulate plant growth and inhibit the development of fungal pathogens would be good candidates for the development of bioactive formulations.
In summary, our findings demonstrate the ability of B. velezensis LC1 to inhibit the growth of harmful fungi and present the novel use of B. velezensis LC1 as a means of controlling southern blight in C. chinensis (Graphical Abstract). These results have significant implications as southern blight has already inflicted substantial harm on C. chinensis cultivation in China and continues to spread.
Data availability statement
The original contributions presented in the study are included in the article/Supplementary material, further inquiries can be directed to the corresponding authors.
Author contributions
TT: Conceptualization, Data curation, Visualization, Writing – original draft. FW: Data curation, Investigation, Writing – original draft. HH: Data curation, Writing – original draft. JG: Data curation, Investigation, Writing – original draft. XG: Funding acquisition, Investigation, Writing – original draft. YD: Conceptualization, Data curation, Writing – original draft. XW: Data curation, Visualization, Writing – original draft. QW: Writing – review & editing. JY: Visualization, Writing – review & editing.
Funding
The author(s) declare financial support was received for the research, authorship, and/or publication of this article. This study was supported by the central government guides local science and technology development fund projects (2022BGE256), Key Research and Development Plan Project of Hubei (2022BBA153), Hubei Technology Innovation Center for Agricultural Sciences-Key Research and Development Project of Science and Technology (2020–620–000-002-04), and Enshi Prefecture Science and technology planning project (D20220055).
Acknowledgments
The authors thank Wang Rufeng (Institute of Chinses Materia Medica, Shanghai University of Traditional Chinese Medicine, Shanghai, China) for providing assistance in the process of MALDI-TOF-MS analysis. The authors also would like to thank Editage (www.editage.cn) for English language editing.
Conflict of interest
The authors declare that the research was conducted in the absence of any commercial or financial relationships that could be construed as a potential conflict of interest.
Publisher’s note
All claims expressed in this article are solely those of the authors and do not necessarily represent those of their affiliated organizations, or those of the publisher, the editors and the reviewers. Any product that may be evaluated in this article, or claim that may be made by its manufacturer, is not guaranteed or endorsed by the publisher.
Supplementary material
The Supplementary material for this article can be found online at: https://www.frontiersin.org/articles/10.3389/fmicb.2024.1337655/full#supplementary-material
Footnotes
References
Amaning Danquah, C., Minkah, P. A. B., Osei Duah Junior, I., Amankwah, K. B., and Somuah, S. O. (2022). Antimicrobial compounds from microorganisms. Antibiotics 11:285. doi: 10.3390/antibiotics11030285
Baard, V., Bakare, O. O., Daniel, A. I., Nkomo, M., Gokul, A., Keyster, M., et al. (2023). Biocontrol potential of Bacillus subtilis and Bacillus tequilensis against four fusarium species. Pathogens 12:254. doi: 10.3390/pathogens12020254
Backer, R., Rokem, J. S., Ilangumaran, G., Lamont, J., Praslickova, D., Ricci, E., et al. (2018). Plant growth-promoting rhizobacteria: context, mechanisms of action, and roadmap to commercialization of biostimulants for sustainable agriculture. Front. Plant Sci. 9:1473. doi: 10.3389/fpls.2018.01473
Bonaterra, A., Badosa, E., Daranas, N., Francés, J., Roselló, G., and Montesinos, E. (2022). Bacteria as biological control agents of plant diseases. Microorganisms 10:1759. doi: 10.3390/microorganisms10091759
Cakmakci, R., Donmez, F., and Aydin, A. (2006). Growth promotion of plants by plant growth-promoting rhizobacteria under greenhouse and two different field soil conditions. Soil Biol. Biochem. 38, 1482–1487. doi: 10.1016/j.soilbio.2005.09.019
Chen, X. H., Koumoutsi, A., Scholz, R., Eisenreich, A., Schneider, K., Heinemeyer, I., et al. (2007). Comparative analysis of the complete genome sequence of the plant growth–promoting bacterium Bacillus amyloliquefaciens FZB42. Nat. Biotechnol. 25, 1007–1014. doi: 10.1038/nbt1325
Chou, S. T., Hsiang, C. Y., Lo, H. Y., Huang, H. F., Lai, M. T., Hsieh, C. L., et al. (2017). Exploration of anti-cancer effects and mechanisms of Zuo-Jin-wan and its alkaloid components in vitro and in orthotopic HepG2 xenograft immunocompetent mice. BMC Complement. Altern. Med. 17:121. doi: 10.1186/s12906-017-1586-6
Gao, X. Y., Liu, Y., Miao, L. L., Li, E. W., Sun, G., Liu, Y., et al. (2017). Characterization and mechanism of anti-Aeromonas salmonicida activity of a marine probiotic strain, Bacillus velezensis V4. Appl. Microbiol. Biotechnol. 101, 3759–3768. doi: 10.1007/s00253-017-8095-x
Ham, S. H., Yoon, A. R., Oh, H. E., and Park, Y. G. (2022). Plant growth-promoting microorganism Pseudarthrobacter sp. NIBRBAC000502770 enhances the growth and flavonoid content of geum aleppicum. Microorganisms 10:1241. doi: 10.3390/microorganisms10061241
Hasan, N., Khan, I. U., Farzand, A., Heng, Z., Moosa, A., Saleem, M., et al. (2022). Bacillus altitudinis HNH7 and Bacillus velezensis HNH9 promote plant growth through upregulation of growth-promoting genes in upland cotton. J. Appl. Microbiol. 132, 3812–3824. doi: 10.1111/jam.15511
Hernández-Huerta, J., Tamez-Guerra, P., Gomez-Flores, R., Delgado-Gardea, M., Robles-Hernández, L., Gonzalez-Franco, A. C., et al. (2023). Pepper growth promotion and biocontrol against Xanthomonas euvesicatoria by Bacillus cereus and Bacillus thuringiensis formulations. PeerJ 11:e14633. doi: 10.7717/peerj.14633
Htwe Maung, C. E., Choub, V., Cho, J.-Y., and Kim, K. Y. (2022). Control of the bacterial soft rot pathogen, Pectobacterium carotovorum by Bacillus velezensis CE 100 in cucumber. Microb. Pathog. 173:105807. doi: 10.1016/j.micpath.2022.105807
Kadiri, M., Sevugapperumal, N., Nallusamy, S., Ragunathan, J., Ganesan, M. V., Alfarraj, S., et al. (2023). Pan-genome analysis and molecular docking unveil the biocontrol potential of Bacillus velezensis VB7 against Phytophthora infestans. Microbiol. Res. 268:127277. doi: 10.1016/j.micres.2022.127277
Kim, J. A., Song, J. S., Kim, P. I., Kim, D. H., and Kim, Y. (2022). Bacillus velezensis TSA32-1 as a promising agent for biocontrol of plant pathogenic fungi. J. Fungi 8:1053. doi: 10.3390/jof8101053
Kovács, Á. T. (2019). Bacillus subtilis. Trends Microbiol. 27, 724–725. doi: 10.1016/j.tim.2019.03.008
Le, K. D., Yu, N. H., Park, A. R., Park, D. J., Kim, C. J., and Kim, J. C. (2022). Streptomyces sp. AN090126 as a biocontrol agent against bacterial and fungal plant diseases. Microorganisms 10:791. doi: 10.3390/microorganisms10040791
Li, Z., Bai, X., Jiao, S., Li, Y., Li, P., Yang, Y., et al. (2021). A simplified synthetic community rescues Astragalus mongholicus from root rot disease by activating plant-induced systemic resistance. Microbiome 9:217. doi: 10.1186/s40168-021-01169-9
Li, X., Wang, X., Shi, X., Wang, B., Li, M., Wang, Q., et al. (2020). Antifungal effect of volatile organic compounds from Bacillus velezensis CT32 against Verticillium dahliae and fusarium oxysporum. PRO 8:1674. doi: 10.3390/pr8121674
Li, X. J., Yao, C. X., Qiu, R., Bai, J. K., Liu, C., Chen, Y. G., et al. (2023). Isolation, identification, and evaluation of the biocontrol potential of a Bacillus velezensis strain against tobacco root rot caused by fusarium oxysporum. J. Appl. Microbiol. 134:lxac049. doi: 10.1093/jambio/lxac049
Li, X., Zeng, S., Wisniewski, M., Munir, S., Yu, L., An, F., et al. (2022). Current and future trends in the biocontrol of postharvest diseases. Crit. Rev. Food Sci. 10, 1–13. doi: 10.1080/10408398.2022.2156977
Liu, Y. L., He, P. B., He, P. F., Munir, S., Ahmed, A., Wu, Y. X., et al. (2022). Potential biocontrol efficiency of Trichoderma species against oomycete pathogens. Front. Microbiol. 13:974024. doi: 10.3389/fmicb.2022.974024
Manuel, P. G., Nelson, V. V., and Camilo, A. R. (2023). Total auxin level in the soil–plant system as a modulating factor for the effectiveness of PGPR inocula. Chem. Biol. Technol. Agric. 10, 1–17. doi: 10.1186/s40538-022-00370-8
Mehta, P., Walia, A., and Kulshrestha, S. (2014). Efficiency of plant growth-promoting P-solubilizing Bacillus circulans CB7 for enhancement of tomato growth under net house conditions. J. Basic Microbiol. 55, 33–44. doi: 10.1002/jobm.201300562
Mikaberidze, A., Paveley, N., Bonhoeffer, S., and van den Bosch, F. (2017). Emergence of resistance to fungicides: the role of fungicide dose. Phytopathology 107, 545–560. doi: 10.1094/PHYTO-08-16-0297-R
Myo, E. M., Liu, B., Ma, J., Shi, L., Jiang, M., Zhang, K., et al. (2019). Evaluation of Bacillus velezensis NKG-2 for bio-control activities against fungal diseases and potential plant growth promotion. Biol. Control 134, 23–31. doi: 10.1016/j.biocontrol.2019.03.017
Nie, L. J., Ye, W. Q., Xie, W. Y., and Zhou, W. W. (2022). Biofilm: new insights in the biological control of fruits with Bacillus amyloliquefaciens B4. Microbiol. Res. 265:127196. doi: 10.1016/j.micres.2022.127196
Oleńska, E., Małek, W., Wójcik, M., Swiecicka, I., Thijs, S., and Vangronsveld, J. (2020). Beneficial features of plant growth-promoting rhizobacteria for improving plant growth and health in challenging conditions: a methodical review. Sci. Total Environ. 743:140682. doi: 10.1016/j.scitotenv.2020.140682
Rabbee, M., Ali, M., Choi, J., Hwang, B., Jeong, S., and Baek, K. (2019). Bacillus velezensis: a valuable member of bioactive molecules within plant microbiomes. Molecules 24:1046. doi: 10.3390/molecules24061046
Raza, W., Ling, N., Yang, L., Huang, Q., and Shen, Q. (2016). Response of tomato wilt pathogen Ralstonia solanacearum to the volatile organic compounds produced by a biocontrol strain Bacillus amyloliquefaciens SQR-9. Sci. Rep. 6:24856. doi: 10.1038/srep24856
Ribeiro, I. D. A., Bach, E., da Silva Moreira, F., Müller, A. R., Rangel, C. P., Wilhelm, C. M., et al. (2021). Antifungal potential against Sclerotinia sclerotiorum (lib.) de Bary and plant growth promoting abilities of Bacillus isolates from canola (Brassica napus L.) roots. Microbiol. Res. 248:126754. doi: 10.1016/j.micres.2021.126754
Ritpitakphong, U., Falquet, L., Vimoltust, A., Berger, A., Métraux, J., and L’Haridon, F. (2016). The microbiome of the leaf surface of Arabidopsis protects against a fungal pathogen. New Phytol. 210, 1033–1043. doi: 10.1111/nph.13808
Ruiz-García, C., Béjar, V., Martínez-Checa, F., Llamas, I., and Quesada, E. (2005). Bacillus velezensis sp. nov., a surfactant-producing bacterium isolated from the river Vélez in Málaga, southern Spain. Int. J. Syst. Evol. Microbiol. 55, 191–195. doi: 10.1099/ijs.0.63310-0
Schmitz, L., Yan, Z., Schneijderberg, M., de Roij, M., Pijnenburg, R., Zheng, Q., et al. (2022). Synthetic bacterial community derived from a desert rhizosphere confers salt stress resilience to tomato in the presence of a soil microbiome. ISME J. 16, 1907–1920. doi: 10.1038/s41396-022-01238-3
Sun, X., Xu, Z., Xie, J., Hesselberg-Thomsen, V., Tan, T., Zheng, D., et al. (2022). Bacillus velezensis stimulates resident rhizosphere Pseudomonas stutzeri for plant health through metabolic interactions. ISME J. 16, 774–787. doi: 10.1038/s41396-021-01125-3
Vahidinasab, M., Adiek, I., Hosseini, B., Akintayo, S. O., Abrishamchi, B., Pfannstiel, J., et al. (2022). Characterization of Bacillus velezensis UTB96, demonstrating improved lipopeptide production compared to the strain B. velezensis FZB42. Microorganisms 10:2225. doi: 10.3390/microorganisms10112225
Venturi, V. (2022). T4BSS-dependent biocontrol by plant-beneficial Pseudomonas. Nat. Microbiol. 7, 1508–1509. doi: 10.1038/s41564-022-01232-7
Vurukonda, S. S. K. P., Giovanardi, D., and Stefani, E. (2018). Plant growth promoting and biocontrol activity of Streptomyces spp. as endophytes. Int. J. Mol. Sci. 19:952. doi: 10.3390/ijms19040952
Wang, J., Qu, F., Liang, J., Yang, M., and Hu, X. (2022). Bacillus velezensis SX13 promoted cucumber growth and production by accelerating the absorption of nutrients and increasing plant photosynthetic metabolism. Sci. Hortic. 301:111151. doi: 10.1016/j.scienta.2022.111151
Wang, J., Wang, L., Lou, G. H., Zeng, H. R., Hu, J., Huang, Q. W., et al. (2019). Coptidis Rhizoma: a comprehensive review of its traditional uses, botany, phytochemistry, pharmacology and toxicology. Pharm. Biol. 57, 193–225. doi: 10.1080/13880209.2019.1577466
Wang, C., Zhao, D., Qi, G., Mao, Z., Hu, X., Du, B., et al. (2020). Effects of Bacillus velezensis FKM10 for promoting the growth of Malus hupehensis Rehd. And inhibiting fusarium verticillioides. Front. Microbiol. 10:2889. doi: 10.3389/fmicb.2019.02889
Wang, J., Zhao, S., Xu, S., Zhao, W., Zhang, X., Lei, Y., et al. (2023). Co-inoculation of antagonistic Bacillus velezensis FH-1 and Brevundimonas diminuta NYM3 promotes rice growth by regulating the structure and nitrification function of rhizosphere microbiome. Front. Microbiol. 14:1101773. doi: 10.3389/fmicb.2023.1101773
Xue, L., Yang, C., Jihong, W., Lin, L., Yuqiang, Z., Zhitong, J., et al. (2022). Biocontrol potential of Burkholderia sp. BV6 against the rice blast fungus Magnaporthe oryzae. J. Appl. Microbiol. 133, 883–897. doi: 10.1111/jam.15605
Yue, Y., Wang, Z., Zhong, T., Guo, M., Huang, L., Yang, L., et al. (2023). Antifungal mechanisms of volatile organic compounds produced by Pseudomonas fluorescens ZX as biological fumigants against Botrytis cinerea. Microbiol. Res. 267:127253. doi: 10.1016/j.micres.2022.127253
Zhang, D., Gao, Y., Ke, X., Yi, M., Liu, Z., Han, X., et al. (2019). Bacillus velezensis LF01: in vitro antimicrobial activity against fish pathogens, growth performance enhancement, and disease resistance against streptococcosis in nile tilapia (Oreochromis niloticus). Appl. Microbiol. Biotechnol. 103, 9023–9035. doi: 10.1007/s00253-019-10176-8
Zhao, J., Liu, D., Wang, Y., Zhu, X., Xuan, Y., Liu, X., et al. (2019). Biocontrol potential of Microbacterium maritypicum Sneb159 against Heterodera glycines. Pest Manag. Sci. 75, 3381–3391. doi: 10.1002/ps.5546
Zhen, Z., Chang, B., Li, M., Lian, F.-M., Chen, L., Dong, L., et al. (2011). Anti-diabetic effects of a Coptis chinensis containing new traditional Chinese medicine formula in type 2 diabetic rats. Am. J. Chin. Med. 39, 53–63. doi: 10.1142/S0192415X11008646
Keywords: Bacillus velezensis, Sclerotium rolfsii, Coptis chinensis, lipopeptide antimicrobial compounds, biocontrol potential
Citation: Tang T, Wang F, Huang H, Guo J, Guo X, Duan Y, Wang X, Wang Q and You J (2024) Bacillus velezensis LT1: a potential biocontrol agent for southern blight on Coptis chinensis. Front. Microbiol. 15:1337655. doi: 10.3389/fmicb.2024.1337655
Edited by:
Daniela Minerdi, University of Turin, ItalyReviewed by:
Vishal Kumar, Yeungnam University, Republic of KoreaViviana Andrea Rapisarda, INSIBIO (CONICET-UNT), and Instituto de Química Biológica “Dr Bernabé Bloj”, Argentina
Copyright © 2024 Tang, Wang, Huang, Guo, Guo, Duan, Wang, Wang and You. This is an open-access article distributed under the terms of the Creative Commons Attribution License (CC BY). The use, distribution or reproduction in other forums is permitted, provided the original author(s) and the copyright owner(s) are credited and that the original publication in this journal is cited, in accordance with accepted academic practice. No use, distribution or reproduction is permitted which does not comply with these terms.
*Correspondence: Jingmao You, jingmaoyou@126.com; Qingfang Wang, 195035892@qq.com