- 1Laboratory Department of Sustainable Aquaculture, WorldFish, Dhaka, Bangladesh
- 2Antimicrobial Resistance Action Center (ARAC), Animal Health Research Division, Bangladesh Livestock Research Institute, Savar, Bangladesh
- 3Department of Sustainable Aquaculture, WorldFish, Penang, Malaysia
- 4Patriot Biotech Sdn Bhd, Bandar Sunway, Malaysia
- 5Weymouth Laboratory, Cefas: Centre for Environment Fisheries and Aquaculture Science, Weymouth, United Kingdom
- 6Veterinary Medicines Directorate FAO Reference Centre for Antimicrobial Resistance, Weybridge, United Kingdom
- 7Bacteriology Department, Animal Plant Health Agency, Weybridge, United Kingdom
- 8Department of Veterinary and Animal Sciences, Faculty of Health and Medical Sciences, University of Copenhagen, Frederiksberg, Denmark
Wet markets in low-and middle-income countries are often reported to have inadequate sanitation resulting in fecal contamination of sold produce. Consumption of contaminated wet market-sourced foods has been linked to individual illness and disease outbreaks. This pilot study, conducted in two major wet markets in Dhaka city, Bangladesh during a 4-month period in 2021 aimed to assess the occurrence and characteristics of Escherichia coli and non-typhoidal Salmonella spp. (NTS) from tilapia (Oreochromis niloticus) and shrimp (Penaeus monodon). Fifty-four individuals of each species were collected. The identity of the bacterial isolates was confirmed by PCR and their susceptibility toward 15 antimicrobials was tested by disk diffusion. The whole genome of 15 E. coli and nine Salmonella spp. were sequenced using Oxford Nanopore Technology. E. coli was present in 60–74% of tilapia muscle tissue and 41–44% of shrimp muscle tissue. Salmonella spp. was found in skin (29%) and gills (26%) of tilapia, and occasionally in muscle and intestinal samples of shrimp. The E. coli had several Multilocus sequence typing and serotypes and limited antimicrobial resistance (AMR) determinants, such as point mutations on glpT and pmrB. One E. coli (BD17) from tilapia carried resistance genes for beta-lactams, quinolones, and tetracycline. All the E. coli belonged to commensal phylogroups B1 and A and showed no Shiga-toxin and other virulence genes, confirming their commensal non-pathogenic status. Among the Salmonella isolates, five belonged to Kentucky serovar and had similar AMR genes and phenotypic resistance patterns. Three strains of this serovar were ST198, often associated with human disease, carried the same resistance genes, and were genetically related to strains from the region. The two undetermined sequence types of S. Kentucky were distantly related and positioned in a separate phylogenetic clade. Two Brunei serovar isolates, one Augustenborg isolate, and one Hartford isolate showed different resistance profiles. This study revealed high fecal contamination levels in tilapia and shrimp sold at two main wet markets in Dhaka. Together with the occurrence of Salmonella spp., including S. Kentucky ST198, a well-known human pathogen, these results stress the need to improve hygienic practices and sanitation standards at markets to improve food safety and protect consumer health.
Introduction
Aquaculture production of tilapia and shrimp in Bangladesh has experienced significant growth in recent years, driven by increasing demands from domestic consumers and export markets (Haque and Belton, 2021; Debnath et al., 2023; El-Sayed and Fitzsimmons, 2023). However, this expansion has raised concerns regarding antimicrobial use (AMU) practices and food safety aspects within the industry. Several studies highlighted the widespread and indiscriminate use of antimicrobials in tilapia and shrimp farms, with a lack of veterinary supervision and adherence to proper dosage and withdrawal periods (Thornber et al., 2020; Dewi et al., 2022). This unregulated AMU in aquaculture settings has been linked to the emergence and spread of antimicrobial resistance (AMR) in bacterial populations, including those present in aquatic environments and seafood products, which can enter the food chain and potentially represent a risk to humans (Lulijwa et al., 2020; Preena et al., 2020; Thornber et al., 2020). Furthermore, studies have reported the presence of AMR bacteria, such as extended-spectrum β-lactamase-producing Escherichia coli, Salmonella spp., and multidrug-resistant Vibrio spp., in aquaculture systems and associated products, posing potential risks to human health (Hamilton et al., 2018; Dewi et al., 2022). Therefore, implementing robust food safety measures from culture ponds to markets is crucial to ensure the sustainability of tilapia and shrimp production and public health safety in Bangladesh.
Wet markets are important points of sales of aquatic food products in Bangladesh and, therefore, play a crucial role in food safety. In Bangladesh, hygiene issues in these markets have always been a major concern. Different factors, including lack of proper sanitation and hygiene practices, inadequate storage and handling, lack of quality control, and extensive use of chemicals contribute to the challenges. Studies have shown that wet markets often have poor hygiene practices and facilities (Alam et al., 2016; Sobuj et al., 2022). In addition, contaminated water may be used to process aquatic produce, leading to the potential contamination of such products by fecal organisms, such as E. coli and Salmonella spp. (Rocha Rdos et al., 2014; Fernandes et al., 2018). These bacteria can cause foodborne illnesses in humans, and their presence in aquatic products has been suggested as an important source of AMR (Khan et al., 2022; Samad et al., 2023).
The origin of AMR in aquatic products can be attributed to various factors, including AMU in aquaculture, contamination with human and animal fecal matter containing AMR bacteria, and cross-contamination with AMR bacteria during processing and handling (Shikongo-Nambambi et al., 2012; Neela et al., 2015; Watts et al., 2017). The presence of AMR bacteria and associated genes in aquatic products raises food safety concerns, especially since aquatic products are often consumed with minimal cooking or even in their raw state. Resistance genes can subsequently be transferred among bacterial populations in the human intestine, leading to the spread of AMR and potential treatment failure of bacterial infections in humans (Okon et al., 2022).
E. coli and Salmonella spp. are two major pathogens causing serious infections in humans (Havelaar et al., 2015). E. coli causes simple gastrointestinal diseases to more severe diseases, while Salmonella spp. are responsible for illnesses, such as typhoid/paratyphoid fever, and non-typhoidal Salmonella spp. (NTS) cause food poisoning. The transmission route of E. coli and Salmonella spp. to humans is mainly via fecally contaminated water and food. E. coli and Salmonella spp. can develop resistance by selective pressures from antimicrobials and horizontally through the transmission of different mobile elements like plasmids and transposons (Butaye et al., 2006).
In this study, we aimed to develop a pilot genomic surveillance for AMR in E. coli and NTS isolated from tilapia and shrimp products purchased at wet markets in Dhaka, Bangladesh. Growth-based methods and PCR were used for bacterial genus identification and their antimicrobial susceptibility was tested by the disk diffusion method. Oxford nanopore sequencing was used to characterize the genomes of E. coli and Salmonella spp. to confirm their serovars, virulence factors, and the genetic basis of resistance. The findings of the study provide important insight into the occurrence and transmission of antimicrobial-resistant E. coli and Salmonella from tilapia and shrimp sold at wet markets. These findings can contribute to initiatives aimed at minimizing the risk of transmitting fecal bacterial pathogens and AMR along the food chain.
Materials and methods
Selection of fish and shrimp wet markets
For this pilot surveillance study of AMR, tilapia and shrimp specimens were purchased from two wet markets, Karwan Bazar wholesale market (KBW), and Karwan Bazar retail market (KBR), both located in the middle of Dhaka city. These markets are among the largest wholesale and retail wet markets in Bangladesh. Additionally, KBW is the main supplier to a range of different retail markets in Dhaka.
Collection and processing of fish and shrimp samples
The target species were tilapia (Oreochromis niloticus) and tiger shrimp (Penaeus monodon), which are commonly sold at the markets. Tilapia, ranging between 250 and 300 g, were mostly sold fresh or live whereas shrimp, 25–30 g each, were displayed on trays occasionally on ice by the traders at the markets. A total of 3 separate visits were made to KBR to collect 27 tilapia. During each visit, nine fresh tilapia were collected from one vendor; however, the vendor changed between the visits. Similarly, four separate visits were made to KBW to collect 27 fresh tilapia. For shrimp sample collection, two visits were made to KBR and two visits were made to KBW to collect 54 shrimp samples (27 from KBR and 27 from KBW). During each sampling, samples were collected from one single vendor; however, vendors were different at every visit. Sample numbers included: KBW: tilapia (n = 27), shrimp (n = 27); KBR: tilapia (n = 27), shrimp (n = 27). The number of shrimps purchased from each vendor between the visits was not the same. A total of 18 and 9 shrimp samples were collected during visit 1 and visit 2 at KBW, respectively. In a similar way, the same number of shrimp samples (18 and 9 samples) were collected during visit 1 and visit 2 at KBR market.
Trained personnel wearing sterile plastic gloves collected the samples at the markets, which were individually placed in sterile labeled zipper plastic bags kept in insulated boxes containing ice packs. Samples were transported to Savar, Dhaka, for further processing and analysis in the Animal Health laboratory of the Bangladesh Livestock Research Institute (BLRI). The duration time from the point of sampling at the market until returning to BLRI to start processing the samples was never more than 3 h.
Surface skin swabs, gill, muscle, and intestinal samples were obtained from tilapia using standardized methods. A sterile cotton swab was used to make three long body swipes while twisting the swab to maximize mucus collection along the body (head to tail). The outer surface of the fish was then sterilized by wiping a gauze pad soaked with 70% ethyl alcohol. Using a sterile scalpel and a pair of forceps, the skin was removed and approximately 50 g of muscle and 10 g of gills were collected (in excess) from both sides of the fish. The liver and other organs were carefully removed, and the gut was obtained by cutting it from both extremities from the pyloric caeca to the anus.
The outer surface of the shrimp was disinfected as done for tilapia. Using sterile scissors or scalpel, the intestine from the hepatopancreas to the anus was removed and the content was carefully extruded into a sterile falcon tube. After removing the shell, a meat sample for each shrimp (approximately 15 g) was collected. The different sample types were placed in separate sterile containers or tubes and labeled with unique designation numbers. All collected tissues from each specimen were processed and analyzed separately. Collected tilapia and shrimp specimens were stored at 4°C within two hours upon arrival at the laboratory.
Bacterial culture and species identification
Each sample type was analyzed for E. coli and Salmonella spp. All the samples were enriched in sterile trypticase soy broth (TSB, Oxoid, Hampshire, UK) following a 1:10 ratio. From each tilapia, approximately 25 g of muscles and 5 g of gill tissues were used and added to 225 mL and 45 mL of TSB, respectively, in separate stomacher bags. Approximately, 1 g of tilapia gut consisting of a mixture of two pieces of foregut and hindgut was added to 9 mL TSB in a 15 mL Falcon tube and the skin swab was placed into a 15 mL Falcon tube containing 5 mL of TSB. Ten grams of shrimp muscle tissue were added to 90 mL TSB in a stomacher bag and the whole intestine of the shrimp was added into a 15 mL Falcon tube containing 3 mL of TSB. All the samples were incubated for 18 ± 2 h at 37 ± 1°C.
A loopful of enriched broth culture was streaked onto different selective and indicative media as follows. E. coli was isolated on eosin methylene blue (EMB; Oxoid, Hampshire, UK) agar plates, which were incubated at 35–37°C for 18–24 h. Salmonella spp. was isolated on xylose lysine deoxycholate agar (XLD; Oxoid, Hampshire, UK) plates incubated at 35–37°C for 18–24 h.
Presumptive E. coli colonies on EMB and Salmonella spp. colonies on XLD agar were streaked onto nutrient agar (NA, Oxoid, Hampshire, UK) to test and ensure purity. The indole test for E. coli and the triple sugar iron (TSI) test for Salmonella spp. were done for biochemical validation. Isolates were stored at-70°C in 30% glycerol with brain heart infusion (BHI; Oxoid, Hampshire, UK) broth. In case the DNA extraction from the bacterial isolates was not done on the same day, a 10 μL loop full of bacterial suspension for each isolate was preserved/fixed in a 1.5 mL tube containing 500 μL of 100% ethanol with the tubes stored at-20°C for future DNA extraction. E. coli ATCC 25922 and S. enteritidis ATCC 4931 were used as positive controls when subculturing on selective agar media and for PCR. All selected isolates were Gram-stained following standard procedures. The identity of the presumptive E. coli and Salmonella spp. was confirmed by PCR (Table 1).
Antimicrobial susceptibility testing (AST)
AST was done using the disk diffusion method following the Clinical & Laboratory Standards Institute (CLSI) guideline VET01S-Ed5 (CLSI, 2020). Bacterial suspensions were prepared in phosphate-buffered saline solution to a 0.5 McFarland standard and spread onto Mueller Hinton agar (MH; Oxoid, Hampshire, UK) plates. Antimicrobial disks were placed on the surface of the MH agar plates, which were incubated at 35–37°C for 18–24 h. Disks with the following antimicrobials were used: ampicillin (AMP; 10 μg), azithromycin (AZM; 15 μg), chloramphenicol (CHL; 30 μg), cefepime (CEP; 30 μg), cefoxitin (FOX; 30 μg), ceftriaxone (CRO; 30 μg), cefuroxime sodium (CXM; 30 μg), ciprofloxacin (CIP; 5 μg), gentamicin (GEN; 10 μg), levofloxacin (LVX; 5 μg), meropenem (MEM; 10 μg), nalidixic acid (NAL; 30 μg), nitrofurantoin (NIT; 100 μg), norfloxacin (NOR; 10 μg), and trimethoprim-sulfamethoxazole (SXT; 1.25/23.75 μg) (Oxoid, Hampshire, UK). Inhibition zones were measured in millimeters using the scan inhibition zone reader (Interscience Scan 4,000, Interscience, France). Interpretation of the data was done using CLSI breakpoints for all antimicrobials except for nitrofurantoin, of which the zone of inhibition was measured in accordance with the European Committee on Antimicrobial Susceptibility Testing (EUCAST) (CLSI, 2020; EUCAST, 2023). Whole genome sequencing and bioinformatic analyses.
DNA extraction and purification
A total of 16 E. coli and 14 Salmonella spp. isolates from tilapia and shrimp samples were selected for whole genome sequencing and further analysis. Bacterial resuspension was pelleted, followed by the removal of supernatant (ethanol) via decantation. DNA extraction was performed as per the method of (Sokolov, 2000) with some modifications. The pellet was resuspended in 500 μL of lysis buffer (50 mM NaCl, 50 mM Tris–HCL pH8, 50 mM EDTA, 2% SDS) and incubated at 60°C for 30 min. Then, 3 μL RNAse A (10 mg/mL) was added to the lysate, followed by incubation at room temperature for 10 min. Salting out was performed via the addition of 333 μL (2/3 vol) saturated NaCl at 4°C for 5 min. The lysate was centrifuged to remove precipitated proteins and lipids and the aqueous layer containing the DNA was transferred to a new tube and mixed with 100 μL of isopropanol via inversion. Fifteen μl of magnetic beads were added and the solution was mixed by inversion, then incubated at room temperature for 10 min to promote the binding of nucleic acid to the beads’ surface. Beads and DNA were separated from the remaining lysate on a magnetic rack and washed twice with 75% ethanol. DNA elution from the bead was performed by the resuspension of the beads with 50 μL of TE buffer followed by incubation at 50°C for 5 min.
A subsample of the extracted gDNA was visualized by 2% agarose gel electrophoresis to assess overall DNA integrity and yield. DNA was purified and size-selected using 0.5X vol of magnetic beads to remove small molecular weight DNA as well as any other carry-over impurities from the initial extraction. The DNA was subsequently measured using a Denovix High sensitivity kit (Denovix, Wilmington, DE, USA) and normalized to approximately 25 ng/μl.
Nanopore library preparation and sequencing
Libraries were prepared using the following two rapid barcoding sequencing kits. The first kit, SQK-RBK004, was used for low throughput, up to 24 samples per library. Approximately 400 ng of DNA as measured by Qubit was fragmented according to the manufacturer’s instructions (Oxford Nanopore, UK). The barcoded samples were pooled in equal ratios to get a final 400 ng of genomic DNA and purified using SPRI Bead, followed by ligation of the rapid adapter (RAP). The second kit, SQK-RBK110.96, for high throughput, 96 samples per library, used approximately 50 ng of DNA per sample, and then samples were pooled, purified, and ligated with RAP. The final pooled library was sequenced on a Flongle flow cell for at least 12 h to assess index distribution. Base calling and demultiplexing of the fast5 files were both performed with Guppy v.5.0.7. Based on the index percentage, the tagmented products were re-pooled or re-prepped for large-scale sequencing on the MinION flow cell and base calling (super accuracy mode) and demultiplexing performed as before to generate the final fastq files for each of the samples prepared.
De novo assembly
Raw nanopore reads were adapter trimmed with a qscore of 9 or higher using porechop (Wick et al., 2017) and then filtered to retain reads longer than 2,000 bp using NanoFilt v2.6.0 (De Coster et al., 2018). The filtered Nanopore reads were assembled de novo using Flye v2.9 (Kolmogorov et al., 2019), followed by one round of polishing with racon (Vaser et al., 2017) and another round of polishing with medaka v1.4.41. Genome assembly statistics were generated using QUAST (Gurevich et al., 2013). Assessment of the genome completeness was done using BUSCO5 (Simão et al., 2015) and identified conserved microbial single-copy genes as listed in the bacteria_odb10 database.
Bacterial species identification and Salmonella serotyping
Whole genome sequence identification was performed using kraken2 to confirm the bacterial species of the sequenced strains (Wood et al., 2019). Out of the 16 E. coli and 14 Salmonella spp. Isolates from tilapia and shrimp samples that were sequenced, only 15 E. coli and 9 Salmonella spp. Genomes were further analyzed based on DNA quality control (e.g., high genomic completeness) (Tables 2, 3).
The genomes confirmed as Salmonella spp. were further analyzed with sistr_cmd and seqsero for confirmation of the serovar (Zhang et al., 2015; Yoshida et al., 2016). Where a discordant serovar was identified by these two tools, the KMER-based taxonomic assignment of kraken2 was used as the third comparison point where the majority was taken to decide on the serovar.
In-silico identification of multilocus sequence typing (MLST), phylogroup analysis, AMR genes and virulence factors
In-silico MLST was performed using the PubMLST database2 developed by Keith Jolley (Jolley and Maiden, 2010) and the open-source software (mlst tool) used to interrogate the database3 (Jolley et al., 2018). AMR genes were determined in the assembled genomes using Resfinder4.1 (Bortolaia et al., 2020) and AMRFinderPlus (Feldgarden et al., 2021). The virulence determinants of E. coli were determined using a virulence factor database (Chen et al., 2005) and the curated VirulenceFinder database for E. coli (Malberg Tetzschner et al., 2020), while Salmonella virulence was determined by looking for their Salmonella Pathogenicity Islands (SPIs) (Roer et al., 2016). Moreover, E. coli genomes were subjected to phylogroup analysis using pathogenwatch4 and clermontyping tools (Beghain et al,. 2018).
Phylogenetic analysis
Salmonella Kentucky was the main serovar determined in the analysis. Strains with this serovar were added to publicly available S. Kentucky genomes from migratory birds (Card et al., 2023), humans, and poultry in Bangladesh and its neighboring countries, such as India (human and sesame seeds), Nepal (human), Pakistan (chili), and Myanmar (human) (Supplementary Table S4). The phylogenetic analysis was run using as reference genome S. Kentucky strain 201,001,922 (accession number CP028357). For this analysis, single-nucleotide variants were called using Snippy version 4.6.06 under the following parameters: mapping quality of 60, a minimum base quality of 13, a minimum read coverage of 4, and a 75% concordance at a locus. We aligned core genome single-nucleotide variants by using Snippy Core version 4.1.0 for phylogeny inference and detected masked putative recombinogenic regions by using Gubbins version 2.4.1. A maximum-likelihood phylogenetic tree was built using RAxML version/8.2.12 and the generalized time-reversible model with 200 bootstraps. The final tree was rooted on the reference genome CP028357 and visualized with Microreact.7
Results
Occurrences of E. coli and Salmonella spp. in tilapia and shrimp
Surface skin swab, gill, muscle, and intestinal samples from tilapia obtained at the KBW and KBR contained E. coli as confirmed by PCR in between 30 and 78% of samples analyzed. There was no apparent association between the different sample types and the different visits to the markets. It is noted that despite muscle tissue samples being obtained following disinfection of the skin surface, 60 to 74% of such samples contained E. coli indicating cross-contamination during sample processing as meat samples normally would be expected to be sterile.
E. coli confirmed by PCR was found in 41 and 44% of muscle tissue and 22 and 33% of intestinal samples from shrimp at the KBW and KBR markets, respectively. There were no apparent differences in findings during the different visits to the markets.
Salmonella spp. confirmed by PCR was found in 29% of skin swabs and 26% of gill samples, but not in muscle tissue and intestinal samples, from 27 tilapia fish collected at KBR (Table 2). Salmonella spp. was isolated in 7% of skin swabs, 52% of gill samples, and one intestinal sample of 27 tilapia fish analyzed from the KBW. No muscle tissue and intestinal samples from shrimp obtained at KBW contained Salmonella spp. as confirmed by PCR whereas Salmonella spp. was isolated and confirmed by PCR in 4/27 (15%) muscle tissue and 2/27 (7%) intestinal samples in shrimp obtained at KBR (Table 2).
Genomic characterization and antimicrobial resistance of E. coli
The genomic characterization (i.e., size, MLST, serovar., phylogroup) and antimicrobial resistance patterns and genes of the 15 E. coli isolates obtained from shrimp and tilapia samples and that yielded quality genomes are shown in Table 3. The E. coli isolates from shrimp had total genome lengths ranging from 4,725,736 to 4,897,945 base pairs, whereas the E. coli isolates from tilapia exhibited total genome lengths ranging from 3,968,775 to 5,077,851 base pairs (see further details in Supplementary Table S1). MLST analysis of the isolates from shrimp revealed the presence of 7 sequence types (STs), including 3,640, 3,501, 1,662, 398, 196, 155, and 48. Serovar classification identified different serovars, such as H49, H19, H16-O103, H9-O8, H21-O159, H16-O8/O160, and H16 (Table 3). The tilapia isolates also display several different MLSTs. Serovar classifications included H7, H9-O49, H7-O18, H19-O8, H26-O8/O80, and H12-O28ab (Table 3).
The phylogenetic analysis of isolates from both shrimp and tilapia revealed that they belonged predominantly to the commensal phylogroups B1 and A (Table 3). The commensal nature of these isolates in warm-blooded animals and humans, but not in fish, was further underlined by the absence of major virulence factors displayed by the known E. coli pathotypes in the genomes (Supplementary Table S2). As commensal strains, the genome sequences did not contain any clinically important AMR genes except for point mutations on the glycerol-3-phosphate transporter (glpT) and the polymyxin resistance gene B (pmrB), known to be associated with potential resistance to fosfomycin and colistin, respectively (Table 3). The isolate BD17 from tilapia harbored the resistance genes, blaTEM-1B, qnrS13, and tet(A) encoding resistance to beta-lactams, quinolones, and tetracycline, respectively. None of the E. coli strains contained any plasmids. This isolate displayed phenotypic resistance to ampicillin and intermediate resistance to nalidixic acid and cefuroxime sodium (Table 3). Several strains showed phenotypic resistance to ampicillin (4 strains) and nitrofurantoin (3 strains), and several strains showed intermediate resistance to some antimicrobial classes, e.g., 13/14 E. coli strains were intermediately resistant to cefuroxime sodium. However, no genes associated with such resistance and intermediate resistance were identified (Table 3). One E. coli strain was resistant to ceftriaxone. Further details on the phenotypic resistance are provided in Supplementary Table S5.
Genomic characterization and antimicrobial resistance of Salmonella spp.
The genomic characteristics and serovars of the nine Salmonella spp. isolates obtained from tilapia samples are shown in Table 4 (see further details in Supplementary Table S1). These strains yielded quality genomes and were isolated between 12 September and 31 December 2021. All strains were Salmonella enterica subspecies enterica.
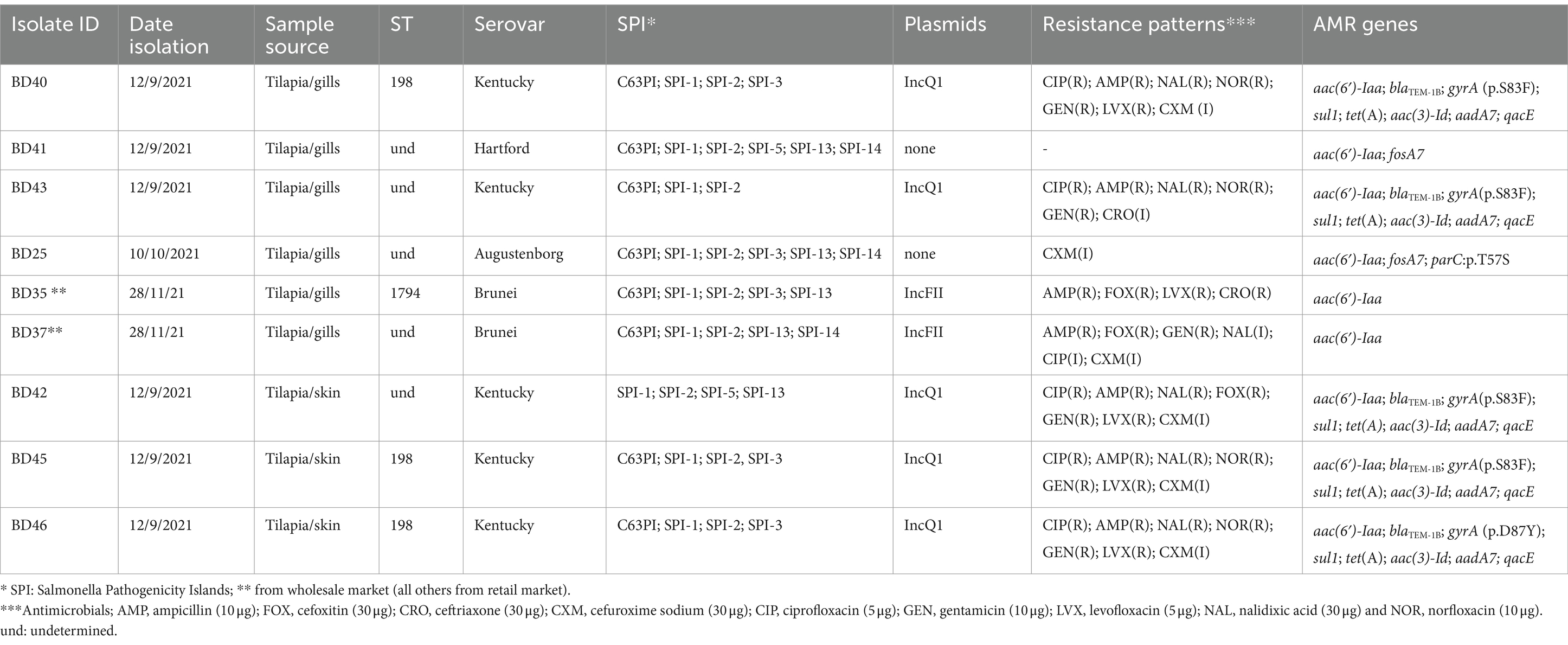
Table 4. Genomic characterization of Salmonella enterica isolates from tilapia sold at wet markets in Bangladesh.
Isolate BD25 exhibited a total genome length of 4,774,230 bp and belonged to the Augustenborg serovar and the AMR genes detected were aac(6′)-Iaa and fosA7, as well as parC:p.T57S mutation. The isolate showed intermediate resistance to CXM. Notably, the pathogenicity islands SPI-1, SPI-13, SPI-14, SPI-2, SPI-3, and C63PI were present, while no plasmids were detected.
Isolate BD35, belonging to the Brunei serovar., had a total genome length of 4,832,090 bp. MLST analysis revealed ST1794 and the presence of the aac(6′)-Iaa gene encoding resistance to aminoglycosides. The isolate was resistant to AMP, FOX, LVX, and CRO. SPI-1, SPI-13, SPI-2, SPI-3, and C63PI were identified, along with the IncFII plasmid. BD37 had also the Brunei serovar. The aminoglycoside resistance gene aac(6′)-Iaa was also detected together with SPI-1, SPI-13, SPI-14, SPI-2, and C63PI. This isolate was resistant to AMP, GEN, and FOX and showed intermediate resistance to other antimicrobials. Both isolates contained the IncFII plasmid.
Isolate BD41 belonged to the Hartford serovar. The AMR genes, aac(6′)-Iaa and fosA7 (fosfomycin) were present, along with C63PI, SPI-1, SPI-13, SPI-14, SPI-2, and SPI-5. No plasmids were observed. The isolate was fully susceptible to all antimicrobials tested.
Isolates BD42, BD40, BD43, BD45, and BD46, were all the Kentucky serovar and exhibited total genome lengths ranging from 4,887,734 to 4,920,732 bp. These isolates shared similar AMR genes including blaTEM-1B, gyrA mutations, sul1, tet(A), aac(3)-Id, and aadA7. The isolates showed similar phenotypic resistance patterns, i.e., to AMP, CIP, GEN, LVX, NAL, and NOR. Some isolates did also show intermediate resistance to other antimicrobials (further details on the phenotypic resistance are provided in Supplementary Table S6). SPI-1, SPI-2, and SPI-5 were identified, along with the IncQ1 plasmid. Three isolates were ST198. They all have the K variant of SGI-1 carrying the same resistance genes all on the same contig in each genome. It should be noted that the plasmids were detected based on their replication proteins not by reconstructing the entire plasmids.
The phylogenetic analysis of the genomes of the five S. Kentucky isolates from this study along with publicly available genomes of S. Kentucky from Bangladesh and neighboring countries revealed different levels of genetic relatedness among the strains circulating in the region and most of the isolates in our study (Figure 1, Supplementary Table S3). Out of all the tilapia isolates, BD40 had the least number of SNPs compared with the non-tilapia isolates (e.g., 30 SNPs with a migratory bird isolate). BD42 shows the greatest number of SNPs compared with BD43 (5,306 SNPs) and all remaining isolates. This was followed by BD43. BD42 and BD43 (non-ST198) clustered together. The Salmonella isolates from tilapia isolates evolved in one phylogenetic cluster from a common ancestor with the migratory bird isolates (SRR24520380/64), which have then further diverged with the remaining 10 migratory birds’ isolates (see further details on pairwise matrix on S. Kentucky Supplementary Table S4).
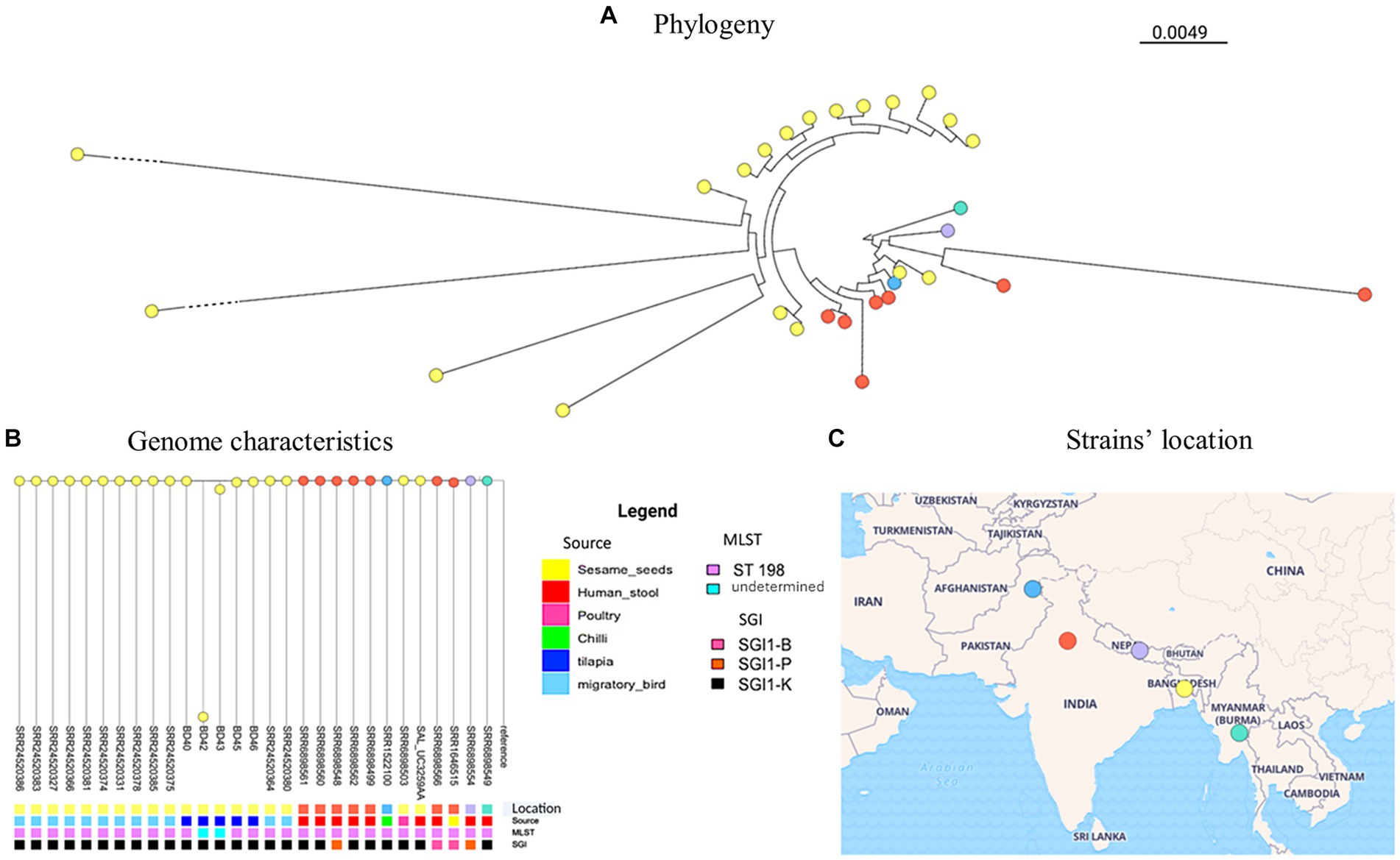
Figure 1. S. Kentucky phylogeny (A) including publicly available genome characteristics of S. Kentucky (B) originating from Bangladesh and surrounding countries. The geographical location of strains included from each country is shown on the map (C).
Discussion
In this study, we assessed the occurrence of E. coli and NTS and the AMR of such isolates obtained from tilapia and shrimp products at retail, to assess the potential food safety and health risk to consumers.
The high occurrences of E. coli in skin swabs, gill, muscle, and intestinal samples from tilapia and shrimp sold at two main wet markets in Dhaka, Bangladesh indicates that fecal pollution and cross-contamination were common at the markets. The findings of E. coli in intestinal samples suggest that the tilapia and shrimp were raised in aquatic systems with fecal pollution, as E. coli is not part of the normal intestinal flora in fish and shrimp (Mohamed Hatha et al., 2003; Duran and Marshall, 2005; Vu et al., 2018), which contrasts with livestock such as in poultry and pigs (Dang and Dalsgaard, 2012; AbuOun et al., 2020; Islam et al., 2023). E. coli in muscle tissue may indicate cross-contamination, e.g., from the fish surface, during collection of the muscle tissue with other tissues before analysis. E. coli may also have entered the muscle tissue, e.g., from the fish gut and surface; however, this seems only to occur under highly stressed environmental aquatic conditions (Dang and Dalsgaard, 2012). It should be noted that the likelihood and levels of fecal contamination at markets may show seasonal variations, e.g., as a result of flooding and decreased hygiene conditions at markets.
On the contrary, there is no apparent explanation of the frequent finding of Salmonella spp. in gill and skin samples and their isolation in muscle tissue and intestinal samples, but the two latter samples are less likely to be fecally contaminated, e.g., during handling of the tilapia at the markets as opposed to surface skin and gills. While fish do not normally have Salmonella as part of their natural flora, they can become passive carriers (Fernandes et al., 2018). Reporting of Salmonella spp. in different types of seafood is relatively common due to fecal cross-contamination during handling and processing, but different serovars have also been found in the skin, gills, muscle, intestine, and feces of live fish (Fernandes et al., 2018; Baniga et al., 2019; Hounmanou et al., 2020, 2022).
The five Salmonella Kentucky strains analyzed in this study carried the SGI1-K island; all had an In4-type class 1 integron that contained only one cassette array and an adjacent mercury resistance module. Other studies have also found such a type of class 1 integrons in S. Kentucky as well as in other serovars (Levings et al., 2007; Doublet et al., 2009). The SGI-K island harbored resistance genes such as aac(6′)-Iaa, blaTEM-1B, sul1, tet(A), aac(3)-Id, and aadA7 that confer resistance to aminoglycosides, tetracycline, sulphonamide, and narrow spectrum beta-lactam antibiotics. The set of genes present in these strains is, however, different from that reported in a previous study (Hawkey et al., 2019); for instance, the presence of blaTEM1 on the SGI-K is uncommon. This may be attributed to the plasticity of the genomes that allows for the loss or gain of segments by homologous recombination and which is responsible for the development of several variants of SGI1 in Salmonella (Hawkey et al., 2019; Jibril et al., 2021). Moreover, on the SGI-K island of the strains, the resistance genes were located on the IS6 transposon as were also found in recent poultry isolates in Nigeria (Jibril et al., 2021), showing the potential distribution of resistance through these islands via various mobile elements.
The identification of SPI-1, SPI-2, and SPI-5 in S. Kentucky, as well as an IncQ1 plasmid, further highlights the genetic diversity and potential for horizontal gene transfer among these isolates. Notably, three of the isolates were found to belong to ST198. Of particular interest are the strains BD42 and BD43, which branched separately on the phylogenetic tree. This finding suggests the presence of distinct genetic lineages or recent evolutionary events contributing to the diversification of S. Kentucky strains in the study area as can already be observed in the distinctive SPIs compared to the other three strains.
When comparing our strains to publicly available genomes of S. Kentucky from countries around Bangladesh, we observed different levels of genetic relatedness among the strains circulating in the region and most of the isolates in our study. This suggests a potential regional dissemination of S. Kentucky strains and emphasizes the importance of continued surveillance and molecular epidemiological studies to monitor the spread and evolution of this pathogen. Since S. Kentucky is rarely isolated from fish, these findings support fecal contamination from human or animal origin at the wet markets where the samples were obtained. Nevertheless, fish can be asymptomatic carriers, where Salmonella spp. have been isolated from surface tissues, muscle, and intestine (Fernandes et al., 2018; Baniga et al., 2019; Hounmanou et al., 2020, 2022). In Bangladesh, S. Kentucky has also been reported in poultry and migratory birds (Card et al., 2023), which show close relatedness with the isolates from this study.
Conclusion
Our study showed a high level of fecal contamination with common findings of commensal E. coli in different sample types of tilapia and shrimp sold at two main wet markets in Dhaka. Together with the occurrence of Salmonella spp. in several products, e.g., S. Kentucky ST198, a well-known human pathogen, stresses the need to improve hygienic practices and sanitation standards at markets as well as in people’s homes to improve food safety and protect consumer health. Transmission of AMR bacteria to humans can occur directly through the consumption of or contact with contaminated products, posing a significant public health concern. Further genomic epidemiological analysis and disease burden estimations are needed in Bangladesh to assess the contribution of seafood to the overall occurrence of human salmonellosis as well as AMR problems in humans.
Scope statement
Retail wet markets in low- and middle-income countries are often reported to have inadequate sanitation resulting in fecal microbial contamination. Such contamination of sold produce with antimicrobial resistant (AMR) bacteria can be a potential risk to public health. In this study, we conducted a pilot genomic surveillance for antimicrobial resistant E. coli and nontyphoidal Salmonella spp. isolated from tilapia and shrimp products purchased at two largest wet markets in Dhaka using Oxford nanopore sequencing. The study's results provide important insights into the prevalence and transmission of antimicrobial-resistant E. coli and Salmonella in tilapia and shrimp sold at wet markets. This has the potential to play a crucial role in efforts aimed at reducing the risk of transmitting fecal bacterial pathogens and antimicrobial resistance throughout the food chain.
Data availability statement
The datasets generated and analyzed during the current study that support our findings are available in the National Center for Biotechnology Information (NCBI) repository at the following persistent web links: demultiplexed FastQ files for all 24 strains of E. coli and Salmonella spp. can be found under BioProject https://dataview.ncbi.nlm.nih.gov/object/PRJNA974206 PRJNA974206 with the corresponding BioSample accession numbers from https://dataview.ncbi.nlm.nih.gov/object/SAMN35176355 SAMN35176355 to https://dataview.ncbi.nlm.nih.gov/object/SAMN35176378 SAMN35176378 and Sequence Read Archive (SRA) accession numbers from https://dataview.ncbi.nlm.nih.gov/object/SRR24673188 SRR24673188 to https://dataview.ncbi.nlm.nih.gov/object/SRR24673211 SRR24673211.
Ethics statement
Ethical approval was not required for the study involving animals in accordance with the local legislation and institutional requirements because the authors confirm that the ethical policies of the journal, as noted on the journal’s author guidelines page, have been adhered to. No ethical approval was required as no live animals were used in this study. The tissues were obtained from fish killed as part of routine commercial food production (i.e., sourced from wet markets).
Author contributions
SR: Conceptualization, Formal analysis, Investigation, Methodology, Validation, Writing – original draft, Writing – review & editing. SH: Conceptualization, Formal analysis, Investigation, Methodology, Writing – original draft, Writing – review & editing. MSS: Methodology, Supervision, Writing – review & editing. FA: Investigation, Methodology, Writing – review & editing. LK: Conceptualization, Writing – review & editing. HMG: Data curation, Formal analysis, Writing – review & editing. AP: Conceptualization, Methodology, Writing – review & editing. RM-C: Writing – review & editing. YM-G-M: Data curation, Formal analysis, Writing – original draft, Writing – review & editing. AD: Formal analysis, Visualization, Writing – original draft. CVM: Conceptualization, Writing – review & editing. ZBB: Investigation, Writing – review & editing. MA-S: Conceptualization, Supervision, Writing – review & editing. DV-J: Methodology, Writing – review & editing. JD-D: Conceptualization, Data curation, Formal analysis, Investigation, Methodology, Supervision, Writing – original draft, Writing – review & editing.
Funding
The author(s) declare financial support was received for the research, authorship, and/or publication of this article. This work was supported by the UK FAO Reference Centre for Antimicrobial Resistance (which receives funding from the Fleming Fund, UK Aid program, the Department of Health and Social Care, Grant Ref: ODA/BANGLADESH) and by the CGIAR Initiative on "Protecting Human Health Through a One Health Approach" (which receives funding from funders who support this research through their contributions to the CGIAR Trust Fund: https://www.cgiar.org/funders/, Grant Number: INIT-07).
Acknowledgments
This study was a collaboration between WorldFish, Centre for Environment Fisheries and Aquaculture Science (CEFAS), and Bangladesh Livestock Research Institute (BLRI) and was conducted as part of the CEFAS project “AMR: Facilitating future collaboration on COVID-19 responses through capacity building on AMR livelihoods” and the CGIAR Initiative on “Protecting human health through a One Health approach.” WorldFish would like to thank its partners from the Fleming Fund Country Grant, Patriot Biotech, the Department of Veterinary and Animal Sciences, Faculty of Health and Medical Sciences, University of Copenhagen, Animal Plant Health Agency (APHA), UK, and Veterinary Medicines Directorate (VMD), UK FAO reference Centre for AMR.
Conflict of interest
The authors declare that the research was conducted in the absence of any commercial or financial relationships that could be construed as a potential conflict of interest.
Publisher’s note
All claims expressed in this article are solely those of the authors and do not necessarily represent those of their affiliated organizations, or those of the publisher, the editors and the reviewers. Any product that may be evaluated in this article, or claim that may be made by its manufacturer, is not guaranteed or endorsed by the publisher.
Supplementary material
The Supplementary material for this article can be found online at: https://www.frontiersin.org/articles/10.3389/fmicb.2024.1329620/full#supplementary-material
Footnotes
1. ^https://github.com/nanoporetech/medaka
3. ^https://github.com/tseemann/mlst
References
AbuOun, M., O’Connor, H. M., Stubberfield, E. J., Nunez-Garcia, J., Sayers, E., Crook, D. W., et al. (2020). Characterizing antimicrobial resistant Escherichia coli and associated risk factors in a cross-sectional study of pig farms in Great Britain. Front. Microbiol. 11:861. doi: 10.3389/fmicb.2020.00861
Alam, M., Haque, M., and Shikha, F. (2016). Studies on public health and hygiene condition of retailers at fish markets in south-Central Bangladesh. J. Bangladesh Agric. Univ. 12, 411–418. doi: 10.3329/jbau.v12i2.28702
Baniga, Z., Mdegela, R. H., Lisa, B., Kusiluka, L. J. M., and Dalsgaard, A. (2019). Prevalence and characterisation of Salmonella Waycross and Salmonella enterica subsp. salamae in Nile perch (Lates niloticus) of Lake Victoria, Tanzania. Food Control 100, 28–34. doi: 10.1016/j.foodcont.2019.01.006
Beghain, J., Bridier-Nahmias, A., Le Nagard, H., Denamur, E., and Clermont, O. (2018). ClermonTyping: An easy-to-use and accurate in silico method for Escherichia genus strain phylotyping. Microbial Genomics 4, e000192. doi: 10.1099/mgen.0.000192
Bortolaia, V., Kaas, R. S., Ruppe, E., Roberts, M. C., Schwarz, S., Cattoir, V., et al. (2020). ResFinder 4.0 for predictions of phenotypes from genotypes. J. Antimicrob. Chemother. 75, 3491–3500. doi: 10.1093/jac/dkaa345
Butaye, P., Michael, G. B., Schwarz, S., Barrett, T. J., Brisabois, A., and White, D. G. (2006). The clonal spread of multidrug-resistant non-typhi Salmonella serotypes. Microbes Infect. 8, 1891–1897. doi: 10.1016/j.micinf.2005.12.020
Card, R. M., Chisnall, T., Begum, R., Sarker, M. S., Hossain, M. S., Sagor, M. S., et al. (2023). Multidrug-resistant non-typhoidal Salmonella of public health significance recovered from migratory birds in Bangladesh. Front. Microbiol. 14:1162657. doi: 10.3389/fmicb.2023.1162657
Chen, L., Yang, J., Yu, J., Yao, Z., Sun, L., Shen, Y., et al. (2005). VFDB: a reference database for bacterial virulence factors. Nucleic Acids Res. 33, D325–D328. doi: 10.1093/nar/gki008
CLSI (2020). Performance standards for antimicrobial susceptibility testing. Thirtieth information supplement (M100). Wayne, PA: CLSI.
Dang, S. T. T., and Dalsgaard, A. (2012). Escherichia coli contamination of fish raised in integrated pig-fish aquaculture systems in Vietnam. J. Food Prot. 75, 1317–1319. doi: 10.4315/0362-028X.JFP-11-501
De Coster, W., D’Hert, S., Schultz, D. T., Cruts, M., and Van Broeckhoven, C. (2018). NanoPack: visualizing and processing long-read sequencing data. Bioinformatics 34, 2666–2669. doi: 10.1093/bioinformatics/bty149
Debnath, S. C., McMurtrie, J., Temperton, B., Delamare-Deboutteville, J., Mohan, C. V., and Tyler, C. R. (2023). Tilapia aquaculture, emerging diseases, and the roles of the skin microbiomes in health and disease. Aquac. Int. 31, 2945–2976. doi: 10.1007/s10499-023-01117-4
Dewi, R. R., Hassan, L., Daud, H. M., Matori, M. F., Nordin, F., Ahmad, N. I., et al. (2022). Prevalence and antimicrobial resistance of Escherichia coli, Salmonella and Vibrio derived from farm-raised red hybrid tilapia (Oreochromis spp.) and Asian sea bass (Lates calcarifer, Bloch 1970) on the west coast of peninsular Malaysia. Antibiotics 11:136. doi: 10.3390/antibiotics11020136
Doublet, B., Praud, K., Weill, F.-X., and Cloeckaert, A. (2009). Association of IS26-composite transposons and complex In4-type integrons generates novel multidrug resistance loci in Salmonella genomic island 1. J. Antimicrob. Chemother. 63, 282–289. doi: 10.1093/jac/dkn500
Duran, G. M., and Marshall, D. L. (2005). Ready-to-eat shrimp as an international vehicle of antibiotic-resistant bacteria. J. Food Prot. 68, 2395–2401. doi: 10.4315/0362-028x-68.11.2395
El-Sayed, A.-F. M., and Fitzsimmons, K. (2023). From Africa to the world—the journey of Nile tilapia. Rev. Aquac. 15, 6–21. doi: 10.1111/raq.12738
EUCAST (2023). The European committee on antimicrobial susceptibility testing. Breakpoint tables for interpretation of MICs and zone diameters. Available at: http://www.eucast.org/clinical_breakpoints/
Feldgarden, M., Brover, V., Gonzalez-Escalona, N., Frye, J. G., Haendiges, J., Haft, D. H., et al. (2021). AMRFinderPlus and the reference gene catalog facilitate examination of the genomic links among antimicrobial resistance, stress response, and virulence. Sci. Rep. 11:12728. doi: 10.1038/s41598-021-91456-0
Fernandes, D. V. G. S., Castro, V. S., Cunha Neto, A. D., and Figueiredo, E. E. D. S. (2018). Salmonella spp. in the fish production chain: a review. Cienc. Rural :e20180141:48. doi: 10.1590/0103-8478cr20180141
Gurevich, A., Saveliev, V., Vyahhi, N., and Tesler, G. (2013). QUAST: quality assessment tool for genome assemblies. Bioinformatics 29, 1072–1075. doi: 10.1093/bioinformatics/btt086
Hamilton, K., Chen, A., Owusu-Ansah, E., Gitter, A., Kozak, S., Niquice, C., et al. (2018). Salmonella risks due to consumption of aquaculture-produced shrimp. Microb. Risk Anal. 9, 22–32. doi: 10.1016/j.mran.2018.04.001
Haque, M., and Belton, B. (2021). Shrimp farming and research in Bangladesh adapts to COVID-19 pandemic. Available at:(https://digitalarchive.worldfishcenter.org/handle/20.500.12348/4433).
Havelaar, A. H., Kirk, M. D., Torgerson, P. R., Gibb, H. J., Hald, T., Lake, R. J., et al. (2015). World Health Organization global estimates and regional comparisons of the burden of foodborne disease in 2010. PLoS Med. 12:e1001923. doi: 10.1371/journal.pmed.1001923
Hawkey, J., Le Hello, S., Doublet, B., Granier, S. A., Hendriksen, R. S., Fricke, W. F., et al. (2019). Global phylogenomics of multidrug-resistant Salmonella enterica serotype Kentucky ST198. Microb. Genom. 5:e000269. doi: 10.1099/mgen.0.000269
Heijnen, L., and Medema, G. (2006). Quantitative detection of E. coli, E. coli O157 and other Shiga toxin producing E. coli in water samples using a culture method combined with real-time PCR. J. Water Health 4, 487–498. doi: 10.2166/wh.2006.0032
Hounmanou, Y. M. G., Baniga, Z., García, V., and Dalsgaard, A. (2022). Salmonella Salamae and S. Waycross isolated from Nile perch in Lake Victoria show limited human pathogenic potential. Sci. Rep. 12:4229. doi: 10.1038/s41598-022-08200-5
Hounmanou, Y. M. G., Dalsgaard, A., Sopacua, T. F., Uddin, G. M. N., Leekitcharoenphon, P., Hendriksen, R. S., et al. (2020). Molecular characteristics and zoonotic potential of Salmonella Weltevreden from cultured shrimp and tilapia in Vietnam and China. Front. Microbiol. 11:1985. doi: 10.3389/fmicb.2020.01985
Islam, M. S., Hossain, M. J., Sobur, M. A., Punom, S. A., Rahman, A. M. M. T., and Rahman, M. T. (2023). A systematic review on the occurrence of antimicrobial-resistant Escherichia coli in poultry and poultry environments in Bangladesh between 2010 and 2021. Biomed. Res. Int. 2023:e2425564. doi: 10.1155/2023/2425564
Jibril, A. H., Okeke, I. N., Dalsgaard, A., Menéndez, V. G., and Olsen, J. E. (2021). Genomic analysis of antimicrobial resistance and resistance plasmids in Salmonella serovars from poultry in Nigeria. Antibiotics 10:99. doi: 10.3390/antibiotics10020099
Jolley, K. A., Bray, J. E., and Maiden, M. C. J. (2018). Open-access bacterial population genomics: BIGSdb software, the PubMLST.org website and their applications. Wellcome Open Res. 3:124. doi: 10.12688/wellcomeopenres.14826.1
Jolley, K. A., and Maiden, M. C. (2010). BIGSdb: scalable analysis of bacterial genome variation at the population level. BMC Bioinformatics 11:595. doi: 10.1186/1471-2105-11-595
Khan, M., Paul, S. I., Rahman, M. M., and Lively, J. A. (2022). Antimicrobial resistant bacteria in shrimp and shrimp farms of Bangladesh. Water 14:3172. doi: 10.3390/w14193172
Kolmogorov, M., Yuan, J., Lin, Y., and Pevzner, P. A. (2019). Assembly of long, error-prone reads using repeat graphs. Nat. Biotechnol. 37, 540–546. doi: 10.1038/s41587-019-0072-8
Levings, R. S., Partridge, S. R., Djordjevic, S. P., and Hall, R. M. (2007). SGI1-K, a variant of the SGI1 genomic island carrying a mercury resistance region, in Salmonella enterica serovar Kentucky. Antimicrob. Agents Chemother. 51, 317–323. doi: 10.1128/aac.01229-06
Lulijwa, R., Rupia, E. J., and Alfaro, A. C. (2020). Antibiotic use in aquaculture, policies and regulation, health and environmental risks: a review of the top 15 major producers. Rev. Aquac. 12, 640–663. doi: 10.1111/raq.12344
Malberg Tetzschner, A. M., Johnson, J. R., Johnston, B. D., Lund, O., and Scheutz, F. (2020). In silico genotyping of Escherichia coli isolates for extraintestinal virulence genes by use of whole-genome sequencing data. J. Clin. Microbiol. 58, e01269–e01220. doi: 10.1128/JCM.01269-20
Malkawi, H. I. (2003). Molecular identification of Salmonella isolates from poultry and meat products in Irbid City, Jordan. World J. Microbiol. Biotechnol. 19, 455–459. doi: 10.1023/A:1025113912366
Mohamed Hatha, A. A., Maqbool, T. K., and Suresh Kumar, S. (2003). Microbial quality of shrimp products of export trade produced from aquacultured shrimp. Int. J. Food Microbiol. 82, 213–221. doi: 10.1016/s0168-1605(02)00306-9
Neela, F., Banu, M., Rahman, M., Rahman, M. A., and Alam, M. (2015). Occurrence of antibiotic resistant bacteria in pond water associated with integrated poultry-fish farming in Bangladesh. Sains Malays. 44, 371–377. doi: 10.17576/jsm-2015-4403-08
Okon, E. M., Okocha, R. C., Adesina, B. T., Ehigie, J. O., Alabi, O. O., Bolanle, A. M., et al. (2022). Antimicrobial resistance in fish and poultry: public health implications for animal source food production in Nigeria, Egypt, and South Africa. Front. Antibiot. 1:1043302. doi: 10.3389/frabi.2022.1043302
Preena, P. G., Swaminathan, T. R., Kumar, V. J. R., and Singh, I. S. B. (2020). Unravelling the menace: detection of antimicrobial resistance in aquaculture. Lett. Appl. Microbiol. 71, 26–38. doi: 10.1111/lam.13292
Rocha Rdos, S., Leite, L. O., de Sousa, O. V., and Vieira, R. H. (2014). Antimicrobial susceptibility of Escherichia coli isolated from fresh-marketed Nile tilapia (Oreochromis niloticus). J. Pathog. 2014:756539. doi: 10.1155/2014/756539
Roer, L., Hendriksen, R. S., Leekitcharoenphon, P., Lukjancenko, O., Kaas, R. S., Hasman, H., et al. (2016). Is the evolution of Salmonella enterica subsp. enterica linked to restriction-modification systems? mSystems 1:e00009-16. doi: 10.1128/mSystems.00009-16
Samad, M. A., Eberson, L., Begum, R., Alam, M. G. S., Talukdar, F., Akter, R., et al. (2023). Microbial contamination and antibiotic resistance in marketed food in Bangladesh: current situation and possible improvements. Antibiotics 12:555. doi: 10.3390/antibiotics12030555
Shikongo-Nambambi, M. N., Shoolongela, A. S., and Schneider, M. B. (2012). Control of bacterial contamination during marine fish processing. Available at:http://www.macrothink.org/journal/index.php/jbls/article/view/1033
Simão, F. A., Waterhouse, R. M., Ioannidis, P., Kriventseva, E. V., and Zdobnov, E. M. (2015). BUSCO: assessing genome assembly and annotation completeness with single-copy orthologs. Bioinformatics 31, 3210–3212. doi: 10.1093/bioinformatics/btv351
Sobuj, M. K. A., Rabby, A. F., Rahman, S., Hasan, S. J., Bhowmik, S., Islam, M. A., et al. (2022). Knowledge, attitudes, and practices on food safety and hygiene of wet and dry fish handlers in Cox’s bazar, Bangladesh. Food Sci. Nutr. 10, 4139–4154. doi: 10.1002/fsn3.3004
Sokolov, E. P. (2000). An improved method for DNA isolation from mucopolysaccharide-rich molluscan tissues. J. Molluscan Stud. 66, 573–575. doi: 10.1093/mollus/66.4.573
Thornber, K., Verner-Jeffreys, D., Hinchliffe, S., Rahman, M. M., Bass, D., and Tyler, C. R. (2020). Evaluating antimicrobial resistance in the global shrimp industry. Rev. Aquac. 12, 966–986. doi: 10.1111/raq.12367
Vaser, R., Sović, I., Nagarajan, N., and Šikić, M. (2017). Fast and accurate de novo genome assembly from long uncorrected reads. Genome Res. 27, 737–746. doi: 10.1101/gr.214270.116
Vu, T. T. T., Alter, T., Roesler, U., Roschanski, N., and Huehn, S. (2018). Investigation of extended-spectrum and AmpC β-lactamase-producing Enterobacteriaceae from retail seafood in Berlin, Germany. J. Food Prot. 81, 1079–1086. doi: 10.4315/0362-028X.JFP-18-029
Watts, J. E. M., Schreier, H. J., Lanska, L., and Hale, M. S. (2017). The rising tide of antimicrobial resistance in aquaculture: sources, sinks and solutions. Mar. Drugs 15:158. doi: 10.3390/md15060158
Wick, R. R., Judd, L. M., Gorrie, C. L., and Holt, K. E. (2017). Completing bacterial genome assemblies with multiplex Minion sequencing. Microb. Genom. 3:e000132. doi: 10.1099/mgen.0.000132
Wood, D. E., Lu, J., and Langmead, B. (2019). Improved metagenomic analysis with kraken 2. Genome Biol. 20:257. doi: 10.1186/s13059-019-1891-0
Yoshida, C. E., Kruczkiewicz, P., Laing, C. R., Lingohr, E. J., Gannon, V. P. J., Nash, J. H. E., et al. (2016). The Salmonella in silico typing resource (SISTR): an open web-accessible tool for rapidly typing and subtyping draft Salmonella genome assemblies. PLoS One 11:e0147101. doi: 10.1371/journal.pone.0147101
Keywords: Salmonella, Escherichia coli, tilapia, shrimp, antimicrobial resistance, whole genome sequencing, food safety, Bangladesh
Citation: Rheman S, Hossain S, Sarker MS, Akter F, Khor L, Gan HM, Powell A, Card RM, Hounmanou YMG, Dalsgaard A, Mohan CV, Bupasha ZB, Samad MA, Verner-Jeffreys DW and Delamare-Deboutteville J (2024) Nanopore sequencing for identification and characterization of antimicrobial-resistant Escherichia coli and Salmonella spp. from tilapia and shrimp sold at wet markets in Dhaka, Bangladesh. Front. Microbiol. 15:1329620. doi: 10.3389/fmicb.2024.1329620
Edited by:
Lucilla Iacumin, University of Udine, ItalyReviewed by:
Keshani Bhushan, Punjab Agricultural University, IndiaManyun Yang, Harvard University, United States
Copyright © 2024 Rheman, Hossain, Sarker, Akter, Khor, Gan, Powell, Card, Hounmanou, Dalsgaard, Mohan, Bupasha, Samad, Verner-Jeffreys and Delamare-Deboutteville. This is an open-access article distributed under the terms of the Creative Commons Attribution License (CC BY). The use, distribution or reproduction in other forums is permitted, provided the original author(s) and the copyright owner(s) are credited and that the original publication in this journal is cited, in accordance with accepted academic practice. No use, distribution or reproduction is permitted which does not comply with these terms.
*Correspondence: Shafiq Rheman, s.rheman@cgiar.org; Jérôme Delamare-Deboutteville, j.delamare@cgiar.org
†These authors have contributed equally to this work