- 1CAS Key Laboratory of Coastal Environmental Processes and Ecological Remediation, Yantai Institute of Coastal Zone Research, Chinese Academy of Sciences, Yantai, China
- 2University of Chinese Academy of Sciences, Beijing, China
- 3Shandong Key Laboratory of Coastal Environmental Processes, Yantai, China
- 4The Yellow River Delta Ecological Research Station of Coastal Wetland, Chinese Academy of Sciences, Yantai, China
- 5School of Geography and Environment, Liaocheng University, Liaocheng, China
- 6The College of Geography and Environmental Science, Henan University, Kaifeng, China
Saltmarsh is widely recognized as a blue carbon ecosystem with great carbon storage potential. Yet soil respiration with a major contributor of atmospheric CO2 can offset its carbon sink function. Up to date, mechanisms ruling CO2 emissions from saltmarsh soil remain unclear. In particular, the effect of precipitation on soil CO2 emissions is unclear in coastal wetlands, due the lack of outdoor data in real situations. We conducted a 7-year field manipulation experiment in a saltmarsh in the Yellow River Delta, China. Soil respiration in five treatments (−60%, −40%, +0%, +40%, and + 60% of precipitation) was measured in the field. Topsoils from the last 3 years (2019–2021) were analyzed for CO2 production potential by microcosm experiments. Furthermore, quality and quantity of soil organic carbon and microbial function were tested. Results show that only the moderate precipitation rise of +40% induced a 66.2% increase of CO2 production potential for the microcosm experiments, whereas other data showed a weak impact. Consistently, soil respiration was also found to be strongest at +40%. The CO2 production potential is positively correlated with soil organic carbon, including carbon quantity and quality. But microbial diversity did not show any positive response to precipitation sizes. r-/K-strategy seemed to be a plausible explanation for biological factors. Overall, our finding reveal that a moderate precipitation increase, not decrease or a robust increase, in a saltmarsh is likely to improve soil organic carbon quality and quantity, and bacterial oligotroph:copiotroph ratio, ultimately leading to an enhanced CO2 production.
1 Introduction
As part of global climate change, precipitation size is constantly changing on a regional or large scale (Alster et al., 2013; IPCC, 2021; Wu et al., 2023). It can alter soil moisture and salinity to influence soil respiration in a variety of ecosystems (Han et al., 2018; Li et al., 2021; Morris et al., 2022). Effects of precipitation events on soil respiration are variable and ecosystem-dependent (Jiang et al., 2013; Su et al., 2023a). For example, strong precipitation is likely to increase soil moisture levels but decrease CO2 fluxes in tropical rain forests (Cleveland et al., 2010; Zhang et al., 2015). Similarly, an observation suggested that precipitation events could decrease soil respiration by increasing soil moisture and inducing anoxic conditions in a coastal wetland (Han et al., 2018). In contrast, coastal wetland became a weaker annual CO2 absorption due to the extreme precipitation event (Wei et al., 2021). Wang et al. (2019) suggested an active soil respiration to changed precipitation. Increased precipitation stimulated soil respiration by 26.1%, while a reduction by 10.8% responding to low precipitation in a desert steppe (Wang et al., 2019). Further, ambient precipitation determined the sensitivity of soil respiration to different treatments (Li et al., 2023). To data, the response regime and governing factor on soil respiration are still not fully constrained in response to changed precipitation size (Su et al., 2023b), especial for coastal wetland soil sensitive to moisture content (Li et al., 2023).
Increased soil respiration rate by a more large size of precipitation was likely due to favorable soil microbial activity and community composition (Huang et al., 2018) or plant growth (Zhang et al., 2019; Zhou et al., 2019), litter decomposition (Canarini et al., 2017; Qin et al., 2019), carbon substrate availability (Wang et al., 2020), and soil salinization (Han et al., 2015; Zhao et al., 2020). Our previous results revealed that precipitation treatments in a coastal wetland significantly affected soil respiration through a series of abiotic and biotic processes, mainly by changing soil water and salt conditions (Li et al., 2021; Huang et al., 2023). In comparison, some studies showed high levels of precipitation caused soil organic carbon to accumulate, due to oxygen-limitation (Kramer and Chadwick, 2018; Possinger et al., 2020). Moreover, variable responses of dissolved organic carbon to precipitation events were also confirmed (Suseela et al., 2013; Warner et al., 2019). In compared to recalcitrant carbon, the labile one is more preferred by microbes (Chen et al., 2022). To this end, soil carbon quantity and quality reserve more attention to explain the link between precipitation and soil respiration.
Precipitation size can directly affect microbial community and function. Currently, aiming to gain a deeper understanding of interaction of soil organic carbon to microorganisms, ecologists have tried to classify microbial taxa based on their life strategies and resource preferences (e.g., copiotrophs vs. oligotrophs) (Fierer et al., 2007; Cotrufo et al., 2015; Chen et al., 2022), in term of r-/K-strategy. Microbial r-strategies (copiotrophic species) refer to organisms that have rapid growth rate and able to make full use of easily decomposed carbon. In contrast, K-strategy bacteria (oligotrophic species) are characterized by slower growth and have a more efficiency to utilize recalcitrant carbon (Fierer et al., 2007; Ho et al., 2017; Singh, 2018). The r-categories and K-categories provide a useful framework for understanding the traits that functionally similar taxa possess (Fierer et al., 2007; Ho et al., 2017). Precipitation size may affect soil respiration by change of microbial r-/K-strategy.
We hypothesized: (1) The response pattern (positive or negative) of CO2 production potential is likely to be distinct across the precipitation gradient; (2) Both quantity and quality of soil organic carbon are involved in the response of soil respiration; (3) Microbial community and r-/K-strategy govern soil respiration. To address the above potential mechanisms of the influence of precipitation change on soil CO2 emissions, we conducted a 7-year (2014–2021) field experiment in the Yellow River Delta, China. There were five treatments for this field experiment (−60%, −40%, +0%, +40%, and +60% of precipitation). First, we sampled topsoils in 2019–2021 and conducted some microcosm experiments. A detailed analysis on soil organic carbon characteristics and microbial function was conducted to verify proposed hypothesis.
2 Materials and methods
2.1 Site description
This study was conducted in the natural marshes (37°45′50″N, 118°59′24″E), which is located in the Yellow River Delta, Shandong, China. The local climate is a warm temperate and continental monsoon climate with a mean annual temperature of 12.9°C and minimum mean temperatures of 26.7°C in July and −2.8°C in January, respectively. The average annual precipitation is 530–630 mm, concentrated mostly in summer (from May to September). The soil texture in this region is sandy clay loam, and the soil type gradually changes from fluvo-aquic soil to saline soil (Han et al., 2018). The main founding species in this place are flood-tolerant Phragmites australis and salt-tolerant Suaeda salsa.
2.2 Experimental design
The field precipitation experiment, initiated in October 2014, included five treatments: a decrease of 60% (−60%) and 40% (−40%), control, and an increase of 40% (+40%) and 60% (+60%) of precipitation. This experiment was established using a completely randomized block design on the natural vegetation and soil at the Yellow River Delta Ecological Research Station of the Coastal Wetland, Chinese Academy of Science.1 In all, 20 plots in total were randomly assigned to the five precipitation treatments, with each treatment being randomly repeated four times. The plot size was 3 × 4 m with 1 m between any two adjacent plots, and a core area of 2 × 3 m was used for measurements. Detailed information is given our previous studies (Li et al., 2021, 2023). Typically, a 21 cm-diameter 8 cm height polyvinyl chloride polymer collar was permanently installed 5 cm into the soil at the center of each plot for the measurement of soil respiration. We measured soil respiration by using an LI-8100 infrared gas analyzer, Li-Cor, Inc., Lincoln, NE, United States, connected to an 8100-103 soil respiration chamber. One or two days before the measurements, all living plants inside the collars were carefully clipped from the soil surface to exclude aboveground plant respiration. To eliminate diurnal variation, the measurements of soil respiration were collected between 8:00 a.m. and 12:00 p.m. (local time).
2.3 CO2 production potential
For CO2 production potential test with microcosm experiment, topsoil samples (0–10 cm) were collected at the end of the 2019–2021 growing seasons (October–November) using a manual stainlesssteel corer of 10 cm diameter. 5 g soils and 5 mL sterile water were added into a anaerobic tube with the total volume of 25 mL. Anaerobic vials were completed with three vacuum/charging cycles of high purity nitrogen, resulting in an anaerobic environment filled with high-purity nitrogen. Then the incubation was conducted at room temperature in a dark environment. The gas was measured and collected every 3 days or so to determine its gas concentration. The incubation was terminated when the gas production concentration peaked or declined. The gas samples were analyzed with a gas chromatograph (GC) (Agilent 7890A, United States) equipped with a flame ionization detector and an automated flow-injection apparatus.
2.4 Soil property analyses
Based on the analysis of CO2 production potential, the samples obtained in 2021 were used to further soil property and microbial analysis. Total carbon (TC) and total nitrogen (TN) were measured on an elemental analyzer (Vario MACRO cube, Elementar Analysensysteme, Germany). We removed total inorganic carbon with hydrochloric acid (HCl, 1 mol L−1) from the samples, and then measured total organic carbon (TOC) on the elemental analyzer. Soil carbon (C) quality was characterized by relative peak area of aliphatic and aromatic hydrocarbons, which was determined using Fourier transform infrared spectroscopy (FTIR, Nicolet iS5, Thermo Scientific). Before the determination, the air-dried soil was screened with a 300-mesh screen for small particles, and ground with an agate mortar and pestle for further homogenization. The reflection spectra of 400 ~ 4,000 cm−1 were obtained by FTIR, and the relative peak areas of different characteristic peaks were calculated. The abundance of other organic carbon functional groups can be represented by the relative peak area of corresponding characteristic peaks. In this paper, soil carbon quality can be represented by the ratio of aliphatic groups to aromatics (rA2930:rA1635 ratio), and the higher the ratio, the better the quality (Chen et al., 2022). For example, the ratio of rA2930:rA1635 is a proxy of the ratio of labile carbon (aliphatic) and not easily decomposed carbon (aromatics). Because aliphatic compounds are usually composed of straight, branched, or annular carbon atoms connected by single or multiple carbon–carbon bonds. In contrast, aromatic compounds are composed of benzene rings and their derivatives with a stable ring structure and high saturation. Therefore, aliphatic reactions are likely to be more active than aromatic reactions.
2.5 Molecular analyses of microbial community composition
We used a PowerSoil DNA Kit (Qiagen) to extract DNA. As is widely acknowledged, V4 region of the 16S rRNA gene with the primers 515F (5′-GTGYCAGCMGCCGCGGTAA-3′) and 806R (5′-GGACTACNVGGGTWTCTAAT-3′) can be used to analyze bacterial community. Similarly, the primers ITS1-F (5′-CTTGGTCATTTAGAGGAAGTAA-3′) and ITS2 (5′-GCTGCGTTCTTCATCGATGC-3′) were used to test fungal community by amplifying the internal transcribed spacer (ITS1) region. To well distinguish the samples after sequencing, barcode sequences were added to the primers. The PCR conditions for both the 16S and ITS amplification procedures can refer to Chen et al. (2022). The Illumina HiSeq platform with a 150 bp paired-end sequencing kit was used to complete amplicons sequence. K-strategy of bacterial communities are in general with fewer rrn copies, whereas those with more rrn copies are classified to a r-strategy (Roller et al., 2016). CO2 production potential under precipitation treatments might intimately rely on microbial community or the activity of certain specific microorganisms to in anaerobic environments. Therefore, metabolically active bacteria and fungal were both tested in our experiments. Only the genes with an abundance at top 10 were presented. Sequence data associated with this project have been deposited in the NCBI Short Read Archive database (Accession Number: PRJNA1060787).
2.6 Statistical analysis
Data were presented as the mean ± standard deviation of triplicate cultures. All statistical analyses were performed using Origin 2021 (Origin Lab Corporation, United States) software. A t-test was used to analyze the significance level. Significance was accepted at the p < 0.05 level of probability.
3 Results and discussion
Here, we conducted in field experiments to simulate −60, −40%, +40%, and +60% precipitation vs. ambient conditions from 2014 in the Yellow River Delta region of China. Soil respiration from a 7-year of simulation of precipitation in 2021 was tested. The following sections present results on soil CO2 production. Further, the physical and chemical properties of soils, the quantity and quality of organic carbon, and the role of microorganisms on CO2 production were discussed.
3.1 Soil CO2 production
There was no significant effect on CO2 production from precipitation sizes in 2019 (Figure 1A), possible due to an extreme rainfall this year (Li et al., 2023). In two consecutive years (2021 and 2022), however, we clearly observed that a + 40% increase of precipitation boosted CO2 production (Figures 1B,C). This stimulated influence was much stronger than that with +60%. Specially, we found a good match between soil respiration from in situ experiment (Li et al., 2023) and the microcosm experiment (Figure 1D). Therefore, we confirmed that a moderate increase of precipitation, around +40%, can stimulate a peak of soil mineralization. Furthermore, the effect of decreased precipitations on soil respiration is less obvious. Our results also further suggested an asymmetric response of soil respiration to precipitation sizes in coastal wetland (Li et al., 2021), same as some terrestrial ecosystems (Du et al., 2020; Li et al., 2020).
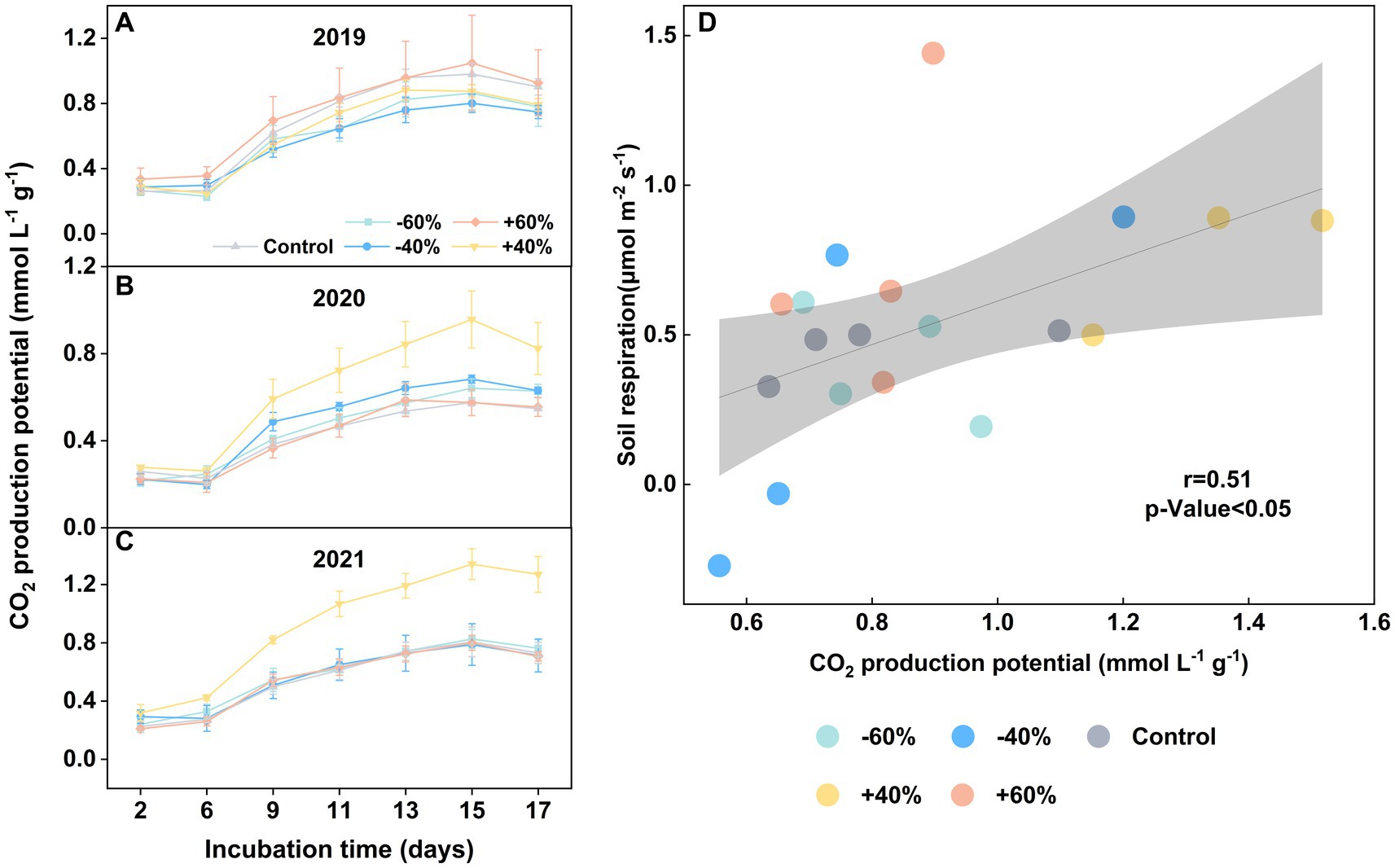
Figure 1. Variation of CO2 production potential in topsoil under precipitation treatments in 2019 ~ 2021 (A–C). CO2 correlation analysis of in situ soil respiration and laboratory incubation for samples from 2021 (D). Vertical bars indicate standard errors. “−60%” and “−40%”: 60 and 40% decreases in precipitation, respectively; “Control”: ambient precipitation; “+40%” and “+60%”: 40 and 60% increases in precipitation, respectively. Note: three replicates were used in this study in “+40%” group.
Precipitation treatment can change the physical and chemical properties of soil. Based on the FTIR spectra data (relative peak area) of the soils, the mineral composition is mainly organosilicon (Supplementary Table S1), and precipitation treatment had no obvious effect on CO2 production (p > 0.05). We found that a moderate increase in precipitation (+40%) decreased soil conductivity (Table 1). And similar results also have been found in some multi-year field manipulation experiments (Han et al., 2021; Huang et al., 2023). However, no relation between soil electric conductivity and CO2 production was discovered (p > 0.05). Moderate increase of precipitation (+40%) can lead to the increase of total nitrogen, total carbon and total plant biomass (Table 1). Precipitation may regulate maximum plant biomass accumulation through water and salt transport (Chu et al., 2021; Li et al., 2023). We also found that soil carbon and nitrogen significantly responded to precipitation size and affected soil respiration.
3.2 Organic carbon quality and quantity
Our study illustrated that a moderate increase in precipitation increased TOC content by +107.6% (Figure 2A). By contrast, the −60%, −40%, and +60% treatments had no significant difference. A positive correlation between CO2 production potential and total organic carbon content was discovered (Supplementary Figure S1A). It was suggested that increased precipitation can increase SOC stocks by reducing soil salinity (Ding et al., 2015; Sun et al., 2023). Moreover, the precipitation effect can be directly associated with an increase of net primary production of ecosystems, thus increasing organic matter deposition in soil (Singh, 2018). Carbon content of plants was improved by high precipitation (Table 1), however, we did not find a direct correlation between plant carbon content and soil respiration (p > 0.05). High soil carbon content may be regarded as a strong sign for soil respiration (Abdalla et al., 2021; Chen et al., 2023). In addition to carbon quantity, we also found that its quality also governed soil respiration (Supplementary Figure S1B). For example, treatment by +40% precipitation significantly enhanced carbon quality by 99.8% in comparison with the control (Figure 2B). Soil organic carbon and labile carbon along a precipitation gradient was also revealed along the Northeast China transect (Wang et al., 2005) and Qinghai-Tibet Plateau (Wang et al., 2022). It is reasonable that the high carbon quality facilitates soil respiration (Li et al., 2023a). In this study, we cannot rule out the effect of total nitrogen on soil respiration (Wang et al., 2019). Because a significant high of total nitrogen was found in the treatment with +40% precipitation. Therefore, as two important sources of microbial growth, carbon and nitrogen, both respond to changes in precipitation, further affecting soil respiration and CO2 emissions. For the detailed carbon components, we did not find any difference for alcohol phenols and polysaccharides (Supplementary Table S1), suggesting that these components had weak response to precipitation size.
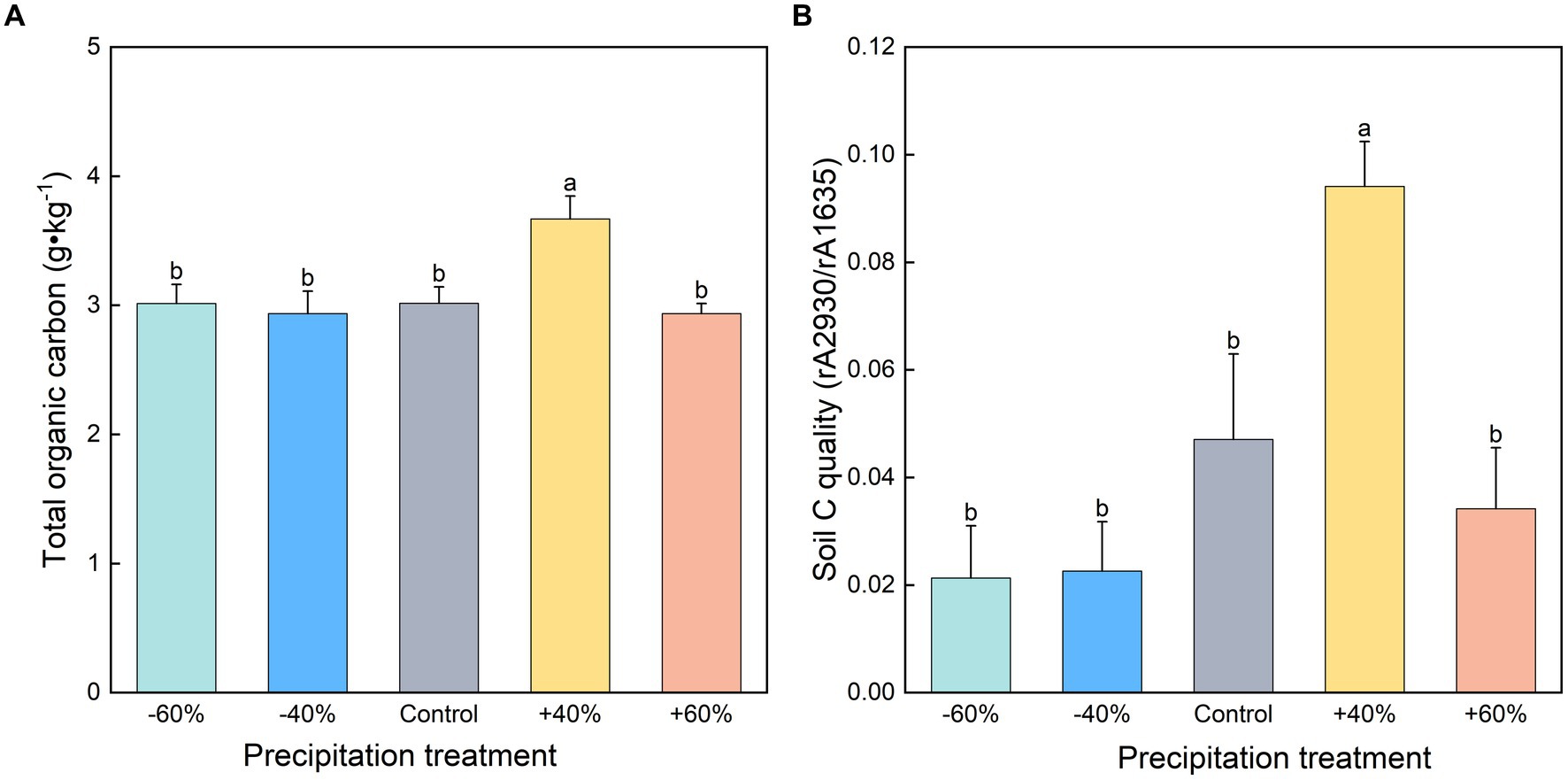
Figure 2. Soil total organic carbon (A) and carbon quality (B) of topsoil under different precipitation treatments. Different small letters indicate significant differences between precipitation treatments at p < 0. 05.
3.3 Bacterial r-/K-strategy related to CO2 production
Out of our expectation, the effect of precipitation on CO2 production potential did not depend on microbial diversity (Figures 3A,B, Supplementary Figure S2). In contrast, bacterial r-/K-selected categories were likely to explain microbial response to precipitation sizes (Figures 3C,D). It was demonstrated that bacterial communities in C-rich soils were dominated by K-strategists, while those in the comparatively C-poor soils are dominated by r-strategists (Fierer et al., 2007; Chen et al., 2022). For other extreme conditions, however, K-strategist microbes were also found in carbon-rich environments (Angel et al., 2010; Cary et al., 2010). In this study, oxygen restriction appears to be a factor affecting r-/K- strategies. Oligotrophic bacteria, such as Actinobacteriota and Chloroflexi, were likely to be survive in harsh environments than polytrophic bacteria under anaerobic conditions. Specially, Actinobacteriota and Chloroflexi microorganisms were positively correlated with CO2 production potential (Figures 3E,F). Oligotroph:copiotroph ratio was also able to use as a characterization and indication of CO2 production potential. With an obvious difference, fungal r/K-selected category was not likely to govern CO2 production (Supplementary Figure S3). Thus, bacteria may be more tolerant of anaerobic environments and actively response to precipitation size change, which will result in corresponding change of CO2 production.
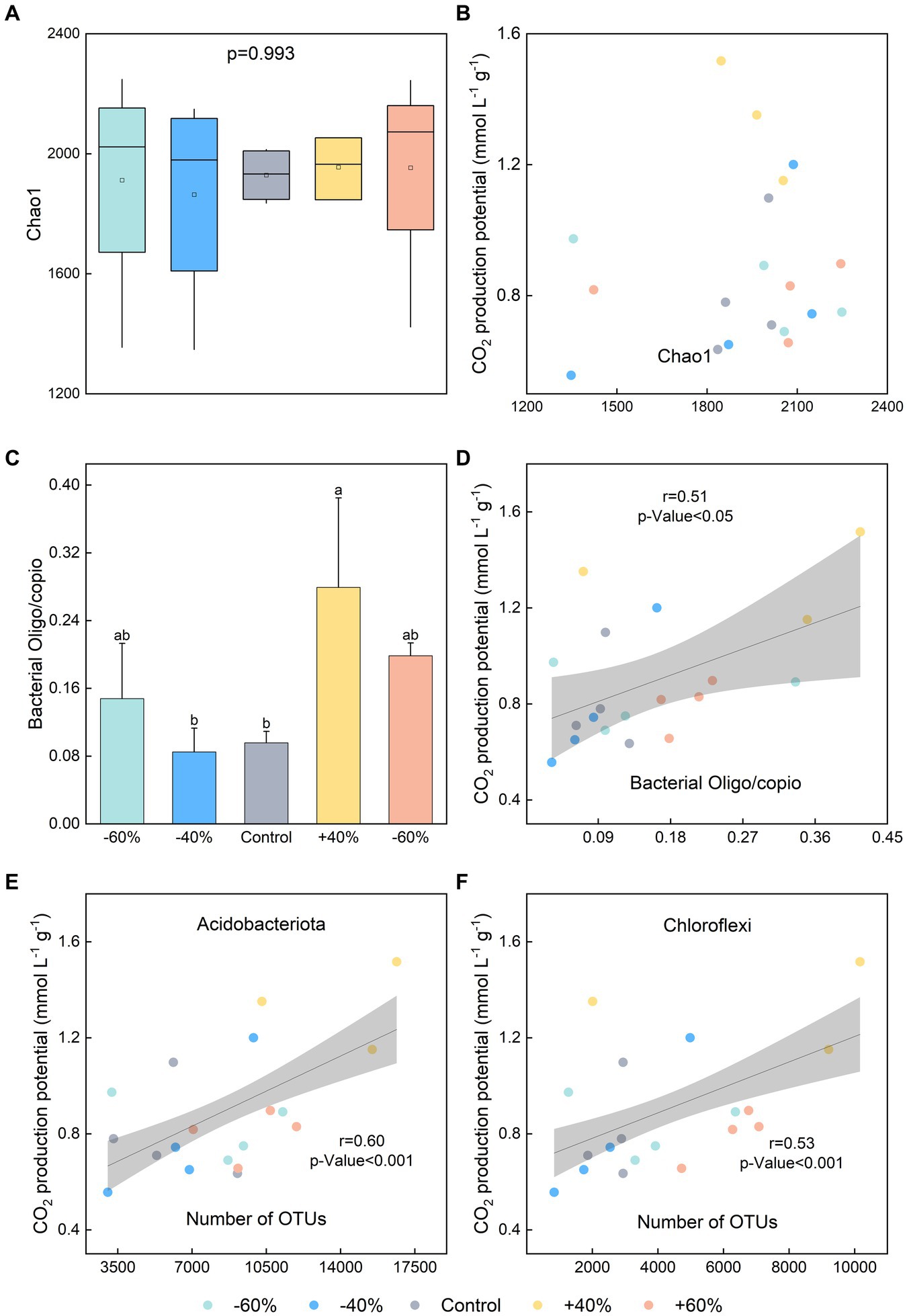
Figure 3. Effects of different precipitation sizes on soil microbial functions and specific species. (A) Soil bacterial diversity. (B) Correlation between soil bacterial diversity and CO2 production potential. (C) Oligotroph:copiotroph ratio of soil bacterial communities. (D) Correlation between oligotroph:copiotroph ratio of soil bacterial communities and CO2 production potential. (E) Correlation between the abundance of Actinobacteriota (Actinobacteria phylum) and CO2 concentration in the topsoil. (F) Correlation between the abundance of Chloroflexi (Chloroflexi phylum) and CO2 concentration. Different letters indicate significant differences between treatments, p < 0.05.
Taken together, precipitation sizes can affect soil organic carbon quality and quantity and microbial r-/K-strategies. For example, a moderate precipitation size, not a decrease or robust increase, can stimulate the strongest CO2 emission. The change of soil carbon resource is likely to regulate bacterial r-/K-strategies. Finally, soil respiration and CO2 production can show different scenario in response to precipitation size. Thus, this work proposes precipitation size affects soil microorganisms through biotic and abiotic factors, ultimately leading to differences in soil respiration and CO2 emissions.
Data availability statement
The 16S rRNA and ITS sequencing data have been deposited in the National Center for Biotechnology Information (NCBI) Short Read Archive database (SRA, https://www.ncbi.nlm.nih.gov/bioproject/PRJNA1060787).
Author contributions
LZha: Data curation, Formal analysis, Investigation, Methodology, Software, Visualization, Writing – original draft, Writing – review & editing. GH: Conceptualization, Data curation, Investigation, Supervision, Writing – original draft, Writing – review & editing. LZho: Data curation, Investigation, Writing – review & editing. XL: Data curation, Investigation, Writing – review & editing. XW: Supervision, Writing – review & editing. XZ: Supervision, Writing – review & editing. LX: Conceptualization, Data curation, Investigation, Supervision, Visualization, Writing – original draft, Writing – review & editing.
Funding
The author(s) declare financial support was received for the research, authorship, and/or publication of this article. LX was supported by Youth Innovation Promotion Association, CAS (2021213) and National Natural Science Foundation of China (42077025). GH received supports from National Natural Science Foundation of China (U2106209, 42071126). We are grateful for the support from the Yellow River Delta Ecological Research Station of Coastal Wetland, and the Chinese Academy of Sciences.
Conflict of interest
The authors declare that the research was conducted in the absence of any commercial or financial relationships that could be construed as a potential conflict of interest.
Publisher’s note
All claims expressed in this article are solely those of the authors and do not necessarily represent those of their affiliated organizations, or those of the publisher, the editors and the reviewers. Any product that may be evaluated in this article, or claim that may be made by its manufacturer, is not guaranteed or endorsed by the publisher.
Supplementary material
The Supplementary material for this article can be found online at: https://www.frontiersin.org/articles/10.3389/fmicb.2024.1328965/full#supplementary-material
Footnotes
References
Abdalla, K., Chivenge, P., Ciais, P., and Chaplot, V. (2021). Long-term (64 years) annual burning lessened soil organic carbon and nitrogen content in a humid subtropical grassland. Glob. Chang. Biol. 27, 6436–6453. doi: 10.1111/gcb.15918
Alster, C. J., German, D. P., Lu, Y., and Allison, S. D. (2013). Microbial enzymatic responses to drought and to nitrogen addition in a southern California grassland. Soil Biol. Biochem. 64, 68–79. doi: 10.1016/j.soilbio.2013.03.034
Angel, R., Soares, M. I. M., Ungar, E. D., and Gillor, O. (2010). Biogeography of soil archaea and bacteria along a steep precipitation gradient. ISME J. 4, 553–563. doi: 10.1038/ismej.2009.136
Canarini, A., Kiær, L. P., and Dijkstra, F. A. (2017). Soil carbon loss regulated by drought intensity and available substrate: A meta-analysis. Soil Biol. Biochem. 112, 90–99. doi: 10.1016/j.soilbio.2017.04.020
Cary, S. C., McDonald, I. R., Barrett, J. E., and Cowan, D. A. (2010). On the rocks: the microbiology of Antarctic Dry Valley soils. Nat. Rev. Microbiol. 8, 129–138. doi: 10.1038/nrmicro2281
Chen, Y., Du, Z., Weng, Z., Sun, K., Zhang, Y., Liu, Q., et al. (2023). Formation of soil organic carbon pool is regulated by the structure of dissolved organic matter and microbial carbon pump efficacy: A decadal study comparing different carbon management strategies. Glob. Chang. Biol. 29, 5445–5459. doi: 10.1111/gcb.16865
Chen, H., Jing, Q., Liu, X., Zhou, X., Fang, C., Li, B., et al. (2022). Microbial respiratory thermal adaptation is regulated by r-/K-strategy dominance. Ecol. Lett. 25, 2489–2499. doi: 10.1111/ele.14106
Chu, X., Han, G., Wei, S., Xing, Q., He, W., Sun, B., et al. (2021). Seasonal not annual precipitation drives 8-year variability of interannual net CO2 exchange in a salt marsh. Agric. For. Meteorol. 308:108557. Available at: https://doi.org/10.1016/j.agrformet.2021.108557
Cleveland, C. C., Wieder, W. R., Reed, S. C., and Townsend, A. R. (2010). Experimental drought in a tropical rain forest increases soil carbon dioxide losses to the atmosphere. Ecology 91, 2313–2323. doi: 10.1890/09-1582.1
Cotrufo, M. F., Soong, J. L., Horton, A. J., Campbell, E. E., Haddix, M. L., Wall, D. H., et al. (2015). Formation of soil organic matter via biochemical and physical pathways of litter mass loss. Nat. Geosci. 8, 776–779. doi: 10.1038/ngeo2520
Ding, J., Zhang, Y., Wang, M., Sun, X., Cong, J., Deng, Y. E., et al. (2015). Soil organic matter quantity and quality shape microbial community compositions of subtropical broadleaved forests. Mol. Ecol. 24, 5175–5185. doi: 10.1111/mec.13384
Du, Y., Wang, Y. P., Su, F., Jiang, J., Wang, C., Yu, M., et al. (2020). The response of soil respiration to precipitation change is asymmetric and differs between grasslands and forests. Glob. Chang. Biol. 26, 6015–6024. doi: 10.1111/gcb.15270
Fierer, N., Bradford, M. A., and Jackson, R. B. (2007). Toward an ecological classification of soil bacteria. Ecology 88, 1354–1364. doi: 10.1890/05-1839
Han, G., Chu, X., Xing, Q., Li, D., Yu, J., Luo, Y., et al. (2015). Effects of episodic flooding on the net ecosystem CO2 exchange of a supratidal wetland in the Yellow River Delta. J. Geophys. Res.-Biogeo 120, 1506–1520. doi: 10.1002/2015JG002923
Han, L., Ding, J., Zhang, J., Chen, P., Wang, J., Wang, Y., et al. (2021). Precipitation events determine the spatiotemporal distribution of playa surface salinity in arid regions: Evidence from satellite data fused via the enhanced spatial and temporal adaptive reflectance fusion model. Catena 206:105546. doi: 10.1016/j.catena.2021.105546
Han, G., Sun, B., Chu, X., Xing, Q., Song, W., and Xia, J. (2018). Precipitation events reduce soil respiration in a coastal wetland based on four-year continuous field measurements. Agric. For. Meteorol. 256, 292–303. doi: 10.1016/j.agrformet.2018.03.018
Ho, A., Di Lonardo, D. P., and Bodelier, P. L. (2017). Revisiting life strategy concepts in environmental microbial ecology. FEMS Microbiol. Ecol. 93:fix0006. doi: 10.1093/femsec/fix0006
Huang, S., Ye, G., Jie, L., Chen, K., Xia, X., Ruan, H., et al. (2018). Autotrophic and heterotrophic soil respiration responds asymmetrically to drought in a subtropical forest in the Southeast China. Soil Biol. Biochem 123, 242–249. doi: 10.1016/j.soilbio.2018.04.029
Huang, W., Chu, X., Li, P., Zhao, M., Zou, N., Wang, X., et al. (2023). Dual asymmetric response of leaf-level CO2 fixation to changes in seasonal precipitation distribution in a coastal marsh. Ecosyst. Health Sust. 9:0067. doi: 10.34133/ehs.0067
IPCC, 2021: Climate Change. (2021). The Physical Science Basis. Contribution of Working Group I to the Sixth Assessment Report of the Intergovernmental Panel on Climate Change. V. Masson-Delmotte, P. Zhai, A. Pirani, S.L. Connors, C. Péan, S. Berger, N. Caud, Y. Chen, L. Goldfarb, M.I. Gomis, M. Huang, K. Leitzell, E. Lonnoy, J.B.R. Matthews, T.K. Maycock, T. Waterfield, O. Yelekçi, R. Yu, and B. Zhou (eds.). Cambridge, United Kingdom Cambridge University Press.
Jiang, H., Deng, Q., Zhou, G., Hui, D., Zhang, D., Liu, S., et al. (2013). Responses of soil respiration and its temperature/moisture sensitivity to precipitation in three subtropical forests in southern China. Biogeosciences 10, 3963–3982. doi: 10.5194/bg-10-3963-2013
Kramer, M. G., and Chadwick, O. A. (2018). Climate-driven thresholds in reactive mineral retention of soil carbon at the global scale. Nat. Clim. Chang. 8, 1104–1108. doi: 10.1038/s41558-018-0341-4
Li, X., Han, G., Eller, F., Hui, D., Zhu, L., Chen, L., et al. (2021). Acclimation of coastal wetland vegetation to salinization results in the asymmetric response of soil respiration along an experimental precipitation gradient. Agric. For. Meteorol. 310:108626. doi: 10.1016/j.agrformet.2021.108626
Li, X., Hou, Y., Chu, X., Zhao, M., Wei, S., Song, W., et al. (2023). Ambient precipitation determines the sensitivity of soil respiration to precipitation treatments in a marsh. Glob. Chang. Biol. 29, 2301–2312. doi: 10.1111/gcb.16581
Li, C., Xiao, C., Li, M., Xu, L., and He, N. (2023). The quality and quantity of SOM determines the mineralization of recently added labile C and priming of native SOM in grazed grasslands. Geoderma 432:116385. doi: 10.1016/j.geoderma.2023.116385
Li, Y., Zhou, Z., Lei, L., Ru, J., Song, J., Zhong, M., et al. (2020). Asymmetric responses of soil respiration in three temperate steppes along a precipitation gradient in northern China revealed by soil-monolith transplanting experiment. Agric. For. Meteorol. 294:108126. doi: 10.1016/j.agrformet.2020.108126
Morris, K. A., Hornum, S., Crystal-Ornelas, R., Pennington, S. C., and Bond-Lamberty, B. (2022). Soil respiration response to simulated precipitation change depends on ecosystem type and study duration. Journal of Geophysical Research: Biogeosciences. 127 no. 11:Art. No. e2022JG006887. PNNL-SA-171042. doi: 10.1029/2022JG006887
Possinger, H. R., Bailey, S. W., Inagaki, T. M., Kögel-Knabner, I., Dynes, J. J., and Arthur, Z. A. (2020). Organo-mineral interactions and soil carbon mineralizability with variable saturation cycle frequency. Geoderma 375, 114483. doi: 10.1016/j.geoderma.2020.114483
Qin, S., Chen, L., Fang, K., Zhang, Q., Wang, J., Futing, L., et al. (2019). Temperature sensitivity of SOM decomposition governed by aggregate protection and microbial communities. Sci. Adv 5. doi: 10.1126/sciadv.aau1218
Roller, B. R., Stoddard, S. F., and Schmidt, T. M. (2016). Exploiting rRNA operon copy number to investigate bacterial reproductive strategies. Nat. Microbiol. 1, 1–7. doi: 10.1038/nmicrobiol.2016.160
Singh, B. (Ed.) (2018). Soil carbon storage: modulators, mechanisms and modeling London: Academic Press.
Su, J., Zhang, Y., and Xu, F. (2023a). Divergent responses of grassland productivity and plant diversity to intra-annual precipitation variability across climate regions: A global synthesis. J. Ecol. 111, 1921–1934. doi: 10.1111/1365-2745.14149
Su, J., Zhao, Y., and Bai, Y. (2023b). Asymmetric responses of leaf litter decomposition to precipitation changes in global terrestrial ecosystem. J. Clean. Prod. 387:135898. doi: 10.1016/j.jclepro.2023.135898
Sun, S., Song, Z., Chen, B., Wang, Y., Ran, X., Fang, Y., et al. (2023). Current and future potential soil organic carbon stocks of vegetated coastal ecosystems and their controls in the Bohai Rim Region, China. Catena 225:107023. doi: 10.1016/j.catena.2023.107023
Suseela, V., Tharayil, N., Xing, B., and Dukes, J. S. (2013). Labile compounds in plant litter reduce the sensitivity of decomposition to warming and altered precipitation. New Phytol. 200, 122–133. doi: 10.1111/nph.12376
Wang, P., Huang, K., and Hu, S. (2020). Distinct fine-root responses to precipitation changes in herbaceous and woody plants: a meta-analysis. New Phytol. 225, 1491–1499. doi: 10.1111/nph.16266
Wang, Z., Mckenna, T. P., Schellenberg, M. P., Tang, S., Zhang, Y., Ta, N., et al. (2019). Soil respiration response to alterations in precipitation and nitrogen addition in a desert steppe in northern China. Sci. Total Environ. 688, 231–242. doi: 10.1016/j.scitotenv.2019.05.419
Wang, H., Wu, J., Li, G., Yan, L., and Wei, X. (2022). Effects of rainfall frequency on soil labile carbon fractions in a wet meadow on the Qinghai-Tibet Plateau. J. Soils Sediments 22, 1489–1499. doi: 10.1007/s11368-022-03170-7
Wang, S. P., Zhou, G. S., Gao, S. H., and Guo, J. P. (2005). Soil organic carbon and labile carbon along a precipitation gradient and their responses to some environmental changes. Pedosphere 15, 676–680.
Warner, K. A., and Saros, J. E. (2019). Variable responses of dissolved organic carbon to precipitation events in boreal drinking water lakes. Water Res. 156, 315–326. doi: 10.1016/j.watres.2019.03.036
Wei, S., Han, G., Chu, X., Sun, B., Song, W., He, W., et al. (2021). Prolonged impacts of extreme precipitation events weakened annual ecosystem CO2 sink strength in a coastal wetland. Agric. For. Meteorol. 310:108655. doi: 10.1016/j.agrformet.2021.108655
Wu, F., Wang, Y., and Wang, J. (2023). Asymmetric responses of soil bacterial community and soil respiration to precipitation changes: A global meta-analysis. Land Degrad. Dev. 34, 1887–1896. doi: 10.1002/ldr.4576
Yang, Y., Li, T., Wang, Y., Cheng, H., Chang, S. X., Liang, C., et al. (2021). Negative effects of multiple global change factors on soil microbial diversity. Soil Biol. Biochem. 156:108229. doi: 10.1016/j.soilbio.2021.108229
Zhang, B., Li, W., Chen, S., Tan, X., Wang, S., Chen, M., et al. (2019). Changing precipitation exerts greater influence on soil heterotrophic than autotrophic respiration in a semiarid steppe. Agric. For. Meteorol. 271, 413–421. doi: 10.1016/j.agrformet.2019.03.019
Zhang, X., Zhang, Y., Sha, L., Wu, C., Tan, Z., Song, Q., et al. (2015). Effects of continuous drought stress on soil respiration in a tropical rainforest in southwest China. Plant Soil 394, 343–353. doi: 10.1007/s11104-015-2523-4
Zhao, M., Han, G., Wu, H., Song, W., Chu, X., Li, J., et al. (2020). Inundation depth affects ecosystem CO2 and CH4 exchange by changing plant productivity in a freshwater wetland in the Yellow River Estuary. Plant Soil 454, 87–102. doi: 10.1007/s11104-020-04612-2
Keywords: precipitation change, saltmarsh, CO2 production potential, carbon quantity and quality, microbial r-/K-strategy
Citation: Zhang L, Han G, Zhou L, Li X, Wang X, Zhang X and Xiao L (2024) Moderate increase of precipitation stimulates CO2 production by regulating soil organic carbon in a saltmarsh. Front. Microbiol. 15:1328965. doi: 10.3389/fmicb.2024.1328965
Edited by:
Ke-Qing Xiao, University of Leeds, United KingdomReviewed by:
Ming Zhang, Huazhong Agricultural University, ChinaSonglin Wu, The University of Queensland, Australia
Copyright © 2024 Zhang, Han, Zhou, Li, Wang, Zhang and Xiao. This is an open-access article distributed under the terms of the Creative Commons Attribution License (CC BY). The use, distribution or reproduction in other forums is permitted, provided the original author(s) and the copyright owner(s) are credited and that the original publication in this journal is cited, in accordance with accepted academic practice. No use, distribution or reproduction is permitted which does not comply with these terms.
*Correspondence: Leilei Xiao, llxiao@yic.ac.cn