- 1INSERM, Université Paris Cité, Sorbonne Université, Centre de Recherche des Cordeliers, Paris, France
- 2Service de Microbiologie, Hôpital Européen Georges Pompidou, AP-HP Centre, Université Paris Cité, Paris, France
- 3Institut Mutualiste Montsouris (IMM), Service de Microbiology, Paris, France
- 4Université Paris-Saclay, UVSQ, Inserm, Infection et Inflammation, Montigny-le-Bretonneux, France
- 5Institute for Integrative Biology of the Cell (I2BC), CEA, CNRS, Université Paris-Saclay, Gif-sur-Yvette, France
Six genes encoding putative high molecular weight penicillin-binding proteins (Pbp) are present in the genome of the β-lactam-resistant strain Corynebacterium jeikeium K411. In this study, we show that pbp2c, one of these six genes, is present in resistant strains of Corynebacteriaceae but absent from sensitive strains. The molecular study of the pbp2c locus from C. jeikeium and its heterologous expression in Corynebacterium glutamicum allowed us to show that Pbp2c confers high levels of β-lactam resistance to the host and is under the control of a β-lactam-induced regulatory system encoded by two adjacent genes, jk0410 and jk0411. The detection of this inducible resistance may require up to 48 h of incubation, particularly in Corynebacterium amycolatum. Finally, the Pbp2c-expressing strains studied were resistant to all the β-lactam antibiotics tested, including carbapenems, ceftaroline, and ceftobiprole.
Introduction
The genus Corynebacterium includes bacteria with very different lifestyles and metabolic abilities (Funke et al., 1997). Certain species, such as Corynebacterium diphtheriae, are human pathogens and others opportunistic pathogens, whereas Corynebacterium glutamicum, a non-pathogenic species, is of great biotechnological importance for the industrial production of amino acids (Hermann, 2003; Liu et al., 2023). Among opportunistic pathogens, Corynebacterium jeikeium and Corynebacterium urealyticum are components of human skin flora. C. jeikeium belong to the lipophilic Corynebacteriaceae sub-family and is a nosocomial pathogen commonly involved in catheter infections, prosthetic endocarditis (Rezaei Bookani et al., 2018; Gupta et al., 2021), periprosthetic joint infections (Seutz et al., 2023), and sepsis (Bernard, 2012), whereas C. urealyticum is mostly involved in urinary tract infections, such as alkaline cystitis (Soriano et al., 1985), granulomatous mastitis (Lu et al., 2023), and post-kidney transplant pyelitis (Soriano and Tauch, 2008). The diagnosis and treatment of Corynebacteriaceae can be complicated by the inability to distinguish colonization from contamination and infection, and by the high prevalence of multidrug resistance in clinical isolates, especially to β-lactams, the most widely used class of antibiotics (Soriano et al., 1995; Fernandez-Roblas et al., 2009; Bluth et al., 2015). For example, the minimal inhibitory concentration (MIC) for penicillin has increased from 0.025 to over 256 mg/L for the most resistant Corynebacterium species and strains (Fernandez-Roblas et al., 2009).
The general cell envelope architecture and synthesis in Corynebacteriaceae is well documented (Burkovski, 2013; Levefaudes et al., 2015). Even if some minor differences exist between different species, the cell envelope is an atypic multilayered one. This envelope is made, from the inner part to the outer part, of a cytoplasmic membrane, a peptidoglycan layer, a connecting glycan layer and an external mycomembrane. The cytoplasmic membrane is an asymetric phospholipid bilayer mainly composed of phosphatidylglycerol and phosphatidylinositol, with palmitic acid (16:0) and decanoic acid (18:1) as dominating fatty acids. Lipomannan and lipoarabinomannan polymers are covalently bound to phospholipids from the outer sheet of the phospholipid bilayer and anchored in the murein sacculus. Overlaying this cytoplasmic membrane is a thin peptidoglycan of the A1γ type, with a glycan part made of alternating β-1,4-linked N-acetylglucosamine and N-acetyl muramic acid units. Cross-linking of glycan polymers occur via direct peptide bounds, without interpeptide bridges. The major peptides found in mature peptidoglycan in Corynebacteriaceae are the tetrapeptide L-Ala-γ-D-Glu-meso-DAP-D-Ala and the tripeptide L-Ala-γ-D-Glu-meso-DAP. In C. jeikeium, the α-carbonyl group of D-Glu and the ε-carbonyl group of meso-DAP residues are partially amidated (Lavollay et al., 2009; Levefaudes et al., 2015).
In Corynebacteriaceae, peptide bridges occur either between meso-DAP and D-Ala (4–3 cross-links) or between two meso-DAP residues (3–3 cross-links), indicating the participation of D,D- and/or L,D-transpeptidases. In C. jeikeium, final steps of peptidoglycan reticulation is mediated by five high molecular weight class A and B penicillin binding proteins (Pbp), responsible for 62% of cross links and two L,D-transpeptidases (Ldt) responsible of the remaining 38% cross links (Lavollay et al., 2009).
The outer part of the envelope in Corynebacteriaceae, with the noticeable exception of C. amycolatum, is made of an additional barrier, the mycomembrane. In Corynebacteriaceae, peptidoglycan is connected to the mycomembrane by complex glycan polymers. These polymers are made of arabinogalactan (D-arabinose and D-galactose containing multimers), attached to linker units made of galactose, rhamnose and N-acetylglucosamine, linker units and arabinogalactan are covalently attached to N-acetyl muramic acid. The inner sheet of mycomembrane is made of corynomycolic acids (circa 30 carbon atoms) covalently linked to arabinogalactan and free corynomycolic acids. The outer sheet of the mycomembrane is mainly made of trehalosyl mono- or di-mycolates. Last, in some Corynebacteriaceae, an outer crystalline surface layer composed of a single protein could exist (Burkovski, 2013).
A crucial step in envelope formation is the transpeptidation step of murein. A failure of transpeptidation leads to cell disruption and cell death due to the internal osmotic pressure. Even if Pbp and Ldt proteins catalyze the formation of similar amide bonds, they are not structurally related and use different substrates. Pbp are serine active enzymes that use the disaccharide-L-Ala-γ-D-Glu-meso-DAP-D-Ala-D-Ala-pentapeptide as the substrate while Ldt are cysteine active enzymes using the disaccharide-L-Ala-γ-D-Glu-meso-DAP-D-Ala-tetrapetide as the susbtrate. Although L,D-transpeptidases are ampicillin-insensitive cross-linking enzymes (Mainardi et al., 2007; Lavollay et al., 2009), they are unlikely to directly contribute to the resistance to this antibiotic in C. jeikeium, the formation of their tetrapeptide substrate being dependent of the low molecular weight Pbp D,D-carboxypeptidase (Pbp4Cjk), inactivated by ampicillin (Lavollay et al., 2009). The C. jeikeium β-lactam resistance may also result from other factors such as the presence of β-lactamases, low affinity Pbp, or the impermeability of the cell wall. In this study, we investigated the putative role of two genes from C. jeikeium (jk0411 and jk0412), only found in strains with significant β-lactam resistance and encoding two proteins with strong analogies to β-lactamases and Pbp, respectively. Six genes encoding putative high-molecular-mass Pbp were previously identified in the genome of the C. jeikeium K411 resistant strain (Lavollay et al., 2009). Only one of these genes, jk0412, predicted to encode a high-molecular class B Pbp, was found in the genome of C. jeikeium resistant strains but absent in susceptible strains (Lavollay et al., 2009).
To directly show the contribution of pbp2c to β-lactams resistance in C. jeikeium, the deletion of the pbp2c gene in C. jeikeium would have been the ideal method. Unfortunately, this was not possible. Although genetic tools for gene deletion in C. glutamicum have been published (Schafer et al., 1994; Hu et al., 2013) they could not be applied to C. jeikeium because of several technical limitations, such as no highly efficient transformation system available (Barzantny et al., 2013), no possibility to use the counter selecting sacB gene for pK18mobsac-mediated edition (Dusch et al., 1999), because of the lack of any PTS system (Tauch et al., 2005), no replicative plasmid in C. jeikeium, with a thermosensitive origin of replication for CRE/LOX edition (Hu et al., 2013). Moreover, C. jeikeium is resistant to a wide range of antibiotics, with MIC over 128 μg/mL for the antibiotics commonly used in molecular biology, namely kanamycin, tetracycline, chloramphenicol, and ampicillin (Rosato et al., 2001). Therefore, because of all these limitations, we decided to study the potential implication in β-lactam resistance of the pbp2c gene from C. jeikeium in a heterologous system based on C. glutamicum, a species taxonomically close to C. jeikeium, highly sensitive to most of β-lactams, and for which all the molecular tools needed for this study are available and functional.
We also report here that all resistant C. jeikeium strains tested possess two additional genes, jk0410 and jk0411, located upstream of the pbp2c gene, and that, one or both, could be involved in the regulation of the expression of pbp2c. Studying the effect of various β-lactams on the induction phenomena highlighted that current European Committee on Antimicrobial Susceptibility Testing (EUCAST) recommendations for detecting β-lactam resistant strains, which do not take into account such inducible resistance, could lead to poorly adapted choices for detection and treatment of infections due to Corynebacteriaceae.
Materials and methods
Bacterial strains and growth conditions
The bacterial strains used in this study are listed in Supplementary Table S1. Escherichia coli and C. glutamicum were grown in Brain Heart Infusion media (BHI, Difco) at 37°C under agitation (180 rpm). Reference strains C. jeikeium CIP82.51 (CjkS), CIP103337 (CjkR), and C. urealyticum DSM7109 (CuR) were grown in BHI medium supplemented with 0.5% Tween 80 (Sigma-Aldrich). Antibiotics used for selection were ampicillin (Ap) at 100 μg/mL (Euromedex, Souffelweyersheim, France), kanamycin (Km) at 50 μg/mL (Duchefa, Haarlem, The Netherlands), and chloramphenicol (Cm) at 30 μg/mL for E. coli and 10 μg/mL for C. glutamicum (Duchefa).
Susceptibility testing
Reference strains and clinical isolates from invasive specimens (hemocultures, deep purulence) were studied in accordance to the recommendations of the Antibiogram Committee of the French Society of Microbiology Version 2.02019 (CA-SFM) using the disk diffusion, broth microdilution, and E-test methods.
For the disk diffusion assay, susceptibility testing was performed on Mueller-Hinton agar supplemented with 5% defibrinated horse blood and 20 mg/L β-NAD (MH-F, BioMérieux, La Balme-Les-Grottes, France) with an inoculum of 0.5 McFarland units. Plates were incubated under 5% CO2 at 35 ± 2°C. The inhibition zones were determined after 24 h of incubation. If a reduction (>2 mm) of the inhibition zone was detected after an additional incubation of 24 h, the diameters observed at 48 h are indicated in brackets (Table 1). Antibiotic disks were purchased from Oxoid (Basingstoke, England) or prepared extemporaneously by loading 10 μg potassium clavulanate (Sigma-Aldrich) onto 6-mm disks (Dutscher, Brumath, France).
Broth microdilution assays were performed according to CA-SFM V 2.02019 guidelines in cation-adjusted Müller-Hinton II broth (BD-Difco, Le Pont-de-Claix, France) supplemented with 5% lysed defibrinated horse blood (Oxoid, Basingstoke, England) and 20 mg/L β-NAD (Sigma-Aldrich). The inoculum containing 5 × 105 CFU/mL in a fresh medium was prepared from an overnight 10 mL preculture under agitation. 96-well plates containing 100 μL were incubated aerobically at 35 ± 2°C for 24 and 48 h. The microtiter plates contained twofold serial dilutions of penicillin G (Panpharma, Boulogne-Billancourt, France), meropenem (AstraZeneca, Rueil-Malmaison, France), or potassium clavulanate (Sigma-Aldrich) from 1,024 to 0.0625 μg/mL. The MICs of penicillin G and meropenem were also determined in combination with a fixed concentration (10 μg/mL) of potassium clavulanate.
E-test assays were performed on MH-F medium according to the recommendations of the manufacturer of the antibiotic-containing strips. Benzylpenicillin, amoxicillin, imipenem, and meropenem MIC Test Strips were provided by BioMérieux (Craponne, France). Ceftaroline and ceftobiprole MIC Test Strips were provided by Liofilchem (Roseto degli Abruzzi, Italy). Benzylpenicillin and meropenem were also tested in association with clavulanate (10 μg/mL) which was added in the media. Plates were incubated under 5% CO2 at 35 ± 2°C for 24 and 48 h.
Preparation of genomic DNA from Corynebacteriaceae
Bacteria from a 24 h culture (2 mL) were harvested by centrifugation (7,500 ×g for 3 min at 4°C), resuspended in 1 mL 25 mM Tris-Cl (pH 8.5) containing 10 mM EDTA, 1% glucose, and 20 mg/mL lysozyme, and incubated at 37°C for 2 h. DNA was extracted using the Wizard Genomic DNA Purification Kit (Promega, Charbonnières-les-Bains, France) or an Ingenius apparatus (ELITechGroup, Puteaux, France) according to the manufacturers’ instructions.
Detection of pbp2c by polymerase chain reaction
The primers used for pbp2c detection are described in Supplementary Table S2. The detection of pbp2c by PCR was carried out in 20 μL of a reaction mixture containing 1 μL genomic DNA extracted from the bacteria, 200 μM of each dNTP, 24 pmol of each primer, 2 units of VENT DNA polymerase (NEB, Evry, France), and 2 μL 10× Thermopol buffer (NEB). The reaction was carried out for 35 cycles according to the following program: denaturation at 95°C for 30 s and annealing and primer extension at 72°C for 90 s. After PCR amplification, 5 μL of the reaction mixture was loaded onto a 1.0% agarose gel containing ethidium bromide (0.5 μg/mL). After electrophoresis, the band of amplified DNA (1,401 bp) was visualized under UV light (254 nm).
Heterologous expression of pbp2c genes in Corynebacterium glutamicum
The pbp2c genes from C. jeikeium (jk0412) and C. urealyticum (cu1571) were amplified using the primers Fwjk0412_1-20 L and Revjk0412_1782–1762 (Supplementary Table S2) and cloned into the vector pBlunt to give pBluntΩjk0412 and pBluntΩcu1571, respectively. The pbp2c genes were subcloned into the vector pET2818 using NcoI and XhoI (NEB) (pET2818Ωjk0412 and pET2818Ωcu1571). The trc promoter (Ptrc) from plasmid pKK388-1 (Brosius, 1988) was amplified using the primers Ptrc_Fw_4980 and Ptrc_Rev_353 and cloned into pBlunt. A 438-bp NcoI-EcoRV fragment from the resulting plasmid pBluntΩPtrc was cloned upstream of the pbp2c genes of pET2818Ωjk0412 and pET2818Ωcu1571 to generate the pET2818ΩPtrc-jk0412 and pET2818ΩPtrc-cu1571 plasmids. For the latter cloning step, pET2818 derivatives were digested by BglII, treated with the Klenow fragment of DNA polymerase I (NEB), and digested with NcoI. The plasmids pET2818ΩPtrc-jk0412 and pET2818ΩPtrc-cu1571 were digested with BamHI and XhoI and the 2,119-bp fragment carrying the Ptrc-pbp2c fusions cloned into the E. coli - C. glutamicum shuttle vector pMM36 (Merkamm et al., 2003). The resulting plasmids pMM36ΩPtrc-jk0412 and pMM36ΩPtrc-cu1571 (Supplementary Table S3) were introduced by electro-transformation into C. glutamicum RES167 as previously described (Bonamy et al., 1990).
Production and purification of recombinant proteins
A fragment of the pbp2c gene from C. jeikeium K411 (jk0412) encoding Pbp2c without the N-terminal transmembrane segment (residues 32–593) was amplified using the primers Fwjk0412_93-113S and Revjk0412_1782-1762 (Supplementary Table S2) and cloned into the vector pET-TEV using the NdeI and XhoI restriction sites (underlined in Supplementary Table S2). pET-TEV is a modified version of pET28a in which the thrombin cleavage site has been replaced by a TEV cleavage site and the T7 tag deleted (Supplementary Table S3) (Houben et al., 2007). The resulting plasmid pET-TEVΩjk0412 encodes the Pbp2c protein with a six-histidine tag at its N-terminus followed by a TEV cleavage site (MGSSHHHHHHSSGENLYFQGHM). Expression of the protein was carried out in E. coli BL21(DE3) harboring pET-TEVΩjk0412. The bacteria were grown at 37°C in three liters of BHI broth supplemented with kanamycin (50 μg/mL). Expression was induced at 16°C for 17 h by adding 0.5 mM isopropyl-β-D-thio-galactopyranoside (IPTG) when the OD600 reached 0.7. The His-tagged Pbp2c protein was purified from a clarified lysate by metal affinity and size exclusion chromatography. Briefly, the crude extract from a 3 L culture of E. coli BL21(DE3) harboring pET-TEVΩjk0412 was clarified by centrifugation (16,600 ×g for 30 min at 4°C). The His-tagged-Pbp2c protein was purified from the clarified lysate by affinity chromatography on Ni2+-nitrilotriacetate-agarose resin (Qiagen GmbH, Hilden, Germany) and elution with 25 mM Tris-Cl (pH 7.5) containing 300 mM NaCl and imidazole at 50, 100, and 250 mM. His-tagged-Pbp2c-containing fractions were identified by 12% SDS-PAGE, pooled, and loaded onto a SuperDex® 200 HL 26/60 column (Cytiva, Uppsala, Sweden) equilibrated with 25 mM Tris-Cl (pH 7.5) containing 300 mM NaCl. The His-tagged-Pbp2c protein was eluted as a monomer according to the calibration curve of the column. The concentrated protein was stored at −20°C in 25 mM Tris-Cl buffer (pH 7.5) containing 300 mM NaCl and complemented with 50% glycerol (v/v). Protein purity was assessed by 12% SDS-PAGE and analyzed by electrospray mass spectroscopy in the positive mode after buffer exchange on a Hitrap®-Desalting column (Cytiva) previously equilibrated in MES buffer (100 mM, pH 6.4). The observed mass of His-tagged-Pbp2c was in agreement with the mass deduced from the Pbp2c sequence without the N-terminal residue Met (calculated: 62,974 Da; observed: 62,975 Da).
The jk0411 gene from C. jeikeium K411 encoding BlaCor was amplified using the primers jk0411_Fw_XbaI and jk0411_Rev_NotI (Supplementary Table S2) and cloned into the pET-TEV as an XbaI-NotI DNA fragment (restriction sites underlined in Supplementary Table S2). Mutagenesis of the jk0411 gene to obtain BlaCor S45A was performed using the QuikChange® site-directed Mutagenesis kit according to the user’s manual (Agilent Technologies). Expression and purification of BlaCor and BlaCor S45A were performed as described for the His-tagged-Pbp2c protein. The observed masses of BlaCor and BlaCor S45A were in agreement with the masses deduced from the protein sequences without the N-terminal residue Met (32,825 and 32,809 Da, respectively).
Growth curves
Corynebacteriaceae species and strains were cultivated overnight in 10 mL BHI-Tween 80 (0.5%) medium in the presence (induced) or absence (uninduced) of a sub-inhibitory concentration (20 μg/mL) of ampicillin or imipenem. Cultures were diluted to an OD600 of 0.1 in the same media (50 mL) and incubated at 37°C with shaking. When the OD600 reached 0.4, meropenem was added to each culture to a final concentration of 32 μg/mL and the OD600 were monitored for an additional 26 h duration.
Detection of Pbp2c-β-lactam adducts by electrospray mass spectrometry
The formation of enzyme-drug adducts between purified Pbp2c and various β-lactams were tested by incubating the protein (20 μM) with a 10-molar excess (200 μM) of antibiotics (amoxicillin, cefoxitin, imipenem, meropenem, ampicillin) for 1 h at 20°C in MES buffer at pH 6.4 (100 mM) and were stored at −20°C prior to mass spectrometry analysis. Protein and protein-β-lactam adduct masses were detected by electrospray mass spectrometry as previously described (Mainardi et al., 2007). Briefly, 5 μL of each reaction mixture was mixed extemporaneously with 5 μL acetonitrile and 1 μL 1% formic acid. The mixture was injected directly into the mass spectrometer (Qstar Pulsar I, Applied Biosystems) in positive mode at a flow rate of 50 μL/min using a buffer containing acetonitrile (50%) and formic acid (0.5%).
Preparation of protein extracts
Bacteria were grown aerobically for 24 h in BHI medium supplemented with 0.5% Tween 80, harvested by centrifugation and resuspended in 100 mM Tris-Cl buffer (pH 7.0) to a turbidity equivalent to 25 OD600 units. This experiment was also performed using the same medium, but containing ampicillin (20 μg/mL) or clavulanate (10 μg/mL). Bacteria were successively washed, suspended in 100 mM Tris-Cl buffer (pH 7.0), and lysed by FastPrep with 0.17 μm glass beads (3 × 30 s, Speed 6.5; QBIOgene, Illkirch, France). Protein concentrations were determined using the Bio-Rad Bradford protein assay according to the manufacturer’s instructions, with bovine serum albumin as the standard.
Mouse anti-Pbp2c antibodies
Purified His-tagged-Pbp2c was subcutaneously injected into five BALB/c mice (Janvier, France) (20 μg per mouse) with incomplete Freund’s adjuvant (1/1, v/v) on days 1, 28, and 57. One week after days 28 and 57, blood samples were obtained from the retroorbital plexus, centrifuged, and stored at −20°C until use. Mouse experiments were performed according to institutional and national ethical guidelines (Agreement n°783,223; approved by the Ministry of Higher Education and Research with APAFIS#11465-2016111417574906v4).
Western blot analysis
Proteins from crude extracts (10 μg) were separated on 15% SDS-PAGE, transferred to a nitrocellulose membrane (Hybond, Amersham Biosciences, Little Chalfont, United Kingdom), and incubated with mouse anti-Pbp2c antiserum (1/5,000). Nitrocellulose membranes were incubated in 10 mM Tris-Cl buffer pH 7.5 containing 150 mM NaCl, 0.025% Tween 20, and 2.5% nonfat dry milk for 1 h at 4°C. Peroxidase-conjugated goat anti-mouse antibodies (Life Technologies, Saint-Aubin, France; 1/6,000) were used as secondary antibodies. Pbp2c-antibodies complexes were detected by chemiluminescence (Amersham ECL Western Blotting Detection Kit, Cytiva) using a film exposure time of 5 min. Purified His-tagged-Pbp2c (3 ng) was used as the positive control.
β-Lactamase activity
β-lactamase activity was assessed by following the hydrolysis of the chromogenic β-lactamase substrate nitrocefin. The assay mixture contained 500 μM nitrocefin (Sigma-Aldrich) and 50 μg protein extract in a final volume of 50 μL of 10 mM phosphate buffer (pH 6.5). Hydrolysis of nitrocefin was monitored spectrophotometrically at 486 nm for 30 min at room temperature (Δε = 15,200 M−1 cm−1). Protein extracts of C. jeikeium CIP82.51 and Mycobacterium chelonae #2445 (generous gift from B. Heym, Institut Pasteur, France) were used as negative and positive controls, respectively.
Results
Heterologous expression of the Corynebacterium jeikeium and Corynebacterium urealyticum pbp2c genes in Corynebacterium glutamicum induce β-lactam resistance
Our first objective was to determine whether the production of Pbp2c was sufficient for β-lactam resistance in a sensitive Corynebacterium host. To obtain Pbp2c production, the pbp2c-encoding genes from C. jeikeium (jk0412) and C. urealyticum (cu1571) were cloned under the control of the trc promoter (Ptrc) into E. coli prior to transfer into the sensitive C. glutamicum strain RES167 using the shuttle transfer plasmid pMM36 (Merkamm et al., 2003). The sensitivity of Corynebacterium strains was determined by disk diffusion assay (Table 1), microdilution assay (Table 2), and E-Test assay (Table 3). The C. glutamicum RES167 strain does not contain any class B Pbp-encoding gene related to pbp2c (Lavollay et al., 2009) and, according to the PK/PD non species related breakpoints of the CA-SFM V2.0 2019, is sensitive to all tested β-lactam antibiotics, with the exception of aztreonam, ceftazidime, and temocillin, known to be inactive against Gram-positive bacteria (Table 1). To ensure that Pbp2c production did not affect the C. glutamicum growth, due to a potential toxicity, we compared the growth of C. glutamicum strains #3278 and #3279 harboring either the empty vector pMM36 or the pMM36ΩPtrc-jk0412 vector (pML2), respectively. No significant difference was observed between these two strains (Supplementary Figure S1). The activity of drugs belonging to glycopeptides, aminoglycosides, fluoroquinolones, macrolides and related, tetracycline and chloramphenicol was not altered by expression of the pbp2c genes (Table 1). Although the introduction of the empty pMM36 vector (Supplementary Table S3) did not alter the sensitivity of RES167 to antibiotics (Strain #3278 in Table 1), constitutive expression of the pbp2c genes from C. jeikeium and C. urealyticum in C. glutamicum resulted in large decreases (at least 12 mm) in the diameter of the inhibition zones for all active β-lactams, including representatives of the penicillin, cephalosporin, and carbapenem classes (Table 1, Strain #3279 and #3322). Determination of the MIC of a subset of β-lactams (Table 2) showed that introducing the gene encoding Pbp2c from either C. jeikeium or C. urealitycum into C. glutamicum increased the MIC to levels equivalent to those observed for resistant strains of C. jeikeium (CjkR or K411) or C. urealyticum (DSM7109). A similar set of experiments was also performed using the E-test® system. In solid medium, the MIC were slightly different and resistance was underestimated (Supplementary Table S4).
Overall, these results showed that expression of the pbp2c genes from C. jeikeium and C. urealyticum confered a broad-spectrum resistance to β-lactams in a sensitive host.
Induction of β-lactam resistance by clavulanate and ampicillin in Corynebacterium jeikeium
A wide range of β-lactam antibiotics have been tested in order to study the activity of the different classes and to determine if the resistance mechanism could be inducible (Table 1; Figure 1). Comparison of the antibiograms of C. jeikeium CIP82.51 (CjkS) (Figure 1A) and C. jeikeium CIP103337 (CjkR) (Figures 1B,C) showed that inhibition zones persisted despite the presence of the pbp2c gene in the resistant strain around disks containing cefoxitin (disk 1, 30 μg), ceftaroline (disk 7, 5 μg), cefepime (disk 12, 30 μg), meropenem (disk 14, 10 μg), and ertapenem (disk 16, 10 μg). We observed antagonism between clavulanate (disk 13, 10 μg) and meropenem (disk 14, 10 μg). We observed such antagonism in the double disk diffusion assay, which showed the typical “D” shape blunting of the inhibition zone around the disk containing meropenem in the vicinity of disks containing clavulanate (Figures 2A,B) or ampicillin (Figures 2C,D). This observation suggested that clavulanate induced resistance to meropenem. Thus, we performed an antibiogram of C. jeikeium CIP103337 (CjkR) in a clavulanate-containing medium (Figure 1D). In the presence of clavulanate, the strain grew in contact with all β-lactam containing disks, suggesting that cefoxitin (disk 1, 30 μg), ceftaroline (disk 7, 5 μg), cefepime (disk 12, 30 μg), meropenem (disk 14, 10 μg), and ertapenem (disk 16, 10 μg) remained partially active against the CjkR strain because they were unable to completely induce β-lactam resistance in 24 h (Figure 1B) and even 48 h for ertapenem (Figure 1C). Thus, a 48 h incubation period was necessary for a correct interpretation of antibiograms and evaluation of resistance to ceftaroline, cefepime, meropenem, and imipenem. For ertapenem, a 48 h incubation was not sufficient and the induction with clavulanate in the media was needed to correctly detect resistant strains.
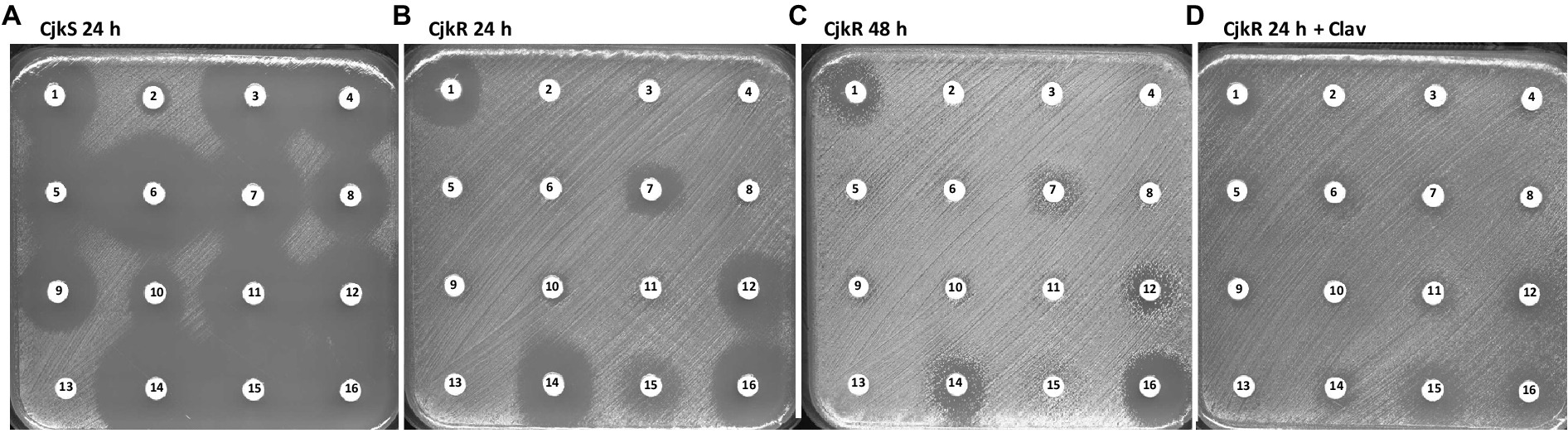
Figure 1. Antibiogram by the disk diffusion assay performed on MH-F agar for (A) Corynebacterium jeikeium strains CIP82.51 (CjkS), (B) CIP103337 (CjkR) incubated 24 h, (C) CIP103337 (CjkR) incubated 48 h, and (D) CIP103337 (CjkR) cultivated in the presence of clavulanate (Clav) at 10 μg/mL and incubated 24 h. Disks contain 1: cefoxitin (30 μg), 2: benzylpenicillin (1 U), 3: ticarcillin (75 μg), 4: ampicillin (10 μg), 5: cefalexin (30 μg), 6: amoxicillin/clavulanate (20 μg/ 10 μg), 7: ceftaroline (5 μg), 8: piperacillin (30 μg), 9: piperacillin/tazobactam (30 μg/6 μg), 10: cefotaxime (5 μg), 11: ticarcillin/clavulanate (75 μg/10 μg), 12: cefepime (30 μg), 13: clavulanate (10 μg), 14: meropenem (10 μg), 15: imipenem (10 μg), or 16: ertapenem (10 μg).
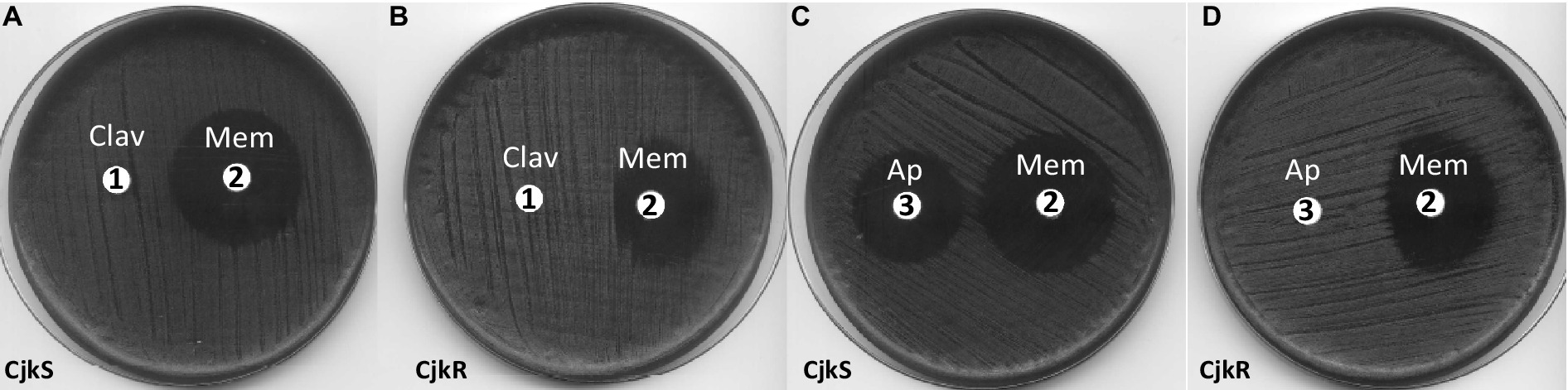
Figure 2. Double disk diffusion assay for C. jeikeium strains CIP82.51 (CjkS) (A,C) and CIP103337 (CjkR) (B,D). Plates were incubated for 24 h on MH-F agar. Disk 1: clavulanate (Clav, 10 μg), disk 2: meropenem (Mem, 10 μg), disk 3: ampicillin (Ap, 10 μg).
To distinguish between inducible resistance, which requires a 24 h prolonged incubation, and standard bacterial growth under subinhibitory antibiotic conditions, we performed experiments in the presence of high inhibitory concentrations of meropenem (32 and 128 μg/mL). We also tested the induction of β-lactam resistance by following growth in the presence or absence of an inducer (Figure 3). We used ampicillin as the inducer because this antibiotic produced the same “D-shape” blunting of the inhibition zone of meropenem as clavulanate does (Figure 2C). From an overnight culture diluted to 0.1 OD600 in fresh medium containing no inducer, the addition of meropenem (32 μg/mL) after 4 h of incubation inhibited growth of C. jeikeium K411 (Figure 3, white triangle). In contrast, prior growth of the strain in the presence of a sub-inhibitory concentration of ampicillin (20 μg/mL) for 4 h completely prevented inhibition by meropenem (Figure 3, black triangle).
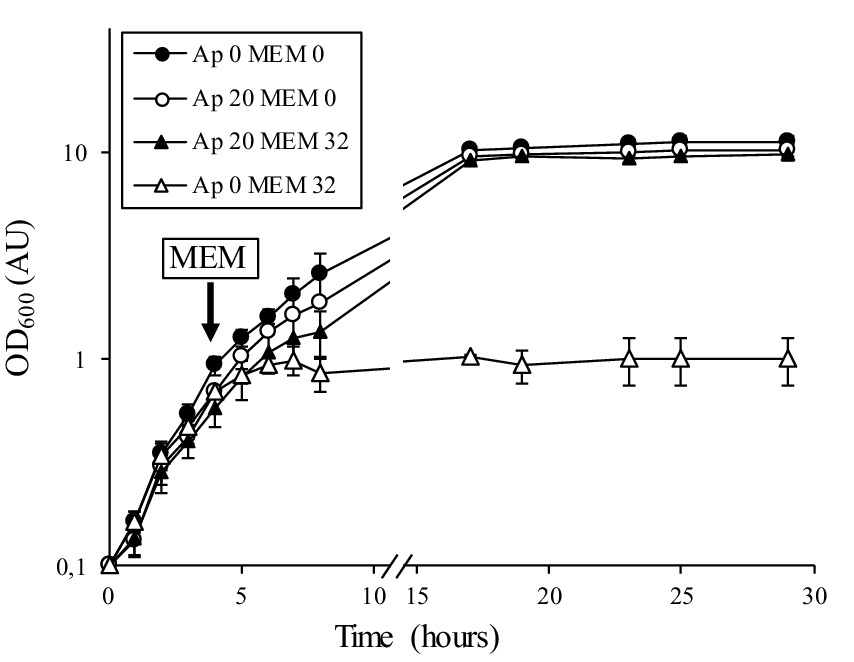
Figure 3. Growth curves of C. jeikeium K411 in the absence or presence of meropenem. An overnight culture of strain K411 diluted to 0.1 OD600 was grown in the absence (Ap 0) or presence (Ap 20) of ampicillin at 20 μg/mL for 4 h (OD600 of approximately 0.4). Meropenem (32 μg/mL, MEM 32) was added to the indicated cultures. OD600 values are the mean ± standard error from three determinations.
We tested induction of the resistance profile by various β-lactam and non-β-lactam antibiotics when performing experiments during which a high inhibitory concentration of meropenem (128 μg/mL) was added to the agar plate medium and the potential inducers loaded onto the disk (Figure 4). In these experiments, inducible resistance was mediated by most β-lactams, not by non-β-lactam antibiotics. Ampicillin (disk 1, 10 μg), oxacillin (disk 2, 5 μg), benzylpenicillin (disk 10, 10 U), amoxicillin/clavulanate (disk 12, 20/10 μg), and imipenem (disk 19, 10 μg) were strong inducers of meropenem resistance, whereas cephalothin (disk 4, 30 μg) and amoxicillin alone (disk 11, 25 μg) appeared to be weaker inducers.
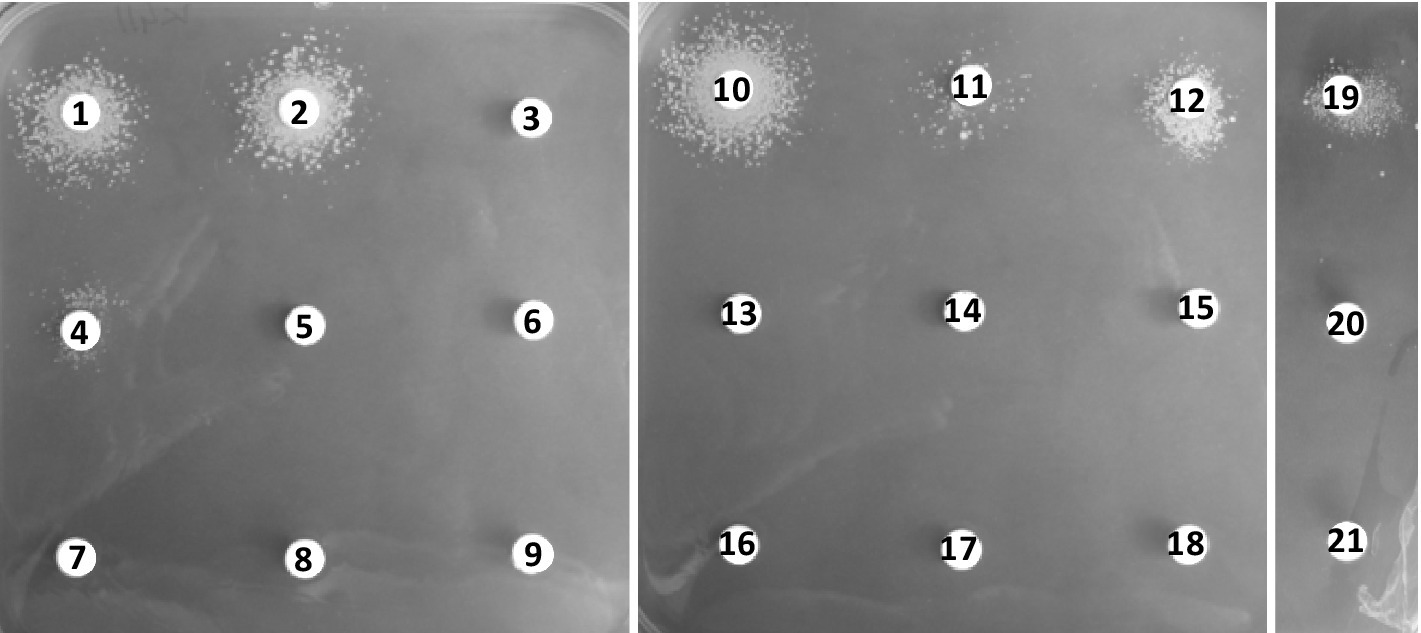
Figure 4. Induction of meropenem resistance by the disk diffusion assay for strain C. jeikeium K411. Plates were incubated for 48 h. The assays were performed on BHI agar containing 0.5% Tween 80 and meropenem at 128 μg/mL. The disks contained 1: ampicillin (10 μg), 2: oxacillin (5 μg), 3: piperacillin (30 μg), 4: cephalothin (30 μg), 5: cefoxitin (30 μg), 6: ceftazidime (30 μg), 7: cefepime (30 μg), 8: colistin (30 μg), 9: chloramphenicol (30 μg), 10: benzylpenicillin (10 U), 11: amoxicillin (25 μg), 12: amoxicillin/clavulanate (20 μg/10 μg), 13: gentamicin (10 μg), 14: erythromycin (15 μg), 15: norfloxacin (10 μg), 16: vancomycin (5 μg), 17: teicoplanin (30 μg), 18: tetracycline (30 μg), 19: imipenem (10 μg), 20: meropenem (10 μg), or 21: ertapenem (10 μg).
Induction of Pbp2c production by clavulanate and ampicillin
We used immunodetection of Pbp2c to determine whether the induction of β-lactam resistance by clavulanate and ampicillin resulted from increased synthesis of Pbp2c in response to these drugs. Bacteria were cultivated under agitation during 24 h in liquid media in the absence or the presence of antibiotics. A polyclonal anti-Pbp2c mouse antiserum was used for Western blot analyses of C. jeikeium protein extracts and revealed a protein band of ca. 63 kDa for C. jeikeium K411 and CIP103337 (CjkR) whith the pbp2c gene (Figure 5). This protein band migrated at an equivalent position as did the purified Pbp2c and was not detectable in protein extract from C. jeikeium CIP82.51 (CjkS) which lacks pbp2c. These results indicated that the mouse antiserum was specific to Pbp2c. The intensity of the signal corresponding to the Pbp2c protein was strongly enhanced in the protein extracts from cultures performed in the presence of ampicillin (Figure 5A) or clavulanate (Figure 5B) but barely detectable in the absence of these drugs.
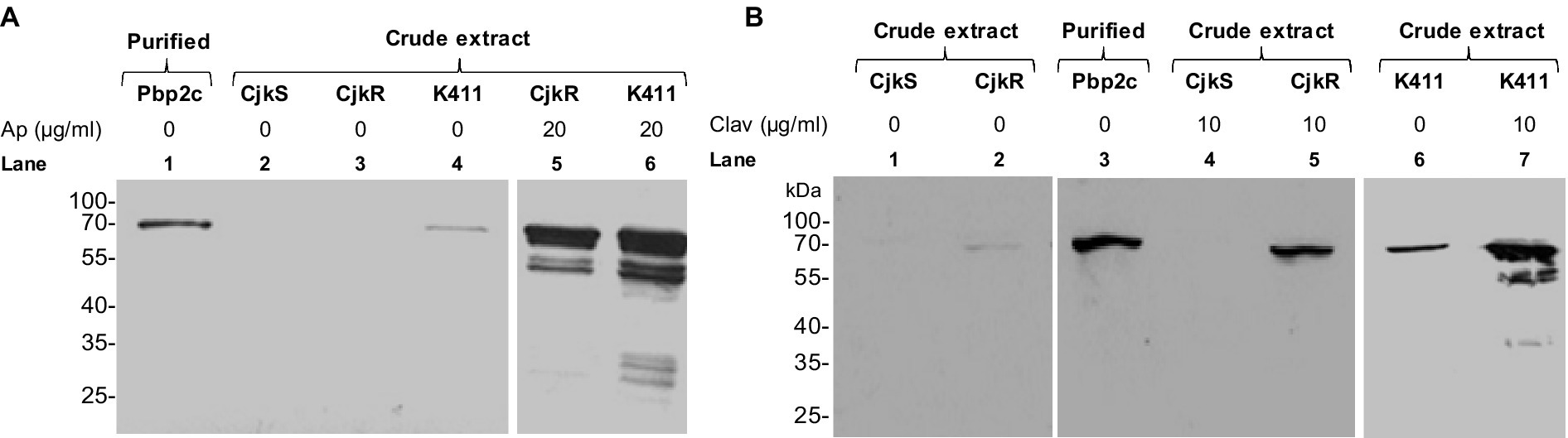
Figure 5. Impact of ampicillin (A) or clavulanate (B) on the level of Pbp2c production. C. jeikeium strains were grown for 24 h in the presence of ampicillin (Ap) or clavulanate (Clav). Crude extracts (10 μg) were analyzed by Western blotting with polyclonal antibodies raised against the Pbp2c protein encoded by jk0412. The extracts were prepared from strain C. jeikeium CIP82.51 (CjkS), a β-lactam-sensitive strain that does not harbor pbp2c, and from the β-lactam resistant strains CIP103337 (CjkR) and K411. Purified Pbp2c (3 ng) was used as a control.
Inactivation of Pbp2c by the formation of adducts with β-lactams
We explored the inactivation of Pbp2c by β-lactams by investigating the formation of adducts in the presence of various β-lactams. Binding of the drugs to the enzyme was tested by electrospray mass spectrometry, as previously described (Mainardi et al., 2007). In these experiments (Figure 6), we detected no adducts between Pbp2c and ampicillin, cefoxitin, or amoxicillin. Incubation of Pbp2c with imipenem allowed the detection of adducts with an average mass matching the addition of the mass of imipenem, but at a low level relative to the signal of the free protein. Adducts matching increments of the average mass of meropenem were also detected but only at a very low level.
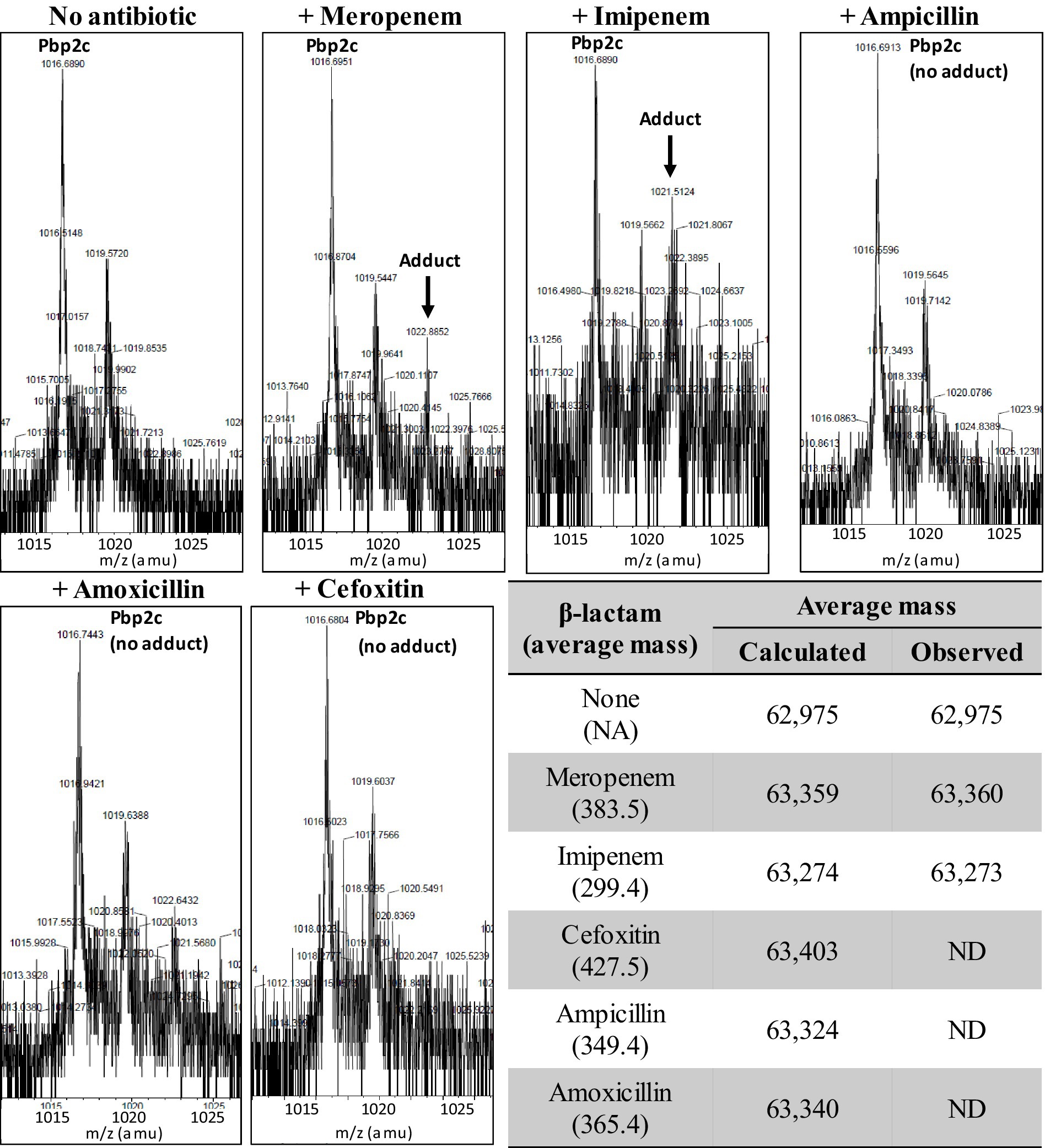
Figure 6. Formation of adducts between Pbp2c and β-lactams. Pbp2c was incubated without or with antibiotics. Peaks at m/z 1,016.7 and 1,019.6 correspond to the [M + 62H]62+ of the native protein (deduced mass average of 62,975 Da) and a spontaneous α-N-6-phosphogluconoylation of the poly histidine tag (Geoghegan et al., 1999). ND: not detected.
β-Lactamase activity
In order to investigate how the level of β-lactam resistance was the result of the presence of an unidentified β-lactamase or not, we tested the presence of a β-lactamase activity on bacterial extracts from C. jeikeium K411 and CjkR, cultivated with or without β-lactam (amoxicillin and/or clavulanate), from CjkS, from the C. glutamicum RES167 strains transformed with either pML1, pML2, pML3, in the purified Pbp2c protein extract, and from M. chelonae as a positive control. With the exception of the M. chelonae positive control, no β-lactam hydrolysis was detected, indicating that the observed resistance was due to the overproduction of a low-affinity Pbp and not from hydrolysis of the β-lactam antibiotics.
Distribution of the Pbp2c-encoding gene among clinical isolates of Corynebacteriaceae
We then investigated the presence of the gene coding for Pbp2c in medical settings. The gene has been detected by PCR using the primer pair Pbp2c_PCR_Fw and Pbp2c_PCR_Rev (Supplementary Table S2), which yielded a 1,401-bp fragment (Supplementary Figure S2) containing the SxxK and SxN catalytic domains of Pbp2c and the positions corresponding to V361 and S372, which were found to be substituted in CjkR-4, a highly resistant strain derived from CjkR against ampicillin (Lavollay et al., 2009). We systematically found the pbp2c gene in strains with a β-lactam-resistance phenotype, whereas it was absent from sensitive isolates of C. jeikeium or other Corynebacteria species (Table 4).
Structure of the pbp2c locus
In order to understand the origin of the pbp2c gene, we carried out in silico genome analyses of Corynebacteriaceae species with jk0412 gene analogs. Sequence comparisons and analyses showed that the pbp2c gene found in Corynebacteriaceae was always associated with the presence of two additional genes, jk0410 and jk0411, namely, not detected in the pbp2c-negative strains. These two genes, located upstream of jk0412 and transcribed in the same orientation (Figure 7A), have the same G/C content as jk0412. This content was found to be 10% lower than the G/C content of the host strains genomes (Figure 7B). This substantial divergence in the G/C content suggests a common and exogenous origin for the locus containing these three genes (referred here as to the pbp2c locus).
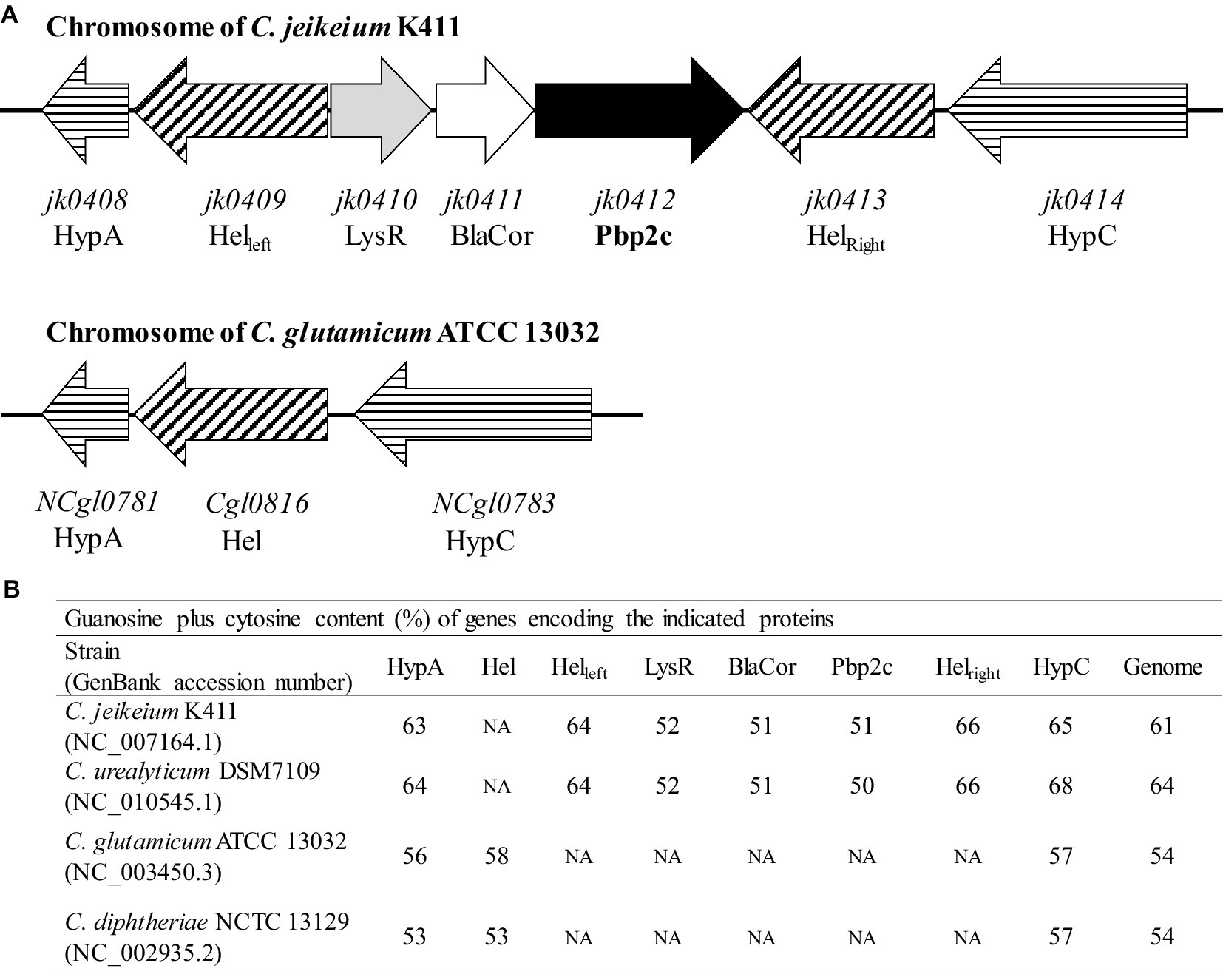
Figure 7. Schematic organization and G + C content of the pbp2c locus. (A) Map of the pbp2c locus in C. jeikeium K411 and comparison with the homologous region of the C. glutamicum ATCC 13032 locus. (B) G + C content of relevant genes and comparison with that of whole genome.
The jk0410 and jk0411 genes are involved in the inducible regulation of Pbp2c production
Similarity analyses of the jk0410 and jk0411 gene products using the protein BLAST program disclosed homology with regulatory proteins of the transcriptional regulator LysR family and the class A β-lactamase family, respectively. We explored the role of the conserved jk0410 and jk0411 genes in the regulation of Pbp2c expression by producing additional constructs (Figure 8A). The first construct, pML1, contained the complete gene set (jk0410, jk0411, and jk0412) incorporated into pMM36. The pMM36 plasmid containing the gene encoding Pbp2c (jk0412) under the control of the exogenous Ptrc promoter was designated pML2. Finally, the pMM36 plasmid construct containing the pbp2c gene alone (jk0412), without the Ptrc promoter, was designated pML3. Each of the three plasmids pML1, pML2, and pML3, were introduced into C. glutamicum RES167. C. glutamicum strains containing either pML1, pML2 or pML3 were cultivated overnight in 10 mL of medium without inducer. Each culture was diluted in fresh culture medium until DO600 reached 0.1 and was cultivated for 24 h in the presence or absence of the inducer (clavulanate, 1 μg/mL). Total proteins were extracted and a Western blot experiment was performed to detect the expression of the jk0412 gene using anti-Pbp2c antibodies (Figure 8B). In the presence of clavulanate, Pbp2c was detected only when bacteria were transformed with pML1(the complete jk0410, jk0411, jk0412 gene cluster). The Pbp2c protein was not detected in the absence of the jk0410 and jk0411 genes or without the Ptrc promoter (pML3), independently of whether the bacteria were grown in the presence or absence of clavulanate. Last, the Pbp2c production was restored by introducing the pb2c gene under the control of the Ptrc promoter, in the presence or absence of clavulanate in the medium.
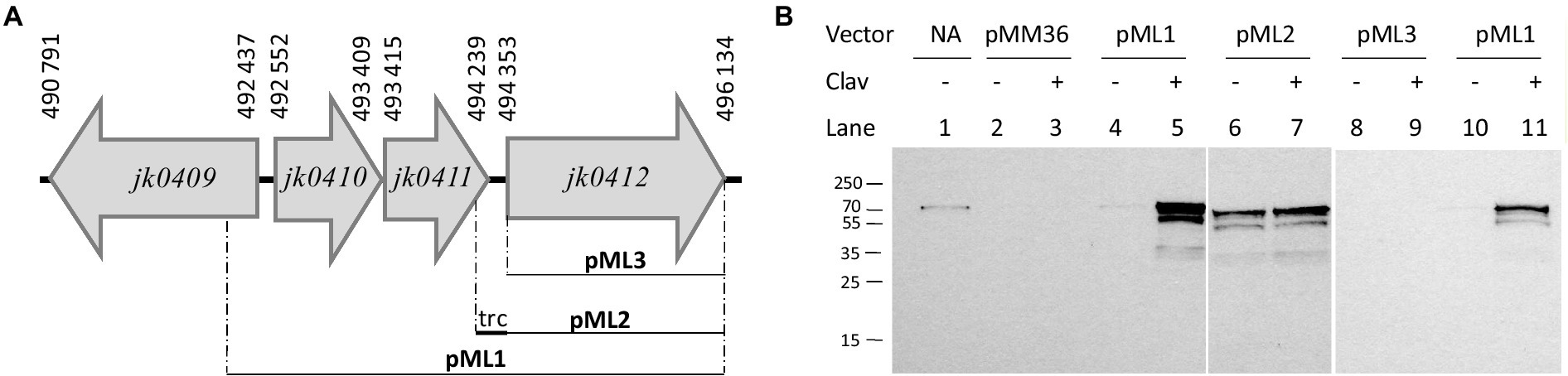
Figure 8. (A) Schematic representation of the constructs pML1, pML2, and pLM3. (B) Western blot of total proteins extracted from C. glutamicum RES167 transformed with pML1, pML2, or pML3. Strains were cultivated overnight without antibiotics, diluted to an OD600 of 0.1 in fresh BHI media complemented (+) or not (−) with clavulanate (clav, 1 μg/mL), and cultivated under agitation for 24 h. Protein extracts (10 μg) were analyzed by Western blotting with polyclonal antibodies raised against the Pbp2c protein encoded by jk0412. Line 1: purified Pbp2C; Line 2–3: RES167 harboring pMM36 (#3278); Line 4–5 and 10–11: RES167 harboring pMM36Ωjk0410-jk0411-jk0412 (#3815); Line 6–7: RES167 harboring pMM36ΩPtrc-jk0412 (#3279); Line 8–9: RES167 harboring pMM36Ωjk0412 (#3812).
Putative roles of the proteins encoded by the jk0410 and jk0411 genes
The conserved motives SxxK, SDx and KTG found in β-lactamases were systematically searched in proteins encoded by the C. jeikeium K411 genome. Twelve genes were identified that could encode proteins with β-lactamase/transpeptidase-like domains (IPR012338). Seven of these genes encode previously identified proteins: Pbp1a (jk2069), Pbp1b (jk1977), Pbp2a (jk0039), Pbp2b (jk1160), Pbp2c (jk0412), FtsI (jk0744), and Pbp4 (jk075) (Lavollay et al., 2009). Out of the five other genes, namely jk0411, jk0584, jk0658, jk1553 and jk2026, only jk0411 encodes a protein with all the three conserved β-lactamase/transpeptidase-like motives (Supplementary Figure S3).
The jk0411 gene encodes a 276 amino acid protein, hereafter referred to as BlaCor. The amino acid sequence of BlaCor display the three amino acid motifs SxxK at positions 45 to 48, SDN at positions 106 to 108, and KTG at positions 220 to 222. The sequence of was also compared with the constitutive β-lactamase BlaC from M. tuberculosis (Supplementary Figure S3). Although the structural alignment (RMS of 9.5 Å) of the AlpahaFold models for BlaCor (AF-Q4JX89) and BlaC (PDB code 6 N14) seemingly indicate that the three conserved domains are positioned in BlaCor as in BlaC (data not shown), BlaCor is 39 amino acids shorter than BlaC, an organization that could contribute to the inability of BlaCor to hydrolyze β-lactams. To assess the ability of BlaCor to hydrolyze β-lactams, the protein in fusion with a 6His tag at the N-terminus position of BlaCor was purified from crude extracts of E. coli expressing jk0411. Size-exclusion chromatography and mass spectroscopy indicated that BlaCor was purified as a monomer. Incubation of purified BlaCor with clavulanate resulted in the formation of a covalent +155 Da trans-enamine adduct (Hugonnet and Blanchard, 2007) which was detected by mass spectrometry (Supplementary Figure S4). A Ser to Ala substitution at position 45 of BlaCor, corresponding to the catalytic Ser residue in Pbp and β-lactamases, abolished the binding of β-lactams to BlaCor (Supplementary Figure S4) indicating that BlaCor is a bona fide Pbp. The incubation of BlaCor with ampicillin resulted in the formation of a covalent +349 Da adduct which was also detected by mass spectrometry (Supplementary Figure S4). To determine whether BlaCor could act as a β-lactamase, the protein was incubated with the chromogenic cephalosporin nitrocefin. Even if the nitrocefin-BlaCor adduct was detected by mass spectrometry (data not shown), incubation with nitrocefin (100 μM) with several concentrations of BlaCor (0, 5, 10, 15, and 20 μM) did not result in nitrocefin hydrolysis. All together these results indicate the absence of β-lactamase activity for this enzyme.
Discussion
Considerable variations in the sensitivity of Corynebacteriaceae species and strains against β-lactams were reported (Soriano et al., 1995). Several species were experimentally shown to be sensitive to penicillin and ampicillin (e.g., C. diphteriae, Corynebacterium pseudotuberculosis, Corynebacterium ulcerans, and Corynebacterium pseudodiphteriticum) (Funke et al., 1997), whereas others have shown low sensitivity to β-lactams. Resistance mechanisms were almost unknown at the beginning of this study (Soriano et al., 1995; Funke et al., 1996; Weiss et al., 1996; Funke et al., 1997).
The pbp2c gene was previously found to be present in the resistant C. jeikeium CIP103337 strain genome (CjkR) but absent in the sensitive C. jeikeium CIP82.51 strain genome (CjkS), suggesting that Pbp2c could be one determinant of the β-lactam resistance observed in C. jeikeium CIP103337 (Lavollay et al., 2009). Here, we showed that the heterologous expression of the Pbp2c-encoding-gene from C. jeikeium CIP103337 in C. glutamicum RES167 confered a β-lactam resistance to this sensitive species and strengthen the role of Pbp2c as a potent, key determinant, of β-lactam resistance in multidrug-resistant C. jeikeium and C. urealyticum. We found that the jk0412 gene, encoding Pbp2c, was present in the genomes of β-lactam-resistant Corynebacterium species, such as C. amycolatum, Corynebacterium tuberculostearicum, and Corynebacterium striatum, (Table 4) and was recently shown to be present in a mobile element in C. diphtheriae (Hennart et al., 2020). The nucleotide sequence of pbp2c was found to be highly conserved, with an identity value ≥99% in multidrug-resistant Corynebacteriaceae species (Hennart et al., 2020). These results highlight the use of a pbp2c-based screening method as a powerful diagnostic tool to improve the detection of infections and the management of patients infected with Corynebacteriaceae.
We tested the induction of β-lactam resistance using several C. glutamicum strains: one carrying the pbp2c gene (jk0412) alone, one with the gene under the control of the P trc promoter, and one in combination with the jk0410 and jk0411 genes (complete pbp2c locus). We showed that jk0412 is one of the main gene involved in high levels of β-lactam resistance in Corynebacteriaceae. We also showed that Pbp2c expression was under the control of the jk0410 and/or jk411 genes which encode for a LysR analog, likely a transcriptional regulator and the β-lactamase analogue (BlaCor), respectively. We showed that BlaCor was able to covalently link β-lactams, but was unable to hydrolyze these compounds. These results suggested that the formation of stable, covalent bonds between BlaCor and β-lactam products could play a role in Pbp2c expression by a mechanism yet to be discovered. We found that the induction was modulated by the β-lactam used. Ampicillin, oxacillin, benzylpenicillin, and imipenem were strong inducers, whereas cefoxitin, ceftaroline, cefepime, meropenem, and ertapenem were weaker inducers. We also found that clavulanate, a β-lactamase inhibitor of the β-lactam family but without antibacterial activity, was also a strong inducer. These differences in resistance induction raised concerns about the protocol for the correct detection of resistant strains of Corynebacteriaceae. In clinical practice, current EUCAST (EUCAST Clinical Breakpoint Tables v. 14.0, 2024)1 and CA-SFM (V1.0 2023) guidelines recommend testing Corynebacteriaceae using benzylpenicillin with a breakpoint at 1 μg/mL and 0.125 μg/mL, respectively (Table 3). As the MIC of this molecule for sensitive strains is 2 μg/mL (Table 2), some strains from the Corynebacteriaceae family could be misclassified as resistant to all β-lactam antibiotics.
We also observed that testing sensitivity to ertapenem or meropenem using a classical 24 or 48 h incubation protocol also failed to reliably detect pbp2c-mediated resistant strains because the induction phenomenon occurred too slowly to be detected under these conditions. A straightforward, rapid, and easily implementable procedure in medical laboratory environments can overcome such drawbacks. This procedure, based on the E-Test strip superimposition method, described for the study of sensitivity to the ceftazidime-avibactam-aztreonam association (Davido et al., 2017), requires pre-incubation of an agar plate for 10 min with an E-Test that contains a powerful inducer (e.g., an amoxicillin-clavulanate association), the removal of the first strip, and its substitution with a new strip containing the carbapenem of interest (ertapenem or meropenem). The result of this E-test revealed the true sensitivity of the strain, whether or not it possesses the gene encoding the Pbp2c protein (Supplementary Figure S5).
In conclusion, this study provides an improved understanding of the mechanism of β-lactam resistance in Corynebacteriaceae. We showed that resistance was mediated by a low affinity Pbp protein (Pbp2c) and not by the acquisition of a β-lactamase-encoding gene. Detection of Corynebacteriaceae strains harboring the pbp2c gene could therefore contribute to improve the management of patients with complex infections implying Corynebacteriaceae. To highly notice, this would make possible avoiding the use of second-line antibiotics, which can expose patients to side effects, such as renal failure with vancomycin (Lu et al., 2023), or the emergence of highly resistant strains with daptomycin (McElvania TeKippe et al., 2014; Mitchell et al., 2021).
Data availability statement
The raw data supporting the conclusions of this article will be made available by the authors, without undue reservation.
Ethics statement
The animal study was approved by institutional and national ethical guidelines (Agreement n°783223; approved by the French Ministry of Higher Education and Research with APAFIS#11465-2016111417574906v4). The study was conducted in accordance with the local legislation and institutional requirements.
Author contributions
ML: Conceptualization, Formal analysis, Investigation, Methodology, Resources, Supervision, Validation, Writing – original draft, Writing – review & editing. CB: Investigation, Methodology, Writing – original draft. VM: Writing – original draft, Investigation. FC: Writing – review & editing. AG: Resources, Writing – review & editing. MF: Writing – review & editing, Writing – original draft, Validation, Methodology, Investigation, Formal analysis.
Funding
The author(s) declare that no financial support was received for the research, authorship, and/or publication of this article.
Acknowledgments
This work was supported by the University Paris-cité, Sorbonne University, and INSERM, Paris, France.
Conflict of interest
The authors declare that the research was conducted in the absence of any commercial or financial relationships that could be construed as a potential conflict of interest.
Publisher’s note
All claims expressed in this article are solely those of the authors and do not necessarily represent those of their affiliated organizations, or those of the publisher, the editors and the reviewers. Any product that may be evaluated in this article, or claim that may be made by its manufacturer, is not guaranteed or endorsed by the publisher.
Supplementary material
The Supplementary material for this article can be found online at: https://www.frontiersin.org/articles/10.3389/fmicb.2024.1327723/full#supplementary-material
Footnotes
References
Barzantny, H., Guttmann, S., Lässig, C., Brune, I., and Tauch, A. (2013). Transcriptional control of lipid metabolism by the MARR -like regulator FAMR and the global regulator GLXR in the lipophilic axilla isolate Corynebacterium jeikeium K 411. Microbial Biotechnol. 6, 118–130. doi: 10.1111/1751-7915.12004
Bernard, K. (2012). The genus Corynebacterium and other medically relevant Coryneform-like Bacteria. J. Clin. Microbiol. 50, 3152–3158. doi: 10.1128/JCM.00796-12
Bluth, M. H., Salem, N., Salem, L., Saber, S., and Ismail, G. A. (2015). Corynebacterium urealyticum: a comprehensive review of an understated organism. Infect Drug Res. 8, 129–145. doi: 10.2147/IDR.S74795
Bonamy, C., Guyonvarch, A., Reyes, O., David, F., and Leblon, G. (1990). Interspecies electro-transformation in Corynebacteria. FEMS Microbiol. Lett. 66, 263–269. doi: 10.1111/j.1574-6968.1990.tb04008.x
Brosius, J. (1988). Expression vectors employing lambda-, trp-, lac-, and lpp-derived promoters. Biotechnology 10, 205–225. doi: 10.1016/B978-0-409-90042-2.50016-7
Burkovski, A. (2013). Cell envelope of Corynebacteria: structure and influence on pathogenicity. ISRN. Microbiology 2013, 1–11. doi: 10.1155/2013/935736
Davido, B., Fellous, L., Lawrence, C., Maxime, V., Rottman, M., and Dinh, A. (2017). Ceftazidime-avibactam and Aztreonam, an interesting strategy to overcome β-lactam resistance conferred by Metallo β-lactamases in Enterobacteriaceae and Pseudomonas aeruginosa. Antimicrob. Agents Chemother. 61, e01008–e01017. doi: 10.1128/AAC.01008-17
Dusch, N., Pühler, A., and Kalinowski, J. (1999). Expression of the Corynebacterium glutamicum panD gene encoding L-aspartate-α-decarboxylase leads to Pantothenate overproduction in Escherichia coli. Appl. Environ. Microbiol. 65, 1530–1539. doi: 10.1128/AEM.65.4.1530-1539.1999
Fernandez-Roblas, R., Adames, H., Martín-de-Hijas, N. Z., García Almeida, D., Gadea, I., and Esteban, J. (2009). In vitro activity of tigecycline and 10 other antimicrobials against clinical isolates of the genus Corynebacterium. Intern J Antimicrobial Agents. 33, 453–455. doi: 10.1016/j.ijantimicag.2008.11.001
Funke, G., Graevenitz, A. V., Iii, J. E. C., and Bernard, K. A. (1997). Clinical microbiology of coryneform bacteria. Clin. Microbiol. Rev. 10, 125–159. doi: 10.1128/CMR.10.1.125
Funke, G., Pünter, V., and Von Graevenitz, A. (1996). Antimicrobial susceptibility patterns of some recently established coryneform bacteria. Antimicrob. Agents Chemother. 40, 2874–2878. doi: 10.1128/AAC.40.12.2874
Geoghegan, K. F., Dixon, H. B. F., Rosner, P. J., Hoth, L. R., Lanzetti, A. J., Borzilleri, K. A., et al. (1999). Spontaneous α-N-6-Phosphogluconoylation of a “his tag” in Escherichia coli: the cause of extra mass of 258 or 178 Da in fusion proteins. Anal. Biochem. 267, 169–184. doi: 10.1006/abio.1998.2990
Gupta, R., Popli, T., Ranchal, P., Khosla, J., Aronow, W., Frishman, W., et al. (2021). Corynebacterium Jeikeium endocarditis: a review of the literature. Cardiol. Rev. 29, 259–262. doi: 10.1097/CRD.0000000000000355
Hennart, M., Panunzi, L. G., Rodrigues, C., Gaday, Q., Baines, S. L., Barros-Pinkelnig, M., et al. (2020). Population genomics and antimicrobial resistance in Corynebacterium diphtheriae. Genome Med. 12:107. doi: 10.1186/s13073-020-00805-7
Hermann, T. (2003). Industrial production of amino acids by coryneform bacteria. J. Biotechnol. 104, 155–172. doi: 10.1016/S0168-1656(03)00149-4
Houben, K., Marion, D., Tarbouriech, N., Ruigrok, R. W. H., and Blanchard, L. (2007). Interaction of the C-terminal domains of Sendai virus N and P proteins: comparison of polymerase-Nucleocapsid interactions within the paramyxovirus family. J. Virol. 81, 6807–6816. doi: 10.1128/JVI.00338-07
Hu, J., Tan, Y., Li, Y., Hu, X., Xu, D., and Wang, X. (2013). Construction and application of an efficient multiple-gene-deletion system in Corynebacterium glutamicum. Plasmid 70, 303–313. doi: 10.1016/j.plasmid.2013.07.001
Hugonnet, J. E., and Blanchard, J. S. (2007). Irreversible inhibition of the Mycobacterium tuberculosis β-lactamase by Clavulanate. Biochemistry 46, 11998–12004. doi: 10.1021/bi701506h
Lavollay, M., Arthur, M., Fourgeaud, M., Dubost, L., Marie, A., Riegel, P., et al. (2009). The β‐lactam‐sensitived,d‐carboxypeptidase activity of Pbp4 controls thel,dandd,dtranspeptidation pathways Incorynebacterium jeikeium. Mol. Microbiol. 74, 650–661. doi: 10.1111/j.1365-2958.2009.06887.x
Levefaudes, M., Patin, D., De Sousa-d’Auria, C., Chami, M., Blanot, D., Hervé, M., et al. (2015). Diaminopimelic acid amidation in corynebacteriales. J. Biol. Chem. 290, 13079–13094. doi: 10.1074/jbc.M115.642843
Liu, J., Ou, Y., Xu, J. Z., Rao, Z. M., and Zhang, W. G. (2023). L-lysine production by systems metabolic engineering of an NADPH auto-regulated Corynebacterium glutamicum. Bioresour. Technol. 387:129701. doi: 10.1016/j.biortech.2023.129701
Lu, C., Marcucci, V., Kibbe, E., and Patel, R. (2023). Granulomatous mastitis secondary to Corynebacterium requiring surgical intervention: a complicated diagnosis. J Surg Case Reports. 2023, 1–3. doi: 10.1093/jscr/rjad211
Mainardi, J. L., Hugonnet, J. E., Rusconi, F., Fourgeaud, M., Dubost, L., Moumi, A. N., et al. (2007). Unexpected inhibition of peptidoglycan LD-Transpeptidase from Enterococcus faecium by the β-lactam imipenem. J. Biol. Chem. 282, 30414–30422. doi: 10.1074/jbc.M704286200
McElvania TeKippe, E., Thomas, B. S., Ewald, G. A., Lawrence, S. J., and Burnham, C. A. D. (2014). Rapid emergence of daptomycin resistance in clinical isolates of Corynebacterium striatum… a cautionary tale. Eur. J. Clin. Microbiol. Infect. Dis. 33, 2199–2205. doi: 10.1007/s10096-014-2188-6
Merkamm, M., Chassagnole, C., Lindley, N. D., and Guyonvarch, A. (2003). Ketopantoate reductase activity is only encoded by ilvC in Corynebacterium glutamicum. J. Biotechnol. 104, 253–260. doi: 10.1016/S0168-1656(03)00145-7
Mitchell, K. F., McElvania, E., Wallace, M. A., Droske, L. E., Robertson, A. E., Westblade, L. F., et al. (2021). Evaluating the rapid emergence of Daptomycin resistance in Corynebacterium: a multicenter study. Simner PJ, éditeur. J. Clin. Microbiol. 59, e02052–e02020. doi: 10.1128/JCM.02052-20
Rezaei Bookani, K., Marcus, R., Cheikh, E., Parish, M., and Salahuddin, U. (2018). Corynebacterium jeikeium endocarditis: a case report and comprehensive review of an underestimated infection. IDCases. 11, 26–30. doi: 10.1016/j.idcr.2017.11.004
Riegel, P., De Briel, D., Prévost, G., Jehl, F., and Monteil, H. (1994). Genomic diversity among Corynebacterium jeikeium strains and comparison with biochemical characteristics and antimicrobial susceptibilities. J. Clin. Microbiol. 32, 1860–1865. doi: 10.1128/jcm.32.8.1860-1865.1994
Rosato, A. E., Lee, B. S., and Nash, K. A. (2001). Inducible macrolide resistance in Corynebacterium jeikeium. Antimicrob Agents Chemother. 45, 1982–1989. doi: 10.1128/AAC.45.7.1982-1989.2001
Schafer, A., Tauch, A., Jsger, W., Kalinowski, J., Thierbachb, G., and Piihler, A. (1994). Small mobilizable multi-purpose cloning vectors derived from the Escherichia coli plasmids pK18 and pK19: selection of defined deletions in the chromosome of Corynebacterium glutumicum. Gene 145, 69–73. doi: 10.1016/0378-1119(94)90324-7
Seutz, Y., Bäcker, H., Akgün, D., Adelhoefer, S., Kriechling, P., Gonzalez, M. R., et al. (2023). Corynebacterium periprosthetic joint infection: a systematic review of 52 cases at 2.5 years follow-up. Arch. Orthop. Trauma Surg. 143, 5527–5538. doi: 10.1007/s00402-023-04844-8
Soriano, F., Ponte, C., Santamaria, M., Aguado, J. M., Wilhelmi, I., Vela, R., et al. (1985). Corynebacterium group D2 as a cause of alkaline-encrusted cystitis: report of four cases and characterization of the organisms. J. Clin. Microbiol. 21, 788–792. doi: 10.1128/jcm.21.5.788-792.1985
Soriano, F., and Tauch, A. (2008). Microbiological and clinical features of Corynebacterium urealyticum: urinary tract stones and genomics as the Rosetta stone. Clin. Microbiol. Infect. 14, 632–643. doi: 10.1111/j.1469-0691.2008.02023.x
Soriano, F., Zapardiel, J., and Nieto, E. (1995). Antimicrobial susceptibilities of Corynebacterium species and other non-spore-forming gram-positive bacilli to 18 antimicrobial agents. Antimicrob. Agents Chemother. 39, 208–214. doi: 10.1128/AAC.39.1.208
Tauch, A., Kaiser, O., Hain, T., Goesmann, A., Weisshaar, B., Albersmeier, A., et al. (2005). Complete genome sequence and analysis of the multiresistant nosocomial pathogen Corynebacterium jeikeium K411, a lipid-requiring bacterium of the human skin Flora. J. Bacteriol. 187, 4671–4682. doi: 10.1128/JB.187.13.4671-4682.2005
Tauch, A., Trost, E., Tilker, A., Ludewig, U., Schneiker, S., Goesmann, A., et al. (2008). The lifestyle of Corynebacterium urealyticum derived from its complete genome sequence established by pyrosequencing. J. Biotechnol. 136, 11–21. doi: 10.1016/j.jbiotec.2008.02.009
Keywords: Corynebacteriaceae , β-lactam, Pbp, Pbp2c, Corynebacterium jeikeium , Corynebacteria
Citation: Lavollay M, Buon C, Le Moigne V, Compain F, Guyonvarch A and Fonvielle M (2024) Exploration of the role of the penicillin binding protein 2c (Pbp2c) in inducible β-lactam resistance in Corynebacteriaceae. Front. Microbiol. 15:1327723. doi: 10.3389/fmicb.2024.1327723
Edited by:
Mona I. Shaaban, Mansoura University, EgyptReviewed by:
Martin S. Pavelka, University of Rochester, United StatesSalome N. Seiffert, Zentrum für Labormedizin (ZLM), Switzerland
Wanda M. Figueroa-Cuilan, The Johns Hopkins Hospital, Johns Hopkins Medicine, United States
Copyright © 2024 Lavollay, Buon, Le Moigne, Compain, Guyonvarch and Fonvielle. This is an open-access article distributed under the terms of the Creative Commons Attribution License (CC BY). The use, distribution or reproduction in other forums is permitted, provided the original author(s) and the copyright owner(s) are credited and that the original publication in this journal is cited, in accordance with accepted academic practice. No use, distribution or reproduction is permitted which does not comply with these terms.
*Correspondence: Marie Lavollay, bWFyaWUubGF2b2xsYXlAaW1t; Matthieu Fonvielle, bWF0dGhpZXUuZm9udmllbGxlQGluc2VybS5mcg==