- 1School of Life Science and Technology, Harbin Normal University, Harbin, China
- 2Hailun National Observation and Research Station of Agroecosystems, State Key Laboratory of Black Soils Conservation and Utilization, Northeast Institute of Geography and Agroecology, Chinese Academy of Sciences, Harbin, China
- 3Jilin Provincial Key Laboratory for Plant Resources Science and Green Production, Jilin Normal University, Siping, China
- 4College of Advanced Agricultural Sciences, University of Chinese Academy of Sciences, Beijing, China
Soil nitrogen (N) availability is one of the limiting factors of crop productivity, and it is strongly influenced by global change and agricultural management practices. However, very few studies have assessed how the winter drought affected soil N availability during the subsequent growing season under chemical fertilization. We conducted a field investigation involving snow removal to simulate winter drought conditions in a Mollisol cropland in Northeast China as part of a 6-year fertilization experiment, and we examined soil physicochemical properties, microbial characteristics, and N availability. Our results demonstrated that chemical fertilization significantly increased soil ammonium and total N availability by 42.9 and 90.3%, respectively; a combined winter drought and fertilization treatment exhibited the highest soil N availability at the end of the growing season. As the growing season continued, the variation in soil N availability was explained more by fertilization than by winter drought. The Mantel test further indicated that soil Olsen-P content and microbial carbon use efficiency (CUE) were significantly related to soil ammonium availability. A microbial community structure explained the largest fraction of the variation in soil nitrate availability. Microbial CUE showed the strongest correlation with soil N availability, followed by soil available C:P and bacteria:fungi ratios under winter drought and chemical fertilization conditions. Overall, we clarified that, despite the weak effect of the winter drought on soil N availability, it cannot be ignored. Our study also identified the important role of soil microorganisms in soil N transformations, even in seasonally snow-covered northern croplands.
1 Introduction
Approximately one-quarter of the world’s arable land is located in snowy and cold regions, which are experiencing more intense and frequent drought stress in the context of global change (Zhou et al., 2017). Winter drought would result in a reduction in snow cover, and soils would be increasingly exposed to cold air, further exacerbating soil freezing, especially during cold spells (Vankoughnett and Henry, 2014). Soil frozen can alter the diversity of soil organisms and biogeochemical cycling in terrestrial ecosystems, thereby affecting the nutrient supply and growth of plants during the subsequent growing season (Makoto et al., 2014; Urakawa et al., 2014).
Soil nitrogen (N) availability is a critical parameter controlling organisms’ growth and ecosystem functions, but it can easily be reduced through leaching or trace gas emissions (Urakawa et al., 2014). A previous meta-analysis revealed that the winter drought reduced the availability of N in the soils and increased the potential nutrient leaching, owing to the cell burst of soil microorganisms and plant roots, which reduced the activities of soil microorganism and plant roots (Song et al., 2017). In order to mitigate the negative effects of extreme climate events on soil quality and reduce available N losses, effective management practices are urgently required to increase soil fertility and N availability. Chemical fertilizer supply is an effective strategy to enhance crop yields and improve soil fertility worldwide (Nguyen et al., 2018), and its large-scale use is also associated with massive disruptions to global biodiversity and N and phosphorus (P) cycles. However, we are still far from fully understanding the responses of soil N availability to the combined effects of the predicted changes in snowfall (e.g., winter drought) and fertilization.
Chemical fertilizer, such as ammonium hydrogen phosphate, as an available N source can directly increase soil N availability. A 28-year maize–soybean rotation experiment showed that, compared to no fertilization, soil available N increased by 18.7% in chemical fertilization (Zhang et al., 2023). However, the dissolved inorganic N (DIN:NH4+-N and NO3−-N) of fluvo-aquic soil was unchanged after 2 years of chemical fertilization (Zhao et al., 2019). Previous findings indicated that the effect of chemical fertilization on soil N availability exhibited a time-scale dependence. Chemical fertilization can also affect soil N availability indirectly via altered soil N transformation rates catalyzed by plants and microorganisms (Gentile et al., 2008; Hu et al., 2022). For example, in urea fertilization soils, the release of organic compounds into the soil can significantly alter microbial metabolism and activity in its immediate surroundings, thus enhancing organic N mineralization (Wiesenbauer et al., 2024). In addition, soil N availability was reported to be increased in response to fertilization in crop drought soils, whereas other studies observed decreased N availability in grassland drought soils (Hartmann et al., 2013; Gelfand et al., 2015). The effect of fertilization on soil N availability may be modified by the effect of winter drought, and we have limited knowledge regarding the potential key factors associated with soil N availability.
Soil N availability has been found to exhibit significant relationships with multiple abiotic (e.g., moisture and temperature) and biotic (i.e., plants and soil microorganisms) factors (Chaves et al., 2021; Liu et al., 2023). There are many pathways through which soil microorganisms can affect soil N availability. Microbial carbon (C) use efficiency (CUE) serves as an integrative index to describe the balance of soil nutrients (Tao et al., 2023). It has been reported that a decrease in soil N availability was accompanied by an increase in microbial CUE (Silva-Sánchez et al., 2019). A high microbial CUE might cause soil microorganisms to allocate more C to growth, thereby resulting in a lower metabolic cost of soil N acquisition (Manzoni et al., 2012). Additionally, soil N availability often depends on the abundance of N-cycling microbial functional genes (Hu et al., 2022), such as those of ammonia-oxidizing archaea and bacteria, which can alter N mineralization rates, thereby positively affecting soil N availability (Okano et al., 2004). Yu et al. (2018) have reported that the increased microbial biomass but not altered microbial community structure was beneficial to soil N transformation in crop rhizosphere. Although soil microorganisms have the potential to be a strong predictor linked to soil N availability, the influence direction is ambiguous. Moreover, the altered stoichiometry of soil substrates by winter drought and chemical fertilization could also affect microbial characteristics and soil N availability (Li et al., 2021). There are open questions about the relationships between soil properties and microorganisms and their effects on soil N availability.
Herein, we used snow removal to simulate winter drought and added chemical fertilizers to soils during spring sowing in a maize–soybean rotation cropland in the Mollisols area in Northeast China. We evaluated the responses of soil ammonium, nitrate, and total N availability to the effects of winter drought and chemical fertilization during the subsequent growing season. To identify potential factors related to soil N availability, we investigated the soil physicochemical properties (e.g., soil water content, pH, dissolved organic carbon (DOC), DIN, Olsen-P, and available potassium (AK) content) and microbial characteristics (e.g., biomass, community structure, CUE, and extracellular enzyme activities). We hypothesized that (1) soil N availability would increase in response to chemical fertilization, but it would decrease in response to winter drought because decreases in soil temperature could reduce the activities of soil microorganisms (Song et al., 2017), which were responsible for N mineralization (Mooshammer et al., 2014) and (2) microbial characteristics, rather than soil properties, would be the primary factor associated with soil N availability, because soil microorganisms were sensitive to changes in temperature, especially in cold regions.
2 Materials and methods
2.1 Site description
The experiment was conducted at the Hailun National Observation and Research Station of Agroecosystems of the Chinese Academy of Science (47°26′N, 126°38′E, 240 m in elevation), which is located in the central region of the Mollisols area in Northeast China. The study area exhibits a typical temperate continental monsoon climate with cold winters and hot summers. The mean annual air temperature is 2.0°C (which has been the same in the past 70 years). The non-growing season lasts from October to April with the lowest mean monthly temperature of −23°C in January, whereas the growing season lasts from May to September with the highest mean monthly temperature of 21°C in July (Hao et al., 2022). The mean annual precipitation is 550 mm, and more than 65% of precipitation is concentrated from June to August. Snowfall generally begins in early to mid-November, with snowmelt occurring by late March or early April. The soil type is typical Mollisol (USDA soil taxonomy) with a silty clay loam texture. The soil had a pH of 5.1, a total carbon (TC) content of 30.8 g kg−1, a total nitrogen (TN) content of 2.3 g kg−1, a total phosphorus (TP) content of 1.0 g kg−1, and a total potassium (TK) content of 22.6 g kg−1 before the start of the experiment in 2017.
2.2 Experimental design
The experiment was initiated in June 2017 with a completely randomized design. We established 12 plots (each has an area of 2.5 m × 2.8 m) and separated plots with a 0.5-m tall white steel plate (0.2 m aboveground, 0.3 m belowground). There were four treatments, namely a non-drought and non-fertilization (control: C), a winter drought (D), a chemical fertilization (F), and a combined winter drought and chemical fertilization (DF), which resulted in three replicates. Each plot was divided into four ridges, with a length and a width of 2.5 and 0.7 m, respectively. Maize (Zea mays L.) was planted in rotation with soybean (Glycine max Merr.) in alternate years. In 2017, maize was planted, and in the following year, soybeans were planted. The planting density of maize and soybean was 12 plants per ridge and 24 plants per ridge, respectively.
For the fertilization treatments, the chemical fertilizers, such as urea, ammonium hydrogen phosphate, and potassium sulfate, were applied at the rates of 138 kg N, 70 kg P2O5, and 20 kg K2O ha−1 y−1 for maize, and 64 kg N, 70 kg P2O5, and 20 kg K2O ha−1 y−1 for soybean (Zhang et al., 2023). For maize planting years, P and kalium (K) fertilizers were applied during the sowing period, and N fertilizer was applied at the sowing and jointing stages with a ratio of 1:2. For soybean planting years, all chemical fertilizers were applied during the sowing period.
In late October 2021, we set up a 1-year winter drought (snow removal) treatment. We attached a black plastic net (1 cm−2 in hole size) to the soil in each plot. To prevent snow accumulation on the snow removal treatments, we used brooms to remove the snow out of the plot down to the level of the plastic net (typically following major snowfall events). Following the final snowmelt, we removed the plastic nets when the snow was completely melted.
Soil temperature and soil water content (SWC) were measured using sensors (MEC10, Zheqin Technology Co., Ltd., Dalian, Liaoning, China) at a depth of 0–5 cm. The measurements were taken continuously from November 2021 to October 2022.
2.3 Soil sample collection
In May and October 2022, we collected five soil cores (3.5 cm in diameter, 0–10 cm depth) randomly from each plot and homogenized them into a composite soil sample. All soil samples were sieved through a 2-mm mesh to remove roots, litter, debris, and stones. The soil samples were divided into three parts, with one part stored at 4°C for measuring SWC, DOC, NH4+-N, NO3−-N, soil extracellular enzyme activity (EEA), and microbial biomass; one part stored at −20°C for phospholipid fatty acid (PLFA) analysis; and the last part air-dried to determine soil pH, Olsen-P, and AK contents.
2.4 Soil properties measurement
SWC was measured after drying 10 g of fresh soil at 105°C until a constant weight was achieved. Soil pH was measured by shaking a 1:2.5 ratio of air-dried soil to deionized water for 30 min. DOC was extracted by 0.5 M K2SO4 and then measured using a TOC analyzer (enviro TOC, Elementar, Germany). Soil NH4+-N and NO3−-N were extracted by 2 M KCl and analyzed by a continuous flow analyzer (AA3, Seal Analytical, Norderstedt, Germany). Olsen-P was determined colorimetrically (malachite green) after extracting samples with 0.5 M NaHCO3. AK was digested with 1 M ammonium acetate and detected by flame photometry.
2.5 Soil enzyme activity
We quantified soil EEAs using a modified fluorometric technique (Yang et al., 2023), including labile C-cycling enzymes: α-1,4-glucosidase (AG), β-1,4-glucosidase (BG), β-xylosidase (BX), and β-D-cellobiosidase (CBH), N-cycling enzymes: β-1,4-N-acetylglucosaminidase (NAG) and L-leucine aminopeptidase (LAP), and P-cycling enzymes: acid phosphatase (AP). The corresponding substrates of these EEAs were as described in Matulich et al. (2015). Soil suspensions were prepared by adding 1 g of fresh soil to 100 mL of 50 mM acetate buffer. Four replicates were used for sample assay wells, blank wells, quench standard wells, negative control wells, and reference standard wells. The 96-deep-well microplates were covered and incubated in the dark at 20°C for 4 h, and the reaction was stopped by adding 10 μL of 1 M NaOH to each well. Fluorescence was measured using a microplate reader (Synergy LX, BioTek Instruments, Winooski, VT, United States) with 360 nm excitation and 460 nm emission filters.
2.6 Microbial biomass and carbon use efficiency
Microbial biomass carbon (MBC), nitrogen (MBN), and phosphorus (MBP) were determined with the chloroform fumigation-extraction method (Joergensen, 1996). Microbial CUE derived from the biogeochemical equilibrium model was calculated as follows (Sinsabaugh and Follstad Shah, 2012):
where KC:N and KC:P represent the half-saturation constants for CUE based on the availability of C, N, and P. We assumed that KC:N and KC:P were 0.5 for the model and CUEmax was 0.6 in this study (Sinsabaugh et al., 2016). EEAC:N was calculated as (AG + BG + BX + CBH)/(NAG + LAP), and EEAC:P was calculated as (AG + BG + BX + CBH)/AP. BC:N and BC:P were the elemental C:N and C:P ratios of microbial biomass. LC:N and LC:P were estimated as DOC:DIN and DOC:Olsen-P, respectively.
2.7 Phospholipid fatty acid analysis
PLFAs were extracted from the soils as described by Yang et al. (2020). The 19:00 was used as an internal standard. The fatty acids (FAs) 14:00, 15:00, 16:00, 17:00, and 18:00 were chosen to represent the non-specific bacteria (Frostegård and Bååth, 1996; Leckie, 2005); i14:0, a15:0, i15:0, i15:1ω6c, i16:0, a17:0, and i17:0 were used to represent the Gram-positive bacteria (G+); 16:0 2OH, 16:1ω7c, 16:1ω9c, cy17:0ω7c, 17:1ω8c, 18:1ω5c, 18:1ω6c, 18:1ω7c, and cy19:0ω7c were chosen to represent the Gram-negative bacteria (G−) (Leckie, 2005; Chen et al., 2017); and 10Me16:0, 10Me17:0, 10Me17:1ω7c, and 10Me 18:0 were used to represent actinomycetes (Ac) (Zhang et al., 2022). The sum of G+, G−, Ac, and non-specific bacteria was used as the total bacteria. In addition, 18:1ω9c, 18:2ω6c, 18:3ω6c, and 16:1ω5c were chosen to represent the fungi (Joergensen and Wichern, 2008; Chang et al., 2021). The total PLFAs were the sum of bacterial and fungal PLFAs, and the B:F ratio was calculated as the bacterial-to-fungal PLFA ratio.
The structure of the microbial community was analyzed using the principal component analysis (PCA) based on the relative molar abundances of the entire PLFA signature (mol-% of the 29 most abundant FAs) after standardizing to unit variance. The PC1 scores for the entire PLFAs were used as indicators for the phylum-level microbial community structure (Chen et al., 2013).
2.8 Soil inorganic N ionic exchange membranes
Soil N availability was measured in situ using ionic exchange membranes (IEMs), which have been shown to be accurate in measuring soil N availability and generate minimal disturbance to the soil microbial community (Liu et al., 2018). We inserted one anion and one cation IEM (2.5 cm × 10 cm) in each sampling quadrat to absorb ammonium ions (NH4+) and nitrate ions (NO3−). Approximately 15 days later, we collected IEMs and immediately rinsed them with deionized water to remove soil. A pair of IEMs were placed into 150-mL flasks for extraction with 70 mL of 2 M KCl by orbital shaking (1 h at 160 rpm). NH4+ and NO3− concentrations were analyzed using a continuous flow analyzer (AA3, Seal Analytical, Norderstedt, Germany). Soil ammonium and nitrate availability were calculated using the following formula: [(concentration in μg N per mL) × (70 mL KCl)]/(50 cm2 area of the strip × days in the ground) (Liu et al., 2018). Soil N availability was determined as the sum of soil ammonium and nitrate availability from the IEMs.
2.9 Statistical analyses
Statistical analysis was performed with IBM SPSS 27.0.1 (SPSS Inc., Chicago, IL, United States). All variables of the data were tested for normal distribution and homogeneity of variance. The effects of winter drought, chemical fertilization, and their interaction on soil properties, microbial characteristics, enzyme activities, and soil N availability were tested using a two-way ANOVA. For each specific parameter, if the interaction between winter drought and chemical fertilization (i.e., D × F) was insignificant, we used the terms non-drought (C and F plots) vs. drought (D and DF plots) for the winter drought main effect, and non-fertilization (C and D plots) vs. fertilization (F and DF plots) for the chemical fertilization main effect; if the interaction was significant, we used the terms non-drought vs. drought in non-fertilization and fertilization and then non-fertilization vs. fertilization in non-drought and drought. At the non-fertilization level, we compared C vs. D plots, and at the fertilization level, we compared F vs. DF plots; at the non-drought level, we compared C vs. F plots, and at the drought level, we compared D vs. DF plots. The Mantel test was performed for a better understanding of the correlation between soil N availability and environmental variables (including soil properties and microbial characteristics). All results were reported as means ± standard errors, and a significance level of p < 0.05 was used for all analyses. The figures were plotted in GraphPad Prism 9.5.0 (GraphPad Software Inc., San Diego, CA, United States) and the ggplot2 package in R software (R Development Core Team, 2023).
3 Results
3.1 Soil microclimate conditions
There was significant seasonal variation in both soil temperature and SWC, and it was described as a low temperature and dry non-growing season with a high temperature and wet growing season (Figure 1). Compared to the ambient snow plots, snow removal significantly decreased the average (−8.6 vs. −3.1°C) and minimal soil temperature (−17.0 vs. −6.8°C) at 5 cm depth over the non-growing season. Moreover, the timing of soil freezing and melting was advanced, and freeze–thaw was increased by two cycles of snow removal (Figure 1A). After 3 weeks of snowmelt, snow removal had no significant effect on SWC (Figure 1B).
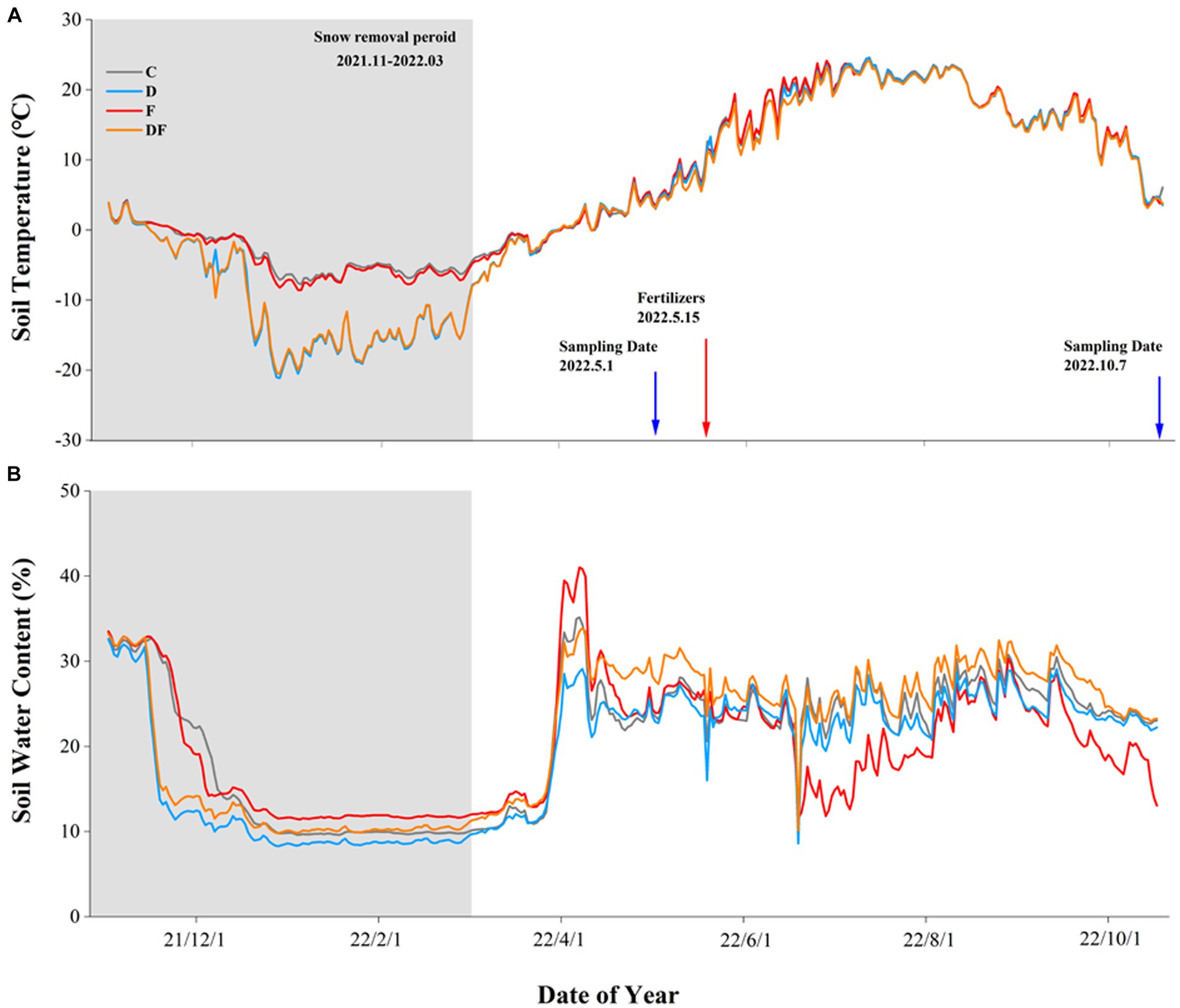
Figure 1. Soil temperature (A) and water content (B) in response to winter drought and chemical fertilization from November 2021 to October 2022 at 5 cm soil depth. C = control, D = winter drought, F = chemical fertilization, and DF = combined winter drought and chemical fertilization.
3.2 Soil physicochemical properties
Neither winter drought nor fertilization had significant effects on SWC, DOC, DIN, and AK on the sampling dates of May and October 2022 (p > 0.05), but the ratio of DOC:DIN increased by 32.2% in response to the winter drought and decreased by 15.8% in response to fertilization in October. Soil pH was significantly decreased by snow removal (p < 0.001) but was not affected by the legacy effect of fertilization in May (p > 0.05). Winter drought, fertilization, and their interaction exhibited no significant impacts on the Olsen-P and DOC:Olsen-P (p > 0.05), but the legacy effect of fertilization profoundly decreased the DIN:Olsen-P by 27.3% in May (p < 0.05). Olsen-P was significantly increased by winter drought and fertilization in October (both p < 0.001), which caused a reduction in the DOC:Olsen-P and DIN:Olsen-P (both p < 0.001). In addition, a significant interactive effect of winter drought and fertilization on the DOC:Olsen-P and DIN:Olsen-P was observed (both p < 0.001; Table 1; Supplementary Table S1).
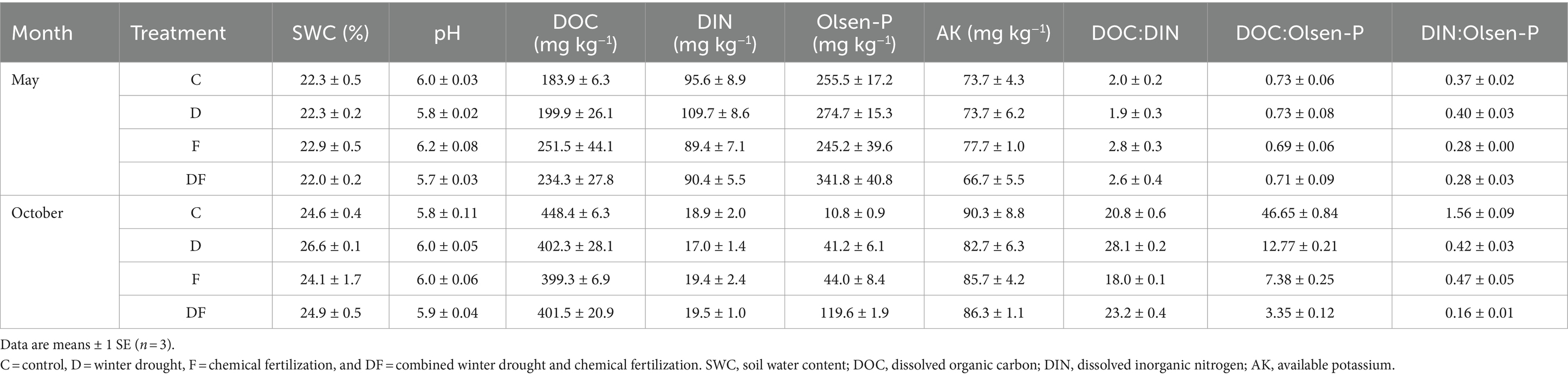
Table 1. Soil physicochemical properties in response to winter drought and chemical fertilization in May and October 2022.
3.3 Soil extracellular enzyme activity
The winter drought profoundly increased the activities of AG (19.9%, p < 0.05), CBH (72.3%, p < 0.05), LAP (p < 0.01), and AP (19.1%, p < 0.05) in spring sampling. Winter drought significantly increased LAP activity by 37.0% (p < 0.05) in non-fertilization plots, but not in fertilization plots, and a significant interactive effect of winter drought and fertilization on LAP (p < 0.05) was observed in May. In October, winter drought significantly increased CBH and NAG activities (both p < 0.05), and fertilization increased NAG activity by 179.4% (p < 0.01). A significant interactive effect of winter drought and fertilization on CBH activity was detected (p < 0.05; Table 2; Supplementary Table S2).
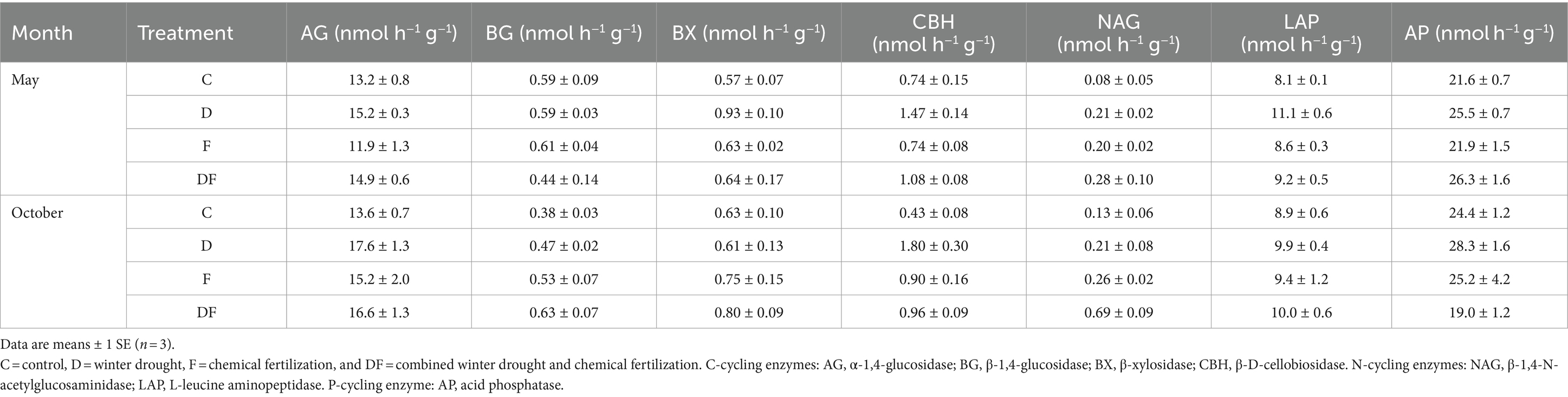
Table 2. Soil C-, N-, and P-acquiring enzyme activities in response to winter drought and chemical fertilization in May and October 2022.
3.4 Soil microbial characteristics
Neither winter drought nor fertilization had significant changes in MBC or MBN (p > 0.05; Figures 2A,B). In May, MBP increased by 66.4% in response to the legacy effect of fertilization (p < 0.001). There was a significant interactive effect of winter drought and fertilization on MBP (p < 0.001), and winter drought significantly decreased MBP (p < 0.01) in October. Winter drought significantly decreased MBP by 63.9% in non-fertilization plots (p < 0.001) but not in fertilization plots (p > 0.05; Figure 2C). We also found that microbial CUE increased by 125% in response to fertilization in October (p < 0.001; Figure 2D).
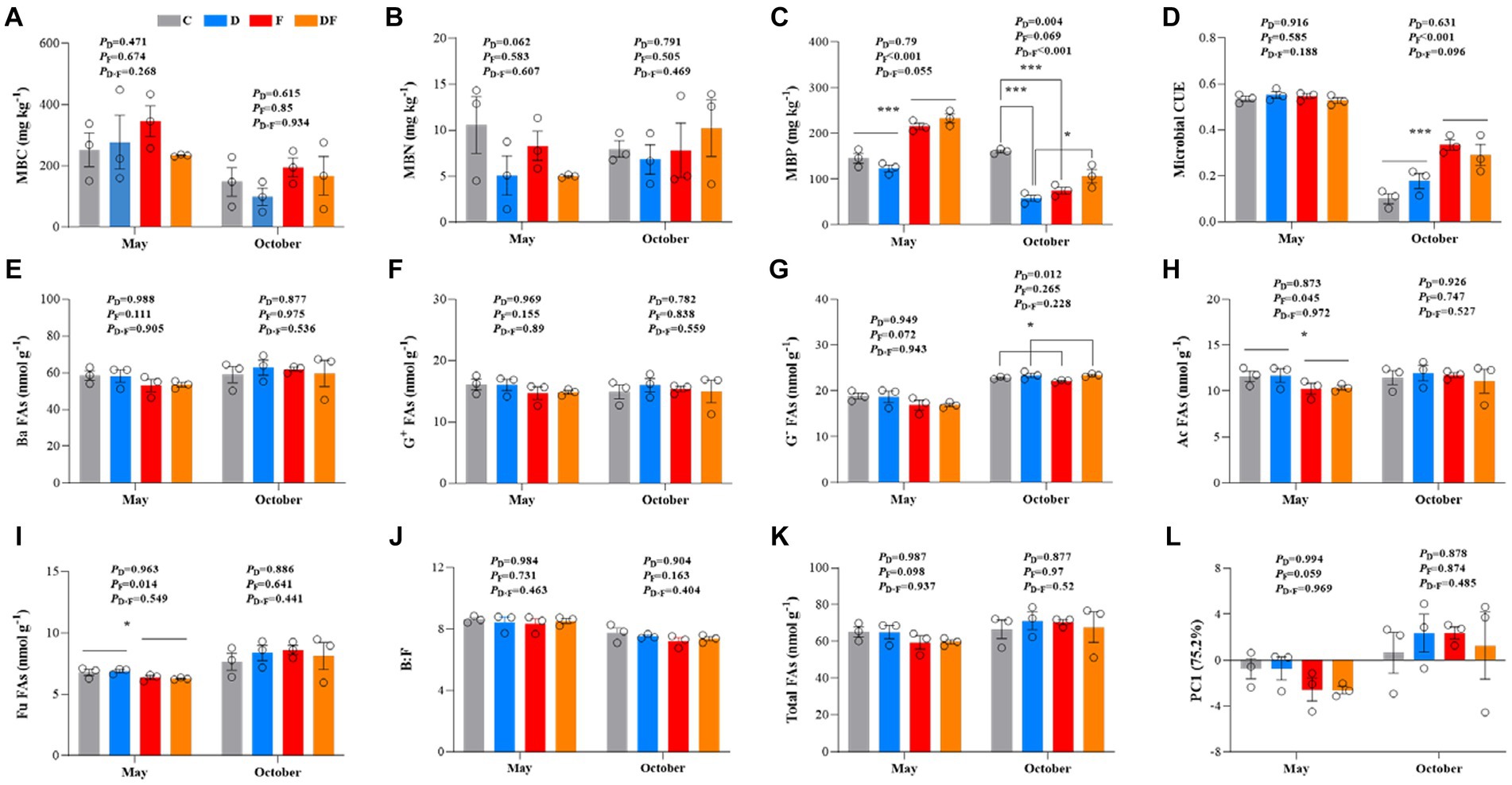
Figure 2. Soil microbial characteristics (A–L) in response to winter drought and chemical fertilization in May and October 2022. C = control, D = winter drought, F = chemical fertilization, and DF = combined winter drought and chemical fertilization. The p-values were expressed as follows: *p < 0.05; **p < 0.01; ***p < 0.001. Data are means ±1 SE (n = 3). MBC, microbial biomass carbon; MBN, microbial biomass nitrogen; MBP, microbial biomass phosphorus; CUE, microbial carbon use efficiency; Ba FAs, bacterial PLFAs; G+ FAs, Gram-positive PLFAs; G− FAs, Gram-negative PLFAs; Ac FAs, actinomycetes PLFAs; Fu FAs, fungal PLFAs; B:F, the ratio of bacterial to fungal PLFAs.
Bacterial FAs, G+ FAs, B:F, and total FAs were not affected by winter drought, fertilization, or their interaction (p > 0.05; Figures 2E,F,J,K). However, there were significant declines in actinomycetes and fungal FAs due to the legacy effect of fertilization; they reduced by 11.6 and 7.3%, respectively, in May (both p < 0.05; Figures 2H,I). The winter drought exerted a significant positive effect on G− FAs in October (increased 4.2%, p < 0.05; Figure 2G). No significant interactive effects of winter drought and fertilization were observed on all FAs (p > 0.05; Figures 2E–K). According to the PCA, there was no significant change in the phylum-level microbial community structure throughout the whole growing season by the treatments (p > 0.05; Figure 2L).
3.5 Soil N availability
The IEM results showed that nitrate availability was higher than ammonium availability in this Mollisol cropland (Figures 3A,B). In spring 2022, winter drought significantly increased soil N availability (May 26–June 6 and June 6–22, p < 0.01 and p < 0.05; Figure 3C). However, as the growing season continued, the dominant treatment explaining variation in soil N availability shifted from the winter drought to fertilization (Figure 3). At the seed filling period of soybean, both nitrate and total N availability showed positive responses to fertilization (Figures 3B,C). At the mature period, ammonium availability decreased by 7.7% in response to winter drought (p < 0.05) and increased by 15.0% in response to fertilization (p < 0.01; Figure 3A). At the last sampling period (September 23–October 7), we found that fertilization significantly increased ammonium availability (p < 0.01; Figure 3A) and soil N availability (p < 0.001; Figure 3C).
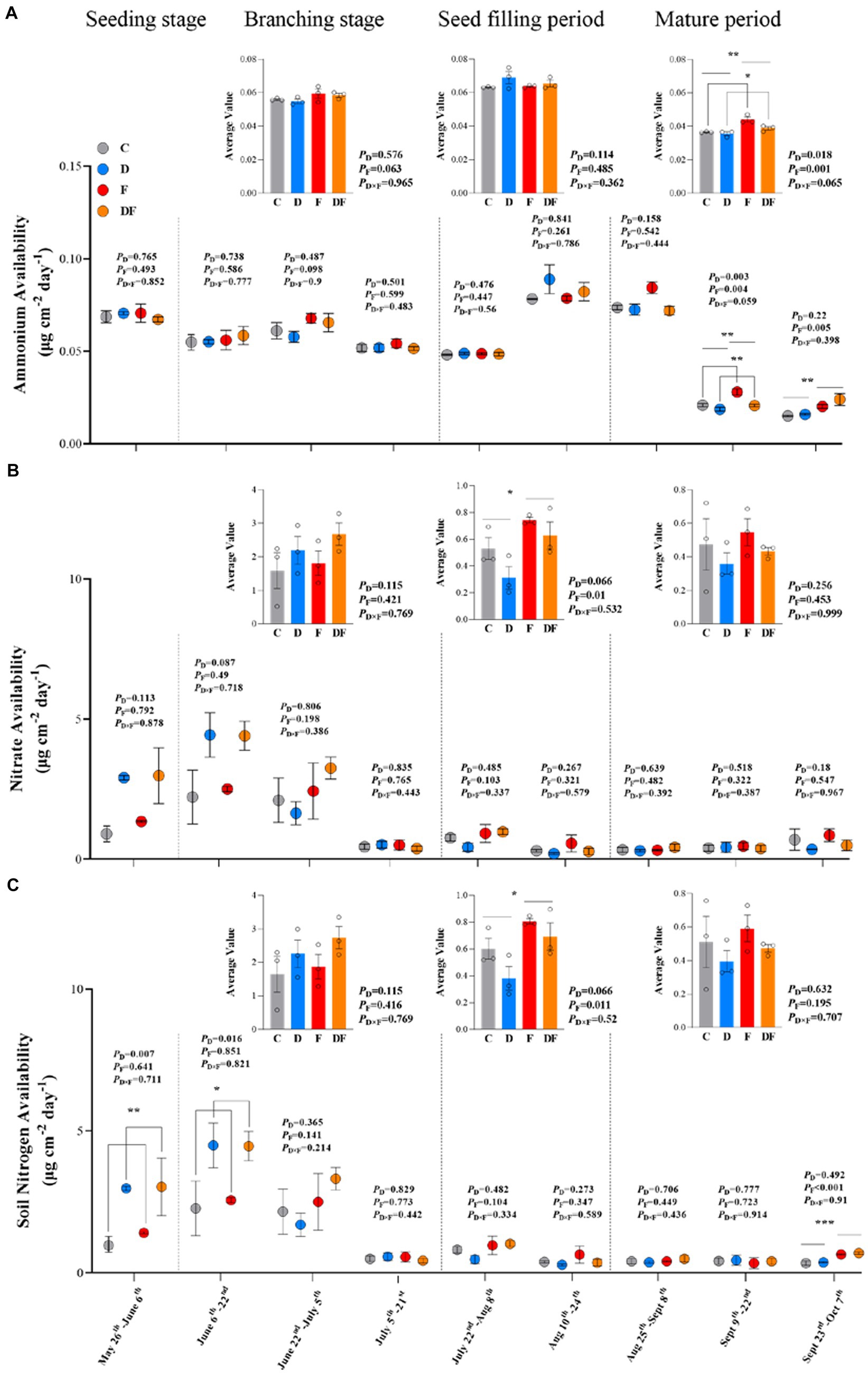
Figure 3. Responses of IEMs measured ammonium (A), nitrate (B), and N (C) availability to winter drought and chemical fertilization from May to October 2022. The inset figures were the changes in the average level of N availability during four growth periods of soybean. C = control, D = winter drought, F = chemical fertilization, and DF = combined winter drought and chemical fertilization. The p-values were expressed as follows: *p < 0.05; **p < 0.01; ***p < 0.001. Data are means ±1 SE (n = 3).
3.6 Influencing factors of soil N availability
The Mantel test and Spearman correlation analysis showed that ammonium availability was significantly correlated with Olsen-P content and microbial CUE (p < 0.05). Microbial factors explained the largest fraction of the variation in nitrate availability; the microbial community structure (PC1) exhibited the strongest correlation (p < 0.01), followed by Ba FAs, G+ FAs, Ac FAs, Fu FAs, and total FAs (all p < 0.05), and we found significant positive correlations among these microbial factors. Soil N availability is significantly related to microbial CUE (p < 0.01), DOC:Olsen-P (p < 0.05), and soil B:F (p < 0.05; Figure 4).
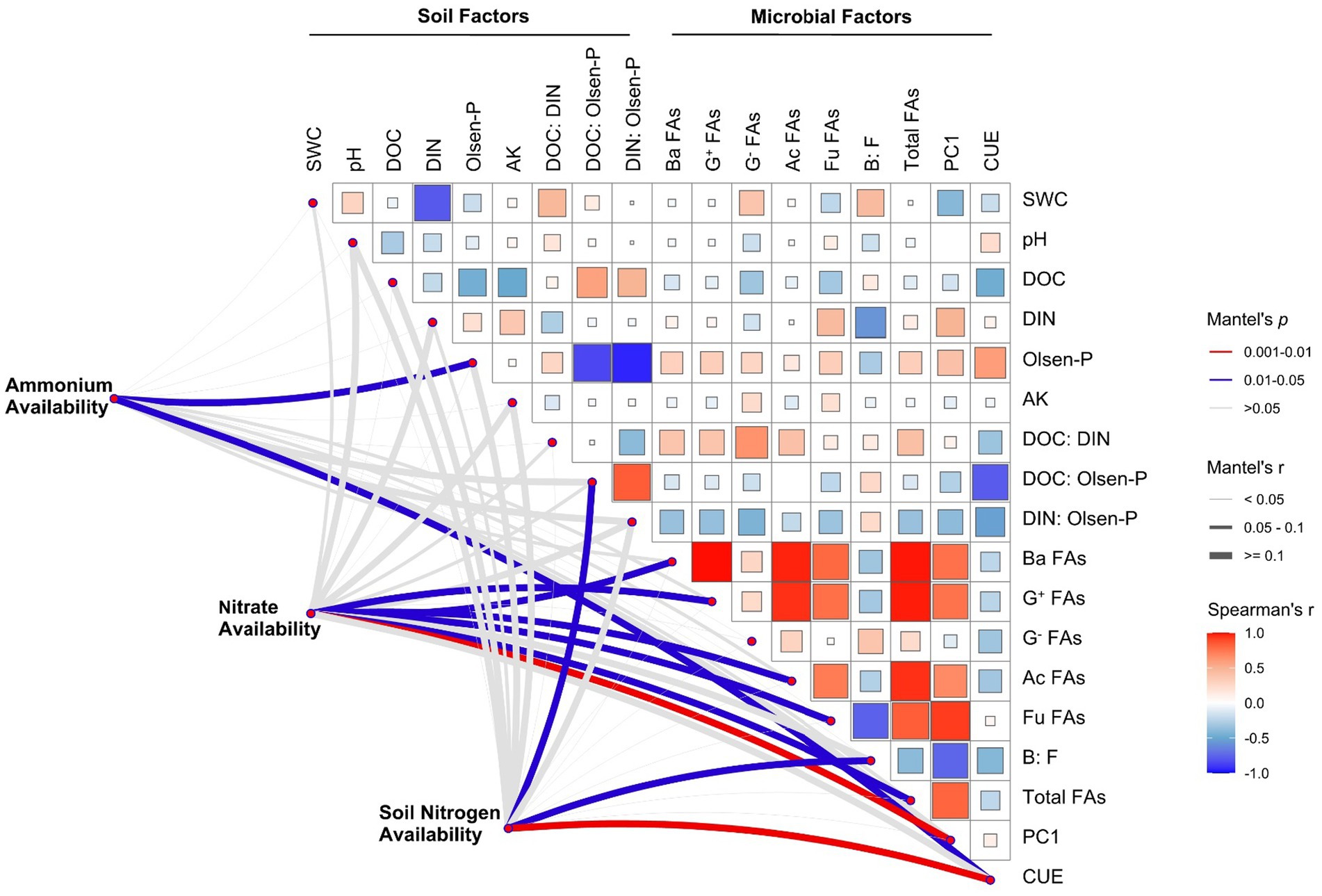
Figure 4. Correlations among soil N availability, soil properties, and microbial characteristics. Pairwise comparisons of soil and microbial factors are shown, with color gradients denoting Spearman’s correlation coefficients. Edge width corresponds to Mantel’s r statistic for the corresponding distance correlations. SWC, soil water content; DOC, dissolved organic carbon; DIN, dissolved inorganic nitrogen; AK, available potassium; Ba FAs, bacterial PLFAs; G+ FAs, Gram-positive PLFAs; G− FAs, Gram-negative PLFAs; Ac FAs, actinomycetes PLFAs; Fu FAs, fungal PLFAs; B:F, the ratio of bacterial to fungal PLFAs; CUE, microbial carbon use efficiency.
4 Discussion
4.1 Soil properties in response to winter drought and chemical fertilization
A number of studies have shown that winter drought could significantly increase soil C, N, and P availability, because an increase in soil freeze–thaw that may promote soil shrinkage and expansion, which gave a direct release of mineralizable organic matters through the fragmentation of soil aggregate and mortality of fine roots and microorganisms (Song et al., 2017; Ji et al., 2022). However, our results showed that soil available C, N, and P remained unchanged after the winter drought (Table 1; Supplementary Table S1). This finding might be attributed to the energy and nutrients absorbed by soil microorganisms in early spring. Watanabe et al. (2019) observed that the concentration of DIN was higher by the treatment than that in control soil after snowmelt, and then, it would gradually decrease over time. This aspect suggested that the winter drought-induced changes in the available nutrients in the soil would dissipate as air and soil temperatures increased in early spring.
Our results indicated that the available C:N:P imbalances increased in the late growing season compared with the early growing season (Table 1; Supplementary Table S1) due to the rapid increase in soil Olsen-P content. We also found that the soil available C:P and N:P ratios (DOC:Olsen-P and DIN:Olsen-P) showed a profound decline by the winter drought in October. The explanation could be due to decreased microbial immobilization (Gao et al., 2021), which was supported by our results showing that winter drought significantly decreased soil MBP (Figure 2C). Soil available C:N, C:P, and N:P ratios exhibited generally consistent responses (reduced) to chemical fertilization in October because chemical fertilization can increase the input of N and P to the soils (Zhang et al., 2019).
4.2 Microbial characteristics in response to winter drought and chemical fertilization
Soil EEAs are usually related to microbial metabolic rates and are widely used to indicate microbial C, N, and P requirements (Sun et al., 2023). It has been reported that soil microorganisms suffer from relative P limitation in Mollisol croplands, and microbial biomass is the key controller for microbial N/P limitation (Yang et al., 2023). In unfertilized plots, MBP was significantly decreased by winter drought at the end of the growing season (Figure 2C), which suggested that the decreased MBP may further exacerbate the microbial P limitation in this Mollisol cropland. Although soil C-, N-, and P-acquiring EEAs were increased in response to winter drought in spring, they were unchanged and remained coupled under the four treatments at the end of the growing season (Table 2; Supplementary Table S2). After the snow removal period, microbial activity was stimulated by dramatic changes in soil temperature in the spring, but the response would be attenuated as the temperature differences decreased among treatments in October.
Microbial CUE is a pivotal index for understanding soil C turnover that is driven by microbial metabolism (Tao et al., 2023). Our results showed that chemical fertilization profoundly increased microbial CUE (Figure 2D). To achieve microbial elemental homeostasis when the soil nutrient (N and P) content was sufficient through fertilization, microorganisms would devote more energy (C) for self-growth and reproduction (Li et al., 2019; Tang et al., 2020). A high soil nutrient content can also alter intracellular C partition, which leads to a lower allocation of C to respiration and a higher allocation of C to growth (Spohn et al., 2016), resulting in an increase in microbial CUE.
However, microbial community composition (bacteria, fungi, and actinomycetes) and structure (phylum level) were not sensitive to both winter drought and chemical fertilization (Figures 2E–L). It is expected that the declines in Fu FAs and Ac FAs in response to the legacy effect of chemical fertilization were detected in the early growing season (Figures 2H,I). In the studied rotation system, the former crop was maize, and it did not have the ability to recruit N-fixers; its N requirement came from N fertilization (Breza et al., 2023). To some extent, crops and microorganisms have a competitive relationship. The high N uptake capacity of maize may lead to the death of fungi and actinobacteria by N deficiency (Liu et al., 2020).
4.3 Soil N availability in response to winter drought and chemical fertilization
Soil ammonium availability was lower than nitrate availability during the growing season (Figures 3A,B). This finding might be due to the lower energetic cost of NH4+, which can be preferentially taken up by the plants (Legay et al., 2020). Inconsistent with our first hypothesis, soil N availability was enhanced by winter drought in the early growing season (Figure 3C). This finding suggested that winter drought-induced soil freezing may increase the mortality of microorganisms, resulting in a large amount of available N in soils (Yang et al., 2020; Ji et al., 2022). Moreover, during the soil thawing process, the surviving organisms may use dead cells as substrates to increase mineralization (Yang et al., 2023). The increased soil N availability is likely to be transient and limited to the early growing season as we did not find evidence of continued soil N accumulation during the peak growing season. This finding was consistent with that of a previous study in a northern hardwood forest, indicating that the labile N from the winter period was insufficient for the demands of both microorganisms and plants in early summer (Watanabe et al., 2019).
By contrast to the winter drought, chemical fertilization had a longer duration to affect soil N availability. During the seed filling and mature period, to some extent, soil ammonium, nitrate, and total N availability showed positive responses to chemical fertilization (Figure 3). First, fertilization was applied in the early growing season, and most of the N-fertilization was dissociated to inorganic N (i.e., NO3− and NH4+) by soil microorganisms in late growing season. Second, plants have a low N requirement during the late growing season, which would be beneficial to the growth and/or activity of soil microorganisms, and further promote the decomposition of organic matter to increase the N availability in soils (Sun et al., 2021). We also found that a combination of winter drought and fertilization has the highest soil N availability (Figures 3C, 5). This finding agrees with a previous study that showed that combined freeze–thaw and fertilization had a larger N mineralization than single fertilization (Lin and Hernandez-Ramirez, 2022).
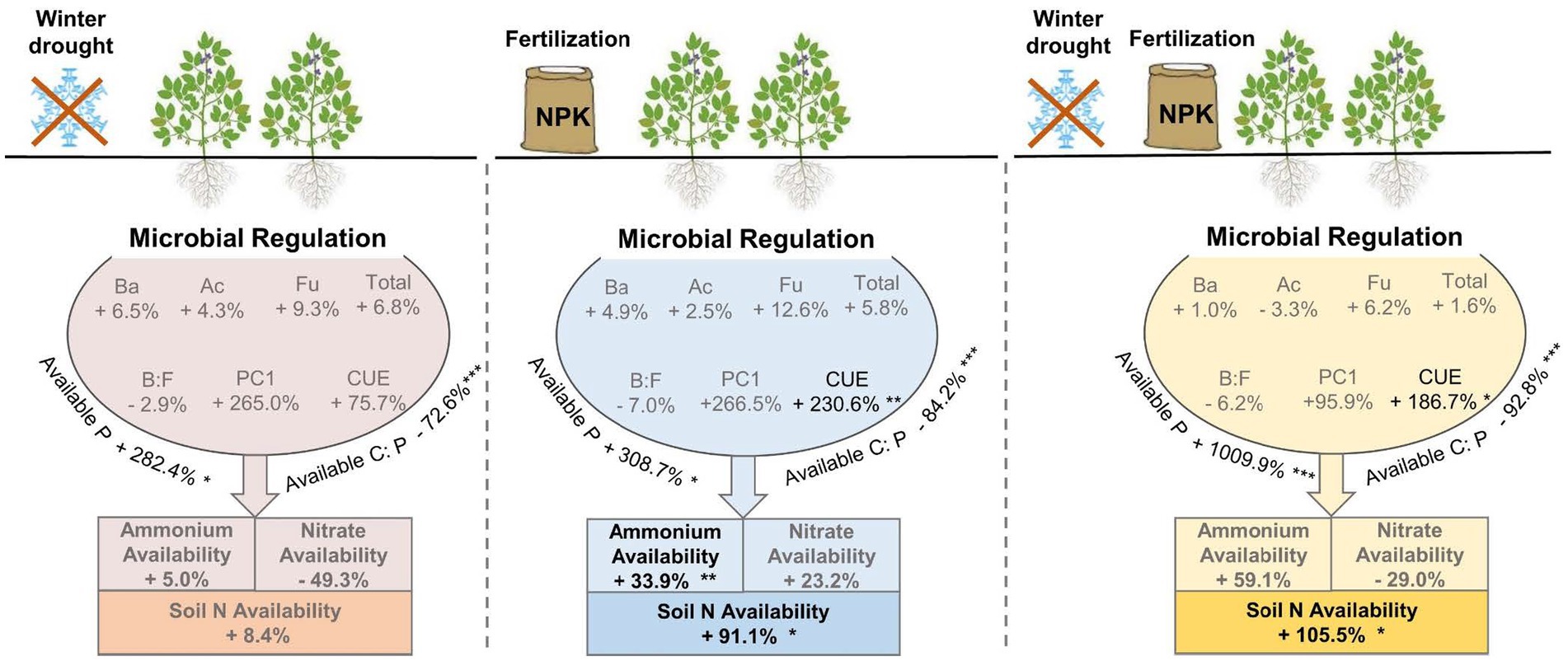
Figure 5. A conceptual diagram showing influences of winter drought and chemical fertilization on soil N availability. Plus and minus signs indicate the increased and decreased percentages of the response variables by winter drought, chemical fertilization, and a combination of winter drought and chemical fertilization, relative to the control plots. The p-values were expressed as follows: *p < 0.05; **p < 0.01; ***p < 0.001.
4.4 Relationships among soil properties, microbial characteristics, and soil N availability
In the present study, Olsen-P content, DOC:Olsen-P, and microbial CUE were significantly related to soil ammonium and total N availability (Figures 4, 5). A recent study conducted in a grassland ecosystem showed that soil available P played a key role in determining the growth and distribution of N-cycling microorganisms, which may improve soil N-fixing potential and increase soil N availability (Xiao et al., 2020). A large body of studies leads us to expect nutrient cycling to be coupled across space and time, especially in the N and P fertilization experiments (Achat et al., 2016). Both winter drought and chemical fertilization significantly increased Olsen-P content, which was a prerequisite for exacerbated soil ammonium and total N availability. The mineralization process releases N in the form of ammonium, which increases the amount of available N in soils.
The process of soil N mineralization has a high energy demand, and microorganisms may use soil available C substrates as an energy source to enable nitrogenase production (Schleuss et al., 2021). Our result showed that soil N availability was strongly correlated with microbial CUE under winter drought and fertilization conditions (Figures 4, 5). Multiple studies have revealed that soil microorganisms with a high CUE may reduce their respiration rate to increase their mineralization rate (Mehnaz et al., 2019; Yuan et al., 2019). Moreover, a high microbial CUE inherently requires more N to maintain the C:N ratio of their biomass (Feng et al., 2022; Zhu et al., 2023). The more assimilation of C in microbial biomass ultimately leads to a higher availability of N produced by microorganisms (Bei et al., 2022) due to the fact that N is generally coupled with C in the soils (Luo et al., 2015). The positive links between microbial CUE and soil N availability have been reported for agricultural soils with different fertilizer regimes (Spohn et al., 2016) and those were also found in unmanaged ecosystems (Tian et al., 2023).
Soil microbial biomass, structure, and composition can, directly and indirectly, influence soil nitrification and nitrate availability (Figure 4; Elrys et al., 2021; Van Huynh et al., 2023). Elrys et al. (2021) revealed that the high nitrification rates and a low soil C:N ratio caused by fertilization were associated with bacteria-dominated communities, and microbial communities produced by the nitrification process always belong to the bacteria genus (Shen et al., 2021). Additionally, although the bacteria:fungi ratio was unchanged by the winter drought and chemical fertilization, our results showed that soil N availability was related to the bacteria:fungi ratio (Figure 4). The fungal community is essential for decomposing cellulose, hemicellulose, and recalcitrant compounds, such as lignin (Shipley and Tardif, 2021). Chen et al. (2021) reported that fungi can drive soil ammonification by regulating fungal ligninolytic capacity, and changes in fungal ligninolytic capacity will, in turn, influence the release of available N. Soil N transformation processes were regulated by different microbial communities, and our results suggested that even a slight change in the ratio of bacteria to fungi would alter soil N availability.
5 Conclusion
In conclusion, our results showed that the effect of chemical fertilization rather than winter drought on soil N availability can continue throughout the growing season in agroecosystems. Chemical fertilization significantly increased microbial CUE and soil N availability, and a combination of winter drought and chemical fertilization had the highest soil N availability at the end of the growing season. Both microbial and soil properties have strong linkages with soil N availability. Although the microbial biomass and extracellular enzyme activities had quick responses to winter drought and chemical fertilization, the durations were less than a growing season. We found that microbial CUE was the most positive correlation factor with soil N availability, followed by soil available C:P and bacteria:fungi ratios. We concluded that the relationship between soil microbial function and N availability was crucial for understanding soil N-cycling processes under global change and agricultural management practices. We also highlighted the need to explicitly incorporate microbial characteristics into biogeochemical models to improve the prediction of ecosystem N cycling.
Data availability statement
The raw data supporting the conclusions of this article will be made available by the authors, without undue reservation.
Author contributions
WB: Data curation, Formal analysis, Investigation, Visualization, Writing – original draft, Writing – review & editing. PH: Investigation, Writing – review & editing. LH: Investigation, Writing – review & editing. XW: Writing – review & editing. LF: Investigation, Writing – review & editing. JZ: Funding acquisition, Writing – review & editing. JW: Funding acquisition, Writing – review & editing. XY: Conceptualization, Data curation, Formal analysis, Funding acquisition, Investigation, Project administration, Visualization, Writing – original draft, Writing – review & editing. L-JL: Conceptualization, Funding acquisition, Project administration, Writing – review & editing.
Funding
The author(s) declare financial support was received for the research, authorship, and/or publication of this article. This study was supported by the National Natural Science Foundation of China (32101396 and 42277350), the Young Scientist Group Project of Northeast Institute of Geography and Agroecology, Chinese Academy of Sciences (2023QNXZ04), the Heilongjiang Science Foundation for Distinguished Young Scholars (JQ2021C004), the China Postdoctoral Science Foundation (2023T160640 and 2021M703201), and the Heilongjiang Provincial Universities Basic Scientific Research Business Expenses Project (2021-KYYWF-0172). XY acknowledges support from the Special Research Assistant Program of the Chinese Academy of Sciences. JZ and WB acknowledge support from the Graduate Innovation Project of Harbin Normal University (HSDSSCX2022-142).
Conflict of interest
The authors declare that the research was conducted in the absence of any commercial or financial relationships that could be construed as a potential conflict of interest.
Publisher’s note
All claims expressed in this article are solely those of the authors and do not necessarily represent those of their affiliated organizations, or those of the publisher, the editors and the reviewers. Any product that may be evaluated in this article, or claim that may be made by its manufacturer, is not guaranteed or endorsed by the publisher.
Supplementary material
The Supplementary material for this article can be found online at: https://www.frontiersin.org/articles/10.3389/fmicb.2024.1304985/full#supplementary-material
References
Achat, D. L., Augusto, L., Gallet-Budynek, A., and Loustau, D. (2016). Future challenges in coupled C-N-P cycle models for terrestrial ecosystems under global change: a review. Biogeochemistry 131, 173–202. doi: 10.1007/s10533-016-0274-9
Bei, S., Li, X., Kuyper, T. W., Chadwick, D. R., and Zhang, J. (2022). Nitrogen availability mediates the priming effect of soil organic matter by preferentially altering the straw carbon-assimilating microbial community. Sci. Total Environ. 815:152882. doi: 10.1016/j.scitotenv.2021.152882
Breza, L. C., Mooshammer, M., Bowles, T. M., Jin, V. L., Schmer, M. R., Thompson, B., et al. (2023). Complex crop rotations improve organic nitrogen cycling. Soil Biol. Biochem. 177:108911. doi: 10.1016/j.soilbio.2022.108911
Chang, R., Liu, S., Chen, L., Li, N., Bing, H., Wang, T., et al. (2021). Soil organic carbon becomes newer under warming at a permafrost site on the Tibetan plateau. Soil Biol. Biochem. 152:108074. doi: 10.1016/j.soilbio.2020.108074
Chaves, B., Redin, M., Giacomini, S. J., Schmatz, R., Léonard, J., Ferchaud, F., et al. (2021). The combination of residue quality, residue placement and soil mineral N content drives C and N dynamics by modifying N availability to microbial decomposers. Soil Biol. Biochem. 163:108434. doi: 10.1016/j.soilbio.2021.108434
Chen, D., Lan, Z., Bai, X., Grace, J. B., and Bai, Y. (2013). Evidence that acidification-induced declines in plant diversity and productivity are mediated by changes in below-ground communities and soil properties in a semi-arid steppe. J. Ecol. 101, 1322–1334. doi: 10.1111/1365-2745.12119
Chen, Z., Li, Y., Chang, S. X., Xu, Q., Li, Y., Ma, Z., et al. (2021). Linking enhanced soil nitrogen mineralization to increased fungal decomposition capacity with Moso bamboo invasion of broadleaf forests. Sci. Total Environ. 771:144779. doi: 10.1016/j.scitotenv.2020.144779
Chen, Z., Wang, H., Liu, X., Zhao, X., Lu, D., Zhou, J., et al. (2017). Changes in soil microbial community and organic carbon fractions under short-term straw return in a rice-wheat cropping system. Soil Till. Res. 165, 121–127. doi: 10.1016/j.still.2016.07.018
Elrys, A. S., Wang, J., Metwally, M., Cheng, Y., Zhang, J. B., Cai, Z. C., et al. (2021). Global gross nitrification rates are dominantly driven by soil carbon-to-nitrogen stoichiometry and total nitrogen. Glob. Chang. Biol. 27, 6512–6524. doi: 10.1111/gcb.15883
Feng, X., Qin, S., Zhang, D., Chen, P., Hu, J., Wang, G., et al. (2022). Nitrogen input enhances microbial carbon use efficiency by altering plant-microbe-mineral interactions. Glob. Chang. Biol. 28, 4845–4860. doi: 10.1111/gcb.16229
Frostegård, A., and Bååth, E. (1996). The use of phospholipid fatty acid analysis to estimate bacterial and fungal biomass in soil. Biol. Fert. Soils 22, 59–65. doi: 10.1007/BF00384433
Gao, D., Bai, E., Yang, Y., Zong, S., and Hagedorn, F. (2021). A global meta-analysis on freeze-thaw effects on soil carbon and phosphorus cycling. Soil Biol. Biochem. 159:108283. doi: 10.1016/j.soilbio.2021.108283
Gelfand, I., Cui, M., Tang, J., and Robertson, G. P. (2015). Short-term drought response of N2O and CO2 emissions from Mesic agricultural soils in the US Midwest. Agric. Ecosyst. Environ. 212, 127–133. doi: 10.1016/j.agee.2015.07.005
Gentile, R., Vanlauwe, B., Chivenge, P., and Six, J. (2008). Interactive effects from combining fertilizer and organic residue inputs on nitrogen transformations. Soil Biol. Biochem. 40, 2375–2384. doi: 10.1016/j.soilbio.2008.05.018
Hao, X., Han, X., Wang, S., and Li, L.-J. (2022). Dynamics and composition of soil organic carbon in response to 15 years of straw return in a Mollisol. Soil Till. Res. 215:105221. doi: 10.1016/j.still.2021.105221
Hartmann, A. A., Barnard, R. L., Marhan, S., and Niklaus, P. A. (2013). Effects of drought and N-fertilization on N cycling in two grassland soils. Oecologia 171, 705–717. doi: 10.1007/s00442-012-2578-3
Hu, X., Gu, H., Liu, J., Wei, D., Zhu, P., Cui, X., et al. (2022). Metagenomics reveals divergent functional profiles of soil carbon and nitrogen cycling under long-term addition of chemical and organic fertilizers in the black soil region. Geoderma 418:115846. doi: 10.1016/j.geoderma.2022.115846
Ji, X., Liu, M., Yang, J., and Feng, F. (2022). Meta-analysis of the impact of freeze-thaw cycles on soil microbial diversity and C and N dynamics. Soil Biol. Biochem. 168:108608. doi: 10.1016/j.soilbio.2022.108608
Joergensen, R. G. (1996). The fumigation-extraction method to estimate soil microbial biomass: calibration of the kEC value. Soil Biol. Biochem. 28, 25–31. doi: 10.1016/0038-0717(95)00102-6
Joergensen, R. G., and Wichern, F. (2008). Quantitative assessment of the fungal contribution to microbial tissue in soil. Soil Biol. Biochem. 40, 2977–2991. doi: 10.1016/j.soilbio.2008.08.017
Leckie, S. E. (2005). Methods of microbial community profiling and their application to forest soils. Forest Ecol. Manag. 220, 88–106. doi: 10.1016/j.foreco.2005.08.007
Legay, N., Grassein, F., Arnoldi, C., Segura, R., Laîné, P., Lavorel, S., et al. (2020). Studies of NH4+ and NO3− uptake ability of subalpine plants and resource-use strategy identified by their functional traits. Oikos 129, 830–841. doi: 10.1111/oik.07282
Li, J., Liu, Y., Hai, X., Shangguan, Z., and Deng, L. (2019). Dynamics of soil microbial C: N: P stoichiometry and its driving mechanisms following natural vegetation restoration after farmland abandonment. Sci. Total Environ. 693:133613. doi: 10.1016/j.scitotenv.2019.133613
Li, J., Sang, C., Yang, J., Qu, L., Xia, Z., Sun, H., et al. (2021). Stoichiometric imbalance and microbial community regulate microbial elements use efficiencies under nitrogen addition. Soil Biol. Biochem. 156:108207. doi: 10.1016/j.soilbio.2021.108207
Lin, S., and Hernandez-Ramirez, G. (2022). Increased soil-derived N2O production following a simulated fall-freeze-thaw cycle: effects of fall urea addition, soil moisture, and history of manure applications. Biogeochemistry 157, 379–398. doi: 10.1007/s10533-021-00880-x
Liu, C., Wang, Y., Chen, H., Sun, Q., Jiang, Q., and Wang, Z. (2023). High level of winter warming aggravates soil carbon, nitrogen loss and changes greenhouse gas emission characteristics in seasonal freeze-thaw farmland soil. Sci. Total Environ. 905:167180. doi: 10.1016/j.scitotenv.2023.167180
Liu, C., Wang, L., Song, X., Chang, Q., Frank, D. A., Wang, D., et al. (2018). Towards a mechanistic understanding of the effect that different species of large grazers have on grassland soil N availability. J. Ecol. 106, 357–366. doi: 10.1111/1365-2745.12809
Liu, J., Zhang, J., Li, D., Xu, C., and Xiang, X. (2020). Differential responses of arbuscular mycorrhizal fungal communities to mineral and organic fertilization. Microbiol. Open 9:e00920. doi: 10.1002/mbo3.920
Luo, W., Dijkstra, F. A., Bai, E., Feng, J., Lü, X.-T., Wang, C., et al. (2015). A threshold reveals decoupled relationship of sulfur with carbon and nitrogen in soils across arid and semi-arid grasslands in northern China. Biogeochemistry 127, 141–153. doi: 10.1007/s10533-015-0174-4
Makoto, K., Kajimoto, T., Koyama, L., Kudo, G., Shibata, H., Yanai, Y., et al. (2014). Winter climate change in plant-soil systems: summary of recent findings and future perspectives. Ecol. Res. 29, 593–606. doi: 10.1007/s11284-013-1115-0
Manzoni, S., Taylor, P., Richter, A., Porporato, A., and Ågren, G. I. (2012). Environmental and stoichiometric controls on microbial carbon-use efficiency in soils. New Phytol. 196, 79–91. doi: 10.1111/j.1469-8137.2012.04225.x
Matulich, K. L., Weihe, C., Allison, S. D., Amend, A. S., Berlemont, R., Goulden, M. L., et al. (2015). Temporal variation overshadows the response of leaf litter microbial communities to simulated global change. ISME J. 9, 2477–2489. doi: 10.1038/ismej.2015.58
Mehnaz, K. R., Corneo, P. E., Keitel, C., and Dijkstra, F. A. (2019). Carbon and phosphorus addition effects on microbial carbon use efficiency, soil organic matter priming, gross nitrogen mineralization and nitrous oxide emission from soil. Soil Biol. Biochem. 134, 175–186. doi: 10.1016/j.soilbio.2019.04.003
Mooshammer, M., Wanek, W., Zechmeister-Boltenstern, S., and Richter, A. A. (2014). Stoichiometric imbalances between terrestrial decomposer communities and their resources: mechanisms and implications of microbial adaptations to their resources. Front. Microbiol. 5:22. doi: 10.3389/fmicb.2014.00022
Nguyen, L. T., Osanai, Y., Lai, K., Anderson, I. C., Bange, M. P., Tissue, D. T., et al. (2018). Responses of the soil microbial community to nitrogen fertilizer regimes and historical exposure to extreme weather events: flooding or prolonged-drought. Soil Biol. Biochem. 118, 227–236. doi: 10.1016/j.soilbio.2017.12.016
Okano, Y., Hristova, K. R., Leutenegger, C. M., Jackson, L. E., Denison, R. F., Gebreyesus, B., et al. (2004). Application of real-time PCR to study effects of ammonium on population size of ammonia-oxidizing bacteria in soil. Appl. Environ. Microb. 70, 1008–1016. doi: 10.1128/AEM.70.2.1008-1016.2004
R Development Core Team (2023). R: A language and environment for statistical. (Vienna, Austria: R Foundation for Statistical Computing). Available at: http://www.R-project.org.
Schleuss, P. M., Widdig, M., Biederman, L. A., Borer, E. T., Crawley, M. J., Kirkman, K. P., et al. (2021). Microbial substrate stoichiometry governs nutrient effects on nitrogen cycling in grassland soils. Soil Biol. Biochem. 155:108168. doi: 10.1016/j.soilbio.2021.108168
Shen, J., Tao, Q., Dong, Q., Luo, Y., Luo, J., He, Y., et al. (2021). Long-term conversion from rice-wheat to rice-vegetable rotations drives variation in soil microbial communities and shifts in nitrogen-cycling through soil profiles. Geoderma 404:115299. doi: 10.1016/j.geoderma.2021.115299
Shipley, B., and Tardif, A. (2021). Causal hypotheses accounting for correlations between decomposition rates of different mass fractions of leaf litter. Ecology 102:e03196. doi: 10.1002/ecy.3196
Silva-Sánchez, A., Soares, M., and Rousk, J. (2019). Testing the dependence of microbial growth and carbon use efficiency on nitrogen availability, pH, and organic matter quality. Soil Biol. Biochem. 134, 25–35. doi: 10.1016/j.soilbio.2019.03.008
Sinsabaugh, R. L., and Follstad Shah, J. J. (2012). Ecoenzymatic stoichiometry and ecological theory. Annu. Rev. Ecol. Evol. Syst. 43, 313–343. doi: 10.1146/annurev-ecolsys-071112-124414
Sinsabaugh, R. L., Turner, B. L., Talbot, J. M., Waring, B. G., Powers, J. S., Kuske, C. R., et al. (2016). Stoichiometry of microbial carbon use efficiency in soils. Ecol. Monogr. 86, 172–189. doi: 10.1890/15-2110.1
Song, Y., Zou, Y., Wang, G., and Yu, X. (2017). Altered soil carbon and nitrogen cycles due to the freeze-thaw effect: a meta-analysis. Soil Biol. Biochem. 109, 35–49. doi: 10.1016/j.soilbio.2017.01.020
Spohn, M., Pötsch, E. M., Eichorst, S. A., Woebken, D., Wanek, W., and Richter, A. (2016). Soil microbial carbon use efficiency and biomass turnover in a long-term fertilization experiment in a temperate grassland. Soil Biol. Biochem. 97, 168–175. doi: 10.1016/j.soilbio.2016.03.008
Sun, L., Li, J., Qu, L., Wang, X., Sang, C., Wang, J., et al. (2023). Phosphorus limitation reduces microbial nitrogen use efficiency by increasing extracellular enzyme investments. Geoderma 432:116416. doi: 10.1016/j.geoderma.2023.116416
Sun, Y., Zang, H., Splettstößer, T., Kumar, A., Xu, X., Kuzyakov, Y., et al. (2021). Plant intraspecific competition and growth stage alter carbon and nitrogen mineralization in the rhizosphere. Plant Cell Environ. 44, 1231–1242. doi: 10.1111/pce.13945
Tang, H., Xiao, X., Li, C., Pan, X., Cheng, K., Li, W., et al. (2020). Microbial carbon source utilization in rice rhizosphere and nonrhizosphere soils with short-term manure N input rate in paddy field. Sci. Rep. 10:6487. doi: 10.1038/s41598-020-63639-8
Tao, F., Huang, Y., Hungate, B. A., Manzoni, S., Frey, S. D., Schmidt, M. W., et al. (2023). Microbial carbon use efficiency promotes global soil carbon storage. Nature 618, 981–985. doi: 10.1038/s41586-023-06042-3
Tian, Y., Schindlbacher, A., Malo, C. U., Shi, C., Heinzle, J., Kengdo, S. K., et al. (2023). Long-term warming of a forest soil reduces microbial biomass and its carbon and nitrogen use efficiencies. Soil Biol. Biochem. 184:109109. doi: 10.1016/j.soilbio.2023.109109
Urakawa, R., Shibata, H., Kuroiwa, M., Inagaki, Y., Tateno, R., Hishi, T., et al. (2014). Effects of freeze-thaw cycles resulting from winter climate change on soil nitrogen cycling in ten temperate forest ecosystems throughout the Japanese archipelago. Soil Biol. Biochem. 74, 82–94. doi: 10.1016/j.soilbio.2014.02.022
Van Huynh, V., Ngo, M. T. T., Itayama, T., Nguyen, M. B., Vo, T.-D.-H., Vo, T.-K.-Q., et al. (2023). Dynamic of microbial community in simultaneous nitrification and denitrification process: a review. Bioresour. Technol. Rep. 22:101415. doi: 10.1016/j.biteb.2023.101415
Vankoughnett, M. R., and Henry, H. A. L. (2014). Soil freezing and N deposition: transient vs multi-year effects on plant productivity and relative species abundance. New Phytol. 202, 1277–1285. doi: 10.1111/nph.12734
Watanabe, T., Tateno, R., Imada, S., Fukuzawa, K., Isobe, K., Urakawa, R., et al. (2019). The effect of a freeze-thaw cycle on dissolved nitrogen dynamics and its relation to dissolved organic matter and soil microbial biomass in the soil of a northern hardwood forest. Biogeochemistry 142, 319–338. doi: 10.1007/s10533-019-00537-w
Wiesenbauer, J., König, A., Gorka, S., Marchand, L., Nunan, N., Kitzler, B., et al. (2024). A pulse of simulated root exudation alters the composition and temporal dynamics of microbial metabolites in its immediate vicinity. Soil Biol. Biochem. 189:109259. doi: 10.1016/j.soilbio.2023.109259
Xiao, D., Xiao, L., Che, R., Tan, Y., Liu, X., Yang, R., et al. (2020). Phosphorus but not nitrogen addition significantly changes diazotroph diversity and community composition in typical karst grassland soil. Agric. Ecosyst. Environ. 301:106987. doi: 10.1016/j.agee.2020.106987
Yang, Y., Geng, J., Cheng, S., Fang, H., Guo, Y., Li, Y., et al. (2023). Linking soil microbial community to the chemical composition of dissolved organic matter in a boreal forest during freeze-thaw cycles. Geoderma 431:116359. doi: 10.1016/j.geoderma.2023.116359
Yang, X., He, P., Zhang, Z., You, M., Wu, X., and Li, L.-J. (2023). Straw return, rather than warming, alleviates microbial phosphorus limitation in a cultivated Mollisol. Appl. Soil Ecol. 186:104821. doi: 10.1016/j.apsoil.2023.104821
Yang, X., Henry, H. A., Zhong, S., Meng, B., Wang, C., Gao, Y., et al. (2020). Towards a mechanistic understanding of soil nitrogen availability responses to summer vs. winter drought in a semiarid grassland. Sci. Total Environ. 741:140272. doi: 10.1016/j.scitotenv.2020.140272
Yu, L., Yu, M., Lu, X., Tang, C., Liu, X., Brookes, P. C., et al. (2018). Combined application of biochar and nitrogen fertilizer benefits nitrogen retention in the rhizosphere of soybean by increasing microbial biomass but not altering microbial community structure. Sci. Total Environ. 640-641, 1221–1230. doi: 10.1016/j.scitotenv.2018.06.018
Yuan, X., Niu, D., Gherardi, L. A., Liu, Y., Wang, Y., Elser, J. J., et al. (2019). Linkages of stoichiometric imbalances to soil microbial respiration with increasing nitrogen addition: evidence from a long-term grassland experiment. Soil Biol. Biochem. 138:107580. doi: 10.1016/j.soilbio.2019.107580
Zhang, Z., He, P., Hao, X., Liu, J., Ge, T., and Li, L.-J. (2023). Rare microbial populations as sensitive indicators of bacterial community dissimilarity under different agricultural management practices. Arch. Agron. Soil Sci. 69, 1013–1026. doi: 10.1080/03650340.2022.2049254
Zhang, S., Liu, P., Zhang, S., Mclaughlin, N. B., Jia, S., Huang, D., et al. (2022). Contribution of rhizodeposit associated microbial groups to SOC varies with maize growth stages. Geoderma 422:115947. doi: 10.1016/j.geoderma.2022.115947
Zhang, Y. L., Sun, C. X., Chen, Z. H., Zhang, G. N., Chen, L. J., and Wu, Z. J. (2019). Stoichiometric analyses of soil nutrients and enzymes in a Cambisol soil treated with inorganic fertilizers or manures for 26 years. Geoderma 353, 382–390. doi: 10.1016/j.geoderma.2019.06.026
Zhao, Z. B., He, J. Z., Geisen, S., Han, L. L., Wang, J. T., Shen, J. P., et al. (2019). Protist communities are more sensitive to nitrogen fertilization than other microorganisms in diverse agricultural soils. Microbiome 7:33. doi: 10.1186/s40168-019-0647-0
Zhou, Y., Berruti, F., Greenhalf, C., and Henry, H. A. (2017). Combined effects of biochar amendment, leguminous cover crop addition and snow removal on nitrogen leaching losses and nitrogen retention over winter and subsequent yield of a test crop (Eruca sativa L.). Soil Biol. Biochem. 114, 220–228. doi: 10.1016/j.soilbio.2017.07.023
Keywords: ammonium availability, Mollisol cropland, nitrate availability, phospholipid fatty acid analysis, snow removal, soil microbial characteristics
Citation: Bao W, He P, Han L, Wei X, Feng L, Zhu J, Wang J, Yang X and Li L-J (2024) Soil nitrogen availability and microbial carbon use efficiency are dependent more on chemical fertilization than winter drought in a maize–soybean rotation system. Front. Microbiol. 15:1304985. doi: 10.3389/fmicb.2024.1304985
Edited by:
Matthias Noll, Hochschule Coburg, GermanyReviewed by:
Zengming Chen, Chinese Academy of Sciences (CAS), ChinaLee H. Dietterich, University of California, Los Angeles, United States
Copyright © 2024 Bao, He, Han, Wei, Feng, Zhu, Wang, Yang and Li. This is an open-access article distributed under the terms of the Creative Commons Attribution License (CC BY). The use, distribution or reproduction in other forums is permitted, provided the original author(s) and the copyright owner(s) are credited and that the original publication in this journal is cited, in accordance with accepted academic practice. No use, distribution or reproduction is permitted which does not comply with these terms.
*Correspondence: Xuechen Yang, eWFuZ3h1ZWNoZW5AaWdhLmFjLmNu; Jihua Wang, d2FuZ2ppaHVhMzMzQGhvdG1haWwuY29t