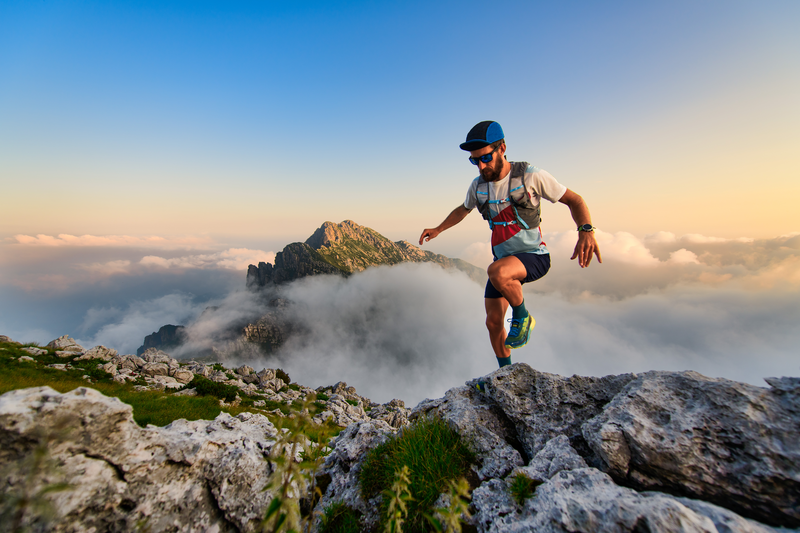
95% of researchers rate our articles as excellent or good
Learn more about the work of our research integrity team to safeguard the quality of each article we publish.
Find out more
ORIGINAL RESEARCH article
Front. Microbiol. , 09 May 2024
Sec. Microbial Symbioses
Volume 15 - 2024 | https://doi.org/10.3389/fmicb.2024.1296512
This article is part of the Research Topic Plant Microbiome: Interactions, Mechanisms of Action, and Applications, Volume III View all 22 articles
Lead (Pb) is a hazardous heavy metal that accumulates in many environments. Phytoremediation of Pb polluted soil is an environmentally friendly method, and a better understanding of mycorrhizal symbiosis under Pb stress can promote its efficiency and application. This study aims to evaluate the impact of two ectomycorrhizal fungi (Suillus grevillei and Suillus luteus) on the performance of Pinus tabulaeformis under Pb stress, and the biomineralization of metallic Pb in vitro. A pot experiment using substrate with 0 and 1,000 mg/kg Pb2+ was conducted to evaluate the growth, photosynthetic pigments, oxidative damage, and Pb accumulation of P. tabulaeformis with or without ectomycorrhizal fungi. In vitro co-cultivation of ectomycorrhizal fungi and Pb shots was used to evaluate Pb biomineralization. The results showed that colonization by the two ectomycorrhizal fungi promoted plant growth, increased the content of photosynthetic pigments, reduced oxidative damage, and caused massive accumulation of Pb in plant roots. The structural characteristics of the Pb secondary minerals formed in the presence of fungi demonstrated significant differences from the minerals formed in the control plates and these minerals were identified as pyromorphite (Pb5(PO4)3Cl). Ectomycorrhizal fungi promoted the performance of P. tabulaeformis under Pb stress and suggested a potential role of mycorrhizal symbiosis in Pb phytoremediation. This observation also represents the first discovery of such Pb biomineralization induced by ectomycorrhizal fungi. Ectomycorrhizal fungi induced Pb biomineralization is also relevant to the phytostabilization and new approaches in the bioremediation of polluted environments.
Lead (Pb) is a hazardous heavy metal that causes severe environmental and human health problems (Rehman et al., 2017; Monchanin et al., 2021). Pb is mainly introduced into soils by human activities such as lead acid battery production, paint production, mining, and leaded petrol production (Jarup, 2003; Li et al., 2014). Pb pollution restricts soil usage and fertility due to the non-degradable and toxic characteristics of Pb (Adriano, 2001). Phytoremediation is an effective and viable method for Pb polluted soils (Sarwar et al., 2017). However, Pb harms plant development and survival due to the production of an excessive amount of reactive oxygen species (ROS) (Reddy et al., 2005; Zhang et al., 2020). Consequently, phytoremediation has some drawbacks, including sluggish development of the accumulator plants, poor biomass production, and low heavy metal absorption, which makes it a lengthy and inefficient procedure (Vergara et al., 2020).
Ectomycorrhizal (ECM) symbiosis is widespread in numerous ecosystems between fungi from Basidiomycota, Ascomycota, and Zygomycota, and the ecologically and economically most important forest trees, including Pinaceae, Fagaceae, Salicaceae, Betulaceae, Caesalpinioideae, Dipterocarpaceae, and Phyllanthaceae (Tedersoo et al., 2010). ECM fungi form Hartig nets inside plant roots, form sheath-like mantles around lateral roots, and form extrametrical mycelia to explore, absorb, and translocate nutrients and water from the surrounding soil. The ECM symbiosis promotes plant nutrition and water uptake, increases plant growth performance, and facilitates the establishment of host plants in harsh environments (Arocena and Glowa, 2000; Baum et al., 2006; Szuba et al., 2017; Wen et al., 2017; Liu et al., 2020). Microbe-enhanced phytoremediation that using ECM is an effective measure for remediating metal-contaminated soils (Shi et al., 2019; Liu et al., 2020). The ECM symbiosis results in enhanced host plants’ tolerance to heavy metals, including alleviation of inhibition of plant photosynthesis caused by heavy metals and a beneficial impact on reducing the metal-induced oxidative stress on plants (Schützendübel and Polle, 2002; Canton et al., 2016; Fernández-Fuego et al., 2017; Mohammadhasani et al., 2017).
In addition to improving the growth and enhancing the heavy metal tolerance of accumulator plants, ECM fungi may play a role in biomineralization. Biomineralization is the process of living organisms’ induced mineral formation. The majority of fungal-involved biomineralization is the consequence of metabolic processes that affect the external environment in a way that facilitates mineral precipitation. Examples of these processes include changes in pH, O2, redox potential, redox transformations of metal species, and excretion of organic and inorganic metabolites like CO2, H+, or organic acids (Gadd, 2021). Fomina et al. (2007) showed that Beauveria caledonica causes uranyl phosphate minerals formation via biomineralization. Bacillus cereus 12-2, which was isolated from lead-zinc mine tailings, could transform the Pb into rod-shaped Ca2.5Pb7.5(OH)2(PO4)6 nanocrystal (Chen et al., 2016). Povedano-Priego et al. (2016) described lead phosphate formation via biomineralization in the interaction of Penicillium chrysogenum with metallic Pb. Phanerochaete chrysoporium participates in Pb biomineralization and transforms Pb into Pb5(PO4)3OH through fungal phosphatase (Zhao et al., 2020).
Pinus tabulaeformis Carr. is one of the most widely distributed pines in northern China (Chen et al., 2008) and it has remarkable drought endurance and great adaptation to poor soil (Wang and Guo, 2010). The well-known ectomycorrhizal species P. tabuliformis, characterized by its strong mycorrhizal dependency and potential colonization by various fungal species (Allen, 1991), can be effectively used for restoration in post-mining areas (Yan et al., 2020; Zhang et al., 2023). It was found that the dominant ECM fungi in the rhizosphere soil of P. tabuliformis, which belongs to the genus Suillus (Wang et al., 2022), serves as a model system for understanding mycorrhizal fungal metal tolerance (Branco et al., 2022). Although there were studies showing that pines with ectomycorrhizal fungi promoted tolerance against heavy metals (Bizo et al., 2017; Liu et al., 2020; Ouatiki et al., 2022), the response of the symbiosis between P. tabulaeformis and ectomycorrhizal fungi to Pb pollution was rarely reported. In this study, we used two ectomycorrhizal fungi (Suillus luteus and Suillus grevillei) to evaluate their ability to (1) form symbiosis with P. tabuleaformis, (2) improve plant growth, (3) promote the activity of antioxidant enzymes and photosynthetic pigment content, (4) regulate Pb uptake and distribution, and (5) biomineralize Pb in vitro.
The ECM fungi (Suillus luteus, NCBI: txid5384 and Suillus grevillea, NCBI: txid5382) were stored in the microbiology lab of the Forestry College, Northwest A&F University. These strains were originally isolated from the ectomycorrhizae of P. tabulaeformis in Ningshan county, Shaanxi Province, China. These fungi were grown on Modified Melin-Norkrans (MMN) solid medium (Fomina et al., 2005). After 2 weeks of growth, four blocks of media for each ECM fungus (1 cm in diameter) were inoculated in 300 mL MMN liquid medium. After a shaking culture (25°C, 150 rpm in darkness) of ECM for 21 days, the mycelia were obtained by filtering. The mycelia were washed five times with sterilized water, homogenized by blender with 500 mL sterilized water, and used as inoculum.
The seeds of Pinus tabulaeformis were obtained from the Forestry Technology Extension Station of the Forestry Department, Shaanxi Province, China. The seeds were surface disinfected by 0.5% KMnO4 for 10 min, washed 3 times with sterilized water, and immersed in sterilized water at 45°C for 1 h. Afterward, seeds were germinated on wet filter papers in Petri dishes at room temperature (20–25°C) in darkness. Germinated seeds were transplanted in seedling trays (50 mL) filled with sterilized vermiculite and cultivated in a greenhouse with a temperature of 20–30°C, a photoperiod of 12 h.
Inoculation of ECM was performed 1 week after the transplantation of the germinated seeds of P. tabulaeformis. Inoculation was achieved by injecting 10 mL inoculum to the base of seedling in the seedling tray. The nonmycorrhizal seedlings received autoclaved inoculum. Inoculation repeated 3 times with interval of 2 weeks. The seedlings were daily watered with deionized water and weekly fertilized with 10 mL of Hoagland’s nutrient solution (Zhang et al., 2020). The success of ECM fungal colonization was proven by the observation of mycorrhizal structure under the microscope after 3 months (not quantified).
For the pot experiment, the substrate was a mixture of quartz river sand, vermiculite, and soil in a ratio of 1:1:1 (v: v: v). The soil was collected from the top layer of the Northwest A&F University campus nursery in Yangling, Shaanxi Province, China. The main soil nutrient characteristics were as follows: 16.15 g/kg organic matter, 30.35 mg/kg available nitrogen, 20.40 mg/kg available phosphorus, and 126.36 mg/kg available potassium. The background value of Pb concentration in soil was 15.83 mg/kg. Soil was ground, passed through a 2 mm sieve, and mixed with thoroughly washed sand and vermiculite. The substrate was autoclaved at 0.11 MPa and 121°C for 2 h.
The Pb2+ concentration in the substrate was adjusted to 1,000 mg/kg by spraying 100 mM Pb(NO3)2 solution to substrate according to the risk intervention values for soil contamination of agricultural land (Soil environmental quality-Risk control standard for soil contamination of agricultural land, GB 15618-2018). The corresponding amount of NH4NO3 was added to the control group to make up for the difference in nitrogen content caused by the addition of Pb(NO3)2. The substrate was used 1 month after the addition of Pb for equilibration.
The experiment consisted of 2 factors (3 × 2): mycorrhizal status, inoculated with S. luteus (SL), S. grevillea (SG), or not (CK); Pb status, extra Pb was mixed with the substrate (1,000 mg/kg, Pb-treated) or not (0 mg/kg, Pb-free). Three randomly selected mycorrhizal or nonmycorrhizal seedlings were transplanted in a plastic pot filled with 0.4 kg Pb-treated or Pb-free substrate and cultivated for 4 months. There were 6 treatments, and 3 replicates for each treatment.
The pot experiment was conducted in a greenhouse at a temperature of 20–35°C, a photoperiod of 12–14 h, and a relative air humidity of 55–78%. The plants were daily watered with deionized water and fertilized every 2 weeks with 50 mL of Hoagland’s nutrient solution.
Four months after the transplantation of seedlings in pots, seedling shoots and roots were harvested separately. Seedling roots were carefully washed with tap water to remove all soil particles and dried with paper towels. The fresh shoot and root weight were recorded. The shoot and root were then randomly divided into 4 parts. One part of the shoot and root was oven dried at 80°C until constant weight and was used to calculate the fresh-to-dry mass ratio. The shoot and root dry weight was calculated according to the fresh weight and fresh-to-dry mass ratio. One part of the shoot and root was oven dried and used to measure the Pb concentration. One part of the shoot was used to measure the photosynthetic pigments content. One part of the root was used to measure the mycorrhizal colonization rate. The rest shoot and root samples were frozen by liquid nitrogen and stored in a refrigerator at −80°C.
The ectomycorrhizal colonization rate was calculated according to the cross-griding method (Püttsepp et al., 2004) after roots were stained with trypan blue (Phillips and Hayman, 1970). Twenty 1 cm root segments were analyzed and a total of 200 intersections were counted for a single replicate.
Pb concentration was analyzed with a microwave mineralizer (Multiwave PRO, Anton Paar, GmbH, Austria) and a flame atomic absorption spectrophotometer (AA7000, Shimadzu, Japan) (Vergara et al., 2020).
Chlorophyll a, chlorophyll b, and carotenoids were determined according to the method described by Lichtenthaler and Wellburn (1983).
Fresh shoots and roots that stored in a refrigerator (−80°C) were ground to powder under liquid nitrogen (three biological replicates) for plant antioxidant capacity and oxidative stress assay. The H2O2 and MDA level was determined with the trichloroacetic acid (TAC) test and the thiobarbituric acid (TBA) test, respectively, according to Velikova et al. (2000). The enzymatic activity (SOD, POD, and CAT) was calculated as the previous study described (Martins et al., 2011; Fernández-Fuego et al., 2017).
In order to assess the Pb transformation by ectomycorrhizal fungi, co-culture of Pb shots (4 mm in diameter) and ectomycorrhizal fungi was carried out in Petri dishes (9 cm in diameter) containing MMN solid medium. The Pb shots were autoclaved and evenly placed on the MMN solid medium, while a block of ectomycorrhizal fungal mycelia taken from the edge of a growing colony was placed on the surface of the MMN solid medium. Dishes that received only Pb shots were used as controls. The Petri dishes were incubated at 25°C in darkness for 2 months.
The elemental composition of secondary minerals formed on the Pb shot surface was analyzed with energy dispersive spectroscopy (EDS, AMETEK, United States). Images of secondary minerals on the Pb shot surface were obtained after the Pb shots were sprayed with gold/palladium using an ion sputter (MC1000, Hitachi, Japan) and then used for SEM analysis by a field emission scanning electron microscope (S-4800, Hitachi, Japan).
The composition of secondary minerals generated on the Pb shot surface was determined using X-ray diffraction (XRD) analysis (Zhao et al., 2020). Mineral phases were identified according to International Centre for Diffraction Data Powder Diffraction File (PDF-4 release 2010).
IBM SPSS® Statistics was used for data analysis (SPSS Version 26, SPSS Inc., United States). Two- and one-way analyses of variance (ANOVA) with Tukey’s honest significant difference (HSD) tests at p < 0.05 were used to confirm statistical significance (Zhao et al., 2020).
After 4 months of pot culture, plant biomass and ECM fungi colonization rate were recorded (Table 1). Under Pb-free condition, inoculation of S. grevillea improved the shoots biomass and total biomass, while inoculation of S. luteus did not contribute to plant growth. Pb addition decreased the growth of P. tabulaeformis. Under Pb-treated condition, the plants colonized by S. grevillea showed superiority in shoots, roots, and total biomass compared with nonmycorrhizal plants, while the improvement of plant biomass by S. luteus was not obvious.
Table 1. Plant growth and ectomycorrhizal colonization of P. tabuliformis 4 months after inoculation.
No mycorrhizal structure was observed in nonmycorrhizal plants. Pb addition decreased the colonization rate of S. luteus but not S. grevillea. More than 50% of the P. tabulaeformis roots were colonized by both ECM fungi, and S. grevillea colonized at a greater rate than S. luteus under both Pb-treated and Pb-free conditions.
Pb treatment and two ECM fungi inoculation had a significant influence on plant Pb concentration and content (Table 2). The Pb concentration in plant shoots and roots was significantly increased after Pb addition. Inoculation with S. grevillea and S. luteus increased Pb concentration in roots and decreased Pb concentration in shoots at Pb-treated condition, which increased 43.6% (SG) and 21.9% (SL, not significant) in roots and decreased 37.1% (SG) and 40.6% (SL) in shoots.
The chlorophyll a concentration was decreased by Pb addition (Figure 1). Compared with nonmycorrhizal plants, S. grevillea inoculated plants had a significant increased chlorophyll a concentration under Pb-free and Pb-treated conditions. For S. luteus inoculated plants, a significant increase in the chlorophyll a concentration was observed under Pb-free condition, while there was no superiority after Pb addition. The chlorophyll b and carotenoids concentrations of needles were not decreased by Pb addition (comparing plants grown in Pb-free or Pb-treated conditions). The chlorophyll b and carotenoids concentrations increased in the needles of S. grevillea colonized plants.
Figure 1. The chlorophyll a (A), chlorophyll b (B), and carotenoid (C) content in shoots of P. tabuliformis, grown for 4 months in Pb-free treatment (0) or Pb addition (1,000) treatment. CK for nonmycorrhizal and SG and SL for inoculation with Suillus grevillei and Suillus luteus. Values are presented as means ± SD (n = 3). Different letters indicate significant differences at p < 0.05 by Tukey’s test.
The activity of SOD in plant tissue was not influenced by Pb stress (comparing plants grown in Pb-free or Pb-treated conditions). A considerable rise in SOD activity was observed in the plants inoculated with the S. grevillea and S. luteus (Figure 2A). Pb stress considerably increased POD activity (comparing plants grown in Pb-free or Pb-treated conditions) in plant tissue. The POD activity in plant tissue further increased significantly for both fungi colonization under Pb stress but showed no difference under Pb-free conditions compared with nonmycorrhizal plants (Figure 2B). The CAT activity in nonmycorrhizal plant tissue was not influenced by Pb stress. Under 2 Pb conditions, a significant increase in CAT activity was observed in the plants that had been colonized by the S. grevillea. When exposed to Pb, S. luteus colonized plants showed a considerable increase in CAT activity, but not in Pb-free circumstances. CAT activity from S. grevillea colonized plants was significantly higher than S. luteus colonized plants (Figure 2C).
Figure 2. Enzymatic activities of superoxide dismutase (SOD) (A), peroxidase (POD) (B), catalase (CAT) (C) and the content of H2O2 (D) and MDA (E) in shoots of P. tabuliformis, grown for 4 months in Pb-free treatment (0) or Pb addition (1,000) treatment. CK for nonmycorrhizal and SG and SL for inoculation with Suillus grevillei and Suillus luteus. Values are presented as means ± SD (n = 3). Different letters indicate significant differences at p < 0.05 by Tukey’s test.
Pb addition increased H2O2 concentrations in plant tissue. The H2O2 concentration in plant tissue was significantly decreased by both fungi (about 41.6 and 48.4% by S. grevillea, about 21.0 and 19.4% by S. luteus under Pb-free or Pb-treated conditions respectively) (Figure 2D). Pb addition increased the MDA level in plant tissue. The MDA concentration in plant tissue was decreased by both fungi (about 37.8 and 65.1% by S. grevillea, about 33.4 and 50.8% by S. luteus under Pb-free or Pb-treated conditions respectively) (Figure 2E).
Two months after incubation, S. grevillea and S. luteus grown on the MMN medium with Pb shots (Figure 3). The Pb shots in control medium became milky, while color change of the Pb shots in medium with S. grevillea or S. luteus was not that obvious.
Figure 3. Pb shots on MMN medium without ECM fungi (A); Pb shots on MMN medium with Suillus grevillei (B); Pb shots on MMN medium with Suillus luteus (C).
Flakes-shaped secondary minerals were observed on the Pb shots in control medium by SEM (Figures 4A,B). Co-culture of Pb shots and S. grevillea resulted in rod-shaped and spindle-shaped secondary minerals (Figures 4C–E). Co-culture of Pb shots and S. luteus resulted in botryoidal shaped (Figure 4F), acicular shaped (Figure 4G), globular shaped (Figure 4H), and granular shaped (Figure 4I) secondary minerals.
Figure 4. SEM images of the secondary minerals on Pb shot surface at different treatment (A,B, non-inoculation; C–E, Suillus grevillei inoculation; F–I, Suillus luteus inoculation).
Inoculation of two ectomycorrhizal fungi changed the elemental composition of secondary minerals on Pb shots (Figure 5). Carbon (C), oxygen (O), and Pb were the elements on Pb shots in control medium, while phosphorus (P) and chlorine (Cl) appeared along with C, O, and Pb when ectomycorrhizal fungi were in the medium.
Figure 5. Elemental composition of secondary minerals on the Pb shots after different treatment. wt% and at% refer to weight percentage and atomic percentage of a particular element, respectively. (A) The elemental composition of non-inoculation treatment showed that secondary minerals were comprised of C, O, and Pb. (B) The elemental composition of Suillus grevillei inoculation treatment showed that secondary minerals were comprised of C, O, P, Pb and Cl. (C) The elemental composition of Suillus luteus inoculation treatment showed that secondary minerals were comprised of C, O, P, Pb, and Cl. The “inset” indicates the weight percentage and atomic percentage of different elements on the Pb shots surface.
The XRD spectra of secondary minerals at different treatments had abundant spectral line characteristics, indicating that the secondary minerals were crystal. The diffraction peaks of secondary minerals were observed and could be identified as PbO, Pb2O3, Pb3(CO3)2(OH)2 in control treatment and PbO, Pb2O3, Pb3(CO3)2(OH)2, Pb5(PO4)3Cl in two ECM fungi treatment (Figure 6).
Figure 6. XRD spectrum of the secondary minerals on Pb shots surface at different treatment (A, non-inoculation; B, Suillus grevillei inoculation; C, Suillus luteus inoculation).
Mycorrhizal plants acquire water and mineral nutrients via fungi hyphae, while supply organic nutrients to fungi (Brundrett, 2002; Kaiser et al., 2010; Pena and Polle, 2014). In this study, we observed an increase in the biomass of P. tabulaeformis inoculated with S. grevillea under both Pb-treated and Pb-free treatment. This improvement caused by S. grevillea can be explained by greater mineral absorption (Canton et al., 2016; Sun et al., 2021) and Pb tolerance (Baum et al., 2006). However, the effect of S. luteus on the growth of P. tabulaeformis was not obvious. Compared with ECM fungi sensitive to heavy metals, the tolerant strains are reported to be more effective in enhancing the metal tolerance and growth of host plants (Adriaensen et al., 2006; Szuba et al., 2017). Thus, we speculated that S. luteus exhibit lower Pb tolerance compared with S. grevillea, which can be corroborated by the fact that the S. luteus colonization rate significantly decreased under Pb Stress (Table 1). Meanwhile, slower growth of S. luteus than S. grevillea was observed on MMN agar medium with lead shots (Figure 3).
The Pb stress response in both ECM fungi treatments showed improvement in P. tabulaeformis, with a decrease in lipid peroxidation (shown by MDA level) and reactive oxygen species (shown by H2O2 concentration) and a significant improvement in antioxidant defense (shown by SOD, POD, and CAT activity). Our findings support the early studies that discovered the promotion of ECM symbiosis through the rise in antioxidant activities in their host plants against Cb and Al stress (Liu et al., 2020; Sun et al., 2021). In this work, S. grevillea inoculation resulted in a considerable rise for carotenes or chlorophylls but not S. luteus, which could be related to the different properties of fungi, as mentioned above. This result is supported by the findings of Szuba et al. (2017), with more chlorophylls and carotenes increments of Populus × canescens colonized by the Pb-tolerant Paxillus involutus. Our results supported the idea that inoculating host plants with ECM fungus considerably increases their Pb tolerance and shields them from heavy metal stress (Szuba et al., 2017).
Pb is a weakly translocated metal. Up to 90% of the Pb absorbed by plants is accumulated in their roots (Kumar et al., 1995; Fahr et al., 2013). The accumulation of Pb in plant roots has been found to be higher than in shoots, particularly in mycorrhizal seedlings where Pb accumulation in roots was significantly elevated compared to non-mycorrhizal seedlings (Table 2). This finding is consistent with previous studies (Bojarczuk et al., 2015; Gu et al., 2017; Hachani et al., 2020; Liu et al., 2020) which have suggested that excess Pb is sequestered through biomineralization in fungus hyphae, often along with colonized roots for analysis. ECM fungi play a crucial role in lead homeostasis within ecosystems by accumulating a substantial amount of lead through their mycelium (Liu et al., 2020; Branco et al., 2022). These mechanisms involve the storage of excessive metals in compartments within cells (González-Guerrero et al., 2008; Ruytinx et al., 2013), and the capture of these metals by proteins or metabolites in ECM fungi (González-Guerrero et al., 2007; Leonhardt et al., 2014). Additionally, excess metals can be exclusion by ECM fungi (Ruytinx et al., 2013; Majorel et al., 2014). Various metal transporters and chelating proteins in mycorrhizal fungi have been functionally studied, providing insight into their role in reducing the harmful effects of excessive environmental metals (Leonhardt et al., 2014; Coninx et al., 2017; Ruytinx et al., 2017; Gómez-Gallego et al., 2019).
To investigate potential interactions between metallic Pb and the ECM fungi, two ECM fungi were incubated with a Pb shot. It was clear from the structural variations between the Pb secondary minerals generated in the presence of the fungus and those formed in the control plates that the fungi had a role in their formation. Subsequently, the particular mineral was identified as pyromorphite (Pb5(PO4)3Cl). Under general geochemical conditions, pyromorphite is the most stable environmental Pb compound (Ksp = 10–79.6 ± 0.15) (Topolska et al., 2016) and pyromorphite deposition has consequently been proposed as a remediation treatment for Pb-contained soil (Miretzky and Fernandez-Cirelli, 2008).
In the natural environment, pyromorphite generation depends on various chemical and biological processes (Rhee et al., 2012). A previous study has demonstrated that Paecilomyces javanicus participates in the biomineralization of Pb metal and transforms Pb into pyromorphite and the authors speculated that this phenomenon is linked with microbial transformations of inorganic and organic phosphorus by extracellular acid phosphatases secreted by fungal (Rhee et al., 2012; Liang et al., 2015). This process produced the main ligands (PO43−) of Pb, which may contribute to biologically induced pyromorphite formation. The variation of pH could change the dissolution of the Pb and P sources and oxidation–reduction potential (Eh), which affect the efficacy of pyromorphite formation (Chen et al., 2003; Ehrlich and Newman, 2009). Fungi can produce organic acids, which can mobilize metal ions while changing pH (Adeyemi and Gadd, 2005). Similarly, we observed less Pb precipitation in the control medium, which may be attributed to the mobilization of PbO, Pb2O3, and Pb3(CO3)2(OH)2 by organic acids secreted by fungal hyphae.
In vivo and in vitro growth conditions may alter the nature of the ligands provided by fungal hyphae. However, here we are lacking information on ECM fungi’ effects on Pb biomineralization in the symbiotic state, but Fomina et al. (2005) reported that ECM fungus provides the same ligands of functional groups (e.g., phosphate, carboxylate, sulfhydryl) in both pure culture and ectomycorrhizal association. Thus, it is likely that ECM fungi hyphae in a symbiotic relationship would also provide functional ligands for Pb precipitation.
Our results revealed a notable, positive impact of inoculating S. grevillei and S. luteus on P. tabulaeformis growth and Pb tolerance, which enhances the establishment of remediation plants under Pb-contaminated soil. Our in vitro experiments confirmed that ECM fungi participated in the bio-mineralization of Pb into pyromorphite. As such, our corroboration of pyromorphite formation represents the first discovery of such Pb biomineralization induced by ECM fungi. This observation increases our understanding of the biogeochemical cycle of Pb. ECM fungi induced Pb biomineralization is also relevant to the new approaches in the bioremediation of polluted environments. Predictably, the space between the soil particles, which is unreachable for the roots, is now filled with hyphae of ECM fungi, meaning more extensive and efficient Pb phytostabilization.
The original contributions presented in the study are included in the article/supplementary material, further inquiries can be directed to the corresponding authors.
KC: Conceptualization, Data curation, Formal Analysis, Investigation, Writing – original draft. YL: Investigation, Writing – original draft. MT: Project administration, Supervision, Writing – review & editing. HZ: Conceptualization, Data curation, Funding acquisition, Project administration, Supervision, Writing – review & editing.
The author(s) declare that financial support was received for the research, authorship, and/or publication of this article. This work was supported by the National Natural Science Foundation of China (42277027, 31700530); the Laboratory of Lingnan Modern Agriculture Project (NZ2021025); and the State Key Laboratory for Conservation and Utilization of Subtropical Agro-bioresources (SKLCUSA-b202007).
The authors declare that the research was conducted in the absence of any commercial or financial relationships that could be construed as a potential conflict of interest.
All claims expressed in this article are solely those of the authors and do not necessarily represent those of their affiliated organizations, or those of the publisher, the editors and the reviewers. Any product that may be evaluated in this article, or claim that may be made by its manufacturer, is not guaranteed or endorsed by the publisher.
Adeyemi, A. O., and Gadd, G. M. (2005). Fungal degradation of calcium-, lead- and silicon-bearing minerals. Biometals 18, 269–281. doi: 10.1007/s10534-005-1539-2
Adriaensen, K., Vangronsveld, J., and Colpaert, J. V. (2006). Zinc-tolerant Suillus bovinus improves growth of Zn-exposed Pinus sylvestris seedlings. Mycorrhiza 16, 553–558. doi: 10.1007/s00572-006-0072-7
Adriano, D. C. (2001). Trace elements in terrestrial environments: Biogeochemistry, bioavailability and risk of metals. 2nd Edn. New York: Springer, 349–410.
Arocena, M. J., and Glowa, R. K. (2000). Mineral weathering in ectomycorrhizosphere of subalpine fir (Abies lasiocarpa (hook.) Nutt.) as revealed by soil solution composition. For. Ecol. Manag. 133, 61–70. doi: 10.1016/S0378-1127(99)00298-4
Baum, C., Hrynkiewicz, K., Leinweber, P., and Meißner, R. (2006). Heavy-metal mobilization and uptake by mycorrhizal and nonmycorrhizal willows (Salix ×dasyclados). J. Plant Nutr. Soil Sci. 169, 516–522. doi: 10.1002/jpln.200521925
Bizo, M. L., Nietzsche, S., Mansfeld, U., Langenhorst, F., Majzlan, J., Göttlicher, J., et al. (2017). Response to lead pollution: mycorrhizal Pinus sylvestris forms the biomineral pyromorphite in roots and needles. Environ. Sci. Pollut. R. 24, 14455–14462. doi: 10.1007/s11356-017-9020-7
Bojarczuk, K., Karliński, L., Hazubska-Przybył, T., and Kieliszewska-Rokicka, B. (2015). Influence of mycorrhizal inoculation on growth of micropropagated Populus × canescens lines in metal-contaminated soils. New For. 46, 195–215. doi: 10.1007/s11056-014-9455-3
Branco, S., Schauster, A., Liao, H. L., and Ruytinx, J. (2022). Mechanisms of stress tolerance and their effects on the ecology and evolution of mycorrhizal fungi. New Phytol. 235, 2158–2175. doi: 10.1111/nph.18308
Brundrett, M. C. (2002). Coevolution of roots and mycorrhizas of land plants. New Phytol. 154, 275–304. doi: 10.1046/j.1469-8137.2002.00397.x
Canton, G. C., Bertolazi, A. A., Cogo, A. J. D., Eutrópio, F. J., Melo, J., Sávio, B. S., et al. (2016). Biochemical and ecophysiological responses to manganese stress by ectomycorrhizal fungus Pisolithus tinctoriusand in association with Eucalyptus grandis. Mycorrhiza 26, 475–487. doi: 10.1007/s00572-016-0686-3
Chen, K., Abbott, R. J., Milne, R. I., Tian, X. M., and Liu, J. (2008). Phylogeography of Pinus tabuliformis Carr (Pinaceae), a dominant species of coniferous forest in northern China. Mol. Ecol. 17, 4276–4288. doi: 10.1111/j.1365-294X.2008.03911.x
Chen, M., Ma, L. Q., Singh, S. P., Cao, R. X., and Melamed, R. (2003). Field demonstration of in situ immobilization of soil Pb using P amendments. Adv. Environ. Res. 8, 93–102. doi: 10.1016/S1093-0191(02)00145-4
Chen, Z., Pan, X., Chen, H., Guan, X., and Lin, Z. (2016). Biomineralization of Pb (II) into Pb-hydroxyapatite induced by Bacillus cereus 12-2 isolated from Lead-zinc mine tailings. J. Hazard. Mater. 301, 531–537. doi: 10.1016/j.jhazmat.2015.09.023
Coninx, L., Thoonen, A., Slenders, E., Morin, E., Arnauts, N., Op De Beeck, M., et al. (2017). The SlZRT1 gene encodes a plasma membrane-located ZIP (Zrt-, Irt-like protein) transporter in the ectomycorrhizal fungus Suillus luteus. Front. Microbiol. 8:2320. doi: 10.3389/fmicb.2017.02320
Ehrlich, H. L., and Newman, D. K. (2009). Geomicrobiology. 4th Edn. Boca Raton, FL: CRC Press/Taylor and Francis Group.
Fahr, M., Laplaze, L., Bendaou, N., Hocher, V., Mzibri, M. E., Bogusz, D., et al. (2013). Effect of lead on root growth. Front. Plant Sci. 4:175. doi: 10.3389/fpls.2013.00175
Fernández-Fuego, D., Keunen, E., Cuypers, A., Bertrand, A., and González, A. (2017). Mycorrhization protects Betula pubescens Ehr. From metal-induced oxidative stress increasing its tolerance to grow in an industrial polluted soil. J. Hazard. Mater. 336, 119–127. doi: 10.1016/j.jhazmat.2017.04.065
Fomina, M. A., Alexander, I. J., Colpaert, J. V., and Gadd, G. M. (2005). Solubilization of toxic metal minerals and metal tolerance of mycorrhizal fungi. Soil Biol. Biochem. 37, 851–866. doi: 10.1016/j.soilbio.2004.10.013
Fomina, M. A., Charnock, J. M., Hillier, S., Alvarez, R., and Gadd, G. M. (2007). Fungal transformations of uranium oxides. Environ. Microbiol. 9, 1696–1710. doi: 10.1111/j.1462-2920.2007.01288.x
Gadd, G. M. (2021). Fungal biomineralization. Curr. Biol. 31, R1557–R1563. doi: 10.1016/j.cub.2021.10.041
Gómez-Gallego, T., Benabdellah, K., Merlos, M. A., Jiménez-Jiménez, A. M., Alcon, C., Berthomieu, P., et al. (2019). The Rhizophagus irregularis genome encodes two CTR copper transporters that mediate cu import into the cytosol and a CTR-like protein likely involved in copper tolerance. Front. Plant Sci. 10:604. doi: 10.3389/fpls.2019.00604
González-Guerrero, M., Cano, C., Azcón-Aguilar, C., and Ferrol, N. (2007). GintMT1 encodes a functional metallothionein in Glomus intraradices that responds to oxidative stress. Mycorrhiza 17, 327–335. doi: 10.1007/s00572-007-0108-7
González-Guerrero, M., Melville, L. H., Ferrol, N., Lott, J. N. A., Azcón-Aguilar, C., and Peterson, R. L. (2008). Ultrastructural localization of heavy metals in the extraradical mycelium and spores of the arbuscular mycorrhizal fungus Glomus intraradices. Can. J. Microbiol. 54, 103–110. doi: 10.1139/w07-119
Gu, H. H., Zhou, Z., Gao, Y. Q., Yuan, X. T., Ai, Y. J., Zhang, J. Y., et al. (2017). The influences of arbuscular mycorrhizal fungus on phytostabilization of lead/zinc tailings using four plant species. Int. J. Phytoremediation 19, 739–745. doi: 10.1080/15226514.2017.1284751
Hachani, C., Lamhamedi, M. S., Cameselle, C., Gouveia, S., Zine El Abidine, A., Khasa, D. P., et al. (2020). Effects of ectomycorrhizal fungi and heavy metals (Pb, Zn, and cd) on growth and mineral nutrition of Pinus halepensis seedlings in North Africa. Microorganisms 8:2033. doi: 10.3390/microorganisms8122033
Jarup, L. (2003). Hazards of heavy metal contamination. Br. Med. Bull. 68, 167–182. doi: 10.1093/bmb/ldg032
Kaiser, C., Koranda, M., Kitzler, B., Fuchslueger, L., Schnecker, J., Schweiger, P., et al. (2010). Belowground carbon allocation by trees drives seasonal patterns of extracellular enzyme activities by altering microbial community composition in a beech forest soil. New Phytol. 187, 843–858. doi: 10.1111/j.1469-8137.2010.03321.x
Kumar, P., Dushenkov, V., Motto, H., and Raskin, I. (1995). Phytoextraction: the use of plants to remove heavy metals from soils. Environ. Sci. Technol. 29, 1232–1238. doi: 10.1021/es00005a014
Leonhardt, T., Sácký, J., Šimek, P., Šantrůček, J., and Kotrba, P. (2014). Metallothionein-like peptides involved in sequestration of Zn in the Zn-accumulating ectomycorrhizal fungus Russula atropurpurea. Metallomics 6, 1693–1701. doi: 10.1039/c4mt00141a
Li, Z., Ma, Z., van der Kuijp, T. J., Yuan, Z., and Huang, L. (2014). A review of soil heavy metal pollution from mines in China: pollution and health risk assessment. Sci. Total Environ. 468-469, 843–853. doi: 10.1016/j.scitotenv.2013.08.090
Liang, X., Kierans, M., Ceci, A., Hillier, S., and Gadd, G. M. (2015). Phosphatase-mediated bioprecipitation of lead by soil fungi. Environ. Microbiol. 18, 219–231. doi: 10.1111/1462-2920.13003
Lichtenthaler, H. K., and Wellburn, R. R. (1983). Determination of total carotenoids and chlorophylls a and b of extracts in different solvents. Biochem. Soc. Trans. 11, 591–592. doi: 10.1042/BST0110591
Liu, B., Wang, S., Wang, J., Zhang, X., Shen, Z., Shi, L., et al. (2020). The great potential for phytoremediation of abandoned tailings pond using ectomycorrhizal Pinus sylvestris. Sci. Total Environ. 719:137475. doi: 10.1016/j.scitotenv.2020.137475
Majorel, C., Hannibal, L., Ducousso, M., Lebrun, M., and Jourand, P. (2014). Evidence of nickel (Ni) efflux in Ni-tolerant ectomycorhizal Pisolithus albus isolated from ultramafic soil. Environ. Microbiol. Rep. 6, 510–518. doi: 10.1111/1758-2229.12176
Martins, L. L., Mourato, M. P., Cardoso, A. I., Pinto, A. P., Mota, A. M., Goncalves, M. L., et al. (2011). Oxidative stress induced by cadmium in Nicotiana tabacum L.: effects on growth parameters, oxidative damage and antioxidant responses in different plant parts. Acta Physiol. Plant. 33, 1375–1383. doi: 10.1007/s11738-010-0671-y
Miretzky, P., and Fernandez-Cirelli, A. (2008). Phosphates for Pb immobilization in soils: a review. Environ. Chem. Lett. 6, 121–133. doi: 10.1007/s10311-007-0133-y
Mohammadhasani, F., Ahmadimoghadam, A., Asrar, Z., and Mohammadi, S. Z. (2017). Effect of Zn toxicity on the level of lipid peroxidation and oxidative enzymes activity in Badami cultivar of pistachio (Pistacia vera L.) colonized by ectomycorrhizal fungus. Plant Physiol. Rep. 22, 206–212. doi: 10.1007/s40502-017-0300-5
Monchanin, C., Devaud, J. M., Barron, A. B., and Lihoreau, M. (2021). Current permissible levels of metal pollutants harm terrestrial invertebrates. Sci. Total Environ. 779:146398. doi: 10.1016/j.scitotenv.2021.146398
Ouatiki, E., Midhat, L., Tounsi, A., Amir, S., Aziz, F., Radi, M., et al. (2022). The association between Pinus halepensis and the ectomycorrhizal fungus scleroderma enhanced the phytoremediation of a polymetal-contaminated soil. Int. J. Environ. Sci Technol. 19, 12537–12550. doi: 10.1007/s13762-022-03993-4
Pena, R., and Polle, A. (2014). Attributing functions to ectomycorrhizal fungal identities in assemblages for nitrogen acquisition under stress. ISME J. 8, 321–330. doi: 10.1038/ismej.2013.158
Perumal, K., Antony, J., and Muthuramalingam, S. (2021). Heavy metal pollutants and their spatial distribution in surface sediments from Thondi coast, Palk Bay, South India. Environ. Sci. Eur. 33, 1–20. doi: 10.1186/s12302-021-00501-2
Phillips, J. M., and Hayman, D. S. (1970). Improved procedures for clearing roots and staining parasitic and vesicular-arbuscular mycorrhizal fungi for rapid assessment of infection. Trans. Brit. Mycol. Soc. 55, 158–IN18. doi: 10.1016/S0007-1536(70)80110-3
Povedano-Priego, C., Martín-Sánchez, I., Jroundi, F., Sánchez-Castro, I., and Merroun, M. L. (2016). Fungal biomineralization of lead phosphates on the surface of lead metal. Miner. Eng. 106, 46–54. doi: 10.1016/j.mineng.2016.11.007
Püttsepp, Ü., Rosling, A., and Taylor, A. F. S. (2004). Ectomycorrhizal fungal communities associated with Salix viminalis L. and S. Dasyclados Wimm. Clones in a short-rotation forestry plantation. For. Ecol. Manag. 196, 413–424. doi: 10.1016/j.foreco.2004.04.003
Reddy, A. M., Kumar, S. G., Jyothsnakumari, G., Thimmanaik, S., and Sudhakar, C. (2005). Lead induced changes in antioxidant metabolism of horse gram (Macrotyloma uniflorum (lam.) Verdc.) and Bengal gram (Cicer arietinum L.). Chemosphere 60:97e104. doi: 10.1016/j.chemosphere.2004.11.092
Rehman, K., Fatima, F., Waheedet, I., and Akash, M. S. H. (2017). Prevalence of exposure of heavy metals and their impact on health consequences. J. Cell. Biochem. 119, 157–184. doi: 10.1002/jcb.26234
Rhee, Y. J., Hillier, S., and Gadd, G. M. (2012). Lead transformation to pyromorphite by fungi. Curr. Biol. 22, 237–241. doi: 10.1016/j.cub.2011.12.017
Ruytinx, J., Coninx, L., Nguyen, H., Smisdom, N., Morin, E., Kohler, A., et al. (2017). Identification, evolution and functional characterization of two Zn CDF-family transporters of the ectomycorrhizal fungus Suillus luteus. Environ. Microbiol. Rep. 9, 419–427. doi: 10.1111/1758-2229.12551
Ruytinx, J., Nguyen, H., Van Hees, M., Op De Beeck, M., Vangronsveld, J., Carleer, R., et al. (2013). Zinc export results in adaptive zinc tolerance in the ectomycorrhizal basidiomycete Suillus bovinus. Metallomics 5, 1225–1233. doi: 10.1039/c3mt00061c
Sarwar, N., Imran, M., Shaheen, M. R., Ishaque, W., Kamran, M. A., Matloob, A., et al. (2017). Phytoremediation strategies for soils contaminated with heavy metals: modifications and future perspectives. Chemosphere 171, 710–721. doi: 10.1016/j.chemosphere.2016.12.116
Schützendübel, A., and Polle, A. (2002). Plant responses to abiotic stresses: heavy metal-induced oxidative stress and protection by mycorrhization. J. Exp. Bot. 53, 1351–1365. doi: 10.1093/jxb/53.372.1351
Shi, L., Deng, X., Yang, Y., Jia, Q., Wang, C., Shen, Z., et al. (2019). A Cr (VI)-tolerant strain, Pisolithus sp1, with a high accumulation capacity of Cr in mycelium and highly efficient assisting Pinus thunbergii for phytoremediation. Chemosphere 224, 862–872. doi: 10.1016/j.chemosphere.2019.03.015
Sun, W., Yang, B., Zhu, Y., Wang, H., Qin, G., and Yang, H. (2021). Ectomycorrhizal fungi enhance the tolerance of phytotoxicity and cadmium accumulation in oak (Quercus acutissima Carruth.) seedlings: modulation of growth properties and the antioxidant defense responses. Environ. Sci. Pollut. Res. Int. 29, 6526–6537. doi: 10.1007/s11356-021-16169-3
Szuba, A., Karliński, L., Krzesłowska, M., and Hazubska-Przybył, T. (2017). Inoculation with a Pb-tolerant strain of Paxillus involutus improves growth and Pb tolerance of Populus × canescens under in vitro conditions. Plant Soil 412, 253–266. doi: 10.1007/s11104-016-3062-3
Tedersoo, L., May, T. W., and Smith, M. E. (2010). Ectomycorrhizal lifestyle in fungi: global diversity, distribution, and evolution of phylogenetic lineages. Mycorrhiza 20, 217–263. doi: 10.1007/s00572-009-0274-x
Topolska, J., Manecki, M., Bajda, T., Borkiewicz, O., and Budzewski, P. (2016). Solubility of pyromorphite Pb5(PO4)3Cl at 5-65°C and its experimentally determined thermodynamic parameters. J. Chem. Thermodyn. 98, 282–287. doi: 10.1016/j.jct.2016.03.031
Velikova, V., Yordanov, I., and Edreva, A. (2000). Oxidative stress and some antioxidant systems in acid rain-treated bean plants: protective role of exogenous polyamines. Plant Sci. 151, 59–66. doi: 10.1016/S0168-9452(99)00197-1
Vergara, C. C., Pignata, M. L., and Rodriguez, J. H. (2020). Effects of co-cropping on soybean growth and stress response in lead-polluted soils. Chemosphere 246:125833. doi: 10.1016/j.chemosphere.2020.125833
Wang, J., Gao, J., Zhang, H., and Tang, M. (2022). Changes in rhizosphere soil fungal communities of Pinus tabuliformis plantations at different development stages on the loess plateau. Int. J. Mol. Sci. 23:6753. doi: 10.3390/ijms23126753
Wang, Q., and Guo, L. D. (2010). Ectomycorrhizal community composition of Pinus tabuliformis assessed by ITS-RFLP and ITS sequences. Botany 88, 590–595. doi: 10.1139/B10-023
Wen, Z. G., Shi, L., Tang, Y., Shen, Z. G., Xia, Y., and Chen, Y. H. (2017). Effects of Pisolithus tinctorius and Cenococcum geophilum inoculation on pine in copper-contaminated soil to enhance phytoremediation. Int. J. Phytoremediation 19, 387–394. doi: 10.1080/15226514.2016.1244155
Yan, M. F., Fan, L. N., and Wang, L. (2020). Restoration of soil carbon with different tree species in a post-mining land in eastern loess plateau, China. Ecol. Eng. 158:106025. doi: 10.1016/j.ecoleng.2020.106025
Zhang, H., Li, X., Xu, Z., Wang, Y., Teng, Z., An, M., et al. (2020). Toxic effects of heavy metals Pb and cd on mulberry (Morus alba L.) seedling leaves: photosynthetic function and reactive oxygen species (ROS) metabolism responses. Ecotoxicol. Environ. Saf. 195:110469. doi: 10.1016/j.ecoenv.2020.110469
Zhang, Q., Ma, J., Gonzalez-Ollauri, A., Yang, Y., and Chen, F. (2023). Soil microbes-mediated enzymes promoted the secondary succession in post-mining plantations on the loess plateau, China. Soil Ecol. Lett. 5, 79–93. doi: 10.1007/s42832-022-0148-0
Keywords: ectomycorrhizal fungi, photosynthetic pigment, antioxidant capacity, XRD analysis, biomineralization
Citation: Cheng K, Liu Y, Tang M and Zhang H (2024) Suillusgrevillei and Suillus luteus promote lead tolerance of Pinus tabulaeformis and biomineralize lead to pyromorphite. Front. Microbiol. 15:1296512. doi: 10.3389/fmicb.2024.1296512
Received: 18 September 2023; Accepted: 22 April 2024;
Published: 09 May 2024.
Edited by:
Albina Ribeiro Franco, University of Minho, PortugalReviewed by:
Sudeep Tiwari, University of Nebraska-Lincoln, United StatesCopyright © 2024 Cheng, Liu, Tang and Zhang. This is an open-access article distributed under the terms of the Creative Commons Attribution License (CC BY). The use, distribution or reproduction in other forums is permitted, provided the original author(s) and the copyright owner(s) are credited and that the original publication in this journal is cited, in accordance with accepted academic practice. No use, distribution or reproduction is permitted which does not comply with these terms.
*Correspondence: Ming Tang, dGFuZ21pbmd5bEAxNjMuY29t; Haoqiang Zhang, emhhbmdoYW9xaWFuZ0Bud3N1YWYuZWR1LmNu
Disclaimer: All claims expressed in this article are solely those of the authors and do not necessarily represent those of their affiliated organizations, or those of the publisher, the editors and the reviewers. Any product that may be evaluated in this article or claim that may be made by its manufacturer is not guaranteed or endorsed by the publisher.
Research integrity at Frontiers
Learn more about the work of our research integrity team to safeguard the quality of each article we publish.