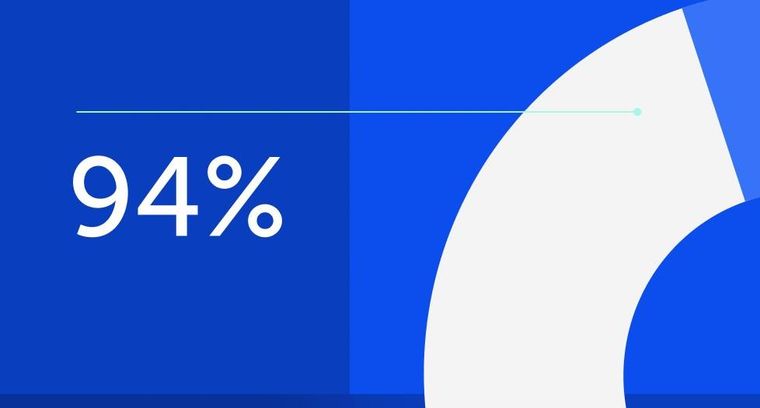
94% of researchers rate our articles as excellent or good
Learn more about the work of our research integrity team to safeguard the quality of each article we publish.
Find out more
REVIEW article
Front. Microbiol., 31 January 2024
Sec. Microbial Symbioses
Volume 15 - 2024 | https://doi.org/10.3389/fmicb.2024.1292004
This article is part of the Research TopicReviews in Gut-Brain Axis: Stress, dysregulation in gut-brain axis function and stress related disordersView all 9 articles
Depression is one of the most prevalent mental disorders today. Over the past decade, there has been considerable attention given to the field of gut microbiota associated with depression. A substantial body of research indicates a bidirectional communication pathway between gut microbiota and the brain. In this review, we extensively detail the correlation between gut microbiota, including Lactobacillus acidophilus and Bifidobacterium longum, and metabolites such as short-chain fatty acids (SCFAs) and 5-hydroxytryptamine (5-HT) concerning depression. Furthermore, we delve into the potential health benefits of microbiome-targeted therapies, encompassing probiotics, prebiotics, and synbiotics, in alleviating depression. Lastly, we underscore the importance of employing a constraint-based modeling framework in the era of systems medicine to contextualize metabolomic measurements and integrate multi-omics data. This approach can offer valuable insights into the complex metabolic host-microbiota interactions, enabling personalized recommendations for potential biomarkers, novel drugs, and treatments for depression.
• This paper reviews reported microbiota-based animal studies related to depression and microbiome-based clinical trials in depression. We also summarized the different combinations of therapies and the resulting efficacy, and explored possible reasons for the poor efficacy.
• We briefly describe certain gut microbial metabolites that influence the onset and progression of depression via MGB axis, including 5-HT, SCFAs, GABA, GP, choline, lactate, BAs and vitamin (folate).
• This paper proposes metabolic models as a means of testing new hypotheses and exploring new microbial-based therapies for depression.
Depression is the most common mental disorder today, characterized by persistent and prolonged feelings of sadness as its main clinical feature. It has a high prevalence, high recurrence rate, and a tendency towards suicide (Belmaker and Agam, 2008), making it one of the most significant types of psychological disorders in modern society. Epidemiological studies from various countries indicate a depression prevalence rate of approximately 7% in the general population (For Anxiety and Depression, R. G. for Treatment, 2017), and up to 10% of individuals with depression may attempt suicide (Bachmann, 2018). Major depressive disorder (MDD) has a devastating impact on global public health, not only causing social and economic burdens but also being a leading cause of severe disability (World Health Organization, 2008). The World Health Organization has identified depression as one of the top public health priorities, estimating that by 2030, it will be the leading cause of death and disability in Western countries (For Anxiety and Depression, R. G. for Treatment, 2017).
Over the past decade, there has been a growing interest in the relationship between gut microbiota and mental disorders (Sanada et al., 2020). The gut microbiota ecosystem contains approximately 1 kg of bacteria, encompassing all microorganisms, their genes, encoded proteins, and metabolites (Eckburg et al., 2005; Korecka and Arulampalam, 2012). Additionally, the gut houses a large number of neurons, second only to the brain, earning the nickname “the second brain” (Ridaura and Belkaid, 2015). Extensive research has established the microbiota-gut-brain (MGB) axis (Bercik, 2011; Camilleri et al., 2012). Along this axis, the microbial community in the gut influences brain function through three bidirectional signaling pathways (Macpherson and Harris, 2004; Bäckhed et al., 2005; Heijtz et al., 2011; Cryan and Dinan, 2012; Breit et al., 2018). Alterations in these signaling pathways may contribute to mental health problems. Therefore, investigating the MGB axis provides a novel approach to exploring the pathogenesis of depression and developing appropriate therapeutic strategies.
In this review, we will summarize the recent advances in gut microbiota research related to depression, focusing on the relationship and role of the MGB axis in depression. We aim to explore the value and potential of the MGB axis in the diagnosis of depression.
There are several pathophysiological hypotheses explaining depression, including the monoamine, brain-derived neurotrophic factor (BDNF), and cytokine hypotheses (Boku et al., 2018). However, these hypotheses have their limitations. Increasing evidence suggests that the gut microbiota may play a role in depression. In a study by McGuinness et al. (2022), it was found that the α-diversity did not significantly differ between the majority of MDD cases and the control group, with only a few reports indicating higher or lower α-diversity between the two groups. However, when statistically tested using β-diversity analysis, 87% of MDD cases showed differences in gut microbiota composition compared to the control group. The study identified 21 bacterial genera with differential abundance at the genus level. Higher abundances of Alistipes, Parabacteroides, Streptococcus, Veillonella, Enterococcus, Flavonifractor, Eggerthella, Escherichia, and lower abundances of Coprococcus, Prevotella, Faecalibacterium, and Ruminococcus were observed in MDD cases. Among them, higher proportions of lactobacilli and lactic acid-producing bacteria, which are generally considered beneficial to the host, were found. These bacteria promote gut microbiota balance (Shi et al., 2017), maintain a normal microbial environment, and have immunomodulatory effects (Pessione, 2012; George et al., 2018). However, in certain circumstances, the production and utilization of lactate can also have detrimental effects on host health. Lactate can accumulate in the gut and cross the blood–brain barrier (Proia et al., 2016), potentially leading to acidosis, arrhythmias, and neurotoxicity (Duncan et al., 2004; Pham et al., 2017). Many psychiatric disorders are also associated with mitochondrial energy dysfunction (Regenold et al., 2009), indicated by increased lactate and decreased pH in the brain. Elevated levels of lactate have been observed in the brains of MDD patients (Ernst et al., 2017), suggesting that an increase in the abundance of lactate-producing bacteria and subsequent lactate accumulation may contribute to the pathophysiology of depression. Additionally, there is evidence suggesting an association between Clostridium difficile and the onset of depression. In a study conducted by Fondden et al. and published in the journal “Nutrients” in 2020, a significant finding indicated that an elevated presence of C. difficile is associated with an increased risk of depression. The study compared individuals with depression to healthy individuals and discovered a 36% higher abundance of C. difficile in those with depression. However, it was also observed that fecal microbiota transplantation proved to be an effective method in reducing the levels of C. difficile, thereby inhibiting the occurrence of depression (van Nood et al., 2013; Austin et al., 2014; Cammarota et al., 2015; Li et al., 2016; Hocquart et al., 2018; Fond et al., 2020). The recent study published in ‘Nature Communications’ provide some of the most compelling evidence to date regarding the relationship between depression and gut microbiota (Radjabzadeh et al., 2022). One study, known as HELIUS, specifically examined health disparities among individuals of different racial backgrounds living in the same urban environment, with the primary aim of investigating the general association between the microbiome and depression. Interestingly, one of the studies within this research did indeed uncover variations in depression risk among different racial groups, but these differences could be explained by individual variations in the composition of one’s microbiome. Overall, the study found a consistent association between overall microbial diversity and depression, transcending racial boundaries. The second study delved more specifically into the types of gut bacteria that may be linked to depression. In a meticulous analysis of fecal samples from approximately 1,000 participants in an ongoing population health study in Rotterdam, 13 microbial species were directly associated with symptoms of depression. The most significant new discovery in this research was the connection between Sellimonas and depression symptoms. Bacterial species belonging to the Sellimonas genus are involved in various inflammatory diseases, potentially linking them to inflammation in individuals with depression. These findings suggest that a causal relationship between the microbiome and depression is entirely plausible, and it is reasonable to consider that depression may lead to other physiological changes, subsequently altering the microbiome (Radjabzadeh et al., 2022).
Multiple studies have demonstrated the involvement of the gut microbiota in the occurrence and development of psychiatric disorders, including depression (Desbonnet et al., 2010; Zheng et al., 2016; Rincel et al., 2019; Pearson-Leary et al., 2020). Transplanting microbiota from depressed patients into normal animals has been found to induce depression-like behaviors (Bravo et al., 2011; Savignac et al., 2014; Liang et al., 2015; Campos et al., 2016; Gacias et al., 2016; Kelly et al., 2016; Zheng et al., 2016). Rats receiving fecal microbiota transplantation from depressed patients displayed anhedonia-like behavior in a sucrose preference test (Kelly et al., 2016). Germ-free mice colonized with microbiota from depressed patients showed increased immobility time in tail suspension and forced swim tests, along with an increased abundance of Actinobacteria, compared to mice colonized with microbiota from healthy individuals (Zheng et al., 2016). These findings, supported by a substantial body of evidence, suggest that alterations in the gut microbiota can contribute to the onset of depression.
While changes in the gut microbiota can contribute to the onset of depression, the gut microbiota also has the potential to improve depressive symptoms. Animal studies (Figure 1) have shown that both antibiotics (Gacias et al., 2016; Guida et al., 2018) and probiotics (Bravo et al., 2011) can significantly alter depression-like behaviors in rats and mice, demonstrating the beneficial effects of gut microbiota as probiotics in alleviating depressive symptoms (Table 1). For instance, in rat studies, a combination therapy of eight probiotic strains (B. bifidum W23, B. lactis W52, L. acidophilus W37, L. brevis W63, L. casei W56, L. salivarius W24, Lactococcus lactis W19, Lactococcus lactis W58) significantly reduced diet-independent depression-like behaviors (Abildgaard et al., 2017). In mouse studies, supplementation of L. helveticus MCC1848 significantly increased interaction time in the social interaction test and sucrose preference ratio in the sucrose preference test (Maehata et al., 2019). Oral administration of L. kefiranofaciens ZW3 improved depression-like behaviors and independent exploration ability, regulating biochemical disorders in the hypothalamic–pituitary–adrenal axis, immune system, and tryptophan metabolism (Sun et al., 2019). Clostridioides butyricum demonstrated significant effects by increasing 5-HT and glucagon-like peptide-1 (GLP-1), upregulating BDNF expression, and promoting GLP-1 secretion and GLP-1 receptor expression (Sun et al., 2018) and GLP-1 has been reported to possess the potential to alleviate depression by regulating neuroinflammation, neurotransmitters, neurogenesis, and synaptic function (Kim et al., 2020). Furthermore, treatment with a multi-strain probiotics approach (L. helveticus R0052, L. plantarum R1012, and B. longum R0175) attenuated anxiety and depression-like behaviors induced by chronic mild stress, significantly increased Lactobacillus abundance, and reversed immune changes in the hippocampus induced by chronic mild stress (Li et al., 2018). Mice subjected to a series of stress stimuli exhibited depression-like behaviors and dysbiosis of the microbiota, but this condition could be reversed by probiotic administration, supplementation with gut bacteria, or antibiotic treatment. Mice subjected to chronic social defeat stress and treated with prebiotics or Bifidobacterium orally showed a reduction in depression-like behaviors in tests such as tail suspension and forced swim (Burokas et al., 2017; Yang et al., 2017). Similarly, mice and rats subjected to chronic restraint stress and treated with minocycline or oral L. helveticus NS8, respectively, reversed the increased depression-like behaviors and altered gut microbiota induced by chronic restraint stress (Liang et al., 2015; Wong et al., 2016). Studies involving mice and rats in models of unpredictable chronic mild stress (Marin et al., 2017), learned helplessness (Mika et al., 2017; Takajo et al., 2019), and maternal separation (Zheng et al., 2016) observed a reduction in depression-like behaviors following treatment with probiotics and supplementation with gut bacteria, while untreated mice and rats showed changes in fecal metabolomic profiles associated with depression-like behaviors (O’Mahony et al., 2009; Jianguo et al., 2019; Zhang et al., 2019). These findings from animal models of depression collectively emphasize the significant role of the gut microbiota and underscore the importance of animal models in microbiota research.
Figure 1. Geographical locations and sample metadata of different animal studies related to depression.
Certain gut microbial metabolites have been shown to influence the occurrence and progression of depression through the MGB axis (Figure 2).
Figure 2. Depression-associated metabolic pathways. Gut microbiota regulates the levels of 5-HT to influence depression. Through dietary or drug interventions, it upregulates the expression of tryptophan hydroxylase 1 (TPH1) in enterochromaffin cells (ECCs), enhancing the production of 5-HT. SCFAs have been shown to affect the production of 5-HT in the gut. An increase in dietary tryptophan induces the synthesis of SCFAs by gut microbiota, leading to an increase in 5-HT production and release in ECCs. Gut microbiota has the ability to increase γ-aminobutyric acid (GABA) levels in the central nervous system (CNS) of mice. GABA crosses the intestinal barrier and is sensed by the vagus nerve, transmitting signals to paraventricular nucleus (PVN) neurons, thereby initiating hypothalamic–pituitary–adrenal (HPA) axis activity and producing an antidepressant effect. In the human body, choline positively influences emotions through promoting SAM-dependent DNA methylation. However, oral choline increases the concentration of acetylcholine in the brain, promoting depression-like behavior. Choline deficiency or excess may both affect depression, highlighting the complexity of the relationship between choline metabolism and depressive behavior. Lactic acid can pass through the blood–brain barrier, and studies in rodents and humans have found a connection between depression and lactic acid abnormalities. About one-third of depression patients exhibit folate deficiency. Folate supplementation has demonstrated its antidepressant effects, but clinical trials have not yet provided strong evidence to support folate as an advantageous adjunctive strategy for depression. The signaling pathway of bile acid metabolism within the gut–liver axis (not depicted in the figure), subject to structural modifications by the gut microbiota, can elicit either severe depression or manifest antidepressant effects based on the specific receptors involved. Created with BioRender.com.
One important factor in depression is tryptophan, and a study focusing on the impact of dietary tryptophan on mood disorders emphasizes that a diet rich in tryptophan helps reduce depressive symptoms and improve an individual’s emotional state. Conversely, a low tryptophan diet can lead to irritability and anxiety (Lindseth et al., 2015). Tryptophan can be metabolized in two crucial pathways of depression: the 5-HT and kynurenine pathways (Comai et al., 2020).
As a key modulator of the gut-brain axis, the neurotransmitter 5-HT plays a crucial role in the communication between the gut and the brain in the signaling of the MGB axis. Recently, an increasing number of studies have indicated the significant role of 5-HT, including its precursor 5-hydroxytryptophan (5-HTP), in the development of depression. Tryptophan hydroxylase (TPH) enzyme plays an important role in various psychiatric disorders, including depression. Research suggests that stress suppresses the expression of this enzyme, thereby reducing the levels of 5-HT (Chen et al., 2017b). Peripheral cells involved in the production of 5-HT exhibit TPH1 dysfunction, leading to insufficient levels of 5-HT in the brain, which in turn triggers a homeostatic response of TPH2, an enzyme that is overexpressed in individuals with suicidal tendencies, in response to low 5-HT levels (Bach-Mizrachi et al., 2006; Fukuda, 2014). This suggests that low 5-HT may contribute to the development of depression. The gut microbiota, in turn, influences depression by regulating the levels of 5-HT. Antibiotic-treated or germ-free mice exhibit reduced synthesis of 5-HT, which can be reversed by the colonization of spore-forming bacteria (Yano et al., 2015). Specific spore-forming bacteria from humans and mice increase the levels of 5-HT in the colon and serum of germ-free mice through the production of SCFAs, thereby upregulating the expression of TPH1 in ECCs and enhancing 5-HT production (Reigstad et al., 2015; Yano et al., 2015). This also improves gut motility disorders associated with germ-free conditions (Furusawa et al., 2013). The communication between ECCs-released 5-HT and the gut microbiota Turicibacter sanguinis, which possesses a 5-HT uptake mechanism, is involved in its colonization and host physiology (Fung et al., 2019). The most common antidepressant medications are based on blocking the reuptake of 5-HT, thereby increasing its levels in the synaptic cleft and promoting antidepressant responses (Cowen and Browning, 2015; Vahid-Ansari and Albert, 2021). This explains the bidirectional effects observed between certain psychotropic drugs, including selective serotonin reuptake inhibitors, and the gut microbiota (Cussotto et al., 2019), suggesting the facilitatory role of high concentrations of 5-HT in antidepressant effects. Furthermore, depression patients show inadequate transport of 5-HTP to the brain (Ryan, 1992; Maffei, 2020), and another study also suggests that the combination of 5-HTP with niacinamide is more effective in combating depression compared to niacinamide alone (López-Ibor Aliño et al., 1976; Maffei, 2020), highlighting the impact of 5-HTP on depression.
Another pathway of tryptophan metabolism is the kynurenine pathway. Excessive pro-inflammatory cytokines produced in depression over activate the enzymes indoleamine 2,3-dioxygnease (IDO) and tryptophan 2,3-dioxygenase (TDO), promoting the kynurenine pathway and consequently reducing the activation of the 5-HT pathway and decreasing the production of 5-HT (Miura et al., 2008). It is worth mentioning that IDO and TDO inhibitors, by inhibiting the activation of IDO and TDO enzymes, can serve as potential drugs for the treatment of depression (Qin et al., 2018). Studies on germ-free mice have shown that the availability of tryptophan increases due to reduced activation of the peripheral kynurenine pathway (Clarke et al., 2013). Moreover, in a rodent model of chronic unpredictable stress, the decrease in stress-induced lactobacillus abundance weakens the inhibition of IDO 1 mediated by hydrogen peroxide. This inhibition leads to an increase in the conversion of tryptophan to kynurenine, resulting in behavioral changes resembling depression in mice exposed to chronic stress (Marin et al., 2017). In contrast to 5-HT, kynurenine can cross the blood–brain barrier and negatively impact brain health through the induction of neuroinflammation and neurodegenerative changes (Kennedy et al., 2017). Therefore, the study of 5-HT and kynurenine metabolism in tryptophan metabolism holds significant importance in depression research, and the balance between the two is closely linked to the physiopathology of depression.
In the gut, short-chain fatty acids (SCFAs) serve as common microbial metabolites and are closely associated with depression. Reports indicate that SCFAs are depleted in patients with MDD (Zheng et al., 2016; Skonieczna-Żydecka et al., 2018). However, administration of SCFAs, especially butyrate, has been shown to improve depression-related gut permeability and HPA axis reactivity, resulting in antidepressant effects (Van De Wouw et al., 2018; Caspani et al., 2019). Research related to depression suggests that the expression of BDNF, can be altered by exogenous SCFAs. Long-term administration of exogenous sodium butyrate in mice has significantly reduced depressive-like behavior, indicating that SCFAs may influence the occurrence and development of depression through their effects on the brain (Schroeder et al., 2007).
SCFAs impact the activity of the enteric nervous system (ENS) and regulate intestinal motility in rodents through free fatty acid receptors present on epithelial cells, enteroendocrine cells (EECs), ECCs, immune cells, and endogenous and exogenous neurons (Muller et al., 2020). These pathways modulate local neuronal cells in the metabolism and/or ENS and the afferent pathway of the vagus nerve, which directly signals to the brain (Morais et al., 2021). A study showed that germ-free mice, lacking gut microbiota, exhibited increased activation of extrinsic neurons connecting the brainstem sensory and enteric sympathetic neurons. However, the activation of these neurons was inhibited by the administration of gut microbiota that produces SCFAs. These findings suggest that gut microbiota can regulate the gut-brain axis neuronal pathway through SCFAs. Additionally, SCFAs have been shown to affect the production of intestinal 5-HT (Bonaz et al., 2018). In humans and mice, increased dietary tryptophan induces the synthesis of SCFAs by gut microbiota, leading to increased production and release of 5-HT in ECCs and enhanced gastrointestinal motility (Reigstad et al., 2015; Yano et al., 2015; Agus et al., 2018; Yu et al., 2019).
However, SCFAs have a relatively short half-life (25 min to 3 h), and further research is needed to determine the extent of the influence of physiologically relevant concentrations of SCFAs on the brain (Margolis et al., 2021).
γ-aminobutyric acid (GABA) is a naturally occurring amino acid found widely in vertebrates, plants, and microorganisms. It is an important inhibitory neurotransmitter in the central nervous system (Rashmi et al., 2018). In recent probiotic research, it has been reported that GABA can be produced by gut microbiota, following a synthesis pathway similar to that in the central nervous system (Janik et al., 2016), and has been shown to alleviate depressive-like behavior in mice (Bravo et al., 2011).
Studies have indicated that certain gut microbiota have the ability to increase GABA levels in the central nervous system of mice, thereby modulating depressive-like behavior. One such microorganism is L. rhamnosus JB-1 (Yunes et al., 2016; Strandwitz et al., 2018), which produces GABA that can cross the intestinal barrier via the proton-coupled amino acid transporter hPAT1 (Nielsen et al., 2012; Bonaz et al., 2017; Yong et al., 2020) and is sensed by the vagus nerve (Bonaz et al., 2017). The vagus nerve activates the GABA signaling pathway to regulate the expression of GABAA and GABAB receptors (Yunes et al., 2016; Strandwitz et al., 2018), allowing the GABA produced by the microbiota to interact with the widely expressed GABA receptors and transporters on the afferent neurons of the vagus nerve (Nielsen et al., 2012; Yong et al., 2020). Additionally, the vagus nerve initiates neural activation in the nucleus tractus solitarius (NTS) of the central nervous system. Sensory gut information transmitted to the NTS is then integrated into its extensive projections, such as the PVN of the hypothalamus, where PVN neurons are responsible for initiating HPA axis activity and producing antidepressant effects (Bravo et al., 2011; Janik et al., 2016).
Some evidence suggests that the host’s lipid metabolism is influenced by the gut microbiota (Blaszczak et al., 2019). Lipids play a crucial role in neuronal function, and the lipid composition of the brain may impact perception and emotional behavior, potentially leading to depression and anxiety (Adibhatla and Hatcher, 2008; Yadav and Tiwari, 2014; Kornhuber et al., 2015). Glycerophospholipids (GP) are major structural lipid components of eukaryotic cell membranes and are involved in numerous cellular processes. Disruption of the gut microbiota, as observed in germ-free mouse experiments, may induce depressive-like behavior by modulating host metabolism (Zheng et al., 2016). Further research has revealed that the gut microbiota primarily influences host GP metabolism (Tian et al., 2022). An experiment found that the hippocampus exhibited the highest degree of disruption in lipid metabolic pathways. The differentially metabolized compounds in the hippocampus were mainly enriched in GP metabolites, with a small proportion belonging to sphingolipid metabolism. Compared to the healthy control (HC) group, the depressive-like (DL) group showed upregulation of most hippocampal metabolites involved in GP metabolism. Furthermore, two metabolites involved in sphingolipid metabolism (dihydroceramide and ceramide-1-phosphate) were significantly decreased in the DL group compared to the HC group. In a chronic unpredictable mild stress rat model of depression, a decrease in lipid metabolism-related enzymes associated with fatty acid synthesis and metabolism, as well as GP metabolism, was observed (Oliveira et al., 2016). These findings indicate an imbalance in hippocampal sphingolipid and GP metabolism associated with depressive-like behavior (Zheng et al., 2021).
Consistent results from studies on humans and non-human primates indicate that dysbiosis of the bacterial phylum Firmicutes may be a hallmark of depression. Zheng et al. found that alterations in microbial and metabolic modules related to fatty acyl, sphingolipid, and GP metabolism were highly correlated with depressive-like behavior (Zheng et al., 2021). Within these microbial modules, several microbial genes involved in fatty acyl, sphingolipid, and GP metabolism were identified, suggesting that the gut microbiota and their regulated host metabolites may play a crucial role in the pathophysiology of depression. Interestingly, most of the unsaturated fatty acids used for the synthesis of brain neuronal membrane GP originate from the gastrointestinal tract rather than the central nervous system, indicating that GP metabolism via the gut-brain axis interferes with depression.
Choline is a constituent of all biological membranes and a precursor of acetylcholine in cholinergic neurons. The acquisition of choline in the body occurs through food sources such as liver and eggs, primarily in the form of phosphatidylcholine (PC), or from endogenously synthesized PC through a continuous methylation process of phosphatidylethanolamine (PE). Choline itself is not a product of bacteria, but under the influence of the gut microbiota, choline can be metabolized into a series of compounds, including trimethylglycine (betaine) and trimethylamine (TMA). In the liver, TMA is converted to trimethylamine N-oxide (TMAO) by flavin monooxygenases (Dumas et al., 2006). Studies have found that the levels of TMA and TMAO in mouse plasma are positively correlated with Clostridiales, Ruminococcus, and Lachnospiraceae in the gut, while negatively correlated with the proportions of S24-7, an abundant family from Bacteroidetes (Wang et al., 2015). In the findings by Romano et al. (2017), choline and its metabolites were found to affect emotional behavior through DNA methylation. Choline regulates the production of the methyl donor S-adenosylmethionine (SAM) to promote DNA methylation. Bacterial consumption of choline reduces the availability of methyl donors, which is consistent with reports of decreased hippocampal DNA methylation and abnormal neurodevelopment in offspring due to maternal choline deficiency (Mellott et al., 2007). In the human body, betaine has a positive impact on emotions by promoting SAM-dependent DNA methylation (Di Pierro and Settembre, 2015). In a rat model of stress, supplementation of choline, betaine, and other methyl donors successfully reversed depressive-like behavior (Paternain et al., 2016).
However, oral administration of choline may promote depressive-like behavior. Choline can actively cross the blood–brain barrier (Sawada et al., 2010), and oral choline increases the concentration of acetylcholine in the brain (Babb et al., 2004), indicating that abnormal choline metabolism may promote depressive-like behavior by altering the choline concentration used for acetylcholine synthesis. Studies have shown that the concentration of the neurotransmitter acetylcholine is significantly higher in patients with MDD than in healthy subjects (Mineur and Picciotto, 2010). All of these findings indicate that choline and its metabolites have a significant impact on emotions through the gut microbiota. Choline deficiency can impair mental health, while excessive choline intake may lead to excessive synthesis of acetylcholine and result in depressive-like behavior. This also suggests the complexity of the relationship between choline metabolism and depressive behavior.
Lactic acid is produced through the fermentation of dietary fiber by mammalian hosts and specific bacteria such as lactic acid bacteria, Bifidobacteria, and Proteobacteria (Ríos-Covián et al., 2016). Although the concentration of lactate in the intestine is low, it can be absorbed into the bloodstream (Tahara et al., 2018) and can cross the blood–brain barrier (Knudsen et al., 1991). Studies in rodents and humans have indicated a connection between depression and lactate abnormalities (Carrard et al., 2018; Karnib et al., 2019), with increased urinary lactate levels observed in severe MDD patients (Chen et al., 2017a). In germ-free mice, elevated lactate concentrations have been observed in the hippocampus, while germ-free rats exhibit increased lactate concentrations in the frontal lobe (Swann et al., 2017).
Karnib et al. (2019) found that lactate salts have a protective and reversing effect on depression. Mice treated with lactate showed increased levels and activity of HDAC2/3 in the hippocampus, while mice not receiving lactate exhibited depressive-like behavior. The efficient exchange of lactate between the peripheral and central nervous systems (Knudsen et al., 1991) suggests the crucial role of the gut microbiota in mediating the antidepressant effects of lactate salts.
Bile acids (BAs), synthesized from liver cholesterol, are pivotal end-products in cholesterol metabolism, constituting essential components of bile and primarily existing in the enterohepatic circulation system. In humans and rats, the main BAs are cholic acid (CA) and chenodeoxycholic acid (CDCA), subject to structural modifications by gut microbiota, leading to the formation of secondary and tertiary BAs (Russell, 2003). Higher levels of cytotoxic secondary BAs, derived from the primary bile acid CDCA by bacterial modifications, have been extensively reviewed in correlation with the severity of anxiety symptoms (Guzior and Quinn, 2021; Mahmoudian Dehkordi et al., 2022; Qu et al., 2022; Sun et al., 2022).
The signaling pathway of BAs is initiated by their binding to farnesoid X receptor (FXR) and Takeda G protein-coupled receptor 5 (Mertens et al., 2017). FXR is involved in bile acid synthesis, secretion, transport, and regulation of cAMP-response element binding protein (CREB) activity. By inhibiting the transcription factor CREB, BAs can suppress the transcription of BDNF, suggesting a potential influence of BAs on depression. Reports have indicated that the abnormal activity of BDNF in individuals with depression may be partially caused by changes in bile acid activity. In the chronic unpredictable mild stress (CUMS) rodent model of depression, an overexpression of FXR in the hippocampus has been observed. Conversely, overexpression of FXR in the rat hippocampus induces depression-like behavior, while the deletion of the FXR gene in juvenile rats inhibits the occurrence of depression-like behavior (Chen et al., 2018). An independent study has also confirmed the antidepressant effect of FXR gene deletion (Huang et al., 2015). Additionally, BAs may disrupt tight junction expression, leading to increased permeability of the intestinal and central epithelial cells, which can result in severe depression (Quinn et al., 2014). However, some BAs, such as ursodeoxycholic acid, have shown good antidepressant effects (Moore et al., 2000; Spedding, 2014), indicating that the impact of BAs on depression-like behavior may depend on specific receptors involved.
Humans heavily rely on the gut microbiota, such as lactobacilli and bifidobacteria, to produce vitamins (Gu and Li, 2016), which play important roles in the human body. In the central nervous system, vitamins can influence neurotransmitter production (Kennedy, 2016), thereby impacting neuronal function.
Folate, a microbiota-derived vitamin, has been widely implicated in depression research (Brocardo et al., 2008, 2009; Molina-Hernández et al., 2011; McCoy et al., 2016; Paternain et al., 2016; Gao et al., 2017), with approximately one-third of individuals with depression showing folate deficiency (Miller, 2008). Administration of folate has demonstrated antidepressant effects in animal models of depression (Brocardo et al., 2008; Molina-Hernández et al., 2011; Gao et al., 2017), and some clinical studies have indicated its potential as an adjunctive therapy for depression in humans (Coppen et al., 1986; Coppen and Bailey, 2000). Folate can synthesize tetrahydrobiopterin, which acts as a cofactor for the conversion of phenylalanine and tryptophan into neurotransmitters dopamine, nzorepinephrine and 5-HT (Wolf et al., 1991), thereby enhancing serotonergic and noradrenergic activity in mice and exerting antidepressant effects. Furthermore, in addition to increasing central 5-HT, folate can induce increased expression of BDNF and glutamate receptor 1 in the hippocampus and associated cortex. The active metabolite of folate, 5-methyltetrahydrofolate, converts homocysteine into methionine, which is used as a methyl donor to produce S-adenosylmethionine (SAM). SAM has been shown to exert antidepressant effects through DNA methylation of phospholipids (Young and Ghadirian, 1989; Kagan et al., 1990; Bottiglieri et al., 1994). Although significant improvements in depression-like behavior have been observed in animal studies, clinical trials have not provided strong evidence supporting the advantage of folate as an adjunctive strategy for depression (Roberts et al., 2018).
The effects of the above metabolites on depression, relevant mechanisms, and reference information have been summarized in Table 2.
So far, probiotics have received significant attention as potential therapies for mood disorders and MDD (Figure 3; Table 3). The occurrence of different probiotics across various clinical trials was explored (Figure 4). L. acidophilus and B. longum are the most widely used probiotics in clinical trials. In healthy individuals, probiotic treatment with L. helveticus R0052 and B. longum R0175 for 30 days has been shown to reduce depression scores on the Hospital Anxiety and Depression (HAD) scale (Messaoudi et al., 2011). In patients with mild to moderate depression, a four-week treatment with a variety of probiotics (Bio-Kult Advanced®) significantly decreased PHQ9 scores (Baião et al., 2023). However, some studies have indicated that probiotic interventions may not effectively alleviate depressive symptoms. In individuals with moderate to severe depression, an eight-week administration of a probiotic mixture containing L. helveticus R0052 and B. longum R0175 as an adjunctive therapy did not improve depressive symptoms (Romijn et al., 2017). This study had a larger sample size and a longer treatment duration compared to previous successful trials of the same probiotic mixture in healthy subjects (Messaoudi et al., 2011). Probiotics are microbial preparations that, when ingested, can improve the balance of gut microbiota (Gibson and Roberfroid, 1995).
Figure 3. Geographical locations and sample metadata of different clinical trials related to depression.
Figure 4. The occurrence of different probiotics across various studies. The left bar in the Upset Plot indicating the number of studies related to each microbe. Higher bars signify stronger evidence linking the microbe to depression. The upper bar displays the number of studies in which several connected microbes simultaneously used. A higher bar indicates that these microbes are found in a greater number of studies.
Prebiotics are compounds that are not broken down, absorbed, or utilized by the human body but promote the growth of gut microbiota, ultimately benefiting the host (Valcheva and Dieleman, 2016). Compounds evaluated in prebiotic trials include Bimuno®-galactooligosaccharide (B-GOS), fructooligosaccharide (FOS), GOS, and short-chain FOS (scFOS). None of the five depression prebiotic trials (Smith, 2005; Silk et al., 2009; Smith et al., 2015; Azpiroz et al., 2017; Kazemi et al., 2019) demonstrated a significant impact on depressive symptoms. Two studies examining the benefits of prebiotic treatment also did not observe a decrease in depression symptom scores during the eight-week follow-up period (Heidarzadeh-Rad et al., 2020; Vaghef-Mehrabany et al., 2021). When studied as standalone therapies, prebiotics yielded non-statistically significant results (Akkasheh et al., 2016; Kazemi et al., 2019; Rudzki et al., 2019). However, no studies have indicated any negative effects of probiotic/prebiotic interventions on depressive symptoms.
The combination of probiotics and prebiotics is known as synbiotics (Sorbara and Pamer, 2022). In a single synbiotic study by Ghorbani et al., a reduction in depressive symptoms was observed after 8 weeks of synbiotic treatment (Ghorbani et al., 2018). In a recent systematic review and meta-analysis by Hofmeister et al., evidence from 50 randomized controlled trials (RCTs) evaluating probiotics, prebiotics, synbiotics, postbiotics, or fecal microbiota transplantation interventions in adult populations was synthesized. Improvement in depressive symptoms was reported based on the Beck Depression Inventory (BDI) and the depression subscale of the Hospital Anxiety and Depression Scale (Hofmeister et al., 2021).
Current evidence suggests that prebiotics as standalone therapies are unlikely to be effective for depression (Akkasheh et al., 2016; Ghorbani et al., 2018; Majeed et al., 2018; Miyaoka et al., 2018; Kazemi et al., 2019). While probiotics and synbiotics appear to be effective in alleviating depressive symptoms, the evidence supporting this observation is mixed. One possible explanation for these mixed findings is that individuals with mild depression may derive more benefits from probiotic and synbiotic treatments compared to those with chronic treatment-resistant depression (Wallace and Milev, 2021). Further studies focusing on different levels of depression severity would help clarify the benefits of these treatments. Additionally, the use of prebiotic and synbiotic therapies for depression is largely understudied, and the evidence is not as specific, necessitating multiple studies analyzing each compound.
The gut microbiota plays a crucial role in regulating human health. Extensive research has shown that the gut microbiota can influence the occurrence and development of depression through the MGB axis, involving neural, immune, and especially metabolic pathways. In reported studies, the gut microbiota has been found to play a significant role in the onset and progression of mental disorders, including depression. For example, when the microbiota from individuals with depression is transplanted into healthy animals, it can induce depression-like behaviors. At the same time, the microbiota can also improve depression-like behaviors. In animal studies, the administration of probiotics has been shown to significantly improve depression-like behaviors in both rats and mice.
Diagnosis and treatment of depression based on the gut microbiota is considered a future research direction. In human studies, administration of probiotics has shown some degree of effectiveness in alleviating depression symptoms. However, prebiotics as a standalone therapy for depression are unlikely to be effective, and the combination of probiotics and prebiotics has not demonstrated significant symptom relief. Yet, there is still limited research on the use of prebiotics and synbiotics in the treatment of depression, and exploring these therapies may uncover beneficial effects.
The CRISPR/Cas9 system is a potent genome editing tool widely utilized in basic, preclinical, and clinical studies as extensively reviewed for genetic disorders (Zhang et al., 2023). While limited studies have employed CRISPR/Cas9 in depression-associated research, there are already CRISPR/Cas-based genome editing tools for Bacteroides (Zheng et al., 2022). These tools significantly aid mechanistic studies of gut commensals and the development of engineered live biotherapeutics.
Constrained-based modeling (Heinken and Thiele, 2015) allows for the versatility needed to simulate bacterial communities under various conditions that cannot be replicated in vivo, such as C. difficile infection. Thus, by employing computational modeling of microbial metabolism using software like MICOM (Diener et al., 2020), a framework is provided to infer the growth rates of selected bacteria and the metabolic interactions within the gut microbiota. Additionally, it offers a high-throughput platform for generating mechanistic hypotheses and testing them in clinical analyses. We believe that the most valuable application of metabolic models in bacterial communities is to provide detailed functional metabolic inferences as a means of testing new hypotheses, thereby laying the groundwork for the development of more accurate models. While these computational approaches have been applied to the study of the gut microbiota, their use in exploring the metabolic consequences of depression is groundbreaking. The outcomes of this approach include the development of more precise models by incorporating information about the ecological relationships between the gut microbiota and its host. From a metabolic perspective, integrating individual differences in genetics and gut microbiota holds promise for personalized recommendations of effective therapies for depression.
MW: Writing – original draft. ZS: Writing – original draft. SL: Writing – original draft, Data curation, Validation, Visualization. FT: Writing – review & editing, Methodology, Supervision, Validation, Investigation. LD: Funding acquisition, Writing – review & editing. FY: Conceptualization, Funding acquisition, Project administration, Writing – review & editing, Writing – original draft.
The author(s) declare financial support was received for the research, authorship, and/or publication of this article. This research was financially supported by the National Natural Science Foundation of China [62102065, 62002242, 62271353, 62001311], joint funds for the Innovation of Science and Technology, Fujian Province (grant number: 2022J05055), Fujian Medical University Research Foundation of Talented Scholars [XRCZX2022003] and Natural Science Foundation of Sichuan Province (2022NSFSC0926).
The authors declare that the research was conducted in the absence of any commercial or financial relationships that could be construed as a potential conflict of interest.
All claims expressed in this article are solely those of the authors and do not necessarily represent those of their affiliated organizations, or those of the publisher, the editors and the reviewers. Any product that may be evaluated in this article, or claim that may be made by its manufacturer, is not guaranteed or endorsed by the publisher.
Abildgaard, A., Elfving, B., Hokland, M., Wegener, G., and Lund, S. (2017). Probiotic treatment reduces depressive-like behaviour in rats independently of diet. Psychoneuroendocrinology 79, 40–48. doi: 10.1016/j.psyneuen.2017.02.014
Adibhatla, R. M., and Hatcher, J. F. (2008). Phospholipase a 2, reactive oxygen species, and lipid peroxidation in CNS pathologies. BMB Rep. 41, 560–567. doi: 10.5483/BMBRep.2008.41.8.560
Agus, A., Planchais, J., and Sokol, H. (2018). Gut microbiota regulation of tryptophan metabolism in health and disease. Cell Host Microbe 23, 716–724. doi: 10.1016/j.chom.2018.05.003
Akkasheh, G., Kashani-Poor, Z., Tajabadi-Ebrahimi, M., Jafari, P., Akbari, H., Taghizadeh, M., et al. (2016). Clinical and metabolic response to probiotic administration in patients with major depressive disorder: a randomized, double-blind, placebo-controlled trial. Nutrition 32, 315–320. doi: 10.1016/j.nut.2015.09.003
Austin, M., Mellow, M., and Tierney, W. M. (2014). Fecal microbiota transplantation in the treatment of Clostridium difficile infections. Am. J. Med. 127, 479–483. doi: 10.1016/j.amjmed.2014.02.017
Azpiroz, F., Dubray, C., Bernalier-Donadille, A., Cardot, J.-M., Accarino, A., Serra, J., et al. (2017). Effects of scFOS on the composition of fecal microbiota and anxiety in patients with irritable bowel syndrome: a randomized, double blind, placebo controlled study. Neurogastroenterol. Motil. 29:e12911. doi: 10.1111/nmo.12911
Babb, S. M., Ke, Y., Lange, N., Kaufman, M. J., Renshaw, P. F., and Cohen, B. M. (2004). Oral choline increases choline metabolites in human brain. Psychiatry Res. Neuroimaging 130, 1–9. doi: 10.1016/S0925-4927(03)00104-5
Bachmann, S. (2018). Epidemiology of suicide and the psychiatric perspective. Int. J. Environ. Res. Public Health 15:1425. doi: 10.3390/ijerph15071425
Bach-Mizrachi, H., Underwood, M. D., Kassir, S. A., Bakalian, M. J., Sibille, E., Tamir, H., et al. (2006). Neuronal tryptophan hydroxylase mRNA expression in the human dorsal and median raphe nuclei: major Depression and suicide. Neuropsychopharmacology 31, 814–824. doi: 10.1038/sj.npp.1300897
Bäckhed, F., Ley, R. E., Sonnenburg, J. L., Peterson, D. A., and Gordon, J. I. (2005). Host-bacterial mutualism in the human intestine. Science 307, 1915–1920. doi: 10.1126/science.1104816
Baião, R., Capitão, L. P., Higgins, C., Browning, M., Harmer, C. J., and Burnet, P. W. J. (2023). Multispecies probiotic administration reduces emotional salience and improves mood in subjects with moderate depression: a randomised, double-blind, placebo-controlled study. Psychol. Med. 53, 3437–3447. doi: 10.1017/S003329172100550X
Belmaker, R. H., and Agam, G. (2008). Major Depressive Disorder. N. Engl. J. Med. 358, 55–68. doi: 10.1056/NEJMra073096
Bercik, P. (2011). The microbiota-gut-brain axis: learning from intestinal bacteria? Gut 60, 288–289. doi: 10.1136/gut.2010.226779
Blaszczak, A. M., Dunaway, S., Ali Alikhan, M., Kelly, S., Levin, D., and Kaffenberger, J. (2019). Safety of systemic therapies in the treatment of psoriasis with concomitant cirrhosis: a retrospective study. Int. J. Dermatol. 58, e228–e229. doi: 10.1111/ijd.14556
Boku, S., Nakagawa, S., Toda, H., and Hishimoto, A. (2018). Neural basis of major depressive disorder: beyond monoamine hypothesis: neural basis of major depressive disorder. Psychiatry Clin. Neurosci. 72, 3–12. doi: 10.1111/pcn.12604
Bonaz, B., Bazin, T., and Pellissier, S. (2018). The Vagus nerve at the Interface of the microbiota-gut-brain Axis. Front. Neurosci. 12:49. doi: 10.3389/fnins.2018.00049
Bonaz, B., Sinniger, V., and Pellissier, S. (2017). The Vagus nerve in the neuro-immune Axis: implications in the pathology of the gastrointestinal tract. Front. Immunol. 8:1452. doi: 10.3389/fimmu.2017.01452
Bottiglieri, T., Hyland, K., and Reynolds, E. H. (1994). The clinical potential of ademetionine (S-Adenosylmethionine) in neurological disorders. Drugs 48, 137–152. doi: 10.2165/00003495-199448020-00002
Bravo, J. A., Forsythe, P., Chew, M. V., Escaravage, E., Savignac, H. M., Dinan, T. G., et al. (2011). Ingestion of Lactobacillus strain regulates emotional behavior and central GABA receptor expression in a mouse via the vagus nerve. Proc. Natl. Acad. Sci. 108, 16050–16055. doi: 10.1073/pnas.1102999108
Breit, S., Kupferberg, A., Rogler, G., and Hasler, G. (2018). Vagus nerve as modulator of the brain-gut Axis in psychiatric and inflammatory disorders. Front. Psych. 9:44. doi: 10.3389/fpsyt.2018.00044
Brocardo, P. S., Budni, J., Kaster, M. P., Santos, A. R. S., and Rodrigues, A. L. S. (2008). Folic acid administration produces an antidepressant-like effect in mice: evidence for the involvement of the serotonergic and noradrenergic systems. Neuropharmacology 54, 464–473. doi: 10.1016/j.neuropharm.2007.10.016
Brocardo, P. S., Budni, J., Lobato, K. R., Santos, A. R. S., and Rodrigues, A. L. S. (2009). Evidence for the involvement of the opioid system in the antidepressant-like effect of folic acid in the mouse forced swimming test. Behav. Brain Res. 200, 122–127. doi: 10.1016/j.bbr.2009.01.004
Burokas, A., Arboleya, S., Moloney, R. D., Peterson, V. L., Murphy, K., Clarke, G., et al. (2017). Targeting the microbiota-gut-brain Axis: prebiotics have anxiolytic and antidepressant-like effects and reverse the impact of chronic stress in mice. Biol. Psychiatry 82, 472–487. doi: 10.1016/j.biopsych.2016.12.031
Camilleri, M., Madsen, K., Spiller, R., Van Meerveld, B. G., and Verne, G. N. (2012). Intestinal barrier function in health and gastrointestinal disease: intestinal barrier function. Neurogastroenterol. Motil. 24, 503–512. doi: 10.1111/j.1365-2982.2012.01921.x
Cammarota, G., Masucci, L., Ianiro, G., Bibbò, S., Dinoi, G., Costamagna, G., et al. (2015). Randomised clinical trial: Faecal microbiota transplantation by colonoscopy vs. vancomycin for the treatment of recurrent Clostridium difficile infection. Aliment. Pharmacol. Ther. 41, 835–843. doi: 10.1111/apt.13144
Campos, A. C., Rocha, N. P., Nicoli, J. R., Vieira, L. Q., Teixeira, M. M., and Teixeira, A. L. (2016). Absence of gut microbiota influences lipopolysaccharide-induced behavioral changes in mice. Behav. Brain Res. 312, 186–194. doi: 10.1016/j.bbr.2016.06.027
Carrard, A., Elsayed, M., Margineanu, M., Boury-Jamot, B., Fragnière, L., Meylan, E. M., et al. (2018). Peripheral administration of lactate produces antidepressant-like effects. Mol. Psychiatry 23, 392–399. doi: 10.1038/mp.2016.179
Caspani, G., Kennedy, S., Foster, J. A., and Swann, J. (2019). Gut microbial metabolites in depression: understanding the biochemical mechanisms. Microbial Cell 6, 454–481. doi: 10.15698/mic2019.10.693
Chen, Y., Xu, H., Zhu, M., Liu, K., Lin, B., Luo, R., et al. (2017b). Stress inhibits tryptophan hydroxylase expression in a rat model of depression. Oncotarget 8, 63247–63257. doi: 10.18632/oncotarget.18780
Chen, W., Zheng, J., Xu, X., Hu, Y., and Ma, Y. (2018). Hippocampal FXR plays a role in the pathogenesis of depression: a preliminary study based on lentiviral gene modulation. Psychiatry Res. 264, 374–379. doi: 10.1016/j.psychres.2018.04.025
Chen, J., Zhou, C., Zheng, P., Cheng, K., Wang, H., Li, J., et al. (2017a). Differential urinary metabolites related with the severity of major depressive disorder. Behav. Brain Res. 332, 280–287. doi: 10.1016/j.bbr.2017.06.012
Clarke, G., Grenham, S., Scully, P., Fitzgerald, P., Moloney, R. D., Shanahan, F., et al. (2013). The microbiome-gut-brain axis during early life regulates the hippocampal serotonergic system in a sex-dependent manner. Mol. Psychiatry 18, 666–673. doi: 10.1038/mp.2012.77
Comai, S., Bertazzo, A., Brughera, M., and Crotti, S. (2020). Tryptophan in health and disease. Adv. Clin. Chem 95, 165–218. doi: 10.1016/bs.acc.2019.08.005
Coppen, A., and Bailey, J. (2000). Enhancement of the antidepressant action of fluoxetine by folic acid: a randomised, placebo controlled trial. J. Affect. Disord. 60, 121–130. doi: 10.1016/S0165-0327(00)00153-1
Coppen, A., Chaudhry, S., and Swade, C. (1986). Folic acid enhances lithium prophylaxis. J. Affect. Disord. 10, 9–13. doi: 10.1016/0165-0327(86)90043-1
Cowen, P. J., and Browning, M. (2015). What has serotonin to do with depression? World Psychiatry 14, 158–160. doi: 10.1002/wps.20229
Cryan, J. F., and Dinan, T. G. (2012). Mind-altering microorganisms: the impact of the gut microbiota on brain and behaviour. Nat. Rev. Neurosci. 13, 701–712. doi: 10.1038/nrn3346
Cussotto, S., Clarke, G., Dinan, T. G., and Cryan, J. F. (2019). Psychotropics and the microbiome: a chamber of secrets …. Psychopharmacology 236, 1411–1432. doi: 10.1007/s00213-019-5185-8
Desbonnet, L., Garrett, L., Clarke, G., Kiely, B., Cryan, J. F., and Dinan, T. G. (2010). Effects of the probiotic Bifidobacterium infantis in the maternal separation model of depression. Neuroscience 170, 1179–1188. doi: 10.1016/j.neuroscience.2010.08.005
Di Pierro, F., and Settembre, R. (2015). Preliminary results of a randomized controlled trial carried out with a fixed combination of S-adenosyl-L-methionine and betaine versus amitriptyline in patients with mild depression. Int. J. Gen. Med. 8, 73–78. doi: 10.2147/IJGM.S79518
Diener, C., Gibbons, S. M., and Resendis-Antonio, O. (2020). MICOM: metagenome-scale modeling to infer metabolic interactions in the gut microbiota. mSystems 5, e00606–e00619. doi: 10.1128/mSystems.00606-19
Dumas, M.-E., Barton, R. H., Toye, A., Cloarec, O., Blancher, C., Rothwell, A., et al. (2006). Metabolic profiling reveals a contribution of gut microbiota to fatty liver phenotype in insulin-resistant mice. Proc. Natl. Acad. Sci. 103, 12511–12516. doi: 10.1073/pnas.0601056103
Duncan, S. H., Louis, P., and Flint, H. J. (2004). Lactate-utilizing Bacteria, isolated from human feces, that produce butyrate as a major fermentation product. Appl. Environ. Microbiol. 70, 5810–5817. doi: 10.1128/AEM.70.10.5810-5817.2004
Eckburg, P. B., Bik, E. M., Bernstein, C. N., Purdom, E., Dethlefsen, L., Sargent, M., et al. (2005). Diversity of the human intestinal microbial Flora. Science 308, 1635–1638. doi: 10.1126/science.1110591
Ernst, J., Hock, A., Henning, A., Seifritz, E., Boeker, H., and Grimm, S. (2017). Increased pregenual anterior cingulate glucose and lactate concentrations in major depressive disorder. Mol. Psychiatry 22, 113–119. doi: 10.1038/mp.2016.73
Fond, G. B., Lagier, J.-C., Honore, S., Lancon, C., Korchia, T., Verville, P.-L. S. D., et al. (2020). Microbiota-orientated treatments for major Depression and schizophrenia. Nutrients 12:1024. doi: 10.3390/nu12041024
For Anxiety and Depression, R. G. for Treatment (2017). Psychotherapies for anxiety and depression: benefits and costs. Res. Psychother. 20:284. doi: 10.4081/ripppo.2017.284
Fukuda, K. (2014). Etiological classification of depression based on the enzymes of tryptophan metabolism. BMC Psychiatry 14:372. doi: 10.1186/s12888-014-0372-y
Fung, T. C., Vuong, H. E., Luna, C. D. G., Pronovost, G. N., Aleksandrova, A. A., Riley, N. G., et al. (2019). Intestinal serotonin and fluoxetine exposure modulate bacterial colonization in the gut. Nat. Microbiol. 4, 2064–2073. doi: 10.1038/s41564-019-0540-4
Furusawa, Y., Obata, Y., Fukuda, S., Endo, T. A., Nakato, G., Takahashi, D., et al. (2013). Commensal microbe-derived butyrate induces the differentiation of colonic regulatory T cells. Nature 504, 446–450. doi: 10.1038/nature12721
Gacias, M., Gaspari, S., Santos, P.-M. G., Tamburini, S., Andrade, M., Zhang, F., et al. (2016). Microbiota-driven transcriptional changes in prefrontal cortex override genetic differences in social behavior. elife 5:e13442. doi: 10.7554/eLife.13442
Gao, L., Liu, X., Yu, L., Wu, J., Xu, M., and Liu, Y. (2017). Folic acid exerts antidepressant effects by upregulating brain-derived neurotrophic factor and glutamate receptor 1 expression in brain. Neuro Report 28, 1078–1084. doi: 10.1097/WNR.0000000000000887
George, F., Daniel, C., Thomas, M., Singer, E., Guilbaud, A., Tessier, F. J., et al. (2018). Occurrence and dynamism of lactic acid Bacteria in distinct ecological niches: a multifaceted functional health perspective. Front. Microbiol. 9:2899. doi: 10.3389/fmicb.2018.02899
Ghorbani, Z., Nazari, S., Etesam, F., Nourimajd, S., Ahmadpanah, M., and Razeghi Jahromi, S. (2018). The effect of Synbiotic as an adjuvant therapy to fluoxetine in moderate depression: a randomized multicenter trial. Arch. Neurosci. 5:e60507. doi: 10.5812/archneurosci.60507
Gibson, G. R., and Roberfroid, M. B. (1995). Dietary modulation of the human colonic microbiota: introducing the concept of prebiotics. J. Nutr. 125, 1401–1412. doi: 10.1093/jn/125.6.1401
Gu, Q., and Li, P. (2016). Biosynthesis of vitamins by probiotic bacteria. Probiotics and Prebiotics in Human Nutrition and Health. 135–48. doi: 10.5772/63117
Guida, F., Turco, F., Iannotta, M., De Gregorio, D., Palumbo, I., Sarnelli, G., et al. (2018). Antibiotic-induced microbiota perturbation causes gut endocannabinoidome changes, hippocampal neuroglial reorganization and depression in mice. Brain Behav. Immun. 67, 230–245. doi: 10.1016/j.bbi.2017.09.001
Guzior, D. V., and Quinn, R. A. (2021). Review: microbial transformations of human bile acids. Microbiome 9:140. doi: 10.1186/s40168-021-01101-1
Heidarzadeh-Rad, N., Gökmen-Özel, H., Kazemi, A., Almasi, N., and Djafarian, K. (2020). Effects of a Psychobiotic supplement on serum brain-derived neurotrophic factor levels in depressive patients: a post hoc analysis of a randomized clinical trial. J. Neurogastroenterol. Motil. 26, 486–495. doi: 10.5056/jnm20079
Heijtz, R. D., Wang, S., Anuar, F., Qian, Y., Björkholm, B., Samuelsson, A., et al. (2011). Normal gut microbiota modulates brain development and behavior. Proc. Natl. Acad. Sci. 108, 3047–3052. doi: 10.1073/pnas.1010529108
Heinken, A., and Thiele, I. (2015). Systematic prediction of health-relevant human-microbial co-metabolism through a computational framework. Gut Microbes 6, 120–130. doi: 10.1080/19490976.2015.1023494
Hocquart, M., Lagier, J.-C., Cassir, N., Saidani, N., Eldin, C., Kerbaj, J., et al. (2018). Early fecal microbiota transplantation improves survival in severe Clostridium difficile infections. Clin. Infect. Dis. 66, 645–650. doi: 10.1093/cid/cix762
Hofmeister, M., Clement, F., Patten, S., Li, J., Dowsett, L. E., Farkas, B., et al. (2021). The effect of interventions targeting gut microbiota on depressive symptoms: a systematic review and meta-analysis. CMAJ Open 9, E1195–E1204. doi: 10.9778/cmajo.20200283
Huang, F., Wang, T., Lan, Y., Yang, L., Pan, W., Zhu, Y., et al. (2015). Deletion of mouse FXR gene disturbs multiple neurotransmitter systems and alters neurobehavior. Front. Behav. Neurosci. 9:70. doi: 10.3389/fnbeh.2015.00070
Janik, R., Thomason, L. A. M., Stanisz, A. M., Forsythe, P., Bienenstock, J., and Stanisz, G. J. (2016). Magnetic resonance spectroscopy reveals oral Lactobacillus promotion of increases in brain GABA, N-acetyl aspartate and glutamate. Neuro Image 125, 988–995. doi: 10.1016/j.neuroimage.2015.11.018
Jianguo, L., Xueyang, J., Cui, W., Changxin, W., and Xuemei, Q. (2019). Altered gut metabolome contributes to depression-like behaviors in rats exposed to chronic unpredictable mild stress. Transl. Psychiatry 9:40. doi: 10.1038/s41398-019-0391-z
Kagan, B. L., Sultzer, D. L., Rosenlicht, N., and Gerner, R. H. (1990). Oral S-adenosylmethionine in depression: a randomized, double-blind, placebo-controlled trial. Am. J. Psychiatr. 147, 591–595. doi: 10.1176/ajp.147.5.591
Karnib, N., El-Ghandour, R., El Hayek, L., Nasrallah, P., Khalifeh, M., Barmo, N., et al. (2019). Lactate is an antidepressant that mediates resilience to stress by modulating the hippocampal levels and activity of histone deacetylases. Neuropsychopharmacology 44, 1152–1162. doi: 10.1038/s41386-019-0313-z
Kazemi, A., Noorbala, A. A., Azam, K., Eskandari, M. H., and Djafarian, K. (2019). Effect of probiotic and prebiotic vs placebo on psychological outcomes in patients with major depressive disorder: a randomized clinical trial. Clin. Nut. 38, 522–528. doi: 10.1016/j.clnu.2018.04.010
Kelly, J. R., Borre, Y., O’Brien, C., Patterson, E., El Aidy, S., Deane, J., et al. (2016). Transferring the blues: Depression-associated gut microbiota induces neurobehavioural changes in the rat. J. Psychiatr. Res. 82, 109–118. doi: 10.1016/j.jpsychires.2016.07.019
Kennedy, D. (2016). B vitamins and the brain: mechanisms, dose and efficacy—a review. Nutrients 8:68. doi: 10.3390/nu8020068
Kennedy, P. J., Cryan, J. F., Dinan, T. G., and Clarke, G. (2017). Kynurenine pathway metabolism and the microbiota-gut-brain axis. Neuropharmacology 112, 399–412. doi: 10.1016/j.neuropharm.2016.07.002
Kim, Y.-K., Kim, O. Y., and Song, J. (2020). Alleviation of Depression by glucagon-like peptide 1 through the regulation of Neuroinflammation, neurotransmitters, neurogenesis, and synaptic function. Front. Pharmacol. 11:1270. doi: 10.3389/fphar.2020.01270
Knudsen, G. M., Paulson, O. B., and Hertz, M. M. (1991). Kinetic analysis of the human blood-brain barrier transport of lactate and its influence by hypercapnia. J. Cereb. Blood Flow Metab. 11, 581–586. doi: 10.1038/jcbfm.1991.107
Korecka, A., and Arulampalam, V. (2012). The gut microbiome: scourge, sentinel or spectator? J. Oral Microbiol. 4:9367. doi: 10.3402/jom.v4i0.9367
Kornhuber, J., Rhein, C., Müller, C. P., and Mühle, C. (2015). Secretory sphingomyelinase in health and disease. Biol. Chem. 396, 707–736. doi: 10.1515/hsz-2015-0109
Li, Y.-T., Cai, H.-F., Wang, Z.-H., Xu, J., and Fang, J.-Y. (2016). Systematic review with meta-analysis: long-term outcomes of faecal microbiota transplantation for Clostridium difficile infection. Aliment. Pharmacol. Ther. 43, 445–457. doi: 10.1111/apt.13492
Li, N., Wang, Q., Wang, Y., Sun, A., Lin, Y., Jin, Y., et al. (2018). Oral probiotics ameliorate the behavioral deficits induced by chronic mild stress in mice via the gut microbiota-inflammation Axis. Front. Behav. Neurosci. 12:266. doi: 10.3389/fnbeh.2018.00266
Liang, S., Wang, T., Hu, X., Luo, J., Li, W., Wu, X., et al. (2015). Administration of Lactobacillus helveticus NS8 improves behavioral, cognitive, and biochemical aberrations caused by chronic restraint stress. Neuroscience 310, 561–577. doi: 10.1016/j.neuroscience.2015.09.033
Lindseth, G., Helland, B., and Caspers, J. (2015). The effects of dietary tryptophan on affective disorders. Arch. Psychiatr. Nurs. 29, 102–107. doi: 10.1016/j.apnu.2014.11.008
López-Ibor Aliño, J. J., Ayuso Gutierrez, J. L., and Montejo Iglesias, M. L. (1976). 5-Hydroxytryptophan (5-HTP) and a MAOI (Nialamide) in the treatment of depressions. Int. Pharmacopsychiatry 11, 8–15. doi: 10.1159/000468207
Macpherson, A. J., and Harris, N. L. (2004). Interactions between commensal intestinal bacteria and the immune system. Nat. Rev. Immunol. 4, 478–485. doi: 10.1038/nri1373
Maehata, H., Kobayashi, Y., Mitsuyama, E., Kawase, T., Kuhara, T., Xiao, J.-Z., et al. (2019). Heat-killed Lactobacillus helveticus strain MCC1848 confers resilience to anxiety or depression-like symptoms caused by subchronic social defeat stress in mice. Biosci. Biotechnol. Biochem. 83, 1239–1247. doi: 10.1080/09168451.2019.1591263
Maffei, M. E. (2020). 5-Hydroxytryptophan (5-HTP): natural occurrence, analysis, biosynthesis, biotechnology, physiology and toxicology. Int. J. Mol. Sci. 22:181. doi: 10.3390/ijms22010181
Mahmoudian Dehkordi, S., Bhattacharyya, S., Brydges, C. R., Jia, W., Fiehn, O., Rush, A. J., et al. (2022). Gut microbiome-linked metabolites in the pathobiology of major Depression with or without anxiety—a role for bile acids. Front. Neurosci. 16:937906. doi: 10.3389/fnins.2022.937906
Majeed, M., Nagabhushanam, K., Arumugam, S., Majeed, S., and Ali, F. (2018). Bacillus coagulans MTCC 5856 for the management of major depression with irritable bowel syndrome: a randomised, double-blind, placebo controlled, multi-Centre, pilot clinical study. Food Nutr. Res. 62:1218. doi: 10.29219/fnr.v62.1218
Margolis, K. G., Cryan, J. F., and Mayer, E. A. (2021). The microbiota-gut-brain Axis: from motility to mood. Gastroenterology 160, 1486–1501. doi: 10.1053/j.gastro.2020.10.066
Marin, I. A., Goertz, J. E., Ren, T., Rich, S. S., Onengut-Gumuscu, S., Farber, E., et al. (2017). Microbiota alteration is associated with the development of stress-induced despair behavior. Sci. Rep. 7:43859. doi: 10.1038/srep43859
McCoy, C. R., Rana, S., Stringfellow, S. A., Day, J. J., Wyss, J. M., Clinton, S. M., et al. (2016). Neonatal maternal separation stress elicits lasting DNA methylation changes in the hippocampus of stress-reactive Wistar Kyoto rats. Eur. J. Neurosci. 44, 2829–2845. doi: 10.1111/ejn.13404
McGuinness, A. J., Davis, J. A., Dawson, S. L., Loughman, A., Collier, F., O’Hely, M., et al. (2022). A systematic review of gut microbiota composition in observational studies of major depressive disorder, bipolar disorder and schizophrenia. Mol. Psychiatry 27, 1920–1935. doi: 10.1038/s41380-022-01456-3
Mellott, T. J., Kowall, N. W., Lopez-Coviella, I., and Blusztajn, J. K. (2007). Prenatal choline deficiency increases choline transporter expression in the septum and hippocampus during postnatal development and in adulthood in rats. Brain Res. 1151, 1–11. doi: 10.1016/j.brainres.2007.03.004
Mertens, K. L., Kalsbeek, A., Soeters, M. R., and Eggink, H. M. (2017). Bile acid signaling pathways from the enterohepatic circulation to the central nervous system. Front. Neurosci. 11:617. doi: 10.3389/fnins.2017.00617
Messaoudi, M., Lalonde, R., Violle, N., Javelot, H., Desor, D., Nejdi, A., et al. (2011). Assessment of psychotropic-like properties of a probiotic formulation (Lactobacillus helveticus R0052 and Bifidobacterium longum R0175) in rats and human subjects. Br. J. Nutr. 105, 755–764. doi: 10.1017/S0007114510004319
Mika, A., Day, H. E. W., Martinez, A., Rumian, N. L., Greenwood, B. N., Chichlowski, M., et al. (2017). Early life diets with prebiotics and bioactive milk fractions attenuate the impact of stress on learned helplessness behaviours and alter gene expression within neural circuits important for stress resistance. Eur. J. Neurosci. 45, 342–357. doi: 10.1111/ejn.13444
Miller, A. L. (2008). The methylation, neurotransmitter, and antioxidant connections between folate and depression. Altern. Med. Rev. 13, 216–226.
Mineur, Y. S., and Picciotto, M. R. (2010). Nicotine receptors and depression: revisiting and revising the cholinergic hypothesis. Trends Pharmacol. Sci. 31, 580–586. doi: 10.1016/j.tips.2010.09.004
Miura, H., Ozaki, N., Sawada, M., Isobe, K., Ohta, T., and Nagatsu, T. (2008). A link between stress and depression: shifts in the balance between the kynurenine and serotonin pathways of tryptophan metabolism and the etiology and pathophysiology of depression. Stress 11, 198–209. doi: 10.1080/10253890701754068
Miyaoka, T., Kanayama, M., Wake, R., Hashioka, S., Hayashida, M., Nagahama, M., et al. (2018). Clostridium butyricum MIYAIRI 588 as adjunctive therapy for treatment-resistant major depressive disorder: a prospective open-label trial. Clin. Neuropharmacol. 41, 151–155. doi: 10.1097/WNF.0000000000000299
Molina-Hernández, M., Téllez-Alcántara, N. P., Olivera-López, J. I., and Jaramillo, M. T. (2011). The folic acid combined with 17-β estradiol produces antidepressant-like actions in ovariectomized rats forced to swim. Prog. Neuro-Psychopharmacol. Biol. Psychiatry 35, 60–66. doi: 10.1016/j.pnpbp.2010.08.022
Moore, L. B., Goodwin, B., Jones, S. A., Wisely, G. B., Serabjit-Singh, C. J., Willson, T. M., et al. (2000). St. John’s wort induces hepatic drug metabolism through activation of the pregnane X receptor. Proc. Natl. Acad. Sci. 97, 7500–7502. doi: 10.1073/pnas.130155097
Morais, L. H., Schreiber, H. L., and Mazmanian, S. K. (2021). The gut microbiota–brain axis in behaviour and brain disorders. Nat. Rev. Microbiol. 19, 241–255. doi: 10.1038/s41579-020-00460-0
Muller, P. A., Schneeberger, M., Matheis, F., Wang, P., Kerner, Z., Ilanges, A., et al. (2020). Microbiota modulate sympathetic neurons via a gut–brain circuit. Nature 583, 441–446. doi: 10.1038/s41586-020-2474-7
Nielsen, C. U., Carstensen, M., and Brodin, B. (2012). Carrier-mediated γ-aminobutyric acid transport across the basolateral membrane of human intestinal Caco-2 cell monolayers. Eur. J. Pharm. Biopharm. 81, 458–462. doi: 10.1016/j.ejpb.2012.03.007
O’Mahony, S. M., Marchesi, J. R., Scully, P., Codling, C., Ceolho, A.-M., Quigley, E. M. M., et al. (2009). Early life stress alters behavior, immunity, and microbiota in rats: implications for irritable bowel syndrome and psychiatric illnesses. Biol. Psychiatry 65, 263–267. doi: 10.1016/j.biopsych.2008.06.026
Oliveira, T. G., Chan, R. B., Bravo, F. V., Miranda, A., Silva, R. R., Zhou, B., et al. (2016). The impact of chronic stress on the rat brain lipidome. Mol. Psychiatry 21, 80–88. doi: 10.1038/mp.2015.14
Paternain, L., Martisova, E., Campión, J., Martínez, J. A., Ramírez, M. J., and Milagro, F. I. (2016). Methyl donor supplementation in rats reverses the deleterious effect of maternal separation on depression-like behaviour. Behav. Brain Res. 299, 51–58. doi: 10.1016/j.bbr.2015.11.031
Pearson-Leary, J., Zhao, C., Bittinger, K., Eacret, D., Luz, S., Vigderman, A. S., et al. (2020). The gut microbiome regulates the increases in depressive-type behaviors and in inflammatory processes in the ventral hippocampus of stress vulnerable rats. Mol. Psychiatry 25, 1068–1079. doi: 10.1038/s41380-019-0380-x
Pessione, E. (2012). Lactic acid bacteria contribution to gut microbiota complexity: lights and shadows. Front. Cell. Infect. Microbiol. 2:86. doi: 10.3389/fcimb.2012.00086
Pham, V. T., Lacroix, C., Braegger, C. P., and Chassard, C. (2017). Lactate-utilizing community is associated with gut microbiota dysbiosis in colicky infants. Sci. Rep. 7:11176. doi: 10.1038/s41598-017-11509-1
Proia, P., Di Liegro, C., Schiera, G., Fricano, A., and Di Liegro, I. (2016). Lactate as a metabolite and a regulator in the central nervous system. Int. J. Mol. Sci. 17:1450. doi: 10.3390/ijms17091450
Qin, Y., Wang, N., Zhang, X., Han, X., Zhai, X., and Lu, Y. (2018). IDO and TDO as a potential therapeutic target in different types of depression. Metab. Brain Dis. 33, 1787–1800. doi: 10.1007/s11011-018-0290-7
Qu, Y., Su, C., Zhao, Q., Shi, A., Zhao, F., Tang, L., et al. (2022). Gut microbiota-mediated elevated production of secondary bile acids in chronic unpredictable mild stress. Front. Pharmacol. 13:837543. doi: 10.3389/fphar.2022.837543
Quinn, M., McMillin, M., Galindo, C., Frampton, G., Pae, H. Y., and DeMorrow, S. (2014). Bile acids permeabilize the blood brain barrier after bile duct ligation in rats via Rac 1-dependent mechanisms. Dig. Liver Dis. 46, 527–534. doi: 10.1016/j.dld.2014.01.159
Radjabzadeh, D., Bosch, J. A., Uitterlinden, A. G., Zwinderman, A. H., Ikram, M. A., van Meurs, J. B. J., et al. (2022). Gut microbiome-wide association study of depressive symptoms. Nat. Commun. 13:7128. doi: 10.1038/s41467-022-34502-3
Rashmi, D., Zanan, R., John, S., Khandagale, K., and Nadaf, A. (2018). γ-Aminobutyric acid (GABA): biosynthesis, role, commercial production, and applications. Stud. Nat. Prod. Chem 57, 413–452. doi: 10.1016/B978-0-444-64057-4.00013-2
Regenold, W. T., Phatak, P., Marano, C. M., Sassan, A., Conley, R. R., and Kling, M. A. (2009). Elevated cerebrospinal fluid lactate concentrations in patients with bipolar disorder and schizophrenia: implications for the mitochondrial dysfunction hypothesis. Biol. Psychiatry 65, 489–494. doi: 10.1016/j.biopsych.2008.11.010
Reigstad, C. S., Salmonson, C. E., Iii, J. F. R., Szurszewski, J. H., Linden, D. R., Sonnenburg, J. L., et al. (2015). Gut microbes promote colonic serotonin production through an effect of short-chain fatty acids on enterochromaffin cells. FASEB J. 29, 1395–1403. doi: 10.1096/fj.14-259598
Ridaura, V., and Belkaid, Y. (2015). Gut microbiota: the link to your second brain. Cell 161, 193–194. doi: 10.1016/j.cell.2015.03.033
Rincel, M., Aubert, P., Chevalier, J., Grohard, P.-A., Basso, L., Monchaux de Oliveira, C., et al. (2019). Multi-hit early life adversity affects gut microbiota, brain and behavior in a sex-dependent manner. Brain Behav. Immun. 80, 179–192. doi: 10.1016/j.bbi.2019.03.006
Ríos-Covián, D., Ruas-Madiedo, P., Margolles, A., Gueimonde, M., De Los Reyes-Gavilán, C. G., and Salazar, N. (2016). Intestinal short chain fatty acids and their link with diet and human health. Front. Microbiol. 7:185. doi: 10.3389/fmicb.2016.00185
Roberts, E., Carter, B., and Young, A. H. (2018). Caveat emptor: folate in unipolar depressive illness, a systematic review and meta-analysis. J. Psychopharmacol. 32, 377–384. doi: 10.1177/0269881118756060
Romano, K. A., Martinez-del Campo, A., Kasahara, K., Chittim, C. L., Vivas, E. I., Amador-Noguez, D., et al. (2017). Metabolic, epigenetic, and transgenerational effects of gut bacterial choline consumption. Cell Host Microbe 22, 279–290.e7. doi: 10.1016/j.chom.2017.07.021
Romijn, A. R., Rucklidge, J. J., Kuijer, R. G., and Frampton, C. (2017). A double-blind, randomized, placebo-controlled trial of Lactobacillus helveticus and Bifidobacterium longum for the symptoms of depression. Aust. N. Z. J. Psychiatry 51, 810–821. doi: 10.1177/0004867416686694
Rudzki, L., Ostrowska, L., Pawlak, D., Małus, A., Pawlak, K., Waszkiewicz, N., et al. (2019). Probiotic Lactobacillus Plantarum 299v decreases kynurenine concentration and improves cognitive functions in patients with major depression: a double-blind, randomized, placebo controlled study. Psychoneuroendocrinology 100, 213–222. doi: 10.1016/j.psyneuen.2018.10.010
Russell, D. W. (2003). The enzymes, regulation, and genetics of bile acid synthesis. Annu. Rev. Biochem. 72, 137–174. doi: 10.1146/annurev.biochem.72.121801.161712
Ryan, N. D. (1992). Neuroendocrine response to L-5-Hydroxytryptophan challenge in Prepubertal major Depression: depressed vs Normal children. Arch. Gen. Psychiatry 49, 843–851. doi: 10.1001/archpsyc.1992.01820110007001
Sanada, K., Nakajima, S., Kurokawa, S., Barceló-Soler, A., Ikuse, D., Hirata, A., et al. (2020). Gut microbiota and major depressive disorder: a systematic review and meta-analysis. J. Affect. Disord. 266, 1–13. doi: 10.1016/j.jad.2020.01.102
Savignac, H. M., Kiely, B., Dinan, T. G., and Cryan, J. F. (2014). Bifidobacteria exert strain-specific effects on stress-related behavior and physiology in BALB/c mice. Neurogastroenterol. Motil. 26, 1615–1627. doi: 10.1111/nmo.12427
Sawada, N., Takanaga, H., Matsuo, H., Naito, M., Tsuruo, T., and Sawada, Y. (2010). Choline uptake by mouse brain capillary endothelial cells in culture. J. Pharm. Pharmacol. 51, 847–852. doi: 10.1211/0022357991773050
Schroeder, F. A., Lin, C. L., Crusio, W. E., and Akbarian, S. (2007). Antidepressant-like effects of the histone deacetylase inhibitor, sodium butyrate, in the mouse. Biol. Psychiatry 62, 55–64. doi: 10.1016/j.biopsych.2006.06.036
Shi, Y., Zhai, Q., Li, D., Mao, B., Liu, X., Zhao, J., et al. (2017). Restoration of cefixime-induced gut microbiota changes by Lactobacillus cocktails and fructooligosaccharides in a mouse model. Microbiol. Res. 200, 14–24. doi: 10.1016/j.micres.2017.04.001
Silk, D. B. A., Davis, A., Vulevic, J., Tzortzis, G., and Gibson, G. R. (2009). Clinical trial: the effects of a trans-galactooligosaccharide prebiotic on faecal microbiota and symptoms in irritable bowel syndrome. Aliment. Pharmacol. Ther. 29, 508–518. doi: 10.1111/j.1365-2036.2008.03911.x
Skonieczna-Żydecka, K., Grochans, E., Maciejewska, D., Szkup, M., Schneider-Matyka, D., Jurczak, A., et al. (2018). Faecal short chain fatty acids profile is changed in polish depressive women. Nutrients 10:1939. doi: 10.3390/nu10121939
Smith, A. (2005). The concept of well-being: relevance to nutrition research. Arthritis Res. Ther. 7:S1. doi: 10.1186/ar1506
Smith, A. P., Sutherland, D., and Hewlett, P. (2015). An investigation of the acute effects of Oligofructose-enriched inulin on subjective wellbeing, mood cognitive performance. Nutrients 7, 8887–8896. doi: 10.3390/nu7115441
Sorbara, M. T., and Pamer, E. G. (2022). Microbiome-based therapeutics. Nature reviews. Microbiology 20, 365–380. doi: 10.1038/s41579-021-00667-9
Spedding, S. (2014). Vitamin D and Depression: a systematic review and Meta-analysis comparing studies with and without biological flaws. Nutrients 6, 1501–1518. doi: 10.3390/nu6041501
Strandwitz, P., Kim, K. H., Terekhova, D., Liu, J. K., Sharma, A., Levering, J., et al. (2018). GABA-modulating bacteria of the human gut microbiota. Nat. Microbiol. 4, 396–403. doi: 10.1038/s41564-018-0307-3
Sun, Y., Geng, W., Pan, Y., Wang, J., Xiao, P., and Wang, Y. (2019). Supplementation with Lactobacillus kefiranofaciens ZW3 from Tibetan kefir improves depression-like behavior in stressed mice by modulating the gut microbiota. Food Funct. 10, 925–937. doi: 10.1039/C8FO02096E
Sun, J., Wang, F., Hu, X., Yang, C., Xu, H., Yao, Y., et al. (2018). Clostridium butyricum attenuates chronic unpredictable mild stress-induced depressive-like behavior in mice via the gut-brain Axis. J. Agric. Food Chem. 66, 8415–8421. doi: 10.1021/acs.jafc.8b02462
Sun, N., Zhang, J., Wang, J., Liu, Z., Wang, X., Kang, P., et al. (2022). Abnormal gut microbiota and bile acids in patients with first-episode major depressive disorder and correlation analysis. Psychiatry Clin. Neurosci. 76, 321–328. doi: 10.1111/pcn.13368
Swann, J. R., Garcia-Perez, I., Braniste, V., Wilson, I. D., Sidaway, J. E., Nicholson, J. K., et al. (2017). Application of 1 H NMR spectroscopy to the metabolic phenotyping of rodent brain extracts: a metabonomic study of gut microbial influence on host brain metabolism. J. Pharm. Biomed. Anal. 143, 141–146. doi: 10.1016/j.jpba.2017.05.040
Tahara, Y., Yamazaki, M., Sukigara, H., Motohashi, H., Sasaki, H., Miyakawa, H., et al. (2018). Gut microbiota-derived short chain fatty acids induce circadian clock entrainment in mouse peripheral tissue. Sci. Rep. 8:1395. doi: 10.1038/s41598-018-19836-7
Takajo, T., Tomita, K., Tsuchihashi, H., Enomoto, S., Tanichi, M., Toda, H., et al. (2019). Depression promotes the onset of irritable bowel syndrome through unique Dysbiosis in rats. Gut Liver 13, 325–332. doi: 10.5009/gnl18296
Tian, T., Mao, Q., Xie, J., Wang, Y., Shao, W., Zhong, Q., et al. (2022). Multi-omics data reveals the disturbance of glycerophospholipid metabolism caused by disordered gut microbiota in depressed mice. J. Adv. Res. 39, 135–145. doi: 10.1016/j.jare.2021.10.002
Vaghef-Mehrabany, E., Ranjbar, F., Asghari-Jafarabadi, M., Hosseinpour-Arjmand, S., and Ebrahimi-Mameghani, M. (2021). Calorie restriction in combination with prebiotic supplementation in obese women with depression: effects on metabolic and clinical response. Nutr. Neurosci. 24, 339–353. doi: 10.1080/1028415X.2019.1630985
Vahid-Ansari, F., and Albert, P. R. (2021). Rewiring of the serotonin system in major Depression. Front. Psych. 12:802581. doi: 10.3389/fpsyt.2021.802581
Valcheva, R., and Dieleman, L. A. (2016). Prebiotics: definition and protective mechanisms. Best Pract. Res. Clin. Gastroenterol. 30, 27–37. doi: 10.1016/j.bpg.2016.02.008
Van De Wouw, M., Boehme, M., Lyte, J. M., Wiley, N., Strain, C., O’Sullivan, O., et al. (2018). Short-chain fatty acids: microbial metabolites that alleviate stress-induced brain-gut axis alterations: SCFAs alleviate stress-induced brain-gut axis alterations. J. Physiol. 596, 4923–4944. doi: 10.1113/JP276431
van Nood, E., Vrieze, A., Nieuwdorp, M., Fuentes, S., Zoetendal, E. G., de Vos, W. M., et al. (2013). Duodenal infusion of donor feces for recurrent Clostridium difficile. N. Engl. J. Med. 368, 407–415. doi: 10.1056/NEJMoa1205037
Wallace, C. J. K., and Milev, R. V. (2021). The efficacy, safety, and tolerability of probiotics on Depression: clinical results from an open-label pilot study. Front. Psych. 12:618279. doi: 10.3389/fpsyt.2021.618279
Wang, Z., Roberts, A. B., Buffa, J. A., Levison, B. S., Zhu, W., Org, E., et al. (2015). Non-lethal inhibition of gut microbial trimethylamine production for the treatment of atherosclerosis. Cell 163, 1585–1595. doi: 10.1016/j.cell.2015.11.055
Wolf, W. A., Ziaja, E., Arthur, R. A., Anastasiadis, P. Z., Levine, R. A., and Kuhn, D. M. (1991). Effect of tetrahydrobiopterin on serotonin synthesis, release, and metabolism in superfused hippocampal slices. J. Neurochem. 57, 1191–1197. doi: 10.1111/j.1471-4159.1991.tb08279.x
Wong, M.-L., Inserra, A., Lewis, M. D., Mastronardi, C. A., Leong, L., Choo, J., et al. (2016). Inflammasome signaling affects anxiety-and depressive-like behavior and gut microbiome composition. Mol. Psychiatry 21, 797–805. doi: 10.1038/mp.2016.46
World Health Organization. (2008). The Global Burden of Disease: 2004 Update. Geneva, Switzerland: World Health Organization 146.
Yadav, R. S., and Tiwari, N. K. (2014). Lipid integration in neurodegeneration: an overview of Alzheimer’s disease. Mol. Neurobiol. 50, 168–176. doi: 10.1007/s12035-014-8661-5
Yang, C., Fujita, Y., Ren, Q., Ma, M., Dong, C., and Hashimoto, K. (2017). Bifidobacterium in the gut microbiota confer resilience to chronic social defeat stress in mice. Sci. Rep. 7:45942. doi: 10.1038/srep45942
Yano, J. M., Yu, K., Donaldson, G. P., Shastri, G. G., Ann, P., Ma, L., et al. (2015). Indigenous bacteria from the gut microbiota regulate host serotonin biosynthesis. Cell 161, 264–276. doi: 10.1016/j.cell.2015.02.047
Yong, S. J., Tong, T., Chew, J., and Lim, W. L. (2020). Antidepressive mechanisms of probiotics and their therapeutic potential. Front. Neurosci. 13:1361. doi: 10.3389/fnins.2019.01361
Young, S. N., and Ghadirian, A. M. (1989). Folic acid and psychopathology. Prog. Neuro-Psychopharmacol. Biol. Psychiatry 13, 841–863. doi: 10.1016/0278-5846(89)90037-7
Yu, Y., Villalobos-Hernandez, E. C., Pradhananga, S., Baker, C. C., Keating, C., Grundy, D., et al. (2019). Deoxycholic acid activates colonic afferent nerves via 5-HT 3 receptor-dependent and-independent mechanisms. American journal of physiology-gastrointestinal and liver. Physiology 317, G275–G284. doi: 10.1152/ajpgi.00016.2019
Yunes, R. A., Poluektova, E. U., Dyachkova, M. S., Klimina, K. M., Kovtun, A. S., Averina, O. V., et al. (2016). GABA production and structure of gadB/gadC genes in Lactobacillus and Bifidobacterium strains from human microbiota. Anaerobe 42, 197–204. doi: 10.1016/j.anaerobe.2016.10.011
Zhang, K., Fujita, Y., Chang, L., Qu, Y., Pu, Y., Wang, S., et al. (2019). Abnormal composition of gut microbiota is associated with resilience versus susceptibility to inescapable electric stress. Transl. Psychiatry 9:231. doi: 10.1038/s41398-019-0571-x
Zhang, S., Wang, Y., Mao, D., Wang, Y., Zhang, H., Pan, Y., et al. (2023). Current trends of clinical trials involving CRISPR/Cas systems. Front. Med. 10:1292452. doi: 10.3389/fmed.2023.1292452
Zheng, L., Tan, Y., Hu, Y., Shen, J., Qu, Z., Chen, X., et al. (2022). CRISPR/Cas-based genome editing for human gut commensal Bacteroides species. ACS Synth. Biol. 11, 464–472. doi: 10.1021/acssynbio.1c00543
Zheng, P., Wu, J., Zhang, H., Perry, S. W., Yin, B., Tan, X., et al. (2021). The gut microbiome modulates gut–brain axis glycerophospholipid metabolism in a region-specific manner in a nonhuman primate model of depression. Mol. Psychiatry 26, 2380–2392. doi: 10.1038/s41380-020-0744-2
Keywords: depression, gut microbiota, metabolites, pathogenesis, clinical trials
Citation: Wang M, Song Z, Lai S, Tang F, Dou L and Yang F (2024) Depression-associated gut microbes, metabolites and clinical trials. Front. Microbiol. 15:1292004. doi: 10.3389/fmicb.2024.1292004
Received: 10 September 2023; Accepted: 16 January 2024;
Published: 31 January 2024.
Edited by:
Avinash Veerappa, University of Nebraska Medical Center, United StatesReviewed by:
Yingli Jing, Capital Medical University, ChinaCopyright © 2024 Wang, Song, Lai, Tang, Dou and Yang. This is an open-access article distributed under the terms of the Creative Commons Attribution License (CC BY). The use, distribution or reproduction in other forums is permitted, provided the original author(s) and the copyright owner(s) are credited and that the original publication in this journal is cited, in accordance with accepted academic practice. No use, distribution or reproduction is permitted which does not comply with these terms.
*Correspondence: Fenglong Yang, eWFuZ2Zlbmdsb25nQGZqbXUuZWR1LmNu; Lijun Dou, ZG91bDJAY2NmLm9yZw==
†These authors have contributed equally to this work and share first authorship
Disclaimer: All claims expressed in this article are solely those of the authors and do not necessarily represent those of their affiliated organizations, or those of the publisher, the editors and the reviewers. Any product that may be evaluated in this article or claim that may be made by its manufacturer is not guaranteed or endorsed by the publisher.
Research integrity at Frontiers
Learn more about the work of our research integrity team to safeguard the quality of each article we publish.