- 1Institute of Special Wild Economic Animal and Plant Sciences, Chinese Academy of Agricultural Sciences, Changchun, China
- 2Jilin Provincial Key Laboratory of Traditional Chinese Medicinal Materials Cultivation and Propagation, Changchun, China
- 3College of Pharmacy and Biological Engineering, Chengdu University, Chengdu, China
Introduction: Ginseng (Panax ginseng C.A. Meyer) has multiple effects on human health; however, soil degradation seriously affects its yield. Trichoderma spp. play an important role in improving plant biomass by influencing the soil environment. Therefore, it is necessary to screen efficient Trichoderma strains that can increase ginseng biomass and determine their mechanisms.
Methods: Herein, we selected six Trichoderma species (T. brevicompactum, T. velutinum, T. viridescens, T. atroviride, T. koningiopsis, and T. saturnisporum) isolated from ginseng rhizosphere soil, and evaluated their growth promoting effects on ginseng and their influence on the microbiome and chemical attributes of the ginseng rhizosphere soil.
Results: Except for T. saturnisporum (F), compared with the control, the other five species increased ginseng biomass. In terms of chemical properties, the pH value, available potassium content, and available phosphorus content in the ginseng rhizosphere soil increased by 1.16–5.85%, 0.16–14.03%, and 3.92–38.64%, respectively, after root irrigation with spores of Trichoderma species. For the soil microbiome, fungal Chao1 and Ace richness indices decreased. Application of Trichoderma enhanced the relative level of Proteobacteria, but reduced the relative level of Ascomycota. At the genus level, application of Trichoderma enhanced the relative levels of Sphingomonas, Blastomonas, and Trichoderma, but reduced the relative level of Fusarium. Available K and available P were the most important elements that affected the structure of the bacterial community, while total K was the most influential element for the structure of the fungal community structure.
Conclusion: The results indicated that the application of Trichoderma spp. could increase soil nutrients and regulate the structure and composition of the soil microbial community, thereby enhancing the biomass of ginseng. The results will provide guidance for soil improvement in ginseng cultivation.
1 Introduction
Ginseng has multiple beneficial effects on human health (Yu et al., 2017; Riaz et al., 2019). Ginseng has been artificially cultivated in large areas because of its high medicinal value (Sun et al., 2017). However, over time, cultivation of ginseng has led to decreased soil nutrients and beneficial soil microorganisms, but an increase in harmful microorganisms, especially those causing soil-borne diseases (Wang et al., 2020). To reduce the incidence of diseases and increase production, chemical fertilizers and pesticides have been widely used; however, this has caused problems such as persistence of pesticide residues and environmental pollution (Tang, 2020; Kai and Adhikari, 2021). Therefore, how to promote ginseng growth safely and effectively has become an important issue in ginseng cultivation (Chen et al., 2016).
Trichoderma spp. are widely distributed in soil, with functions such as inhibiting soil borne pathogens, improving soil, and promoting plant growth (Javeria et al., 2020; Jamil, 2021). Wu et al. (2022) found that Trichoderma applied after fumigation significantly improved cucumber yield and the soil chemical properties. Liu L. et al. (2022) found that biofertilizer containing T. harzianum increased the yield and quality of Bupleurum chinense, increased the content of available nutrients in the rhizosphere soil, and enhanced the activities of sucrase and catalase. Trichoderma spp. isolated from ginseng rhizosphere soil could improve the soil nutrient status and further affect the diversity of the soil fungal community (Ma et al., 2023).
However, there are few reports on whether Trichoderma species have broad spectrum biomass promoting effects on ginseng, and their relationship with the microbiome and physicochemical properties of ginseng rhizosphere soil. In the present study, we selected six Trichoderma species isolated from ginseng rhizosphere soil to evaluate their biomass promoting effects on ginseng and their influence on the microbiome and physicochemical properties of the ginseng rhizosphere soil. The results will provide guidance to improve the soil for ginseng cultivation and the development of biological fertilizers.
2 Materials and methods
2.1 Experimental materials
The Trichoderma strains were provided by the Institute of Special Wild Economic Animal and Plant Sciences, Chinese Academy of Agricultural Sciences (Changchun, China), and were also isolated from ginseng roots collected in Wanliang Town, Fusong County, Changbai City, Jilin Province, China. After morphological and molecular biological analyses, they were identified as: T. brevicompactum, T. velutinum, T. viridescens, T. atroviride, T. koningiopsis, and T. saturnisporum. Among them, T. brevicompactum, T. velutinum, T. viridescens, T. atroviride, and T. koningiopsis have been preserved in the China General Microbiological Culture Collection Center (preservation numbers: CGMCC NO. 23213, CGMCC No. 23211, CGMCC No. 23212, CGMCC No. 23214, and CGMCC No. 23210, respectively) (Wang et al., 2022a,b, c, d, e).
The Trichoderma strains were inoculated onto 90 mm potato dextrose agar (PDA) plates and incubated at 25°C for 7 days. After the spores were fully grown on the plate, an appropriate amount of sterile water was added to wash them off gently, followed by dispersal of the spores into a 6 g⋅L–1 sodium carboxymethyl cellulose (CMC) solution to obtain a spore suspension at 1 × 107 colony forming units (CFU)⋅mL–1.
2.2 Experimental design
The experiment was conducted at the Wild Economic Animals and Plants Institute of CAAS, Changchun, China (E125°24′53″, N43°46′19″). Farmland soil was added to a 20 cm diameter pot, with 2 kg of soil per pot. The basic information of the foundation soils is shown in the FS treatment in Table 1. Same sized, healthy 1-year-old ginseng plants were selected for transplantation into the pots. The plants were grown in a room under a luminous intensity of 100 lux at 25°C and 60% humidity, and watered every 5 days. 7 treatments were set: A. T. brevicompactum, B. T. velutinum, C. T. viridescens, D. T. atroviride, E. T. koningiopsis, F. T. saturnisporum and CK [untreated plants (control)], respectively. Each treatment was performed using 4 replicates, with 5 plants per replicate. A total of 30 mL of prepared spore suspension of each fungal species was used for root irrigation after planting. Soil samples were collected after 90 days of ginseng seedling growth. We collected the rhizosphere soil and bulk soil in sterile plastic bags. The soil present within approximately 3 cm around the root of the ginseng seedling was considered the bulk soil, and the remaining soil attached to the ginseng roots was considered the rhizosphere soil. A portion of each soil sample was placed at −80°C for subsequent extraction of DNA, and the rest of each sample was dried naturally before analysis of its chemical attributes. The whole ginseng plant was washed thoroughly, and the fresh weight of the whole plant and roots were measured. The dry weight of the whole plant and roots were then measured after drying at 105°C.
2.3 Analysis of soil chemical properties
The soil pH value was measured using a pH/oxidation reduction potential (ORP) acidity meter. The ammonium nitrogen (NH4+-N), nitrate nitrogen (NO3–-N), total nitrogen (TN), and total carbon (TC) contents were determined as described previously (Jin et al., 2022a). The salt content (S) was determined using a conductivity meter, and the agricultural soil chemical analysis method (Bao, 2000) was used to determine the total potassium (TK), total phosphorus (TP), available potassium (AK), and available phosphorus (AP).
2.4 Soil DNA extraction, PCR, and sequencing
A MoBio Laboratories PowerSoil DNA Isolation Kit (MoBio Laboratories, Carlsbad, CA, USA) was employed to extract DNA. The 16S rRNA in the DNA sample was amplified using the primers 806R (GGACTACHVGGGTWTCTAAT) and 338F (ACTCCTACGGGAGGCAGCA) (Fu et al., 2023) using the following reaction conditions: 95°C for 10 min, followed by 40 cycles of 15 s at 95°C, 60 s at 55°C, and 90 s at 72°C, with a final extension of 7 min at 72°C. Likewise, primers ITS1F (CTTGGTCATTTAGAGGAAGTAA) and ITS2 (GCTGCGTTCTTCATCGATGC) (White et al., 1990; Gardes and Bruns, 1993) were used to amplify the internal transcribed spacer (ITS) region as follows: 95°C for 5 min, then 35 cycles of 95°C for 1 min, 53°C for 45 s, and 72°C for 1 min. The amplicons were purified and then subjected to Illumina MiSeq sequencing (Illumina Inc., San Diego, CA, USA).
First, the original data was filtered employing Trimmomatic 0.33 (Bolger et al., 2014), and primer sequences were removed employing Cutadapt 1.9.1 (Martin, 2011). Subsequently, Usearch (version 10) (Edgar, 2013) was employed to splice the double-ended reads, with chimeras being removed using UCHIME (version 4.2) (Edgar et al., 2011) to leave sequence of high quality for subsequent analysis. Using a cutoff of 97% similarity, the sequences were clustered into operational taxonomic units (OTUs) using Usearch. OTUs with counts less than two in all samples were filtered out. Taxonomy annotation of the resulting OTUs was carried out using the Naive Bayes classifier in QIIME2 (Bolyen et al., 2019), utilizing the SILVA database (release 138.1) (Quast et al., 2013), with a confidence threshold of 70%. Meanwhile, Alpha and Beta diversity analyses were performed using the QIIME2 software to assess the species diversity within each sample. This involved calculating the Shannon, Simpson, ACE, and Chao1 indices to obtain information on the diversity of species within the samples. Additionally, information on the common and unique OTUs between different samples or groups was obtained.
2.5 Statistical analysis
SAS version 9.1 (SAS institute, Cary, NC, USA) was employed to analyze the soil bacteria and fungi diversity indices (e.g., Shannon and Chao1) and soil chemical attributes. One-way analysis of variance (ANOVA) with the least significance difference (LSD) test were used to compare the mean vales for the samples and variability in the data was expressed as the standard error (n = 4). Differences at P < 0.05 or P < 0.01 were considered statistically significant. We performed linear discriminant analysis Effect Size (LEfSe) analysis according to the method of Segata et al. (2011). Redundancy analysis (RDA) of soil chemical factors and microbial diversity was carried out using CANOCO 5.0. The environmental variables were evaluated using a partial Monte Carlo permutation test (499 permutations) with an unrestricted permutation to investigate their statistical significance (Huang et al., 2017).
3 Results
3.1 Effects of different treatments on the fresh weight and dry weight of ginseng plants
As shown in Figure 1, the control group showed symptoms of ginseng wilt disease, with dry leaf tips and edges, while the symptoms of ginseng wilt disease in Trichoderma treatment groups were almost non-existent or reduced. Compared with CK, the fresh and dry weight of ginseng under A and E treatments increased significantly (P < 0.05), while the dry weight of ginseng under B, C, and D treatments increased significantly (P < 0.05). The fresh weight of ginseng under A treatment increased by 20.73% and the dry weight increased by 49.02%. The fresh weight of ginseng under E treatment increased by 15.45% and the dry weight increased by 35.30%, the dry weight of ginseng under B, C, and D treatments increased by 45.10, 35.30, and 49.02%, respectively (Table 2). Thus, treatment with A had the best effect on increasing the fresh and dry weight of ginseng, followed by E treatment, and B, C, and D treatments were beneficial only for the dry weight accumulation of ginseng. There was no significant difference between F treatment and the control group.
3.2 Comparisons of soil chemical properties among different treatments
The soil chemical properties of 1-year-old ginseng treated with different Trichoderma spp. after a 90-day growth period are shown in Table 1. The pH value of the soil increased by 1.16–5.85% compared with CK. The TC under B, C, and D treatments was increased compared with that in CK; the TN under B treatment increased significantly compared with that of CK (P < 0.05); the TP under B and D treatments increased compared with that of CK; and the contents of TK and NH4+-N under D treatment increased compared with that of CK. The NO3–-N content under D and F treatments increased compared with that of CK. The contents of AP and AK under all treatments increased by 0.16–14.03% and 3.9–38.64% compared with CK, respectively. The S content under each treatment was decreased compared with that of CK, demonstrating that Trichoderma application reduced the S content, in which treatments A, C, and E resulted in significant differences compared with CK (P < 0.05).
3.3 Diversity analysis of bacteria and fungi in the soil after various treatments
The indexes of diversity coverage of microorganisms in the soil were all > 0.97, showing that the results of sequencing accurately represented the actual situation of the soil bacteria and fungi. For bacteria, except for treatment C, the Shannon index was higher in all treatments compared with that of CK. The Ace indices of treatments A, B, and D were higher than that of CK. The Chao1 indices of treatments A and B were higher than that of CK. For fungi, except for treatment E, the Shannon index was lower than that in CK for all treatments, while the Simpson indices of treatments D and E were higher than that of CK. The Ace and Chao1 indices of all treatments were lower than that of CK (P < 0.05). Therefore, overall, the application of Trichoderma increased bacterial diversity and reduced fungal diversity (Table 3).
3.4 Soil microbial community composition among different treatments
3.4.1 Phylum level classification of bacteria and fungi
After applying Trichoderma, there was no change in the top 10 bacterial population categories compared with those in CK; however, there were certain changes in their relative abundances, and the changes varied with different Trichoderma treatments. The top 10 soil bacterial phyla by relative abundance under the various treatments were: Proteobacteria, Acidobacteria, Chloroflexi, Gemmatimonadetes, Actinobacteria, Verrucomicrobia, Bacteroidetes, Firmicutes, Patescibacteria, and Cyanobacteria. The sum of the comparative levels of Acidobacteria and Proteobacteria reached 58.48–62.45% (Figure 2A). The comparative levels of Chloroflexi and Proteobacteria in each Trichoderma treatment group were increased compared with those in CK. Among them, the relative abundance of Proteobacteria under E treatment was the highest, and the relative abundance of Chloroflexi under B treatment was the highest; however, the relative abundance of Acidobacteria under all treatments was decreased compared with that in CK. Except for treatment B, the relative abundance of Gemmatimonadetes under all treatments was increased compared with that CK.
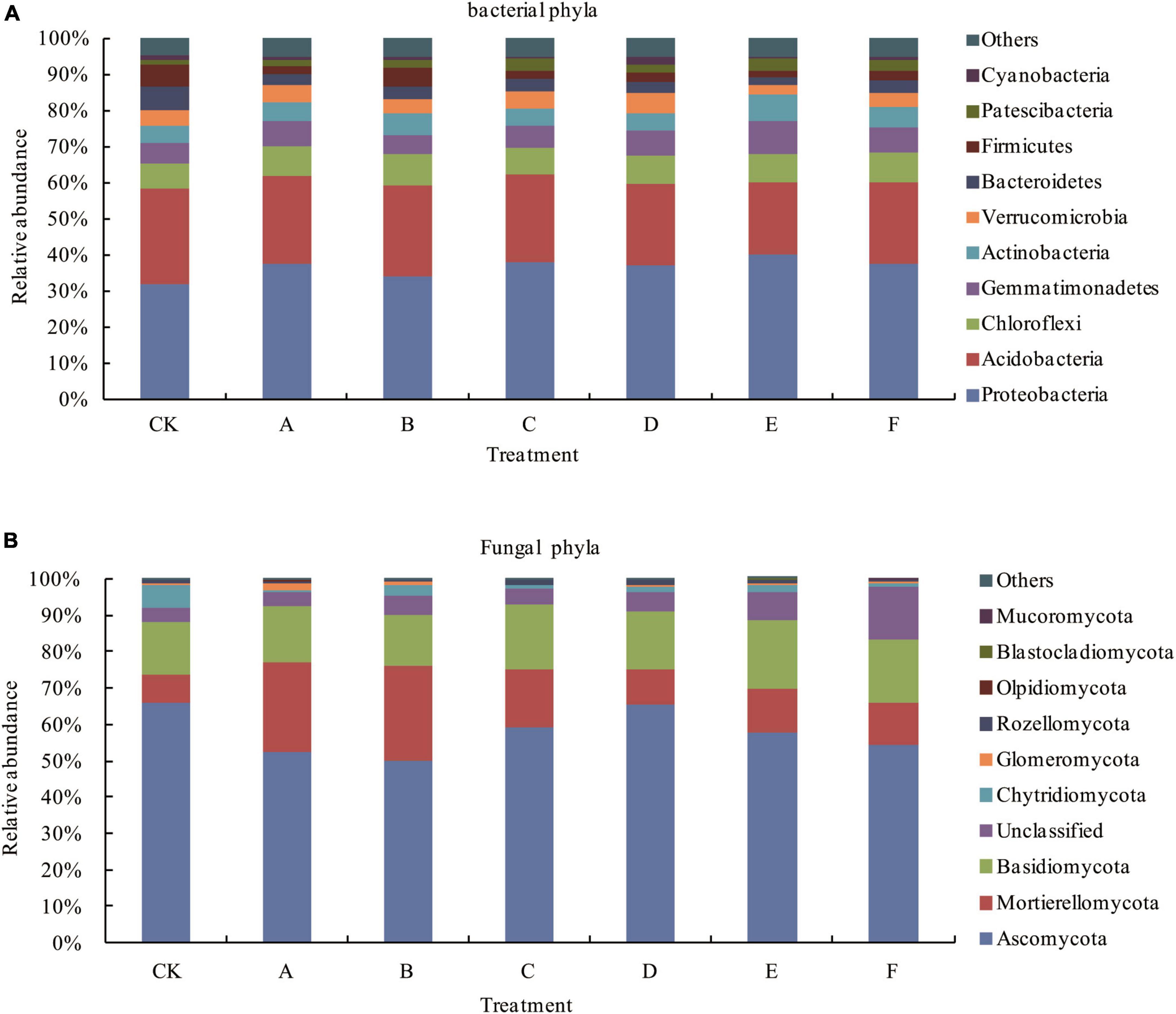
Figure 2. Comparative levels of bacterial (A) and fungal (B) phyla. Others: all species other than the top 10 species according to their relative abundance levels.
After applying Trichoderma, there was no change in the top 10 fungal population categories compared with that in CK; however, there were certain changes in their relative abundances, and the changes varied with different Trichoderma treatments. The top 10 soil fungal phyla by relative abundance under the various treatments were: Ascomycota, Mortierellomycota, Basidiomycota, Unclassified, Chytridiomycota, Glomeromycota, Rozellomycota, Olpidiomycota, Blastocladiomycota, and Mucoromycota. The sum of the comparative levels of Ascomycota, Mortierellomycota, and Basidiomycota reached 83.52–93.01% (Figure 2B). The relative abundance of Ascomycota in each Trichoderma treatment group was lower than that in CK and the relative abundance of Mortierellomycota was higher than that in CK. Except for treatment B, the relative abundance of Basidiomycota under all treatments was increased compared with that in CK.
3.4.2 Genus level classification of bacteria and fungi
As shown in Figure 3A, the bacterial community comprised a large number of rare species (Other) and Uncultured bacteria, with relatively low relative abundance and rich species. Except for treatment B, the relative abundance of Sphingomonas under Trichoderma treatment was increased compared with that in CK. The relative abundance of Gemmatimonas under each treatment was increased compared with that in CK, and except for treatments E and F, the comparative level of Bryobacter was increased compared with that in CK.
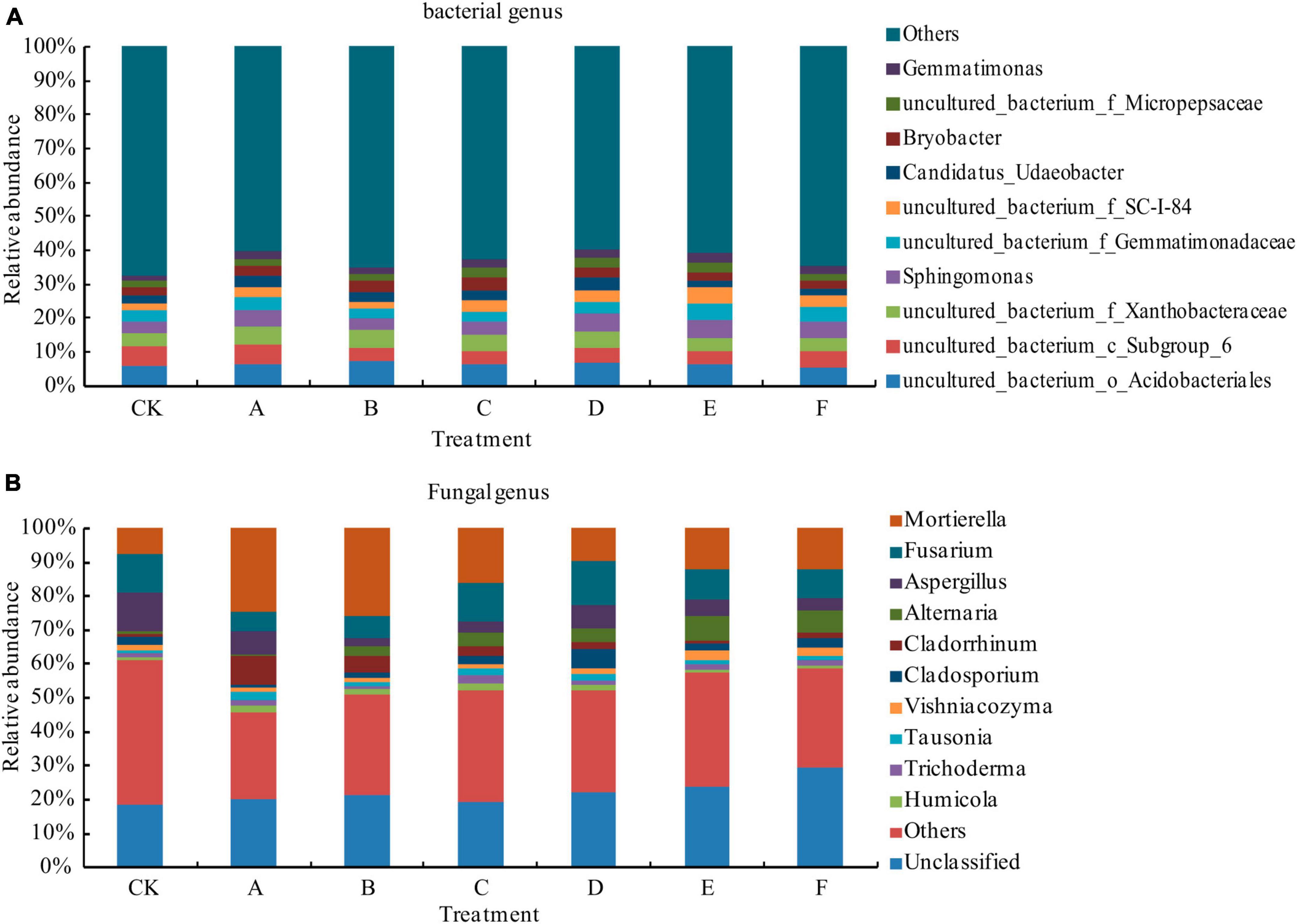
Figure 3. Comparative levels of bacterial (A) and fungal (B) genera. Others: all species other than the top 10 species according to their relative abundance levels.
As shown in Figure 3B, for fungal genera, the comparative level of Unclassified and Other fungi were high, indicating that there were more unclassified flora and rare species under each treatment, and the sum of their relative abundances reached 45.8–61.3%. The comparative level of Mortierella under each Trichoderma treatment was higher than that in CK. Except for treatment D, the comparative level of Fusarium was lower under Trichoderma treatment than that in CK, and except under treatment D, the comparative level of Trichoderma was increased compared with that in CK.
3.5 Difference analysis of the microbial communities in the soil
The LEfSe statistical result analysis (Figure 4) indicated that the bacterial species under C and F treatments did not differ significantly, thus these two groups were omitted from the analysis. Xanthobacteraceae and Rhizobiales in group A; Bifidobacterium in group B; Udaeobacter and Chthoniobacterales in group D; Proteobacteria, Gemmatimonadaceae, Sphingomonadaceae, and Thermoleophilia in Group E; and Acidobacteria and Bacteroidia in Group CK were identified as biomarkers. The fungal species under A, C, and F treatments did not differ significantly, thus these groups were omitted from the analysis. Mortierella in group B, Pyronemataceae in group D, Alternaria and Pleosporaceae in group E, and Aspergillus in group CK were identified as biomarkers (Figure 5).
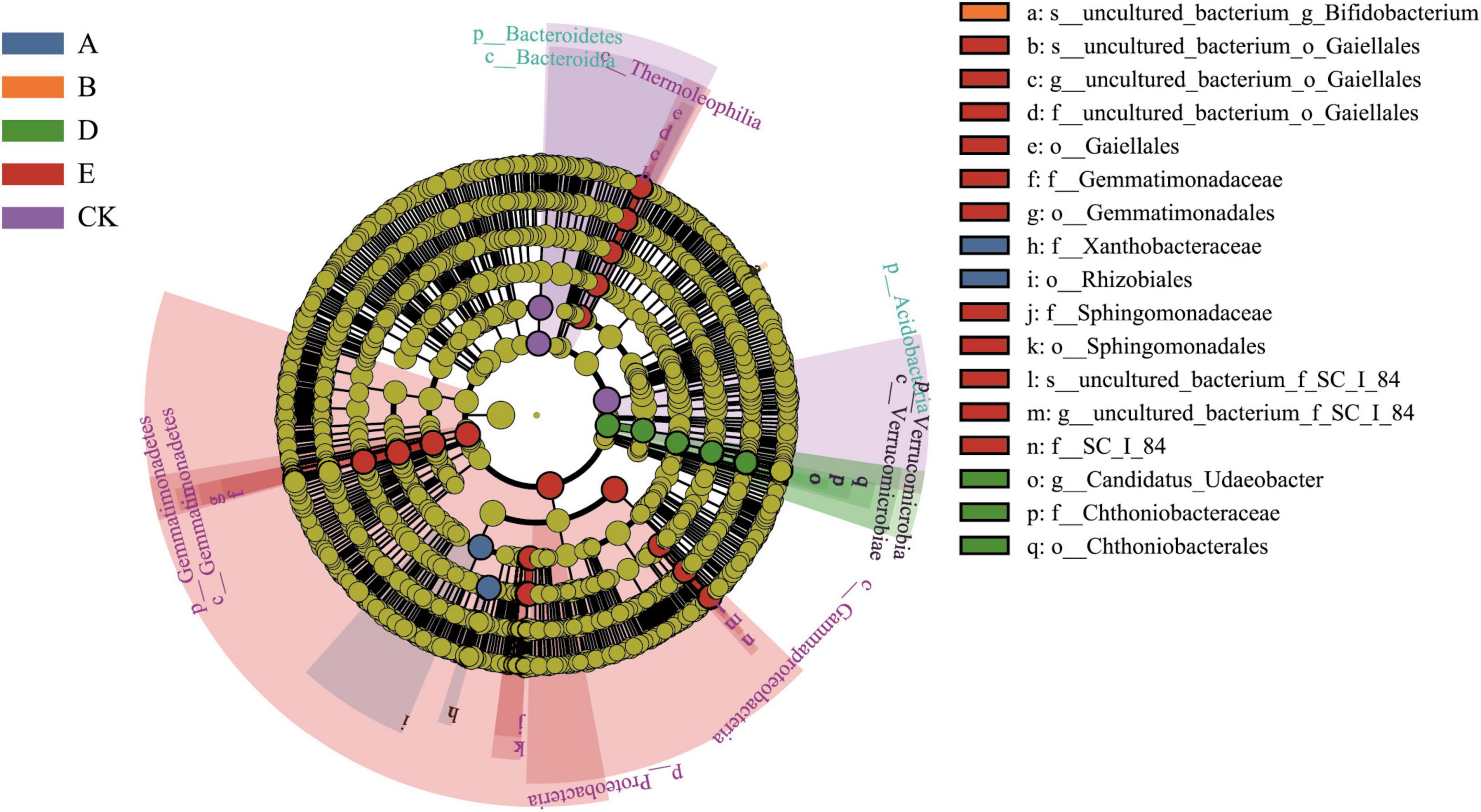
Figure 4. LEfSe analysis of the structure of the soil sample bacterial communities. The circle, from inside to outside, indicates bacteria from phylum to species, respectively. Yellow points indicate that bacteria had no notable differences among the treatment groups, and biomarker bacteria in the different treatments were classified using different colors.
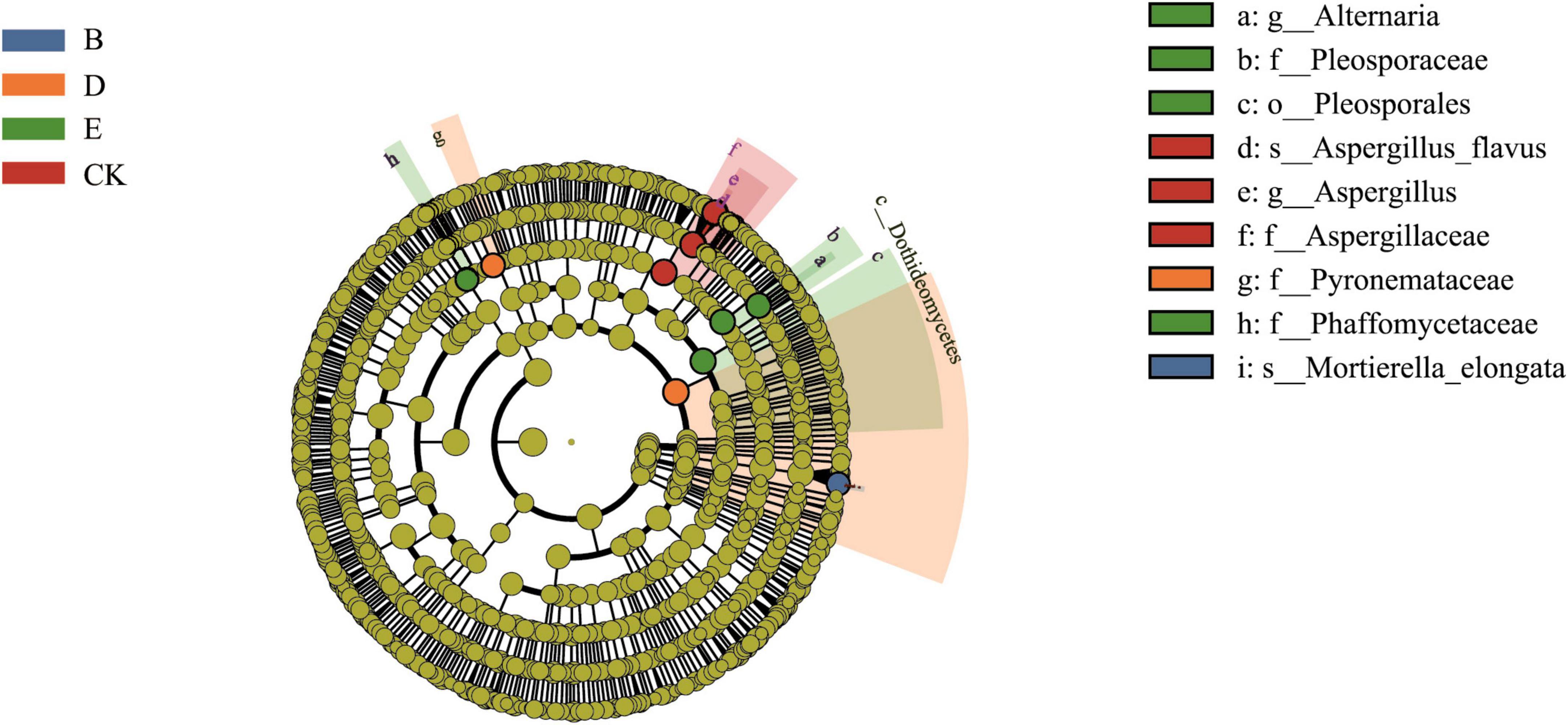
Figure 5. LEfSe analysis of the fungal community in soil samples. The circle, from inside to outside, indicates fungi from phylum to species, respectively. Yellow points indicate that fungi had no notable differences among the treatment groups, and biomarker fungi in the different treatments were classified using different colors.
3.6 RDA of the microbial communities and nutrients in the soil under different treatments
Figure 6A shows the RDA of the soil bacterial community structure and soil chemical properties. The first two axes of the RDA plot explain 53.31 and 22.09% of the total variance, respectively (75.40% combined). The Monte Carlo test results showed that available potassium (F = 2.7, P = 0.018) was the most important factor affecting the soil bacterial community, and 95% of the bacterial community variation between samples could be explained by this environmental factor. The environmental factors exerted their effects, from large to small, in the order: available potassium, salt, available phosphorus, pH value, and total nitrogen. As shown in Figure 6A, available potassium and available phosphorus correlated positively with the relative abundance of Proteobacteria and Gemmatimonadetes, and negatively with the relative abundance of Firmicutes.
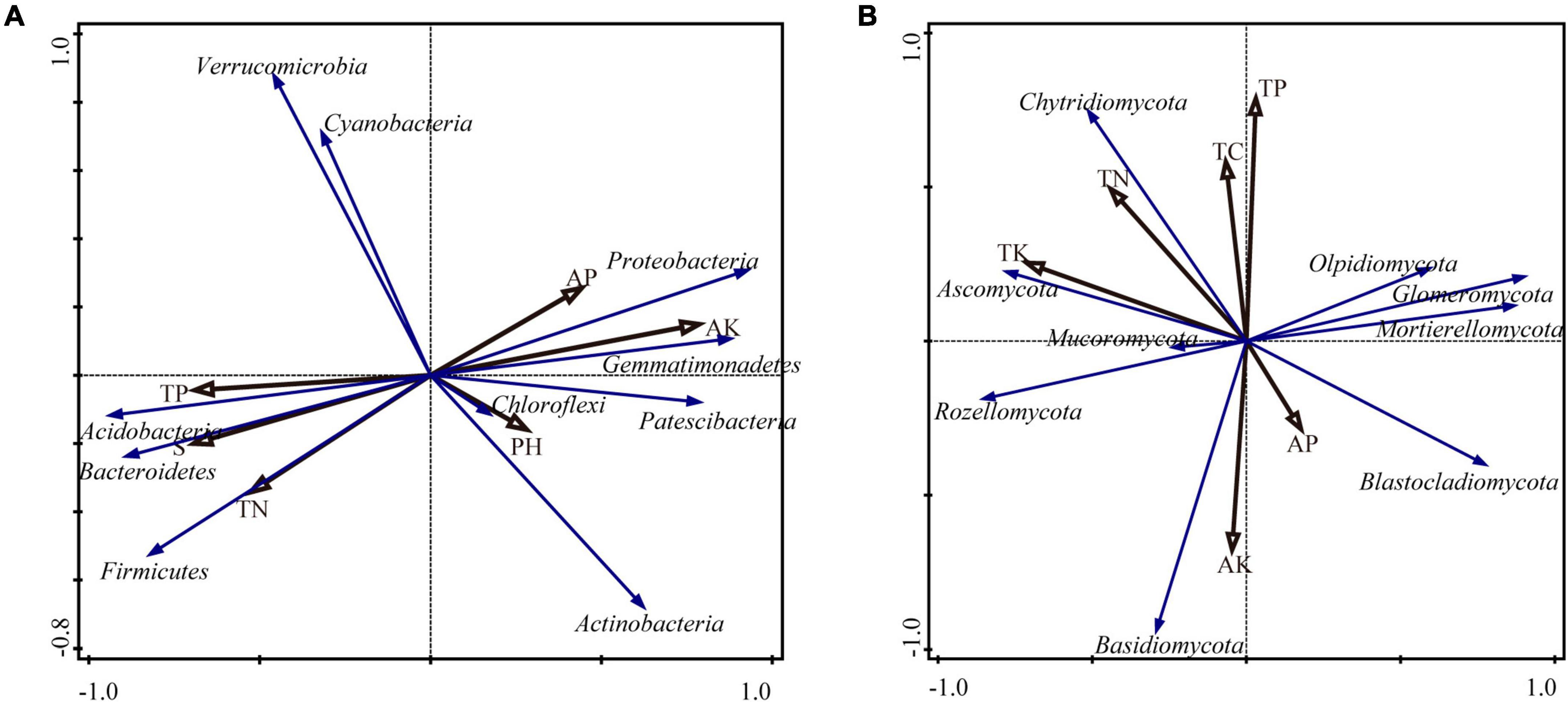
Figure 6. Analysis of redundancy for soil nutrients and the soil bacterial (A) and fungal (B) communities.
Figure 6B shows the RDA analysis of the soil structure of the fungal community structure and chemical properties of the soil. The first two axes of the RDA plot explain 47.29 and 20.00% of the total variance, respectively (67.29% combined). The Monte Carlo test results showed that total potassium (F = 2.3, P = 0.064) and total nitrogen (F = 3.5, P = 0.062) were the most important factors affecting the soil fungal community, and 98.3% of the fungal community variation between samples could be explained by these environmental factors. The environmental factors exerted their effects, from large to small, in the order: total potassium, total nitrogen, total phosphorus, available phosphorus, and available potassium. As shown in Figure 6B, total potassium and total nitrogen were positively correlated with Ascomycota and Chytridiomycota and negatively correlated with Blastocladiomycota.
4 Discussion
Soil degradation seriously affects the yield and quality of ginseng. Trichoderma spp. play an important role in improving plant biomass by influencing the soil environment (Meng et al., 2019; Wang et al., 2021). Therefore, it is necessary to screen high-efficiency Trichoderma strains that can increase the biomass of ginseng. Previous research demonstrated that T. koningiopsis could promote plant growth via increased levels of volatile organic compounds (You et al., 2022). After treatment with T. atroviride, the aerial and root dry weights of tomato increased (Rao et al., 2022). Herein, we found that five out of six Trichoderma species isolated from ginseng rhizosphere soil showed biomass promoting effects on ginseng plants, among which T. brevicompactum had the best effect on increasing ginseng fresh and dry weight, followed by T. velutinum and T. koningiopsis; and T. atroviride was beneficial to dry weight accumulation of ginseng. These results identified excellent Trichoderma materials to improve ginseng production.
Microorganisms are an important component of soil, and are closely related to soil health and quality (de Vries et al., 2020). Studies have shown that Trichoderma spp. can alter the soil microbiome. Zhang et al. (2020) found that inoculation with T. asperellum reduced fungal diversity and increased bacterial diversity. In particular, it increased the relative abundance of rhizosphere microorganisms that promote plant growth, such as Sphingomonas,Trichoderma,Actinomadura, Pseudomonas, and Rhodanobacter. Li et al. (2023) found that dual inoculation with dark septate endophytes and T. koningiopsis altered the microbial community structure in the rhizosphere, in which the levels of Acidobacteriae, Ascomycota, Firmicutes, and Actinobacteriota increased significantly, resulting in Vicinamibacteria and Trichoderma being enriched in the soil. After fumigation, Trichoderma application enhanced the relative abundance of beneficial microorganisms, which can improve the soil microbiome (Wu et al., 2022). In this study, we found that Trichoderma application reduced fungal richness, which might have been caused by the antagonism of Trichoderma against some pathogenic fungi. At the phylum level, all six Trichoderma species enhanced Proteobacteria levels, which were highest under treatment with T. koningiopsis (E). Certain Proteobacteria have major functions in increasing plant yield, such as nitrogen fixation, phosphorus solubilization, and plant growth promotion (Huang et al., 2020; Martínez, 2023), which help ginseng to absorb and utilize nutrients in the soil. At the genus level, all six Trichoderma species increased the relative abundance of Gemmatimonas. Except for treatment B, they increased the relative abundance of Sphingomonas and Trichoderma, and except for treatment D, they decreased the relative abundance of Fusarium. There was a significant and positive correlation between Gemmatimonas and soil nutrient components, and thus Gemmatimonas might be an indicator genus in response to changes in soil nutrient contents. Besides, Gemmatimonas can solubilize insoluble elements, such as phosphorus, and induce plant stress resistance or produce antifungal antibiotics, which have been proven to promote plant growth and enhance nutrient uptake (Shang and Liu, 2020; Liu C. et al., 2022). Recent studies have detailed the role of Sphingomonas species in plant growth promotion via the production of phytohormones and increased stress tolerance (Matsumoto et al., 2021; Jin et al., 2022b; Lombardino et al., 2022). Meanwhile, the relative abundance of Trichoderma increased after inoculation, indicating that Trichoderma could grow and reproduce in the treated soil. Root rot is the main disease responsible for decreases in the yield and quality of ginseng, and the main pathogen causing root rot is Fusarium (Guan et al., 2014; Wang et al., 2016). Fusarium is a common plant pathogen that can hinder the growth of many crops (Pegg et al., 2019; Srinivas et al., 2019; Kong et al., 2023). The observed decrease ofFusarium in the soil showed that the addition of Trichoderma inhibited Fusarium and could prevent root rot of ginseng. Therefore, the results of our study, combined with those of previous studies, indicated that inoculation with Trichoderma could promote the growth of beneficial microorganisms and decrease the proliferation of deleterious microbes.
Soil pH value is a key indicator of ginseng planting site selection (Kim et al., 2015), and many soil borne diseases are affected by the soil pH value. A decreased in soil pH might increase the incidence of ginseng root diseases (Jin et al., 2022a). Herein, we demonstrated that Trichoderma inoculation increased soil PH value, and the available phosphorus and available potassium contents, which was conducive to the prevention and control of ginseng soil-borne diseases and the promotion of ginseng growth. Thus, inoculation with Trichoderma, which colonized and grew in the soil and would secrete organic acids and other factors, changed the pH and increased the available phosphorus and available potassium content (Tekaya et al., 2018). In this study, Trichoderma species had different effects on soil physicochemical properties, among which T. velutinum (B), T. atroviride (D), and T. koningiopsis (E) improved the physicochemical properties. Parada et al. (2019) showed that inoculating microbials into acidic soil could alleviate soil acidification, which agreed with our findings. The enhancement of available phosphorus and available potassium contents might also have been caused by the activation of beneficial microbial populations in the soil after inoculation with Trichoderma (Qi et al., 2022). Beneficial microorganisms and their activities facilitate the transformation of plant nutrients from non-effective forms to effective forms, thereby improving soil fertility (Jain et al., 2015; Yuan et al., 2016). Saravanakumar et al. (2013) found that Trichoderma has a solubilizing effect on phosphate. Mao and Jiang (2021) reported that the contents of alkali-hydrolysable nitrogen, organic matter, available potassium, and available phosphorus in soil increased after application of T. hamatum, which was consistent with our results.
Soil properties might influence the structure and diversity of soil microbial communities (Khan et al., 2016; Sun et al., 2019). In this study, RDA was carried out at the species level for bacteria and fungi, and among soil nutrients, available K and available P were the most influential elements for the structure of the bacterial community, whereas total K had the largest influence the structure of the fungal community. This was consistent with the results of Zhu and Yu (2022). Available potassium and available phosphorus correlated positively with Proteobacteria and Blastomonas levels, indicating that application of Trichoderma could increase the contents of available potassium and phosphorus in ginseng soil, and increase the comparative levels of Proteobacteria and Blastomonas in soil, thus promoting ginseng growth.
5 Conclusion
The results of this study indicated that different Trichoderma spp. have different effects on the biomass of ginseng, and the chemical properties and microbiome of ginseng soil. Most of the selected Trichoderma spp. had beneficial effects on ginseng biomass, improved soil nutrients, increased the relative abundance of beneficial microbial populations (such as Gemmatimonas, Sphingomonas, and Trichoderma), and reduced the relative abundance of harmful microbial populations (such as Fusarium). In particular, T. koningiopsis (E) was superior to the other tested species and has potential for application. This study provides a theoretical basis for soil improvement and biological control of diseases in ginseng cultivation. However, the limitations of greenhouse cultivation mean that additional long-term field experiments should be conducted with different climatic conditions.
Data availability statement
The datasets presented in this study can be found in online repositories. The names of the repository/repositories and accession number(s) can be found below: https://www.ncbi.nlm.nih.gov/, PRJNA1064685, PRJNA1064678.
Author contributions
LZ: Data curation, Methodology, Validation, Visualization, Writing—original draft. QJ: Data curation, Formal analysis, Software, Writing—review and editing. YG: Conceptualization, Data curation, Formal analysis, Writing—review and editing. ZL: Data curation, Formal analysis, Methodology, Writing—review and editing. XP: Resources, Validation, Writing—review and editing. YuZ: Data curation, Methodology, Software, Writing—review and editing. YaZ: Data curation, Methodology, Validation, Writing—review and editing. QW: Conceptualization, Formal analysis, Funding acquisition, Methodology, Project administration, Resources, Supervision, Validation, Visualization, Writing—review and editing.
Funding
The author(s) declare financial support was received for the research, authorship, and/or publication of this article. This work was supported by the Jilin Provincial Science and Technology Department (No. 20220202114NC).
Conflict of interest
The authors declare that the research was conducted in the absence of any commercial or financial relationships that could be construed as a potential conflict of interest.
Publisher’s note
All claims expressed in this article are solely those of the authors and do not necessarily represent those of their affiliated organizations, or those of the publisher, the editors and the reviewers. Any product that may be evaluated in this article, or claim that may be made by its manufacturer, is not guaranteed or endorsed by the publisher.
References
Bolger, A. M., Lohse, M., and Usadel, B. (2014). Trimmomatic: a flexible trimmer for Illumina sequence data. Bioinformatics 30, 2114–2120. doi: 10.1093/bioinformatics/btu170
Bolyen, E., Rideout, J. R., Dillon, M. R., Bokulich, N. A., Abnet, C. C., Al-Ghalith, G. A., et al. (2019). Reproducible, interactive, scalable and extensible microbiome data science using QIIME 2. Nat. Biotechnol. 37, 852–857. doi: 10.1038/s41587-019-0209-9
Chen, J. L., Sun, S. Z., Miao, C. P., Wu, K., Chen, Y. W., Xu, L. H., et al. (2016). Endophytic Trichoderma gamsii YIM PH30019: a promising biocontrol agent with hyperosmolar, mycoparasitism, and antagonistic activities of induced volatile organic compounds on root-rot pathogenic fungi of Panax notoginseng. J. Ginseng Res. 40, 315–324. doi: 10.1016/j.jgr.2015.09.006
de Vries, F. T., Griffiths, R. I., Knight, C. G., Nicolitch, O., and Williams, A. (2020). Harnessing rhizosphere microbiomes for drought-resilient crop production. Science 368, 270–274. doi: 10.1126/science.aaz5192
Edgar, R. C. (2013). UPARSE: highly accurate OTU sequences from microbial amplicon reads. Nat. Methods 10, 996–998. doi: 10.1038/nmeth.2604
Edgar, R. C., Haas, B. J., Clemente, J. C., Quince, C., and Knight, R. (2011). UCHIME improves sensitivity and speed of chimera detection. Bioinformatics 27, 2194–2200. doi: 10.1093/bioinformatics/btr381
Fu, J. C., Wu, J., Xu, M., Ma, J., Long, L. L., Chen, C., et al. (2023). Effects of simulated warming on soil properties and bacterial community composition in the Bashania faberi ecosystem. Appl. Soil Ecol. 192:105091. doi: 10.1016/j.apsoil.2023.105091
Gardes, M., and Bruns, T. D. (1993). ITS primers with enhanced specificity for basidiomycetes-application to the identification of mycorrhizae and rusts. Mol. Ecol. 2, 113–118. doi: 10.1111/j.1365-294x.1993.tb00005.x
Guan, Y. M., Lu, B. H., Wang, Y., Gao, J., and Wu, L. J. (2014). First report of root rot caused by Fusarium redolens on ginseng (Panax ginseng) in Jilin Province of China. Plant Dis. 98, 844–844. doi: 10.1094/PDIS-09-13-0922-PDN
Huang, M., Tian, A., Chen, J., Cao, F., Chen, Y., and Liu, L. (2020). Soil bacterial communities in three rice-based cropping systems differing in productivity. Sci. Rep. 10:9867. doi: 10.1038/s41598-020-66924-8
Huang, Z., Zhao, F., Li, Y. H., Zhang, J. W., and Feng, Y. Z. (2017). Variations in the bacterial community compositions at different sites in the tomb of Emperor Yang of the Sui Dynasty. Microbiol. Res. 196, 26–33. doi: 10.1016/j.micres.2016.12.004
Jain, A., Singh, A., Singh, S., and Singh, H. B. (2015). Biological management of Sclerotinia sclerotiorum in pea using plant growth promoting microbial consortium. J. Basic Microbiol. 55, 961–972. doi: 10.1002/jobm.201400628
Jamil, A. (2021). Antifungal and plant growth promoting activity of Trichoderma spp. against Fusarium oxysporum f. sp. lycopersici colonizing tomato. J. Plant Protection Res. 61, 243–253. doi: 10.24425/jppr.2021.137950
Javeria, S., Kumar, A., Kharkwal, A. C., Varma, A., and Sharma, P. (2020). Exploiting plant growth promoting Trichoderma for lentil wilt management. Indian J. Agric. Sci. 90, 1020–1024. doi: 10.56093/ijas.v90i5.104387
Jin, Q., Zhang, Y. Y., Ma, Y. Y., Sun, H., Guan, Y. M., Liu, Z. B., et al. (2022a). The composition and function of the soil microbial community and its driving factors before and after cultivation of Panax ginseng in farmland of different ages. Ecol. Indic. 145:109748. doi: 10.1016/j.ecolind.2022.109748
Jin, Q., Zhang, Y. Y., Wang, Q. X., Li, M. J., Sun, H., Liu, N., et al. (2022b). Effects of potassium fulvic acid and potassium humate on microbial biodiversity in bulk soil and rhizosphere soil of Panax ginseng. Microbiol. Res. 254:126914. doi: 10.1016/j.micres.2021.126914
Kai, T., and Adhikari, D. (2021). Effect of organic and chemical fertilizer application on apple nutrient content and orchard soil condition. Agriculture 11:340.
Khan, K. S., Mack, R., Castillo, X., Kaiser, M., and Joergensen, R. G. (2016). Microbial biomass, fungal and bacterial residues, and their relationships to the soil organic matter C/N/P/S ratios. Geoderma 271, 115–123. doi: 10.1016/j.geoderma.2016.02.019
Kim, C., Choo, G. C., Cho, H. S., and Lim, J. T. (2015). Soil properties of cultivation sites for mountain-cultivated ginseng at local level. J. Ginseng Res. 39, 76–80. doi: 10.1016/j.jgr.2014.06.004
Kong, W. B., Xie, Z. J., Huo, H. R., Jia, P. F., Zhang, A. M., Liang, J. Y., et al. (2023). Anti-Fusarium activity of essential oil distilled from artemisinin (Artemisia annua L.) extraction residues. S. Afr. J. Bot. 158, 180–189. doi: 10.1016/j.sajb.2023.05.010
Li, S., Shang, X. J., Luo, Q. X., Yan, Q., and Hou, R. (2023). Effects of the dual inoculation of dark septate endophytes and Trichoderma koningiopsis on blueberry growth and rhizosphere soil microorganisms. FEMS Microbiol. Ecol. 99:fiad008. doi: 10.1093/femsec/fiad008
Liu, C., Zhuang, J. Y., Wang, J., Fan, G. H., Feng, M., and Zhang, S. T. (2022). Soil bacterial communities of three types of plants from ecological restoration areas and plant-growth promotional benefits of Microbacterium invictum (strain X-18). Front. Microbiol. 13:926037. doi: 10.3389/fmicb.2022.926037
Liu, L., Xu, Y. S., Cao, H., Fan, Y., Du, K., Bu, X., et al. (2022). Effects of Trichoderma harzianum biofertilizer on growth, yield, and quality of Bupleurum chinense. Plant Direct 6:e461. doi: 10.1002/pld3.461
Lombardino, J., Bijlani, S., Singh, N. K., Wood, J. M., Barker, R., and Gilroy, S. (2022). Genomic characterization of potential plant growth-promoting features of sphingomonas strains isolated from the international space station. Microbiol. Spectr. 10:e0199421. doi: 10.1128/spectrum.01994-21
Ma, Y. Y., Jin, Q., Guan, Y. M., Zhang, P., Pan, X. X., Zhang, Y., et al. (2023). Effects of Trichoderma hamatum and T. harzianum on the physicochemical properties and fungal community structure in Panax ginseng-growing soil. Soil Fertil. Sci. China 7, 100–107. doi: 10.11838/sfsc.1673-6257.22370
Mao, T. T., and Jiang, X. L. (2021). Changes in microbial community and enzyme activity in soil under continuous pepper cropping in response to Trichoderma hamatum MHT1134 application. Sci. Rep. 11:21585. doi: 10.1038/S41598-021-00951-X
Martin, M. (2011). Cutadapt removes adapter sequences from high-throughput sequencing reads. EMBnet J. 17, 10–12. doi: 10.14806/ej.17.1.200
Martínez, S. (2023). Soil microbial community structure in rice rotation systems with crops and pastures in temperate Uruguay. Appl. Soil Ecol. 188:104929. doi: 10.1016/j.apsoil.2023.104929
Matsumoto, H., Fan, X., and Wang, Y. (2021). Bacterial seed endophyte shapes disease resistance in rice. Nat. Plants 7, 60–72. doi: 10.1038/S41477-020-00826-5
Meng, X. H., Miao, Y. Z., Liu, Q. M., Ma, L., Guo, K., Liu, D. Y., et al. (2019). TgSWO from Trichoderma guizhouense NJAU4742 promotes growth in cucumber plants by modifying the root morphology and the cell wall architecture. Microb. Cell Fact. 18:148. doi: 10.1186/s12934-019-1196-8
Parada, J., Valenzuela, T., Gómez, F., Tereucán, G., García, S., Cornejo, P., et al. (2019). Effect of fertilization and arbuscular mycorrhizal fungal inoculation on antioxidant profiles and activities in Fragaria ananassa fruit. J. Sci. Food Agric. 99, 1397–1404. doi: 10.1002/jsfa.9316
Pegg, K. G., Coates, L. M., O’Neill, W. T., and Turner, D. W. (2019). The epidemiology of Fusarium wilt of banana. Front. Plant Sci. 10:1395. doi: 10.3389/fpls.2019.01395
Qi, Y. Q., Liu, H. L., Zhang, B. P., Geng, M. X., Cai, X. X., Wang, J. H., et al. (2022). Investigating the effect of microbial inoculants Frankia F1 on growth-promotion, rhizosphere soil physicochemical properties, and bacterial community of ginseng. Appl. Soil Ecol. 172:104369. doi: 10.1016/j.apsoil.2021.104369
Quast, C., Pruesse, E., Yilmaz, P., Gerken, J., Schweer, T., Yarza, P., et al. (2013). The SILVA ribosomal RNA gene database project: improved data processing and web-based tools. Nucleic Acids Res. 41, D590–D596. doi: 10.1093/nar/gks1219
Rao, Y. X., Zeng, L. Z., Jiang, H., Mei, L., and Wang, Y. J. (2022). Trichoderma atroviride LZ42 releases volatile organic compounds promoting plant growth and suppressing Fusarium wilt disease in tomato seedlings. BMC Microbiol. 22:88. doi: 10.1186/s12866-022-02511-3
Riaz, M., Rahman, N. U., Zia-Ul-Haq, M., Jaffar, H. Z. E., and Manea, R. (2019). Ginseng: a dietary supplement as immune-modulator in various diseases. Trends Food Sci. Technol. 83, 12–30. doi: 10.1016/j.tifs.2018.11.008
Saravanakumar, K., Shanmuga Arasu, V., and Kathiresan, K. (2013). Effect of Trichoderma on soil phosphate solubilization and growth improvement of Avicennia marina. Aquat. Bot. 104, 101–105. doi: 10.1016/j.aquabot.2012.09.001
Segata, N., Lzard, J., Waldron, L., Gevers, D., Miropolsky, L., Garrett, W., et al. (2011). Metagenomic biomarker discovery and explanation. Genome Biol. 12:R60. doi: 10.1186/gb-2011-12-6-r60
Shang, J., and Liu, B. (2020). Application of a microbial consortium improves the growth of Camellia sinensis and influences the indigenous rhizosphere bacterial communities. J. Appl. Microbiol. 130, 2029–2040. doi: 10.1111/jam.14927
Srinivas, C., Devi, D. N., Murthy, K. N., Mohan, C. D., and Nayaka, S. C. (2019). Fusarium oxysporum f. sp. lycopersici causal agent of vascular wilt disease of tomato: biology to diversity–a review. Saudi J. Biol. Sci. 26, 1315–1324. doi: 10.1016/j.sjbs.2019.06.002
Sun, H., Wang, Q. X., Liu, N., Li, L., Zhang, C. G., Liu, Z. B., et al. (2017). Effects of different leaf litters on the physicochemical properties and bacterial communities in Panax ginseng-growing soil. Appl. Soil Ecol. 111, 17–24. doi: 10.1016/j.apsoil.2016.11.008
Sun, H., Wang, Q. X., Zhang, L. L., Liu, N., Liu, Z. B., Lu, L., et al. (2019). Distinct leaf litter drive the fungal communities in Panax ginseng-growing soil. Ecol. Indic. 104, 184–194. doi: 10.1016/j.ecolind.2019.04.083
Tang, J. (2020). Research on scientific strategy and technology of agricultural non-point source pollution control. IOP Conf. Series Earth Environ. Sci. 514:032024. doi: 10.1088/1755-1315/514/3/032024
Tekaya, S. B., Guerra, T., Rodriguez, D., Dawson, J. O., and Hahn, D. (2018). Frankia diversity in host plant root nodules is independent of abundance or relative diversity of frankia populations in corresponding rhizosphere soils. Appl. Environ. Microbiol. 84:e02248-17. doi: 10.1128/AEM.02248-17
Wang, Q. X., Ma, Y. Y., Jin, Q., Guan, Y. M., Pan, X. X., Liu, N., et al. (2022a). A Trichoderma koningiopsis, root soak and its application. ZL 202111349818.0. Beijing: China National Intellectual Property Administration.
Wang, Q. X., Ma, Y. Y., Jin, Q., Pan, X. X., Liu, Z. B., and Zhang, S. N. (2022b). A Trichoderma viridescens, ginseng soil amendment and its application. ZL202111347796.4. Beijing: China National Intellectual Property Administration.
Wang, Q. X., Ma, Y. Y., Jin, Q., Zhang, Y., Liu, Z. B., and Yang, K. X. (2022c). A Trichoderma velutinum, fungicide and its application. ZL 202111347777.1. Beijing: China National Intellectual Property Administration.
Wang, Q. X., Ma, Y. Y., Jin, Q., Zhang, Y., Zhang, L. L., and Yang, K. X. (2022d). A Trichoderma brevicompactum, bacteriostatic agent for pathogenic bacteria of ginseng and its application. ZL202111349835.4. Beijing: China National Intellectual Property Administration.
Wang, Q. X., Ma, Y. Y., Jin, Q., Zhang, Y., Zhang, L. L., and Zhang, S. N. (2022e). A Trichoderma atroviride and its application in inhibiting pathogenic bacteria of ginseng. ZL 202111349795.3. Beijing: China National Intellectual Property Administration.
Wang, Q. X., Sun, H., Li, M. J., Xu, C. L., and Zhang, Y. Y. (2020). Different age-induced changes in rhizosphere microbial composition and function of Panax ginseng in transplantation mode. Front. Plant Sci. 11:563240. doi: 10.3389/fpls.2020.563240
Wang, R., Chen, D., Khan, R. A. A., Cui, J., Hou, J., and Liu, T. (2021). A novel Trichoderma asperellum strain DQ-1 promotes tomato growth and induces resistance to gray mold caused by Botrytis cinerea. FEMS Microbiol. Lett. 368, 125–128. doi: 10.1093/femsle/fnab140
Wang, Y., Guan, Y. M., Lu, B. H., and Gao, J. (2016). First report of Ginseng (Panax ginseng) root rot caused by Fusarium acuminatum in China. Plant Dis. 100, 525–526. doi: 10.1094/PDIS-03-15-0273-PDN
White, T. J., Bruns, T., Lee, S., and Taylor, J. (1990). “38–Amplification and direct sequencing of fungal ribosomal RNA genes for phylogenetics,” in PCR protocols, eds M. A. Innis, D. H. Gelfand, J. J. Sninsky, and T. J. White (New York, NY: Academic Press, Inc.), 315–322. doi: 10.1016/B978-0-12-372180-8.50042-1
Wu, J. J., Zhu, J. H., Zhang, D. Q., Cheng, H. Y., Hao, B. Q., Cao, A. C., et al. (2022). Beneficial effect on the soil microenvironment of Trichoderma applied after fumigation for cucumber production. PLoS One 17:e0266347. doi: 10.1371/journal.pone.0266347
You, J. Q., Li, G. Q., Li, C. H., Zhu, L. H., Yang, H. J., Song, R. H., et al. (2022). Biological control and plant growth promotion by volatile organic compounds of Trichoderma koningiopsis T-51. J. Fungi 8:131. doi: 10.3390/jof8020131
Yu, T., Yang, Y. Y., Kwak, Y. S., Song, G. G., Kim, M. Y., Rhee, M. H., et al. (2017). Ginsenoside Rc from Panax ginseng exerts anti-inflammatory activity by targeting TANK-binding kinase 1/interferon regulatory factor-3 and p38/ATF-2. J. Ginseng Res. 41, 127–133. doi: 10.1016/j.jgr.2016.02.001
Yuan, S. F., Li, M. Y., Fang, Z. Y., Liu, Y., Shi, W., Pan, B., et al. (2016). Biological control of tobacco bacterial wilt using Trichoderma harzianum amended bioorganic fertilizer and the arbuscular mycorrhizal fungi Glomus mosseae. Biol. Control 92, 164–171. doi: 10.1016/j.biocontrol.2015.10.013
Zhang, Y., Tian, C., Xiao, J. L., Wei, L., Tian, Y., and Liang, Z. H. (2020). Soil inoculation of Trichoderma asperellum M45a regulates rhizosphere microbes and triggers watermelon resistance to Fusarium wilt. AMB Express 10:189. doi: 10.1186/s13568-020-01126-z
Keywords: ginseng, Trichoderma spp., biomass promotion, soil nutrients, rhizosphere microbiome
Citation: Zhang L, Jin Q, Guan Y, Liu Z, Pan X, Zhang Y, Zhang Y and Wang Q (2024) Trichoderma spp. promotes ginseng biomass by influencing the soil microbial community. Front. Microbiol. 15:1283492. doi: 10.3389/fmicb.2024.1283492
Received: 26 August 2023; Accepted: 08 January 2024;
Published: 31 January 2024.
Edited by:
Puneet Singh Chauhan, National Botanical Research Institute (CSIR), IndiaCopyright © 2024 Zhang, Jin, Guan, Liu, Pan, Zhang, Zhang and Wang. This is an open-access article distributed under the terms of the Creative Commons Attribution License (CC BY). The use, distribution or reproduction in other forums is permitted, provided the original author(s) and the copyright owner(s) are credited and that the original publication in this journal is cited, in accordance with accepted academic practice. No use, distribution or reproduction is permitted which does not comply with these terms.
*Correspondence: Qiuxia Wang, wangqiuxia1018@126.com