- 1School of Biochemistry and Biotechnology, University of the Punjab, Lahore, Pakistan
- 2Faculty of Medicine, MAHSA University, Kuala Lumpur, Malaysia
- 3Department of Zoology, College of Science, King Saud University, Riyadh, Saudi Arabia
- 4Department of Cell Biology, School of Life Sciences, Central South University, Changsha, China
Poultry production occupies an important place in the economy of any country. High broiler production in recent years has badly affected its profitability due to bad feed quality, excessive use of chemotherapeutic agents, emergence of diverse pathogens, and the deficiencies in management practices during rearing cycle. Microbiological improvement of the meat quality using potential probiotics can be beneficial for broiler farming. Present study was initiated to isolate chicken gastrointestinal tract (GIT) bacteria with probiotic potential. To isolate probiotics from chicken gut, alimentary canal of chickens of known sizes and ages was suspended in ringers soln. Under shaking conditions for overnight followed by serial dilutions of ringers soln. Bacterial isolates were analyzed via growth curve analysis, biochemical testing using RapID™ NF Plus Panel kit, molecular characterization, antimicrobial activity assay, antibiotic sensitivity assay, GIT adherence assay, bile salt and gastric acid resistant assay, and cholesterol assimilation assay. Four bacteria isolated in present study were identified as Limosilactobacillus antri strain PUPro1, Lactobacillus delbrueckii strain PUPro2, Lacticaseibacillus casei strain PUPro3, and Ligilactobacillus salivarius strain PUPro4. L. delbrueckii strain PUPro2 grew extremely fast. All isolates exhibited exceptional resistance to increasing concentrations of NaCl and bile salts with value of p >0.5. L. delbrueckii strain PUPro2 adhered to chicken ileum epithelial cells and demonstrated the highest viable counts of 320 colony forming units (CFUs). Antagonistic action was found in all isolates against P. aeruginosa, B. subtilis, B. proteus, and S. aureus, with value of p >0.5. Antibiotic susceptibility testing showed sensitivity to all the antibiotics used. Cholesterol assimilation was detected in all bacteria, with values ranging from 216.12 to 192.2 mg/dL. All isolates exhibited γ-hemolysis. In future, these bacteria might be tested for their impact on broilers meat quality and growth and can be recommended for their use as supplements for broilers diet with positive impact on poultry production.
1 Introduction
In Pakistan, poultry is a dynamic livestock sector which has contributed to employment opportunities to 1.5 million people in last few years due to its massive development, i.e., at the rate of 7.5%. It contributes 40% to the total annual meat production, 5.76% to agricultural sector, and 12.6% to overall GDP of Pakistan (Akbar et al., 2023). At present, Pakistan poultry turnover is 1,190 billion rupees (Alam et al., 2023). In global poultry industry, current filet yield of 16 and 22% has been recorded by the slow and fast growing chickens, respectively (Baéza et al., 2022). Poultry goods production in Pakistan during last 3 years is described in detail in Supplementary Table S1 (Henchion et al., 2021).
However, this industry has been on the brink of collapse in Pakistan as in seven tehsils of Rawalpindi and District Mansehra due to limitations associated with broilers farming (Saman et al., 2023). These limitations include high cost of broilers feed, mortality due to diseases, improper nutrition, lack of disease control programs, poor hatching conditions, improper sanitation, and poor immunity in chickens. Additionally, farmers use antibiotics to reduce enteric illnesses and increase feed conversion ratio (FCR) and body weight gain (BWG) to meet increased demand for poultry products (Desiere et al., 2018; Ogbuewu et al., 2022). As a consequence, broiler meat is saturated with carcinogenic hormones and antibiotic growth promoters (AGPs). As antibiotic abuse has virtually outlawed them, so breeders are under pressure to find more ecologically benign ways to promote the broilers growth (Arsène et al., 2021). Poor waste management practices at poultry farms expose broilers during rearing procedures to deep litter producing a filthy environment where young chicks are more likely to die immediately after delivery (Yan et al., 2021).
To compensate these losses, chicken gut microbiome is being targeted by the veterinarians and microbiologists. Replacing the gut bacteria with potential probiotics has been reported to be an emerging alternative to AGPs (Dowarah et al., 2018; Reuben et al., 2019; Melese et al., 2023). Probiotics are the live microorganisms which improve host health when given in sufficient amounts (Reid et al., 2019). Probiotics, employed in poultry industry, are the inhabitants of chicken gut in most of the cases. They improve serum calcium (Ca) and phosphorus (P) availability thus strengthening the tibial bone (Rizzoli and Biver, 2020). They boost amino acid levels in broiler chickens, improving their flavor (Yadav et al., 2018). Probiotic-fed broilers have been observed to exhibit the reduced midsection fat and increased breast muscle (Zhang et al., 2020). Metabolites of probiotics have also been found to be associated with health promotion activities such as tryptamine, short chain fatty acids (SCFAs), tryptophan, vitamins, and bacteriocins play role in immune system homeostasis, cell to cell communication, and microbiota cross talk, provide resistance to pathogens gut colonization, promote integrity, structure, and function of gut, and activate toll-like receptors (TLRs) on binding with enterocytes leading to cytokine expression (Agus et al., 2018; Khan et al., 2020; Liu et al., 2022; He et al., 2023; Li et al., 2023). In addition to this, metabolites such as lauric acid, pantothenate acid, L-glutamic acid, and N-acetyl-L-aspartic acid have been reported to positively regulate the lipid metabolism in broilers (Wu et al., 2021; Zhang et al., 2021). Probiotic supplements, at an approximate amount of 0.5 g/kg, are reported to improve meat texture and pH in broilers (Mohammed et al., 2021).
The probiotics reported so far in literature include L. monocytogenes, E. faecalis, S. enteritidis, S. typhimurium, Bifidobacterium, Roseburia, Akkermansia, Propionibacterium, Faecalibacterium, L. reuteri I2, Pediococcus acidilactici I5, P. acidilactici C3, P. acidilactici I8, P. acidilactici I13, L. acidophilus, L. plantarum, L. brevis, L. salivarius and L. fermentum and Enterococcus faecium C14, Brevibacillus laterosporus S62, B. coagulans, B. licheniformis, and E. coli (Sanders et al., 2019; Sorescu et al., 2021; Tang et al., 2021; Gupta et al., 2023; Li et al., 2023). Among these, B. subtilis supplementation reduced broiler belly fat in a project (Liu et al., 2020). Lactobacillus sp. boosted chicken feed protein and crude protein retention (Wu et al., 2019). E. faecium NCIMB 11181 supplementation increased FCR (Wu et al., 2019; Yang et al., 2023). Limosilactobacillus fermentum PC-76 and PC-10 were found to be anti-Salmonella gallinarum (Mehmood et al., 2023).
Under natural circumstances, young chickens are well protected through feeding on parent feces which contain efficient microflora for protection against pathogenic microbes, but the chickens being reared under commercial settings are usually deprived of such beneficial microbes due to cleanliness maintained at the hatching place, i.e., incubators. Shell contamination of pathogens and the gastric secretion of HCl at eighteenth day of incubation exacerbate the composition of gut microflora. Therefore, the post-birth probiotic feed supplementation is crucial in chickens (Lutful, 2009; Ivanova and Denisenko, 2019). Although literature reports various chicken gut-associated bacteria with different probiotic potentials, however, in majority of the cases, a single bacterium does not exhibit wide range of probiotic characteristics simultaneously and cannot be recommended as a sole nutritional tool. They can only be used in combination with other bacteria. Present study has been initiated to isolate and characterize the GIT inhabiting bacteria which might exhibit maximum potential of exerting probiotic effects on chicken health as a single bacterium. For this purpose, we selected the 10 broilers with improved performance and hypothesized that we might find wholesome probiotic bacteria from their guts. These bacteria might be administered via broiler feed as a sole zootechnical tool for the wholesomeness of meat, better FCR, high-quality egg production, healthy weight gain, effective establishment of intestinal microflora, immune system modulation, and pathogen resistance.
2 Materials and methods
2.1 Sample collection and preparation
Healthy broiler chickens (n = 10) at 21 days of age were purchased from a butcher and slaughtered. The chickens were washed twice with distilled water followed by 70% ethanol, before the GIT sampling. Followed this, gut was aseptically removed and washed with 0.9% NaCl. Washed gut was minced in 225 mL of ringer solution and mixed well for an hour (Musikasang et al., 2009; Shamsudin et al., 2019).
2.2 Isolation and culturing of probiotics
De Man–Rogosa–Sharpe agar (MRS) (Merck KGaA, 64271 Darmstadt, EMD Millipore Corporation, Germany) and Mueller Hinton agar (MHA) (M0203, FLINN SCIENTIFIC) were used for isolation and characterization of probiotics, respectively. MRS was prepared and poured into petri plates and allowed to get solidified (Kumar and Kumar, 2015; Soemarie et al., 2022; Gupta et al., 2023). The original sample (200 μL) and its six serial dilutions (10−6 to 10−1) were poured on solidified agar plates. Plates were incubated at 37°C. Bacterial colonies obtained after 24 h were enumerated to determine CFUs/ml using the formula:
The isolated bacteria were preserved in the form of glycerol stocks for later use (Fadanka et al., 2022).
2.3 Molecular characterization by 16S rRNA gene sequence analysis
Using an organic extraction method, DNA was isolated from bacterial pellet (Gupta, 2019). Agarose (RXP 10121, RxBiosciences, Rockville) gels assessed DNA quality. Forty-five min after activation at 90 volts, the Gel Documentation System (4,191,354, BIO-RAD) revealed DNA bands under UV light (Green and Sambrook, 2019). Using particular primers F1 and R1 (Supplementary Table S2), 16S rDNA was amplified by polymerase chain reaction (PCR) equipment (MyGeneTM Series Thermal Cycler) (Beckers et al., 2016). The 12.5 μL master mix, 1 μL forward primer, 1 μL reverse primer, 2 μL template, 8 μL injection water, and 0.5 μL Taq polymerase were added to 20 μL PCR reaction mixture. PCR conditions used are as follows: initial denaturation at 94°C for 10–15 min, followed by 38 cycles of denaturation at 94°C for 30–40 s, annealing at 94°C for 30–40 s, extension at 72°C for 30 s, and final extension at 72°C for 10 min. The results of the PCR amplification were verified by gel electrophoresis. After inspecting the gel in UV light, the Gel Documentation System captured an image of it (Lorenz, 2012). PCR products were shipped to Macrogen, Inc., Korea, for DNA sequence analysis using Sanger chain termination method (Young, 2018).
2.4 Bioinformatics analysis
Sequencing results were downloaded in FASTA format. Basic Local Alignment Search Tool (BLAST) program1 from the National Center for Biotechnology Information (NCBI)2 was used to align and scan these sequences against bacterial database. Clustal Omega3 multiple sequence alignment was performed using NCBI consensus sequences of isolates and related bacteria. Phylogenetic tree was constructed using MEGA 11,4 after aligning the gaps. The neighbor joining statistical approach, maximum likelihood substitution method, and 1,000-repeat Bootstrap analysis were used to build phylogenetic trees (Sievers and Higgins, 2014).
2.5 Submission of DNA sequences to GenBank
Sequences of four isolates have been deposited in the publically accessible NCBI GenBank database. DNA sequences and their corresponding accession codes are listed in Supplementary Table S3.
2.5.1 Growth analysis
Bacterial growth was monitored by measuring the optical density of the cultures at 600 nm (OD600) at different time intervals (0, 3, 6, 9, 24, 27, 30, 51, 54, 57 h) using a UV–visible spectrophotometer (BioTek Equipment, Inc.). A growth curve was obtained by plotting the OD600 versus time. Growth assay was performed in triplicates using synchronized cultures.
2.6 Biochemical characterization by RapID™ NF plus panel system
For this biochemical study, we used the Remel RapID™ NF Plus Panel kit (Thermo Scientific™) qualitative micromethod (Maloney et al., 2014). RapID™ NF Plus Panel kit screened the isolates for the presence of arginine dihydrolase (ADH), aliphatic thiol (TRD), triglyceride (EST), p-nitrophenyl-phosphoesterase (PHS), p-nitrophenyl-N-acetyl-β-D-glucosaminidase (NAG), p-nitrophenyl-D-α glucosidase (α-Glu), p-nitrophenyl-β-D-glucosidase (β-Glu), p-nitrophenyl-β-D-galactosidase (ONPG), urea hydrolysis test (URE), glucose fermentation test (GLU), proline β-naphthylamidase test (PRO), pyrrolidonyl β-naphthylamidase test (PYR), γ-glutamyl β-naphthylamidase test (GGT), tryptophan β-naphthylamidase (TRY), N-benzyl-arginine naphthylamidase test (BANA), tryptophan fermentation test (IND), and nitrate utilization test (NO3).
2.7 Assays of probiotics
All the assays performed to assess the probiotic potential of present study bacteria were carried out in triplicates. This helped to validate the observed results. Synchronized cultures were used as inoculum in triplicate experiments.
2.7.1 NaCl tolerance assay
To test salt tolerance of present study bacteria, NaCl (ONLINESCIENCEMALL, Clay Palmerdale Road, Pinson, United States) concentrations of 0.2, 2, and 5% were selected. To get synchronized cultures, fresh overnight grown cultures (100 μL) were inoculated to MRS broth supplemented with different NaCl concentration. The OD600 was measured at 0 h. Following this, bacteria were cultured in shaking incubator (Bio-Techne, China) at 150 rpm and 37°C until the log phase was achieved. After that, bacterial growth was assessed by measuring OD600 at the end of exponential phase (Rocha-Ramírez et al., 2021).
2.7.2 HCl tolerance assay
Present study bacterial isolates were grown for overnight at 37°C under shaking conditions. Cultures were centrifuged using centrifuge machine (HERMLE, Z380) at 6,000 rpm, 4°C, and 10 min to get the pellet. MRS medium of three different pH values, i.e., 2, 3, and 5, was prepared in three separate tubes, each tube containing 2 mL medium. The pH was adjusted with HCl (AQUABOND Inc.). Each of these tubes were inoculated with pelleted bacterial cells and incubated for 3 h at shaker at 37°C. The OD600 was measured at 0 h (before incubation) and 3 h (after incubation) to measure the tolerance of bacteria against different pH values (Nawaz et al., 2017).
2.7.3 Bile salt tolerance assay
Bile salt tolerance was tested at 0.3% concentration of bile salt (Research Products International, United States). MRS broth without bile salts was used as control. Experimental solution was made by adding 0.3 g bile salts to 100 mL MRS broth. Both MRS broth and control were inoculated with 100 μL fresh overnight cultures. It was then cultured at 37°C and 250 rpm until the log phase was achieved. Using a spectrophotometer, OD600 was measured at 0 h and at log phase to test the bile salt tolerance in bacteria (Xu et al., 2020).
2.7.4 Cell adhesion assay
The chicken gut was kept at 4°C for 30 min in phosphate buffer saline (PBS) (G-Biosciences, United States) to release surface mucus and was cut into four 1 cm pieces of disinfected ileum. Each component was added in probiotic cell suspension at 37°C for different time intervals, i.e., 0, 30, 60, and 90 min. Ileum was collected and macerated to remove non-adherent bacteria, seeded on MHA agar plates, and incubated for 24 h at 37°C. CFUs in each plate were counted (Reuben et al., 2019).
2.7.5 Growth in the presence of pathogens
Isolates were tested against different pathogens, i.e., Pseudomonas aeruginosa, Bacillus subtilis, Bacillus proteus, and Staphylococcus aureus. The pathogen glycerol stocks (60 μL) and overnight grown cultures of probiotics (60 μL) were used as inoculum and added to MRS broth (5 mL). Control comprised of MRS broth inoculated with overnight grown probiotic culture (60 μL). Cultures were incubated under shaking conditions for 24 h at 37°C and 150 rpm. Growth of probiotics in the presence of pathogens was assessed by measuring OD600 (Cizeikiene and Jagelaviciute, 2021).
2.7.6 Antibiotic sensitivity profiling
The disk diffusion method was used to determine the antibiotic sensitivity profile of all isolates by measuring the inhibitory zone for each antibiotic. Tested antibiotics included amoxicillin (DMS Chemical Pharmaceutical Inc., Ltd, China), azithromycin (Jenpharm Life Sciences, Pakistan), cefadroxil (Wellona Pharma, India), velosef (GlaxoSmithKline, London), kanamycin (Kanto Chemical Co., Inc., Tokyo, Japan), and augmentin (MEDSAFE, New Zealand) (Jomehzadeh et al., 2020). Three concentrations of each of these antibiotics were consulted, i.e., 5, 10, and 15 μL.
2.7.7 Hemolytic assay
Autoclaved blood agar (Millipore, India) was poured into petri plates. After solidification, overnight bacterial cultures were streaked. These plates were incubated at 37°C for 24 h, and hemolytic activities were estimated. The presence of a clear, colorless, or light-yellow zone surrounding the colonies will show total red blood cell (RBC) lysis, i.e., β-hemolysis. A greenish to brownish discoloration of the media will reflect reduction of hemoglobin, i.e., α-hemolysis, and no change in media color will reflect the ϒ-hemolysis (Kumari VB et al., 2023).
2.7.8 Cholesterol assay
Cholesterol breakdown characteristics were evaluated by CHOD-PAP method using cholesterol liquicolor kit (PATHOZYME DIAGNOSTICS, India). The probiotic culture cuvette received 10 μL of cholesterol standard (STD). The standard was mixed with culture and incubated at 20–25°C for 5 min. Blank cuvette contained 10 μL STD. OD was measured at 500 nm. Cholesterol concentration was measured as follows:
2.8 Statistical analysis
After each test, the findings were summarized as a mean ± SD as triplicates of each experiment were conducted separately. One-way ANOVA statistical tests were run using SPSS version 18.0. Threshold for statistical significance was set at p ≤ 0.05.
3 Results
3.1 Isolation of probiotics
Probiotics were isolated from chicken intestines using MRS broth. Clear bacterial colonies appeared on the plates after 24 h of incubation at 37°C.
3.2 Molecular characterization
Present study bacteria were identified using molecular analysis (Supplementary Figure S1). Sequences of 16S rRNA gene have been deposited in GenBank database, and accession numbers were assigned (Supplementary Table S3).
3.3 Phylogeny analysis
Phylogenetic analysis revealed the close relation of L. delbrueckii strain PUPro2 with L. delbrueckii strain sample AAA with bootstrap value of 100. L. antri strain PUPro1 and L. panis strain DSM 6035 both originated from the shared clade of L. antri strain DSM 106038 and L. antri strain Kx146A4. L. casei strain PUPro3 originated from the same branch point as that of L. casei strain TA001 with strong bootstrap value of 100 showing their close relatedness. L. salivarius strain PUPro4 was closely related to Lactobacillus species OTU343 through strong bootstrap value of 100 (Figure 1).
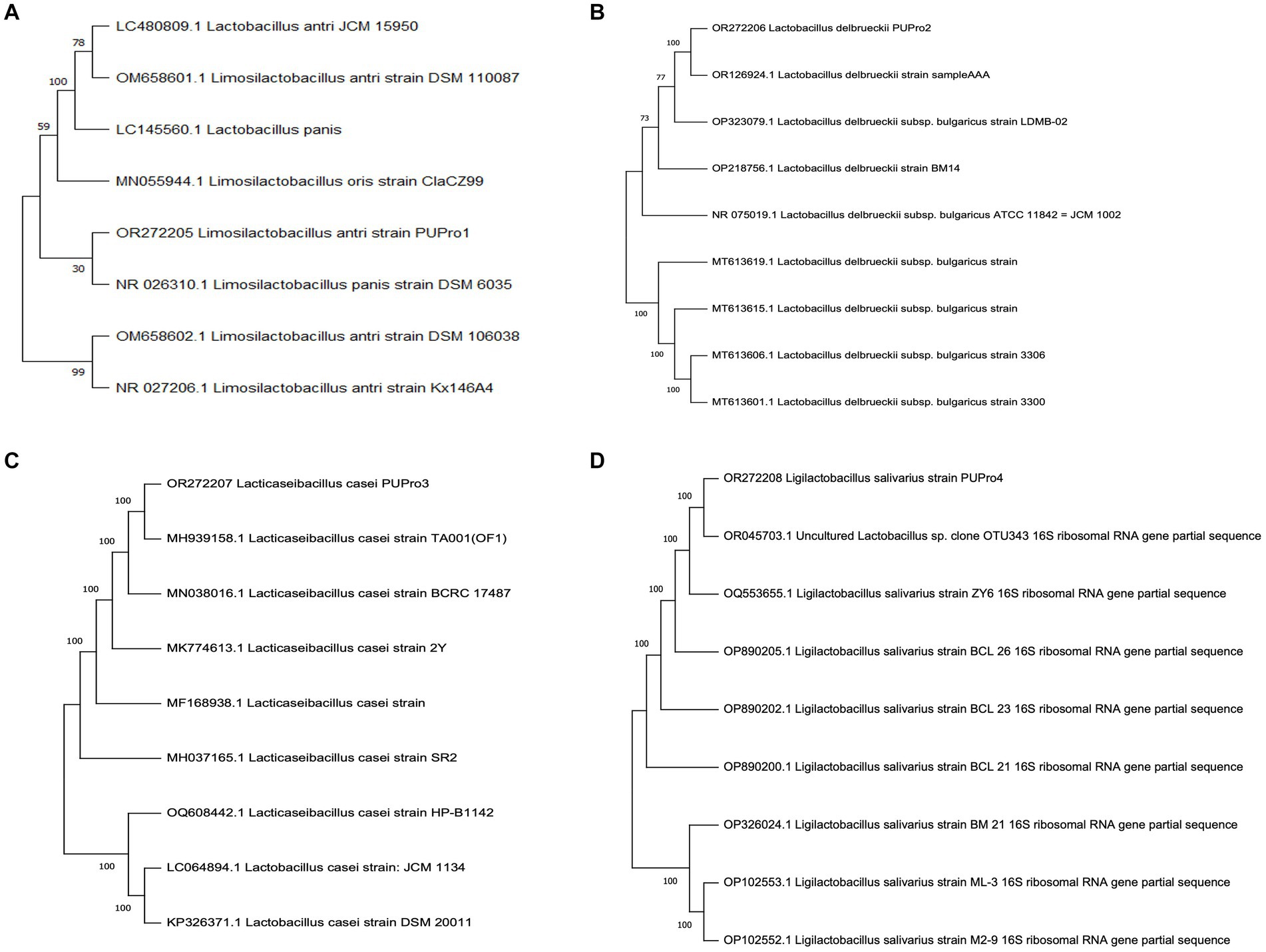
Figure 1. Phylogenetic trees constructed for present study bacteria using MEGA11. (A) Limosilactobacillus antri strain PUPro1. (B) Lactobacillus delbrueckii strain PUPro2. (C) Lacticaseibacillus casei strain PUPro3. (D) Ligilactobacillus salivarius strain PUPro4.
3.4 Growth analysis
All bacterial isolates were fast growing. L. antri strain PUPro1 entered the logarithmic phase at 24 h and exited after 57 h. L. delbrueckii strain PUPro2 grew logarithmically from 3 to 24 h. L. salivarius strain PUPro4 exhibited log phase of 9–24 h. L. casei strain PUPro3 grew logarithmically between 6 and 24 h (Figure 2; Supplementary Tables S4, S5).
3.5 Biochemical characterization
All isolates were tested positive for TRD and EST tests. Only L. antri strain PUPro1 was positive for PHS. All isolates fermented glucose except L. delbrueckii strain PUPro2 and L. casei strain PUPro3. All isolates were nitrate-reducing and NO3−positive. All the isolates showed negative results for ADH, NAG, α-GLU, β-GLU, PRO, PYR, GGT, TRY, BANA, IND, and ONPG assays (Supplementary Figure S2; Supplementary Table S6).
3.6 Assays of probiotics
3.6.1 NaCl tolerance assay
Each isolate survived at 0.2, 2, and 5% concentration of NaCl, and OD600 dropped slightly as NaCl increased from 0.2 to 2 and 5% (Figure 3). L. salivarius strain PUPro4 had the highest tolerance at 0.2 and 2% NaCl with OD600 = 0.984 and 0.929, respectively, while L. casei strain PUPro3 had the lowest OD600. L. salivarius strain PUPro4, with an OD600 of 0.922, was the most tolerant to 5% NaCl. Least tolerance was observed in case of L. antri strain PUPro1 with 0.714 (Table 1). Statistics showed value of p >0.05.
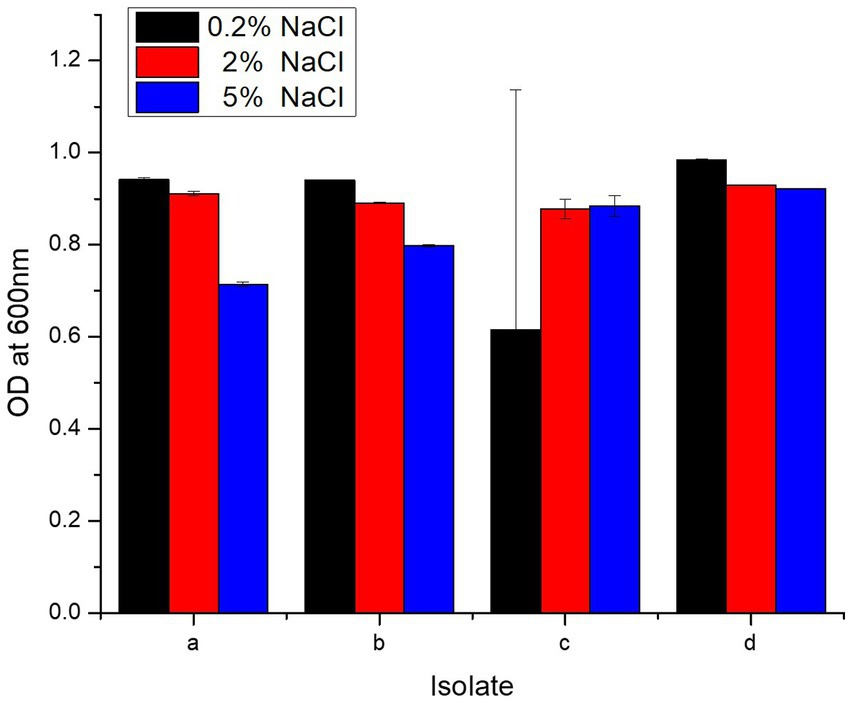
Figure 3. NaCl resistance potential of present study bacteria performed measuring OD600 at different NaCl concentrations. (a) L. antri strain PUPro1, (b) L. delbrueckii strain PUPro2, (c) L. casei strain PUPro3, (d) L. salivarius strain PUPro4.
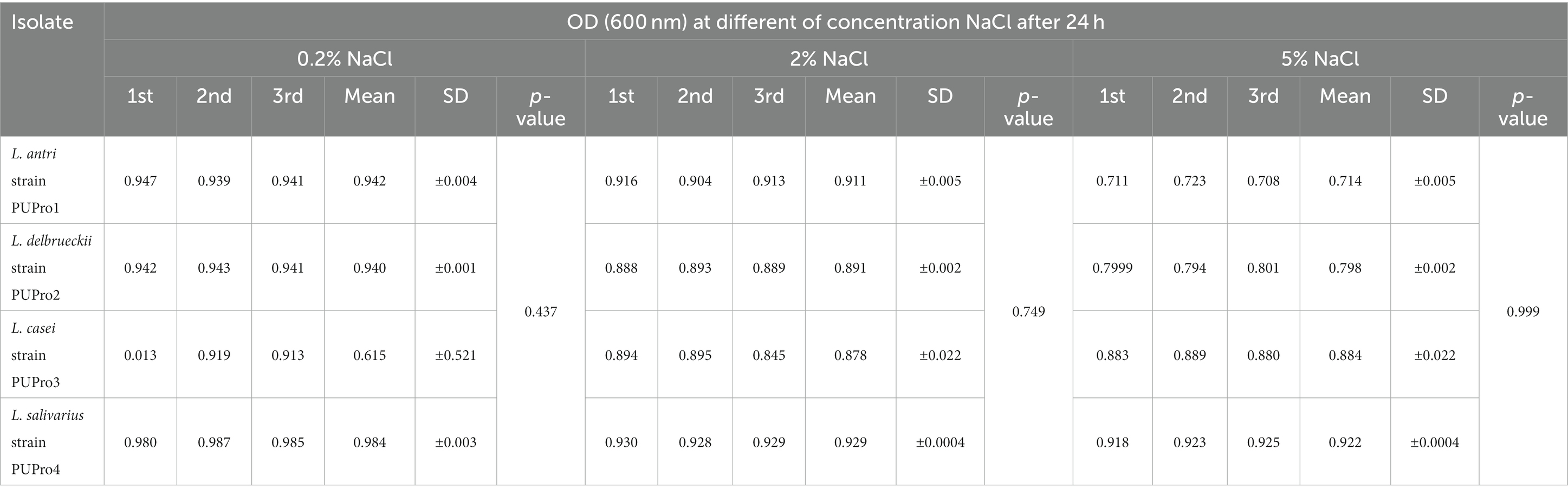
Table 1. Survival of present study bacterial strains at 0.2, 2, and 5% NaCl concentration estimated through measuring OD600 in the presence of bile salts.
3.6.2 HCl tolerance assay
Present study bacteria were analyzed for resistance against HCl via incubation in media of three different pH values, i.e., 2, 3, and 5. All the four isolates showed maximum tolerance to HCl at pH value of 3. Highest growth measured in terms of O.D600 at pH 3 was 1.60 ± 0.003, 1.56 ± 0.04, 1.54 ± 0.004, and 1.44 ± 0.001 for L. casei strain PUPro3, L. delbrueckii strain PUPro2, L. antri strain PUPro1, and L. salivarius strain PUPro4, respectively (Figure 4; Supplementary Table S7). These results showed that these bacteria are capable of surviving the acidity of stomach before reaching the host chicken intestine.
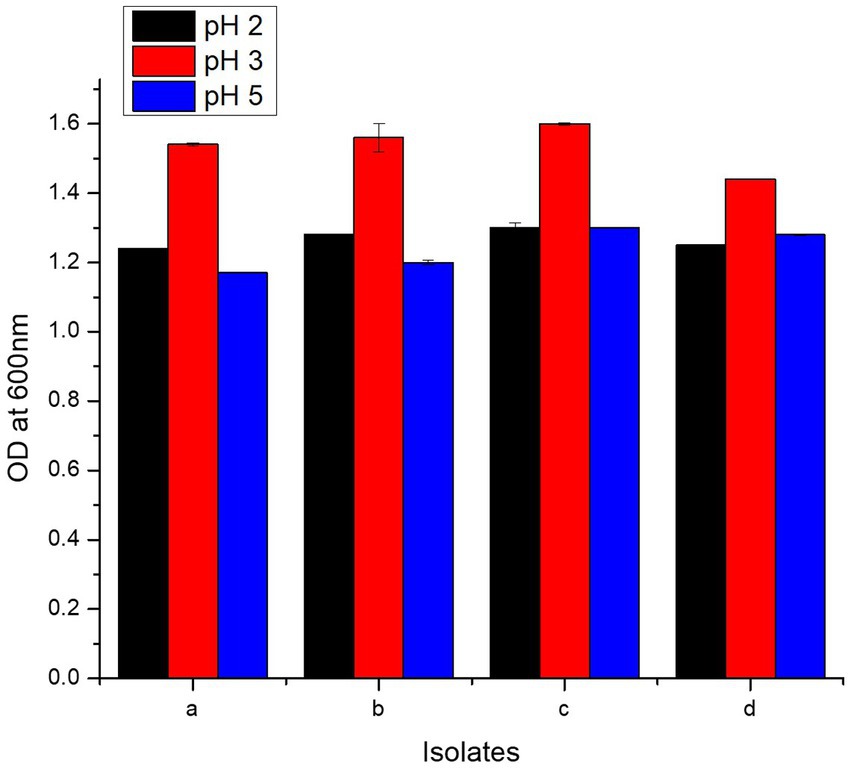
Figure 4. Comparison of HCl resistance potential of present study bacteria performed via measuring OD600 at different pH values. (a) Limosilactobacillus antri strain PUPro1. (b) Lactobacillus delbrueckii strain PUPro2. (c) Lacticaseibacillus casei strain PUPro3. (d)Ligilactobacillus salivarius strain PUPro4.
3.6.3 Bile salt tolerance assay
In bile salt tolerance test, all isolates performed well. After 24 h incubation at 37°C, the isolates with bile salts showed a marked increase in the OD600 value (Figure 5). L. casei strain PUPro3 exhibited the greatest OD600, i.e., 0.973 in the presence of bile salts, while L. delbrueckii strain PUPro2 had the lowest value of 0.851 (Table 2). Statistical analysis revealed significant results with value of p greater than 0.05.
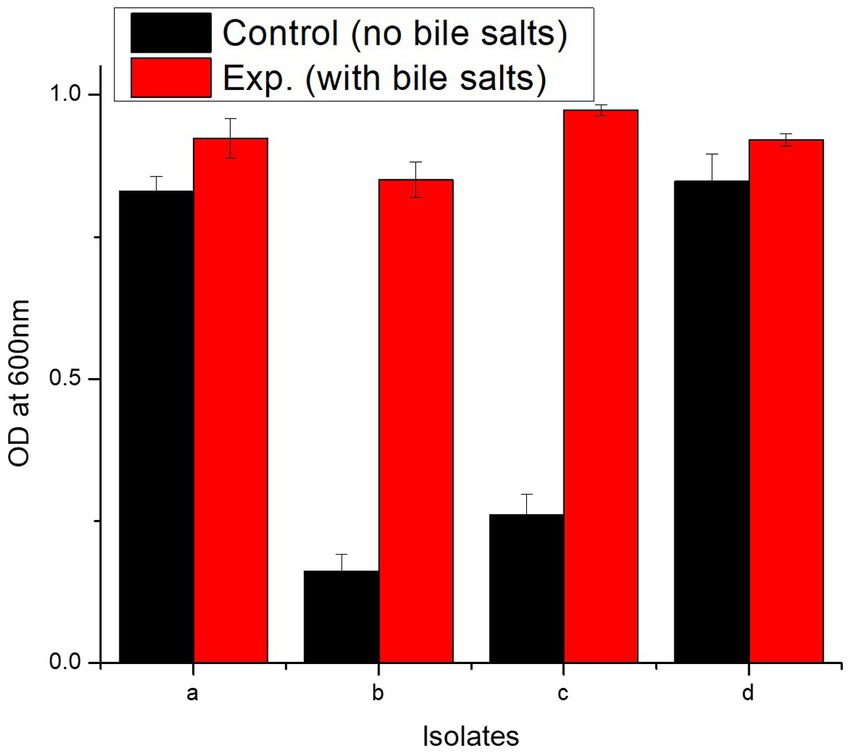
Figure 5. Characterization of probiotics reported in present study using bile salt tolerance assay and antimicrobial assay. (a) Comparison of OD600 of isolates in the absence and presence of bile salts. (b) Graphical representation of antimicrobial resistance of probiotic isolates against Pseudomonas aeruginosa, Bacillus subtilis, Bacillus proteus, and Staphylococcus aureus. (a) L. antri strain PUPro1, (b) L. delbrueckii strain PUPro2, (c) L. casei strain PUPro3, (d) L. salivarius strain PUPro4.
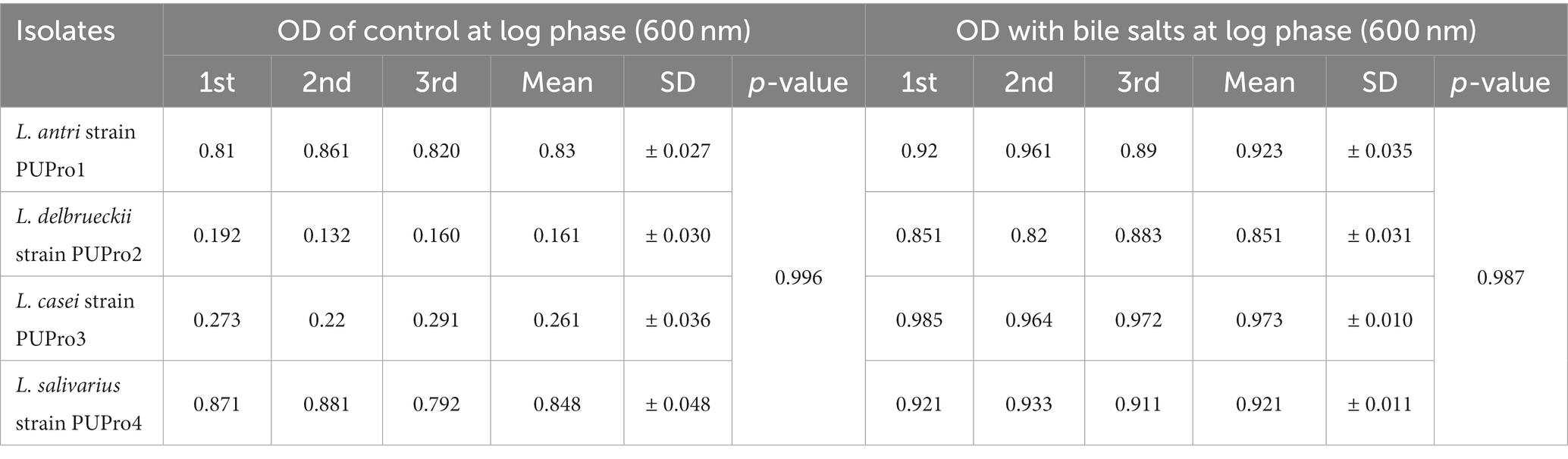
Table 2. Bile salt resistance estimated in present study bacterial strains through measurement of OD600 in the presence and absence of bile salts.
3.6.4 Cell adhesion assay
All isolates adhered to chicken ileum epithelial cells, and the number of CFUs increased as incubation time raised from 0 to 90 min (Supplementary Figure S3; Figure 6). Incubation for 0 min yielded zero CFU. L. delbrueckii strain PUPro2 adhered chicken ileum epithelial cells best and had the highest CFUs, i.e., 102.33, 261.33, and 312.67 after 30, 60, and 90 min of incubation, respectively. Least tendency of adherence was observed in case of L. casei strain PUPro3 (Table 3). The results were significant with p > 0.05.
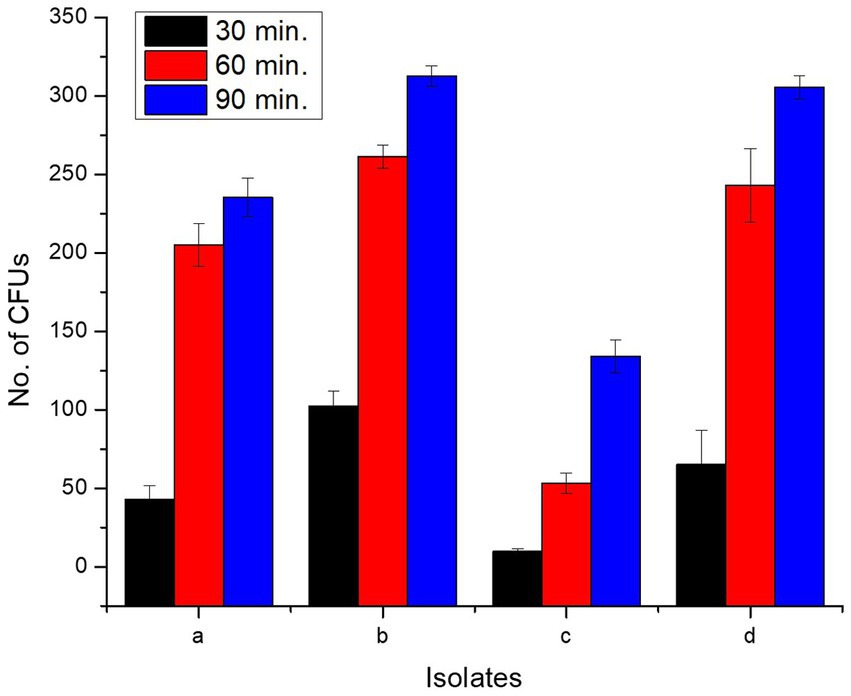
Figure 6. Comparison of CFUs of present study bacteria formed at different time intervals during cell adhesion assay indicating their ileum adhering tendency. (a) Limosilactobacillus antri strain PUPro1. (b) Lactobacillus delbrueckii strain PUPro2. (c) Lacticaseibacillus casei strain PUPro3. (d) Ligilactobacillus salivarius strain PUPro4.
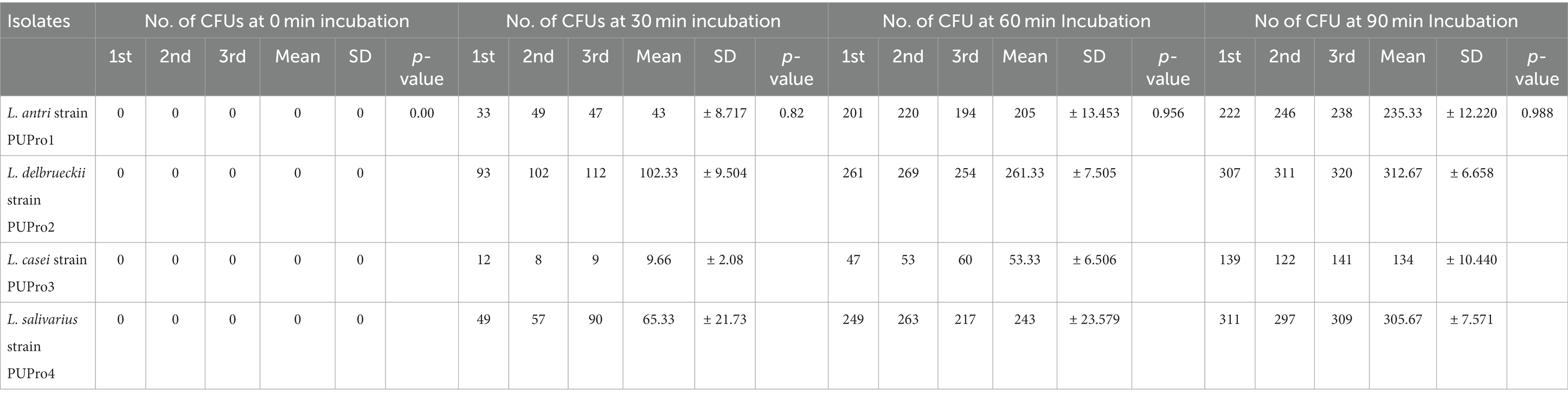
Table 3. Analysis of intestinal adhesion potential of present study isolates through determination of colony forming units (CFUs) at 0, 30, 60, and 90 min in cell adhesion assay.
3.6.5 Growth in the presence of pathogens
All isolates showed excellent tolerance against pathogens. L. delbrueckii strain PUPro2 had the highest tolerance against Pseudomonas aeruginosa with OD600 of 1.226, whereas L. casei strain PUPro3 had the lowest resistance with OD600 = 1.017 (Supplementary Table S8). L. casei strain PUPro3 exhibited highest resistance against B. subtilis with OD600 = 1.394, while L. delbrueckii strain PUPro2 showed least tolerance with OD600 of 1.127 (Supplementary Table S9). L. casei strain PUPro3 had highest OD600 value of 1.033 against B. proteus (Supplementary Table S10). L. salivarius strain PUPro4 had the highest OD600 (1.321) against Staphylococcus aureus, while in case of L. casei strain PUPro3, the value was 0.861 (Supplementary Table S11). Statistical analysis demonstrated the p-value of above 0.05 (Figure 7).
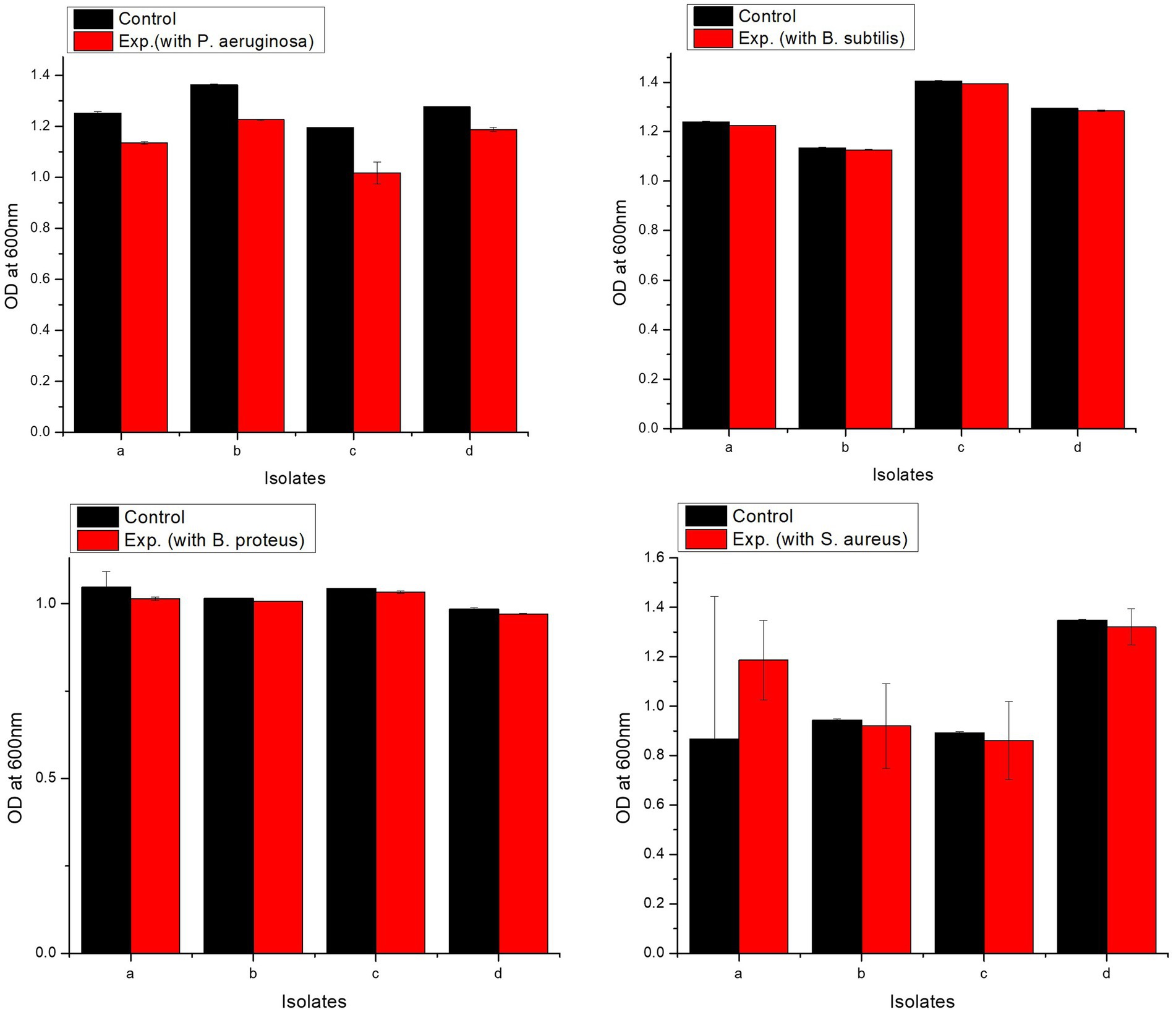
Figure 7. Analysis of resistance against pathogenic bacteria in present study isolates. Control comprised of medium and bacterium, while experimental tube contained medium, bacterium, and pathogen. (a) L. antri strain PUPro1, (b) L. delbrueckii strain PUPro2, (c) L. casei strain PUPro3, (d) L. salivarius strain PUPro4.
3.6.6 Antibiotic sensitivity profiling
All isolates were antibiotic-sensitive and exhibited zones of inhibition (Figure 8). In case of amoxicillin, cefadroxil, and kanamycin, highest zones of inhibition were observed in L. casei strain PUPro3, i.e., 34.33, 24.33, and 30.33 mm, respectively, while for azithromycin in L. antri strain PUPro1 (28.67 mm). For velosef and augmentin, largest zones were observed in L. salivarius strain PUPro4, i.e., 33.67 and 39.33 mm (Supplementary Table S12). Significant p > 0.05 were obtained for all the antibiotics.
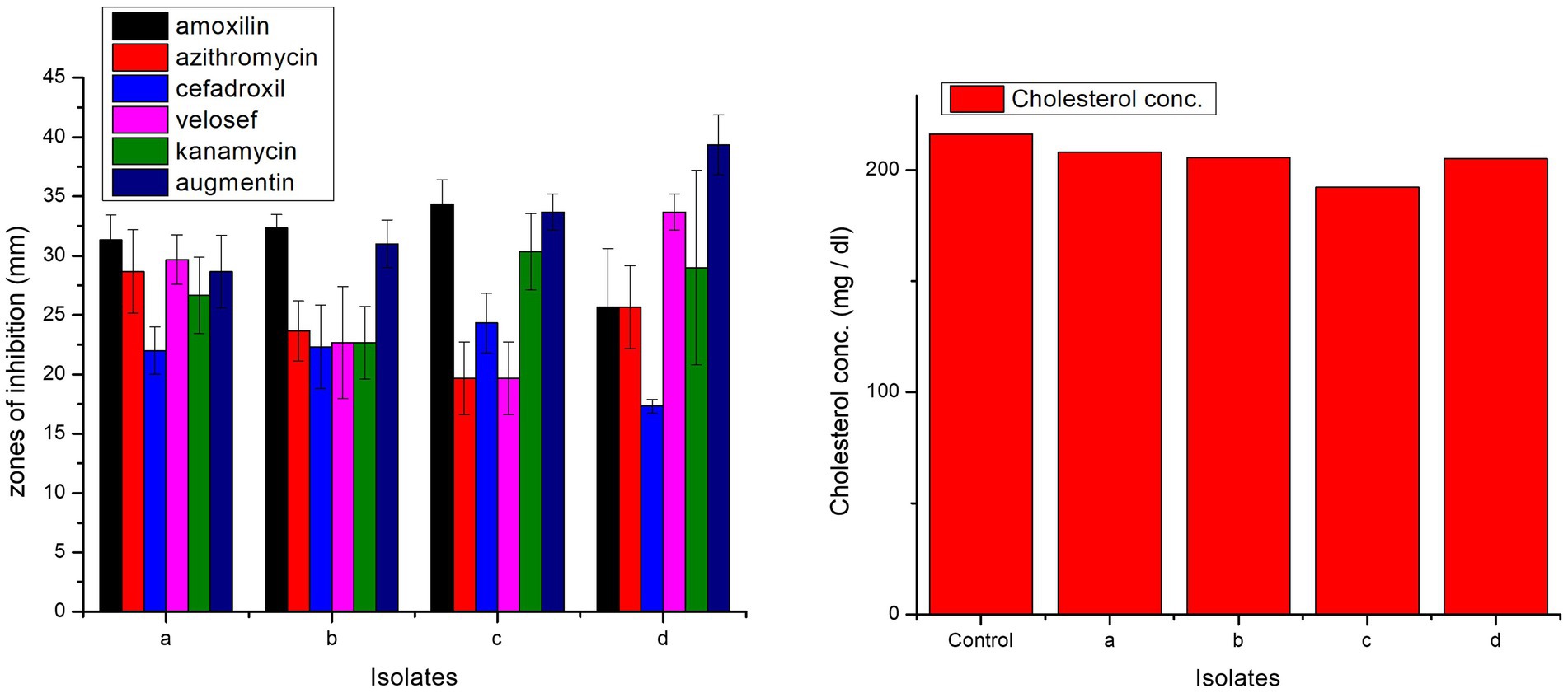
Figure 8. Comparison of zones of inhibition exhibited by present study bacteria against different antibiotics and comparison of cholesterol degradation potential of present study bacteria evaluated by CHOD-PAP method using cholesterol liquicolor kit. (a) L. antri strain PUPro1, (b) L. delbrueckii strain PUPro2, (c) L. casei strain PUPro3, (d) L. salivarius strain PUPro4.
3.6.7 Hemolytic assay
Since there was no change in color of media, so all isolates were found to show ϒ-hemolysis (Supplementary Table S13). No zones were observed around the colonies (Supplementary Figure S4), indicating no RBC lysis, making these isolates the safest and best.
3.6.8 Cholesterol assay
Among the four present study bacteria, L. casei strain PUPro3 significantly degraded cholesterol from 216.12 to 192.2 mg/dL. L. antri strain PUPro1 showed the lowest cholesterol assimilation, i.e., 208 mg/dL (Figure 8).
4 Discussion
Poultry serves as major source of animal protein in the form of meat and eggs all over the world. In 2018, per person consumption of broiler meat has been estimated to be 14 kg globally and 48 kg in USA (Thorp, 2021). In Pakistan, both the private and public sectors have increased their poultry investment from 1.28 billion $ in 2015 to 4.47 billion $ in 2018 (Aslam et al., 2020). Protein production from animal agriculture in a sustainable way is becoming crucial need because the global food demand is expected to increase by 35 to 56% between 2010 and 2050 (Van Dijk et al., 2021; Castro et al., 2023), but these days poultry industry is suffering from major challenges which can be overcome through direct-fed microbial (DFM) probiotics which might equip chickens with beneficial properties such as tolerance to heat stress, reduction in foodborne illnesses, competitive exclusion of pathogens, enhanced immunity, and prebiotics with positive physiological impact (Abd El-Hack et al., 2020; Ebeid et al., 2021).
Keeping in view the role of probiotics in overcoming the challenges of poultry industry, present project was initiated to isolate bacteria from broiler chicken GIT and their in vitro assessment for probiotic potential. Bacteria qualifying the in vitro assessment assays, as described in methodology of this study, might contribute to upgrade the economic status of Pakistan associated with poultry performance by providing hygienic and proper broilers diet in cost effective way. These might serve for the production of disease-resistant broilers with reduce cooking, pressing, and shear losses. They may also enhance bone mass, meat quality (amino acids, fat content, protein content, texture), and FCR (Pourakbari et al., 2016; Fekadie et al., 2022).
In addition to abovementioned benefits, the prebiotics derived from these probiotics also positively regulate the chicken metabolism in variety of ways, e.g., compound probiotics have been observed to contribute to growth performance of broilers exposed to heat stress, through increased rate of arginine, amino sugar, alanine, aspartate and glutamate, and beta alanine biosynthesis (Zhang et al., 2023). Metabolomics has also confirmed the formation of same metabolites in chicken intestine as formed in the presence of antibiotics, i.e., amino acids, sugars, organic acids, disaccharides, and trisaccharides, confirming their use as alternative to antibiotics (Li et al., 2023). Among the probiotics reported in the literature, Lactobacillus have been found to have strong involvement in the regulation of gut metabolites which in turn improves homoeostasis in chickens. In a study, chickens were fed on L. acidophilus supplemented diet which positively affected the amino acid and lipid metabolism along with increased production of immunoglobulin G (IgG) (Chen et al., 2023). Another study has reported enhanced concentration of acetate in broiler chickens fed with diet supplemented with L. salivarius. When this probiotic was combined with phytobiotics, it reduced extended-spectrum beta-lactamase (ESBL) producing E. coli (Ren et al., 2019). In a study, broilers fed with L. plantarum-derived metabolites considerably increased height of villi and growth (Gao et al., 2017). Dietary supplementation with L. plantarum along with fructooligosaccharide (FOS) showed increased levels of short chain fatty acids (SCFAs), IgA, and IgG and efficient growth performance in E. coli O78 challenged broilers (Ding et al., 2019). Another study has reported increased expression of genes involved in sulfur and nitrogen metabolism, flagella assembly and chemotaxis, and vitamin and cofactor synthesis, in lincomycin exposed broilers, supplemented with L. plantarum P-8 (Gao et al., 2017). Literature has also reported efficient role of L. plantarum-derived postbiotics in enhancing carcass yield, immunity, intestinal microbial composition, and growth performance of broilers exposed to heat stress (Humam et al., 2019).
In addition to above health benefits, probiotics through interfering the metabolic pathways of host chickens enable them to withstand heat and oxidative stresses. Probiotics, in intestinal epithelial cells, activate the heat shock proteins (HSPs), glutathione S-transferase (GST), and glutathione (GSH) and inactivates iNOS, COX-2, NF-KB, and toll-like receptor-4 (TLR-4) when there is high reactive oxygen species (ROS) accumulation. ROS are produced in intestine under oxidative stress. Activation of GST by probiotics results in production of GSH which serves as an antioxidant and removes ROS. Inhibition of iNOS by probiotics inhibits synthesis of nitric oxide synthase (NOS) resulting in decreased nitric oxide (NO) thus reducing ROS. Inhibition of COX-2, NF-KB, and TLR-4 reduces heat shock-induced inflammation in intestinal epithelium thus protecting the digestive system from permanent damage. To reduce the heat-induced epithelium disruption, probiotics accelerate the production of mucous and tight junction-adherent junction (TJ-AJ) pathway which seals intracellular space between neighboring cells (Abd El-Hack et al., 2020).
Keeping in view, these beneficial effects of probiotics, we attempted to isolate bacteria from chicken GIT. Four fast growing isolates were processed for detailed in vitro characterization. These isolates were identified to be L. antri, L. delbrueckii, L. casei, and L. salivarius, respectively. As all of these had different species and also showed variations during characterization, so were designated as different strains, i.e., PUPro1, PUPro2, PUPro3, and PUPro4, respectively. Our study is consistent with the previous one because most of the broilers have been reported to have Lactobacilli as gut flora (Boonkumklao et al., 2006). L. casei, L. johnsonii, L. acidophilus, L. crispatus, L. salivarius, and L. aviaries are the most reported Lactobacillus species in poultry (Sanders and Klaenhammer, 2001; Tharmaraj and Shah, 2003; Neville and O’Toole, 2010; Pieniz et al., 2014).
All isolates were found fast growing. Log phases comprising of 24–57 h, 3–51 h, 6–24 h, and 9–24 h were observed in L. antri strain PUPro1, L. delbrueckii strain PUPro2, L. casei strain PUPro3, and L. salivarius strain PUPro4, respectively. These findings are in line with previously reported chicken gut-associated bacteria which exhibited a rapid growth (Vinderola et al., 2002; Śliżewska and Chlebicz-Wójcik, 2020).
Literature describes various biochemical features of probiotics (Bansal et al., 2019; Samedi and Charles, 2019; Abid et al., 2022), but this is the first study to use the RapID™ NF Plus Panel for biochemical testing. The ability of a bacterium to use arginine as a carbon source can be tested biochemically by ADH test (Kwon et al., 2005). In present analysis, none of the isolates tested positive for ADH. All isolates were positive in EST and GLU test results. In the EST test, lipid break down releases fatty acids which drop the pH and change the color (MacFaddin, 2000). With the exception of L. antri strain PUPro1, all of the probiotic strains tested positive for PHS assay (Gilardi et al., 1975). None of the isolates showed positive results for GGT, NAG, α-GLU, β-GLU, PRO, BANA, IND, TRY, PYR, ONPG, and URE tests that detects the presence of glutamyl aminopeptidase, p-nitrophenyl-N-acetyl-β-D-glucosaminidase, ρ-nitrophenyl-D-glucosidase, ρ-nitrophenyl-β-D-glucosidase, proline aminopeptidase, N-benzyl-arginine aminopeptidase, potential of tryptophan to indole conversion, tryptophan aminopeptidase, pyrrolidonyl aminopeptidase, ρ-nitrophenyl-β-D-galactosidase, and urea hydrolysis potential, respectively (Westley et al., 1967; Mulczyk and Szewczuk, 1970; Gilardi et al., 1975; Kilian and Bülo, 1976; Nagatsu et al., 1976; Humble et al., 1977; Holt et al., 1984; Kwon et al., 2005). L. antri strain PUPro1 and L. salivarius strain PUPro4 tested positive for NO3 test that identifies nitrate reductase activity (Mulczyk and Szewczuk, 1970).
All the isolates were found to be resistant to NaCl at 0.2, 2, and 5% concentration. L. salivarius strain PUPro4 was the most resistant, with OD600 of 0.984, 0.929, and 0.922 for 0.2, 2, and 5% NaCl concentration, respectively. A previous study indicated that LAB from milk and milk products, meats, chicken dung, and other sources can grow in 1–10% NaCl treatments (Hoque et al., 2010; Shehata et al., 2016). Our findings are consistent with the literature and imply that present study bacteria can survive higher concentrations of NaCl and stress in vitro (Ye et al., 2020).
Probiotic strains must bind to host intestinal cells for better colonization (Collado et al., 2007). Probiotics’ antimicrobial and cholesterol-lowering benefits require strong colon epithelial cell attachment (Shokryazdan et al., 2014). In this study, all isolates adhered to chicken ileum epithelial cells with increased viability over 90 min L. casei strain PUPro3, with a maximum CFUs count of 134, showed the lowest adhesion ability. Our findings are consistent with those of literature that observed rumen LAB isolates’ adherence increased with incubation period (Setyawardani et al., 2014; Jose et al., 2015).
Stay of probiotics in different parts of chicken GIT varies as per the pH values of these parts. Generally, pH varies between 2 and 6.5, i.e., pH values for crop, proventriculus, gizzard, small intestine, and large intestine have been reported to be 4.8, 4.4, 2.6, 6.2, and 6.3, respectively (Svihus, 2014). Keeping in view these values, acidity tolerance of present study bacteria was estimated at three pH values, i.e., 2, 3, and 5. Maximum survival during interval of 3 h was observed in L. delbrueckii strain PUPro2 (pH = 2), in L. antri strain PUPro1, L. delbrueckii strain PUPro2 and L. casei strain PUPro3 (pH = 3), and in L. casei strain PUPro3 (pH = 6). No survival was observed in L. antri strain PUPro1 at pH = 6. These results were according to the previous research study reporting good survival of L. salivarius and L. fermentum at pH = 2.5 for 3 h (Hutari et al., 2011). Other studies have also reported survival of probiotics at the same pH (Reuben et al., 2019; Salehizadeh et al., 2020; Gupta et al., 2023). These findings confirmed the survival of present study bacteria in chicken gut during passage through stomach.
Hydrophobic bile salts play crucial roles in digestive system including fat emulsification and resistance against antimicrobials. So, when taken orally, probiotics must survive the severe environment of small intestine (Olejnik et al., 2005). In chicken gut, bile salt concentration varies, i.e., 0.0085% (caecum), 0.17% (duodenum), and 0.7% (jejunum). Typical 0.3% bile salt level has been considered in many probiotic bile salt tolerance studies (Shokryazdan et al., 2014; García-Hernández et al., 2016). The current study’s isolates survived in 0.3% bile salts and exhibited enhanced growth after 24 h. In this study, L. casei strain PUPro3 had the highest OD value of 0.973 in the presence of bile salts. Present finding is in accordance with previous literature reporting tolerance to 0.3% concentration of bile salts (Shin et al., 2008; Salehizadeh et al., 2020). Our findings also support prior research that revealed bile salts increased probiotic proliferation (Reuben et al., 2019).
The chicken industry loses a lot of revenue due to zoonotic and foodborne disease illnesses (Nallala et al., 2017). Potential probiotics need to have antimicrobial activity against such pathogens. All of the present study isolates were highly antagonistic to four distinct pathogens: Pseudomonas aeruginosa, Bacillus subtilis, Bacillus proteus, and Staphylococcus aureus. Antimicrobial activity against P. aeruginosa, B. subtilis, B. proteus, and S. aureus was highest for L. delbrueckii strain PUPro2, L. casei strain PUPro3, L. casei strain PUPro3, and L. salivarius strain PUPro4. This finding is consistent with previous study. Our results are also supported by the observation that chicken-derived probiotics have a broad-spectrum antagonistic impact against a wide range of infections (García-Hernández et al., 2016; Oyewole et al., 2018).
Antibiotic-resistant genes in probiotic could cause gut infections, so antibiotic profiling of isolated bacteria was crucial (Nallala et al., 2017). All isolates were ecologically benign and showed unique inhibitory zones against augmentin, azithromycin, amoxicillin, kanamycin, cefadroxil, and velosef. The obtained inhibitory zones had diameters between 17.33 mm and 39.33 mm. The literature reports antibiotic susceptibility in chicken probiotic cultures against antibiotics such as penicillin, ampicillin, chloramphenicol, ceftriaxone, and novobiocin supporting our findings (Gueimonde Fernández et al., 2013; Anandharaj and Sivasankari, 2014; Puniya et al., 2016; Dowarah et al., 2018). However, contrary to our findings, the literature also reports resistance against kanamycin, aminoglycosides and glycopeptides, streptomycin, gentamicin, and vancomycin in Lactobacilli (Jose et al., 2015; Zavišić et al., 2023).
According to FAO guidelines, probiotic microbial strains should not pose any host safety risks (Borras et al., 2021). Hemolytic activity destroys the protective epithelial layer, allowing infections to penetrate easily. Non-pathogenic strains must be non-hemolytic (De Vuyst et al., 2003). None of the isolates tested in this study produced the toxic chemical hemolysin, and all were non-hemolytic. Non-hemolytic LAB strains have been reported in the literature, which is in line with our findings (Chang et al., 2013; Oloyede and Afolabi, 2013; Oyewole et al., 2018).
Hypercholesterolemia or high blood cholesterol is a primary cause of deaths caused by heart diseases in poultry which can be decreased by lowering serum cholesterol (Shehata et al., 2016). Cholesterol lowering potential of isolates was measured using a cholesterol liquicolor kit. All of the isolated bacteria showed degradation potential toward cholesterol. The L. casei strain PUPro3 was the most effective at degrading cholesterol, reducing cholesterol levels from 216.12 to 192.2 mg/dL. L. antri strain PUP1 degraded cholesterol at a rate of just 208 mg/dL. These results are consistent with the literature (Miremadi et al., 2014; Shehata et al., 2016).
5 Conclusion
Probiotic strains separated from their natural host are more likely to establish themselves in the GIT and exert their therapeutic benefits. Present study is significant because the bacteria isolated might have potential as antibiotic substitutes and broiler productivity boosters. To further test and confirm their possible effect on broilers meat quality, they can be cultured for the estimation of CFU production followed by their immobilization in approximate quantity on any suitable substrate. This will enhance their shelf life. Afterward, these probiotics can be fed to broilers through feed supplementation. In addition to this, probiotic metabolomics and its integration with proteomics might also prove helpful in getting insight into their possible contributions to sustainable poultry production.
Data availability statement
The datasets presented in this study can be found in online repositories. The names of the repository/repositories and accession number(s) can be found in the article/Supplementary material.
Author contributions
FS: Data curation, Writing – original draft. FM: Conceptualization, Writing – original draft. AS: Formal analysis, Writing – original draft. SS: Writing – original draft. SA: Formal analysis, Writing – original draft. AQ: Writing – review & editing.
Funding
The authors declare that financial support was received for the research work and publication of this article from King Saud University, Riyadh, Saudi Arabia.
Acknowledgments
The authors would like to thank the Researchers Supporting Project number (RSP2024R35), King Saud University, Riyadh, Saudi Arabia.
Conflict of interest
The authors declare that the research was conducted in the absence of any commercial or financial relationships that could be construed as a potential conflict of interest.
Publisher’s note
All claims expressed in this article are solely those of the authors and do not necessarily represent those of their affiliated organizations, or those of the publisher, the editors and the reviewers. Any product that may be evaluated in this article, or claim that may be made by its manufacturer, is not guaranteed or endorsed by the publisher.
Supplementary material
The Supplementary material for this article can be found online at: https://www.frontiersin.org/articles/10.3389/fmicb.2024.1278439/full#supplementary-material
Footnotes
References
Abd El-Hack, M. E., El-Saadony, M. T., Shafi, M. E., Qattan, S. Y. A., Batiha, G. E., Khafaga, A. F., et al. (2020). Probiotics in poultry feed: a comprehensive review. J. Anim. Physiol. Anim. Nutr. 104, 1835–1850. doi: 10.1111/jpn.13454
Abid, S., Farid, A., Abid, R., Rehman, M. U., Alsanie, W. F., Alhomrani, M., et al. (2022). Identification, biochemical characterization, and safety attributes of locally isolated Lactobacillus fermentum from Bubalus bubalis (buffalo) milk as a probiotic. Microorganisms 10:954. doi: 10.3390/microorganisms10050954
Agus, A., Planchais, J., and Sokol, H. (2018). Gut microbiota regulation of tryptophan metabolism in health and disease. Cell Host Microbe 23, 716–724. doi: 10.1016/j.chom.2018.05.003
Akbar, M., Akbar, A., Yaqoob, H. S., Hussain, A., Svobodová, L., and Yasmin, F. (2023). Islamic finance education: current state and challenges for Pakistan. Cogent. Econ. Finance 11:2164665. doi: 10.1080/23322039.2022.2164665
Alam, W., Khan, S., Sultan, A., Ahmad, N., Tanweer, A. J., Abbas, G., et al. (2023). Effect of dietary supplementation of animal blood plasma powder on production performance, dressed weight and immune response of broiler. Pak. J. Sci. 75, 419–426. doi: 10.57041/pjs.v75i02.8
Anandharaj, M., and Sivasankari, B. (2014). Isolation of potential probiotic Lactobacillus oris HMI68 from mother's milk with cholesterol-reducing property. J. Biosci. Bioeng. 118, 153–159. doi: 10.1016/j.jbiosc.2014.01.015
Arsène, M. M., Davares, A. K., Andreevna, S. L., Vladimirovich, E. A., Carime, B. Z., Marouf, R., et al. (2021). The use of probiotics in animal feeding for safe production and as potential alternatives to antibiotics. Vet. World. 14:319. doi: 10.14202/vetworld.2021.319-328
Aslam, H. B., Alarcon, P., Yaqub, T., Iqbal, M., and Häsler, B. (2020). A value chain approach to characterize the chicken sub-sector in Pakistan. Front. Vet. Sci. 7:361. doi: 10.3389/fvets.2020.00361
Baéza, E., Guillier, L., and Petracci, M. (2022). Production factors affecting poultry carcass and meat quality attributes. Animal 16:100331. doi: 10.1016/j.animal.2021.100331
Bansal, P., Kumar, R., Singh, J., and Dhanda, S. (2019). Next generation sequencing, biochemical characterization, metabolic pathway analysis of novel probiotic Pediococcus acidilactici NCDC 252 and it’s evolutionary relationship with other lactic acid bacteria. Mol. Biol. Rep. 46, 5883–5895. doi: 10.1007/s11033-019-05022-z
Beckers, B., Op De Beeck, M., Thijs, S., Truyens, S., Weyens, N., Boerjan, W., et al. (2016). Performance of 16s rDNA primer pairs in the study of rhizosphere and endosphere bacterial microbiomes in metabarcoding studies. Front. Microbiol. 7:650. doi: 10.3389/fmicb.2016.00650
Boonkumklao, P., Kongthong, P., and Assavanig, A. (2006). Acid and bile tolerance of Lactobacillus thermotolerans, a novel species isolated from chicken feces. Agric. Nat. Resour. 40, 13–17.
Borras, L., Valiño, E., Elías, A., Martínez, J., Sanabria, A., and Becerra, M. (2021). Effect of fibrous materials inclusion on the solid-state fermentation of post harvested wastes of Solanum tuberosum. Cuba. J. Agric. Sci. 55, 31–42.
Castro, F. L. S., Chai, L., Arango, J., Owens, C. M., Smith, P. A., Reichelt, S., et al. (2023). Poultry industry paradigms: connecting the dots. J. Appl. Poult. Res. 32:100310. doi: 10.1016/j.japr.2022.100310
Chang, S. M., Tsai, C. L., Wee, W. C., and Yan, T. R. (2013). Isolation and functional study of potentially probiotic lactobacilli from Taiwan traditional paocai. Afr. J. Microbiol. Res. 7, 683–691. doi: 10.5897/AJMR12.2196
Chen, X., Chen, W., Ci, W., Zheng, Y., Han, X., Huang, J., et al. (2023). Effects of dietary supplementation with Lactobacillus acidophilus and Bacillus subtilis on mucosal immunity and intestinal barrier are associated with its modulation of gut metabolites and microbiota in late-phase laying hens. Probiot.Antimicrob. Proteins 15, 912–924. doi: 10.1007/s12602-022-09923-7
Cizeikiene, D., and Jagelaviciute, J. (2021). Investigation of antibacterial activity and probiotic properties of strains belonging to Lactobacillus and Bifidobacterium genera for their potential application in functional food and feed products. Probiot. Antimicrob. Proteins 13, 1387–1403. doi: 10.1007/s12602-021-09777-5
Collado, M. C., Meriluoto, J., and Salminen, S. (2007). In vitro analysis of probiotic strain combinations to inhibit pathogen adhesion to human intestinal mucus. Food Res. Int. 40, 629–636. doi: 10.1016/j.foodres.2006.11.007
De Vuyst, L., Moreno, M. F., and Revets, H. (2003). Screening for enterocins and detection of hemolysin and vancomycin resistance in enterococci of different origins. Int. J. Food Microbiol. 84, 299–318. doi: 10.1016/S0168-1605(02)00425-7
Desiere, S., Hung, Y., Verbeke, W., and D’Haese, M. (2018). Assessing current and future meat and fish consumption in sub-Sahara Africa: learnings from FAO food balance sheets and LSMS household survey data. Glob. Food Sec. 16, 116–126. doi: 10.1016/j.gfs.2017.12.004
Ding, S., Wang, Y., Yan, W., Li, A., Jiang, H., and Fang, J. (2019). Effects of Lactobacillus plantarum 15-1 and fructooligosaccharides on the response of broilers to pathogenic Escherichia coli O78 challenge. PLoS One 14:e0212079. doi: 10.1371/journal.pone.0223971
Dowarah, R., Verma, A. K., Agarwal, N., Singh, P., and Singh, B. R. (2018). Selection and characterization of probiotic lactic acid bacteria and its impact on growth, nutrient digestibility, health and antioxidant status in weaned piglets. PLoS One 13:e0192978. doi: 10.1371/journal.pone.0192978
Ebeid, T. A., Al-Homidan, I. H., and Fathi, M. M. (2021). Physiological and immunological benefits of probiotics and their impacts in poultry productivity. World Poultry Sci. J. 77, 883–899. doi: 10.1080/00439339.2021.1960239
Fadanka, S., Minette, S., and Mowoh, N. (2022). Preparation of Bacteria glycerol stocks. Available at: https://www.protocols.io/view/preparation-of-bacteria-glycerol-stocks-b85iry4e.
Fekadie, A., Abera, A., and Yitbarek, C. (2022). Effective microbes (EM) supplementation effect on feed intake digestibility and live weight changes of Washera sheep fed wheat straw. Iran. J. Appl. Anim. Sci. 4, 713–722.
Gao, P., Hou, Q., Kwok, L. Y., Huo, D., Feng, S., and Zhang, H. (2017). Effect of feeding Lactobacillus plantarum P-8 on the faecal microbiota of broiler chickens exposed to lincomycin. Sci. Bull. 62, 105–113. doi: 10.1016/j.scib.2017.01.001
García-Hernández, Y., Pérez-Sánchez, T., Boucourt, R., Balcázar, J. L., Nicoli, J. R., Moreira-Silva, J., et al. (2016). Isolation, characterization and evaluation of probiotic lactic acid bacteria for potential use in animal production. Res. Vet. Sci. 108, 125–132. doi: 10.1016/j.rvsc.2016.08.009
Gilardi, G., Hirschl, S., and Mandel, M. (1975). Characteristics of yellow-pigmented nonfermentative bacilli (groups Ve-1 and Ve-2) encountered in clinical bacteriology. J. Clin. Microbiol. 1, 384–389. doi: 10.1128/jcm.1.4.384-389.1975
Green, M. R., and Sambrook, J. (2019). Analysis of DNA by agarose gel electrophoresis. Cold Spring Harbor Protoc 2019:pdb.top100388. doi: 10.1101/pdb.top100388
Gueimonde Fernández, M., Sánchez García, B., De Los, G., Reyes-Gavilán, C., and Margolles Barros, A. (2013). Antibiotic resistance in probiotic bacteria. Front. Microbiol. :202:4. doi: 10.3389/fmicb.2013.00202
Gupta, N. (2019). DNA extraction and polymerase chain reaction. J. Cytol. 36, 116–117. doi: 10.4103/JOC.JOC_110_18
Gupta, M., Raut, R., Manandhar, S., Chaudhary, A., Shrestha, U., Dangol, S., et al. (2023). Identification and characterization of probiotics isolated from indigenous chicken (Gallus domesticus) of Nepal. PLoS One 18:e0280412. doi: 10.1371/journal.pone.0280412
He, Y., Li, J., Wang, F., Na, W., and Tan, Z. (2023). Dynamic changes in the gut microbiota and metabolites during the growth of Hainan Wenchang chickens. Animals 13:348. doi: 10.3390/ani13030348
Henchion, M., Moloney, A. P., Hyland, J., Zimmermann, J., and Mccarthy, S. (2021). Trends for meat, milk and egg consumption for the next decades and the role played by livestock systems in the global production of proteins. Animal 15:100287. doi: 10.1016/j.animal.2021.100287
Holt, J. G., Krieg, N., Sneath, P., Staley, J., and Williams, S. (1984). Bergey's manual of systematic bacteriology. The Williams and Wilkins Co., Baltimore.
Hoque, M., Akter, F., Hossain, K., Rahman, M., Billah, M., and Islam, K. (2010). Isolation, identification and analysis of probiotic properties of Lactobacillus spp. from selective regional yoghurts. World J. Dairy Food Sci. 5, 39–46.
Humam, A. M., Loh, T. C., Foo, H. L., Samsudin, A. A., Mustapha, N. M., Zulkifli, I., et al. (2019). Effects of feeding different postbiotics produced by Lactobacillus plantarum on growth performance, carcass yield, intestinal morphology, gut microbiota composition, immune status, and growth gene expression in broilers under heat stress. Animals 9:644. doi: 10.3390/ani9090644
Humble, M., King, A., and Phillips, I. (1977). API ZYM: a simple rapid system for the detection of bacterial enzymes. J. Clin. Pathol. 30, 275–277. doi: 10.1136/jcp.30.3.275
Hutari, A., Jaseem, W. S., Hamid, A. A., and Yusoff, W. M. W. (2011). Screening of Lactobacillus strains against Salmonella both isolated from Malaysian free-range chicken intestine for use as probiotic. Sains Malays 40, 1115–1122.
Ivanova, N. N., and Denisenko, L. I. (2019). “The role of probiotics in the poultry industry” in Urgent issues of agricultural science, production and education, 209–212. Available at: https://www.elibrary.ru/item.asp?id=37404056
Jomehzadeh, N., Javaherizadeh, H., Amin, M., Saki, M., Al-Ouqaili, M. T., Hamidi, H., et al. (2020). Isolation and identification of potential probiotic Lactobacillus species from feces of infants in Southwest Iran. Int. J. Infect. Dis. 96, 524–530. doi: 10.1016/j.ijid.2020.05.034
Jose, N. M., Bunt, C. R., and Hussain, M. A. (2015). Comparison of microbiological and probiotic characteristics of lactobacilli isolates from dairy food products and animal rumen contents. Microorganisms 3, 198–212. doi: 10.3390/microorganisms3020198
Khan, S., Moore, R. J., Stanley, D., and Chousalkar, K. K. (2020). The gut microbiota of laying hens and its manipulation with prebiotics and probiotics to enhance gut health and food safety. Appl. Environ. Microbiol. 86, e00600–e00620. doi: 10.1128/AEM.00600-20
Kilian, M., and Bülo, P. (1976). Rapid diagnosis of Enterobacteriaceae: I. Detection of bacterial glycosidases. Acta Pathol. Microbiol. Scand. Sect. B Microbiol. 84, 245–251.
Kumar, A., and Kumar, D. (2015). Characterization of Lactobacillus isolated from dairy samples for probiotic properties. Anaerobe 33, 117–123. doi: 10.1016/j.anaerobe.2015.03.004
Kumari Vb, C., Huligere, S. S., Alotaibi, G., Al Mouslem, A. K., Bahauddin, A. A., Shivanandappa, T. B., et al. (2023). Antidiabetic activity of potential probiotics limosilactobacillus spp., levilactobacillus spp., and lacticaseibacillus spp. isolated from fermented sugarcane juice: a comprehensive in vitro and in silico study. Nutrients 15:1882. doi: 10.3390/nu15081882
Kwon, K. K., Lee, H.-S., Yang, S. H., and Kim, S. J. (2005). Kordiimonas gwangyangensis gen. Nov., sp. nov., a marine bacterium isolated from marine sediments that forms a distinct phyletic lineage (Kordiimonadales Ord. Nov.) in the ‘Alphaproteobacteria’. Int. J. Syst. Evol. Microbiol. 55, 2033–2037. doi: 10.1099/ijs.0.63684-0
Li, Y., Zhang, J. L., Deng, J. J., Chen, Z., Liu, S., Liu, J., et al. (2023). Integrative analysis of the microbiome and metabolome of broiler intestine: insights into the mechanisms of probiotic action as an antibiotic substitute. doi: 10.21203/rs.3.rs-3456870/v1
Liu, J., Wang, J., Zhou, Y., Han, H., Liu, W., Li, D., et al. (2022). Integrated omics analysis reveals differences in gut microbiota and gut-host metabolite profiles between obese and lean chickens. Poultry Sci. 101:102165. doi: 10.1016/j.psj.2022.102165
Liu, Q., Yu, Z., Tian, F., Zhao, J., Zhang, H., Zhai, Q., et al. (2020). Surface components and metabolites of probiotics for regulation of intestinal epithelial barrier. Microb. Cell Factories 19, 1–11. doi: 10.1186/s12934-020-1289-4
Lorenz, T. C. (2012). Polymerase chain reaction: basic protocol plus troubleshooting and optimization strategies. J. Vis. Exp. :63:e3998. doi: 10.3791/3998
Lutful, K. S. M. (2009). The role of probiotics in the poultry industry. Int. J. Mol. Sci. 10, 3531–3546. doi: 10.3390/ijms10083531
MacFaddin, J. (2000). Biochemical tests for identification of medical bacteria. Lippincott Williams & Wilkins Philadelphia, PA.
Maloney, S., Engler, C., and Norton, R. (2014). Evaluation of the Remel RapID NF plus rapid biochemical method for identification of Burkholderia pseudomallei. J. Clin. Microbiol. 52, 2175–2176. doi: 10.1128/JCM.00025-14
Mehmood, A., Nawaz, M., Rabbani, M., and Mushtaq, M. H. (2023). In vitro characterization of probiotic potential of Limosilactobacillus fermentum against Salmonella gallinarum causing fowl typhoid. Animals 13:1284. doi: 10.3390/ani13081284
Melese, K., Alemu, T., and Desalegn, A. (2023). Isolation, characterization and in-vitro evaluation of potential probiotic lactic acid bacteria isolated from chicken gut. [Preprint]. doi: 10.21203/rs.3.rs-2697471/v1
Miremadi, F., Ayyash, M., Sherkat, F., and Sojanovska, L. (2014). Cholesterol reduction mechanisms and fatty acid composition of cellular membranes of probiotic lactobacilli and Bifidobacteria. J. Funct. Foods 9, 295–305. doi: 10.1016/j.jff.2014.05.002
Mohammed, A., Zaki, R., Negm, E., Mahmoud, M., and Cheng, H. (2021). Effects of dietary supplementation of a probiotic (Bacillus subtilis) on bone mass and meat quality of broiler chickens. Poult. Sci. 100:100906. doi: 10.1016/j.psj.2020.11.073
Mulczyk, M., and Szewczuk, A. (1970). Pyrrolidonyl peptidase in bacteria: a new colrimetric test for differentiation of enterobacteriaceae. J. Gen. Microbiol. 61, 9–13.
Musikasang, H., Tani, A., Kittikun, A.-H., and Maneerat, S. (2009). Probiotic potential of lactic acid bacteria isolated from chicken gastrointestinal digestive tract. World J. Microbiol. Biotechnol. 25, 1337–1345. doi: 10.1007/s11274-009-0020-8
Nagatsu, T., Hino, M., Fuyamada, H., Hayakawa, T., Sakakibara, S., Nakagawa, Y., et al. (1976). New chromogenic substrates for X-prolyl dipeptidyl-aminopeptidase. Anal. Biochem. 74, 466–476. doi: 10.1016/0003-2697(76)90227-X
Nallala, V., Sadishkumar, V., and Jeevaratnam, K. (2017). Molecular characterization of antimicrobial Lactobacillus isolates and evaluation of their probiotic characteristics in vitro for use in poultry. Food Biotechnol. 31, 20–41. doi: 10.1080/08905436.2016.1269289
Nawaz, A. S. N., Jagadeesh, K. S., and Krishnaraj, P. U. (2017). Isolation and screening of lactic acid bacteria for acidic pH and bile tolerance. Int. J. Curr. Microbiol. Appl. Sci. 6, 3975–3980. doi: 10.20546/ijcmas.2017.607.411
Neville, B., and O’Toole, P. (2010). Probiotic properties of Lactobacillus salivarius and closely related Lactobacillus species. Future Microbiol. 5, 759–774. doi: 10.2217/fmb.10.35
Ogbuewu, I. P., Mabelebele, M., Sebola, N. A., and Mbajiorgu, C. (2022). Bacillus probiotics as alternatives to in-feed antibiotics and its influence on growth, serum chemistry, antioxidant status, intestinal histomorphology, and lesion scores in disease-challenged broiler chickens. Front. Vet. Sci. 9:876725. doi: 10.3389/fvets.2022.876725
Olejnik, A., Lewandowska, M., Obarska, M., and Grajek, W. (2005). Tolerance of Lactobacillus and Bifidobacterium strains to low pH, bile salts and digestive enzymes. Food Sci. Technol. 8:5.
Oloyede, A., and Afolabi, O. (2013). Phenotypic characterization and in vitro screening of lactic acid bacteria from goat milk for probiotic USE. J. Agric. Sci. Environ. 13, 50–61. doi: 10.51406/jagse.v13i1.1210
Oyewole, O. F., Maria, C. O., Tope, P. S., and FunmI, O. O. (2018). In vitro study of potential probiotic lactic acid bacteria isolated from the gut of chickens in Abeokuta, Nigeria. Alex. J. Vet. Sci 58. doi: 10.5455/ajvs.290499
Pieniz, S., Andreazza, R., Anghinoni, T., Camargo, F., and Brandelli, A. (2014). Probiotic potential, antimicrobial and antioxidant activities of Enterococcus durans strain LAB18s. Food Control 37, 251–256. doi: 10.1016/j.foodcont.2013.09.055
Pourakbari, M., Seidavi, A., Asadpour, L., and Martínez, A. (2016). Probiotic level effects on growth performance, carcass traits, blood parameters, cecal microbiota, and immune response of broilers. An. Acad. Bras. Ciênc. 88, 1011–1021. doi: 10.1590/0001-3765201620150071
Puniya, M., Ravinder Kumar, M., Panwar, H., Kumar, N., and Ramneek, A. K. P. (2016). Screening of lactic acid bacteria of different origin for their probiotic potential. J. Food Process. Technol. 7:545.
Reid, G., Gadir, A. A., and Dhir, R. (2019). Probiotics: reiterating what they are and what they are not. Front. Microbiol. 10:424. doi: 10.3389/fmicb.2019.00424
Ren, H., Vahjen, W., Dadi, T., Saliu, E. M., Boroojeni, F. G., and Zentek, J. (2019). Synergistic effects of probiotics and phytobiotics on the intestinal microbiota in young broiler chicken. Microorganisms 7:684. doi: 10.3390/microorganisms7120684
Reuben, R. C., Roy, P. C., Sarkar, S. L., Alam, R.-U., and Jahid, I. K. (2019). Isolation, characterization, and assessment of lactic acid bacteria toward their selection as poultry probiotics. BMC Microbiol. 19, 1–20. doi: 10.1186/s12866-019-1626-0
Rizzoli, R., and Biver, E. (2020). Are probiotics the new calcium and vitamin D for bone health? Curr. Osteoporos. Rep. 18, 273–284. doi: 10.1007/s11914-020-00591-6
Rocha-Ramírez, L. M., Hernández-Chiñas, U., Moreno-Guerrero, S. S., Ramírez-Pacheco, A., and Eslava, C. A. (2021). Probiotic properties and immunomodulatory activity of Lactobacillus strains isolated from dairy products. Microorganisms 9:825. doi: 10.3390/microorganisms9040825
Salehizadeh, M., Modarressi, M. H., Mousavi, S. N., and Ebrahimi, M. T. (2020). Evaluation of lactic acid bacteria isolated from poultry feces as potential probiotic and its in vitro competitive activity against Salmonella typhimurium. Vet. Res. Forum 11, 67–75. doi: 10.30466/vrf.2018.84395.2110
Saman, A., Chaudhry, M., Ijaz, M., Shaukat, W., Zaheer, M. U., Mateus, A., et al. (2023). Assessment of knowledge, perception, practices and drivers of antimicrobial resistance and antimicrobial usage among veterinarians in Pakistan. Prev. Vet. Med. 212:105836. doi: 10.1016/j.prevetmed.2022.105836
Samedi, L., and Charles, A. L. (2019). Isolation and characterization of potential probiotic lactobacilli from leaves of food plants for possible additives in pellet feeding. Ann. Agric. Sci. 64, 55–62. doi: 10.1016/j.aoas.2019.05.004
Sanders, M., and Klaenhammer, T. (2001). Invited review: the scientific basis of Lactobacillus acidophilus NCFM functionality as a probiotic. J. Dairy Sci. 84, 319–331. doi: 10.3168/jds.S0022-0302(01)74481-5
Sanders, M. E., Merenstein, D. J., Reid, G., Gibson, G. R., and Rastall, R. A. (2019). Probiotics and prebiotics in intestinal health and disease: from biology to the clinic. Nat. Rev. Gastroenterol. Hepatol. 16, 605–616. doi: 10.1038/s41575-019-0173-3
Setyawardani, T., Rahayu, W., Maheswari, R., and Palupi, N. (2014). Antimicrobial activity and adhesion ability of indigenous lactic acid bacteria isolated from goat milk. Int. J. Food Res. 21.
Shamsudin, W. N. F., San Loo, S., Ho, Y. W., Abdullah, N., Saad, W. Z., and Wan, K. L. (2019). Probiotic properties of Lactobacillus isolates from chicken intestines. J. Biochem. Microbiol. Biotechnol. 7, 8–13. doi: 10.54987/jobimb.v7i2.476
Shehata, M., El-Sohaimy, S., El-Sahn, M. A., and Youssef, M. (2016). Screening of isolated potential probiotic lactic acid bacteria for cholesterol lowering property and bile salt hydrolase activity. Anna. Agric. Sci. 61, 65–75. doi: 10.1016/j.aoas.2016.03.001
Shin, M., Han, S., Ji, A., Kim, K., and Lee, W. (2008). Isolation and characterization of bacteriocin-producing bacteria from the gastrointestinal tract of broiler chickens for probiotic use. J. Appl. Microbiol. 105, 2203–2212. doi: 10.1111/j.1365-2672.2008.03935.x
Shokryazdan, P., Kalavathy, R., Sieo, C., Alitheen, N., Liang, J., Jahromi, M., et al. (2014). Isolation and characterization of Lactobacillus strains as potential probiotics for chickens. Pertanika J. Trop. Agric. Sci 37, 141–157. doi: 10.1155/2014/927268
Sievers, F., and Higgins, D. G. (2014). Clustal omega, accurate alignment of very large numbers of sequences. Methods Mol. Biol. 1079, 105–116. doi: 10.1007/978-1-62703-646-7_6
Śliżewska, K., and Chlebicz-Wójcik, A. (2020). Growth kinetics of probiotic Lactobacillus strains in the alternative, cost-efficient semi-solid fermentation medium. Biology 9:423. doi: 10.3390/biology9120423
Soemarie, Y. B., Milanda, T., and Barliana, M. I. (2022). Isolation, characterization, and identification candidate of probiotic bacteria isolated from Wadi Papuyu (Anabas testudineus Bloch.) a fermented fish product from Central Kalimantan, Indonesia. Int. J. Food Sci. 2022:4241531. doi: 10.1155/2022/4241531
Sorescu, I., Dumitru, M., and Ciurescu, G. (2021). Lactobacillus spp. strains isolation, identification, preservation and quantitative determinations from gut content of 45-day-old chickens broilers. Braz. J. Poultry Sci. 23, 1–7. doi: 10.1590/1806-9061-2020-1378
Svihus, B. (2014). Function of the digestive system. J. Appl. Poult. Res. 23, 306–314. doi: 10.3382/japr.2014-00937
Tang, X., Liu, X., and Liu, H. (2021). Effects of dietary probiotic (Bacillus subtilis) supplementation on carcass traits, meat quality, amino acid, and fatty acid profile of broiler chickens. Front. Vet. Sci. 8:767802. doi: 10.3389/fvets.2021.767802
Tharmaraj, N., and Shah, N. (2003). Selective enumeration of Lactobacillus delbrueckii ssp. bulgaricus, Streptococcus thermophilus, Lactobacillus acidophilus, Bifidobacteria, Lactobacillus casei, Lactobacillus rhamnosus, and Propionibacteria. J. Dairy Sci. 86, 2288–2296. doi: 10.3168/jds.S0022-0302(03)73821-1
Thorp, B. (2021). The poultry industry: poultry health: a guide for professionals. CABI, Wallingford.
Van Dijk, M., Morley, T., Rau, M. L., and Saghai, Y. (2021). A meta-analysis of projected global food demand and population at risk of hunger for the period 2010–2050. Nature Food 2, 494–501. doi: 10.1038/s43016-021-00322-9
Vinderola, C. G., Mocchiutti, P., and Reinheimer, J. A. (2002). Interactions among lactic acid starter and probiotic bacteria used for fermented dairy products. J. Dairy Sci. 85, 721–729. doi: 10.3168/jds.S0022-0302(02)74129-5
Westley, J., Anderson, P., Close, V., Halpern, B., and Lederberg, E. (1967). Aminopeptidase profiles of various bacteria. Appl. Microbiol. 15, 822–825. doi: 10.1128/am.15.4.822-825.1967
Wu, Y., Zhang, H., Zhang, R., Cao, G., Li, Q., Zhang, B., et al. (2021). Serum metabolome and gut microbiome alterations in broiler chickens supplemented with lauric acid. Poultry Sci. 100:101315. doi: 10.1016/j.psj.2021.101315
Wu, Y., Zhen, W., Geng, Y., Wang, Z., and Guo, Y. (2019). Effects of dietary Enterococcus faecium NCIMB 11181 supplementation on growth performance and cellular and humoral immune responses in broiler chickens. Poult. Sci. 98, 150–163. doi: 10.3382/ps/pey368
Xu, Y., Zhou, T., Tang, H., Li, X., Chen, Y., Zhang, L., et al. (2020). Probiotic potential and amylolytic properties of lactic acid bacteria isolated from Chinese fermented cereal foods. Food Control 111:107057. doi: 10.1016/j.foodcont.2019.107057
Yadav, M., Dubey, M., Yadav, M., and Shankar, K. S. (2018). Effect of supplementation of probiotic (Bacillus subtilis) on growth performance and carcass traits of broiler chickens. Int. J. Curr. Microbiol. Appl. Sci. 7, 3440–4849. doi: 10.20546/ijcmas.2018.708.510
Yan, L., Lv, Z., An, S., Xing, K., Wang, Z., Lv, M., et al. (2021). Effects of rearing system and narasin on growth performance, gastrointestinal development, and gut microbiota of broilers. Poult. Sci. 100:100840. doi: 10.1016/j.psj.2020.10.073
Yang, S., Yang, Y., Long, X., Zhang, F., and Wang, Z. (2023). Integrated analysis of the effects of cecal microbiota and serum metabolome on market weights of Chinese native chickens. Animals 13:3034. doi: 10.3390/ani13193034
Ye, K., Li, P., and Gu, Q. (2020). Complete genome sequence analysis of a strain Lactobacillus pentosus ZFM94 and its probiotic characteristics. Genomics 112, 3142–3149. doi: 10.1016/j.ygeno.2020.05.015
Young, J. (2018). Sanger sequencing–a hands-on simulation. Genet. Soc. Am. Peer Rev. Educ. Port. 2018:3. doi: 10.1534/gsaprep.2018.003
Zavišić, G., Popović, M., Stojkov, S., Medić, D., Gusman, V., Jovanović Lješković, N., et al. (2023). Antibiotic resistance and probiotics: knowledge gaps, market overview and preliminary screening. Antibiotics 12:1281. doi: 10.3390/antibiotics12081281
Zhang, T., Ding, H., Chen, L., Lin, Y., Gong, Y., Pan, Z., et al. (2021). Antibiotic-induced dysbiosis of microbiota promotes chicken lipogenesis by altering metabolomics in the cecum. Meta 11:487. doi: 10.3390/metabo11080487
Zhang, M., Liu, L., Chen, D., Zhang, X., Zhou, C., Gan, Q., et al. (2020). Functional microRNA screening for dietary vitamin E regulation of abdominal fat deposition in broilers. Br. Poult. Sci. 61, 344–349. doi: 10.1080/00071668.2020.1736265
Keywords: probiotics, bile salts, gut, chicken, poultry, hemolysis, adhesion
Citation: Shahbaz F, Muccee F, Shahab A, Safi SZ, Alomar SY and Qadeer A (2024) Isolation and in vitro assessment of chicken gut microbes for probiotic potential. Front. Microbiol. 15:1278439. doi: 10.3389/fmicb.2024.1278439
Edited by:
Ajay Kumar, Amity University, IndiaReviewed by:
Alireza Seidavi, Islamic Azad University, Rasht Branch, IranAtte Johannes Von Wright, University of Eastern Finland, Finland
Copyright © 2024 Shahbaz, Muccee, Shahab, Safi, Alomar and Qadeer. This is an open-access article distributed under the terms of the Creative Commons Attribution License (CC BY). The use, distribution or reproduction in other forums is permitted, provided the original author(s) and the copyright owner(s) are credited and that the original publication in this journal is cited, in accordance with accepted academic practice. No use, distribution or reproduction is permitted which does not comply with these terms.
*Correspondence: Fatima Muccee, fatima.sbb@pu.edu.pk