- 1Department of Precision Medicine, University of Campania “Luigi Vanvitelli”, Naples, Italy
- 2Department of Science, Università degli Studi della Basilicata, Potenza, Italy
- 3Spinoff TNcKILLERS, Potenza, Italy
- 4School of Agricultural, Forestry, Food and Environmental Sciences (SAFE), Università degli Studi della Basilicata, Potenza, Italy
- 5Laboratory of Molecular and Precision Oncology, Biogem Scarl, Institute of Genetic Research, Ariano Irpino, Italy
Introduction: Many lactic acid bacteria (LAB) strains are currently gaining attention in the food industry and various biological applications because of their harmless and functional properties. Given the growing consumer demand for safe food, further research into potential probiotic bacteria is beneficial. Therefore, we aimed to characterize Pediococcus pentosaceus DSPZPP1, a LAB strain isolated from traditional fermented sausages from the Basilicata region of Southern Italy.
Methods: In this study, we analyzed the whole genome of the P. pentosaceus DSPZPP1 strain and performed in silico characterization to evaluate its applicability for probiotics and use in the food industry.
Results and Discussion: The whole-genome assembly and functional annotations revealed many interesting characteristics of the DSPZPP1 strain. Sequencing raw reads were assembled into a draft genome of size 1,891,398 bp, with a G + C content of 37.3%. Functional annotation identified 1930 protein-encoding genes and 58 RNAs including tRNA, tmRNA, and 16S, 23S, and 5S rRNAs. The analysis shows the presence of genes that encode water-soluble B-group vitamins such as biotin, folate, coenzyme A, and riboflavin. Furthermore, the analysis revealed that the DSPZPP1 strain can synthesize class II bacteriocin, penocin A, adding importance to the food industry for bio-enriched food. The DSPZPP1 genome does not show the presence of plasmids, and no genes associated with antimicrobial resistance and virulence were found. In addition, two intact bacteriophages were identified. Importantly, the lowest probability value in pathogenicity analysis indicates that this strain is non-pathogenic to humans. 16 s rRNA-based phylogenetic analysis and comparative analysis based on ANI and Tetra reveal that the DSPZPP1 strain shares the closest evolutionary relationship with P. pentosaceus DSM 20336 and other Pediococcus strains. Analysis of carbohydrate active enzymes (CAZymes) identified glycosyl transferases (GT) as a main class of enzymes followed by glycoside hydrolases (GH). Our study shows several interesting characteristics of the isolated DSPZPP1 strain from fermented Italian sausages, suggesting its potential use as a promising probiotic candidate and making it more appropriate for selection as a future additive in biopreservation.
1 Introduction
LAB include a group of Gram-positive rods and cocci, non-sporing, and catalase-negative microorganisms that are of great importance in fermented food industries for their biochemical, physiological, and genetic features (Shukla and Goyal, 2014). LAB are widely present in foods and used as biopreservatives as well as aroma, texture, and flavor enhancers. Various studies have demonstrated the close connection between the LAB properties and their ability to produce, through fermentation, a wide range of sugars and metabolites, such as lactic and acetic acid, ethanol, acetone, diacetyl, exopolysaccharides, specific proteases, and protein antimicrobials, called bacteriocins (Papagianni, 2012; Cotter et al., 2013; Gudiña et al., 2015; Barbosa et al., 2017; Zommiti et al., 2018). Screening for beneficial bacteria from complex microbial communities is becoming more common. Of the many known microorganisms, few have been tested to determine their characteristics and applications. Among these, microorganisms called probiotics are receiving much interest due to their beneficial effects on plants, animals, humans, and foods, and there is an increasing effort to identify other new bacteria that may be both functional and harmless.
Currently, only a limited number of probiotics are used for the daily maintenance of intestinal microbial balance and in clinical treatment. Among them, some LABs have proved worthy of exploration in human health promotion (Jiang et al., 2021).
Although several species of Lactobacillus, Bifidobacterium, and Clostridium possess potent probiotic characteristics, these strains are not sufficient for the various needs of humans and industry (Min et al., 2017). Therefore, the identification of additional bacterial species is required to enrich the application spectrum of probiotics and their applications in food and agriculture (Jiang et al., 2021). Several studies have tested and used bacterial species belonging to the genus Pediococcus that have highlighted the importance of Pediococcus in the field of probiotics, indicating that new strains, isolated from different food matrices and belonging to this genus, can play a key role in the development of new functional foods (Martino et al., 2013; Vidhyasagar and Jeevaratnam, 2013; Savedboworn et al., 2014; Dubey et al., 2015; Chen et al., 2017; Zommiti et al., 2018; Todhanakasem et al., 2020; Hu et al., 2021). Numerous studies have reported that strains of Pediococcus (P.) pentosaceus are frequently isolated from various food sources and biotopes encompassing plant materials, bacterial ripened cheese, beverages, pickles, wine, dairy, and meat products, with a potent role as starter cultures involved in the manufacturing of fermented foods (Halami et al., 2005; Midha et al., 2012; García-Ruiz et al., 2014; Lv et al., 2014; Carafa et al., 2015; Ilavenil et al., 2016; Zommiti et al., 2018).
Pediococcus pentosaceus, belonging to the family Lactobacillaceae, genus Pediococcus, is a species of cocci-shaped LAB in pairs or quadruplets and is a non-motile facultative anaerobic Gram-positive bacterium (Chen et al., 2020). Previous studies have shown that P. pentosaceus occurs naturally in fermented products and may have an active function in product quality, food safety, and production efficiency (Balakrishnan and Agrawal, 2014; Jang et al., 2015; Gong and Qi, 2020; Montemurro et al., 2020; Jiang et al., 2021; Xu et al., 2021). Furthermore, different research has reported that P. pentosaceus has probiotic functions including anti-inflammatory, anti-cancer, antioxidant, detoxifying, and cholesterol-lowering activities (Zhao et al., 2012; Thirabunyanon and Hongwittayakorn, 2013; Sellamani et al., 2016; Asami et al., 2017; Kim et al., 2019). However, the use of LAB does not support the different needs in food processing, so the demand for more types of probiotic strains is growing and P. pentosaceus represents a good candidate due to its application value and probiotic effect attracting great interest by the food industry (Qi et al., 2021). Researchers from various countries have studied the probiotic potential of P. pentosaceus in local foods to determine the best use of P. pentosaceus in the food industry, maintaining its probiotic effect in the human body (Shukla and Goyal, 2014; Sellamani et al., 2016; Nanasombat et al., 2017; Ghosh et al., 2019).
For this reason, in many studies (Cocolin et al., 2004; Ammor et al., 2005; Comi et al., 2005; Aymerich et al., 2006; Baruzzi et al., 2006; Bonomo et al., 2008), the microbial diversity identification and characterization were assessed. In Italy, different typical meat products, such as the traditional fermented sausages of the Basilicata region, were analyzed to identify suitable starter cultures for use in the production process and necessary to guarantee food safety and to standardize product properties (Lebert et al., 2007). It has been observed that artisanal products have greater quality than sausages from controlled fermentations; commercial starter cultures are often not able to compete well with the house flora so their use often results in the loss of desirable sensory properties (Bonomo et al., 2008, 2013). Therefore, the tendency has been to select appropriate cultures from indigenous microorganisms that are more competitive and well adapted to the particular product and the specific production technology, and with high metabolic capacities, which can beneficially affect product quality and safety, preserving their typicity (Ammor et al., 2005; Drosinos et al., 2005; Aymerich et al., 2006; Leroy et al., 2006; Bonomo et al., 2008).
In a previous study, Parente et al. (2001) evaluated the phenotypic diversity of lactic acid bacteria (LAB) populations in traditional fermented sausages produced in artisanal and industrial plants in Basilicata (Southern Italy). In total, 414 LAB cultures were isolated from samples of sausages at different stages of ripening and a set of 28 tests was used to classify and identify the isolates; 50% of the isolates were identified as Lactobacillus (L.) sakei, 22% as Pediococcus pentosaceus, and 7% as Leuconostoc spp. and other Lactobacillus spp., were present in lower percentages.
Among these tested isolates, there is also our strain (P. pentosaceus DSPZPP1) belonging to a cluster that proved interesting properties. So, further analyses were conducted on some strains selected among these isolates investigated. In the previous study by Bonomo et al. (2008), 49 lactic acid bacteria (LAB) isolated from traditional fermented sausages of the Basilicata region were molecularly investigated for taxonomic identification at species and strain levels and technologically characterized on the basis of salt tolerance, acid production ability, growth at different temperatures, proteolytic, antimicrobial, and nitrate reductase activities. Lactobacillus sakei was the predominant species (67%) followed by Pediococcus pentosaceus (16%), Leuconostoc carnosum (8%), Lb. plantarum (4%), Lb. brevis (2%), and Leuc. pseudomesenteroides (2%). The technological characterization was conducted to highlight the primary purpose of LAB starter cultures used in meat fermentation, which is to enhance product safety and extend shelf life. LAB achieve this by reducing pH, growing, and being competitive in the conditions of sausage manufacturing, as well as by producing antimicrobial compounds. Moreover, a no-hierarchical cluster analysis by k-means procedure that classified strains in eight clusters on the basis of their technological profile was carried out. One of the three clusters that included the strains with the most interesting technological characteristics was the number 7 which included our strain P. pentosaceus DSPZPP1 (already labeled DBPZ0346). These strains presented a good growth, even in the presence of salt and nitrite. They also demonstrated a strong acidifying ability, moderate proteolytic and nitrate reductase activities, and displayed an antimicrobial activity against Staphylococcus epidermidis DSM1798 and Kocuria varians LMG14231 strains (Bonomo et al., 2008). Therefore, based on the information on the abilities of this bacterial strain, we decided to deepen the study of the P. pentosaceus DSPZPP1 strain to better understand in a more precise and in-depth way the characteristics of the strain, which can then be used for various applications. This study arises from the need to use different and customized screening methods for P. pentosaceus based on individual characteristics and different applications of the product (Jiang et al., 2020) and from the limitation related to the source and species of P. pentosaceus, which pushes us to select strains with probiotic activities from different sources. Although numerous studies have found that P. pentosaceus has very important uses, systematic and relevant studies on the genetic research and application of P. pentosaceus are still lacking (Qi et al., 2021).
In this study, the whole-genome assembly and functional annotations of P. pentosaceus DSPZPP1 strain were performed to gain a thorough understanding of the functions and related mechanisms of the strain and to reveal interesting characteristics of the strain, suggesting its potential use as a promising probiotic candidate and making it more appropriate for selection as a future additive in biopreservation.
2 Materials and methods
2.1 Bacterial strain and culture conditions
The P. pentosaceus DSPZPP1 strain was isolated from traditional fermented sausage (Basilicata region, Southern Italy) (Parente et al., 2001; Bonomo et al., 2008). The strain was maintained as freeze-dried stocks in reconstituted (11% w/v) skim milk, containing 0.1% (w/v) ascorbic acid in the culture collection of the Microorganisms Genetics Laboratory of the Department of Sciences, University of Basilicata (Potenza, Italy), and routinely propagated in MRS broth at 37°C for 16 h before the analyses.
2.2 Whole-genome sequencing and annotation
Genomic DNA was extracted from 5 mL of late-exponential phase (A600 = 0.7–0.8) bacterial culture using The quick-DNATM fungal/bacterial MiniPrep Kit, provided By Zymo research Europe GmbH (Freiburg, Germany). DNA integrity Was confirmed through agarose electrophoresis, while Its concentration and purity were assessed By readings obtained using a NanoDrop ND-1000 spectrophotometer (NanoDrop technologies Inc., Wilmington, USA). The indexed libraries were generated using The Illumina Nextera DNA flex library preparation Kit. A whole-genome sequencing experiment was performed on The Illumina NextSeq 500 platform with a 2x150bp paired-end module at The biogem research institute, medicinal investigational research—M.I.R. (Ariano Irpino-AV, Italy). Prior quality control assessment Is performed using FASTQC v0.12.1.1 High-quality (HQ) filtered reads from The Raw data were obtained using NGS QC toolkit v2.3.3 (Patel and Jain, 2012) with The quality score cutoff ≥Q20. Primary assembly (contigs) were generated from high-quality filtered reads using short read de-novo assembler VELVET v1.2.10 (Zerbino, 2010). Scaffolding of pre-assembled contigs Was performed using SSPACE v2.0 (Boetzer et al., 2011). Output scaffolds were further anchored using Pediococcus representative genome P. pentosaceus ATCC 25745 [NCBI RefSeq ID: NC_008525.1] For correct ordering and orientation using MeDuSa WebServer: http://combo.dbe.unifi.it/medusa, Accessed February 2, 2023 (Bosi et al., 2015) To generate a final draft genome. Gene prediction and annotation Are performed using The online subsystem-based bacterial genome annotation server RAST: https://rast.nmpdr.org/, Accessed February 7, 2023 (Aziz et al., 2008). Ribosomal RNAs (rRNAs) and transfer RNAs (tRNAs) were detected using barrnap (Seemann, 2023) and ARAGORN (Laslett and Canback, 2004) tools, respectively.
2.3 Phylogenetic analysis and genome visualization
Phylogenetic analysis was performed using the 16 s rRNA gene to study the evolutionary relationship of the DSPZPP1 strain with other Pediococcus strains, obtaining dendrograms by the unweighted pair group method (UPGMA) clustering algorithm. 16 s rRNA gene sequence of DSPZPP1 was screened against the ‘16 s rRNA sequence database’ in EZBioCloud: https://www.ezbiocloud.net/, Accessed February 9, 2023 (Yoon et al., 2017a). Multiple sequence alignment (MSA) was performed using the MUSCLE program (Edgar, 2004) in MEGA11 standalone software (Tamura et al., 2021). Output MEGA alignment file was used to construct the phylogeny tree using the neighbor-joining (Mailund et al., 2006) method with a bootstrap value of 500. Graphical visualization of different sequence features for the circular genome of P. pentosaceus DSPZPP1 was performed using CGView’s PROKSEE server: https://proksee.ca/, Accessed February 9, 2023 (Stothard et al., 2019).
2.4 Comparative genome analysis
To evaluate the similarity between the DSPZPP1 draft genome and other Pediococcus strains, a pairwise genome sequence comparison was performed. This was measured in terms of average nucleotide identity (ANI) (Yoon et al., 2017b), and correlation indexes of tetra-nucleotide signatures (Tetra) (Noble et al., 1998) were studied by comparing the draft genome sequence of DSPZPP1 strain against complete genome sequences of other Pediococcus strains published on NCBI. Average nucleotide identity was calculated using BLAST (ANIb) (McGinnis and Madden, 2004) and MUMMER (ANIm) (Marçais et al., 2018), and analysis was performed using JSpeciesWS: https://jspecies.ribohost.com/jspeciesws/, Accessed February 9, 2023 (Richter et al., 2016).
2.5 Identification of plasmids and bacteriophages
DSPZPP1 Draft genome was screened for presence of plasmids and bacteriophages using PlasmidFinder v2.1: https://cge.food.dtu.dk/services/PlasmidFinder/, Accessed February 7, 2023 (Carattoli et al., 2014) and PHAge Search Tool Enhanced Release PHASTER webserver: https://phaster.ca/, Accessed February 9, 2023 (Arndt et al., 2016), respectively.
2.6 Identification of antimicrobial resistance genes, virulence genes, and prediction of pathogenicity
Screening of antimicrobial resistance genes was performed against different databases including Comprehensive Antibiotic Resistance Database (CARD) (Alcock et al., 2023), ResFinder (Florensa et al., 2022), ARG_ANNOT (Gupta et al., 2014), and NCBI AMRFinder Plus (Feldgarden et al., 2021). In addition, virulence factor genes in the draft genome were identified using the Virulence Factor Database (VFDB; Chen et al., 2005). Screening of both antimicrobial resistance and virulence genes against different mentioned databases is performed using the standalone tool ABRicate.2 In addition, the pathogenicity of sequenced strain toward the human host is assessed using PathogenFinder v1.1: https://cge.food.dtu.dk/services/PathogenFinder/, Accessed February 9, 2023 (Cosentino et al., 2013).
2.7 Analysis of carbohydrate-active enzymes (CAZymes)
Identification of different classes of carbohydrate active enzymes (CAZymes) was performed using an HMMER-based search (Wheeler and Eddy, 2013) against the carbohydrate-active enzyme database (CAZyDB) (Drula et al., 2022). Draft genome assembly of DSPZPP1 was scanned in dbCAN3 webserver: https://bcb.unl.edu/dbCAN2/index.php, Accessed February 13, 2023 (Yin et al., 2012) for automated annotation of CAZymes with E-value and coverage cutoff of 1e-15 and 0.35, respectively.
2.8 Identification of bacteriocins
Bacteriocins in the DSPZPP1 draft genome were analyzed using the web tool BAGEL4: http://bagel4.molgenrug.nl/, Accessed February 14, 2023 (van Heel et al., 2018). Subsequently, the bacteriocin domains were manually confirmed using BLASTp: https://blast.ncbi.nlm.nih.gov/Blast.cgi, Accessed February 14, 2023 (McGinnis and Madden, 2004) against databases of non-redundant protein sequences (nr) with 30% identity and 80% coverage thresholds.
3 Results
3.1 Draft genome assembly characteristics of Pediococcus pentosaceus DSPZPP1
Whole-genome sequencing using Illumina NextSeq 500 generated approximately 6.14 million paired-end reads. After quality control analysis, approximately 5.15 million HQ reads were obtained which were assembled into 15 scaffolds to generate the final draft genome of size 1,891,324 bp, with a G + C content of 37.3%. A total of 1988 protein-coding RNA genes were identified. Detailed genome assembly statistics and other characteristics are described in Table 1.
3.2 Functional annotation
Gene prediction and functional annotation of the DSPZPP1 draft genome are performed using RAST3 (Aziz et al., 2008), a subsystem-based classification system. A total of 1930 protein-encoding genes (PEGs) and 58 RNAs were predicted [see Supplementary Table S1 (ProteinEncodingGene_Annotation)]. Of 1930 coding sequences, 1,531 were functional (79.32%) and 399 were hypothetical/unknown proteins (20.6%). A total of four ribosomal RNAs, including one 16 s, one 23 s, and two 5 s (one partial sequence), 53 TRNAs, and one tmRNA, were identified. Predicted 1930 protein-encoding genes were assigned to 23 different SEED subsystem categories (Figure 1). It is observed that the maximum pool of protein-encoding genes contributed to “Protein Metabolism” (111) followed by “Carbohydrates” (97) and “Biosynthesis of Nucleosides & Nucleotides” (88). Different LAB strains are known to synthesize water-soluble B-group vitamins, which emphasizes its use in the production of effective vitamin-enriched products (LeBlanc et al., 2011). Our analysis reveals that the DSPZPP1 strain can synthesize B-group vitamins, including biotin, riboflavin, folate, and coenzyme A [see Supplementary Table S1 (RASTDetailedSubsystemAnnotation)], making DSPZPP1, a stronger probiotic candidate.
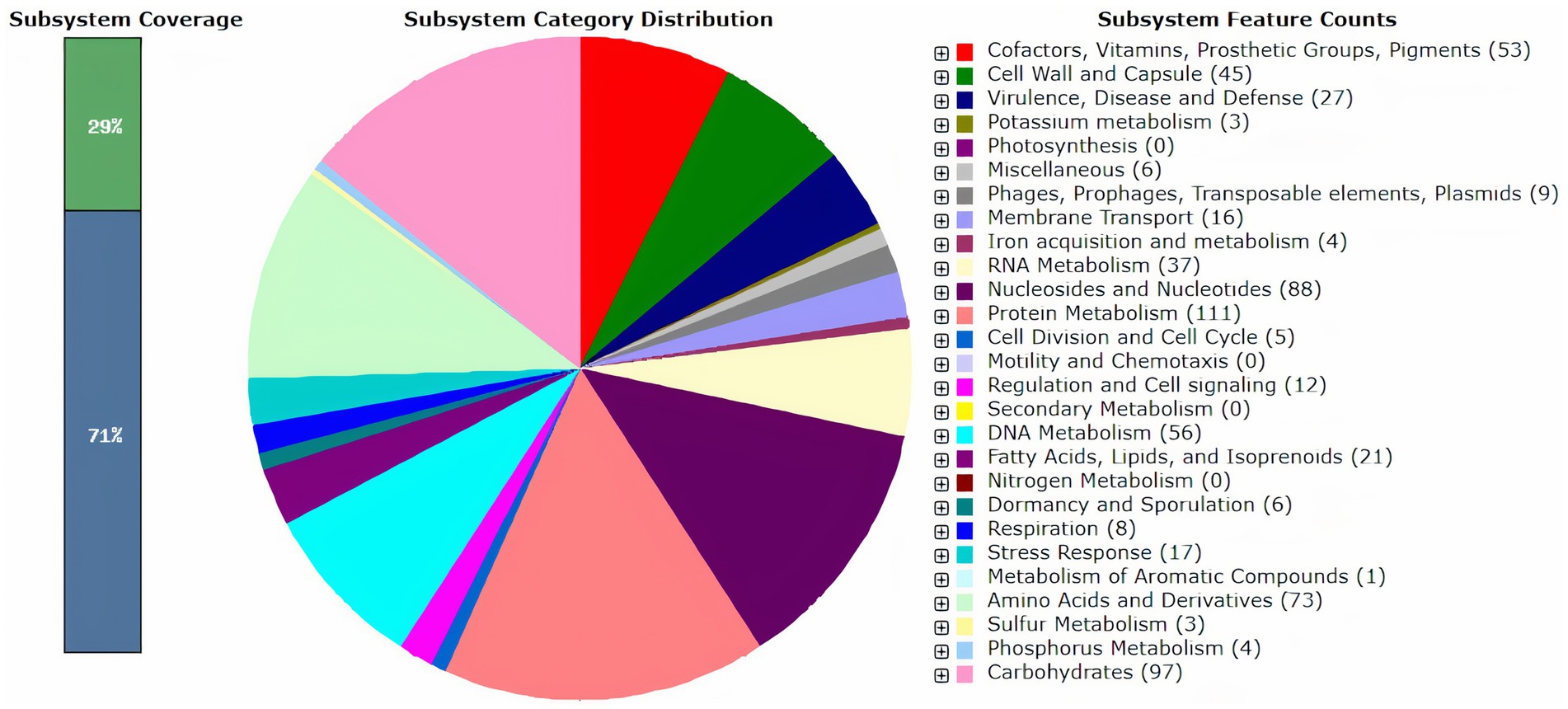
Figure 1. Overview of RAST functional annotation results for the draft genome of P. pentosaceus DSPZPP1. The number in parentheses for each subsystem category indicates the count of protein-encoding genes (PEGs) involved in the respective subsystem processes.
3.3 Taxonomy analysis and genome visualization
The UPGMA tree derived using 16S rRNA gene sequence shows that the DSPZPP1 strain has a closer phylogenetic relationship with other strains from the Pediococcus genus. P. pentosaceus strains are clustered together forming different branches compared to another genus. The phylogenetic tree derived using MEGA11 (Tamura et al., 2021) is depicted in Figure 2. Phylogeny analysis identified P. pentosaceus DSM 20336 strain [NCBI RefSeq accession: NZ_JQBF00000000.1] as the closest homolog of DSPZPP1. The phylogeny tree is depicted in Figure 2. Genomic features of the annotated draft genome of P. pentosaceus DSPZPP1 can be visualized using the circular genome map depicted in Figure 3.
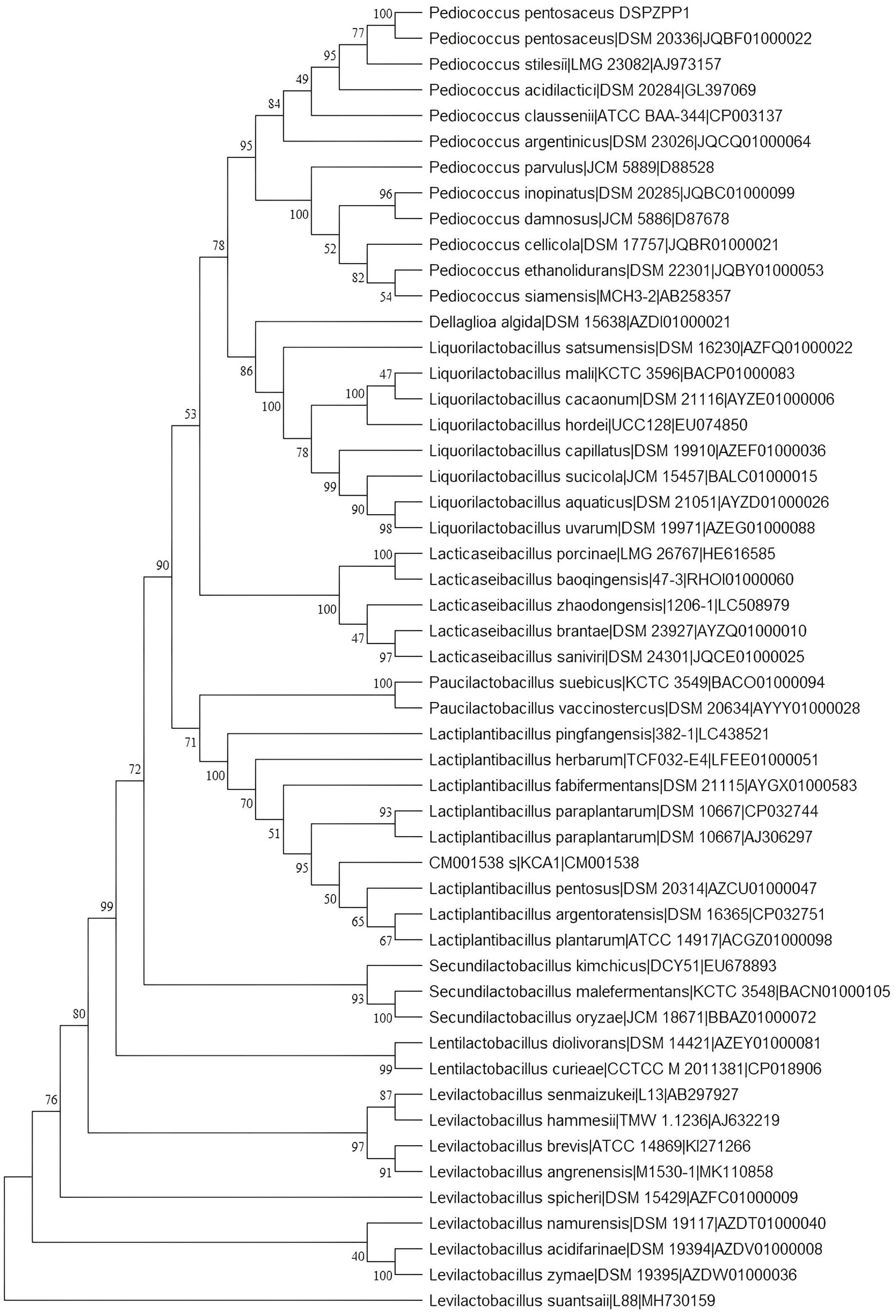
Figure 2. 16s rRNA gene-based phylogeny tree constructed using MEGA11. All the Pediococcus strains form a separate branch confirming the closest evolutionary relationship. The DSPZPP1 strain is identified as the closest evolutionary neighbor of P. pentosaceus DSM 20336.
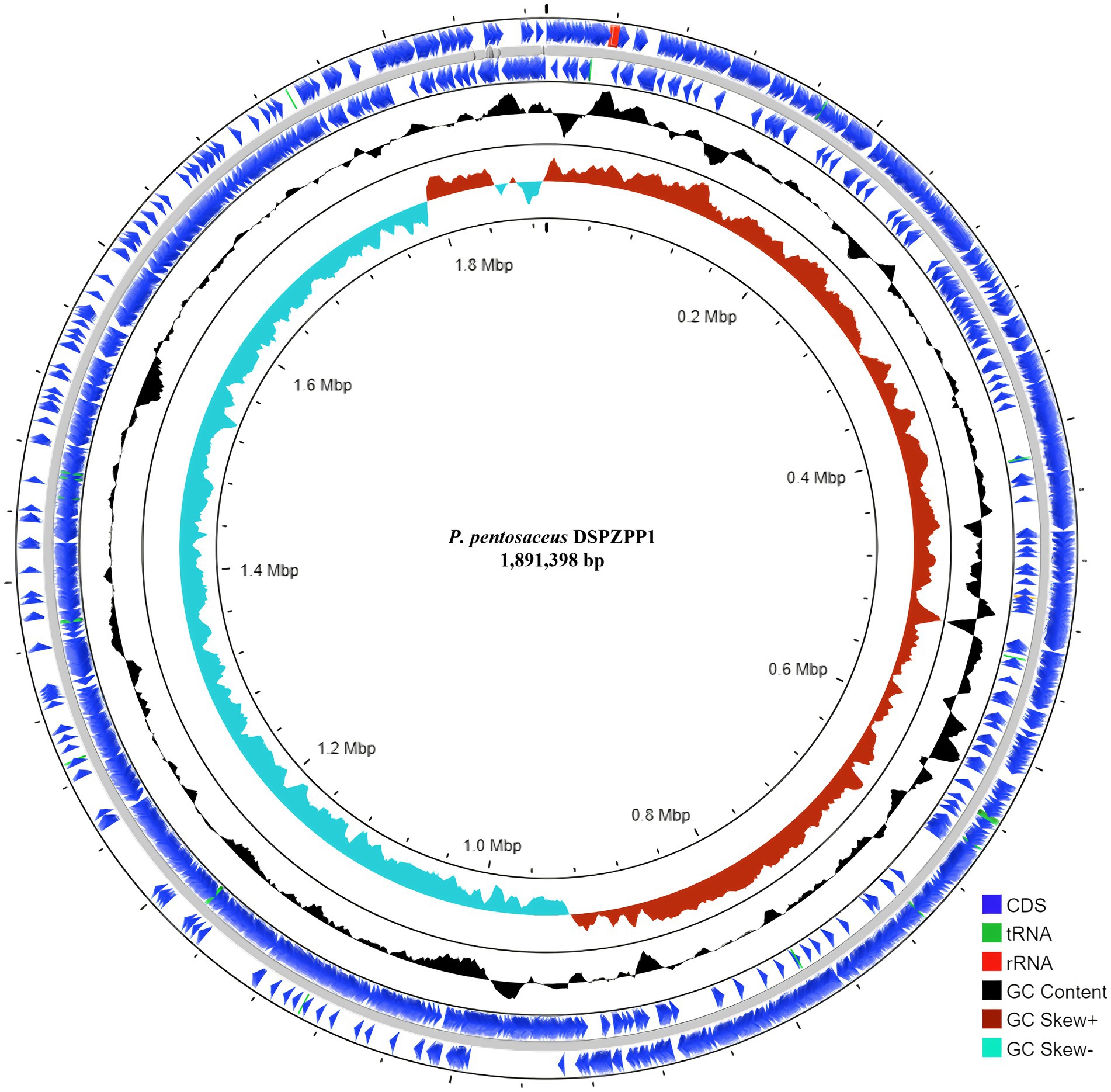
Figure 3. Circular genome map of P. pentosaceus DSPZPP1 of size 1.89 Mbp. Genome visualization consists of five rings. The outer concentric circle followed by the second circle represents the position of protein-coding genes and RNAs on forward and reverse strands, respectively. The middle circle represents draft genome scaffolds colored in gray followed by a ring showing GC content (black). Next, the inner ring shows GC Skew [(G–C)/(G+C)] distribution. Positive GC Skew is indicated in red and negative GC skew in sky blue.
3.4 Comparative genome analysis
Average nucleotide identity (ANI) analysis using BLAST (McGinnis and Madden, 2004) and MUMMER (Marçais et al., 2018), along with tetra correlation analysis study, confirms P. pentosaceus DSM 20336 strain is the closest phylogenetic neighbor of DSPZPP1. This conclusion is supported by the highest tetra correlation coefficient value of 0.9985. The ANI values obtained are 98.54 and 99.04% using BLAST and MUMMER, respectively. Detailed percent identity statistics of this analysis are described in Table 2.
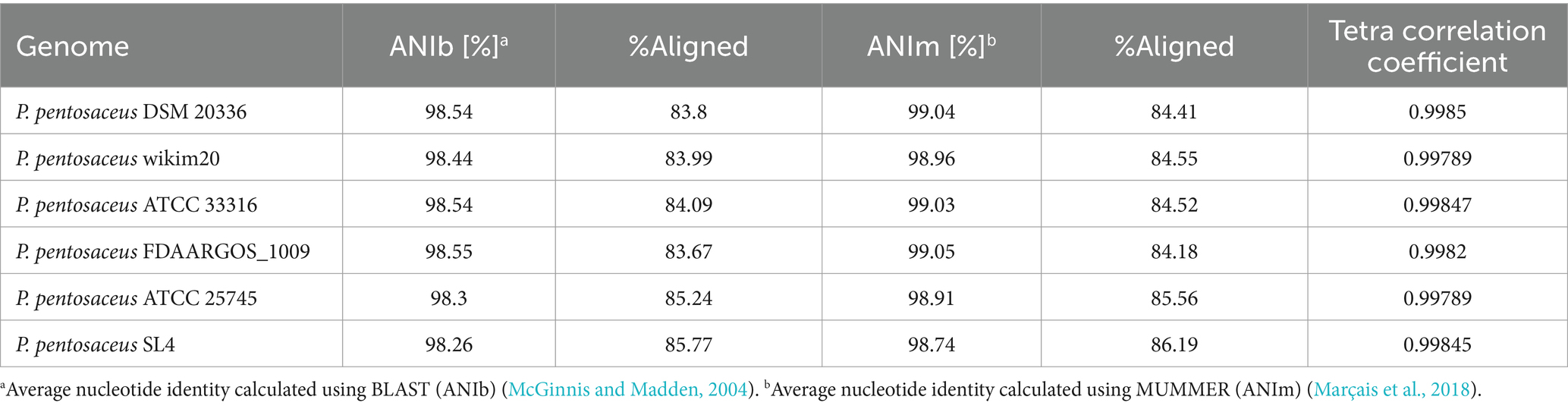
Table 2. Average nucleotide identity (ANI) and tetra frequency analysis P. pentosaceus DSPZPP1 genome in comparison with other P. pentosaceus strains.
3.5 Analysis of antimicrobial resistance (AMR), virulence genes, and pathogenicity
Different LAB strains including P. pentosaceus are used in various food industry applications (Lv et al., 2014; Torres-Miranda et al., 2022; Oliveira et al., 2023). Therefore, it is important to conduct a safety assessment prior to its use as a probiotic or within the food industry. Therefore, for the safety evaluation of the DSPZPP1 strain, the draft genome sequence was analyzed for the presence of antimicrobial resistance (AMR) genes, virulence genes, and pathogenicity toward the human host. Analysis shows the absence of antimicrobial resistance (Supplementary File S1) and virulence gene/s. Furthermore, pathogenicity analysis gives a probability value of 0.173, indicating a very low chance of this strain being a human pathogen (Supplementary File S2). Based on these findings, we can safely postulate that the P. pentosaceus DSPZPP1 strain is suitable for use as a probiotic and in different food industry applications.
3.6 Identification of plasmids and bacteriophages
Pediococcus pentosaceus DSPZPP1 does not show the presence of any plasmid sequences. Bacteriophage analysis identified the presence of two intact, one incomplete, and one questionable bacteriophage in the genome. All the identified bacteriophages are located on different regions of scaffold 1 (Figure 4) in the genome. Identified two intact plasmids—region 1 of length 43.4 Kb (Figure 5A) and region 2 of length 57.4 Kb (Figure 5B)—contain significant genes to be functional are described in Table 3. Detailed results of identified bacteriophages are described in Table 3.

Figure 4. Positions of four identified bacteriophages in draft genome sequence of P. pentosaceus DSPZPP1. The four identified bacteriophages include two intact, one questionable, and one incomplete which are located on scaffold 1 having genome coordinates as Scaffold_1:616309 bp - 629709 bp, Scaffold_1:652710 bp −696,134 bp, Scaffold_1:925982–948,780, and Scaffold_1:948804–1,006,219, for bacteriophage one, two, three and four, respectively.
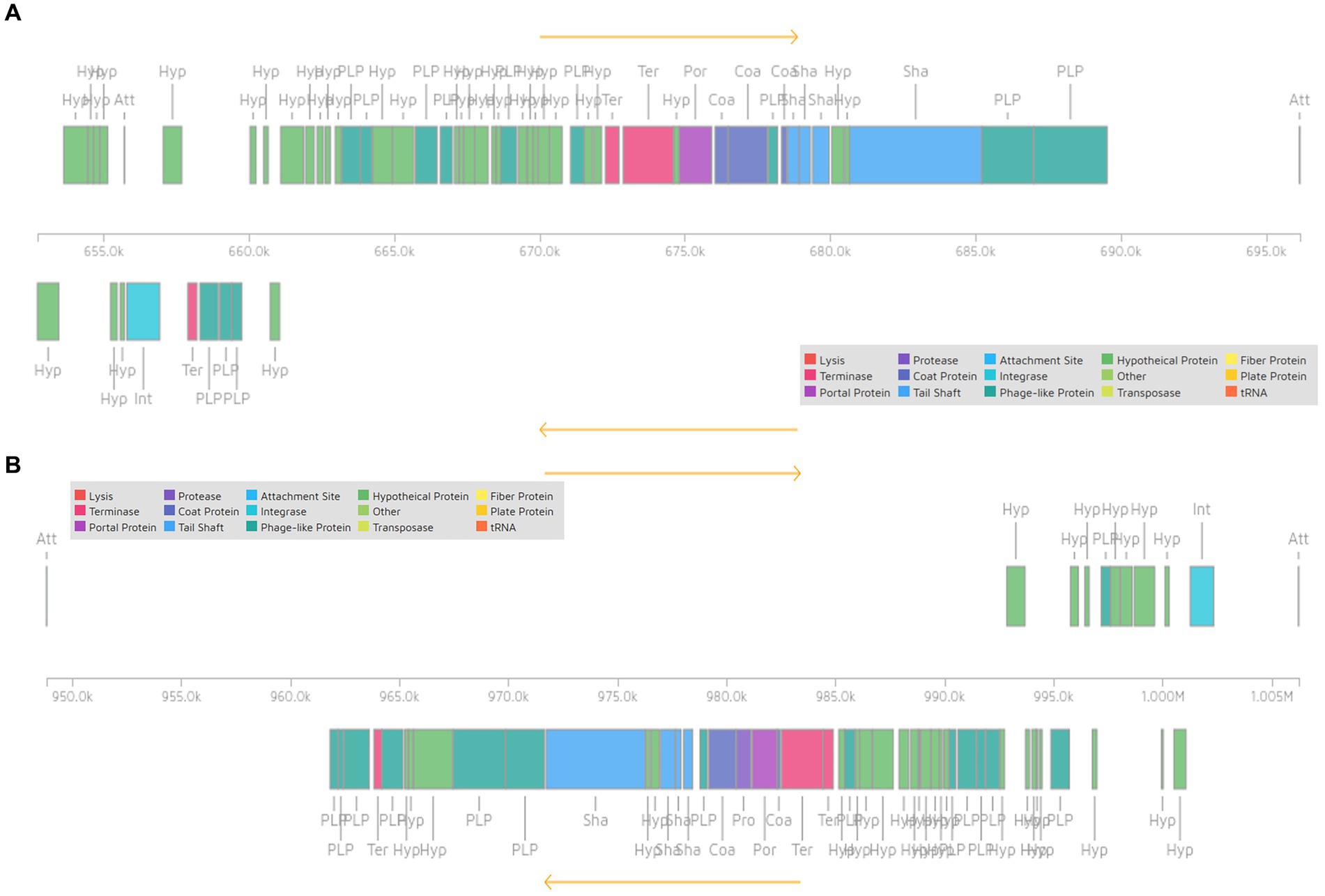
Figure 5. Representation of intact bacteriophage region 1 [Scaffold_1:652710–696,134] (A) and region 2 [Scaffold_1:948804–1,006,219] (B) showing functional genes.
3.7 Analysis of carbohydrate-active enzymes (CAZymes)
HMMER-based screening in dbCAN3 identified 46 carbohydrate activity-related enzymes in the DSPZPP1 draft genome from four different CAZyme classes, namely glycosyl transferases (GTs), glycoside hydrolases (GHs), carbohydrate esterases (CEs), and auxillary activities (AAs) (Figure 6). Highest number (21) of CAZymes were identified from glycosyl transferases class, which are known to be involved in the formation of glycosidic bonds, followed by glycoside hydrolases (20), modulating the hydrolysis and rearrangement of glycosidic bonds along with few other classes depicted in Figure 6. A list of all identified CAZymes is available in Supplementary Table S2.
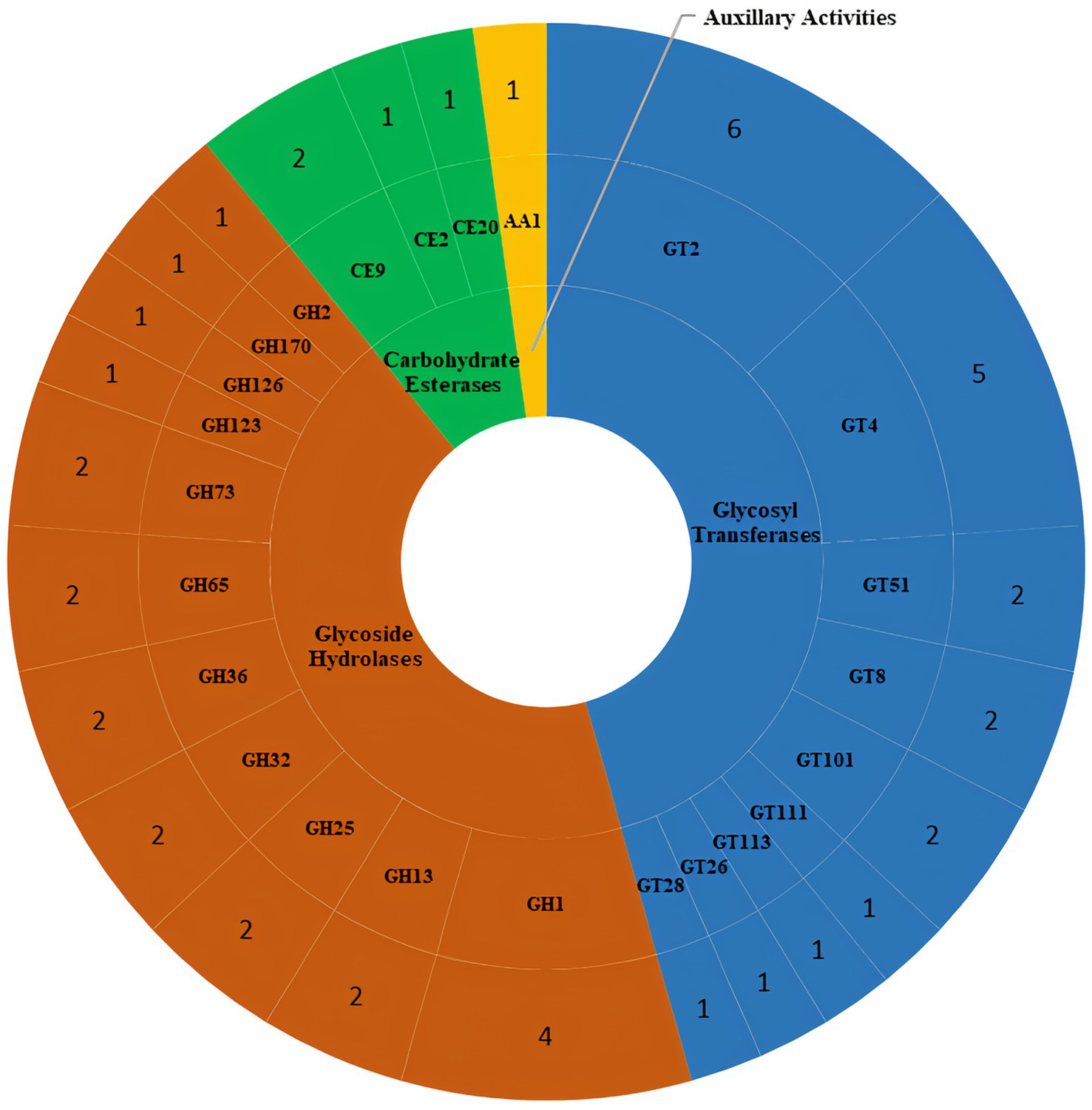
Figure 6. Distribution of different classes of carbohydrate-active enzymes (CAZymes) identified in the P. pentosaceus DSPZPP1 draft genome. Different CAZymes classes are represented in different colors. From the inner to the outer ring, the representation includes the Class of CAZyme, CAZyme families, and count of genes identified in each CAZyme family, respectively.
3.8 Bacteriocin identification
Pediococcus pentosaceus is a lactic acid bacterium, which produces small bactericidal peptides called bacteriocins. Pediococcus strains are used as natural preservatives to extend the shelf life and hygienic quality of food because of their characteristic property of producing bacteriocins. It acts against different food contamination-causing bacteria such as Staphylococcus aureus, Listeria monocytogenes, and Clostridium botulinum. DSPZPP1 draft genome was assessed for the presence of bacteriocin compounds. The analysis identified the presence of pediocin-like bacteriocin gene, penocin A, class II bacteriocin (Diep et al., 2006) on scaffold 1 from position 1916 bp to 22,093 bp (Figure 7). Identified bacteriocin peptide sequence is used for BLASTp analysis. Penocin A of the DSPZPP1 genome shows an exact match (100% identity) with the “class II bacteriocin” protein of Pediococcus (NCBI Accession ID: WP_011672822.1) having 8e-36 E-value and 100% query coverage. This confirms the presence of bacteriocin penocin A in the draft genome sequence of P. pentosaceus DSPZPP1. The analysis concludes the ability of the DSPZPP1 strain to produce the class II bacteriocin, confirming its significance in selection as a probiotic strain.
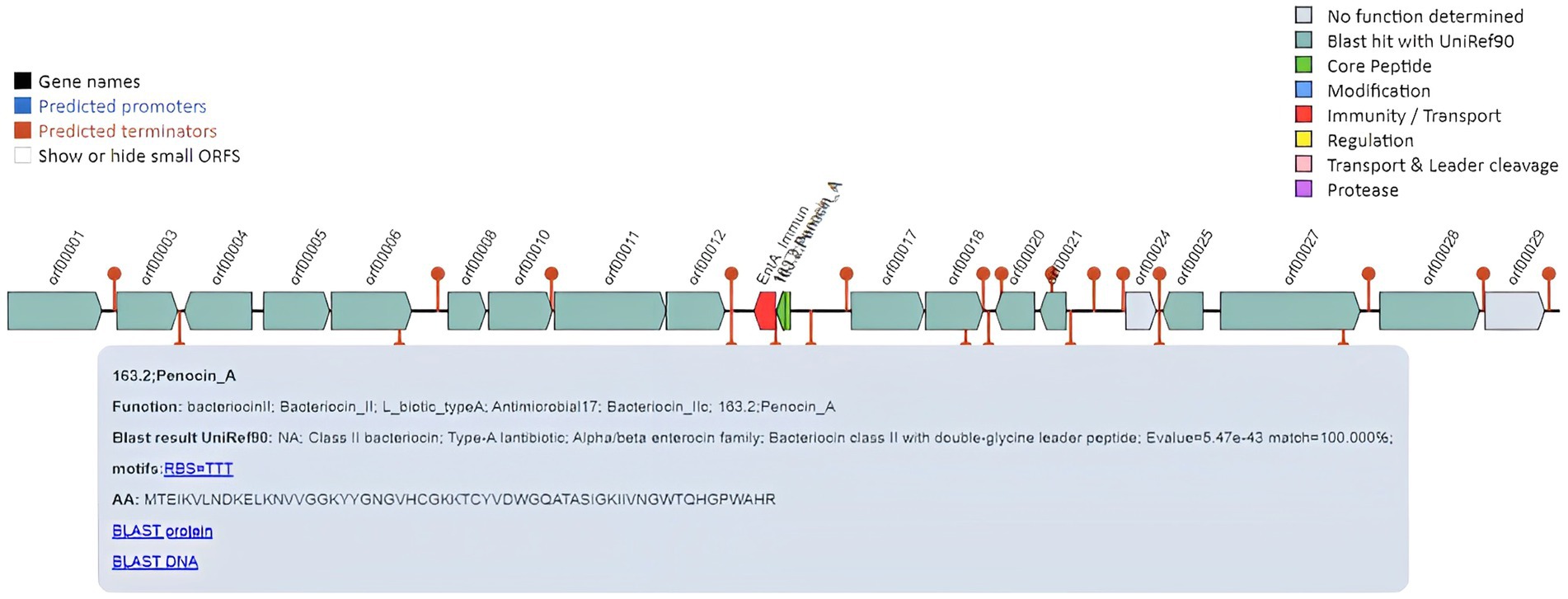
Figure 7. Overview of pediocin-like bacteriocin gene Penocin A in the draft genome of P. pentosaceus DSPZPP1.
4 Discussion
Currently, numerous P. pentosaceus strains have been isolated and identified from different fermented food sources such as milk and sausages. Most of the food-derived strains were shown to be safe and very stable, confirming to have probiotic effects (Min et al., 2017). In the recent years, different P. pentosaceus strains were studied (Martino et al., 2013; Vidhyasagar and Jeevaratnam, 2013; Savedboworn et al., 2014; Dubey et al., 2015; Chen et al., 2017) and tested having promising potential as a biological preservative for foods and flavor additive appearing as a potentially predominant probiotic strain (Jiang et al., 2021). It has also been observed that it possesses interesting properties such as antimicrobial ability, resistance to stresses, oxidative level reduction, bile tolerance, β-galactosidase activity, impact on gut microbiome composition, and resistance to gastrointestinal tract conditions (Osmanagaoglu et al., 2010; Dubey et al., 2016; Li et al., 2021). Such investigations support the use of Pediococcus strains in the field of probiotics, suggesting role of newly isolated strains from varied food products belonging to the same genus may play a key role in being used in a new generation of functional foods. Looking simultaneously at the continuous demand for safe food by consumers and the unique properties of different LAB strains leading them to potential probiotic candidates, it is necessary to expand the list of potential probiotic bacteria. Therefore, before practical use of bacteria as a probiotic, it is important to perform specific study, which focuses on different aspects involving microorganisms’ safety evaluation, acquired antibiotic resistance, virulence factors, and probiotic viability at the end of shelf life to claim suggested single strain as GRAS (generally regarded as safe) or QPS (qualified presumption of safety) (Dobson et al., 2002; Todorov and Dicks, 2009; Zommiti et al., 2018; Li et al., 2021).
This study conducted on the bacterial strain P. pentosaceus DSPZPP1 is the continuation and deepening of previous in vitro studies carried out to isolate and investigate the microflora from traditional fermented sausages of Basilicata region, in order to formulate autochthonous starter cultures and to standardize the production process of sausages, to preserve their typical organoleptic and sensory characteristics, and to improve the quality of final product (Parente et al., 2001; Bonomo et al., 2008). In these products, LAB play an important role in meat preservation and fermentation processes and are considered technologically fundamental, providing diversity by the modification of raw material to obtain new sensory properties and improve the safety, stability, and shelf life of meat products (Fontana et al., 2005) and contribute to the flavor, color, and texture development (Aymerich et al., 2006). The identification and technological characterization of LAB involved in meat fermentation are crucial to selecting the best strains to use as starters (Ammor et al., 2005; Rantsiou et al., 2006).
The results of Bonomo et al. (2008) highlighted that the LAB population plays a significant role in influencing the organoleptic and sensory characteristics of the final product. This influence is exerted through specific and important activities that enhance the quality and safety of fermented sausages. Moreover, the LAB ecosystem is characterized by a species-site-dominance, and this dominance is closely related to the environmental parameters. Traditional fermented sausages show a distinctive organoleptic profile, which can be attributed to the isolated strains. Although these strains belong to the species commonly associated with sausage fermentation, they possess specific physiological and technological characteristics that contribute to the uniqueness of these traditional products (Bonomo et al., 2008). Therefore, this study evaluated the complete genome sequence of P. pentosaceus DSPZPP1 and performed a complete characterization of the assembled draft genome. Functional annotation of protein-encoding genes predicted from assembled draft genome shows the presence of gene sequences responsible for the metabolism of carbohydrates and proteins, which is an important probiotic and technological feature in LAB (Franz et al., 2006; LeBlanc et al., 2011). Functional annotation analysis highlights the presence of gene sequences responsible for central carbohydrates and metabolism of proteins/amino acids, biosynthesis, and degradation of different compounds, such as cell envelope components, and for transport mechanisms, marking important features in probiotic strains (Oliveira et al., 2023). Moreover, the tolerance to acids and bile was another significant characteristic in LAB because the strains must resist transit and the complexity of the gastrointestinal tract to perform its probiotic functional role. In the P. pentosaceus DSPZPP1 genome, the F0F1-ATPase synthase complex proteins and general stress response genes are bacterial defense mechanisms that allow strains to respond and thrive in rapidly changing environmental conditions ensuring the proper functioning of DNA repair and structure pathways (Cotter and Hill, 2003; Sanchez et al., 2008; Oliveira et al., 2023). Functional annotation analysis also revealed the potential of P. pentosaceus DSPZPP1 strain to biosynthesize different naturally occurring water-soluble vitamin B molecules including riboflavin, biotin, and folate [see Supplementary Table S1 (RASTDetailedSubsystemAnnotation)].
Many lactic acid bacteria (LAB) strains such as L. fermentum, L. gasseri, and L. lactis are known to produce B-group vitamins (Sybesma et al., 2004; Jayashree et al., 2010; LeBlanc et al., 2011). This property makes LAB strains promising supplements to enhance the biological activity of compounds (Nguyen et al., 2015) and are currently being used in different applications in the food industry (LeBlanc et al., 2011; Nguyen et al., 2015; Levit et al., 2021; Wang et al., 2021). The property of vitamin B biosynthesis by isolated DSPZPP1 strain implies its significant potential use as a vitamin supplement along with its other applications in the food industry. 16s rRNA-based phylogeny analysis integrated with ANI and TETRA analysis indicates the closest evolutionary relationship between sequenced P. pentosaceus DSPZPP1 strain and other P. pentosaceus strains available at NCBI.
In total, 46 CAZymes from four different classes have been identified that play a key role in sugar metabolism. Different types of GTs are involved in the biosynthesis of disaccharides, oligosaccharides, and polysaccharides, which contribute to the formation of glycosidic bonds (Drula et al., 2022). CAZyme analysis identified 21 enzymes belonging to 9 GT families, belonging to GT2 and GT4 families representing 52.4% of the total GTs, which are responsible for the synthesis of sucrose, cellulose and chitin synthases, glucosyltransferase, and galactosyltransferase. Another majority class of enzymes involved in carbohydrate metabolism was that of GHs with an important role in the hydrolysis of the carbohydrate glycosidic bonds; among the 20 GHs belonging to 11 families, the genes responsible for the synthesis of β-glucosidase (GH1), β-galactosidase (GH2), α-glucosidase (GH13) are present in the genome of DSPZPP1 strain. These enzymes are fundamental in the development of the strain in different environments as they act in the metabolism of sugars, such as lactose, sucrose, and oligosaccharides (Vera et al., 2020; Drula et al., 2022; Oliveira et al., 2023). Moreover, enzymes of the family GH73 were also detected, which are responsible for the cleavage of the β-1,4-glycosidic linkage between N-acetylglucosamine (NAG) and N-acetylmuramic acid (NAM) of bacterial peptidoglycan; because of their cleavage specificity, they are commonly described as β-N-acetylglucosaminidases. Enzyme lysozyme (GH25) was also identified as involved in the cleavage of the β-1,4-glycosidic bond of bacterial cell walls. The characterized lysozymes from this family exhibit both β-1,4-N-acetyl- and β-1,4-N,6-O-diacetylmuramidase activities and degrade O-acetylated peptidoglycan present in Staphylococcus aureus and other pathogens (Levit et al., 2021). These enzymes work as a primary line of defense against bacterial infections. Additionally, the P. pentosaceus DSPZPP1 genome also encodes GH36 enzymes that exhibit α-galactosidase and α-N-acetylgalactosaminidase activity, GH32 family that contains invertases and enzymes hydrolyze fructose-containing different polysaccharides, and enzymes belonging to GH65 act on substrates containing α-glucosidic linkages and contains mainly phosphorylases (Vera et al., 2020). The genome-encoded CAZymes play an important role in carbohydrate synthesis and hydrolysis during the fermentation process; so, the ability of the bacteria to use carbohydrates is a significant indicator of the functionality of the strain and puts the basics for the cultivation and selection of the strain (Jiang et al., 2020). A further class of enzymes identified with the CAZymes analysis was CEs belonging to three families. CE2 enzymes are α/β-hydrolases, containing an N-terminal β-sheet “jelly-roll” domain that acts as a carbohydrate-binding domain (CBM) and is linked to a C-terminal domain that contains the α/β-hydrolase fold (SGNH-hydrolase motif) (Vollmer, 2008). CE family 9 esterases catalyze the deacetylation of N-acetylglucosamine-6-phosphate to glucosamine-6-phosphate and this reaction has been demonstrated to be important for both amino sugar metabolism and peptidoglycan cell wall recycling in bacteria (Egloff et al., 2001). Carbohydrate esterase family 20 (CE20) comprises xyloglucan acetyl esterases (XacXaeA) putatively related to arabinoxylan deacetylation. XacXaeA is the founding member of the family, specific to O-acetylation, as it is not capable of cleaving N-acetylated carbohydrates. It shows activity on a broad range of O-acetylated mono- and disaccharides and did not show a positional preference for acetylated oxygens. XacXaeA was active toward cell wall extracted xyloglucan oligosaccharides, deacetylating distinct types of structures (Montanier et al., 2009).
The effective probiotic LAB strains mediate antagonistic effects against pathogens by producing internal antimicrobial substances, influencing the metabolism, or stimulating the host immunity. These strains can tolerate acid and bile, adhere to epithelial surfaces, and exhibit antimicrobial properties and antagonistic activity against intestinal pathogens (Park, 2001; Liu et al., 2022). Among all effective probiotic LABs, P. pentosaceus is a known potential probiotic strain and has shown broad-spectrum antibacterial activity against external foodborne pathogens by preventing the adhesion of pathogenic bacteria in the gut (Bambirra et al., 2007; Savard et al., 2011). P. pentosaceus strains have been studied to produce bacteriocins or other antimicrobial compounds, such as extracellular polysaccharides, when they form biofilms or are attached to the surface, and have been found to show antagonistic effects against various foodborne pathogens (Papagianni and Anastasiadou, 2009; Ladha and Jeevaratnam, 2018; Todhanakasem et al., 2020). In our analysis, the P. pentosaceus DSPZPP1 genome shows the presence of the pediocin-like bacteriocin gene penocin A, a class II bacteriocin. Class II pediocin-like bacteriocins are an important and dominant group of antimicrobial peptides that have common features in gene clusters, the structural genes coding for the central peptide, the gene coding for the immune protein and the transporter genes (Papagianni and Anastasiadou, 2009; Cui et al., 2012; Collins et al., 2017; Chen et al., 2020; Tatsaporn and Kornkanok, 2020; Oliveira et al., 2023). In this study, the identified operon of penocin A presented the necessary genes and it is a cluster of biosynthetic genes encoding bacteriocin (Miller et al., 2005; Drider et al., 2006).
In addition to this probiotic characteristic, the draft genome does not show the presence of virulence genes and antimicrobial resistance (AMR) genes. Low pathogenicity value characterizes sequenced strains as non-human pathogens and can be safely used in biological applications. Therefore, these properties make the P. pentosaceus DSPZPP1 strain an excellent candidate to be used safely as a probiotic as well as a natural preservative to improve the quality of food. The presence of mobile genetic elements is an important factor to be investigated in microorganisms destined for food application as the presence of plasmids and prophages can be vehicles of horizontal gene transfer of pathogenic genes or antimicrobial resistance genes to other microorganisms (Canchaya et al., 2003; Ferri et al., 2017; Oliveira et al., 2023). The absence of plasmid sequences indicates the stability of the DSPZPP1 genome. Four bacteriophages were identified located at different positions in the genome described in Table 3.
In conclusion, our analysis revealed that the P. pentosaceus DSPZPP1 strain, isolated from Italian fermented sausages, can serve as a promising probiotic bacterium. Additionally, it may be used as a future additive in biopreservation to enhance food quality, extend shelf life, and contribute to the final technological properties of the biological product. Furthermore, detailed elucidation of the DSPZPP1 draft genome sequence provides deeper insights into the characteristics of isolated strains, which may contribute to the development of probiotics and future additives in future.
Data availability statement
The datasets presented in this study can be found in online repositories. The names of the repository/repositories and accession number(s) can be found in the article/Supplementary material.
Author contributions
MT: Investigation, Writing – original draft, Data curation, Formal analysis. MGB: Investigation, Writing – original draft, Conceptualization, Methodology. SZ: Conceptualization, Methodology, Writing – original draft. SM: Investigation, Writing – original draft. MB: Formal analysis, Methodology, Writing – original draft. IC: Supervision, Writing – review & editing. MC: Project administration, Supervision, Validation, Writing – review & editing. GS: Project administration, Supervision, Validation, Writing – review & editing.
Funding
The author(s) declare that no financial support was received for the research, authorship, and/or publication of this article.
Conflict of interest
MGB and GS were employed by Spinoff TNcKILLERS.
The remaining authors declare that the research was conducted in the absence of any commercial or financial relationships that could be construed as a potential conflict of interest.
The author(s) declared that they were an editorial board member of Frontiers, at the time of submission. This had no impact on the peer review process and the final decision.
Publisher’s note
All claims expressed in this article are solely those of the authors and do not necessarily represent those of their affiliated organizations, or those of the publisher, the editors and the reviewers. Any product that may be evaluated in this article, or claim that may be made by its manufacturer, is not guaranteed or endorsed by the publisher.
Supplementary material
The Supplementary material for this article can be found online at: https://www.frontiersin.org/articles/10.3389/fmicb.2024.1268216/full#supplementary-material
Footnotes
1. ^https://www.bioinformatics.babraham.ac.uk/projects/fastqc/
References
Alcock, B. P., Huynh, W., Chalil, R., Smith, K. W., Raphenya, A. R., Wlodarski, M. A., et al. (2023). CARD 2023: expanded curation, support for machine learning, and resistome prediction at the comprehensive antibiotic resistance database. Nucleic Acids Res. 51, D690–D699. doi: 10.1093/nar/gkac920
Ammor, S., Rachman, C., Chaillou, S., Prévost, H., Dousset, X., Zagorec, M., et al. (2005). Phenotypic and genotypic identification of lactic acid bacteria isolated from a small-scale facility producing traditional dry sausages. Food Microbiol. 22, 373–382. doi: 10.1016/j.fm.2004.11.005
Arndt, D., Grant, J. R., Marcu, A., Sajed, T., Pon, A., Liang, Y., et al. (2016). PHASTER: a better, faster version of the PHAST phage search tool. Nucleic Acids Res. 44, W16–W21. doi: 10.1093/nar/gkw387
Asami, K., Kondo, A., Suda, Y., Shimoyamada, M., and Kanauchi, M. (2017). Neutralization of lipopolysaccharide by heat shock protein in Pediococcus pentosaceus AK-23. J. Food Sci. 82, 1657–1663. doi: 10.1111/1750-3841.13679
Aymerich, T., Martin, B., Garriga, M., Vidal-Carou, M. C., Bover-Cid, S., and Hugas, M. (2006). Safety properties and molecular strain typing of lactic acid bacteria from slightly fermented sausages. J. Appl. Microbiol. 100, 40–49. doi: 10.1111/j.1365-2672.2005.02772.x
Aziz, R. K., Bartels, D., Best, A. A., DeJongh, M., Disz, T., Edwards, R. A., et al. (2008). The RAST server: rapid annotations using subsystems technology. BMC Genomics 9:75. doi: 10.1186/1471-2164-9-75
Balakrishnan, G., and Agrawal, R. (2014). Antioxidant activity and fatty acid profile of fermented milk prepared by Pediococcus pentosaceus. J. Food Sci. Technol. 51, 4138–4142. doi: 10.1007/s13197-012-0891-9
Bambirra, F. H. S., Lima, K. G. C., Franco, B. D. G. M., Cara, D. C., Nardi, R. M. D., Barbosa, F. H. F., et al. (2007). Protective effect of Lactobacillus sakei 2a against experimental challenge with Listeria monocytogenes in gnotobiotic mice. Lett. Appl. Microbiol. 45, 663–667. doi: 10.1111/j.1472-765X.2007.02250.x
Barbosa, A. A. T., Mantovani, H. C., and Jain, S. (2017). Bacteriocins from lactic acid bacteria and their potential in the preservation of fruit products. Crit. Rev. Biotechnol. 37, 852–864. doi: 10.1080/07388551.2016.1262323
Baruzzi, F., Matarante, A., Caputo, L., and Morea, M. (2006). Molecular and physiological characterization of natural microbial communities isolated from a traditional southern Italian processed sausage. Meat Sci. 72, 261–269. doi: 10.1016/j.meatsci.2005.07.013
Boetzer, M., Henkel, C. V., Jansen, H. J., Butler, D., and Pirovano, W. (2011). Scaffolding pre-assembled contigs using SSPACE. Bioinformatics 27, 578–579. doi: 10.1093/bioinformatics/btq683
Bonomo, M. G., Milella, L., Martelli, G., and Salzano, G. (2013). Stress response assessment of Lactobacillus sakei strains selected as potential autochthonous starter cultures by flow cytometry and nucleic acid double staining analyses. J. Appl. Microbiol. 115, 786–795. doi: 10.1111/jam.12271
Bonomo, M. G., Ricciardi, A., Zotta, T., Parente, E., and Salzano, G. (2008). Molecular and technological characterization of lactic acid bacteria from traditional fermented sausages of Basilicata region (southern Italy). Meat Sci. 80, 1238–1248. doi: 10.1016/j.meatsci.2008.05.032
Bosi, E., Donati, B., Galardini, M., Brunetti, S., Sagot, M. F., Lió, P., et al. (2015). MeDuSa: a multi-draft based scaffolder. Bioinformatics 31, 2443–2451. doi: 10.1093/bioinformatics/btv171
Canchaya, C., Proux, C., Fournous, G., Bruttin, A., and Brüssow, H. (2003). Prophage genomics. Microbiol. Mol. Biol. Rev. 67, 238–276. doi: 10.1128/MMBR.67.2.238-276.2003
Carafa, I., Nardin, T., Larcher, R., Viola, R., Tuohy, K., and Franciosi, E. (2015). Identification and characterization of wild lactobacilli and pediococci from spontaneously fermented mountain cheese. Food Microbiol. 48, 123–132. doi: 10.1016/j.fm.2014.12.003
Carattoli, A., Zankari, E., García-Fernández, A., Voldby Larsen, M., Lund, O., Villa, L., et al. (2014). In silico detection and typing of plasmids using plasmid finder and plasmid multilocus sequence typing. Antimicrob. Agents Chemother. 58, 3895–3903. doi: 10.1128/AAC.02412-14
Chen, T., Wang, L., Li, Q., Long, Y., Lin, Y., Yin, J., et al. (2020). Functional probiotics of lactic acid bacteria from Hu sheep milk. BMC Microbiol. 20:228. doi: 10.1186/s12866-020-01920-6
Chen, L., Yang, J., Yu, J., Yao, Z., Sun, L., Shen, Y., et al. (2005). VFDB: a reference database for bacterial virulence factors. Nucleic Acids Res. 33, D325–D328. doi: 10.1093/nar/gki008
Chen, F., Zhu, L., and Qiu, H. (2017). Isolation and probiotic potential of Lactobacillus salivarius and Pediococcus pentosaceus in specific pathogen free chickens. Braz. J. Poult. Sci. 19, 325–332. doi: 10.1590/1806-9061-2016-0413
Cocolin, L., Rantsiou, K., Iacumin, L., Urso, R., Cantoni, C., and Comi, G. (2004). Study of the ecology of fresh sausages and characterization of populations of lactic acid bacteria by molecular methods. Appl. Environ. Microbiol. 70, 1883–1894. doi: 10.1128/AEM.70.4.1883-1894.2004
Collins, F. W. J., O’Connor, P. M., O’Sullivan, O., Gómez-Sala, B., Rea, M. C., Hill, C., et al. (2017). Bacteriocin gene-trait matching across the complete Lactobacillus pan-genome. Sci. Rep. 7:3481. doi: 10.1038/s41598-017-03339-y
Comi, G., Urso, R., Iacumin, L., Rantsiou, K., Cattaneo, P., Cantoni, C., et al. (2005). Characterisation of naturally sausages produced in the north east of Italy. Meat Sci. 69, 381–392. doi: 10.1016/j.meatsci.2004.08.007
Cosentino, S., Larsen, M. V., Aarestrup, F. M., and Lund, O. (2013). PathogenFinder - distinguishing friend from foe using bacterial whole genome sequence data. PLoS One 8:e77302. doi: 10.1371/journal.pone.0077302
Cotter, P. D., and Hill, C. (2003). Surviving the acid test: responses of gram-positive bacteria to low pH. Microbiol. Mol. Biol. Rev. 67, 429–453. doi: 10.1128/MMBR.67.3.429-453.2003
Cotter, P. D., Ross, R. P., and Hill, C. (2013). Bacteriocins — a viable alternative to antibiotics? Nat. Rev. Microbiol. 11, 95–105. doi: 10.1038/nrmicro2937
Cui, Y., Zhang, C., Wang, Y., Shi, J., Zhang, L., Ding, Z., et al. (2012). Class IIa bacteriocins: diversity and new developments. Int. J. Mol. Sci. 13, 16668–16707. doi: 10.3390/ijms131216668
Diep, D. B., Godager, L., Brede, D., and Nes, I. F. (2006). Data mining and characterization of a novel pediocin-like bacteriocin system from the genome of Pediococcus pentosaceus ATCC 25745. Microbiol. 152, 1649–1659. doi: 10.1099/mic.0.28794-0
Dobson, C. M., Deneer, H., Lee, S., Hemmingsen, S., Glaze, S., and Ziola, B. (2002). Phylogenetic analysis of the genus Pediococcus, including Pediococcus claussenii sp. nov., a novel lactic acid bacterium isolated from beer. Int. J. Syst. Evol. Microbiol. 52, 2003–2010. doi: 10.1099/00207713-52-6-2003
Drider, D., Fimland, G., Héchard, Y., McMullen, L. M., and Prévost, H. (2006). The continuing story of class IIa bacteriocins. Microbiol. Mol. Biol. Rev. 70, 564–582. doi: 10.1128/MMBR.00016-05
Drosinos, E. H., Mataragas, M., Xiraphi, N., Moschonas, G., Gaitis, F., and Metaxopoulos, J. (2005). Characterization of the microbial flora from a traditional fermented sausage. Meat Sci. 69, 307–317. doi: 10.1016/j.meatsci.2004.07.012
Drula, E., Garron, M. L., Dogan, S., Lombard, V., Henrissat, B., and Terrapon, N. (2022). The carbohydrate-active enzyme database: functions and literature. Nucleic Acids Res. 50, D571–D577. doi: 10.1093/nar/gkab1045
Dubey, V., Ghosh, A. R., Bishayee, K., and Khuda-Bukhsh, A. R. (2015). Probiotic Pediococcus pentosaceus strain GS4 alleviates azoxymethane-induced toxicity in mice. Nut. Res. 35, 921–929. doi: 10.1016/j.nutres.2015.08.001
Dubey, V., Ghosh, A. R., Bishayee, K., and Khuda-Bukhsh, A. (2016). Appraisal of the anti-cancer potential of probiotic Pediococcus pentosaceus GS4 against colon cancer: in vitro and in vivo approaches. J. Funct. Foods 23, 66–79. doi: 10.1016/j.jff.2016.02.032
Edgar, R. C. (2004). MUSCLE: a multiple sequence alignment method with reduced time and space complexity. BMC Bioinformatics 5:113. doi: 10.1186/1471-2105-5-113
Egloff, M. P., Uppenberg, J., Haalck, L., and van Tilbeurgh, H. (2001). Crystal structure of maltose phosphorylase from Lactobacillus brevis: unexpected evolutionary relationship with glucoamylases. Structure 9, 689–697. doi: 10.1016/S0969-2126(01)00626-8
Feldgarden, M., Brover, V., Gonzalez-Escalona, N., Frye, J. G., Haendiges, J., Haft, D. H., et al. (2021). AMRFinderPlus and the reference gene catalog facilitate examination of the genomic links among antimicrobial resistance, stress response, and virulence. Sci. Rep. 11:12728. doi: 10.1038/s41598-021-91456-0
Ferri, M., Ranucci, E., Romagnoli, P., and Giaccone, V. (2017). Antimicrobial resistance: a global emerging threat to public health systems. Crit. Rev. Food Sci. Nutr. 57, 2857–2876. doi: 10.1080/10408398.2015.1077192
Florensa, A. F., Kaas, R. S., Clausen, P. T. L. C., Aytan-Aktug, D., and Aarestrup, F. M. (2022). ResFinder – an open online resource for identification of antimicrobial resistance genes in next-generation sequencing data and prediction of phenotypes from genotypes. Microb. Genomics 8:000748. doi: 10.1099/mgen.0.000748
Fontana, C., Cocconcelli, P. S., and Vignolo, G. (2005). Monitoring the bacterial population dynamics during fermentation of artisanal Argentinean sausages. Int. J. Food Microbiol. 103, 131–142. doi: 10.1016/j.ijfoodmicro.2004.11.046
Franz, C., Ludwig, W., Back, W., and Dicks, L. (2006). “The genera Pediococcus and Tetragenococcus” in The prokaryotes. 3rd ed (Berlin: Springer), 229–266.
García-Ruiz, A., González de Llano, D., Esteban-Fernández, A., Requena, T., Bartolomé, B., and Moreno-Arribas, M. V. (2014). Assessment of probiotic properties in lactic acid bacteria isolated from wine. Food Microbiol. 44, 220–225. doi: 10.1016/j.fm.2014.06.015
Ghosh, B., Sukumar, G., and Ghosh, A. R. (2019). Purification and characterization of pediocin from probiotic Pediococcus pentosaceus GS4, MTCC 12683. Folia Microbiol. 64, 765–778. doi: 10.1007/s12223-019-00689-0
Gong, Y., and Qi, X. (2020). A study revealing volatile aroma produced by Pediococcus pentosaceus in dough fermentation. Food Sci. Nutr. 8, 5077–5085. doi: 10.1002/fsn3.1807
Gudiña, E. J., Fernandes, E. C., Teixeira, J. A., and Rodrigues, L. R. (2015). Antimicrobial and anti-adhesive activities of cell-bound biosurfactant from Lactobacillus agilis CCUG31450. RSC Adv. 5, 90960–90968. doi: 10.1039/C5RA11659G
Gupta, S. K., Padmanabhan, B. R., Diene, S. M., Lopez-Rojas, R., Kempf, M., Landraud, L., et al. (2014). ARG-ANNOT, a new bioinformatic tool to discover antibiotic resistance genes in bacterial genomes. Antimicrob. Agents Chemother. 58, 212–220. doi: 10.1128/AAC.01310-13
Halami, P. M., Ramesh, A., and Chandrashekar, A. (2005). Fermenting cucumber, a potential source for the isolation of pediocin-like bacteriocin producers. World J. Microbiol. Biotechnol. 21, 1351–1358. doi: 10.1007/s11274-005-4858-0
Hu, D., Wu, J., Jin, L., Yuan, L., Li, J., Chen, X., et al. (2021). Evaluation of Pediococcus pentosaceus strains as probiotic adjunct cultures for soybean milk post-fermentation. Food Res. Int. 148:110570. doi: 10.1016/j.foodres.2021.110570
Ilavenil, S., Vijayakumar, M., Kim, D. H., Valan Arasu, M., Park, H. S., Ravikumar, S., et al. (2016). Assessment of probiotic, antifungal and cholesterol lowering properties of Pediococcus pentosaceus KCC-23 isolated from Italian ryegrass. J. Sci. Food Agric. 96, 593–601. doi: 10.1002/jsfa.7128
Jang, S., Lee, D., Jang, I. S., Choi, H. S., and Suh, H. J. (2015). The culture of Pediococcus pentosaceus T1 inhibits Listeria proliferation in salmon fillets and controls maturation of kimchi. Food Technol. Biotechnol. 53, 29–37. doi: 10.17113/ftb.53.01.15.3754
Jayashree, S., Jayaraman, K., and Gurumurthy, K. (2010). Isolation, screening and characterization of riboflavin producing lactic acid bacteria from Katpadi, Vellore district. Recent Res. Sci. Technol. 2, 83–88.
Jiang, S., Cai, L., Lv, L., and Li, L. (2021). Pediococcus pentosaceus, a future additive or probiotic candidate. Microb. Cell Factories 20:45. doi: 10.1186/s12934-021-01537-y
Jiang, J., Yang, B., Ross, R. P., Stanton, C., Zhao, J., Zhang, H., et al. (2020). Comparative genomics of Pediococcus pentosaceus isolated from different niches reveals genetic diversity in carbohydrate metabolism and immune system. Front. Microbiol. 11:253. doi: 10.3389/fmicb.2020.00253
Kim, J. S., Kim, J. H., Palaniyandi, S. A., Lee, C. C., You, J. W., Yang, H., et al. (2019). Yak-Kong soybean (glycine max) fermented by a novel Pediococcus pentosaceus inhibits the oxidative stress-induced monocyte-endothelial cell adhesion. Nutrients 11:1380. doi: 10.3390/nu11061380
Ladha, G., and Jeevaratnam, K. (2018). Probiotic potential of Pediococcus pentosaceus LJR1, a bacteriocinogenic strain isolated from rumen liquor of goat (Capra aegagrus hircus). Food Biotechnol. 32, 60–77. doi: 10.1080/08905436.2017.1414700
Laslett, D., and Canback, B. (2004). ARAGORN, a program to detect tRNA genes and tmRNA genes in nucleotide sequences. Nucleic Acids Res. 32, 11–16. doi: 10.1093/nar/gkh152
Lebert, I., Leroy, S., Giammarinaro, P., Lebert, A., Chacornac, J. P., Bover-Cid, S., et al. (2007). Diversity of microorganisms in the environment and dry fermented sausages of small traditional French processing units. Meat Sci. 76, 112–122. doi: 10.1016/j.meatsci.2006.10.019
LeBlanc, J. G., Laiño, J. E., del Valle, M. J., Vannini, V., van Sinderen, D., Taranto, M. P., et al. (2011). B-group vitamin production by lactic acid bacteria - current knowledge and potential applications. J. Appl. Microbiol. 111, 1297–1309. doi: 10.1111/j.1365-2672.2011.05157.x
Leroy, F., Verluyten, J., and De Vuyst, L. (2006). Functional meat starter cultures for improved sausage fermentation. Int. J. Food Microbiol. 106, 270–285. doi: 10.1016/j.ijfoodmicro.2005.06.027
Levit, R., Savoy de Giori, G., Moreno de LeBlanc, A., and LeBlanc, J. G. (2021). Recent update on lactic acid bacteria producing riboflavin and folates: application for food fortification and treatment of intestinal inflammation. J. Appl. Microbiol. 130, 1412–1424. doi: 10.1111/jam.14854
Li, H., Xie, X., Li, Y., Chen, M., Xue, L., Wang, J., et al. (2021). Pediococcus pentosaceus IM96 exerts protective effects against Enterohemorrhagic Escherichia coli O157:H7 infection in vivo. Food Secur. 10:2945. doi: 10.3390/foods10122945
Liu, N., Gagnot, S., Denis, Y., Byrne, D., Faulds, C., Fierobe, H. P., et al. (2022). Selfish uptake versus extracellular arabinoxylan degradation in the primary degrader Ruminiclostridium cellulolyticum, a new string to its bow. Biotechnol. Biofuels Bioprod. 15:127. doi: 10.1186/s13068-022-02225-8
Lv, L. X., Li, Y. D., Hu, X. J., Shi, H. Y., and Li, L. J. (2014). Whole-genome sequence assembly of Pediococcus pentosaceus LI05 (CGMCC 7049) from the human gastrointestinal tract and comparative analysis with representative sequences from three food-borne strains. Gut Pathogens 6:36. doi: 10.1186/s13099-014-0036-y
Mailund, T., Brodal, G. S., Fagerberg, R., Pedersen, C. N. S., and Phillips, D. (2006). Recrafting the neighbor-joining method. BMC Bioinformatics 7:29. doi: 10.1186/1471-2105-7-29
Marçais, G., Delcher, A. L., Phillippy, A. M., Coston, R., Salzberg, S. L., and Zimin, A. (2018). MUMmer4: A fast and versatile genome alignment system. PLOS Comp. Biol. 14:e1005944. doi: 10.1371/journal.pcbi.1005944
Martino, M. E., Maifreni, M., Marino, M., Bartolomeoli, I., Carraro, L., Fasolato, L., et al. (2013). Genotypic and phenotypic diversity of Pediococcus pentosaceus strains isolated from food matrices and characterisation of the penocin operon. Antonie Van Leeuwenhoek 103, 1149–1163. doi: 10.1007/s10482-013-9897-1
McGinnis, S., and Madden, T. L. (2004). BLAST: at the core of a powerful and diverse set of sequence analysis tools. Nucleic Acids Res. 32, W20–W25. doi: 10.1093/nar/gkh435
Midha, S., Ranjan, M., Sharma, V., Kumari, A., Singh, P. K., Korpole, S., et al. (2012). Genome sequence of Pediococcus pentosaceus strain IE-3. J. Bacteriol. 194:4468. doi: 10.1128/JB.00897-12
Miller, K. W., Ray, P., Steinmetz, T., Hanekamp, T., and Ray, B. (2005). Gene organization and sequences of pediocin AcH/PA-1 production operons in Pediococcus and Lactobacillus plasmids. Lett. Appl. Microbiol. 40, 56–62. doi: 10.1111/j.1472-765X.2004.01627.x
Min, B. E., Hwang, H. G., Lim, H. G., and Jung, G. Y. (2017). Optimization of industrial microorganisms: recent advances in synthetic dynamic regulators. J. Ind. Microbiol. Biotechnol. 44, 89–98. doi: 10.1007/s10295-016-1867-y
Montanier, C., Money, V. A., Pires, V. M. R., Flint, J. E., Pinheiro, B. A., Goyal, A., et al. (2009). The active site of a carbohydrate esterase displays divergent catalytic and noncatalytic binding functions. PLoS Biol. 7:e1000071:e71. doi: 10.1371/journal.pbio.1000071
Montemurro, M., Celano, G., de Angelis, M., Gobbetti, M., Rizzello, C. G., and Pontonio, E. (2020). Selection of non-Lactobacillus strains to be used as starters for sourdough fermentation. Food Microbiol. 90:103491. doi: 10.1016/j.fm.2020.103491
Nanasombat, S., Treebavonkusol, P., Kittisrisopit, S., Jaichalad, T., Phunpruch, S., Kootmas, A., et al. (2017). Lactic acid bacteria isolated from raw and fermented pork products: identification and characterization of catalase-producing Pediococcus pentosaceus. Food Sci. Biotechnol. 26, 173–179. doi: 10.1007/s10068-017-0023-4
Nguyen, N. K., Dong, N. T. N., Nguyen, H. T., and Le, P. H. (2015). Lactic acid bacteria: promising supplements for enhancing the biological activities of kombucha. Springer Plus 4:91. doi: 10.1186/s40064-015-0872-3
Noble, P. A., Citek, R. W., and Ogunseitan, O. A. (1998). Tetranucleotide frequencies in microbial genomes. Electrophoresis 19, 528–535. doi: 10.1002/elps.1150190412
Oliveira, F. S., da Silva Rodrigues, R., de Carvalho, A. F., and Nero, L. A. (2023). Genomic analyses of Pediococcus pentosaceus ST65ACC, a bacteriocinogenic strain isolated from artisanal raw-milk cheese. Probiotics Antimicrob. Prot. 15, 630–645. doi: 10.1007/s12602-021-09894-1
Osmanagaoglu, O., Kiran, F., and Ataoglu, H. (2010). Evaluation of in vitro probiotic potential of Pediococcus pentosaceus OZF isolated from human breast Milk. Probiotics Antimicrob. Prot. 2, 162–174. doi: 10.1007/s12602-010-9050-7
Papagianni, M. (2012). Metabolic engineering of lactic acid bacteria for the production of industrially important compounds. Comp. Struct. Biotechnol. J. 3:e201210003. doi: 10.5936/csbj.201210003
Papagianni, M., and Anastasiadou, S. (2009). Pediocins: the bacteriocins of Pediococci. Sources, production, properties and applications. Microb. Cell Factories 8:3. doi: 10.1186/1475-2859-8-3
Parente, E., Grieco, S., and Crudele, M. A. (2001). Phenotypic diversity of lactic acid bacteria isolated from fermented sausages produced in Basilicata (southern Italy). J. Appl. Microbiol. 90, 943–952. doi: 10.1046/j.1365-2672.2001.01328.x
Park, J. T. (2001). Identification of a dedicated recycling pathway for anhydro-N-acetylmuramic acid and N-acetylglucosamine derived from Escherichia coli cell wall murein. J. Bacteriol. 183, 3842–3847. doi: 10.1128/JB.183.13.3842-3847.2001
Patel, R. K., and Jain, M. (2012). NGS QC toolkit: a toolkit for quality control of next generation sequencing data. PLoS One 7:e30619. doi: 10.1371/journal.pone.0030619
Qi, Y., Huang, L., Zeng, Y., Li, W., Zhou, D., Xie, J., et al. (2021). Pediococcus pentosaceus: screening and application as probiotics in food processing. Front. Microbiol. 12:12. doi: 10.3389/fmicb.2021.762467
Rantsiou, K., Drosinos, E. H., Gialitaki, M., Metaxopoulos, I., Comi, G., and Cocolin, L. (2006). Use of molecular tools to characterize Lactobacillus spp. isolated from Greek traditional fermented sausages. I. J. Food Microbiol. 112, 215–222. doi: 10.1016/j.ijfoodmicro.2006.09.001
Richter, M., Rosselló-Móra, R., Oliver Glöckner, F., and Peplies, J. (2016). JSpeciesWS: a web server for prokaryotic species circumscription based on pairwise genome comparison. Bioinformatics 32, 929–931. doi: 10.1093/bioinformatics/btv681
Sanchez, B., Ruiz, L., de los Reyes-Gavilan, C., and Margolles, A. (2008). Proteomics of stress response in Bifidobacterium. Front. Biosci. 13, 6905–6919. doi: 10.2741/3198
Savard, P., Lamarche, B., Paradis, M. E., Thiboutot, H., Laurin, É., and Roy, D. (2011). Impact of Bifidobacterium animalis subsp. lactis BB-12 and, Lactobacillus acidophilus LA-5-containing yoghurt, on fecal bacterial counts of healthy adults. Int. J. Food Microbiol. 149, 50–57. doi: 10.1016/j.ijfoodmicro.2010.12.026
Savedboworn, W., Riansa-ngawong, W., Sinlapacharoen, W., Pajakang, S., Patcharajarukit, B., and Tipkanon, S. (2014). Assessment of probiotic properties in lactic acid bacteria isolated from fermented vegetables. Appl. Sci. Eng. Progress 7, 53–65. doi: 10.14416/j.ijast.2014.10.001
Sellamani, M., Kalagatur, N. K., Siddaiah, C., Mudili, V., Krishna, K., Natarajan, G., et al. (2016). Antifungal and zearalenone inhibitory activity of Pediococcus pentosaceus isolated from dairy products on fusarium graminearum. Front. Microbiol. 7:890. doi: 10.3389/fmicb.2016.00890
Shukla, R., and Goyal, A. (2014). Probiotic potential of Pediococcus pentosaceus CRAG3: a new isolate from fermented cucumber. Probiotics Antimicrob. Prot. 6, 11–21. doi: 10.1007/s12602-013-9149-8
Stothard, P., Grant, J. R., and Van Domselaar, G. (2019). Visualizing and comparing circular genomes using the CGView family of tools. Brief. Bioinform. 20, 1576–1582. doi: 10.1093/bib/bbx081
Sybesma, W., Burgess, C., Starrenburg, M., van Sinderen, D., and Hugenholtz, J. (2004). Multivitamin production in Lactococcus lactis using metabolic engineering. Metab. Eng. 6, 109–115. doi: 10.1016/j.ymben.2003.11.002
Tamura, K., Stecher, G., and Kumar, S. (2021). MEGA11: molecular evolutionary genetics analysis version 11. Mol. Biol. Evol. 38, 3022–3027. doi: 10.1093/molbev/msab120
Tatsaporn, T., and Kornkanok, K. (2020). Using potential lactic acid bacteria biofilms and their compounds to control biofilms of foodborne pathogens. Biotechnol. Rep. (Amst.) 26:e00477. doi: 10.1016/j.btre.2020.e00477
Thirabunyanon, M., and Hongwittayakorn, P. (2013). Potential probiotic lactic acid bacteria of human origin induce antiproliferation of colon cancer cells via synergic actions in adhesion to cancer cells and short-chain fatty acid bioproduction. Appl. Biochem. Biotechnol. 169, 511–525. doi: 10.1007/s12010-012-9995-y
Todhanakasem, T., Triwattana, K., Pom, J., Havanapan, P., Koombhongse, P., and Thitisak, P. (2020). Physiological studies of the Pediococcus pentosaceus biofilm. Lett. Appl. Microbiol. 72:2. doi: 10.1111/lam.13351
Todorov, S. D., and Dicks, L. M. T. (2009). Bacteriocin production by Pediococcus pentosaceus isolated from Marula (Scerocarya birrea). Int. J. Food Microbiol. 132, 117–126. doi: 10.1016/j.ijfoodmicro.2009.04.010
Torres-Miranda, A., Melis-Arcos, F., and Garrido, D. (2022). Characterization and identification of probiotic features in Lacticaseibacillus paracasei using a comparative genomic analysis approach. Probiotics Antimicrob. Prot. 14, 1211–1224. doi: 10.1007/s12602-022-09999-1
van Heel, A. J., de Jong, A., Song, C., Viel, J. H., Kok, J., and Kuipers, O. P. (2018). BAGEL4: a user-friendly web server to thoroughly mine RiPPs and bacteriocins. Nucleic Acids Res. 46, W278–W281. doi: 10.1093/nar/gky383
Vera, C., Guerrero, C., Aburto, C., Cordova, A., and Illanes, A. (2020). Conventional and non-conventional applications of β-galactosidases. Biochim. Biophys. Acta Proteins Prot. 1868:140271. doi: 10.1016/j.bbapap.2019.140271
Vidhyasagar, V., and Jeevaratnam, K. (2013). Evaluation of Pediococcus pentosaceus strains isolated from idly batter for probiotic properties in vitro. J. Funct. Foods 5, 235–243. doi: 10.1016/j.jff.2012.10.012
Vollmer, W. (2008). Structural variation in the glycan strands of bacterial peptidoglycan. FEMS Microbiol. Rev. 32, 287–306. doi: 10.1111/j.1574-6976.2007.00088.x
Wang, Y., Wu, J., Lv, M., Shao, Z., Hungwe, M., Wang, J., et al. (2021). Metabolism characteristics of lactic acid bacteria and the expanding applications in food industry. Front. Bioeng. Biotechnol. 9:612285. doi: 10.3389/fbioe.2021.612285
Wheeler, T. J., and Eddy, S. R. (2013). Nhmmer: DNA homology search with profile HMMs. Bioinformatics 29, 2487–2489. doi: 10.1093/bioinformatics/btt403
Xu, X., Bi, S., Lao, F., Chen, F., Liao, X., and Wu, J. (2021). Comprehensive investigation on volatile and non-volatile metabolites in broccoli juices fermented by animal- and plant-derived Pediococcus pentosaceus. Food Chem. 341:128118. doi: 10.1016/j.foodchem.2020.128118
Yin, Y., Mao, X., Yang, J., Chen, X., Mao, F., and Xu, Y. (2012). dbCAN: a web resource for automated carbohydrate-active enzyme annotation. Nucleic Acids Res. 40, W445–W451. doi: 10.1093/nar/gks479
Yoon, S. H., Ha, S. M., Kwon, S., Lim, J., Kim, Y., Seo, H., et al. (2017a). Introducing EzBioCloud: a taxonomically united database of 16S rRNA gene sequences and whole-genome assemblies. Int. J. Syst. Evol. Microbiol. 67, 1613–1617. doi: 10.1099/ijsem.0.001755
Yoon, S. H., Ha, S., Lim, J., Kwon, S., and Chun, J. (2017b). A large-scale evaluation of algorithms to calculate average nucleotide identity. Antonie Van Leeuwenhoek 110, 1281–1286. doi: 10.1007/s10482-017-0844-4
Zerbino, D. R. (2010). Using the Velvet de novo assembler for short-read sequencing technologies. Curr. Protoc. Bioinformatics 31:5. doi: 10.1002/0471250953.bi1105s31
Zhao, X., Higashikawa, F., Noda, M., Kawamura, Y., Matoba, Y., Kumagai, T., et al. (2012). The obesity and fatty liver are reduced by plant-derived Pediococcus pentosaceus LP28 in high fat diet-induced obese mice. PLoS One 7:e30696. doi: 10.1371/journal.pone.0030696
Zommiti, M., Bouffartigues, E., Maillot, O., Barreau, M., Szunerits, S., Sebei, K., et al. (2018). In vitro assessment of the probiotic properties and Bacteriocinogenic potential of Pediococcus pentosaceus MZF16 isolated from artisanal Tunisian meat “dried Ossban”. Front. Microbiol. 9:2607. doi: 10.3389/fmicb.2018.02607
Keywords: Pediococcus pentosaceus, probiotics, whole genome sequencing, antimicrobial resistance, bacteriocins
Citation: Tathode MS, Bonomo MG, Zappavigna S, Mang SM, Bocchetti M, Camele I, Caraglia M and Salzano G (2024) Whole-genome analysis suggesting probiotic potential and safety properties of Pediococcus pentosaceus DSPZPP1, a promising LAB strain isolated from traditional fermented sausages of the Basilicata region (Southern Italy). Front. Microbiol. 15:1268216. doi: 10.3389/fmicb.2024.1268216
Edited by:
Arun K. Bhunia, Purdue University, United StatesReviewed by:
Gabriela N. Tenea, Universidad Técnica del Norte, EcuadorPierre Marie Kaktcham, University of Dschang, Cameroon
Copyright © 2024 Tathode, Bonomo, Zappavigna, Mang, Bocchetti, Camele, Caraglia and Salzano. This is an open-access article distributed under the terms of the Creative Commons Attribution License (CC BY). The use, distribution or reproduction in other forums is permitted, provided the original author(s) and the copyright owner(s) are credited and that the original publication in this journal is cited, in accordance with accepted academic practice. No use, distribution or reproduction is permitted which does not comply with these terms.
*Correspondence: Maria Grazia Bonomo, mariagrazia.bonomo@unibas.it
†These authors have contributed equally to this work and share first authorship