- 1Medical Research and Development, River Engineering, Noida, India
- 2Department of Physics, Faculty of Science, King Khalid University, Abha, Saudi Arabia
- 3Department of Home Economics, Faculty of Science and Arts in Tihama, King Khalid University, Muhayil, Saudi Arabia
- 4ITCS, Karnal, Haryana, India
- 5Hemchandracharya North Gujarat University, Patan, Gujarat, India
- 6Department of Clinical Laboratory Sciences, Faculty of Applied Medical Sciences, University of Umm Al-Qura University, Makkah, Saudi Arabia
Gut microbiota dysbiosis has been a serious risk factor for several gastric and systemic diseases. Recently, gut microbiota’s role in aging was discussed. Available preclinical evidence suggests that the probiotic bacteria Lactiplantibacillus plantarums (LP) may influence the aging process via modulation of the gut microbiota. The present review summarized compelling evidence of LP’s potential effect on aging hallmarks such as oxidative stress, inflammation, DNA methylation, and mitochondrial dysfunction. LP gavage modulates gut microbiota and improves overall endurance in aging animal models. LP cell constituents exert considerable antioxidant potential which may reduce ROS levels directly. In addition, restored gut microbiota facilitate a healthy intestinal milieu and accelerate multi-channel communication via signaling factors such as SCFA and GABA. Signaling factors further activate specific transcription factor Nrf2 in order to reduce oxidative damage. Nrf2 regulates cellular defense systems involving anti-inflammatory cytokines, MMPs, and protective enzymes against MAPKs. We concluded that LP supplementation may be an effective approach to managing aging and associated health risks.
1 Introduction
The human gut microbiota (GM) plays a crucial role in multiple physiological activities, so a healthy composition of GM has been a widely discussed subject for overall health. Factors such as diet, environment, and inappropriate medication (antibiotics and xenobiotics) may alter the intrinsic composition of gut microbiota and induce microbial dysbiosis-associated health anomalies including arthritis, atherosclerosis, cirrhosis, intestinal cancer, hypertension, and diabetes (Li et al., 2017; Gupta et al., 2022). Growing evidence indicates gut microbiome dysbiosis may influence aging and associated health complications. Hitherto, little is understood about the significance of human GM in the complex aging process (Maynard and Weinkove, 2018; Ragonnaud and Biragyn, 2021; Ghosh et al., 2022). Aging is an inevitable, multifactorial complex process that influences almost every organ and tissue (Campisi et al., 2019; Schumacher et al., 2021). GM’s role in aging may be a helpful approach to influencing and understanding the other dimensions of the aging process. Microbial therapeutic interventions such as probiotics have been the most promising agents in restoring those guts-friendly bacteria, in cases of repeated infectious diarrheas and irritable bowel syndrome; in recent years, the use of probiotics has been widened to many other health conditions such as lowering debilitating side effects of radio and chemotherapy. The concept of ‘bugs to drugs’ seems relevant as the use of probiotics extends (O’Hara and Shanahan, 2007; Vivarelli et al., 2019; Rahman et al., 2022).
The identification or development of new probiotics has been an alluring arena of microbiology. Members of Lactobacillaceae have been important probiotic agents (Azad et al., 2018). Probiotics associated with several actions of mechanism, such as the host’s innate and adaptive immune modulation, considerable anti-inflammatory effects by TLR4/TLR2/MyD88/NFKB signaling, decreased gut permeability, and alterations in inflammasome, have been proposed by researchers (Van Zyl et al., 2020). Lactobacilli species are known to produce proteins p40 and p75 in the host, which showed immunomodulation; they trans-activated the epidermal growth factor receptor (EGFR) in intestinal epithelial cells of the host, and antimicrobial factors such as Aggregation-promoting factor (APF) and Bacteriocins decreased pathogens such as Salmonella typhimurium, Clostridium sporogenes, and Enterococcus faecalis colonization in host (García-Peña et al., 2017; Du et al., 2021). In particular, Lactobacillus plantarum (LP) or Lactiplantibacillus plantarum (updated name) has been a well-studied probiotic model subjected to multiple health effects such as anti-microbial, anti-cancerous, and anti-inflammatory. LP supplementation associated with positive outcomes is observed in bowel disease, atopic dermatitis, intestinal infections, and maternal and neonatal hematological issues. LP is one of the most plausible lactic acid bacterium species found in diverse ecological conditions (Seddik et al., 2017; Zhou et al., 2021; Jeong et al., 2023; OjiNjideka Hemphill et al., 2023). LP’s effect on aging is in the initial stage (Lin et al., 2021; Ding et al., 2022; Kumar et al., 2022). Available studies showed LP may influence longevity and healthy aging via enhancing gut and host integrity. However, the role of LP supplementation in aging and associated health conditions is scarcely understood so far (Biagi et al., 2012). This review discusses the significance of LP supplementation in managing signs of aging-associated health complications.
2 Materials and method (searching strategies)
The present review is comprised of recent relevant articles including pre-clinical and clinical studies. Searching strategies mainly include online research databases such as Google Scholar, PubMed, and Science Direct. Overall, 17,209 results were obtained when specific filters such as time period (2015 to 2023) and keywords (‘Probiotic Lactobacillus plantarum’, ‘Lactobacillus plantarum and aging’, ‘Lactobacillus plantarum effect on aging’, ‘Lactobacillus plantarum medicinal uses’) were applied. More specific keywords were also recruited to fill the potential gap. Figures and illustrative works were original and designed according to compiled research outcomes, and in case of retrieval, significant modifications were employed. For instance, some vector images were retrieved from Pixabay under the no attribution required category and modified completely (99%) using tools such as Adobe Photoshop and Microsoft PowerPoint. Articles selection and shortlisting criteria were based on the significant impact factors and citations of relevant publications (Figure 1). A total of 645 relevant articles were selected initially. Duplicate and irrelevant articles were removed. Finally, 112 articles were incorporated in the present review.
3 Gut microbiota, microbial dysbiosis, and aging
Humans have been reviewed as ‘metaorganisms’ due to their integral symbiotic association with the intestinal microbiota (Dam et al., 2019). Noticeably, most diseases might be linked to gut microbiota dysbiosis (Langille et al., 2014). Evidence of aging and microbiome association is also growing (Lustgarten, 2016; Wei et al., 2022). Perturbed GM composition could be responsible for several geriatric degenerative diseases such as Parkinson’s disease, Alzheimer’s disease, sarcopenia, and physical frailty (Figure 2; Lakshminarayanan et al., 2014; Nadeem et al., 2015; Mossad et al., 2021). GM’s role in the aging process could be important in minimizing the risk of aging-associated ailments. However, the diversity and specificity of the human gut microbiome have been influenced by multiple intrinsic and extrinsic factors. A cohort analysis showed that gut microbiome biodiversity is positively associated with age in young adults in the United Kingdom, the United States, and Colombia (Heintz and Mair, 2014). It was observed that 16S rRNA gene sequencing of the infant to elderly macaques microbiome indicates microbiome composition variation and connectivity responsible for age-dependent network changes and altered metabolic functions of amino acids, carbohydrates, lipids, and phenotypes in the microbial community (De la Cuesta-Zuluaga et al., 2019). Moreover, abnormal DNA methylation, characterized by gradual genome demethylation and hypermethylation was observed during the aging process. Usually, DNA methylation is an inevitable normal process that occurs throughout the lifetime. However, why changes occur in DNA methylation is not explained in detail. DNA methylation is susceptible to external factors such as environment, smoking, and lifestyle. So, DNA methylation biomarkers may predict tissue’s biological age across the human lifespan, including development (Jung and Pfeifer, 2015; Zampieri et al., 2015; Salameh et al., 2020). GM’s role in DNA methylation is insufficiently understood. Recent studies show that DNA methylation is significantly associated with GM composition and intestinal homeostasis. In addition, GM may also induce histone modification and the immune system (Jones et al., 2015; Ye et al., 2017; Ansari et al., 2020). Microbiota modulation approaches such as fecal microbiota transplantation (FMT) have been helpful in the recovery of abnormal hypomethylation (Ramos-Molina et al., 2019). Probiotic LP’s modulating role in methylation has been studied (Spath et al., 2012; Zhang B. et al., 2023), so probiotic bacteria such as LP may be a useful agent to modulate microbial dysbiosis and associated aging signs.
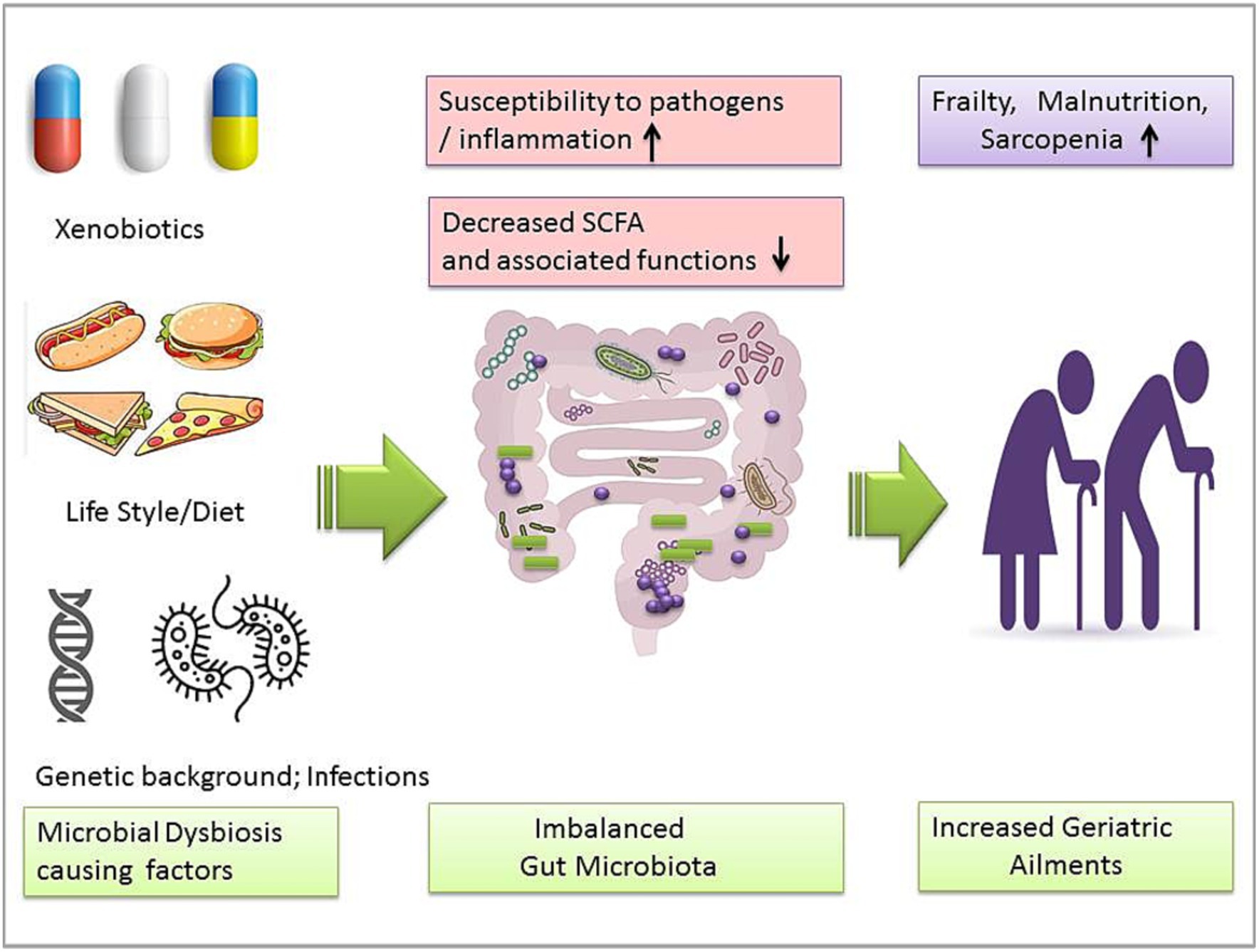
Figure 2. Gut microbiota dysbiosis-causing factors and imbalanced microbiota-associated geriatric ailments.
The evidence of probiotics in aging is growing. Usually, lower animals such as invertebrate Caenorhabditis elegans are used as aging models to observe potential bacterial intervention (Derrien et al., 2019; Teame et al., 2020). Probiotic bacteria may influence some drug’s efficacies. For instance, metformin is the most prescribed anti-hyperglycemic drug that acts as a potential pro-longevity molecule due to its key target being adenosine mono-phosphate-activated protein kinase (AMPK). However, feeding metformin to C. elegans increases significant lifespan only in the presence of bacteria. Interestingly, Metformin showed no effect on worms when cultured without bacteria or killed bacteria (Cabreiro et al., 2013; Zhang A. et al., 2023).
4 Lactiplantibacillus plantarum effect on aging hallmarks
Management of aging and associated health burdens has been challenging and complex. In modern medical research, great success has been observed in lowering mortality; consequently, the lifespan of humans has been extended remarkably as the aged population grows rapidly in the modern world (Cong et al., 2021). Aging is a complex, natural process influenced by multiple factors including oxidative stress, inflammation, environmental factors (UV, pollution, and geography), lifestyle, genetics, and epigenetics factors (Rodríguez-Rodero et al., 2011; Trist et al., 2019; Welker et al., 2020).
By managing those factors, the aging process can be improved. For instance, one of the age-promoting factors of DNA methylation, which is susceptible to bioactive nutrients, probiotic supplementation, and gut microbiota composition, may affect the DNA methylation (Halloran and Underwood, 2019; Vähämiko et al., 2019; Allison et al., 2021).
Probiotic bacterial intervention in aging in the early stage is limited to a few preclinical studies. In particular, Lactobacillus spp. showed promising effects on the aging model (Ni et al., 2019; Vaiserman et al., 2020). Compounds derived from microbes such as melleolide and microbial invertase may confer rejuvenating and positive effects on several types of aging cells (Gupta et al., 2019; Salekeen et al., 2021). A recent experiment suggests that gut-microbes-associated compounds such as indole-3-propionic acid, dihydropteroate, phenyllactic acid, phenylpyruvic acid, all-trans-retinoic acid, and multiple deoxy-, methyl-, and cyclic nucleotides from gut microbiota are the considerable regulators of NAD+ metabolism and could be the indirect markers for aging-associated degeneration in humans (Rabe et al., 2006; Chen et al., 2023). Fermented soya food items and L-ergothioneine may also provide a series of beneficial antioxidant effects to minimize aging-associated damage (Das et al., 2020). A perturbing consortium of gut microbiota (due to environmental factors, diet, and long-term antibiotics) may weaken the host barrier against pathogens and consequently decrease SCFA production and cause frailty, diabetes, malnutrition, and sarcopenia in the elderly (Nakaya et al., 2014). Probiotics such as LP may help to modulate altered gut microbiota and associated aging hallmarks. Studies suggest that gut microbiota dysbiosis is significantly associated with aging. A recent experimental study in China indicated that a pathogenic alteration in intestinal microbiota composition could decrease telomerase mRNA in mice, while gut microbiota modulation may improve the signs of the aging model (Shenghua et al., 2019). The gut microbiota is one of the most discussed aspects of aging. Altered or disarranged gut microbiota increases age-related disorders, while a healthy composition contributes to longevity and minimization of age-related diseases (Vaiserman et al., 2017; Cӑtoi et al., 2020; Mossad et al., 2022). In addition, the role of organ-specific microbiota could be associated with aging. For instance, skin microbiota composition significantly changes according to age (Li et al., 2020; Vaiserman et al., 2020).
LP’s role in the gut microbiota-associated aging process showed promising antioxidant potential against aging models (Das and Goyal, 2015; Luan et al., 2021). LP can modulate perturbed microbiota and stimulate SCFA levels to build gut microbiota organ-specific crosstalk, which further allows the transmission/activation of activated p38 mitogen-activated protein kinase (MAPKs) and other factors to minimize aging hallmarks such as oxidative stress and inflammation in aging models (Zhang et al., 2019; Lin et al., 2021; Lee et al., 2021a,b).
LP has shown significant antioxidant potential in the D-galactose-induced aging mice model. LP gavage can increase antioxidant activities; reduce malondialdehyde, alanine aminotransferase, aspartate aminotransferase, blood urea, and muscle glycogen levels; and increase overall endurance in mice (Cabreiro et al., 2013; Lee et al., 2021a,b). LP-based probiotic supplementations may influence immune-related genes and toll-like receptor 4 expression. Particularly, Lactiplantibacillus plantarum WCFS1 and Lactobacillus casei BL23 increased regulatory T-cell frequencies in mesenteric lymph nodes and specific antibody production to support T cell-dependent immune response, which prevents age-related decline in the intestinal mucus of mice (Chen et al., 2017).
Oxidative stress is one of the most intrinsic factors associated with aging (Tan et al., 2018), and its corollary reactive oxygen species (ROS) can induce the overall aging process including skin aging. Esthetically, the skin is the outermost and largest organ of the human body showing signs of aging directly (Figure 3). Comparatively, the skin aging process may also be accelerated by extrinsic factors such as ultraviolet radiation. Such long-term sun exposure and UV radiation are associated with skin aging known as photo-aging. People with lighter skin are the most sensitive to photo-aging (Sun et al., 2021). UV light exposure may provoke the production of ROS and accelerate photo-aging. ROS hyperactivation causes a signaling pathway that activates matrix metalloproteinases (MMPs) and reduces collagen production. Low collagen level promotes the degrading of connective tissues. On the other hand, induced autophagy and mitochondrial ROS may be able to contribute to aging (Lee et al., 2021a,b; Kumar et al., 2022). Also, a master regulator of redox homeostasis Nrf2 deficiency exacerbates age-related mitochondrial oxidative stress (Chen et al., 2016). A healthy composition of gut microbiota may influence ROS hyperactivation. An oncological study suggests gut microbiota may modulate ROS generation in tumor cells (Kim I. S. et al., 2019). Probiotic intervention has been a promising approach to modulating gut microbiota. Studies show the probiotic antioxidant effect can check ROS and maintain gut homeostasis (Liguori et al., 2018; Yu et al., 2022). In particular, probiotic bacteria LP cell wall constituents such as lipoteichoic and exopolysaccharides can regulate MMP1, MMP2, MMP3, MMP9, and MMP10 expression, decrease the high level of reactive oxygen species, and show promising anti-collagenase and antioxidant activities (Figure 4) (Zhang et al., 2013; Hong et al., 2015; Raza et al., 2017; Shirzad et al., 2018; Kitaoka et al., 2019; Gu et al., 2020; Warraich et al., 2020; Kang et al., 2021; Singh et al., 2022). Therefore, LP supplementation may decrease free radicals and oxidative stress and control the expression levels of TGF-β-related transcription factors in human dermal fibroblasts (Sun et al., 2021). LP also regulates the tight junction in human intestinal epithelial cells and is able to retain moisture in human dermal fibroblast cells by serving as a functional substance in skin-gut axis communication (Lee et al., 2021a,b).
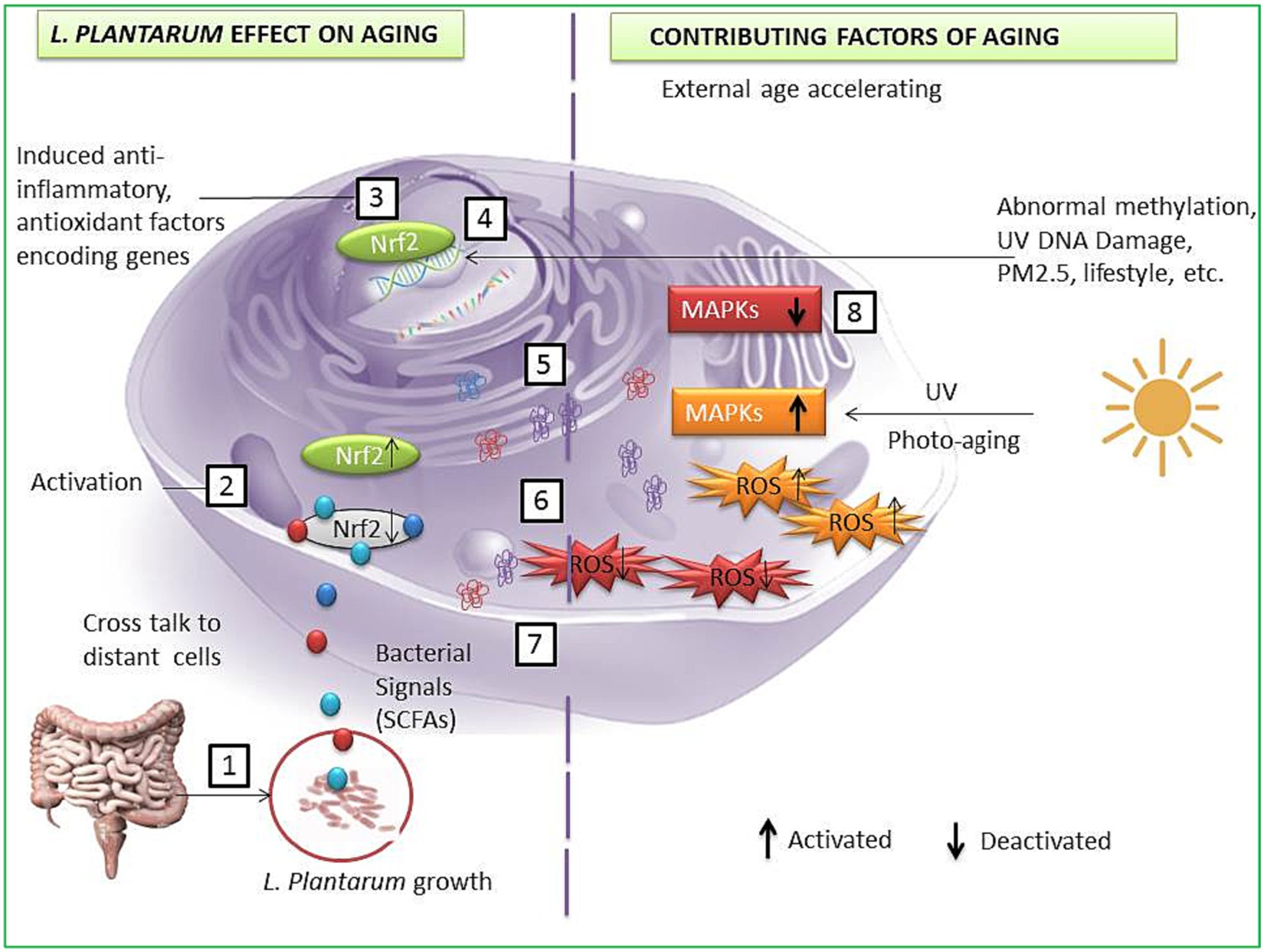
Figure 3. A possible anti-aging pathway induced by L. plantarum strains. LP interacts with gut cells and induces signals to other cells such as SCFAs (1); bacterial and host cells crosstalk lead to Nrf2 activation in host cell (2); activated Nrf2 enters the nucleus and activates specific genes to encode enzymes and protein to protect cells from aging factors (3); transcription and DNA repair (4); translated specific anti-inflammatory, antioxidant protein, and oxidative stress-reducing enzymes (5); translocation of cell-protecting proteins and enzymes (6); reduction of ROS (7); deactivation of the MAPKs and other photo-aging and DNA damaging factors (8).
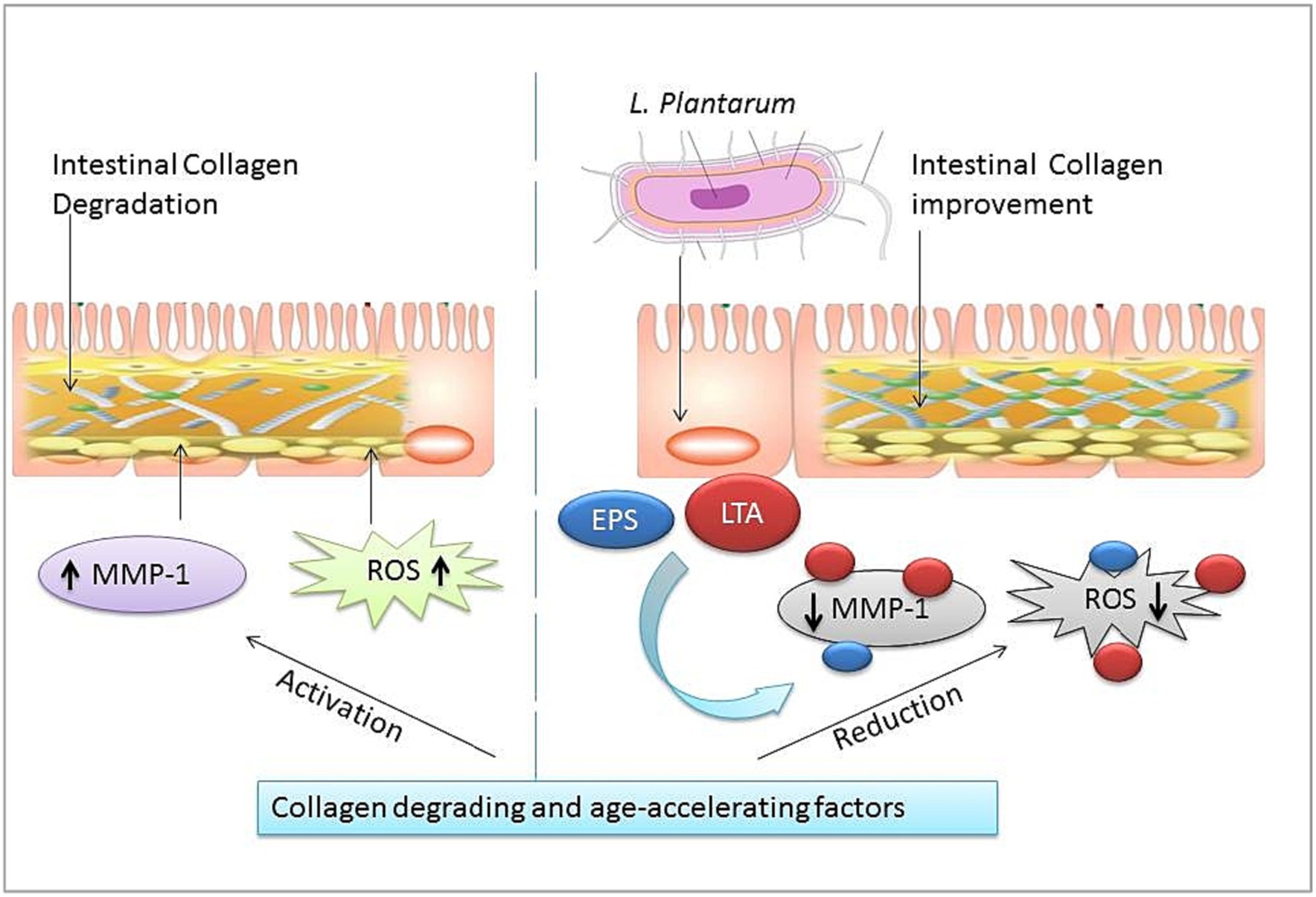
Figure 4. Intestinal collagen and oxidation stress-reducing effect of L. plantarum (LP). The right half shows age-promoting factors of matrix metalloproteinases (MMP-1) and reactive oxygen species (ROS). The left half shows LP cell wall constituents: lipoteichoic acid (LTA), exopolysaccharides (EPS) oxidation damage, and collagen degradation reducing effect.
5 Lactiplantibacillus plantarum associated potential age-ameliorating pathway.
According to the available evidence, LP strains may play an important role in the aging process via gut microbiota modulation, Table 1. LP may help to maintain healthy gut microbiota which maintains intestinal homeostasis by regulating the SCFA and GABA levels (Ni et al., 2019). SCFAs are bacterial signals, crucial to maintain numerous physiological functions. Induced SCFA levels facilitate gut-organ crosstalk, which helps to relocate various essential factors to distant host cells, in order to control cell-damaging factors such as inflammatory responses (Nogal et al., 2021). SCFAs facilitate redox signaling between host and bacterium for normal physiology of the cells (Figure 5).
SCFAs may also activate the nuclear factor erythroid 2-related factor 2 (Nrf2) production (González-Bosch et al., 2021). Nrf2 is known to trigger a significant antioxidant pathway in cells. Usually, Nrf2 remains inactive in normal physiologic conditions, while in the activated form, it can enter into the nucleus from the cytoplasm and further activate the antioxidant response element (ARE) to prevent oxidative stress in the host cell. LP supplementation can activate nuclear factor erythroid 2-related factor 2 (Nrf2) in the various types of cells like the skin, liver, and spleen of aging mice (Zhou et al., 2021). Activated Nrf2 may regulate hundreds of genes encoding proteins with anti-inflammatory, antioxidant, drug-metabolizing, and other homeostatic aspects (Figure 2; Liu et al., 2019; Dinkova-Kostova and Copple, 2023). We have discussed that excess accumulation of reactive oxygen species (ROS) is also associated with age-related degenerative disorders such as neurological ailments, chronic sarcopenia, and frailty (Zhang et al., 2017; Hong et al., 2021). LP effect on aging mainly involves the regulation of reactive oxygen species (ROS), stimulation of anti-inflammatory cytokines, and DNA repairing by activating Nrf2 (Tiwari and Wilson, 2019). LP cell wall constituents such as polysaccharides (EPS) are usually responsible for recovering the altered gut microbiota and conferring anti-oxidative effect in aging cells by stimulating enzymes like glutathione peroxidase, superoxide dismutase, and catalase and improving signs of premature aging such as collagen elastin, skin diseases such as atopic dermatitis, and metabolic disorders such as obesity (Zhang et al., 2017; Kim H. R. et al., 2019; Ge et al., 2021; Fu et al., 2022; Yu et al., 2022). Overall, the LP effect against the aging model is positive. LP supplementation can reduce important aging hallmarks such as oxidative stress, inflammation, and mitochondrial dysfunctions via gut microbiota modulation (Figure 2). Although most available research is preclinical, more extensive studies are suggested to explore the possibilities of LP supplementation in aging-associated risk management.
6 Conclusion
Human gut microbiota modulation by probiotic LP may influence several aging hallmarks. LP has shown significant antioxidant effects, which prevent oxidative damage in aging cells. Compelling outcomes from available preclinical research suggest that LP strains may induce gut-microbiota-associated signaling such as SCFA and GABA and activate inducible transcription factor Nrf2. Nrf2 induces a cell-intrinsic defense system by regulating multiple specific genes resulting in the production of anti-inflammatory cytokines and protective/antioxidant enzymes (against MAPKs) and reducing ROS. This review suggests LP supplementation may help to manage some aging hallmarks. Yet, significant studies are required to explore LP possibilities to manage the complex aging process.
Author contributions
NG: Conceptualization, Methodology, Visualization, Writing – original draft. NE-G: Funding acquisition, Writing – review & editing. LM: Validation, Funding acquisition, Writing – review & editing. HG: Investigation, Software, Writing – review & editing. VY: Supervision, Writing – review & editing. SA: Funding acquisition, Writing – review & editing. NQ: Funding acquisition, Resources.
Funding
The current study was supported financially by the Dean of Science and Research at King Khalid University via the large Group Project under grant no. RGP.2/456/44.
Acknowledgments
The authors extend their appreciation to the Deanship of Scientific Research at King Khalid University for funding this work through the large Group Project under grant number RGP.2/456/44. The authors are also thankful to the R&D of River Engineering Pvt. Ltd. for providing support for this article.
Conflict of interest
The authors declare that the research was conducted in the absence of any commercial or financial relationships that could be construed as a potential conflict of interest.
Publisher’s note
All claims expressed in this article are solely those of the authors and do not necessarily represent those of their affiliated organizations, or those of the publisher, the editors and the reviewers. Any product that may be evaluated in this article, or claim that may be made by its manufacturer, is not guaranteed or endorsed by the publisher.
References
Allison, J., Kaliszewska, A., Uceda, S., Reiriz, M., and Arias, N. (2021). Targeting DNA methylation in the adult brain through diet. Nutrients 13:3979. doi: 10.3390/nu13113979
Ansari, I., Raddatz, G., Gutekunst, J., Ridnik, M., Cohen, D., Abu-Remaileh, M., et al. (2020). The microbiota programs DNA methylation to control intestinal homeostasis and inflammation. Nat. Microbiol. 5, 610–619. doi: 10.1038/s41564-019-0659-3
Azad, M. A. K., Sarker, M., Li, T., and Yin, J. (2018). Probiotic species in the modulation of gut Micro-biota: An overview. Biomed. Res. Int. 2018:9478630. doi: 10.1155/2018/9478630
Biagi, E., Candela, M., Fairweather-Tait, S., Franceschi, C., and Brigidi, P. (2012). Aging of the human metaorganism: the microbial counterpart. Age (Dordr.) 34, 247–267. doi: 10.1007/s11357-011-9217-5
Cabreiro, F., Au, C., Leung, K. Y., Vergara-Irigaray, N., Cochemé, H. M., Noori, T., et al. (2013). Metformin retards aging in C. elegans by altering micro-bial folate and methionine metabolism. Cell 153, 228–239. doi: 10.1016/j.cell.2013.02.035
Campisi, J., Kapahi, P., Lithgow, G. J., Melov, S., Newman, J. C., and Verdin, E. (2019). From discoveries in ageing research to therapeutics for healthy ageing. Nature 571, 183–192. doi: 10.1038/s41586-019-1365-2
Cӑtoi, A. F., Corina, A., Katsiki, N., Vodnar, D. C., Andreicuț, A. D., Stoian, A. P., et al. (2020). (2020) gut microbiota and aging-a focus on centenarians. Biochim. Biophys. Acta Mol. basis Dis. 1866:165765. doi: 10.1016/j.bbadis.2020.165765
Chen, L., Liu, R., Li, S., Wu, M., Yu, H., and Ge, Q. (2023). Metabolism of hydrogen peroxide by Lacti-plantibacillus plantarum NJAU-01: a proteomics study. Food Microbiol. 112:104246. doi: 10.1016/j.fm.2023.104246
Chen, Q., Liu, C., Zhang, Y., Wang, S., and Li, F. (2022). Effect of Lactiplantibacillus plantarum KSFY01 on the exercise capacity of D-galactose-induced oxidative stress-aged mice. Front. Microbiol. 13:1030833. doi: 10.3389/fmicb.2022.1030833
Chen, J., Ou, Y., Li, Y., Hu, S., Shao, L. W., and Liu, Y. (2017). Metformin extends C. elegans lifespan through lysosomal pathway. elife 6:e31268. doi: 10.7554/eLife.31268
Chen, Y. M., Wei, L., Chiu, Y. S., Hsu, Y. J., Tsai, T. Y., Wang, M. F., et al. (2016). Lactiplantibacillus plantarum TWK10 Supplemen-tation improves exercise performance and increases muscle mass in mice. Nutrients 8:205. doi: 10.3390/nu8040205
Cong, P., Wang, J., Luo, W., and Zhang, Y. (2021). Effects of aging on the properties of SBS modified asphalt binders containing anti-aging agents. Constr. Build. Mater. 302:124413. doi: 10.1016/j.conbuildmat.2021.124413
Dam, B., Misra, A., and Banerjee, S. (2019). Role of gut microbiota in combating oxidative stress. Oxid. Stress Microb. Dis. 2019, 43–82. doi: 10.1007/978-981-13-8763-0_4
Das, D., and Goyal, A. (2015). Antioxidant activity and γ-aminobutyric acid (GABA) producing ability of probiotic Lactiplantibacillus plantarum DM5 isolated from Marcha of Sikkim. Food Sci. Technol. 61, 263–268. doi: 10.1016/j.lwt.2014.11.013
Das, G., Paramithiotis, S., Sundaram Sivamaruthi, B., Wijaya, C. H., Suharta, S., Sanlier, N., et al. (2020). Traditional fer-mented foods with anti-aging effect: a concentric review. Food Res. Int. 134:109269. doi: 10.1016/j.foodres.2020.109269
De la Cuesta-Zuluaga, J., Kelley, S. T., Chen, Y., Escobar, J. S., Mueller, N. T., Ley, R. E., et al. (2019). Age-and sex-dependent patterns of gut microbial diversity in human adults. mSystems 4, e00261–e00219. doi: 10.1128/mSystems.00261-19
Derrien, M., Alvarez, A. S., and De Vos, W. M. (2019). The gut microbiota in the first decade of life. Trends Microbiol. 27, 997–1010. doi: 10.1016/j.tim.2019.08.001
Ding, Q., Cao, F., Lai, S., Zhuge, H., Chang, K., Valencak, T. G., et al. (2022). Lactiplanti-bacillus plantarum ZY08 relieves chronic alcohol-induced hepatic steatosis and liver injury in mice via restoring intestinal flora homeostasis. Food Res. Int. 157:111259. doi: 10.1016/j.foodres.2022.111259
Dinkova-Kostova, A. T., and Copple, I. M. (2023). Advances and challenges in therapeutic targeting of NRF2. Trends Pharmacol. Sci. 44, 137–149. doi: 10.1016/j.tips.2022.12.003
Du, Y., Gao, Y., Zeng, B., Fan, X., Yang, D., and Yang, M. (2021). Effects of anti-aging interventions on intestinal microbiota. Gut Microbes 13:1994835. doi: 10.1080/19490976.2021.1994835
Fu, H., Zhang, Y., An, Q., Wang, D., You, S., Zhao, D., et al. (2022). Anti-Photoaging effect of Rhodiola rosea Fer-mented by Lactiplantibacillus plantarum on UVA-damaged fibroblasts. Nutrients 14:2324. doi: 10.3390/nu14112324
Gan, Y., Chen, X., Yi, R., and Zhao, X. (2021). Antioxidative and anti-inflammatory effects of Lacti-plantibacillus plantarum ZS62 on alcohol-induced subacute hepatic damage. Oxidative Med. Cell. Longev. 2021:7337988. doi: 10.1155/2021/7337988
García-Peña, C., Álvarez-Cisneros, T., Quiroz-Baez, R., and Friedland, R. P. (2017). Microbiota and aging. A review and commentary. Arch. Med. Res. 48, 681–689. doi: 10.1016/j.arcmed.2017.11.005
Ge, Q., Yang, B., Liu, R., Jiang, D., Yu, H., Wu, M., et al. (2021). Antioxidant activity of Lacti-plantibacillus plantarum NJAU-01 in an animal model of aging. BMC Microbiol. 21:182. doi: 10.1186/s12866-021-02248-5
Ghosh, T. S., Shanahan, F., and O'Toole, P. W. (2022). The gut microbiome as a modulator of healthy ageing. Nat. Rev. Gastroenterol. Hepatol. 19, 565–584. doi: 10.1038/s41575-022-00605-x
González-Bosch, C., Boorman, E., Zunszain, P. A., and Mann, G. E. (2021). Short-chain fatty acids as modulators of redox signaling in health and disease. Redox Biol. 47:102165. doi: 10.1016/j.redox.2021.102165
Gu, Y., Han, J., Jiang, C., and Zhang, Y. (2020). Biomarkers, oxidative stress and autophagy in skin aging. Ageing Res. Rev. 59:101036. doi: 10.1016/j.arr.2020.101036
Gupta, P. L., Rajput, M., Oza, T., Trivedi, U., and Sanghvi, G. (2019). Eminence of microbial products in cosmetic industry. Nat. Prod. Bioprospect. 9, 267–278. doi: 10.1007/s13659-019-0215-0
Gupta, N., Yadav, V. K., Gacem, A., Al-Dossari, M., Yadav, K. K., Abd El-Gawaad, N. S., et al. (2022). Deleterious effect of air pollution on human microbial community and bacterial Flora: a short review. Int. J. Environ. Res. Public Health 19:15494. doi: 10.3390/ijerph192315494
Halloran, K., and Underwood, M. A. (2019). Probiotic mechanisms of action. Early Hum. Dev. 135, 58–65. doi: 10.1016/j.earlhumdev.2019.05.010
Heintz, C., and Mair, W. (2014). You are what you host: microbiome modulation of the aging pro-cess. Cell 156, 408–411. doi: 10.1016/j.cell.2014.01.025
Hong, Y. F., Hy, L., Jung, B. J., Jang, S., Chung, D. K., and Kim, H. (2015). Lipoteichoic acid isolated from Lactiplantibacillus plantarum down-regulates UV-induced MMP-1 expression and up-regulates type I procollagen through the inhibition of reactive oxygen species generation. Mol. Immunol. 67, 248–255. doi: 10.1016/j.molimm.2015.05.019
Hong, S. M., Kang, M. C., Jin, M., Lee, T. H., Lim, B. O., and Kim, S. Y. (2021). Fermented blueberry and black rice containing Lactiplanti-bacillus plantarum MG 4221: a novel functional food for particulate matter (PM2.5)/dinitrochlorobenzene (DNCB)-induced atopic dermatitis. Food Funct. 12, 3611–3623. doi: 10.1039/d0fo02966a
Jeong, S., Kim, Y., Park, S., Lee, D., Lee, J., Hlaing, S. P., et al. (2023). Lactiplanti-bacillus plantarum metabolites elicit anticancer effects by inhibiting autophagy-related re-sponses. Molecules 28:1890. doi: 10.3390/molecules28041890
Jones, M. J., Goodman, S. J., and Kobor, M. S. (2015). DNA methylation and healthy human aging. Aging Cell 14, 924–932. doi: 10.1111/acel.12349
Jung, M., and Pfeifer, G. P. (2015). Aging and DNA methylation. BMC Biol. 13:7. doi: 10.1186/s12915-015-0118-4
Kang, X., Gao, Z., Zheng, L., Zhang, X., and Li, H. (2021). Regulation of Lactiplantibacillus plantarum on the reactive oxygen species related metabolisms of Saccharomyces cerevisiae. LWT 147:111492. doi: 10.1016/j.lwt.2021.111492
Kim, H. R., Jeong, D. H., Kim, S., Lee, S. W., Sin, H. S., Yu, K. Y., et al. (2019). Fermentation of blackberry with L. plantarum JBMI F5 enhance the protection effect on UVB-mediated Photoaging in human foreskin fibroblast and hairless mice through regulation of MAPK/NF-κB signaling. Nutrients 11:2429. doi: 10.3390/nu11102429
Kim, I. S., Lee, S. H., Kwon, Y. M., Adhikari, B., Kim, J. A., Yu, D. Y., et al. (2019). Oral administration of β-glucan and Lactiplantibacillus plantarum alleviates atopic dermatitis-like symptoms. J. Microbiol. Biotechnol. 29, 1693–1706. doi: 10.4014/jmb.1907.07011
Kitaoka, Y., Tamura, Y., Takahashi, K., Takeda, K., Takemasa, T., and Hatta, H. (2019). Effects of Nrf2 deficiency on mitochondrial oxidative stress in aged skeletal muscle. Physiol. Rep. 7:e13998. doi: 10.14814/phy2.13998
Kumar, A., Joishy, T., Das, S., Kalita, M. C., Mukherjee, A. K., and Khan, M. R. (2022). A potential probiotic Lactiplantibacillus plantarum JBC5 improves longevity and healthy aging by modulating Antioxidative, innate immunity and serotonin-signaling pathways in Caenorhabditis elegans. Antioxidants (Basel). 11:268. doi: 10.3390/antiox11020268
Lakshminarayanan, B., Stanton, C., O’Toole, P. W., and Ross, R. P. (2014). Compositional dynamics of the human intestinal microbiota with aging: implications for health. J. Nutr. Health Aging 18, 773–786. doi: 10.1007/s12603-014-0549-6
Langille, M. G., Meehan, C. J., Koenig, J. E., Dhanani, A. S., Rose, R. A., Howlett, S. E., et al. (2014). Microbial shifts in the aging mouse gut. Microbiome 2:50. doi: 10.1186/s40168-014-0050-9
Lee, K., Kim, H. J., Kim, S. A., Park, S. D., Shim, J. J., and Lee, J. L. (2021a). Exopolysaccharide from Lacti-plantibacillus plantarum HY7714 protects against skin aging through skin-gut Axis communication. Molecules 26:1651. doi: 10.3390/molecules26061651
Lee, K., Kim, J., Park, S. D., Shim, J. J., and Lee, J. L. (2021b). Lactiplantibacillus plantarum HY7715 ameliorates sarcopenia by improving skeletal muscle mass and function in aged Balb/c mice. Int. J. Mol. Sci. 22:10023. doi: 10.3390/ijms221810023
Li, H., Liu, F., Lu, J., Shi, J., Guan, J., Yan, F., et al. (2020). Probiotic mixture of Lactiplanti-bacillus plantarum strains improves lipid metabolism and gut microbiota structure in high fat diet-fed mice. Front. Microbiol. 11:512. doi: 10.3389/fmicb.2020.00512
Li, J., Zhao, F., Wang, Y., Chen, J., Tao, J., Tian, G., et al. (2017). Gut microbiota dysbiosis con-tributes to the development of hypertension. Microbiome 5:14. doi: 10.1186/s40168-016-0222-x
Liguori, I., Russo, G., Curcio, F., Bulli, G., Aran, L., Della-Morte, D., et al. (2018). Oxidative stress, aging, and diseases. Clin. Interv. Aging 13, 757–772. doi: 10.2147/CIA.S158513
Lin, S. W., Tsai, Y. S., Chen, Y. L., Wang, M. F., Chen, C. C., Lin, W. H., et al. (2021). Lactiplantibacillus plantarum GKM3 promotes Lon-gevity, memory retention, and reduces brain oxidation stress in SAMP8 mice. Nutrients 13:2860. doi: 10.3390/nu13082860
Liu, Y. W., Liong, M. T., Chung, Y. E., Huang, H. Y., Peng, W. S., Cheng, Y. F., et al. (2019). Effects of Lactiplantibacillus plantarum PS128 on children with autism Spectrum disorder in Taiwan: a randomized, double-blind, placebo-controlled trial. Nutrients 11:820. doi: 10.3390/nu11040820
Luan, X., Feng, M., and Sun, J. (2021). Effect of Lactiplantibacillus plantarum on antioxidant activity in fermented sausage. Food Res. Int. 144:110351. doi: 10.1016/j.foodres.2021.110351
Lustgarten, M. S. (2016). Classifying aging as a disease: the role of microbes. Front. Genet. 7:212. doi: 10.3389/fgene.2016.00212
Maynard, C., and Weinkove, D. (2018). The gut microbiota and ageing. Subcell. Biochem. 90, 351–371. doi: 10.1007/978-981-13-2835-0_12
Mossad, O., Batut, B., Yilmaz, B., Dokalis, N., Mezö, C., Nent, E., et al. (2022). Gut microbiota drives age-related oxidative stress and mitochondrial damage in microglia via the metabolite N6-carboxymethyllysine. Nat. Neurosci. 25, 295–305. doi: 10.1038/s41593-022-01027-3
Mossad, O., Nent, E., Woltemate, S., Folschweiller, S., Buescher, J. M., Schnepf, D., et al. (2021). Micro-biota-dependent increase in δ-valerobetaine alters neuronal function and is responsible for age-related cognitive decline. Nat. Aging 1, 1127–1136. doi: 10.1038/s43587-021-00141-4
Nadeem, H., Rashid, M. H., Siddique, M. H., Azeem, F., Muzammil, S., Javed, M. R., et al. (2015). Microbial invertases: a review on kinetics, thermodynamics, physiochemical properties. Process Biochem. 50, 1202–1210. doi: 10.1016/j.procbio.2015.04.015
Nakaya, S., Kobori, H., Sekiya, A., Kawagishi, H., and Ushimaru, T. (2014). Anti-aging and an-ti-microbial effects of melleolide on various types of yeast. Biosci. Biotechnol. Biochem. 78, 455–457. doi: 10.1080/09168451.2014.885826
Ni, Y., Yang, X., Zheng, L., Wang, Z., Wu, L., Jiang, J., et al. (2019). Lactobacillus and Bifidobacterium improves physiological function and cognitive ability in aged mice by the regulation of gut microbiota. Mol. Nutr. Food Res. 63:e1900603. doi: 10.1002/mnfr.201900603
Nogal, A., Valdes, A. M., and Menni, C. (2021). The role of short-chain fatty acids in the interplay between gut microbiota and diet in car-dio-metabolic health. Gut Microbes 13, 1–24. doi: 10.1080/19490976.2021.1897212
O’Hara, A. M., and Shanahan, F. (2007). Gut microbiota: mining for therapeutic potential. Clin. Gastroenterol. Hepatol. 5, 274–284. doi: 10.1016/j.cgh.2006
OjiNjideka Hemphill, N., Pezley, L., Steffen, A., Elam, G., Kominiarek, M. A., Odoms-Young, A., et al. (2023). Feasibility study of Lactiplanti-bacillus plantarum 299v probiotic supplementation in an urban academic facility among diverse pregnant individuals. Nutrients 15:875. doi: 10.3390/nu15040875
Rabe, J. H., Mamelak, A. J., McElgunn, P. J., Morison, W. L., and Sauder, D. N. (2006). Photoaging: mecha-nisms and repair. J. Am. Acad. Dermatol. 55, 1–19. doi: 10.1016/j.jaad.2005.05.010
Ragonnaud, E., and Biragyn, A. (2021). Gut microbiota as the key controllers of "healthy" aging of elderly people. Immun. Ageing 18:2. doi: 10.1186/s12979-020-00213-w
Rahman, M. M., Islam, M. R., Shohag, S., Ahasan, M. T., Sarkar, N., Khan, H., et al. (2022). Microbiome in cancer: role in carcinogenesis and impact in therapeutic strategies. Biomed. Pharmacother. 149:112898. doi: 10.1016/j.biopha.2022.112898
Ramos-Molina, B., Sánchez-Alcoholado, L., Cabrera-Mulero, A., Lopez-Dominguez, R., Carmo-na-Saez, P., Garcia-Fuentes, E., et al. (2019). Gut microbiota composition is associated with the global DNA methylation pattern in obesity. Front. Genet. 10:613. doi: 10.3389/fgene.2019.00613
Raza, M. H., Siraj, S., Arshad, A., Waheed, U., Aldakheel, F., Alduraywish, S., et al. (2017). ROS-modulated therapeutic ap-proaches in cancer treatment. J. Cancer Res. Clin. Oncol. 143, 1789–1809. doi: 10.1007/s00432-017-2464-9
Rodríguez-Rodero, S., Fernández-Morera, J. L., Menéndez-Torre, E., Calvanese, V., Fernández, A. F., and Fraga, M. F. (2011). Aging genetics and aging. Aging Dis. 2, 186–195.
Salameh, Y., Bejaoui, Y., and El Hajj, N. (2020). DNA methylation biomarkers in aging and age-related diseases. Front. Genet. 11:171. doi: 10.3389/fgene.2020.00171
Salekeen, R., Siam, M. H. B., Sharif, D. I., Lustgarten, M. S., Billah, M. M., and Islam, K. M. D. (2021). In silico in-sights into potential gut microbial modulation of NAD+ metabolism and longevity. J. Biochem. Mol. Toxicol. 35:e22925. doi: 10.1002/jbt.22925
Schumacher, B., Pothof, J., Vijg, J., and Hoeijmakers, J. H. J. (2021). The central role of DNA damage in the ageing process. Nature 592, 695–703. doi: 10.1038/s41586-021-03307-7
Seddik, H. A., Bendali, F., Gancel, F., Fliss, I., Spano, G., and Drider, D. (2017). Lactiplantibacillus plantarum and its probiotic and food potentialities. Probiotics Antimicrob. Proteins. 9, 111–122. doi: 10.1007/s12602-017-9264-z
Shenghua, P., Ziqin, Z., Shuyu, T., Huixia, Z., Xianglu, R., and Jiao, G. (2019). An integrated fecal microbiome and metabolome in the aged mice reveal anti-aging effects from the intestines and biochemical mechanism of FuFang zhenshu TiaoZhi (FTZ). Biomed. Pharmacother. 121:109421. doi: 10.1016/j.biopha.2019.109421
Shirzad, M., Hamedi, J., Motevaseli, E., and Modarressi, M. H. (2018). Anti-elastase and anti-collagenase potential of lactobacilli ex-opolysaccharides on human fibroblast. Artif. Cells Nanomed. Biotechnol. 46, 1051–1061. doi: 10.1080/21691401.2018.1443274
Singh, V., Ahlawat, S., Mohan, H., Gill, S. S., and Sharma, K. K. (2022). Balancing reactive oxygen species generation by rebooting gut microbiota. J. Appl. Microbiol. 132, 4112–4129. doi: 10.1111/jam.15504
Spath, K., Heinl, S., and Grabherr, R. (2012). Direct cloning in Lactobacillus plantarum: electro-poration with non-methylated plasmid DNA enhances transformation efficiency and makes shuttle vectors obsolete. Microb. Cell Factories 11:141. doi: 10.1186/1475-2859-11-141
Sun, Q., Liu, P., You, S., Zhao, D., Wang, C., Zhang, J., et al. (2021). Protective effects of LPL-EPS-02 on human dermal fi-broblasts damaged by UVA radiation. J. Funct. Foods 83:104544. doi: 10.1016/j.jff.2021.104544
Tan, B. L., Norhaizan, M. E., Liew, W. P., and Sulaiman, R. H. (2018). Antioxidant and oxidative stress: a mutual interplay in age-related diseases. Front. Pharmacol. 9:1162. doi: 10.3389/fphar.2018.01162
Teame, T., Wang, A., Xie, M., Zhang, Z., Yang, Y., Ding, Q., et al. (2020). Paraprobiotics and postbiotics of probiotic lactobacilli, their positive effects on the host and action mechanisms: a review. Front. Nutr. 7:570344. doi: 10.3389/fnut.2020.570344
Tiwari, V., and Wilson, D. M. (2019). DNA damage and associated DNA repair defects in disease and premature aging. Am. J. Hum. Genet. 105, 237–257. doi: 10.1016/j.ajhg.2019.06.005
Trist, B. G., Hare, D. J., and Double, K. L. (2019). Oxidative stress in the aging substantia nigra and the etiology of Parkinson’s disease. Aging Cell 18:e13031. doi: 10.1111/acel.13031
Vähämiko, S., Laiho, A., Lund, R., Isolauri, E., Salminen, S., and Laitinen, K. (2019). The impact of pro-biotic supplementation during pregnancy on DNA methylation of obesity-related genes in mothers and their children. Eur. J. Nutr. 58, 367–377. doi: 10.1007/s00394-017-1601-1
Vaiserman, A. M., Koliada, A. K., and Marotta, F. (2017). Gut microbiota: a player in aging and a target for anti-aging intervention. Ageing Res. Rev. 35, 36–45. doi: 10.1016/j.arr.2017.01.001
Vaiserman, A., Koliada, A., Zayachkivska, A., and Lushchak, O. (2020). Nanodelivery of natural An-tioxidants: An anti-aging perspective. Front. Bioeng. Biotechnol. 7:447. doi: 10.3389/fbioe.2019.00447
Van Beek, A. A., Sovran, B., Hugenholtz, F., Meijer, B., Hoogerland, J. A., Mihailova, V., et al. (2016). Supplementation with Lactiplantibacillus plantarum WCFS1 prevents decline of mucus barrier in Colon of accelerated aging Ercc 1−/Δ7 mice. Front. Immunol. 7:408. doi: 10.3389/fimmu.2016.00408
Van Zyl, W. F., Deane, S. M., and Dicks, L. M. T. (2020). Molecular insights into probiotic mechanisms of action employed against intestinal pathogenic bacteria. Gut Microbes 12:1831339. doi: 10.1080/19490976.2020.1831339
Vivarelli, S., Salemi, R., Candido, S., Falzone, L., Santagati, M., Stefani, S., et al. (2019). Gut Micro-biota and Cancer: from pathogenesis to therapy. Cancers 11:38. doi: 10.3390/cancers11010038
Wang, W., Liu, F., Xu, C., Liu, Z., Ma, J., Gu, L., et al. (2021). Lactiplantibacillus plantarum 69-2 combined with Galac-to-oligosaccharides alleviates d-galactose-induced aging by Regu-lating the AMPK/SIRT1 signaling pathway and gut mi-crobiota in mice. J. Agric. Food Chem. 69, 2745–2757. doi: 10.1021/acs.jafc.0c06730
Warraich, U. E., Hussain, F., and Kayani, H. U. R. (2020). Aging-oxidative stress, antioxidants and computational modeling. Heliyon 6:e04107. doi: 10.1016/j.heliyon.2020.e04107
Wei, Z. Y., Rao, J. H., Tang, M. T., Zhao, G. A., Li, Q. C., Wu, L. M., et al. (2022). Characterization of changes and driver microbes in gut microbiota during healthy aging using a captive monkey model. Genomics Proteomics Bioinformatics 20, 350–365. doi: 10.1016/j.gpb.2021.09.009
Welker, D. L., Crowley, B. L., Evans, J. B., Welker, M. H., Broadbent, J. R., Roberts, R. F., et al. (2020). Transformation of Lactobacil-lusplantarum and Apilactobacillus kunkeei is influenced by recipient cell growth temperature, vector replicon, and DNA methylation. J. Microbiol. Methods 175:105967. doi: 10.1016/j.mimet.2020.105967
Ye, J., Wu, W., Li, Y., and Li, L. (2017). Influences of the gut microbiota on DNA methylation and histone modification. Dig. Dis. Sci. 62, 1155–1164. doi: 10.1007/s10620-017-4538-6
Yu, J., Ma, X., Wang, X., Cui, X., Ding, K., Wang, S., et al. (2022). Application and mechanism of probiotics in skin care: a review. J. Cosmet. Dermatol. 21, 886–894. doi: 10.1111/jocd.14734
Zampieri, M., Ciccarone, F., Calabrese, R., Franceschi, C., Bürkle, A., and Caiafa, P. (2015). Reconfiguration of DNA methylation in aging. Mech. Ageing Dev. 151, 60–70. doi: 10.1016/j.mad.2015.02.002
Zhang, X., Duan, W., Zou, J., Zhou, H., Liu, C., and Yang, H. (2019). Flavor and antioxidant activity improvement of carrot juice by fermentation with Lactiplantibacillus plantarum WZ-01. J. Food Meas. Charact. 13, 3366–3375. doi: 10.1007/s11694-019-00260-y
Zhang, A., Hsiung, K. C., Kern, C. C., Wang, Y., Girtle, A. L., Xu, N., et al. (2023). Unraveling effects of anti-aging drugs on C. elegans using liposomes. Geroscience 4, 1–21. doi: 10.1007/s11357-023-00800-x.Epub
Zhang, L., Liu, C., Li, D., Zhao, Y., Zhang, X., Zeng, X., et al. (2013). Antioxidant activity of an exopolysaccharide isolated from Lactiplantibacillus plantarum C88. Int. J. Biol. Macromol. 54, 270–275. doi: 10.1016/j.ijbiomac.2012.12.037
Zhang, J., Zhao, X., Jiang, Y., Zhao, W., Guo, T., Cao, Y., et al. (2017). An-tioxidant status and gut microbiota change in an aging mouse model as influenced by exopoly-saccharide produced by Lactiplantibacillus plantarum YW11 iso-lated from Tibetan kefir. J. Dairy Sci. 100, 6025–6041. doi: 10.3168/jds.2016-12480
Zhang, B., Zhou, W., Liu, Q., Huang, C., Hu, Z., Zheng, M., et al. (2023). Effects of fecal microbiota transplant on DNA methylation in patients with systemic lupus erythematosus. J. Autoimmun. 141:103047. doi: 10.1016/j.jaut.2023.103047
Zhou, X., Sun, H., Tan, F., Yi, R., Zhou, C., Deng, Y., et al. (2021). Anti-aging effect of Lacti-plantibacillus plantarum HFY09-fermented soymilk on D-galactose-induced oxidative aging in mice through modulation of the Nrf2 signaling pathway. J. Funct. Foods 78:104386. doi: 10.1016/j.jff.2021.104386
Keywords: microbial dysbiosis, probiotic, photo-aging, anti-oxidative stress pathway, Lactiplantibacillus plantarums strains, collagen improvement
Citation: Gupta N, El-Gawaad NSA, Mallasiy LO, Gupta H, Yadav VK, Alghamdi S and Qusty NF (2024) Microbial dysbiosis and the aging process: a review on the potential age-deceleration role of Lactiplantibacillus plantarum. Front. Microbiol. 15:1260793. doi: 10.3389/fmicb.2024.1260793
Edited by:
Antonios Koutelidakis, University of the Aegean, GreeceCopyright © 2024 Gupta, El-Gawaad, Mallasiy, Yadav, Alghamdi and Qusty. This is an open-access article distributed under the terms of the Creative Commons Attribution License (CC BY). The use, distribution or reproduction in other forums is permitted, provided the original author(s) and the copyright owner(s) are credited and that the original publication in this journal is cited, in accordance with accepted academic practice. No use, distribution or reproduction is permitted which does not comply with these terms.
*Correspondence: Nishant Gupta, bWljcm9uaXNoYW50QHlhaG9vLmlu