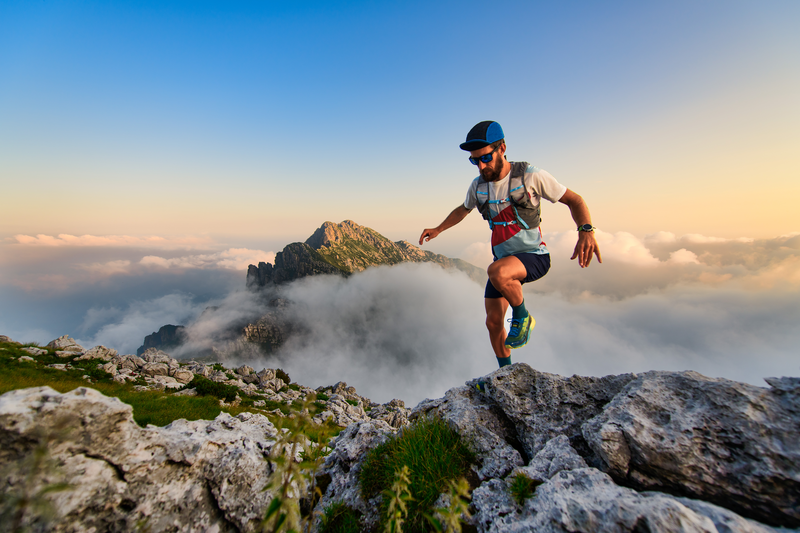
94% of researchers rate our articles as excellent or good
Learn more about the work of our research integrity team to safeguard the quality of each article we publish.
Find out more
MINI REVIEW article
Front. Microbiol. , 10 February 2023
Sec. Antimicrobials, Resistance and Chemotherapy
Volume 14 - 2023 | https://doi.org/10.3389/fmicb.2023.948092
This article is part of the Research Topic Photonics-based Diagnosis and Treatment of Infectious and Inflammatory Diseases View all 9 articles
Infectious diseases remain a serious global challenge threatening human health. Oral infectious diseases, a major neglected global problem, not only affect people’s lifestyles but also have an intimate association with systemic diseases. Antibiotic therapy is a common treatment. However, the emergence of new resistance problems hindered and enhanced the complication of the treatment. Currently, antimicrobial photodynamic therapy (aPDT) has long been the topic of intense interest due to the advantage of being minimally invasive, low toxicity, and high selectivity. aPDT is also becoming increasingly popular and applied in treating oral diseases such as tooth caries, pulpitis, periodontal diseases, peri-implantitis, and oral candidiasis. Photothermal therapy (PTT), another phototherapy, also plays an important role in resisting resistant bacterial and biofilm infections. In this mini-review, we summarize the latest advances in photonics-based treatments of oral infectious diseases. The whole review is divided into three main parts. The first part focuses on photonics-based antibacterial strategies and mechanisms. The second part presents applications for photonics-based treatments of oral infectious diseases. The last part discusses present problems in current materials and future perspectives.
Oral infectious diseases are often ignored, and they threaten human oral health and systemic health. Some oral infectious diseases such as periodontitis become a major public health problem because of their high prevalence, dental and maxillofacial deformation and functional impairment, high dental care cost, and negative impact on general health (Papapanou et al., 2018). These diseases are usually caused by plaque or dysbiosis. Because most oral infectious diseases are deep tissue infections and the complexity of anatomical structures, the treatment effect is often unsatisfactory.
Biophotonics is defined as the science of imaging, detecting, and manipulating biological materials by generating and using light or photons. It is used successfully in medicine and dentistry to help diagnose and treat various diseases (Besegato et al., 2022). Recently, antibacterial photodynamic therapy (aPDT) and photothermal therapy (PTT) and their derived multimodal synergistic treatments have received extensive attention. Due to its strong bactericidal effect and no bacterial resistance, it is also gradually used in the dental field. The development of some photonics-based materials will be beneficial for the clinical treatment of oral infectious diseases. This mini-review will provide a succinct summary of the mechanism and strategies of photonics-based treatment and applications in oral infectious diseases.
Antibacterial photodynamic therapy is a promising strategy to eradicate pathogenic microbes or biofilm and has the advantages of simple operation, wide application, and no drug resistance. aPDT involves three components: light, a photosensitizer (PS), and oxygen. The light source that excites the corresponding PS, usually from visible light to near-infrared spectrum, in which visible light greater than 430 nm is irradiated outside the tooth, can be used for the extinction of Enterococcus faecalis in root canal treatment (Cieplik et al., 2016). However, the excitation light is usually in a fixed wavelength band with limited penetration, which limits its application in deep tissue (Lin et al., 2021). To solve this problem, near-infrared-II light with less energy decay and increased tissue depth is recommended (Zhang G. et al., 2021). Electric-driven aPDT using in situ chemiluminescence instead of an external light source to activate PSs is another strategy (Liu et al., 2018). Another limitation of aPDT application is due to the hydrophobicity and aggregation of some PSs. Therefore, a series of hydrophilic PSs were developed to improve the above problems (Sun et al., 2019; Gao et al., 2021). Generally, aPDT has broad-spectral antibacterial activity instead of non-selective one, which may kill off beneficial bacteria. Accordingly, targetable aPDT (Im et al., 2021) or promotion of type I photoreactions (Li et al., 2018) were conducted to be a selective antibacterial strategy. The excellent photoantimicrobials should have the following characteristics: positive charge, hydrophilicity, low molecular weight, High 1O2 quantum yield, photostability, and appropriate therapeutic window (Maisch, 2020). Nowadays, a growing number of researchers pay more attention to aPDT-based synergistic therapies, which include aPDT and antibiotic therapy (Jiang et al., 2020), enzyme-aPDT (Li et al., 2021b; Hu et al., 2022), photodynamic ion therapy (Li J. et al., 2021), aPDT and low-level laser therapy (LLLT) (He et al., 2021), aPDT and Chemotherapy (Zhang et al., 2015; Hamblin, 2016; Chen H. et al., 2019); aPDT and Chemodynamic Therapy (CDT) (Ma et al., 2021), and aPDT gas therapy (Sun J. et al., 2021; Zhu et al., 2021; Zhou et al., 2022). However, remarkably, synergistic aPDT and antibiotic therapy may be a controversial strategy, since low reactive oxygen species (ROS) concentrations are beneficial for bacteria and can induce resistance in the antibiotic-mediated killing of bacteria (Van Acker and Coenye, 2017). Synergistic therapy is generally not only to improve sterilization ability but also to propose personalized treatment solutions for specific clinical problems, such as generating more oxygen to improve aPDT effect or promoting tissue healing or immunomodulatory. Most of the synergistic therapies have excellent antibacterial effects, especially aPDT and antibiotic therapy (Teic, 4.9 logs reduction against Staphylococcus aureus (S. aureus; Jiang et al., 2020) and aPDT and chemotherapy [C60-fullerene with KI, 4.5 logs reduction against A. baumanii and 4.1 logs reduction against Methicillin-resistant S. aureus (MRSA); Zhang et al., 2015]. The details of the above aPDT-based synergistic therapies are summarized in Table 1.
Antibacterial properties of aPDT-based materials are derived from ROS. ROS including superoxide anion (O2.−), hydrogen peroxide (H2O2), and hydroxyl radical (OH˙) generated via the type I mechanism (electron transfer) and singlet oxygen (1O2) generated via the type II mechanism (energy transfer). The possible antibacterial mechanisms of aPDT may include the following (Liu W. et al., 2020; Fan et al., 2021; Huang et al., 2022): (1) altered outer membrane permeability, (2) oxidation of Lipids, (3) protein or DNA damage, (4) interfere with bacterial metabolism, and (5) irreversible bacterial destruction. These antibacterial mechanisms of aPDT are displayed in Figure 1.
Figure 1. (A) PDT mechanism including type I mechanism (electron transfer) and type II mechanism (energy transfer); (B) Antibacterial mechanism of aPDT including (1) altered outer membrane permeability, (2) oxidation of lipids, (3) protein or DNA damage, (4) interfere with bacterial metabolism, and (5) irreversible bacterial destruction.
PTT is a non-invasive therapy to combat drug-resistant bacteria and plaque biofilm and has the advantages of minimal systemic toxicity, broad-spectrum antibacterial activity, and no drug resistance. When PTT is applied alone, high-power laser excitation and high-dose photothermal agent (PTA) are often required, which may cause tissue damage. Notably, a localized thermal management strategy based on the thermal-disrupting interface-induced mitigation (TRIM) for accurate topical antibacterial therapy was developed (Hu et al., 2020). TRIM film contains a critical dimension for surface features, which leads to species-specific spatial confinement. Bacteria are attached to the microvalleys while host cells can only attach to the microtidges. After infrared irradiation, the phase change material poly(N-isopropylacrylamide) (pNIPAM) polymerized and made the PTA gold nanostars gather near the bacteria without host cell damage. pNIPAM is photothermal-responsive and could transfer from the hydrophilic phase to the hydrophobic phase when the temperature is up to a lower critical solution temperature. Taking advantage of this property, such material could trap bacteria (Yang et al., 2018, 2019). Notably, while PTA exerts its bactericidal properties, the local temperature should be controlled to avoid the aggravation of local tissue inflammation or secondary damage. Therefore, many researchers have proposed the strategy of mild temperature PTT. Usually, a mild temperature is no more than 45°C, which can act as an antibacterial or promote drug release (Ye et al., 2020; Zhang G. et al., 2021). In addition, there are various antibacterial strategies for PTT-based synergistic therapy including synergistic PTT and antibiotic therapy (Huang et al., 2020; Wang et al., 2020), enzyme-PTT (Wang et al., 2021; Xie et al., 2021), photothermal ion therapy (Li et al., 2019), synergistic PTT and CDT (Liu Z. et al., 2021), sonodynamic therapy (SDT) and PTT (Bi et al., 2022), and PTT gas therapy (Liu Y. N. et al., 2020).
Generally, PTAs transform light energy into heat energy in three ways including plasmonic heating, electron–hole generation and relaxation, and thermal vibration of molecules (Chen C. J. et al., 2019). Antibacterial mechanisms of PTT include (1) increased membrane permeability, (2) bacterial protein denaturation, and (3) irreversible bacterial destruction. These antibacterial mechanisms of PTT are displayed in Figure 2.
Figure 2. (A) Photothermal conversion mechanism including (1) plasmonic heating, (2) electron–hole generation and relaxation, and (3) thermal vibration of molecules. (B) Antibacterial mechanisms of PTT include (1) increased membrane permeability, (2) bacterial protein denaturation, and (3) irreversible bacterial destruction.
There were several disadvantages of aPDT or PTT being applied alone. Since ROS has a short half-life and narrow diffusion distance, it can hardly invade into compact bacterial biofilm (Qi et al., 2022). To obtain a good sterilization effect, more dosages of PSs or PTAs and greater light intensity are needed, which may cause tissue damage. To achieve more function and minimal side effects, aPDT- and PTT-derived multimodal synergistic therapies attract attention. Heat can accelerate the release and penetration of drugs, and promote the release of ROS and some chemical reactions. Currently, antibacterial strategies for aPDT/PTT synergistic therapies include synergistic aPDT/PTT (Liu Y. X. et al., 2021; Mo et al., 2022), aPDT/PTT and antibiotic therapy (Zeng et al., 2020), aPDT/PTT and gas therapy (Cai et al., 2021; Qi et al., 2022), aPDT/PTT and ion therapy, and aPDT/PTT/CDT (Zhang J. C. et al., 2021). For aPDT/PTT materials, there are huge differences between different materials in antibacterial activity. The materials with the same composition but different exposed facets could show different antibacterial properties. In Mo’s work, two Cu7S4 nanosheets with (304) and (224) exposed facets were designed for antibacterial strategy. The antibacterial effects of Escherichia coli and Bacillus subtilis were 72.2% and 40.7%, respectively, in the former one, while were nearly 100 and 96.8%, respectively, in the latter one. The latter one could produce richer ROS and have more efficient photothermal conversion and stronger adsorption due to different structures (Mo et al., 2022). In the above-mentioned research, it is worth noting that aPDT/PTT/CDT and aPDT/PTT/gas therapy can achieve over 99% sterilization efficiency. It was reported that CuFeO4/graphene oxide coatings with aPDT/PTT/CDT functions showed very high antibacterial effciency of 99.94 ± 1.20% against S. aureus and 99.57 ± 0.86% against E. coli, respectively (Zhang J. C. et al., 2021). In addition, gas therapy (such as CO and NO) can further enhance sterilization by dissipating biofilm. The aPDT/PTT/NO gas group can lead to an additional reduction of about 1.5 log against Porphyromonas gingivalis (P. gingivalis) biofilm and over 2 log against Fusobacterium nucleatum (F. nucleatum) biofilm, compared with the aPDT/PTT group (Qi et al., 2022). Interestingly, Cai et al. (2021) revealed similar results that all tested bacteria including E. coli, S. aureus, and MRSA could be additionally reduced over 2 log in the aPDT/PTT/CO gas group than aPDT/PTT group.
Low-Level Laser Therapy or PBM is a term used to describe a medical procedure that uses low-power lasers (0.5 W), sometimes known as “cold lasers,” so that the effects are a result of the light rather than heat. When mitochondria are stimulated by a light source, PBM reacts photochemically with target cells (Dompe et al., 2020). The cytochrome c oxidase enzyme, which is found in the unit IV respiratory chain of the mitochondria, absorbs the application of red light (600–810 nm). As inhibitory nitric oxide is separated from the enzyme by photons, electron transport, mitochondrial membrane potential, and ATP synthesis are all increased. On the other hand, near-infrared light (810–1,064 nm) application stimulates light-sensitive ion channels and raises Ca2+ levels; Then, the Ca2+ interacts with ROS and cAMP. All these processes promote cell motility, proliferation, and differentiation (de Freitas and Hamblin, 2016; Dompe et al., 2020).
Although most studies concentrate on the intracellular effects of visible and near-infrared light on mitochondrial cytochrome c oxidase, recent research has shown that transforming growth factor (TGF)-b1 and its downstream targets, Human Beta-Defensin-2 (HBD-2), have direct antimicrobial effects and indirectly control inflammation and encourage tissue regeneration in the peri-implant or periodontal tissues. Laser-mediated HBD-2 expression involves the Smad and non-Smad components of the TGF-b1 signaling pathway (Tang et al., 2017).
Oral infectious diseases such as caries, pulpitis, periodontal diseases, peri-implantitis, and oral candidiasis are caused by plaque biofilm or dysbacteriosis. Therefore, effective antimicrobials are key to treating these diseases. However, the infection site of oral infectious diseases is deep and the anatomical structure is often complicated, which makes it difficult to completely remove bacterial plaque and the recurrence of infection. aPDT or PTT as a non-invasive treatment plays an important role in oral infectious diseases.
Antimicrobial photodynamic therapy can effectively inhibit the growth of a variety of microorganisms associated with cariogenic biofilms, including Streptococcus, Streptococcus mutans (S. mutans), Lactobacillus, and yeast (Garcia et al., 2021). A nanoplatform composed of assembling toluidine blue O (TBO) and superparamagnetic iron oxide nanoparticles (MagTBO) was designed to achieve enhanced antimicrobial activity (Balhaddad et al., 2021). Under a magnetic field, 2.5% MagTBO microemulsions showed a 6-log reduction of S. mutans monospecies biofilm and a 4.5- to 5.5-log reduction of multispecies biofilms. In addition, an amphiphilic and pH-responsive PS (Polyethylene glycol-b-poly(2-(diisopropylamino)ethyl methacrylate)) (MPEG-b-P(PDA) loaded with Ce6 (MPP-Ce6) was developed for inhibiting multispecies cariogenic biofilms (Liu et al., 2022). MPP-Ce6 had over 99% inhibition against S. mutans, Streptococcus sobrinus, and Streptococcus sanguinis. Although numerous laboratory studies have demonstrated the relative effectiveness of aPDT in reducing the number of cariogenic bacteria in biofilms in vitro, preclinical (orthotopic models) and clinical studies have shown that bacterial reduction in aPDT is not significant, especially in dentin carious lesions (Reis et al., 2019).
PTT-based researches on dental caries are rare. Recently, removable photothermal antibacterial “warm paste” nanoagents became striking (Xu X. et al., 2022). In brief, polydopamine (PDA), Ag, and glycol chitosan (GCs) were sequentially modified on the surface of Fe3O4 to form FePAgPG nanoparticles (NPs). In a cariogenic acid environment, due to the pH-responsive effect, FePAgPG NPs targeted cariogenic bacteria. The FePAgPG NPs inhibited over 95% of biofilm formed by Streptococcus mutants via the Ag-assisted PTT strategy. Multimodal synergistic treatments could achieve the best antibacterial effect. A pH-responsive nanoplatform had a triple function with synergetic pharmacological therapy and aPDT/PTT for enhanced biofilm eradication and caries prevention (Yu et al., 2022). The combination of ciprofloxacin and IR780 showed an amazing killing rate of 99.8% and considerably high biofilm dispersion of about 70–80%. Moreover, under an acidic oral biofilm microenvironment, the nanoplatform suffered from degradation, anchored bacteria, and released drugs on demand.
Incomplete cleaning of infected dental pulp causes failure of endodontic treatment and refractory apical periodontitis. In terms of bacterial control, aPDT has proven to be a positive option as an adjunct to conventional root canal techniques (Lopes et al., 2022). Indocyanine Green (ICG) was regarded as the best PS for endodontic infection compared with TBO and methylene blue (MB). The enterococcal surface protein (esp) gene was related to colonization and bacterial resistance in endodontic infections. The expression of esp was significantly downregulated to approximately ~5.2-fold by ICG (Chiniforush et al., 2018). Nano-MOF Fe-101 loading ICG (Fe-101-ICG) could downregulate the expression of esp to 6.2-fold (Golmohamadpour et al., 2018). Notably, ICG is not only a PS but also a PTA. Some researchers ignored the additional bactericidal effect of the photothermal effect of ICG. Besides, some Zn-based PSs were developed to combat Enterococcus faecalis (E. faecalis) (Diogo et al., 2017; Schuenck-Rodrigues et al., 2020). The MIC of nanoemulsion containing zinc phthalocyanines (ZnPc-NE) against E. faecalis was only 1.09 μg ml−1. Under a 60 or 90 s irradiation, Zn(II)chlorin e6 methyl ester (Zn(II)e6Me) could eliminate around 60% of the biofilm’s biomass. Sodium hypochlorite (NaClO) remains the most commonly used irrigant for root canal treatment worldwide. However, a higher concentration of NaClO with high antibacterial activity could cause severe complications. To address this issue, a visible light-guided root canal cleaning system consisting of a mixture of neutralized 0.5% NaClO solution and TiO2-x NPs was developed. The system produced various ROS including ·OH, ·Cl, and ·ClO, and after treatment with this system for 5 min, the killing rate of planktonic E. faecalis was 99.3% and that of biofilm was 100% (Liu et al., 2022). In another study, PTT utilizations with 1% NaClO solution showed superior performance in tooth root canal therapy (Duan et al., 2022). A novel D-A semiconducting conjugated polymer (PBDT-DIID) was designed, showing 70.6% photothermal conversion efficiency. The 1% NaClO solution with 25 μg ml−1 and 50 μg ml−1 could kill 99.7 and 99.6% of the bacteria, respectively. Meanwhile, the temperature elevation outside the root canal was controlled within 10°C, protecting periodontal membrane cells from damage.
Among all oral infectious diseases, researchers have studied the most about photodynamic materials for the treatment of periodontal disease. Different kinds of materials are designed according to the characteristics of the disease. Generally, the lesions formed in the periodontal pocket are irregular and periodontitis tends to occur in deep tissue. NPs with amphiphilic silane containing Chlorin e6 (Fe3O4-silane@Ce6/C6) were designed to kill periodontitis-related pathogens. The ratio metric fluorescence of Ce6/C6 could monitor the aPDT effect of Ce6 and Fe3O4 and realize the magnetically targeting function. Near-infrared (NIR) light has a strong penetration ability in tissue. Taking advantage of this feature, nanomaterials based on upconversion nanoparticles (UCNPs) were developed to combat periodontitis-related pathogens such as P. gingivalis, Prevotella intermedia, and F. nucleatum. NaYF4-Mn30%@Ce6@silane reached the maximum CFU reduction by more than 2 log (Zhang et al., 2019). Another work realized the conversion from NIR light to ultraviolet light and triggered the luminescence catalytic material titanium dioxide (TiO2), to realize the deep aPDT. UCNPs@TiO2 could reduce the three single-species biofilm CFU by about 4 orders of magnitude. Deep tissues are often in a hypoxic state, and most of the pathogenic bacteria of periodontitis are anaerobic bacteria. The process of PSs producing 1O2 via the type II mechanism needs consuming oxygen. Therefore, an oxygen self-sufficient nanoplatform (Fe3O4@Ce6-MnO2) was designed to solve the above problems (Sun X. et al., 2021). MnO2 nanolayer could react with hydrogen peroxide in the environment to generate O2 to enhance the efficacy of aPDT. In addition, since not all aPDT could achieve the ideal treatment effect, aPDT-based synergistic therapies were considered as strategies. Tinidazole-loaded TAT-Ce6 conjugate showed remarkable synergistic anti-periodontitis effects of aPDT and antibiotic therapy (Li et al., 2021a). Rapid killing of periodontal pathogens can also be achieved through the synergistic effect of aPDT and released ions. This 2D MOF CuTCPP-Fe2O3 nanosheet could also alleviate inflammation and promote angiogenesis (Li J. et al., 2021). However, too much ROS could cause serious oxidative stress and may aggravate tissue damage. The temporal sequence of ROS generation and scavenging is used to realize the antibacterial and anti-inflammatory functions. In the first stage, PSs produce ROS to kill bacteria. After that, the ROS scavenger removed excess ROS which is beneficial to reduce inflammation and tissue repair. Materials reported with the above functions are CeO2@Ce6 with 4 log CFU reduction and cyanobacteria loading Ce6 and Cu5.4O (CeCyan-Cu5.4O) with about 100% killing of the anaerobic bacteria biofilm (Sun Y. et al., 2021; Wang et al., 2022).
Hydrogels are the most popular polymers applied as delivery scaffolds for tissue generation and function as carriers for PTA loading. Au NPs such as Au nanorods (AuNRs), Au nanobipyramids (Au NBPs), and gold nanocages (GNC), as common PTAs, have high photothermal conversion efficiency. Chitosan hydrogels embedded with AuNRs showed significant anti-biofilm activity (Bermudez-Jimenez et al., 2020). With an increase of 10°C, there was a 5–8 log reduction against Streptococcus oralis (S oralis) and E faecalis biofilms. In another study, minocycline-loaded Au NBPs@SiO2 was incorporated into hydrogels and the synergetic antibiotic and photothermal treatment could kill 90 and 66.7% of P. gingivalis on the 3rd and 5th days (Lin et al., 2020). Zhang et al. (2020) designed a nano-antibiotic platform (TC-PCM@GNC-PND), which is composed of GNC, phase-change materials (PCM), poly(N-isopropylacrylamide-co-diethylaminoethyl methacrylate) (PND), and tetracycline (TC). Two thermos-sensitive interactions of PCM and PND could precisely control the release of the encapsulated drugs. Meanwhile, the platform could act as injectable hydrogel in situ, promoting the retention of antimicrobial agents in local infectious sites. In addition, chlorhexidine-incorporated hydrogels composed of curdlan and PDA were fabricated for periodontal treatment (Tong et al., 2020). The bactericidal effect comes from the synergistic effect of photothermal action and antibacterial agent. The best antibacterial rate is as high as 99.9%.
While considering the antibacterial treatment of periodontitis, attention should also be paid to inflammatory regulation. A baicalein-loaded mesoporous Prussian blue (MPB-BA), which has antioxidant, anti-inflammatory, and antibacterial effects, was designed for bacteria-induced periodontitis treatment (Tian et al., 2022). No CFU could be found on blood agar plates of the MPB-BA group, suggesting excellent antibacterial activity. Macrophages could be switched to M2 phenotype and MPB-BA-regulated inflammation via the inhibition of the Nrf2/NF-κB pathway. The multimodal synergistic treatment of aPDT/PTT combined with other treatments can further improve the bactericidal and anti-biofilm effects and can obtain multiple therapeutic effects at the same time. Self-assembled NPs (sPDMA@ICG) with aPDT/PTT and ion therapy were applied for the alveolar bone resorption inhibition and inflammatory alleviation of periodontitis (Shi et al., 2021). The star-shaped brush poly(2-(dimethylamino)ethyl methacrylate) (sPDMA) with a positive charge could make whole NPs tightly absorbed on the surface of P. gingivalis. NPs containing 10 μg ml−1 ICG almost inhibited the bacterial and biofilm growth due to aPDT/PTT effects. Another research is on aPDT/PTT and nitric oxide (NO) gas synergistic therapy platforms for biofilm eradication and inflammation regulation against periodontal diseases (Qi et al., 2022). AuNRs and ICG acted as heat-source, controlling the generation of NO. Meanwhile, ICG could produce ROS under NIR irradiation. The nanoplatform showed excellent antibiofilm functions with an about 4-log reduction in CFU of multi-species biofilm. In addition, expressions of adhesin molecule genes and virulence factor genes of P. gingivalis significantly downregulated after treatment.
In addition, LLLT or PBM is a therapy that utilizes low-level laser irradiation on cells or tissues to regenerate tissue, reduce inflammation, and reduce pain (He et al., 2021). One study showed that both aPDT and LLLT treatments improved the clinical parameters of periodontal disease and there were no significant differences between the two treatments (Freire et al., 2020).
Both aPDT and PTT were proven to be effective treatments for peri-implantitis (Wang et al., 2019; Xu B. et al., 2022), oral candidiasis (or Candida albicans; Dos Santos et al., 2019; Vila-Nova et al., 2022), oral lichen planus (Al-Maweri et al., 2018), jawbone infections (or methicillin-resistant S. aureus) (Araujo et al., 2018; Nie et al., 2022), and alveolar repair (Ervolino et al., 2019). However, research on these diseases mainly focused on killing pathogens in vitro or evaluating the evaluation of commercially available PSs for clinical treatment. The development of therapeutic materials for these diseases is required in the future.
Although research and development of aPDT and PTT materials have entered a climax in recent years, there are still some problems that have to be mentioned. For example, as far as PSs or PTAs are concerned, the concentration, light intensity, and irradiation time used are also different due to different materials. Notably, high-dose NIR light could activate Transcription Factor-4 (ATF-4)-mediated endoplasmic reticulum stress and autophagy, inducing NIR light phototoxicity (Khan et al., 2015). There is currently no unified standard or safety threshold for these parameters. Still, most experiments remain in vitro experiments, and there is still a long way to go before product transformation. Compared with the development of photonics-based materials in other medical disciplines, the development in the field of stomatology is still in its infancy. The oral microenvironment is extremely complex, such as different pH, temperature tolerance of different parts, fluidity of saliva, and gingival crevicular fluid. Therefore, combined with the characteristics of oral infectious diseases, personalized photonics-based oral materials and oral materials integrated with diagnosis and treatment will be the development trend and the focus of future research. It is expected that more products can be transformed into clinical practice for the benefit of human beings.
SH: writing-original draft and data curation. MQ: conceptualization, methodology, visualization, supervision, and writing-reviewing and editing. YC: writing-original draft. All authors contributed to the article and approved the submitted version.
The authors declare that the research was conducted in the absence of any commercial or financial relationships that could be construed as a potential conflict of interest.
All claims expressed in this article are solely those of the authors and do not necessarily represent those of their affiliated organizations, or those of the publisher, the editors and the reviewers. Any product that may be evaluated in this article, or claim that may be made by its manufacturer, is not guaranteed or endorsed by the publisher.
Al-Maweri, S. A., Ashraf, S., Kalakonda, B., Halboub, E., Petro, W., and AlAizari, N. A. (2018). Efficacy of photodynamic therapy in the treatment of symptomatic oral lichen planus: a systematic review. J. Oral Pathol. Med. 47, 326–332. doi: 10.1111/jop.12684
Araujo, T. S. D., Rodrigues, P. L. F., Santos, M. S., de Oliveira, J. M., Rosa, L. P., Bagnato, V. S., et al. (2018). Reduced methicillin-resistant Staphylococcus aureus biofilm formation in bone cavities by photodynamic therapy. Photodiagn. Photodyn. Ther. 21, 219–223. doi: 10.1016/j.pdpdt.2017.12.011
Balhaddad, A. A., Xia, Y., Lan, Y., Mokeem, L., Ibrahim, M. S., Weir, M. D., et al. (2021). Magnetic-responsive photosensitizer nanoplatform for optimized inactivation of dental caries-related biofilms: technology development and proof of principle. ACS Nano 15, 19888–19904. doi: 10.1021/acsnano.1c07397
Bermudez-Jimenez, C., Nino-Martinez, N., Patino-Marin, N., Martinez-Gutierrez, F., Ruiz, F., Bach, H., et al. (2020). Effective control of biofilms by photothermal therapy using a gold nanorod hydrogel. J. Biomed. Mater. Res. B Appl. Biomater. 108, 333–342. doi: 10.1002/jbm.b.34392
Besegato, J. F., de Melo, P. B. G., Tamae, P. E., Alves, A. P. A. R., Rondon, L. F., Leanse, L. G., et al. (2022). How can biophotonics help dentistry to avoid or minimize cross infection by SARS-CoV-2? Photodiagn. Photodyn. Ther. 37:102682. doi: 10.1016/j.pdpdt.2021.102682
Bi, X., Bai, Q., Liang, M., Yang, D., Li, S., Wang, L., et al. (2022). Silver peroxide nanoparticles for combined antibacterial sonodynamic and photothermal therapy. Small 18:e2104160. doi: 10.1002/smll.202104160
Cai, X. J., Tian, J., Zhu, J. W., Chen, J. P., Li, L., Yang, C., et al. (2021). Photodynamic and photothermal co-driven CO-enhanced multi-mode synergistic antibacterial nanoplatform to effectively fight against biofilm infections. Chem. Eng. J. 426:131919. doi: 10.1016/j.cej.2021.131919
Chen, C. J., Kuang, Y. D., and Hu, L. B. (2019). Challenges and opportunities for solar evaporation. Joule 3, 683–718. doi: 10.1016/j.joule.2018.12.023
Chen, H., Yang, J., Sun, L., Zhang, H., Guo, Y., Qu, J., et al. (2019). Synergistic chemotherapy and photodynamic therapy of endophthalmitis mediated by Zeolitic Imidazolate framework-based drug delivery systems. Small 15:e1903880. doi: 10.1002/smll.201903880
Chiniforush, N., Pourhajibagher, M., Parker, S., Benedicenti, S., Shahabi, S., and Bahador, A. (2018). The effect of sublethal photodynamic therapy on the expression of Enterococcal surface protein (esp) encoding gene in enterococcus faecalis: quantitative real-time PCR assessment. Photodiagn. Photodyn. Ther. 24, 311–317. doi: 10.1016/j.pdpdt.2018.10.008
Cieplik, F., Pummer, A., Leibl, C., Regensburger, J., Hiller, K.-A., Schmalz, G., et al. (2016). Photodynamic inactivation of root canal bacteria by light activation through human dental hard and simulated surrounding tissue. Front. Microbiol. 7:929. doi: 10.3389/fmicb.2016.00929
de Freitas, L. F., and Hamblin, M. R. (2016). Proposed mechanisms of Photobiomodulation or low-level light therapy. IEEE J. Sel. Top Quantum Electron. 22, 348–364. doi: 10.1109/JSTQE.2016.2561201
Diogo, P., Fernandes, C., Caramelo, F., Mota, M., Miranda, I. M., Faustino, M. A. F., et al. (2017). Antimicrobial photodynamic therapy against endodontic enterococcus faecalis and Candida albicans mono and mixed biofilms in the presence of photosensitizers: a comparative study with classical endodontic Irrigants. Front. Microbiol. 8:498. doi: 10.3389/fmicb.2017.00498
Dompe, C., Moncrieff, L., Matys, J., Grzech-Lesniak, K., Kocherova, I., Bryja, A., et al. (2020). Photobiomodulation-underlying mechanism and clinical applications. J. Clin. Med. 9:1724. doi: 10.3390/jcm9061724
Dos Santos, K. F., Sousa, M. S., Valverde, J. V. P., Olivati, C. A., Souto, P. C. S., Silva, J. R., et al. (2019). Fractal analysis and mathematical models for the investigation of photothermal inactivation of Candida albicans using carbon nanotubes. Colloid. Surface. B. 180, 393–400. doi: 10.1016/j.colsurfb.2019.05.002
Duan, X., Zhang, Q., Jiang, Y., Wu, X., Yue, X., Geng, Y., et al. (2022). Semiconducting polymer nanoparticles with Intramolecular motion-induced Photothermy for tumor phototheranostics and tooth root canal therapy. Adv. Mater. 34:e2200179. doi: 10.1002/adma.202200179
Ervolino, E., Statkievicz, C., Toro, L. F., de Mello-Neto, J. M., Cavazana, T. P., Issa, J. P. M., et al. (2019). Antimicrobial photodynamic therapy improves the alveolar repair process and prevents the occurrence of osteonecrosis of the jaws after tooth extraction in senile rats treated with zoledronate. Bone 120, 101–113. doi: 10.1016/j.bone.2018.10.014
Fan, X., Yang, F., Nie, C., Ma, L., Cheng, C., and Haag, R. (2021). Biocatalytic Nanomaterials: a new pathway for bacterial disinfection. Adv. Mater. 33:e2100637. doi: 10.1002/adma.202100637
Freire, A. E. N., Macedo Iunes Carrera, T., de Oliveira, G., Pigossi, S. C., and Vital Ribeiro Junior, N. (2020). Comparison between antimicrobial photodynamic therapy and low-level laser therapy on non-surgical periodontal treatment: a clinical study. Photodiagn. Photodyn. Ther. 31:101756. doi: 10.1016/j.pdpdt.2020.101756
Gao, Q., Huang, D. N., Deng, Y. Y., Yu, W. J., Jin, Q., Ji, J., et al. (2021). Chlorin e6 (Ce6)-loaded supramolecular polypeptide micelles with enhanced photodynamic therapy effect against Pseudomonas aeruginosa. Chem. Eng. J. 417:129334. doi: 10.1016/j.cej.2021.129334
Garcia, M. T., Ward, R., Goncalves, N. M. F., Pedroso, L. L. C., Neto, J., Strixino, J. F., et al. (2021). Susceptibility of dental caries microcosm biofilms to photodynamic therapy mediated by Fotoenticine. Pharmaceutics 13:1907. doi: 10.3390/pharmaceutics13111907
Golmohamadpour, A., Bahramian, B., Khoobi, M., Pourhajibagher, M., Barikani, H. R., and Bahador, A. (2018). Antimicrobial photodynamic therapy assessment of three indocyanine green-loaded metal-organic frameworks against Enterococcus faecalis. Photodiagn. Photodyn. Ther. 23, 331–338. doi: 10.1016/j.pdpdt.2018.08.004
Hamblin, M. R. (2016). Antimicrobial photodynamic inactivation: a bright new technique to kill resistant microbe. Curr. Opin. Microbiol. 33, 67–73. doi: 10.1016/j.mib.2016.06.008
He, Y., Leng, J., Li, K., Xu, K., Lin, C., Yuan, Z., et al. (2021). A multifunctional hydrogel coating to direct fibroblast activation and infected wound healing via simultaneously controllable photobiomodulation and photodynamic therapies. Biomaterials 278:121164. doi: 10.1016/j.biomaterials.2021.121164
Hu, B., Berkey, C., Feliciano, T., Chen, X., Li, Z., Chen, C., et al. (2020). Thermal-disrupting Interface mitigates intercellular cohesion loss for accurate topical antibacterial therapy. Adv. Mater. 32:e1907030. doi: 10.1002/adma.201907030
Hu, Y. Q., Wang, W. B., Huang, S. Y., Li, J., Zhang, Y. F., Gao, Y. C., et al. (2022). A targeted nanozyme based on multiple porphyrins for enhanced photodynamic antibacterial application. Chem. Eng. J. 431:133704. doi: 10.1016/j.cej.2021.133704
Huang, Y., Gao, Q., Li, X., Gao, Y. F., Han, H. J., Jin, Q., et al. (2020). Ofloxacin loaded MoS2 nanoflakes for synergistic mild-temperature photothermal/antibiotic therapy with reduced drug resistance of bacteria. Nano Res. 13, 2340–2350. doi: 10.1007/s12274-020-2853-2
Huang, S., Xu, S., Hu, Y., Zhao, X., Chang, L., Chen, Z., et al. (2022). Preparation of NIR-responsive, ROS-generating and antibacterial black phosphorus quantum dots for promoting the MRSA-infected wound healing in diabetic rats. Acta Biomater. 137, 199–217. doi: 10.1016/j.actbio.2021.10.008
Im, B. N., Shin, H., Lim, B., Lee, J., Kim, K. S., Park, J. M., et al. (2021). Helicobacter pylori-targeting multiligand photosensitizer for effective antibacterial endoscopic photodynamic therapy. Biomaterials 271:120745. doi: 10.1016/j.biomaterials.2021.120745
Jiang, Q. E. F., Tian, J., Yang, J., Zhang, J., and Cheng, Y. (2020). Light-excited antibiotics for potentiating bacterial killing via reactive oxygen species generation. ACS Appl. Mater. Inter. 12, 16150–16158. doi: 10.1021/acsami.0c02647
Khan, I., Tang, E., and Arany, P. (2015). Molecular pathway of near-infrared laser phototoxicity involves ATF-4 orchestrated ER stress. Sci. Rep. 5:10581. doi: 10.1038/srep10581
Li, X., Lee, D., Huang, J. D., and Yoon, J. (2018). Phthalocyanine-assembled Nanodots as photosensitizers for highly efficient type I photoreactions in photodynamic therapy. Angew. Chem. Int. Ed. Engl. 57, 9885–9890. doi: 10.1002/anie.201806551
Li, J., Liu, X., Tan, L., Cui, Z., Yang, X., Liang, Y., et al. (2019). Zinc-doped Prussian blue enhances photothermal clearance of Staphylococcus aureus and promotes tissue repair in infected wounds. Nat. Commun. 10:4490. doi: 10.1038/s41467-019-12429-6
Li, Z., Lu, S., Liu, W. Z., Dai, T., Ke, J. X., Li, X. J., et al. (2021b). Synergistic lysozyme-photodynamic therapy against resistant bacteria based on an intelligent upconversion nanoplatform. Angew. Chem. Int. Edit. 60, 19201–19206. doi: 10.1002/anie.202103943
Li, Z., Pan, W., Shi, E., Bai, L., Liu, H., Li, C., et al. (2021a). A multifunctional nanosystem based on bacterial cell-penetrating photosensitizer for fighting periodontitis via combining photodynamic and antibiotic therapies. ACS Biomater Sci. Eng. 7, 772–786. doi: 10.1021/acsbiomaterials.0c01638
Li, J., Song, S., Meng, J., Tan, L., Liu, X., Zheng, Y., et al. (2021). 2D MOF periodontitis photodynamic ion therapy. J. Am. Chem. Soc. 143, 15427–15439. doi: 10.1021/jacs.1c07875
Lin, J., He, Z., Liu, F., Feng, J., Huang, C., Sun, X., et al. (2020). Hybrid hydrogels for synergistic periodontal antibacterial treatment with sustained drug release and NIR-responsive photothermal effect. Int. J. Nanomedicine Volume 15, 5377–5387. doi: 10.2147/IJN.S248538
Lin, H. X., Lin, Z. X., Zheng, K. H., Wang, C. L., Lin, L. S., Chen, J. X., et al. (2021). Near-infrared-II nanomaterials for fluorescence imaging and photodynamic therapy. Adv. Opt. Mater. 9:2002177. doi: 10.1002/adom.202002177
Liu, Y. N., Li, F., Guo, Z. R., Xiao, Y. Q., Zhang, Y. L., Sun, X. Y., et al. (2020). Silver nanoparticle-embedded hydrogel as a photothermal platform for combating bacterial infections. Chem. Eng. J. 382:122990. doi: 10.1016/j.cej.2019.122990
Liu, D., Ma, X., Ji, Y., Chen, R., Zhou, S., Yao, H., et al. (2022). Bioresponsive nanotherapy for preventing dental caries by inhibiting multispecies cariogenic biofilms. Bioact. Mater. 14, 1–14. doi: 10.1016/j.bioactmat.2021.12.016
Liu, Y. X., Tian, Y., Han, Q. Y., Yin, J., Zhang, J. C., Yu, Y., et al. (2021). Synergism of 2D/1D MXene/cobalt nanowire heterojunctions for boosted photo-activated antibacterial application. Chem. Eng. J. 410:128209. doi: 10.1016/j.cej.2020.128209
Liu, S., Yuan, H., Bai, H., Zhang, P., Lv, F., Liu, L., et al. (2018). Electrochemiluminescence for electric-driven antibacterial therapeutics. J. Am. Chem. Soc. 140, 2284–2291. doi: 10.1021/jacs.7b12140
Liu, W., Zhang, Y., You, W., Su, J., Yu, S., Dai, T., et al. (2020). Near-infrared-excited upconversion photodynamic therapy of extensively drug-resistant Acinetobacter baumannii based on lanthanide nanoparticles. Nanoscale 12, 13948–13957. doi: 10.1039/d0nr01073a
Liu, Z., Zhao, X., Yu, B., Zhao, N., Zhang, C., and Xu, F. J. (2021). Rough carbon-iron oxide Nanohybrids for near-infrared-II light-responsive synergistic antibacterial therapy. ACS Nano 15, 7482–7490. doi: 10.1021/acsnano.1c00894
Lopes, C. B., Motta, P. B., Campos, T. M., Leite, D. P. V., Araki Yamamoto, A. T., Mota, M. S. A., et al. (2022). Protocol for the clinical practice of photodynamic therapy in endodontics: assessment of guideline quality using the AGREE II instrument. Photodiagn. Photodyn. Ther. 38:102835. doi: 10.1016/j.pdpdt.2022.102835
Ma, Y., Xu, H., Sun, B., Du, S., Cui, S., Zhang, L., et al. (2021). pH-responsive oxygen and hydrogen peroxide self-supplying Nanosystem for photodynamic and Chemodynamic therapy of wound infection. Acs Appl. Mater. Inter. 13, 59720–59730. doi: 10.1021/acsami.1c19681
Maisch, T. (2020). Photoantimicrobials—an update. Transl. Biophotonics 2:e201900033. doi: 10.1002/tbio.201900033
Mo, S., Zhao, Y., Wen, J., Sun, J., Zhang, Z., Yu, Q., et al. (2022). Efficient photothermal and photodynamic synergistic antibacterial therapy of Cu7S4 nanosheets regulated by facet engineering. J. Hazard. Mater. 432:128662. doi: 10.1016/j.jhazmat.2022.128662
Nie, R., Sun, Y., Lv, H., Lu, M., Huangfu, H., Li, Y., et al. (2022). 3D printing of MXene composite hydrogel scaffolds for Photothermal antibacterial activity and bone regeneration in infected bone defect models. Nanoscale 14, 8112–8129. doi: 10.1039/D2NR02176E
Papapanou, P. N., Sanz, M., Buduneli, N., Dietrich, T., Feres, M., Fine, D. H., et al. (2018). Periodontitis: consensus report of workgroup 2 of the 2017 world workshop on the classification of periodontal and Peri-implant diseases and conditions. J. Clin. Periodontol. 45, S162–S170. doi: 10.1111/jcpe.12946
Qi, M. L., Ren, X., Li, W., Sun, Y., Sun, X. L., Li, C. Y., et al. (2022). NIR responsive nitric oxide nanogenerator for enhanced biofilm eradication and inflammation immunotherapy against periodontal diseases. Nano Today 43:101447. doi: 10.1016/j.nantod.2022.101447
Reis, A. C. M., Regis, W. F. M., and Rodrigues, L. K. A. (2019). Scientific evidence in antimicrobial photodynamic therapy: An alternative approach for reducing cariogenic bacteria. Photodiagn. Photodyn. Ther. 26, 179–189. doi: 10.1016/j.pdpdt.2019.03.012
Schuenck-Rodrigues, R. A., de Oliveira de Siqueira, L. B., Dos Santos Matos, A. P., da Costa, S. P., da Silva Cardoso, V., Vermelho, A. B., et al. (2020). Development, characterization and photobiological activity of nanoemulsion containing zinc phthalocyanine for oral infections treatment. J. Photochem. Photobiol. B 211:112010. doi: 10.1016/j.jphotobiol.2020.112010
Shi, E., Bai, L., Mao, L., Wang, H., Yang, X., Wang, Y., et al. (2021). Self-assembled nanoparticles containing photosensitizer and polycationic brush for synergistic photothermal and photodynamic therapy against periodontitis. J. Nanobiotechnol. 19:413. doi: 10.1186/s12951-021-01114-w
Sun, J., Fan, Y., Ye, W., Tian, L. M., Niu, S. C., Ming, W. H., et al. (2021). Near-infrared light triggered photodynamic and nitric oxide synergistic antibacterial nanocomposite membrane. Chem. Eng. J. 417:128049. doi: 10.1016/j.cej.2020.128049
Sun, Y., Sun, X., Li, X., Li, W., Li, C., Zhou, Y., et al. (2021). A versatile nanocomposite based on nanoceria for antibacterial enhancement and protection from aPDT-aggravated inflammation via modulation of macrophage polarization. Biomaterials 268:120614. doi: 10.1016/j.biomaterials.2020.120614
Sun, X., Sun, J., Sun, Y., Li, C., Fang, J., Zhang, T., et al. (2021). Oxygen self-sufficient Nanoplatform for enhanced and selective antibacterial photodynamic therapy against anaerobe-induced periodontal disease. Adv. Funct. Mater. 31:2101040. doi: 10.1002/adfm.202101040
Sun, X., Wang, L., Lynch, C. D., Sun, X., Li, X., Qi, M., et al. (2019). Nanoparticles having amphiphilic silane containing Chlorin e6 with strong anti-biofilm activity against periodontitis-related pathogens. J. Dent. 81, 70–84. doi: 10.1016/j.jdent.2018.12.011
Tang, E., Khan, I., Andreana, S., and Arany, P. R. (2017). Laser-activated transforming growth factor-beta1 induces human beta-defensin 2: implications for laser therapies for periodontitis and peri-implantitis. J. Periodontal Res. 52, 360–367. doi: 10.1111/jre.12399
Tian, Y., Li, Y., Liu, J., Lin, Y., Jiao, J., Chen, B., et al. (2022). Photothermal therapy with regulated Nrf2/NF-kappaB signaling pathway for treating bacteria-induced periodontitis. Bioact. Mater. 9, 428–445. doi: 10.1016/j.bioactmat.2021.07.033
Tong, X., Qi, X., Mao, R., Pan, W., Zhang, M., Wu, X., et al. (2020). Construction of functional curdlan hydrogels with bio-inspired polydopamine for synergistic periodontal antibacterial therapeutics. Carbohydr. Polym. 245:116585. doi: 10.1016/j.carbpol.2020.116585
Van Acker, H., and Coenye, T. (2017). The role of reactive oxygen species in antibiotic-mediated killing of bacteria. Trends Microbiol. 25, 456–466. doi: 10.1016/j.tim.2016.12.008
Vila-Nova, T. E. L., Leao, R. S., Santiago Junior, J. F., Pellizzer, E. P., Vasconcelos, B., and Moraes, S. L. D. (2022). Photodynamic therapy in the treatment of denture stomatitis: A systematic review and meta-analysis. J. Prosthet. Dent. in press. doi: 10.1016/j.prosdent.2021.11.028
Wang, H., Li, W., Zhang, D., Li, W., and Wang, Z. (2019). Adjunctive photodynamic therapy improves the outcomes of peri-implantitis: a randomized controlled trial. Aust. Dent. J. 64, 256–262. doi: 10.1111/adj.12705
Wang, X., Shi, Q., Zha, Z., Zhu, D., Zheng, L., Shi, L., et al. (2021). Copper single-atom catalysts with photothermal performance and enhanced nanozyme activity for bacteria-infected wound therapy. Bioact. Mater. 6, 4389–4401. doi: 10.1016/j.bioactmat.2021.04.024
Wang, W. N., Zhang, C. Y., Zhang, M. F., Pei, P., Zhou, W., Zha, Z. B., et al. (2020). Precisely photothermal controlled releasing of antibacterial agent from Bi2S3 hollow microspheres triggered by NIR light for water sterilization. Chem. Eng. J. 381:122630. doi: 10.1016/j.cej.2019.122630
Wang, B., Zhou, L., Guo, Y., Guo, H., Zhong, Y., Huang, X., et al. (2022). Cyanobacteria-based self-oxygenated photodynamic therapy for anaerobic infection treatment and tissue repair. Bioact. Mater. 12, 314–326. doi: 10.1016/j.bioactmat.2021.10.032
Xie, X. H., Wang, R., Zhang, X. X., Ren, Y. R., Du, T., Ni, Y. S., et al. (2021). A photothermal and self-induced Fenton dual-modal antibacterial platform for synergistic enhanced bacterial elimination. Appl. Catal. B-Environ. 295:120315. doi: 10.1016/j.apcatb.2021.120315
Xu, X., Fan, M., Yu, Z., Zhao, Y., Zhang, H., Wang, J., et al. (2022). A removable photothermal antibacterial “warm paste” target for cariogenic bacteria. Chem. Eng. J. 429:132491. doi: 10.1016/j.cej.2021.132491
Xu, B., Li, Z., Li, G., Xu, M., Li, Y., Liu, L., et al. (2022). Mild photothermal effect of titania nanotubes array as a promising solution for peri-implantitis. Mater. Design 217:110641. doi: 10.1016/j.matdes.2022.110641
Yang, Y., Deng, Y. Y., Huang, J. B., Fan, X., Cheng, C., Nie, C. X., et al. (2019). Size-transformable metal-organic framework-derived Nanocarbons for localized chemo-Photothermal bacterial ablation and wound disinfection. Adv. Funct. Mater. 29:1900143. doi: 10.1002/adfm.201900143
Yang, Y., Ma, L., Cheng, C., Deng, Y. Y., Huang, J. B., Fan, X., et al. (2018). Nonchemotherapic and robust dual-responsive Nanoagents with on-demand bacterial trapping, ablation, and release for efficient wound disinfection. Adv. Funct. Mater. 28:1705708. doi: 10.1002/adfm.201705708
Ye, Y., He, J., Qiao, Y., Qi, Y., Zhang, H., Santos, H. A., et al. (2020). Mild temperature photothermal assisted anti-bacterial and anti-inflammatory nanosystem for synergistic treatment of post-cataract surgery endophthalmitis. Theranostics 10, 8541–8557. doi: 10.7150/thno.46895
Yu, Y., Zhang, Y., Cheng, Y., Wang, Y., Chen, Z., Sun, H., et al. (2022). NIR-activated nanosystems with self-modulated bacteria targeting for enhanced biofilm eradication and caries prevention. Bioact. Mater. 13, 269–285. doi: 10.1016/j.bioactmat.2021.10.035
Zeng, J. K., Wang, Y. T., Sun, Z. Y., Chang, H. S., Cao, M., Zhao, J., et al. (2020). A novel biocompatible PDA/IR820/DAP coating for antibiotic/photodynamic/photothermal triple therapy to inhibit and eliminate Staphylococcus aureus bio film. Chem. Eng. J. 394:125017. doi: 10.1016/j.cej.2020.125017
Zhang, Y., Dai, T., Wang, M., Vecchio, D., Chiang, L. Y., Maisch, T., et al. (2015). Potentiation of antimicrobial photodynamic inactivation mediated by a cationic fullerene by added iodide: in vitro and in vivo studies. Nanomedicine 10, 603–614. doi: 10.2217/nnm.14.131
Zhang, J. C., Gao, X. Y., Ma, D. C., He, S., Du, B. W., Yang, W. Z., et al. (2021). Copper ferrite heterojunction coatings empower polyetheretherketone implant with multi-modal bactericidal functions and boosted osteogenicity through synergistic photo/Fenton-therapy. Chem. Eng. J. 422:130094. doi: 10.1016/j.cej.2021.130094
Zhang, L., Wang, Y., Wang, C., He, M., Wan, J., Wei, Y., et al. (2020). Light-Activable on-demand release of Nano-antibiotic platforms for precise synergy of Thermochemotherapy on periodontitis. ACS Appl. Mater. Inter. 12, 3354–3362. doi: 10.1021/acsami.9b17335
Zhang, G., Yang, Y., Shi, J., Yao, X., Chen, W., Wei, X., et al. (2021). Near-infrared light II - assisted rapid biofilm elimination platform for bone implants at mild temperature. Biomaterials 269:120634. doi: 10.1016/j.biomaterials.2020.120634
Zhang, T., Ying, D., Qi, M., Li, X., Fu, L., Sun, X., et al. (2019). Anti-biofilm property of bioactive Upconversion Nanocomposites containing Chlorin e6 against periodontal pathogens. Molecules 24, 2692–2709. doi: 10.3390/molecules24152692
Zhou, B., Sun, X., Dong, B., Yu, S., Cheng, L., Hu, S., et al. (2022). Antibacterial PDT nanoplatform capable of releasing therapeutic gas for synergistic and enhanced treatment against deep infections. Theranostics 12, 2580–2597. doi: 10.7150/thno.70277
Keywords: photonics, oral infectious diseases, antibacterial photodynamic therapy, photothermal therapy, photobiomodulation
Citation: Huang S, Qi M and Chen Y (2023) Photonics-based treatments: Mechanisms and applications in oral infectious diseases. Front. Microbiol. 14:948092. doi: 10.3389/fmicb.2023.948092
Received: 19 May 2022; Accepted: 19 January 2023;
Published: 10 February 2023.
Edited by:
Fabian Cieplik, University Medical Center Regensburg, GermanyReviewed by:
Praveen Arany, University at Buffalo, United StatesCopyright © 2023 Huang, Qi and Chen. This is an open-access article distributed under the terms of the Creative Commons Attribution License (CC BY). The use, distribution or reproduction in other forums is permitted, provided the original author(s) and the copyright owner(s) are credited and that the original publication in this journal is cited, in accordance with accepted academic practice. No use, distribution or reproduction is permitted which does not comply with these terms.
*Correspondence: Manlin Qi, ✉ cW1sMTk5MkAxMjYuY29t;
†These authors have contributed equally to this work
Disclaimer: All claims expressed in this article are solely those of the authors and do not necessarily represent those of their affiliated organizations, or those of the publisher, the editors and the reviewers. Any product that may be evaluated in this article or claim that may be made by its manufacturer is not guaranteed or endorsed by the publisher.
Research integrity at Frontiers
Learn more about the work of our research integrity team to safeguard the quality of each article we publish.