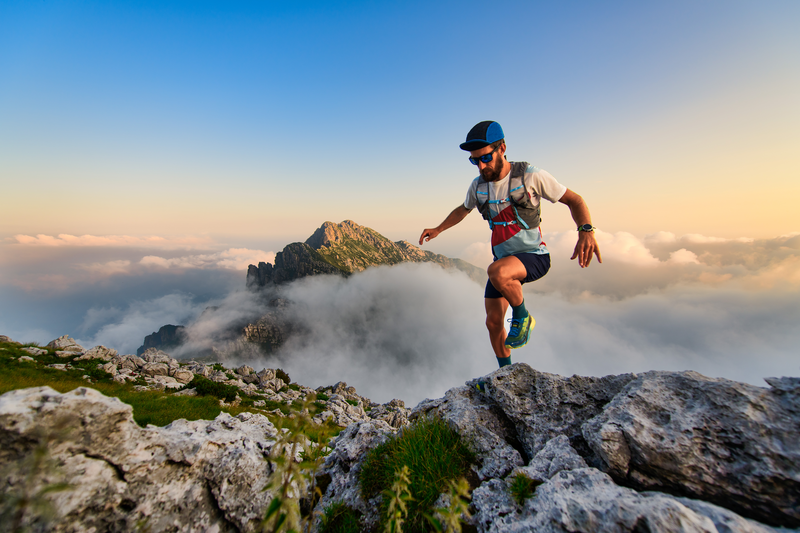
94% of researchers rate our articles as excellent or good
Learn more about the work of our research integrity team to safeguard the quality of each article we publish.
Find out more
EDITORIAL article
Front. Microbiol. , 08 January 2024
Sec. Systems Microbiology
Volume 14 - 2023 | https://doi.org/10.3389/fmicb.2023.1357125
This article is part of the Research Topic Environments-Pathogens-The Gut Microbiota and Host Diseases View all 12 articles
Editorial on the Research Topic
Environments-pathogens-the gut microbiota and host diseases
Half a century ago, the tripartite interaction “disease is the outcome of imbalanced interplay among host, gut microbiome, and environmental variables” [SIC] was proposed (Snieszko, 1974). This paradigm has been supported by subsequent studies, which have revealed that the gut microbiota is a central hub that integrates environmental exposures with host genetic and immune signals, thereby determining host health outcomes (Kamada et al., 2013; Xiong et al., 2019). In particular, the transplantation of the gut microbiota from diseased individuals to healthy recipients induces the same disease and vice versa, revealing the causal role of dysbiosis in the gut microbiota and host health (Huang et al., 2020; Pandey et al., 2023). In this scenario, targeting the gut microbiota is a promising way to improve host health and treat disease. To achieve this, however, requires prior knowledge of the factors that affect the host gut microbiome. In addition, the “one pathogen, one disease” or “one virulence gene, one disease” paradigm is insufficient to validate the causal roles of polymicrobial pathogens in a disease. Accordingly, “ecological Koch's postulates” (one dysbiosis, one gut microbiota, one disease) are proposed to interpret these infectious diseases (Pascale et al., 2018; Xiong, 2018). It is apparent that the term “dysbiosis” is too simple and vague to explain complex disease states, thereby limiting the mechanistic understanding of etiology and the definition of causal agents. Therefore, unraveling the mechanisms behind dysbiosis is fundamental to better comprehending disease progression within a “pathobiome” concept and ultimately to guiding strategies for disease prevention.
Recently, ecological approaches have been applied to identify polymicrobial pathogens and their etiologies. For example, by integrating ecological features of primary colonizers, keystone taxa that drive gut networks from healthy to diseased cohorts, biomarkers of health status, as well as their encoding virulence gene, polymicrobial pathogens are inferred for shrimp white feces syndrome (Lu et al., 2020), coral white band disease (Gignoux-Wolfsohn et al., 2017), and human carcinogenesis (Cai et al., 2023). In addition, interkingdom phagotroph predator-prey interactions intimately kill the winning pathogens, thereby sustaining host health (Lu et al., 2022; Wu et al., 2022). A common observation is that disease symptoms lag far behind disease onset. Unfortunately, once disease symptoms appear, it is difficult to regain health in advanced disease stages, including shrimp and fish diseases (Xiong et al., 2017; Mougin and Joyce, 2023), and especially human cancers (Sung et al., 2021). There is evidence that disruption in the gut microbiota gradually worsens during disease progression, preceding host disease symptoms (Xiong et al., 2017; Shen et al., 2021; Cai et al., 2023). In fact, the gut microbiome is a key etiological element in the onset and progression of disease (Feng et al., 2015; Xiong et al., 2017). For these reasons, early detection of these adverse disorders in the gut microbiota is of paramount importance for predicting disease incidence in the host. The disease onset stage provides a better opportunity for disease biocontrol, such as antagonizing probiotics, synbiotics (Xiong, 2018; Goh et al., 2022), and even personalized treatments (Rodríguez-Fernández et al., 2022), although the designation of gut microbiota-based therapies is still a slow journey to primetime.
In light of the above-mentioned concerns, this Research Topic aimed to explore recent developments in this area with a focus on (1) exploring the underlying mechanisms governing the interrelationships between Environments-Pathogens-The gut microbiota and host disease from a molecular and ecological perspective; (2) identifying and validating causative relationships between polymicrobial pathogens and host disease progression; and (3) establishing approaches for diagnosing the incidence and/or outcome of disease, especially in the early (“subclinical”) stages, and biocontrol strategies for preventing host disease.
In one study, Liao et al. explore how host-gut microbiota interactions respond to decapod iridescent virus 1 (DIV1) infection using a lethal concentration 50 (LC50) assay. DIV1 infection causes dose-dependent mortality in shrimp (Metapenaeus ensis). In this study, the authenticity of the gut transcriptome is selectively validated by RT-qPCR, revealing that the expression patterns of the tested genes are comparable between mRNA sequencing and qPCR. Thus, RNA-Seq-assayed gene expression profiles are reliable for evaluating the effects of DIV1 infection on the shrimp transcriptome. DIV1 infection activates pathways involved in virus invasion, replication, and host antiviral infection, including lncRNAs. Specifically, shrimp fight DIV1 infection by potentiating the expression of the Wnt signaling pathway, the p53 signaling pathway, the C-type lectin receptor signaling pathway, and others. However, DIV1 infection up-regulates shrimp NF-κB inhibitor cactus-like and toll-interacting proteins through the proliferation of gut pathogenic Vibrio and Photobacterium genera, which suppress the toll-like receptor (TLR)-mediated immune response. Invertebrate shrimp cells recognize pathogen-associated molecular patterns on microbial pathogens through TLRs; thus, the inhibited TLRs create a favorable condition for immune escape and further DIV1 infection.
A second study by Su et al. uses publicly available datasets with large sample sizes of gut microbiota and gut microbial metabolites, thereby enabling them to obtain precise estimates and high statistical power. In this study, gut microbiota and gut microbial metabolites are deployed as exposure, while host health (here, low back pain, LBP) is used as the outcome. To correct for measured confounders, a multivariate Mendelian randomization analysis is conducted to obtain causal inferences between gut microbiota, gut microbial metabolites, and LBP outcome. As a result, 20 gut bacterial taxa and 2 gut microbial metabolites causally affecting LBP are examined. The results are consistent with the most available evidence. The workflow provides a causal effect of the gut microbiota-mediated mechanism of host health. Using similar procedures, Shi et al. identify causal relationships between gut bacterial taxa and five chronic respiratory diseases.
A review by Guevara-Ramírez et al. summarizes intrinsic and extrinsic factors that affect gut microbiota and hematologic cancer. Intrinsic variables, including host genetics, immunity, age, and health status, govern the gut microbiome. For example, host genetics can modify the expression of microbial receptors, determining the establishment of specific microbial species. Immune disorders disrupt microbial imbalances that contribute to carcinogenesis. Extrinsic variables, such as environmental exposures, diet, lifestyle, anticancer therapy, and stress, also affect the gut microbiota. Lymphoma is marked by an increased abundance of Escherichia coli and Clostridium butyricum, while leukemia is characterized by a decreased abundance of Lachnospiraceae and Ruminococcaceae. In contrast, myeloma is characterized by an enrichment of Pseudomonas aeruginosa and Clostridium leptum strains, with higher levels of C. leptum in the advanced stages of myeloma. C. leptum is a producer of butyrate that suppresses interleukin 17 (IL-17). Based on this knowledge, treatment with antibiotics or antibodies that block IL-17/IL-17R interactions delays myeloma progression. Thus, disease-specific strains may indicate different types and/or stages of hematologic disease. Moreover, the biology of disease-specific strains could guide the identification of therapeutic strategies.
Recently, there has been increasing evidence for the role of gut microbes in promoting host health and preventing host disease through biocontrol strategies. In support of this, Chen et al. investigate how different dietary compositions affect the gut microbiota and digestive enzyme activities of black soldier fly larvae. High-protein, high-fat, and high-starch diets significantly alter the gut microbiota, leading to subsequent changes in digestive enzyme activities. Among these diets, a high-oil diet stimulates the diversity of gut microbiota, which is associated with better growth and survival of black soldier fly larvae. By this logic, oil supplementation could improve the performance of black soldier flies in treating food waste for environmental protection. Geo et al. use Qishen Granule (QSG) to treat rats with heart failure. QSG significantly enriches gut Bacteroidetes and Prevotellaceae populations, thereby improving gut structure and barrier protection. In addition, QSG arranges mitochondria, alleviates swelling, and improves crest structural integrity. As a result, cardiac function and cardiomyocyte alignment are improved in heart failure rats. Therefore, QSG may potentiate cardiac function by regulating gut microecology, providing a viable therapeutic strategy for heart failure. Moreover, a review by Feng et al. summarizes the application of probiotics in the treatment of autism spectrum disorders (ASD) in humans from the perspective of a gut-brain axis. There are strong and positive associations between gut microbiota dysbiosis, gastrointestinal abnormalities, and ASD symptom severity. Dysbiosis in the gut microbiota causes immune system disorders and gut microbial metabolites. For example, elevated gut Clostridia species and Pseudomonas stutzeri strains produce high levels of p-cresol in individuals with ASD, leading to restricted social behavior and recognition. Conversely, suppressed gut Lactobacillus reuteri and Bacteroides dentium cause a reduction in aminobutyric acid, resulting in anxiety and depression-like behavior and stress responsiveness. Accordingly, supplementation with B. longum ameliorates microglia activity. Lactobacillus strains reverse valproic acid-induced apoptosis and degeneration in the cerebellum. Thus, probiotic supplementation improves ASD by regulating the gut–brain axis. Currently, an established probiotic protocol is lacking, resulting in a diversity of probiotic strains, concentrations, and treatment frequencies among studies. However, preclinical evidence has demonstrated recovery of brain function and improvement in ASD after probiotic supplementation.
In conclusion, this Research Topic provides multidisciplinary knowledge on host-gut microbiota and gut microbial metabolites in response to disease and potential therapeutic strategies, such as a high-oil diet, QSG, and probiotics. Emphasis is placed on strategies targeting the gut microbiome to mitigate host disease. Nevertheless, the available data are cross-sectional, thus limiting the capacity to establish a cause-and-effect relationship between the gut microbiota and host health. Therefore, conducting longitudinal studies that integrate the microbiota across disease progression is essential to gaining a comprehensive understanding of this causal relationship. In addition, although the gut microbiome data have been deposited in public databases, the related covariates are descriptive or missing, making additional subgroup analyses necessary to rule out the confounders in an unbiased manner.
JX: Conceptualization, Project administration, Writing—original draft, Writing—review & editing. ZS: Writing—original draft.
The author(s) declare financial support was received for the research, authorship, and/or publication of this article. This work was funded by the National Natural Science Foundation of China (32071549, 32371596, U23A200206, and 32061123004), the K.C. Wong Magna Fund in Ningbo University, the Fundamental Research Funds for the Leading Scientist Project of Qinghai Province (2023-NK-147), and the Open Fund Project of Guangxi Key Laboratory of Precision Medicine for Genetic Diseases (GXWCH-ZDKF-2022-15, Maternal and Child Health Hospital of Guangxi Zhuang Autonomous Region).
We deeply appreciate all the authors and reviewers who have contributed to this Research Topic.
The authors declare that the research was conducted in the absence of any commercial or financial relationships that could be construed as a potential conflict of interest.
The author(s) declared that they were an editorial board member of Frontiers, at the time of submission. This had no impact on the peer review process and the final decision.
All claims expressed in this article are solely those of the authors and do not necessarily represent those of their affiliated organizations, or those of the publisher, the editors and the reviewers. Any product that may be evaluated in this article, or claim that may be made by its manufacturer, is not guaranteed or endorsed by the publisher.
Cai, P., Xiong, J., Sha, H., Dai, X., and Lu, J. (2023). Tumor bacterial markers diagnose the initiation and four stages of colorectal cancer. Front. Cell. Infect. Microbiol. 13, 1123544. doi: 10.3389/fcimb.2023.1123544
Feng, Q., Liang, S., Jia, H., Stadlmayr, A., Tang, L., Lan, Z., et al. (2015). Gut microbiome development along the colorectal adenoma-carcinoma sequence. Nat. Commun. 6, 6528. doi: 10.1038/ncomms7528
Gignoux-Wolfsohn, S. A., Aronson, F. M., and Vollmer, S. V. (2017). Complex interactions between potentially pathogenic, opportunistic, and resident bacteria emerge during infection on a reef-building coral. FEMS Microbiol. Ecol. 93, fix080. doi: 10.1093/femsec/fix080
Goh, J. H., Loh, T. T., Law, J. F., Ser, H. L., Khaw, K., Letchumanan, V., et al. (2022). Harnessing the potentialities of probiotics, prebiotics, synbiotics, paraprobiotics, and postbiotics for shrimp farming. Rev. Aquac. 14, 1478–1557. doi: 10.1111/raq.12659
Huang, Z., Zeng, S., Xiong, J., Hou, D., Zhou, R., Xing, C., et al. (2020). Microecological Koch's postulates reveal that intestinal microbiota dysbiosis contributes to shrimp white feces syndrome. Microbiome 8, 32. doi: 10.1186/s40168-020-00802-3
Kamada, N., Seo, S. U., Chen, G. Y., and Núñez, G. (2013). Role of the gut microbiota in immunity and inflammatory disease. Nat. Rev. Immunol. 13, 321–335. doi: 10.1038/nri3430
Lu, J., Li, X., Qiu, Q., Chen, J., and Xiong, J. (2022). Gut interkingdom predator-prey interactions are key determinants of shrimp health. Aquaculture 546, 737304. doi: 10.1016/j.aquaculture.2021.737304
Lu, J., Zhang, X., Qiu, Q., Chen, J., and Xiong, J. (2020). Identifying potential polymicrobial pathogens: Moving beyond differential abundance to driver taxa. Microb. Ecol. 80, 447–458. doi: 10.1007/s00248-020-01511-y
Mougin, J., and Joyce, A. (2023). Fish disease prevention via microbial dysbiosis-associated biomarkers in aquaculture. Rev. Aquac. 15, 579–594. doi: 10.1111/raq.12745
Pandey, H., Jain, D., Tang, D., Wong, S., and Lal, D. (2023). Gut microbiota in pathophysiology, diagnosis, and therapeutics of inflammatory bowel disease. Intestinel Research doi: 10.5217/ir.2023.00080
Pascale, V., Mark, A., and Sansonetti, P. J. (2018). Pathogens, microbiome and the host: emergence of the ecological Koch's postulates. FEMS Microbiol. Rev.42, fuy003. doi: 10.1093/femsre/fuy003
Rodríguez-Fernández, C., Iglesias, M., de Domingo, B., Conde-Pérez, K., Vallejo, J., Rodríguez-Martínez, L., et al. (2022). Microbiome in immune-mediated uveitis. Int. J. Mol. Sci. 33, 7020.
Shen, H., Song, T., Lu, J., Qiu, Q., Chen, J., and Xiong, J. (2021). Shrimp AHPND causing Vibrio anguillarum infection: quantitative diagnosis and identifying antagonistic bacteria. Mar. Biotechnol. 23, 964–975. doi: 10.1007/s10126-021-10079-8
Snieszko, S. F. (1974). The effects of environmental stress on outbreaks of infectious diseases of fishes. J. Fish Biol. 6, 197–208. doi: 10.1111/j.1095-8649.1974.tb04537.x
Sung, H., Ferlay, J., Siegel, R. L., Laversanne, M., Soerjomataram, I., Jemal, A., et al. (2021). Global cancer statistics 2020: GLOBOCAN Estimates of incidence and mortality worldwide for 36 cancers in 185 countries. CA: Cancer J. Clin.71, 209–249. doi: 10.3322/caac.21660
Wu, C., Ge, C., Wang, F., Zhang, H., Zhu, Z., Lesueur, D., et al. (2022). Phagotrophic protist-mediated control of Polymyxa graminisin the wheat rhizosphere. Plant Soil 485, 333–347. doi: 10.1007/s11104-022-05829-z
Xiong, J. (2018). Progress in the gut microbiota in exploring shrimp disease pathogenesis and incidence. Appl. Microbiol. Biotechnol. 102, 7343–7350. doi: 10.1007/s00253-018-9199-7
Xiong, J., Nie, L., and Chen, J. (2019). Current understanding on the roles of gut microbiota in fish disease and immunity. Zool. Res. 40, 70. doi: 10.24272/j.issn.2095-8137.2018.069
Keywords: gut microbiome, host health, bioindicators, causal relationships, therapeutic strategies
Citation: Xiong J and Shi Z (2024) Editorial: Environments-pathogens-the gut microbiota and host diseases. Front. Microbiol. 14:1357125. doi: 10.3389/fmicb.2023.1357125
Received: 17 December 2023; Accepted: 20 December 2023;
Published: 08 January 2024.
Edited and reviewed by: Matthias Hess, University of California, Davis, United States
Copyright © 2024 Xiong and Shi. This is an open-access article distributed under the terms of the Creative Commons Attribution License (CC BY). The use, distribution or reproduction in other forums is permitted, provided the original author(s) and the copyright owner(s) are credited and that the original publication in this journal is cited, in accordance with accepted academic practice. No use, distribution or reproduction is permitted which does not comply with these terms.
*Correspondence: Jinbo Xiong, eGlvbmdqaW5ib0BuYnUuZWR1LmNu; Zunji Shi, c2hpempAbHp1LmVkdS5jbg==
Disclaimer: All claims expressed in this article are solely those of the authors and do not necessarily represent those of their affiliated organizations, or those of the publisher, the editors and the reviewers. Any product that may be evaluated in this article or claim that may be made by its manufacturer is not guaranteed or endorsed by the publisher.
Research integrity at Frontiers
Learn more about the work of our research integrity team to safeguard the quality of each article we publish.