- 1Yunnan Key Laboratory of Precision Nutrition and Personalized Food Manufacturing, Yunnan Agricultural University, Kunming, China
- 2College of Food Science and Technology, Yunnan Agricultural University, Kunming, China
- 3Engineering Research Center of Development and Utilization of Food and Drug Homologous Resources, Ministry of Education, Yunnan Agricultural University, Kunming, China
- 4Department of Hotel Management, Chongqing Vocational Institute of Tourism, Chongqing, China
- 5College of Pu’er Tea West Yunnan University of Applied Sciences, Puer, China
- 6Division of Science and Technology, Yunnan Agricultural University, Kunming, China
Moringa oleifera Lam. leaf is not only a new food resource in China, but also a traditional medicinal plant. It is commonly used in the folk to alleviate constipation, but its laxative mechanism is not fully understood. Hence we investigated it in loperamide-induced functional constipation (FC) mice. The results showed that MOAE significantly regulated not only gastrointestinal hormones and neurotransmitters in serum but also important gastrointestinal motility factors in the enteric nervous system (ENS)-interstitial cells of Cajal (ICCs)-smooth muscle cell (SMC) network. Meanwhile, MOAE attenuated intestinal inflammation, increased cecal short-chain fatty acid levels and colonic antimicrobial peptide expression, and improved the impaired intestinal barrier function in loperamide-induced FC mice. In addition, MOAE also increased fecal water content by inhibiting the mRNA expression of colonic aquaporins (Aqp3 and Aqp4) in FC mice. Interestingly and importantly, MOAE affected the intestinal microbiota by inhibiting some key “constipation-causing” microbiota, such as Bacteroidaceae, Clostridiaceae, Bacteroides, and Ruminococcus, and promoting the growth of other important “constipation-curing” microbiota, such as Butyricoccus, Tyzzerella, and Desulfovibrio. These important taxa are significantly associated with a variety of indicators of constipation. These findings suggest that MOAE can promote defecation through its rich chemical composition to modulate the ENS-ICCs-SMCs network and the gut microecosystem.
Highlights
• MOAE can relieve the loperamide-induced FC by modulating ENS-ICCs-SMCs network.
• MOAE can restore the gut microbiota imbanlance induced by loperamide.
• Gut microbiota regulated by MOAE was correlated with constipation phenotype.
Introduction
Functional constipation (FC) is a common gastrointestinal disorder in the population, and its prevalence has been shown to vary in cross-sectional surveys (Barberio et al., 2021). It can be divided into three subgroups based on different pathological mechanisms, including defecation disorder, slow transit constipation (STC), and normal transit constipation (Vriesman et al., 2020). Human defecation is a complex physiological movement process that involves multiple systems in the body; disorder in any link will lead to constipation. FC is multifactorial in its pathophysiology. General pathophysiology includes genetic factors, parental factors, psychological and behavioral factors, lifestyle factors, colonic motility factors, anorectal factors, and the microbiome (Vriesman et al., 2020).
The treatment of FC remains a difficult problem. The limitations of conventional drugs provide opportunities for the application of important traditional resources including both medicine and food. Native to western and sub-Himalayan regions, Moringa oleifera Lam.(MO) is known for its diverse medicinal and nutritional uses (Gao et al., 2017). As a traditional medicinal plant, it has been used in folk medicine to relieve constipation (Senthilkumar et al., 2018). However, MO leaves were also authenticated as new food resources. Based on pharmacodynamics and serum metabolomics, Li C. et al. found that M. oleifera leaves have long-lasting and mild laxative effects, increase the defecation volume and water content of feces, and may be a potential medicine for treating constipation (Li C. et al., 2022).
Accumulating evidence has highlighted the link between imbalances in the gut microbiota and constipation (Pan et al., 2022). The development and maturation of the enteric nervous system (ENS) has been shown to depend on bacterial colonization of the gut (Joly et al., 2021). The ENS is structurally similar to the central nervous system, as it independently regulates neurons for gut motility and brain-gut communication (Liu et al., 2009). Disruption of the microbiota-gut-brain axis signalling may lead to altered gut motility (Kennedy et al., 2012; Carabotti et al., 2015). Some important microbes can influence defecation behavior by regulating intestinal motility, such as Lactobacillus rhamnosus, L Lactobacillus paracasei and Bacillus coagulans (Chen et al., 2020; Wang G. et al., 2020; Zhou et al., 2022). LPS and metabolites of gut microbes, such as deoxycholic acid and Short-chain fatty acids (SCFAs), can also affect intestinal motility (Rebollar et al., 2002; Fukumoto et al., 2003; Yano et al., 2015).
Although intestinal microecology is closely related to constipation, there is no report on whether M. oleifera leaves affect defecation behavior by regulating intestinal microecology. Therefore, we evaluated the laxative effect of M. oleifera leaf aqueous extract (MOAE) in loperamide-induced slow transit constipation (STC) mice and systematically explored the laxative mechanism of MOAE from the aspects of neurotransmitters, gastrointestinal hormones, intestinal motility related factors, gut aquaporins, intestinal inflammation, gut barrier factors, intestinal antimicrobial peptides, the gut microbiota and their metabolites.
Materials and methods
Preparation and chemical composition of Moringa oleifera leaf aqueous extract
The dry MO leaf powder was provided by Yunnan Tianyou Technology Development Co., Ltd. Dry M. oleifera leaf powder (400 g) was accurately weighed, exposed to boiled water (95°C, RO water) for 1 min at a ratio of 1:9 (g/mL), and then filtered immediately. After filtration was completed, the filtrate was continuously centrifuged three times in a high-speed centrifuge (4,500 rpm/m for 5 min), and the supernatant was taken. The filter residue was poured into boiling water for 1 min and then filtered immediately. The above step was repeated twice. After three rounds of centrifugation, the supernatants were poured together and vacuum freeze-dried to obtain M. oleifera leaf aqueous extract (MOAE). The yield was 20.0%. The methods listed in Supplementary Table S1 were used to determine the main nutritional components and phytochemical composition of MOAE.
Experimental design for animal study
Seventy-two male C57BL/6 J mice (5–6 weeks old, 20–22 g) were purchased from Liaoning Changsheng Biotechnology Co., Ltd. The mice were housed in a controlled environment (24 ± 1°C, 12-h daylight cycle, lights off at 20,00) with ad libitum access to food and water. Mice were divided into the following six groups (n = 12) after adaptive feeding for 7 days, including the control group (CON, saline solution), the loperamide model group (LOP, 4 mg/kg loperamide), the positive control group (POS, 4 mg/kg loperamide and 900 mg/kg Maren pill from Beijing Tongrentang Pharmaceutical Co., Ltd.), the low-dose MOAE group (LMO, received 4 mg/kg loperamide and 250 mg/kg MOAE), the middle-dose MOAE group (MMO, received 4 mg/kg loperamide and 500 mg/kg MOAE), and the high-dose MOAE group (HMO, received 4 mg/kg loperamide and 750 mg/kg MOAE). All groups received daily oral gavage with the corresponding test substance at 9:30 a.m. for 7 days. During the experiment, the mice had ad libitum access to water, the body weight and food intake were monitored once every 2 days, and the padding and drinking water were changed every 4 days. Animal Ethics Committee of Yunnan Agriculture University approved the protocols of animal experiments (No.: 202001025).
Defecation test
On the evening of the sixth day, the mice were fasted overnight for 12 h. All groups were subjected to oral gavage with the corresponding test substance at 8:00 a.m. the next day. Sixty minutes later, all mice were administered approximately 0.4 mL ink, placed in empty, clean cages and watered ad libitum. The defection time of the first black stool (FBST), the fecal number (FN) and the fecal wet weight (FW) were observed and recorded over 6 h. The feces of each mouse were collected and dried in an oven at 80°C for 36 h, and the fecal water content (FWC) was calculated. All these defecation parameters were used to assess the laxative effect. After the defection test, all the mice were placed back into their original cages for the gastrointestinal transit test the next day.
Gastrointestinal transit test and tissue collection
On the evening of the seventh day, the mice were fasted overnight for 12 h. All groups were subjected to oral gavage with the corresponding test substance at 8:00 a.m. the next day. Sixty minutes later, all mice were gavage administered approximately 0.4 mL ink. Twenty minutes later, the mice were sacrificed to collect whole blood and the small intestinal segments, and then the ink advance distance was measured to calculate the gastrointestinal transmission rate (GTR). Serum was prepared by incubating the collected blood at 37°C for 30 min and centrifuged at 4°C and 3,500 rpm for 10 min. The distal ileum and proximal colon of each mouse were precisely dissected simultaneously, and their contents were rinsed with cold PBS. They were then snap frozen in liquid nitrogen and stored at -80°C for further use.
Enzyme-linked immunosorbent assay
The levels of motilin (MTL) and vasoactive intestinal peptide (VIP) in serum were examined using kits purchased from Sangon Biotech (Shanghai, China). The levels of gastrin (Gas) and somatostatin (SS) in serum were measured using mouse Enzyme-linked immunosorbent assay (ELISA) kits obtained from Cusabio Biotech Co., Ltd. (Wuhan, China).
Histopathology investigation
10% neutral formaldehyde fixative were used to fixed the ileum and colon of mice. Tissues were sectioned at 5 mm thickness and embedded in paraffin. Hematoxylin and eosin (H&E) staining was performed on 5-μm paraffin sections. An Olympus CX43 microscope with CellSens Entry software was used to capture the H&E staining images.
RNA preparation and quantitative PCR analysis of gene expression
Total RNA was extracted from frozen tissue of both the ileum and colon by using the TaKaRa MiniBEST Universal RNA Extraction Kit (TaKaRa, 9,767, Dalian), and then complementary DNA was obtained by using the PrimeScript™ RT Reagent Kit with gDNA Eraser (Perfect Real Time) (TaKaRa, RR047A, Dalian). Quantitative PCR analysis of gene expression was conducted using a LightCycler/LightCycler480 System (Roche Diagnostics) with TB Green™ Premix ExTaq™II (TaKaRa, RR820A, Dalian) in both the ileum and colon. The 2-△△Ct method was used for data analysis. The primer sequences are presented in Supplementary Table S2.
Metabolite extraction and GC–MS analysis of short-chain fatty acids
A 0.10 mL sample was added into 1.5 mL EP tubes, 0.05 mL of 50% H2SO4 and 0.2 mL of 2-methylvaleric acid (25 mg/L stock in methyl tertbutyl ether) were added as an internal standard; the samples were vortexed for 30 s, oscillated for 10 min, and then ultrasonicated for 10 min (incubated in ice water). After centrifuging for 15 min at 10,000 rpm and 4°C, the mixture was kept at −20°C for 30 min. For GC–MS analysis, the supernatant was then transferred to a fresh 2 mL glass vial. An Agilent 7890B gas chromatograph coupled to an Agilent 5977B mass spectrometer was used in the assay, and the specific parameters were in general agreement with a previous report (Wang Y. et al., 2020). The content of various short-chain fatty acids (SCFAs) in the samples was calculated by constructing standard curves.
16S rRNA gene sequencing and bioinformatics analysis of the gut microbiota
Metagenomic DNA from the cecal content was extracted using a QIAamp-DNA stool mini-kit (Qiagen, 51,604, Germany). DNA samples were then shipped on dry ice to Majorbio Biotechnology Co., Ltd. (Shanghai, China). The detailed methods for sequencing gut microbiota 16S rRNA genes and bioinformatic analysis were performed using our previously described methods (Gao et al., 2020; Gao X. et al., 2022; Hu et al., 2023).
Statistical analysis
The data are presented as the means ± standard errors of the means (SEMs). The Student’s t-test (unpaired two-tailed) was performed to analyze two independent groups. Spearman’s r coefficients were used for bivariate correlations. The heatmaps were generated using HemI 1.0 software. Unless otherwise stated in the figure legends, the results were considered statistically significant when the value of p is less than 0.05.
Results
Phytochemical composition and nutritional components of MOAE
As shown in Supplementary Table S1, the macronutrients included water (6.92%), fat (1.75%), protein (20.20%), carbohydrate (54.33%), and crude polysaccharide (5.14%). MOAE was also rich in mineral elements and vitamin C (720 mg/kg). The ash and total acid contents were 16.80 and 0.40%, respectively. Supplementary Table S3 shows the most abundant chemical compound categories found in MOAE. Flavonoids, amino acids, nucleotides, lipids, and alkaloids were the main classifications. There were 23 monomes with relative abundances greater than 0.50% (Supplementary Table S4). The monomes with a abundance of more than 5% were L-phenylalanine (11.84%), isoquercitrin (6.38%), astragalin (8.73%), and vidarabine (5.33%).
MOAE alleviated the symptoms of constipation induced by loperamide in mice
To assess the laxative effect of MOAE, defecation tests and gastrointestinal transit tests were carried out (Figure 1A). In the defecation tests, we examined FBST, FN, FW and FWC within 6 h. Our results showed that the LOP group had a longer FBST than the CON group (p < 0.001), and the FBST was shorter in the MMO and HMO groups than in the LOP group (p < 0.01) (Figure 1B). Similarly, the FN and FWC were significantly higher in the HMO group than in the LOP group (p < 0.05) but there was not a significant increase in FW (Figures 1C–E). The fecal morphology in these groups, except the LOP group, was similar to that in the normal group; meanwhile, the feces appeared to have a larger bulk and higher surface moisture in the HMO group (Figure 1F).
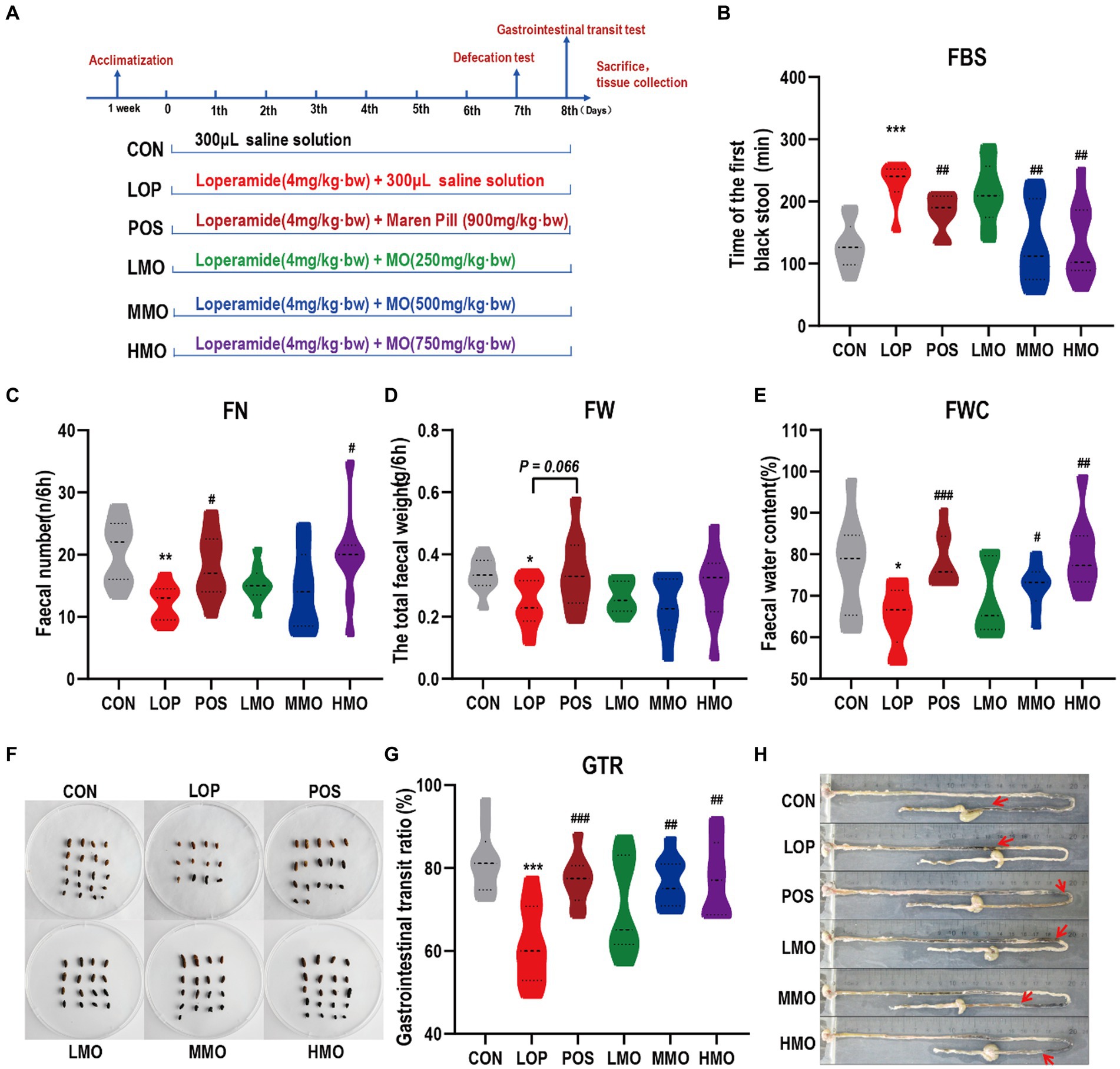
Figure 1. Effects of MOAE on the symptoms of FC in C57BL/6J mice. (A) Basic workflow and grouping; (B) The defecation time of the first black stool, FBST; (C) The fecal number, FN; (D) The fecal wet weight, FW; (E) The fecal water content, FWC; (F) The representative fecal morphology; (G) The gastrointestinal transit rate, GTR; (H) Representative pictures of ink advance. The data are presented as the mean ± SEM (n = 10–12). *, vs. CON group; #, vs. LOP group. **p < 0.01; ***p < 0.001. #p < 0.05; ##p < 0.01; ###p < 0.001.
In the gastrointestinal transit tests, loperamide effectively inhibited the movement of the small intestine (p < 0.001), and medium and high doses of MOAE significantly reversed the inhibitory effect of loperamide on intestinal motility (Figures 1G,H, P < 0.01). Notably, we also investigated the effect of MOAE on body weight (Supplementary Figure S1A), feeding behavior (Supplementary Figures S1B,C), and the organ index (Supplementary Figures S1D–H) of FC mice. MOAE treatment did not show any adverse effects on these parameters. These results suggested that MOAE could alleviate FC by promoting small intestinal peristalsis, shortening the defecation time, and increasing the water content of feces. The effect of MOAE was comparable to that of the positive drug.
MOAE reversed loperamide-induced gastrointestinal hormone and neurotransmitter secretion abnormalities
Gastrin (Gas), motilin (MTL), Vasoactive intestinal polypeptide (VIP) and somatostatin (SS) are important neurotransmitters and gastrointestinal hormones. As important components of ENS, they play important roles in the regulation of gastrointestinal motility. Gas, MTL, SS, and VIP levels in serum were measured by ELISA. Loperamide significantly decreased the contents of the excitatory gastrointestinal hormones Gas and MTL (Figures 2A,B, P < 0.05), significantly increased the content of the inhibitory gastrointestinal hormone SS (Figure 2C, P < 0.01), and increased the content of the inhibitory neurotransmitter VIP (Figure 2D, P = 0.066). Different doses of MOAE reversed loperamide-induced gastrointestinal hormone and neurotransmitter disorders to varying degrees, and the HMO group showed the best regulation.
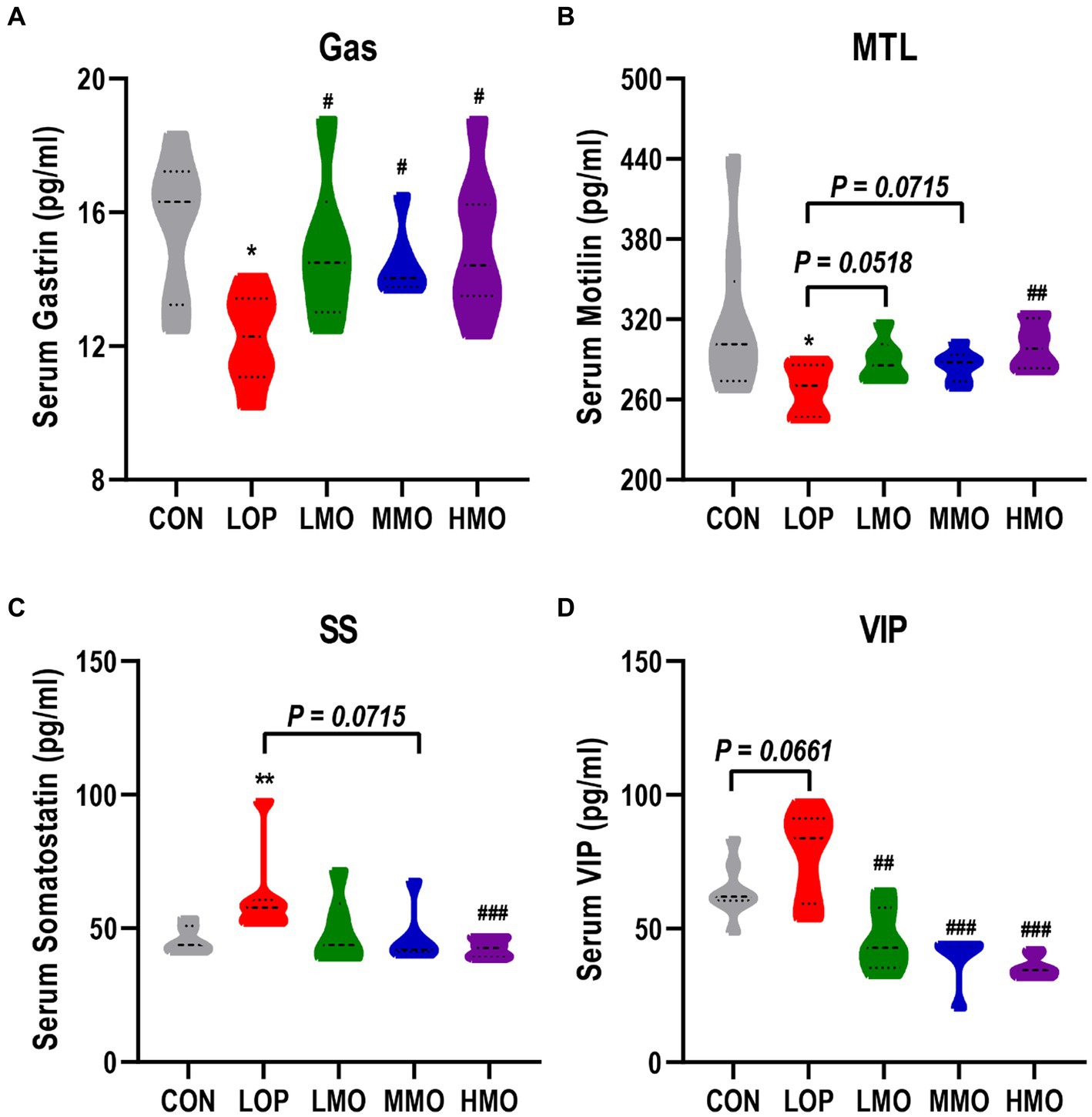
Figure 2. The effect of MOAE on the serum levels of neurotransmitters and gastrointestinal hormones. (A) Gastrin, (B) Motilin, (C) Somatostatin, and (D) vasoactive intestinal polypeptide levels in mice serum. The data are presented as the mean ± SEM (n = 8). *, vs. CON group; #, vs. LOP group. *p < 0.05; **p < 0.01. #p < 0.05; ##p < 0.01; ###p < 0.001. MOEA enhanced the expression of gastrointestinal motility-related factors.
5-Hydroxytryptamine (5-HT) and acetylcholine (Ach) are both exciting neurotransmitters that can stimulate bowel muscle contractions and gastrointestinal smooth muscle peristalsis. In our experiments, the mRNA expression of acetylcholinesterase (AchE) and 5-hydroxytryptamine receptor 4 (5-HT4R) in the colon could be significantly upregulated by treatment with a medium dose of MOEA and a high dose of MOEA, respectively (Figures 3A,B).
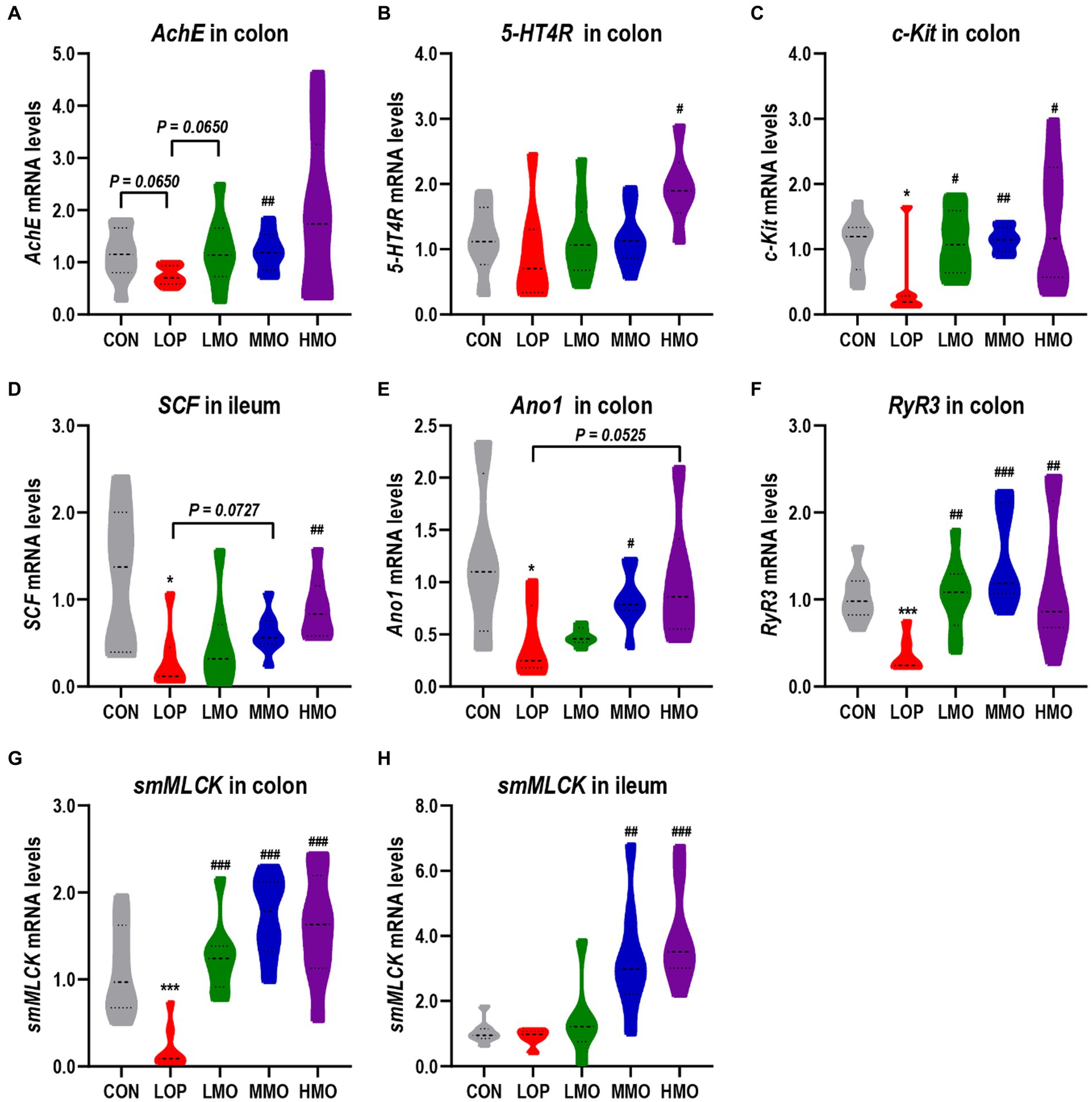
Figure 3. MOAE increased the mRNA expression of neurotransmitter receptors and gastrointestinal motility factors in mice. (A–C) The mRNA expression of AchE, 5-HT4R, and c-Kit in the colon; (D) The mRNA expression of SCF in the ileum; (E–G) Colonic mRNA expression of Ano1, RyR3, and smMLCK; (H) The mRNA expression of smMLCK in the ileum. The data are presented as the mean ± SEM (n = 8). *, vs. CON group; #, vs. LOP group. *p < 0.05; ***p < 0.001. #p < 0.05; ##p < 0.01; ###p < 0.001.
Interstitial cells of Cajal (ICCs) are the pacemaker cells of smooth muscle. The stem cell factor (SCF) and stem cell factor receptor (c-Kit) are important malers of ICCs. Our results showed that the mRNA expression of c-Kit in the colon and SCF in the ileum were significantly inhibited by loperamide, while high-dose MOAE treatment significantly upregulated their expression (Figures 3C,D).
The opening of the ryanodine receptor 3 (RyR3) can cause smooth muscle contraction through the activation of myosin light chain kinase (MLCK); in addition, anoctamin 1 (Ano1), a calcium-activated chloride channel, is also present in ICCs and plays an important role in intestinal motility (Gao X. et al., 2022). Similarly, loperamide significantly reduced the expression of Ano1, RyR3 and smooth muscle myosin light chain kinase (smMLCK), whereas MOAE reversed their expression to different extent (Figures 3E–H), even to levels exceeding that of the CON group (Figures 3F–H). In all, MOAE could promote defecation by enhancing the expression of key factors associated with the motility of gastrointestinal tract.
MOAE alleviated intestinal inflammation and damaged gut barrier function induced by loperamide
The process of functional constipation is often accompanied by intestinal inflammation and impaired intestinal barrier function (Vriesman et al., 2020). Colonic H&E staining and pro-inflammatory factor mRNA assay showed that loperamide induced inflammatory cell infiltration and high expression of pro-inflammatory factors (Figure 4A), while MOAE treatment inhibited the expression of proinflammatory cytokines (Figures 4B–D and Supplementary Figures S2K–L). Meanwhile, MOAE also restored the downregulation of several intestinal barrier function factors by loperamide, including Muc2, ZO-1, and Occludin (Figures 4E–G).
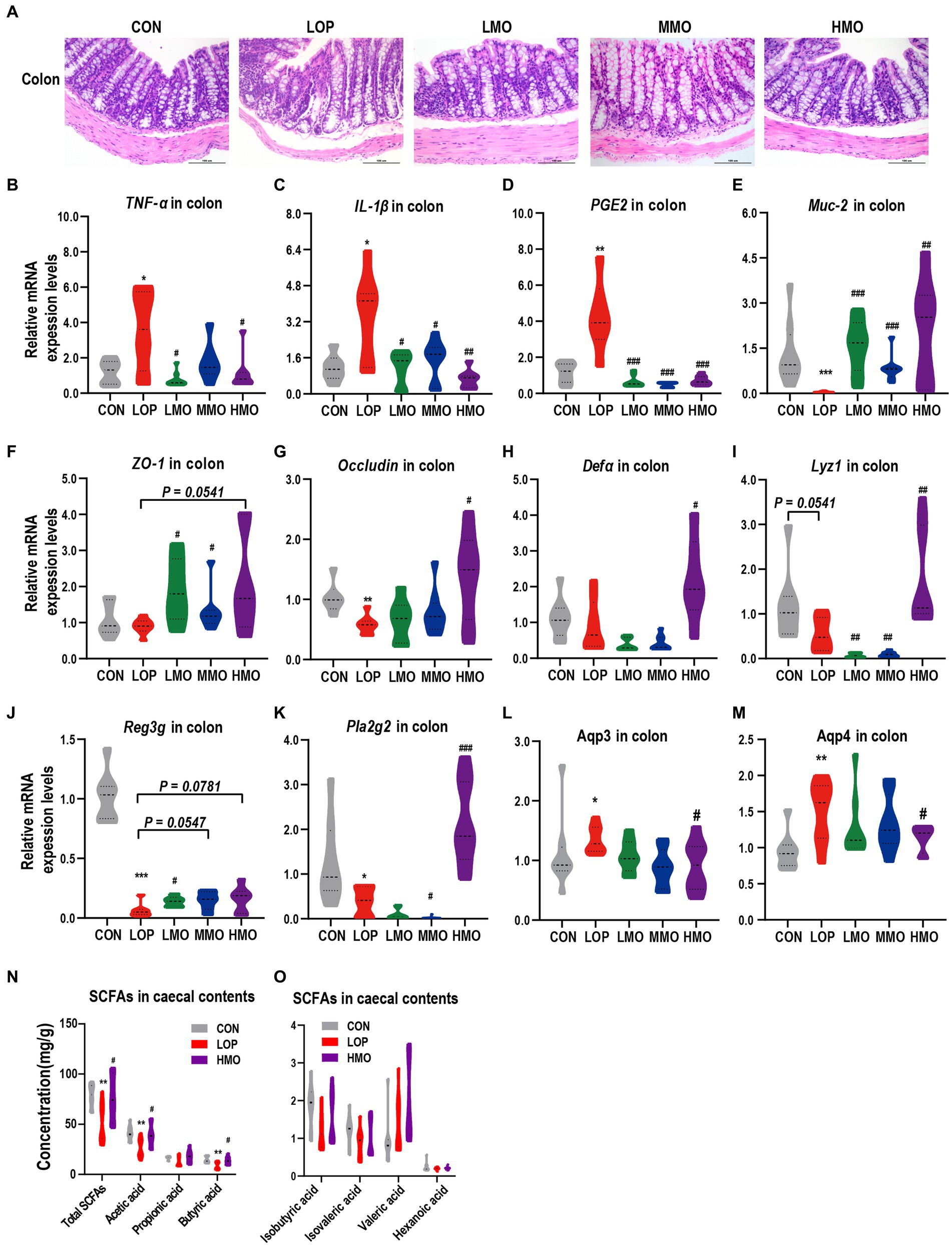
Figure 4. Effects of MOAE on intestinal inflammation, barrier, aquaporins and SCFAs in loperamide-induced FC mice. (A) Representative photographs of H&E staining of proximal colon sections. (B–D) Expression of TNF-α, IL-1β, and PGE2 in the colon. (E–G) Expression of Muc2, ZO-1, and Occludin in the colon. (H–K) Expression of Defa, Lyz1, Reg3g, and Pla2g2 in the colon. (L,M) Expression of the aquaporins (Aqp3 and Aqp4) in the colon. (N) SCFAs in cecal contents, including total SCFAs, acetic acid, propionic acid, and butyric acid. (O) SCFAs in ceacal contents, including isobutyric acid, isovaleric acid, valeric acid, and hexanoic acid. The data are presented as the mean ± SEM (n = 6 or 8). *, vs. CON group; #, vs. LOP group. *p < 0.05; ***p < 0.001. #p < 0.05; ##p < 0.01; ###p < 0.001.
Antimicrobial peptides (AMPs) in the gut are important for defense against pathogens and for maintaining the homeostasis between the microbiota and the host (Gao et al., 2020). Loperamide reduced all the colonic mRNA expression of alpha-defensins (Defa), lysozyme C (Lyz1), regenerating islet-derived 3-gamma (Reg3g) and phospholipase A2 group II (Pla2g2) to varying degrees (Figures 4H–K); interestingly, high doses of MOAE significantly up-regulated these AMPs (Figures 4H–K), except for Reg3g, for which the change was not statistically significant (Figure 4J, p = 0.0781). Notably, LOP and MOAE had similar effects on the mouse ileum, but the overall effects were not as significant as their effects on the colon (Supplementary Figures S2A–J). This may be due to the fact that different intestinal segments have different histological structures, intestinal microecological environments and levels of MOAE utilization. In summary, MOAE alleviated intestinal inflammation and damaged gut barrier function in loperamide induced FC mice.
Effects of MOAE on colonic aquaporins and cecal SCFAs in loperamide-induced FC mice
Given the ability of MOAE to increase fecal water content in loperamide-induced FC mice (Figure 1E), we examined the mRNA expression levels of two important aquaporins, Aqp3 and Aqp4, in colonic tissue. Loperamide significantly up-regulated Aqp3 and Aqp4, while a high dose of MOAE treatment reversed these changes, and the expression levels of Aqp3 and Aqp4 in the HMO group were close to those in the CON group (Figures 4L,M). At the same time, high-dose MOAE treatment also significantly reversed the decrease in the levels of total SCFAs, acetic acid, propionic acid, and butyric acid in the cecal content caused by LOP (Figure 4N). The effects of LOP and MOAE on several other SCFAs were not significant (Figure 4O). These results suggest that MOAE might improve the intestinal environment by regulating water balance and increasing the content of SCFAs.
MOAE ameliorated loperamide-induced gut microbiota disorder in FC mice
To assess the impact of loperamide and MOAE on the cecal microbiota, 16S rRNA gene sequencing was performed. We obtained 1,366,660 sequences from 23 samples in the three groups, and each sample contained more than 41,924 valid sequences, and identified 700 OTUs, 135 genera, 58 families, and 11 phyla. The Sobs index rarefaction curve of each sample indicated that the sequencing result was reliable (Supplementary Figure S3A). Although MOAE had a limited effect on α diversity (Supplementary Figures S3B–F), MOAE altered β diversity (Figure 5A). Meanwhile, MOAE changed the relative abundance of many cecal microbial taxa (Supplementary Figures S4A–C).
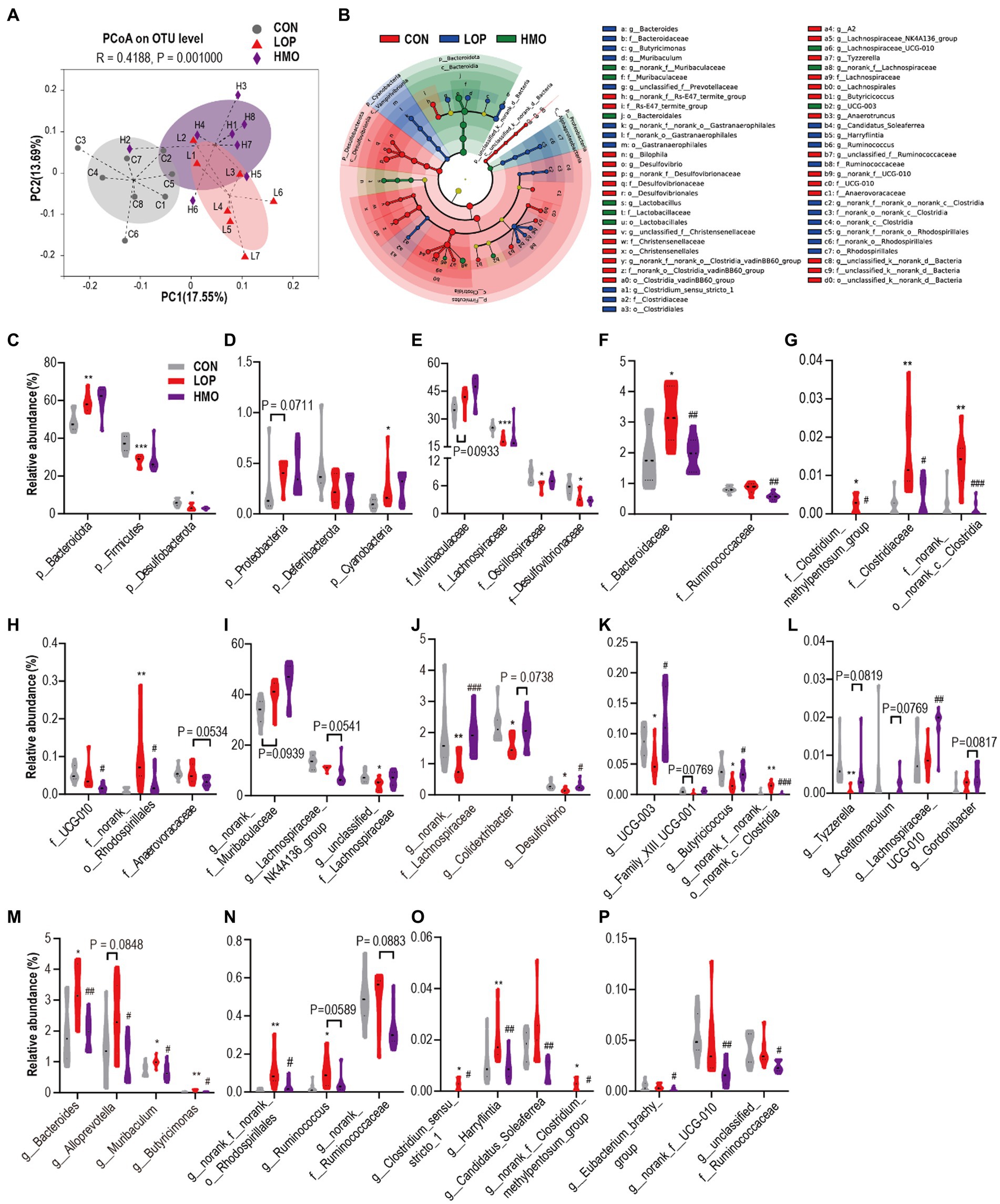
Figure 5. MOAE restored the gut microbial community structural and composition shift in FC mice (A) PCoA plot (Bray–Curtis dissimilarity). (B) LEfSe analyses (phylum to genera, LDA score > 2.0). (C–P) The relative abundance of gut microbiota at the phylum (C,D), family (E–H), and genus levels (I–P). The data are presented as the mean ± SEM, n = 7–8. * vs. NCD group, # vs. LOP group. *p ≤ 0.05, **p ≤ 0.01, ***p ≤ 0.001; #p ≤ 0.05, ##p ≤ 0.01, ###p ≤ 0.001.
The dominant microbiota in each group was determined by linear discriminant analysis effect size (LEfSe) analyses (Figure 5B and Supplementary Figures S5A–D). It shows 69 different taxa in in the three groups (Supplementary Figures S5A–D). Our focus was on taxa that were significantly affected by loperamide or MOAE, particularly those with MOAE reversal (Supplementary Figures S5A–D).
At the phylum level, Bacteroidota, Firmicutes, Desulfobacterota and Cyanobacteria were significantly changed by loperamide treatment; however, MOAE had less impact on them (Figures 5C,D). At the family level, loperamide significantly altered the abundance of some dominant taxa (>1%, Figures 5E,F), including Lachnospiraceae, Oscillospiraceae, Desulfovibrionaceae, and Bacteroidaceae (p < 0.05); however, MOAE only significantly reversed the changes in the abundance of Bacteroidaceae. MOAE also significantly suppressed the increase in abundance of some relatively less abundant taxa induced by loperamide, such as Clostridium_methylpentosum_group, Clostridiaceae, norank_o_norank_C_Clostridia and norank_o_Rhodospirillales. Although the effects of loperamide on Ruminococcaceae and UCG-010 were small, MOAE treatment significantly reduced their abundance (Figures 5F,H).
At the genus level, norank_f_Muribaculaceae was the most abundant; however, neither loperamide nor MOAE had significant effects on this genera (Figure 5I). Loperamide reduced the relative abundance of two important taxa of Lachnospiraceae, Lachnospiraceae_NK4A136_group and unclassified_f_Lachnospiraceae. However, MOAE treatment restored the relative abundance of unclassified_f_Lachnospiraceae to control levels to some extent (Figure 5I). Compared with CON, loperamide also significantly reduced the abundance of norank_f_Lachnospiraceae, Colidextribacter, Desulfovibrio, UCG-003, Family_XIII_UCG-001, Butyricicoccus, norank_f_norank_o_norank_c_Clostridia, and Tyzzerella (Figures 5J–L), while MOAE significantly or nearly significantly increased the relative abundance of these taxa. Notably, MOAE also significantly increased the relative abundance of Lachnospiraceae_UCG-010 in the caecum of FC mice, although loperamide had little effect on it (Figure 5L).
Loperamide significantly (or nearly significantly) increased the four genera of Bacteroidota (Figure 5M), Bacteroides, Alloprevotella, Muribaculum, and Butyricimonas, while MOAE significantly reduced the abundance of them. Loperamide also significantly increased the relative abundance of norank_f_norank_o_ Rhodospirillales (a genus of Proteobacteria), while MOAE treatment significantly decreased its relative abundance in the caecum of FC mice (Figure 5N). Meanwhile, loperamide increased Ruminococcus, Clostridium_sensu_stricto_1, Harryflintia, Candidatus_Soleaferrea, and norank_f__Clostridium_methylpentosum_group (genera of Firmicutes), while MOAE significantly (or nearly significantly) decreased their abundance (Figures 5N,O). MOAE also significantly reduced the relative abundance of Eubacterium_brachy_group, norank_f_UCG-010, and unclassified_f_Ruminococcaceae in the caecum of FC mice, although loperamide had little effect on these bacteria (Figure 5P).
Correlations between the specific gut bacterial taxa and key host parameters
Based on the significant relief of constipation symptoms, gastrointestinal motility and the intestinal microecosystem in FC mice by MOAE, we established correlations between specific gut bacteria taxa (at the family and genus levels) and core host parameters (Figures 6A–C).
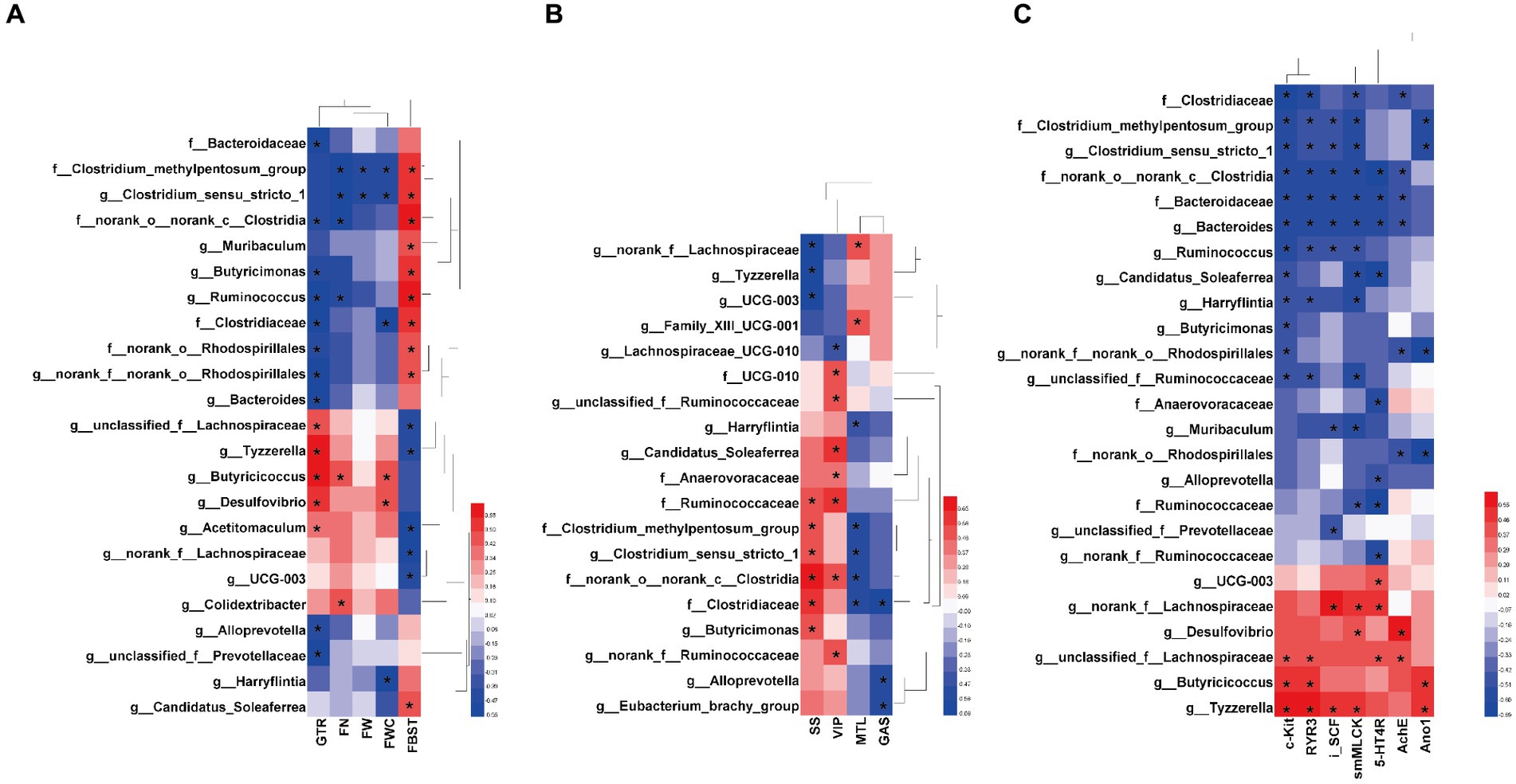
Figure 6. Heatmaps showing correlations between the specific gut bacterial taxa and key host parameters. (A) Correlations between the specific taxa and laxative phenotypic indicators, including FBST, FN, FW, FWC and GTR. (B) Correlations between gut bacteria and gastrointestinal hormones and neurotransmitters in serum, including GAS, MTL, SS, and VIP. (C) Correlations between the specific taxa and neurotransmitter receptors and intestinal motility related factors, including AchE, 5-HT4R, c-Kit,RyR3, smMLCK, and Ano1 in the colon, and SCF in the ileum (iSCF). The color at each point of intersection indicates the value of the r coefficient; The Bonferroni and Hochberg procedures were used to adjust p-values for multiple testing. * Indicates that there is a significant correlation between these two parameters (p < 0.05).
There are clear and strong correlations between specific taxa and laxative phenotypic indicators (Figure 6A). At the family level, Bacteroidaceae, Clostridiaceae, Clostridium_methylpentosum_group, norank_o_norank_c_Clostridia, and norank_o_Rhodospirillales were positively correlated with FBST or negatively correlated with GTR (p < 0.05). At the genus level, Clostridium_sensu_stricto_1, Muribaculum, Butyricimonas, Ruminococcus, Bacteroides, Alloprevotella, unclassified_f_Prevotellaceae, and Candidatus_Soleaferrea also showed similar correlations (p < 0.05). This suggests that these taxa might promote in the development of loperamide-induced constipation. Interestingly, MOAE reduced their relative abundance in the cecal microbiome of FC mice (Figures 5F–H,J,M–O). On the other hand, some taxa at the genus level showed opposite correlations, including unclassified_f_Lachnospiraceae, Tyzzerella, Butyricicoccus, Desulfovibrio, Acetitomaculum, norank_f__Lachnospiraceae, and UCG-003. Again, this suggests that these taxa might be potentially beneficial bacteria. Importantly, MOAE treatment increased their relative abundances (Figures 5I–L,O). Of course, other laxative phenotypes such as FN, FW and FWC were also strongly associated with these specific taxa. All of these numerous correlations support the important role of the gut microbiota in the relief of constipation by MOAE.
Correlations between the specific taxa and intestinal motility related factors, intestinal inflammation, gut barrier, and cecal SCFAs were also systematically analyzed. We found that Ruminococcaceae, Clostridium_methylpentosum_group, Clostridium_sensu_stricto_1, norank_o__norank_c_Clostridia, and Clostridiaceae were significantly correlated with more than two serum gut hormones (or neurotransmitters), and they were all positively correlated with SS and negatively correlated with MTL, while norank_f_Lachnospiraceae, Tyzzerella and UCG-003 showed the opposite correlations (Figure 6B). Meanwhile, serum VIP and Gas also showed strong correlations with a variety of taxa (Figure 6B). Figure 6C clearly shows the correlations between 25 taxa and the mRNA expression of seven gastrointestinal motility-related factors in the colon. Clostridiaceae, Clostridium_methylpentosum_group, Clostridium_sensu_stricto_1, norank_o__norank_c__Clostridia, Bacteroidaceae, Bacteroides, and Ruminococcus were negatively and significantly correlated with more than four related factors. Norank_f__Lachnospiraceae, Desulfovibrio, unclassified_f__Lachnospiraceae, Butyricicoccus, and Tyzzerella were negatively and significantly correlated with more than two related factors. Colonic c-kit and smMLCK show more correlations with gut microbes. All these results supported the notion that MOAE could affect gastrointestinal motility by regulating these microbial groups.
Correlations between the specific taxa and intestinal inflammation are shown in Supplementary Figure S6A. Bacteroidaceae, Clostridium_methylpentosum_group, norank_o__norank_c__Clostridia, norank_o__Rhodospirillales, Clostridium_sensu_stricto_1, Muribaculum, Bacteroides, norank_f__norank_o__Rhodospirillales and Ruminococcus were all positively correlated with TNF-α, IL-1β and PGE2 in the colon, while Desulfovibrio, Acetitomaculum, UCG-003, and Lachnospiraceae_UCG-010 were negatively correlated with these proinflammatory factors.
The correlations between 18 taxa and three important gut barrier-related factors are shown in Supplementary Figure S6B. Among them, 13 taxa showed significant and negative correlations with Occludin or Muc2, including 5 families and 8 genera, which were norank_o__norank_c_Clostridia, Clostridium_methylpentosum_group, UCG-010, Bacteroidaceae, Clostridiaceae, Butyricimonas, unclassified_f_Ruminococcaceae, Clostridium_sensu_stricto_1, Muribaculum, Alloprevotella, norank_f__norank_o_Rhodospirillales, Ruminococcus, and Bacteroides. The other 5 taxa showed the opposite correlations and included unclassified_f__Lachnospiraceae, Butyricicoccus, Tyzzerella, norank_f__Lachnospiraceae, and Desulfovibrio. It is worth emphasizing that Tyzzerella showed the strongest correlation with gut barrier factors and was the only taxon that had a significant positive correlation with ZO-1.
Twenty-eight specific taxa also showed significant correlations with the expression of intestinal AMP genes (Supplementary Figure S6C). Reg3g shows the closest correlation with these microbial groups. Ruminococcaceae, norank_o__norank_c__Clostridia, Bacteroidaceae, Candidatus_Soleaferrea, norank_f__Ruminococcaceae, Harryflintia, Muribaculum, Bacteroides, Alloprevotella, and unclassified_f__Ruminococcaceae showed significant negative correlations with Reg3g in the ileum, while norank_o__Rhodospirillales, Clostridium_methylpentosum_group, Clostridiaceae, norank_f__norank_o__Rhodospirillales, Ruminococcus, Clostridium_sensu_stricto_1, and unclassified_f__Prevotellaceae showed significant negative correlations with Reg3g in the colon. Notably, Tyzzerella, Butyricicoccus, Desulfovibrio, UCG-003, and Family_XIII_UCG-001 showed significant negative correlations with Reg3g in the colon or ileum.
In addition, we found significant correlations between SCFAs and some microbial groups (Supplementary Figure S6D). Desulfovibrio showed significant positive correlations with T-SCFAs, acetic acid and butyric acid, while unclassified_f_Lachnospiraceae showed significant positive correlations with only butyric acid. Muribaculum, norank_f_norank_o_norank_c_Clostridia, Butyricimonas, norank_f_norank_o_Rhodospirillales, Ruminococcus, Harryflintia, and norank_o_norank_c_Clostridia were all negatively correlated with T-SCFAs, acetic acid and butyric acid. Taken together, the preventive effect of MOAE in loperamide-induced FC mice can be explained in part by this complex but clear network that exists between specific gut bacterial taxa and key host parameters.
Discussion
Functional constipation (FC) is a common, highly prevalent gastrointestinal disorder that significantly reduces patients’ quality of life. Humans have searched for many natural products from edible and medicinal plants that are effective in preventing and treating FC. Modern scientific research has confirmed the laxative effect of M. oleifera leaves (Li R. et al., 2022; Li C. et al., 2022; Li L. et al., 2022), but the mechanism of the laxative effect of M. oleifera leaves is not yet systematically understood. MOAE was found to be low in fat and high in protein and carbohydrates and contained a certain amount of polysaccharides (Supplementary Table S1). Polysaccharides from many plants have been reported to have significant laxative effects, such as Durio zibethinus rind, Chrysanthemum morifolium, and Spirulina platensis (Ma et al., 2019; Jiang et al., 2020; Wang et al., 2021).
All of these plant polysaccharides were capable of modulating the host intestinal microbiota while reducing constipation symptoms. M. oleifera leaf polysaccharides have various biological activities, such as the prevention of colitis and obesity, the enhancement of immunity, and the regulation of gut microbiota (Li et al., 2020; Li L. et al., 2022; Tian et al., 2022), which suggests that they may also have laxative effects, but further studies are needed to confirm this.
MOAE is also rich in vitamin C and mineral elements, including sodium, potassium, magnesium, calcium, phosphorus, iron, and zinc (Supplementary Table S1). Vitamin C has abundant biological activities, but its laxative properties have not been reported. Through a systematic literature review, we found that the presence of sodium, potassium, calcium, magnesium and phosphorus in nature in various forms have different degrees of relief for constipation, including calcium sennosides, magnesium oxide, magnesium sulfate, sodium hyaluronate, and potassium binder (Dupont et al., 2019; Ali et al., 2021; Mori et al., 2021; Imamura and Kinugawa, 2022; Vacca et al., 2022). In particular, dietary magnesium and phosphorus are closely related to the occurrence of constipation (Zhang et al., 2021; Zhao et al., 2023). Therefore, whether M. oleifera leaves exert laxative effects through their rich mineral elements is an interesting scientific question worthy of further investigation.
Flavonoids and amino acids are the two main classifications of the phytochemical compounds in MOAE (Supplementary Table S3). Flavonoids in Allium mongolicum Regel, Amomum tsaoko and Maojian green tea can relieve constipation (Chen et al., 2019; Hu et al., 2023; Wu et al., 2023). It is unknown whether the flavonoids in M. oleifera leaves have laxative effects. Isoquercitrin, astragalin, vitexin and rutin are the four flavonoids with relatively high abundance in MOAE. These four flavonoids have biological activities such as antioxidant, anti-inflammatory and gut microbiota regulation (Valentová et al., 2014; Riaz et al., 2018; Peng et al., 2021; Muvhulawa et al., 2022), but there is no report on their laxative activity thus far.
Phenylalanine (L-Phe) and L-arginine (L-Arg) are the two main amino acids in MOAE, with L-Phe being the monomeric compound with the highest relative abundance of 11.84% (Supplementary Table S4). Recent findings suggest that L-Phe can improve constipation in rats by remodeling the structure of the intestinal microbial community and altering metabolite levels (Yang et al., 2022). In addition, it has been reported that L-Phe can improve intestinal barrier health and the immune status of young grass carp, which is associated with regulating the gene expression of cytokines, tight junction proteins, and antioxidant enzymes (Feng et al., 2015). L-Phe is able to stimulate the secretion of gastric and humoral proteins in pigs through calcium-sensing receptors (Xian et al., 2018), and inhibit the activity of acetylcholinesterase in the brains and diaphragms of rats (Tsakiris et al., 1998; Tsakiris, 2001). These previous results support the idea that L-Phe is one of the important laxative compounds of MOAE.
L-Arg can be converted to NO in vivo, and there is also strong evidence that L-Arg supplementation can promote gastrointestinal motility and relieve constipation. For example, diarrhoea was found after oral administration of large amounts of L-Arg in clinical studies (Grimble, 2007), animal experimental studies also showed that intraperitoneal injection of L-Arg could reverse the constipation caused by morphine (Calignano et al., 1991), and long-term administration of L-Arg could also reverse the decline in gastrointestinal motility caused by morphine in mice (Karan et al., 2000). In addition, it has also been shown that dietary L-Arg can promote Aquaporin-3 expression and water transport in porcine trophoblast cells (Zhu et al., 2022) and attenuate colonic barrier damage, oxidative stress, and inflammation in intrauterine growth-retarded lactating lambs by modulating the gut microbiota (Zhang et al., 2022). In summary, MOAE may relieve constipation through a variety of components and pathways, only some of which we have discussed here, and more in-depth studies may be needed in the future.
Gastrointestinal hormones, neurotransmitters and gastrointestinal motility factors play an important role in the maintenance of gastrointestinal motility. They are often abnormally expressed in patients with constipation. Previous research results showed that MOAE could increase serum substance P and colonic GAS levels and decrease serum growth inhibitory hormone and colonic VIP levels in rats (Li et al., 2019). We also found that high doses of MOAE reversed loperamide-induced disturbances in gut hormones and neurotransmitters, including GAS, MTL, SS, and VIP, in serum, as well as the mRNA expression of AchE and 5-HT4R in colonic tissues. These results fully confirmed that MOAE could promote the secretion of excitatory gastrointestinal hormones and neurotransmitters and reduce the secretion of inhibitory gastrointestinal hormones and neurotransmitters to promote gastrointestinal motility.
This study also examined the changes of important factors within the ENS-ICCs-SMCs network, which is primarily organized by ICCs (Gao X. et al., 2022). Acting as a kind of pacemaker cell, ICCs are facilitators of gastrointestinal electrical activity and regulators of neurotransmitter transmission (Zhu et al., 2021). The results of this study showed that high-dose MOAE treatment significantly upregulated c-Kit in the colon and SCF in the ileum (Figure 3). We also found that MOAE could restore loperidine-induced downregulation of Ano1, RyR3 (a receptor in calcium channels) and smMLCK to varying degrees. These findings suggest that MOAE may alleviate FC by modulating the ENS-ICCs-SMCs network.
Patients with constipation often have impaired intestinal barrier function and varying degrees of intestinal inflammation. Previous studies have shown that a reduction in constipation symptoms is accompanied by the reduction of inflammation (Liu et al., 2020; Gao H. et al., 2022; Shatri et al., 2023). M. oleifera leaf aqueous extract was able to alleviate high-fat diet-induced intestinal inflammation (Mohamed Husien et al., 2022). M. oleifera polysaccharide aided intestinal barrier maintenance and reduced symptoms of experimental colitis (Elabd et al., 2018; Pan et al., 2022). In the present study, high doses of MOAE significantly increased the gene expression of mechanical barrier factors and chemical barrier factors and inhibited colonic inflammatory factors which may be related to the reduction in bacteria and toxic products that enter the immune barrier. Notably, MOAE may also increase the fecal water content of FC mice by decreasing the expression of colonic Aqp3 and Aqp4, which in turn affects the entire intestinal microecosystem.
As an important environmental factor of the intestinal microecosystem, research on the role of the intestinal microbiota in constipation has received increasing attention. Intestinal microecological dysbiosis can lead to various functional gastrointestinal disorders, especially constipation. However, the mechanisms by which the gut microbiota and their metabolites affect intestinal motility are still poorly understood. In the present study, we found that MOAE can affect the gut microbial community structure and composition.
At the family level, Bacteroidaceae was the most noteworthy dominant microbial group, and MOAE reversed the significant increase of Bacteroidaceae in loperamide-induced FC mice, which is consistent with some clinical observations. For example, a higher relative abundance of Bacteroidacea was observed in constipated pediatric patients than in healthy children (Niu et al., 2020). Similarly, MOAE also reversed the significant increase in Clostridiaceae in FC mice induced by loperamide. Enrichment of Clostridiaceae in patients with slow transit constipation (Yu et al., 2023) and in loperamide-induced constipated rats (Huang et al., 2022) was also observed; Clostridiaceae bacteria are also considered to be characteristic of arthritis (Wang et al., 2017). Our correlation analysis also found that Bacteroidaceae and Clostridiaceae were significantly and negatively associated with the laxative phenotype. Although many studies support the hypothesis that Ruminococcaceae is a potentially beneficial organism, MOAE significantly reduces its abundance. A clinical study found that the relative abundance of Bacteroidaceae and Ruminococcaceae in chronic constipation patients with uncoordinated defecation was higher than that in chronic constipation patients with coordinated defecation (Yu et al., 2023), which laterally supports our findings. These results suggest that Bacteroidaceae, Clostridiaceae and Ruminococcaceae might play an important role in MOAE defecation at the family level, especially the dominant taxon Bacteroidaceae. Of course, we cannot exclude other taxa that are significantly altered by MOAE, and it is difficult for us to access their association with constipation.
Through a systematic literature review, we found that among the genus-level microbial groups significantly affected by MOAE, Alloprevotella, Bacteroides, Butyricimonas, Muribaculum, Ruminococcus, Clostridium_sensu_stricto_1, Desulfovibrio and Butyricicoccus were associated with constipation. Among them, Alloprevotella, Bacteroides, Muribaculum and Butyricimonas belong to the phylum Bacteroidetes. Many of these organisms are opportunistic pathogens. They were significantly enriched in loperamide-induced FC mice and significantly inhibited by MOAE.
Bacteroides, one of the most abundant members of the intestinal tract in most mammals, plays an important role in normal intestinal physiology and is most closely related to constipation. Oligosaccharides, konjac glucomannan, and yellow tea extracts all significantly reduced the abundance of Bacteroides in animals with constipation (Wang et al., 2017; Hayeeawaema et al., 2020; Cao et al., 2021). The results of clinical studies have also shown that FC patients have a higher abundance of Bacteroides, especially elderly FC patients (Guo et al., 2020; Wang et al., 2023). A study evaluating the safety and efficacy of fecal microbiota transplantation for chronic FC suggested that a high abundance of Bacteroides may be one of the causes of constipation (Tian et al., 2020). However, the genus Bacteroides is diverse and complex. Colonization with B. thetaiotaomicron, which was designed to produce tryptamine, led to accelerated gastrointestinal transport in GF mice (Bhattarai et al., 2018). B. ovatus can consume tryptophan and glutamate in vitro and synthesize the neuroactive compounds glutamine and GABA (Horvath et al., 2022).
Beneficial interactions with the host may be mediated by polysaccharide A or outer membrane vesicles of non-toxigenic B. fragilis, whereas systemic inflammation may be induced by toxigenic B. fragilis toxins or lipopolysaccharides (Sun et al., 2019); B. fragilis, on the other hand, requires only one step to turn from good to bad (Valguarnera and Wardenburg, 2020). Our correlation analysis revealed not only the correlation of Bacteroides with phenotypic indicators but also the significant correlation of Bacteroides with gastrointestinal motility factors, serum neurotransmitters, intestinal inflammatory factors and barrier factors, which further supports the hypothesis that a high abundance of Bacteroides causes functional constipation.
Alloprevotella, Muribaculum, and Butyricimonas have also been associated with constipation to varying degrees. It has been reported that elderly FC patients have a higher abundance of Butyricimonas (Guo et al., 2020), while Muribaculum is more abundant in complement 3 knockout mice with a constipation phenotype (Choi et al., 2021), and bamboo shavings-derived O-acetylated xylan alleviates constipation in mice while also reducing the levels of the potentially pathogenic bacteria Alloprevotella (Huang et al., 2022). Although the relative abundance of these microorganisms is low, their role cannot be ignored, and they also show a strong correlation with various types of host indicators.
Ruminococcus is a genus of Ruminococcaceae that is similar to Bacteroides. Ruminococcus was significantly enriched in loperamide-induced FC mice, while MOAE inhibited this genera. The relationship between microbes of the genus Ruminococcus and gastrointestinal motility is complex and seems to be paradoxical. Clinical studies have concluded that the relative abundance of Ruminococcus is higher in FC patients (Guo et al., 2020), and it is positively correlated with medical history in patients with slow-transit constipation (Tian et al., 2021); however, R. gnavus can play a pathogenic role in diarrhoeal irritable bowel syndrome by increasing 5-HT biosynthesis and promoting intestinal motility (Zhai et al., 2023). However, correlation analysis revealed not only the correlation between Ruminococcus and constipation phenotypic indicators but also the significant correlation between Ruminococcus and gastrointestinal motility factors, serum neurotransmitters, intestinal inflammatory factors, intestinal barrier factors and short-chain fatty acids, all of which support previous findings in clinical studies. Therefore, we speculate that the paradox may be related to the specificity of species function.
In the present study, another conditional pathogen, Clostridium_sensu_stricto_1, was significantly inhibited by MOAE. Clostridium_sensu_stricto_1 was strongly associated with intestinal inflammation (Wang et al., 2018; Ma et al., 2022), which is also supported by our correlation analysis results. The significant correlations of Clostridium_sensu_stricto_1 with the constipation phenotype, gastrointestinal hormones, neurotransmitters, intestinal motility factors, intestinal inflammatory factors and barrier factors in FC mice support its role in the relief of constipation by MOAE.
In addition to the significantly inhibited microbial taxa mentioned above, a number of microbial taxa were significantly enriched by MOAE treatment during the mitigation of FC, including norank_f_Lachnospiraceae, Lachnospiraceae_UCG-010, Colidextribacter, Desulfovibrio, UCG-003, Family_XIII_UCG-001, Butyricicoccus, norank_f_norank_o_norank_c_Clostridia, and Tyzzerella. Although we did not review their direct association with constipation, some of these taxa may play important roles in alleviating inflammation and protecting the intestinal barrier.
Butyricicoccus is a genus of butyrate-producing bacteria. The relative abundance of Butyricicoccus in the stool of patients with inflammatory bowel disease is low. Administration of B. pullicaecorum attenuated TNBS-induced colitis in rats, and the supernatant of Butyricoccus cultures enhanced intestinal epithelial barrier function (Eeckhaut et al., 2013). Cannabis sativa L. aqueous extracts significantly elevated the abundance of B. spp. while relieving constipation (Li R. et al., 2022; Li C. et al., 2022; Li L. et al., 2022). Our correlation analysis results also support the idea that Butyricoccus might play a beneficial role in the process of FC mitigation by MOAE.
To date, there are few reports on Tyzzerella. However, it has been reported that Tyzzerella can produce a large number of aromatic amines through the action of aromatic amino acid decarboxylase (Sugiyama et al., 2022), and many aromatic amines are able to promote intestinal motility. Our correlation analysis showed that Tyzzerella was positively correlated with GTR and various intestinal barrier factors and negatively correlated with FBST, SS and various gastrointestinal motility factors. Together, these results suggest that Tyzzerella might also play an important role in FC alleviation by MOAE.
Most studies have observed a possible facilitative role of Desulfovibrio in the development of constipation (Xu et al., 2022), and some drugs have been shown to reduce the abundance of Desulfovibrio in the treatment of constipation (Liu et al., 2019; Jiang et al., 2020). However, it has also been shown that D. vulgaris from the genus Desulfovibrio is an effective producer of acetic acid, which may be effective in reducing hepatic steatosis in mice by producing acetic acid and regulating hepatic lipid metabolism (Hong et al., 2021). In addition, some probiotics or prebiotics could also significantly enhance the abundance of Desulfovibrio in mice (Cui et al., 2022; Dong et al., 2022). Our results suggest that Desulfovibrio may play a beneficial role in the alleviation of FC by MOAE, as Desulfovibrio was significantly positively correlated with GTR and intestinal barrier factors and negatively correlated with FBST, gastrointestinal motility factors, and short-chain fatty acid content.
In addition, several other microbial taxa were significantly enriched under MOAE treatment, including norank_f_Lachnospiraceae, Lachnospiraceae_UCG-010, Colidextribacter, UCG-003, Family_XIII_UCG-001, and norank_ f_norank_o_norank_c_Clostridia. They also showed many correlations with host indicators, but due to the lack of literature, we cannot objectively comment on their role in the mitigation of FC by MOAE.
Microbes themselves affect gastrointestinal motility, and metabolites and components of microbes interact with the ENS. SCFAs can act directly on colonic and ileal smooth muscle (Rondeau et al., 2003), and SCFAs can also regulate 5-HT biosynthesis, thereby affecting colonic peristalsis (Fukui et al., 2018). Notably, the modulation of intestinal motility by SCFAs may be biphasic (Zhu et al., 2014), with low concentrations of SCFAs promoting intestinal motility and high concentrations of SCFAs triggering intestinal motility disorders (Shaidullov et al., 2021). In summary, the regulation of gut microbes and their metabolites is important for MOAE treatment of loperamide-induced constipation.
In summary, we explored the mechanism of MOAE in improving FC induced by loperamide from multiple perspectives related to the ENS and the intestinal microecosystem. We believe that MOAE could relieve constipation through regulating the ENS-ICCs-SMCs network, intestinal inflammation, the intestinal barrier, intestinal aquaporins, cecal SCFAs and the gut microbiota. Interestingly and importantly, MOAE could affect the entire intestinal microecosystem by inhibiting the growth of some key “constipation-causing” microbiota, such as Bacteroidaceae, Clostridiaceae, Bacteroides, and Ruminococcus, and promoting that of other important “constipation-curing” microbiota, such as Butyricoccus, Tyzzerella, and Desulfovibrio. Meanwhile, the correlations established between the important different gut microbes and core host parameters provide a basis for further elucidating the gut microbiota-host metabolism relationship in FC.
Data availability statement
The raw reads of 16S rRNA gene sequence data were deposited into the NCBI Sequence Read Archive (SRA) database under BioProject accession number PRJNA994313.
Ethics statement
The animal study was approved by Animal Ethics Committee of Yunnan Agriculture University. The study was conducted in accordance with the local legislation and institutional requirements.
Author contributions
XG: Conceptualization, Data curation, Formal analysis, Funding acquisition, Methodology, Visualization, Writing – original draft, Writing – review & editing. WQY: Data curation, Formal analysis, Methodology, Writing – original draft. SiL: Methodology, Writing – original draft. ShL: Writing – original draft. WXY: Methodology, Writing – original draft. SS: Methodology, Writing – original draft. JS: Conceptualization, Funding acquisition, Supervision, Writing – review & editing. YZ: Conceptualization, Supervision, Visualization, Writing – review & editing. YT: Conceptualization, Supervision, Visualization, Writing – review & editing.
Funding
The author(s) declare financial support was received for the research, authorship, and/or publication of this article. This research was funded by the Xingdian talent plan of Yunnan Province (Young talents project), selection projects of high-level scientific and technological talents and innovative team (202305AS350025), Yunnan Province-City Integration Project (202302AN360002), and Yunnan Agricultural Joint Special Project (Key Project, 202301BD070001-026).
Conflict of interest
The authors declare that the research was conducted in the absence of any commercial or financial relationships that could be construed as a potential conflict of interest.
Publisher’s note
All claims expressed in this article are solely those of the authors and do not necessarily represent those of their affiliated organizations, or those of the publisher, the editors and the reviewers. Any product that may be evaluated in this article, or claim that may be made by its manufacturer, is not guaranteed or endorsed by the publisher.
Supplementary material
The Supplementary material for this article can be found online at: https://www.frontiersin.org/articles/10.3389/fmicb.2023.1315402/full#supplementary-material
Abbreviations
AchE Acetylcholinesterase Ano1 Anoctamin 1 Aqp3 Aquaporins 3 Aqp4 Aquaporins 4 ENS Enteric nervous system FC Functional constipation FN Fecal number FW Fecal wet weight GTR Gastrointestinal transit rate Gas Gastrin ICCs Interstitial cells of Cajal IL-1β Interleukin-1β MTL Motilin Muc2 Mucin 2 MO Moringa oleifera Lam. MOAE Moringa oleifera Lam. leaf aqueous extract PGE2 Prostaglandin E2 RyR3 Ryanodine receptor 3 SS Somatostatin SCFAs Short-chain fatty acids SCF Stem cell factor SMCs Smooth muscle cells smMLCK Smooth muscle myosin light chain kinase c-Kit Stem cell factor receptor FBST The defecation time of the first black stool TNF-α Tumor necrosis factor-alpha VIP Vasoactive intestinal polypeptide ZO-1 Zonula occludens protein 1 5-HTR4 5-hydroxytryptamine receptor 4
References
Ali, R., Irfan, M., Akram, U., Vaince, M., Hassan, K., Maqsood, A., et al. (2021). Efficacy of natural formulation containing activated charcoal, calcium Sennosides, peppermint oil, fennel oil, rhubarb extract, and purified sulfur (Nucarb®) in relieving constipation. Cureus 13:e18419. doi: 10.7759/cureus.18419
Barberio, B., Judge, C., Savarino, E. V., and Ford, A. C. (2021). Global prevalence of functional constipation according to the Rome criteria: a systematic review and meta-analysis. Lancet Gastroenterol. Hepatol. 6, 638–648. doi: 10.1016/S2468-1253(21)00111-4
Bhattarai, Y., Williams, B. B., Battaglioli, E. J., Whitaker, W. R., Till, L., Grover, M., et al. (2018). Gut microbiota-produced tryptamine activates an epithelial G-protein-coupled receptor to increase colonic secretion. Cell Host Microbe 23, 775–785.e5. doi: 10.1016/j.chom.2018.05.004
Calignano, A., Moncada, S., and Di Rosa, M. (1991). Endogenous nitric oxide modulates morphine-induced constipation. Biochem. Biophys. Res. Commun. 181, 889–893. doi: 10.1016/0006-291x(91)91274-g
Cao, P. Q., Li, X. P., Ou-Yang, J., Jiang, R. G., Huang, F. F., Wen, B. B., et al. (2021). The protective effects of yellow tea extract against loperamide-induced constipation in mice. Food Funct. 12, 5621–5636. doi: 10.1039/d0fo02969f
Carabotti, M., Scirocco, A., Maselli, M. A., and Severi, C. (2015). The gut-brain axis: interactions between enteric microbiota, central and enteric nervous systems. Ann. Gastroenterol. 28, 203–209.
Chen, C. L., Chao, S. H., and Pan, T. M. (2020). Lactobacillus paracasei subsp. paracasei NTU 101 lyophilized powder improves loperamide-induced constipation in rats. Heliyon 6:e03804. doi: 10.1016/j.heliyon.2020.e03804
Chen, Y., Ding, Z., Wu, Y., Chen, Q., Liu, M., Yu, H., et al. (2019). Effects of Allium mongolicum regel and its flavonoids on constipation. Biomol. Ther. 10:14. doi: 10.3390/biom10010014
Choi, Y. J., Kim, J. E., Lee, S. J., Gong, J. E., Son, H. J., Hong, J. T., et al. (2021). Dysbiosis of fecal microbiota from complement 3 knockout mice with constipation phenotypes contributes to development of defecation delay. Front. Physiol. 12:650789. doi: 10.3389/fphys.2021.650789
Cui, S., Guo, W., Chen, C., Tang, X., Zhao, J., Mao, B., et al. (2022). Metagenomic analysis of the effects of Lactiplantibacillus plantarum and Fructooligosaccharides (FOS) on the fecal microbiota structure in mice. Foods 11:1187. doi: 10.3390/foods11091187
Dong, J., Ping, L., Zhang, K., Tang, H., Liu, J., Liu, D., et al. (2022). Immunomodulatory effects of mixed Lactobacillus plantarum on lipopolysaccharide-induced intestinal injury in mice. Food Funct. 13, 4914–4929. doi: 10.1039/d1fo04204a
Dupont, C., Constant, F., Imbert, A., Hébert, G., Zourabichvili, O., and Kapel, N. (2019). Time to treatment response of a magnesium-and sulphate-rich natural mineral water in functional constipation. Nutrition 65, 167–172. doi: 10.1016/j.nut.2019.02.018
Eeckhaut, V., Machiels, K., Perrier, C., Romero, C., Maes, S., Flahou, B., et al. (2013). Butyricicoccus pullicaecorum in inflammatory bowel disease. Gut 62, 1745–1752. doi: 10.1136/gutjnl-2012-303611
Elabd, E. M. Y., Morsy, S. M., and Elmalt, H. A. (2018). Investigating of Moringa Oleifera role on gut microbiota composition and inflammation associated with obesity following high fat diet feeding. Open Access Maced J Med Sci 6, 1359–1364. doi: 10.3889/oamjms.2018.313
Feng, L., Li, W., Liu, Y., Jiang, W. D., Kuang, S. Y., Jiang, J., et al. (2015). Dietary phenylalanine-improved intestinal barrier health in young grass carp (Ctenopharyngodon idella) is associated with increased immune status and regulated gene expression of cytokines, tight junction proteins, antioxidant enzymes and related signalling molecules. Fish Shellfish Immunol. 45, 495–509. doi: 10.1016/j.fsi.2015.05.001
Fukui, H., Xu, X., and Miwa, H. (2018). Role of gut microbiota-gut hormone Axis in the pathophysiology of functional gastrointestinal disorders. J Neurogastroenterol Motil 24, 367–386. doi: 10.5056/jnm18071
Fukumoto, S., Tatewaki, M., Yamada, T., Fujimiya, M., Mantyh, C., Voss, M., et al. (2003). Short-chain fatty acids stimulate colonic transit via intraluminal 5-HT release in rats. Am. J. Physiol. Regul. Integr. Comp. Physiol. 284, R1269–R1276. doi: 10.1152/ajpregu.00442.2002
Gao, X., Chang, S., Liu, S., Peng, L., Xie, J., Dong, W., et al. (2020). Correlations between α-linolenic acid-improved multitissue homeostasis and gut microbiota in mice fed a high-fat diet. mSystems 5, e00391–e00320. doi: 10.1128/mSystems.00391-20
Gao, H., He, C., Hua, R., Liang, C., Wang, B., Du, Y., et al. (2022). Underlying beneficial effects of rhubarb on constipation-induced inflammation, disorder of gut microbiome and metabolism. Front. Pharmacol. 13:1048134. doi: 10.3389/fphar.2022.1048134
Gao, X., Hu, Y., Tao, Y., Liu, S., Chen, H., Li, J., et al. (2022). Cymbopogon citratus (DC.) Stapf aqueous extract ameliorates loperamide-induced constipation in mice by promoting gastrointestinal motility and regulating the gut microbiota. Front. Microbiol. 13:1017804. doi: 10.3389/fmicb.2022.1017804
Gao, X., Xie, Q., Liu, L., Kong, P., Sheng, J., and Xiang, H. (2017). Metabolic adaptation to the aqueous leaf extract of Moringa oleifera Lam.-supplemented diet is related to the modulation of gut microbiota in mice. Appl. Microbiol. Biotechnol. 101, 5115–5130. doi: 10.1007/s00253-017-8233-5
Grimble, G. K. (2007). Adverse gastrointestinal effects of arginine and related amino acids. J. Nutr. 137, 1693S–1701S. doi: 10.1093/jn/137.6.1693S
Guo, M., Yao, J., Yang, F., Liu, W., Bai, H., Ma, J., et al. (2020). The composition of intestinal microbiota and its association with functional constipation of the elderly patients. Future Microbiol. 15, 163–175. doi: 10.2217/fmb-2019-0283
Hayeeawaema, F., Wichienchot, S., and Khuituan, P. (2020). Amelioration of gut dysbiosis and gastrointestinal motility by konjac oligo-glucomannan on loperamide-induced constipation in mice. Nutrition 73:110715. doi: 10.1016/j.nut.2019.110715
Hong, Y., Sheng, L., Zhong, J., Tao, X., Zhu, W., Ma, J., et al. (2021). Desulfovibrio vulgaris, a potent acetic acid-producing bacterium, attenuates nonalcoholic fatty liver disease in mice. Gut Microbes 13, 1–20. doi: 10.1080/19490976.2021.1930874
Horvath, T. D., Ihekweazu, F. D., Haidacher, S. J., Ruan, W., Engevik, K. A., Fultz, R., et al. (2022). Bacteroides ovatus colonization influences the abundance of intestinal short chain fatty acids and neurotransmitters. iScience 25:104158. doi: 10.1016/j.isci.2022.104158
Hu, Y., Gao, X., Zhao, Y., Liu, S., Luo, K., Fu, X., et al. (2023). Flavonoids in Amomum tsaoko Crevost et Lemarie ameliorate Loperamide-induced constipation in mice by regulating gut microbiota and related metabolites. Int. J. Mol. Sci. 24:7191. doi: 10.3390/ijms24087191
Huang, J., Lin, B., Zhang, Y., Xie, Z., Zheng, Y., Wang, Q., et al. (2022). Bamboo shavings derived O-acetylated xylan alleviates loperamide-induced constipation in mice. Carbohydr. Polym. 276:118761. doi: 10.1016/j.carbpol.2021.118761
Imamura, T., and Kinugawa, K. (2022). Successful conversion from conventional potassium binder to sodium zirconium Cyclosilicate in a patient with refractory constipation. Medicina (Kaunas) 58:635. doi: 10.3390/medicina58050635
Jiang, H., Dong, J., Jiang, S., Liang, Q., Zhang, Y., Liu, Z., et al. (2020). Effect of Durio zibethinus rind polysaccharide on functional constipation and intestinal microbiota in rats. Food Res. Int. 136:109316. doi: 10.1016/j.foodres.2020.109316
Joly, A., Leulier, F., and De Vadder, F. (2021). Microbial modulation of the development and physiology of the enteric nervous system. Trends Microbiol. 29, 686–699. doi: 10.1016/j.tim.2020.11.007
Karan, R. S., Kumar, R., and Pandhi, P. (2000). Effect of acute and chronic administration of L-arginine on morphine induced inhibition of gastrointestinal motility. Indian J. Physiol. Pharmacol. 44, 345–349.
Kennedy, P. J., Clarke, G., Quigley, E. M., Groeger, J. A., Dinan, T. G., and Cryan, J. F. (2012). Gut memories: towards a cognitive neurobiology of irritable bowel syndrome. Neurosci. Biobehav. Rev. 36, 310–340. doi: 10.1016/j.neubiorev.2011.07.001
Li, C., Dong, Z., Zhang, B., Huang, Q., Liu, G., and Fu, X. (2020). Structural characterization and immune enhancement activity of a novel polysaccharide from Moringa oleifera leaves. Carbohydr. Polym. 234:115897. doi: 10.1016/j.carbpol.2020.115897
Li, R., Li, M., Li, B., Chen, W. H., and Liu, Z. (2022). Cannabis sativa L. alleviates loperamide-induced constipation by modulating the composition of gut microbiota in mice. Front. Pharmacol. 13:1033069. doi: 10.3389/fphar.2022.1033069
Li, C., Li, Z., Wu, H., Tang, S., Zhang, Y., Yang, B., et al. (2022). Therapeutic effect of Moringa oleifera leaves on constipation mice based on pharmacodynamics and serum metabonomics. J. Ethnopharmacol. 282:114644. doi: 10.1016/j.jep.2021.114644
Li, M., Liu, Y., Gu, W., Li, X., Wen, H., Long, X., et al. (2019). Influence of Moringa oleifera and Moringa oleifera purgative compound on improving defecation and gastrointestinal hormones in constipated rats. Drug Dev. Res. 42, 1291–1296. doi: 10.7501/j.issn.1674-6376.2019.07.006
Li, L., Ma, L., Wen, Y., Xie, J., Yan, L., Ji, A., et al. (2022). Crude polysaccharide extracted from Moringa oleifera leaves prevents obesity in association with modulating gut microbiota in high-fat diet-fed mice. Front. Nutr. 9:861588. doi: 10.3389/fnut.2022.861588
Liu, M. T., Kuan, Y. H., Wang, J., Hen, R., and Gershon, M. D. (2009). 5-HT4 receptor-mediated neuroprotection and neurogenesis in the enteric nervous system of adult mice. J. Neurosci. 29, 9683–9699. doi: 10.1523/JNEUROSCI.1145-09.2009
Liu, D., Lin, L., Lin, Y., Zhong, Y., Zhang, S., Liu, W., et al. (2019). Zengye decoction induces alterations to metabolically active gut microbiota in aged constipated rats. Biomed. Pharmacother. 109, 1361–1371. doi: 10.1016/j.biopha.2018.11.013
Liu, G., Wang, Z., Li, X., Liu, R., Li, B., Huang, L., et al. (2020). Total glucosides of paeony (TGP) alleviates constipation and intestinal inflammation in mice induced by Sjögren's syndrome. J. Ethnopharmacol. 260:113056. doi: 10.1016/j.jep.2020.113056
Ma, H., Xiong, H., Zhu, X., Ji, C., Xue, J., Li, R., et al. (2019). Polysaccharide from Spirulina platensis ameliorates diphenoxylate-induced constipation symptoms in mice. Int. J. Biol. Macromol. 133, 1090–1101. doi: 10.1016/j.ijbiomac.2019.04.209
Ma, L., Zhao, X., Liu, T., Wang, Y., Wang, J., Kong, L., et al. (2022). Xuanfei Baidu decoction attenuates intestinal disorders by modulating NF-κB pathway, regulating T cell immunity and improving intestinal microbiota. Phytomedicine 101:154100. doi: 10.1016/j.phymed.2022.154100
Mohamed Husien, H., Peng, W., Su, H., Zhou, R., Tao, Y., Huang, J., et al. (2022). Moringa oleifera leaf polysaccharide alleviates experimental colitis by inhibiting inflammation and maintaining intestinal barrier. Front. Nutr. 9:1055791. doi: 10.3389/fnut.2022.1055791
Mori, H., Tack, J., and Suzuki, H. (2021). Magnesium oxide in constipation. Nutrients 13:421. doi: 10.3390/nu13020421
Muvhulawa, N., Dludla, P. V., Ziqubu, K., Mthembu, S. X. H., Mthiyane, F., Nkambule, B. B., et al. (2022). Rutin ameliorates inflammation and improves metabolic function: a comprehensive analysis of scientific literature. Pharmacol. Res. 178:106163. doi: 10.1016/j.phrs.2022.106163
Niu, J., Xu, L., Qian, Y., Sun, Z., Yu, D., Huang, J., et al. (2020). Evolution of the gut microbiome in early childhood: a cross-sectional study of Chinese children. Front. Microbiol. 11:439. doi: 10.3389/fmicb.2020.00439
Pan, R., Wang, L., Xu, X., Chen, Y., Wang, H., Wang, G., et al. (2022). Crosstalk between the gut microbiome and colonic motility in chronic constipation: potential mechanisms and microbiota modulation. Nutrients 14:3704. doi: 10.3390/nu14183704
Peng, Y., Gan, R., Li, H., Yang, M., McClements, D. J., Gao, R., et al. (2021). Absorption, metabolism, and bioactivity of vitexin: recent advances in understanding the efficacy of an important nutraceutical. Crit. Rev. Food Sci. Nutr. 61, 1049–1064. doi: 10.1080/10408398.2020.1753165
Rebollar, E., Arruebo, M. P., Plaza, M. A., and Murillo, M. D. (2002). Effect of lipopolysaccharide on rabbit small intestine muscle contractility in vitro: role of prostaglandins. Neurogastroenterol. Motil. 14, 633–642. doi: 10.1046/j.1365-2982.2002.00364.x
Riaz, A., Rasul, A., Hussain, G., Zahoor, M. K., Jabeen, F., Subhani, Z., et al. (2018). Astragalin: a bioactive phytochemical with potential therapeutic activities. Adv. Pharmacol. Sci. 2018, 9794625–9794615. doi: 10.1155/2018/9794625
Rondeau, M. P., Meltzer, K., Michel, K. E., McManus, C. M., and Washabau, R. J. (2003). Short chain fatty acids stimulate feline colonic smooth muscle contraction. J. Feline Med. Surg. 5, 167–173. doi: 10.1016/S1098-612X(03)00002-0
Senthilkumar, A., Karuvantevida, N., Rastrelli, L., Kurup, S. S., and Cheruth, A. J. (2018). Traditional uses, pharmacological efficacy, and Phytochemistry of Moringa peregrina (Forssk.). Rev. Front. Pharmacol. 9:465. doi: 10.3389/fphar.2018.00465
Shaidullov, I. F., Sorokina, D. M., Sitdikov, F. G., Hermann, A., Abdulkhakov, S. R., and Sitdikova, G. F. (2021). Short chain fatty acids and colon motility in a mouse model of irritable bowel syndrome. BMC Gastroenterol. 21:37. doi: 10.1186/s12876-021-01613-y
Shatri, H., Faisal, E., Abdullah, M., Syam, A. F., Utari, A. P., Muzellina, V. N., et al. (2023). Depression symptoms and inflammation in chronic functional constipation patients. Acta Med. Indones. 55, 33–39.
Sugiyama, Y., Mori, Y., Nara, M., Kotani, Y., Nagai, E., Kawada, H., et al. (2022). Gut bacterial aromatic amine production: aromatic amino acid decarboxylase and its effects on peripheral serotonin production. Gut Microbes 14:2128605. doi: 10.1080/19490976.2022.2128605
Sun, F., Zhang, Q., Zhao, J., Zhang, H., Zhai, Q., and Chen, W. (2019). A potential species of next-generation probiotics? The dark and light sides of Bacteroides fragilis in health. Food Res. Int. 126:108590. doi: 10.1016/j.foodres.2019.108590
Tian, H., Chen, Q., Yang, B., Qin, H., and Li, N. (2021). Analysis of gut microbiome and metabolite characteristics in patients with slow transit constipation. Dig. Dis. Sci. 66, 3026–3035. doi: 10.1007/s10620-020-06500-2
Tian, H., Wen, Z., Liu, Z., Guo, Y., Liu, G., and Sun, B. (2022). Comprehensive analysis of microbiome, metabolome and transcriptome revealed the mechanisms of Moringa oleifera polysaccharide on preventing ulcerative colitis. Int. J. Biol. Macromol. 222, 573–586. doi: 10.1016/j.ijbiomac.2022.09.100
Tian, Y., Zuo, L., Guo, Q., Li, J., Hu, Z., Zhao, K., et al. (2020). Potential role of fecal microbiota in patients with constipation. Ther. Adv. Gastroenterol. 13:175628482096842. doi: 10.1177/1756284820968423
Tsakiris, S. (2001). Effects of L-phenylalanine on acetylcholinesterase and Na(+), K(+)-ATPase activities in adult and aged rat brain. Mech. Ageing Dev. 122, 491–501. doi: 10.1016/s0047-6374(01)00217-2
Tsakiris, S., Kouniniotou-Krontiri, P., and Schulpis, K. H. (1998). L-phenylalanine effect on rat diaphragm acetylcholinesterase and Na+, K(+)-ATPase. Z. Naturforsch. C. J. Biosci. 53, 1055–1060. doi: 10.1515/znc-1998-11-1219
Vacca, L., Proietti, S., Bravi, D., Radicati, F. G., and Stocchi, F. (2022). Effectiveness of an herbaceous derivatives, PHGG, plus sodium hyaluronate in the treatment of chronic constipation in patients with Parkinson's disease: a pilot study. Neurol. Sci. 43, 1055–1059. doi: 10.1007/s10072-021-05342-8
Valentová, K., Vrba, J., Bancířová, M., Ulrichová, J., and Křen, V. (2014). Isoquercitrin: pharmacology, toxicology, and metabolism. Food Chem. Toxicol. 68, 267–282. doi: 10.1016/j.fct.2014.03.018
Valguarnera, E., and Wardenburg, J. B. (2020). Good gone bad: one toxin away from disease for Bacteroides fragilis. J. Mol. Biol. 432, 765–785. doi: 10.1016/j.jmb.2019.12.003
Vriesman, M. H., Koppen, I. J. N., Camilleri, M., Di Lorenzo, C., and Benninga, M. A. (2020). Management of functional constipation in children and adults. Nat. Rev. Gastroenterol. Hepatol. 17, 21–39. doi: 10.1038/s41575-019-0222-y
Wang, L., Hu, L., Yan, S., Jiang, T., Fang, S., Wang, G., et al. (2017). Effects of different oligosaccharides at various dosages on the composition of gut microbiota and short-chain fatty acids in mice with constipation. Food Funct. 8, 1966–1978. doi: 10.1039/c7fo00031f
Wang, J., Ji, H., Wang, S., Liu, H., Zhang, W., Zhang, D., et al. (2018). Probiotic Lactobacillus plantarum promotes intestinal barrier function by strengthening the epithelium and modulating gut microbiota. Front. Microbiol. 9:1953. doi: 10.3389/fmicb.2018.01953
Wang, Y., Li, N., Yang, J. J., Zhao, D. M., Chen, B., Zhang, G. Q., et al. (2020). Probiotics and fructo-oligosaccharide intervention modulate the microbiota-gut brain axis to improve autism spectrum reducing also the hyper-serotonergic state and the dopamine metabolism disorder. Pharmacol. Res. 157:104784. doi: 10.1016/j.phrs.2020.104784
Wang, J., Liang, Q., Zhao, Q., Tang, Q., Ahmed, A. F., Zhang, Y., et al. (2021). The effect of microbial composition and proteomic on improvement of functional constipation by Chrysanthemum morifolium polysaccharide. Food Chem. Toxicol. 153:112305. doi: 10.1016/j.fct.2021.112305
Wang, J., Wang, L., Yu, Q., Tang, N., Mei, C., Zhang, H., et al. (2023). Characteristics of the gut microbiome and serum metabolome in patients with functional constipation. Nutrients 15:1779. doi: 10.3390/nu15071779
Wang, G., Yang, S., Sun, S., Si, Q., Wang, L., Zhang, Q., et al. (2020). Lactobacillus rhamnosus strains relieve Loperamide-induced constipation via different pathways independent of short-chain fatty acids. Front. Cell. Infect. Microbiol. 10:423. doi: 10.3389/fcimb.2020.00423
Wu, L., Jin, X., Zheng, C., Ma, F., Zhang, X., Gao, P., et al. (2023). Bidirectional effects of Mao Jian green tea and its flavonoid glycosides on gastrointestinal motility. Foods 12:854. doi: 10.3390/foods12040854
Xian, Y., Zhao, X., Wang, C., Kang, C., Ding, L., Zhu, W., et al. (2018). Phenylalanine and tryptophan stimulate gastrin and somatostatin secretion and H+-K+-ATPase activity in pigs through calcium-sensing receptor. Gen. Comp. Endocrinol. 267, 1–8. doi: 10.1016/j.ygcen.2018.05.022
Xu, Y., Shao, M., Fang, X., Tang, W., Zhou, C., Hu, X., et al. (2022). Antipsychotic-induced gastrointestinal hypomotility and the alteration in gut microbiota in patients with schizophrenia. Brain Behav. Immun. 99, 119–129. doi: 10.1016/j.bbi.2021.09.014
Yang, C., Bai, X., Hu, T., Xue, X., Su, X., Zhang, X., et al. (2022). Integrated metagenomics and targeted-metabolomics analysis of the effects of phenylalanine on loperamide-induced constipation in rats. Front. Microbiol. 13:1018008. doi: 10.3389/fmicb.2022.1018008
Yano, J. M., Yu, K., Donaldson, G. P., Shastri, G. G., Ann, P., Ma, L., et al. (2015). Indigenous bacteria from the gut microbiota regulate host serotonin biosynthesis. Cells 161, 264–276. doi: 10.1016/j.cell.2015.02.047
Yu, T., Ding, Y., Qian, D., Lin, L., and Tang, Y. (2023). Characteristics of fecal microbiota in different constipation subtypes and association with colon physiology, lifestyle factors, and psychological status. Ther. Adv. Gastroenterol. 16:175628482311541. doi: 10.1177/17562848231154101
Zhai, L., Huang, C., Ning, Z., Zhang, Y., Zhuang, M., Yang, W., et al. (2023). Ruminococcus gnavus plays a pathogenic role in diarrhea-predominant irritable bowel syndrome by increasing serotonin biosynthesis. Cell Host Microbe 31, 33–44.e5. doi: 10.1016/j.chom.2022.11.006
Zhang, L., Du, Z., Li, Z., Yu, F., and Li, L. (2021). Association of dietary magnesium intake with chronic constipation among US adults: evidence from the National Health and nutrition examination survey. Food Sci. Nutr. 9, 6634–6641. doi: 10.1002/fsn3.2611
Zhang, H., Zheng, Y., Zha, X., Ma, Y., Liu, X., Elsabagh, M., et al. (2022). Dietary L-arginine or N-Carbamylglutamate alleviates colonic barrier injury, oxidative stress, and inflammation by modulation of intestinal microbiota in intrauterine growth-retarded suckling lambs. Antioxidants (Basel) 11:2251. doi: 10.3390/antiox11112251
Zhao, X., Wang, L., and Quan, L. (2023). Association between dietary phosphorus intake and chronic constipation in adults: evidence from the National Health and nutrition examination survey. BMC Gastroenterol. 23:24. doi: 10.1186/s12876-022-02629-8
Zhou, X., Chen, Y., Ma, X., Yu, Y., Yu, X., Chen, X., et al. (2022). Efficacy of Bacillus coagulans BC01 on loperamide hydrochloride-induced constipation model in Kunming mice. Front. Nutr. 9:964257. doi: 10.3389/fnut.2022.964257
Zhu, G. Y., Jia, D. D., Yang, Y., Miao, Y., Wang, C., and Wang, C. M. (2021). The effect of Shaoyao Gancao decoction on sphincter of Oddi dysfunction in Hypercholesterolemic rabbits via protecting the enteric nervous system-interstitial cells of Cajal-smooth muscle cells network. J. Inflamm. Res. 14, 4615–4628. doi: 10.2147/JIR.S326416
Zhu, L., Liu, W., Alkhouri, R., Baker, R. D., Bard, J. E., Quigley, E. M., et al. (2014). Structural changes in the gut microbiome of constipated patients. Physiol. Genomics 46, 679–686. doi: 10.1152/physiolgenomics.00082.2014
Keywords:
Citation: Gao X, Yang W, Li S, Liu S, Yang W, Song S, Sheng J, Zhao Y and Tian Y (2023) Moringa oleifera leaf alleviates functional constipation via regulating the gut microbiota and the enteric nervous system in mice. Front. Microbiol. 14:1315402. doi: 10.3389/fmicb.2023.1315402
Edited by:
Faming Zhang, Nanjing Medical University, ChinaCopyright © 2023 Gao, Yang, Li, Liu, Yang, Song, Sheng, Zhao and Tian. This is an open-access article distributed under the terms of the Creative Commons Attribution License (CC BY). The use, distribution or reproduction in other forums is permitted, provided the original author(s) and the copyright owner(s) are credited and that the original publication in this journal is cited, in accordance with accepted academic practice. No use, distribution or reproduction is permitted which does not comply with these terms.
*Correspondence: Jun Sheng, shengj@ynau.edu.cn; Yan Zhao, 2021013@ynau.edu.cn; Yang Tian, tianyang@ynau.edu.cn
†These authors have contributed equally to this work