- Agro-Products Quality Safety and Testing Technology Research Institute, Guangxi Academy of Agricultural Sciences, Nanning, China
Sugarcane (Saccharum officinarum L.) may be infected with Apiospora, which can produce the toxin 3-nitropropionic acid (3-NPA) during improper transportation and storage. The consumption of sugarcane that contains 3-NPA can lead to food poisoning. Therefore, this study sought to explore a novel biocontrol agent to prevent and control Apiospora mold. Bacteria were isolated from the soil of healthy sugarcane and identified as Bacillus velezensis T9 through colony morphological, physiological and biochemical characterization and molecular identification. The inhibitory effect of B. velezensis T9 on Apiospora mold on sugarcane was analyzed. Assays of the cell suspension of strain T9 and its cell-free supernatant showed that T9 had significant in vitro antifungal activities against Apiospora arundinis and thus, would be a likely antagonist. Scanning electron microscopy and transmission electron microscopy showed that treatment with T9 significantly distorted the A. arundinis mycelia, perforated the membrane, contracted the vesicles, and decomposed most organelles into irregular fragments. A re-isolation experiment demonstrates the ability of T9 to colonize the sugarcane stems and survive in them. This strain can produce volatile organic compounds (VOCs) that are remarkably strong inhibitors, and it can also form biofilms. Additionally, the cell-free supernatant significantly reduced the ability of A. arundinis to produce 3-NPA and completely inhibited its production at 10%. Therefore, strain T9 is effective at controlling A. arundinis and has the potential for further development as a fungal prevention agent for agricultural products.
Introduction
Sugarcane (Saccharum officinarum L.) is the primary sugar crop that is cultivated in tropical and subtropical regions (Souza et al., 2019; Dhansu et al., 2023). As a fresh product, chewing cane is widely cultivated in many countries of the world due to its high contents of amino acids, vitamins, and trace elements that are essential for human survival. In China, the area planted to fresh sugarcane reaches 230,000 hm2 per year, with an output that exceeds 35 million tons (Wang et al., 2020). However, it is easily infected by various pathogens during transportation and storage. Ever since the first case report of poisoning due to the consumption of moldy sugarcane in northern China in 1972 (Liu et al., 1984), more cases have been recorded almost every year. Apiospora has been identified as the etiological fungus that causes moldy sugarcane poisoning (MSP) during improper postharvest storage (Liu et al., 1984; Hou et al., 1989; Liu et al., 1993). Notably, the toxic metabolite 3-nitropropionic acid (3-NPA) produced by Apiospora has been identified as the causal agent of poisoning (Hu et al., 1986). Based on the morphological characteristics, such as the shape of conidia and conidiophores, species of Apiospora that have been isolated from moldy poisoned sugarcane can be classified and identified as Apiospora. sacchari, Apiospora. saccharicola and Apiospora. phaeospermum (Liu et al., 1988). In our previous study, A. arundinis that produced 3-NPA was isolated from moldy sugarcane (Liao et al., 2022).
3-NPA is a small molecular biotoxin with a simple structure, and it primarily originates from moldy sugarcane caused by Apiospora. However, it can also be found in some plants infected with other fungi (Hajnal et al., 2020). Large amounts of 3-NPA are reported to be highly toxic to humans and animals and cause central nervous system (CNS) lesions. The clinical manifestations of 3-NPA include mild gastrointestinal symptoms, such as nausea, vomiting, abdominal pain, and diarrhea, that occur at approximately 2 h to 5 h after the consumption of moldy sugarcane. Some patients have vertigo, blurred vision, and an inability to stand, with trembling limbs, prophylaxis tetanus, and coma in severe cases. More people with severe cases die within 1–3 d or develop neurological sequelae that are similar to encephalitis and lose the ability to care for themselves (He et al., 1995; Nony et al., 1999; Birkelund et al., 2021). Therefore, preventing sugarcane from fungal colonization during storage or transportation has become an urgent scientific problem.
Currently, the main measures to ensure the safety and edibility of chewing cane are to observe whether the chewing cane has symptoms of mold, such as light yellow brown, dark brown, or red patches, and control the storage period. However, there are few reports on chemical or biological methods to control A. arundinis. The prolonged use of chemical fungicides will lead to the resistance of pathogenic fungi, which could potentially harm human health and environmental safety. In this regard, biocontrol agents have emerged as a potential alternative to chemical fungicides to prevent and control various diseases due to their green and pollution-free characteristics (Niem et al., 2020; Huang et al., 2021; Yuan et al., 2022). The antagonistic microorganisms that can inhibit the postharvest diseases of fruits and vegetables include species of Candida, Bacillus, Pseudomonas, yeast, and Wickerhamomyces (Spadaro and Droby, 2016; Kang et al., 2019; Khalifa et al., 2022). Among them, Bacillus has been widely isolated and utilized as an effective biocontrol agent. For example, B. subtilis JK14 isolated from the surface of peach (Prunus persica [L.] Batsch) fruits has excellent effects at controlling postharvest rot of peaches and can be used as an environmentally safe biological control agent for this purpose (Zhang et al., 2019). Similarly, the endophytic strain CC09 of B. velezensis is effective at controlling wheat (Triticum aestivum [L.]) diseases (Kang et al., 2018).
In this study, 10 strains of bacteria with antagonistic ability against A. arundinis were isolated from the soil of healthy sugarcane roots. Among them, one strain of B. velezensis designated T9 was highly effective at inhibiting A. arundinis, the causal agent of sugarcane mold. Overall, this research provides a novel microbial material to control sugarcane mold during postharvest storage and a theoretical basis to develop and broaden the application of microbial preservatives.
Materials and methods
Fungal preparation
A. arundinis LX1918, isolated from moldy sugarcane in our preliminary study and stored in the laboratory, was used as the research object (Liao et al., 2022). A. arundinis LX1918 was cultured on potato dextrose agar (PDA) at 28°C.
Isolation of antagonistic bacterial strains
The soil was collected from the rhizosphere soil of healthy sugarcane at the Guangxi Academy of Agricultural Sciences (Nanning, China), and the bacteria in the soil samples examined were isolated by dilution plate separation (Filippi et al., 2011). A total of 10.0 g of soil was weighed and placed in a triangular bottle that contained glass beads and 90 mL of sterile water, shaken at 28°C and 180 rpm for 30 min and diluted with gradients of 10−3, 10−4, and 10−5. The nutrient broth (NB) plate that contained each diluent was cultured in a constant temperature incubator at 28°C for 48 h, and single colonies with obvious morphological differences were selected and purified. The purified strains were stored at 4°C for later use.
In vitro screening of potential fungal biocontrol agents
The antagonistic ability of the isolated strains toward plant fungal pathogens was determined through a modified dual-culture test using A. arundinis LX1918 as an indicator fungus (Yu et al., 2011; Liu et al., 2021). The isolated strains were cultured overnight in LB at 180 rpm, 28°C. Under aseptic conditions, the mycelial plugs (5 mm in diameter) of a 5 days-old culture of A. arundinis were inoculated in the center of PDA plates, and 2 μL of a suspension of strain T9 (OD600 = 1.0) were inoculated 2.5 cm from the center on each side of the PDA media in petri dishes. The negative control was inoculated with the pathogenic fungal isolate. The plates were incubated at 28°C for 6 d, and the colony diameter of the pathogenic fungus of each plate was measured. The experiment was repeated three times, and each sample (all the isolates) was treated in triplicate.
The morphology and ultrastructure of the A. arundinis mycelia exposed to T9 were observed by scanning electron microscopy (SEM) and transmission electron microscopy (TEM). The samples were fixed with 2.5% glutaraldehyde at 4°C for 24 h and dehydrated in an ethanol series (30, 50, 70, 80, 95, and 100%) for 20 min at each stage. After being frozen and coated with gold, the hyphae were observed under SEM (S-3400 N; Hitachi, Tokyo, Japan) and TEM (HT7700; Hitachi).
Identification of the antagonistic bacterium T9
Strain T9 that exhibited the strongest antifungal activity was identified by morphological and molecular identification as described in Berger’s Manual of Bacterial Identification (Holt et al., 1994) and the Common Bacterial Systems Identification Manual (Dong and Cai, 2001; Vos et al., 2011). The physiological and biochemical experiments were performed on strain T9, including gram staining, growth temperature, pH tolerance, salt tolerance, starch hydrolysis, VP test, carbon source utilization, and contact enzymes among others. The experiment was performed in triplicate.
The genomic DNA was extracted and purified using a Bacterial Genome DNA Extraction Kit. The 16S rDNA, gyrA, and ropB gene sequences of strain T9 were amplified using the primers listed in Table 1. After 1% agarose gel electrophoresis, the PCR amplification products were sent for sequencing, and the sequencing results were submitted to GenBank. The comparison of similarity was performed with the known sequences in the GenBank database, and the phylogenetic tree was constructed by neighbor-joining using MEGA 7.0 (Kumar et al., 2016). The bootstrap value was set to 1,000.
Effect of the supernatant of Bacillus velezensis T9 on the mycelial growth of Apiospora arundinis
A suspension of strain T9 (OD600 = 1.0) was inoculated in a triangular flask that contained 100 mL of NB and a volume of 1% inoculum and cultured at 28°C for 72 h. The samples were then centrifuged at 3500 rpm for 20 min, and the fermentation supernatant was filtered through a 0.22 μm microporous membrane to obtain the cell-free supernatant.
PDA that contained 1, 3, 5, and 10% of the T9 cell-free supernatant was prepared at 40°C and poured into a plate. Each plate was inoculated with A. arundinis plugs (5 mm in diameter). The culture was repeated three times at 28°C for 6 d with three replicates. The colony diameter was measured by cross-over trials, and the mycelial growth rate and rate of inhibition of each treatment were calculated using the following equations:
Approximately 100 mL of potato dextrose broth (PDB) that contained 1, 3, 5, and 10% T9 cell-free supernatant was prepared, and 100 μL of an A. arundinis spore suspension (1 × 105 spores mL−1) was added to the PDB and cultured at 28°C, 180 rpm for 7 d. Cultures that lacked T9 cell-free supernatant were used as the control. The treatment was performed in triplicate. The PDB was filtered through four layers of sterile gauze to obtain the wet hyphae. The hyphae were dried at 50°C and weighed. The amount of mycelial growth and its rate of inhibition were calculated using the following equations:
Control effect of Bacillus velezensis strain T9 on sugarcane infected with Apiospora arundinis
The ability of strain T9 to control disease on sugarcane infected with A. arundinis was identified using sugarcane stems as previously described with minor modifications (Huang et al., 2021). Wounds (3 mm deep and 5 mm wide) were made on each sugarcane stem using a sterile borer. Each wound was injected with a 1 × 108 CFU/mL suspension of B. velezensis T9 (100 μL) or supernatant, while sterile water and protamine were used in the negative and positive control group, respectively. After standing for 24 h, the A. arundinis cake with a diameter of 5 mm was inoculated on the sugarcane wound, and the diameter of lesions was measured with Vernier calipers on days 5 and 10. A small piece of the sugarcane tissue (3 mm × 3 mm) was taken from the distal end of the disease site, disinfected with 75% ethanol, and washed with sterile water. Finally, the tissue was placed on PDA and cultured in a 28°C incubator for 5 d.
Inhibitory effect of Bacillus velezensis T9 on the production of 3-NPA By Apiospora arundinis
The suspension of A. arundinis spores (105 CFU/mL) was added to PDB that contained 1, 3, 5, or 10% of cell-free supernatant from strain T9, and the cultures were incubated at 28°C for 15 d with shaking at 140 rpm. A volume of 2 mL of the PDB culture filtered through four layers of gauze was added with 10 mL of acetonitrile and then vortexed for 10 min. After a period of time, 1.5 g of NaCl was added to the mixture after ultrasonic extraction for 20 min, vortexed for 2 min, and centrifuged at 4000 rpm for 5 min. Afterward, 5 mL of the cell-free supernatant was taken and evaporated under a stream of N2, and the residue was dissolved in 1 mL of distilled water. It was then passed through a filtration membrane. The filtrate was analyzed using HPLC (Waters, Milford, MA, United States) coupled to a two-stage array detector and an Agilent XDB-C18 column (250 × 4.6 mm, 5 μm particle size) (Agilent Technologies, Santa Clara, CA, United States). The mobile phase (phosphoric acid: methanol [97: 3]) was pumped at a flow rate of 1.0 mL/min. The detection wavelength was 208 nm. The amount of 3-NPA produced by the three fungal isolates was calculated by determining the peak area of the compound in the chromatogram and comparing it to a known standard (Wei et al., 1994). The rate of inhibition of 3-NPA was calculated using the following equation:
Effect of VOCs on the mycelial growth of Apiospora arundinis
The inhibitory effects of the volatile organic compounds (VOCs) produced by strain T9 on the growth of A. arundinis were studied using the two-sealed-base-plates method (Gao et al., 2017; Grzegorczyk et al., 2017).
A bacterial T9 suspension (108 cells/mL) was prepared, and 300 μL of the suspension was seeded on a nutrient agar (NA) plate. After 2 h, a 5 mm-diameter plug of A. arundinis was placed on the center of the PDA plate. The two plates were covered and sealed with polyethylene stretch wrap and then cultured at 28°C for 6 d. The NA plate without T9 inoculation was used as the control, and each treatment was performed in triplicate. The colony diameter of the pathogenic fungus was measured (cross-crossing method), and the rate of inhibition was calculated as follows:
Biofilm formation by the antagonists
The formation of solid surface-associated biofilm was quantified using the crystal violet staining method with minor modifications (Parafati et al., 2015; Zhang et al., 2020). A suspension of strain T9 (108 CFU/mL) was inoculated into glass tubes that contained 5 mL of LB. Then, the tubes were incubated at 28°C for 3 h, 24 h, 48 h, and 72 h in a shaker at 75 rpm. The cultures under the biofilm in the tubes were carefully removed, leaving only the biofilm, and dried at room temperature. Later, 3.0 mL of 0.1% (w/v) crystal violet was added to each tube for 30 min. The crystal violet was suctioned out, rinsed 10 times with distilled water, and decolorized with 3.0 mL of 95% ethanol. Finally, the solution was measured at 570 nm. Each treatment was performed in triplicate.
Colonization of Bacillus velezensis T9 in sugarcane wounds
Artificial wounds were made on superficially sanitized sugarcane stems using a hole punch, and 80 μL of a suspension of T9 cells (108 CFU/mL) was inoculated into the wound. The sugarcane stems were then incubated at 25°C. Later, the wounded tissue samples were collected using a sterile knife at various times (3 h and 1, 2, 3, 4, 5, 6, 7, 8, 9, and 10 d) after inoculation and weighed. The wounded tissues were then ground. Serial dilutions of each sample were prepared, and the dilutions were plated onto NA. Finally, the bacterial colonies were counted after 2 d of incubation at 28°C, and three replicates were used for each treatment. The experiment was repeated twice.
Detection of enzyme activity and the secondary metabolites of strain T9
A 1 μL suspension of strain T9 (OD600 = 1) was spotted onto agar plates that contained skim milk, carboxymethyl cellulase, and β-1,3-glucanase and cultured at 28°C for 2–4 d to determine the ability of strain T9 to produce and secrete cell wall lytic enzymes, including cellulase, glucanase, and protease (Shakeel et al., 2015). The ability of antagonists to produce various hydrolases was evaluated by observing the size of the hydrolase circle around the colony. The hydrolysis circle of cellulase was observed after staining with Congo red (Nagpure et al., 2014; Zhou et al., 2022). The ability of B. velezensis T9 to produce siderophores was determined by observing chrome azurol S (CAS) medium. A suspension of 1 μL of T9 (OD600 = 1) was inoculated onto CAS plates and cultured at 28°C. A yellow-orange zone that formed after 10 d was considered to indicate the presence of a siderophore.
Statistical analysis
All the assays were performed in triplicate, and the results are presented as the mean ± SD. The significant differences between the mean values were determined by a one-way analysis of variance (ANOVA) using Duncan’s multiple range test (p < 0.05). The statistical analyses were performed with SPSS.
Results
Isolation of the antagonistic bacteria
A total of 10 strains with antagonistic effects on A. arundinis were isolated from the soil. Among them, T9 showed an average rate of inhibition of 78.8% with a zone of inhibition of 9.1 mm in diameter (Supplementary Figure S1). The antagonistic effect of strain T9 on A. arundinis was assessed by scanning electron microscopy (SEM) (Figure 1), which showed that the mycelia were round with uniform thickness and a smooth surface in the control group. In contrast, the mycelia treated with T9 became thinner, shriveled, and folded, and the surface integrity of the mycelia was damaged. The spores were also shriveled and deformed. Transmission electron microscopy (TEM) showed that the untreated mycelia were full, and the fungal wall was complete, with a uniform thickness and dense structure. Additionally, the fungal membrane was intact, and no cytoplasmic wall separation was observed. In contrast, the treated mycelia were deformed and disintegrated. The cell wall showed a fuzzy structure with uneven thickness, and the surface mucous layer was thin and discrete outside of the cell wall. The cell membrane was blurred and thin with perforations, and there was severe separation of the cytoplasm from the cell wall. Most of the organelles were disintegrated and appeared as irregular fragments. The vacuole was contracted, and the thallus was poor with signs of breakage and disintegration.
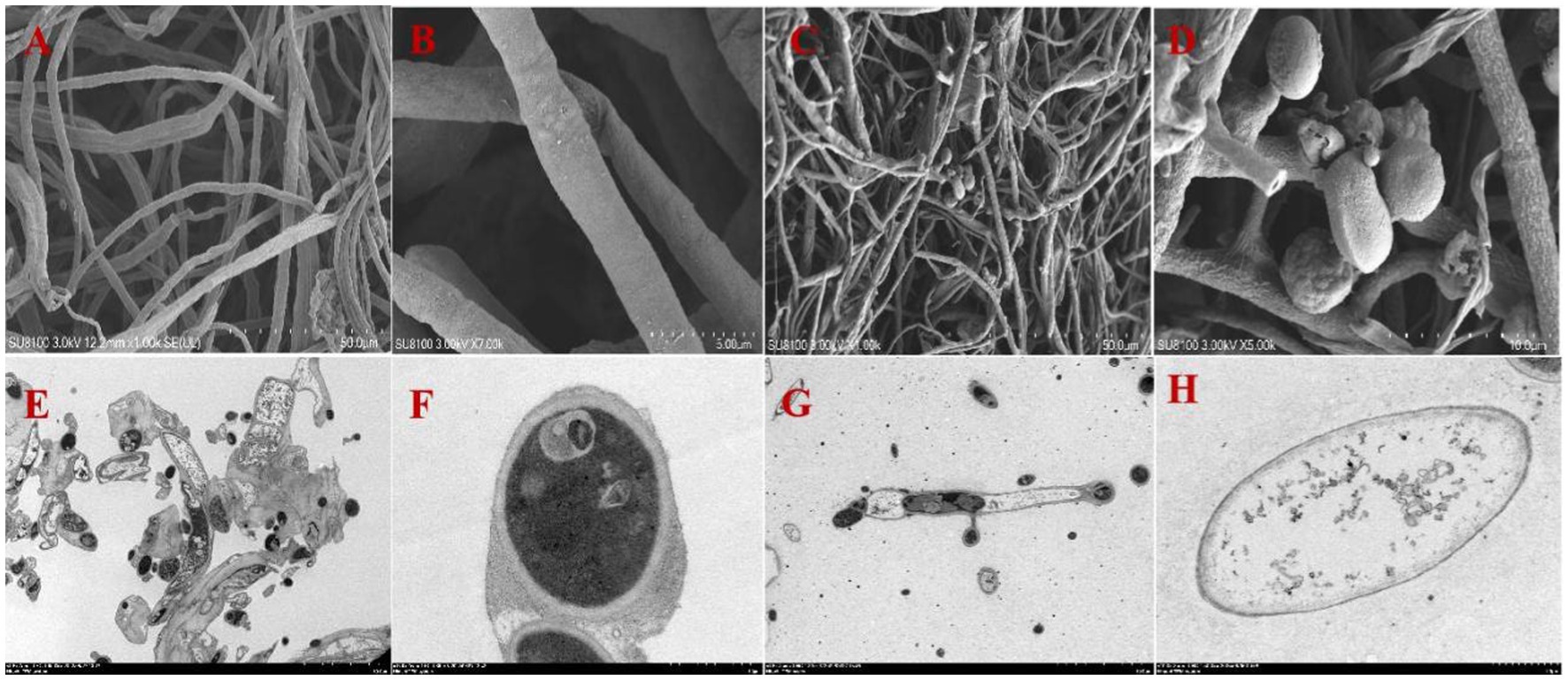
Figure 1. Mycelial morphology and ultrastructure observation of A. arundinis using SEM and TEM. SEM of the control group hyphae (A,B), and hyphae treated with strain T9 group (C,D). TEM of the control group (E,F), and treated with strain T9 group (G,H). The micrographs were taken at the magnification of (A,C) 1,000×, (B) 7,000×, (D) 5,000×, (E,G) 1,500× (F,H) 15,000×, respectively.
Identification of the antagonistic bacterium T9
The growth of strain T9 cultured in the NA medium is shown in Supplementary Figure S2. The morphological observation showed that the single colonies of T9 were milky white, dried, not smooth, and with a wrinkled surface that developed as the culture time increased. The gram stain was positive, and the bacteria were rod-shaped with slightly rounded ends. The physiological and biochemical assays showed that T9 could utilize sucrose, glucose, D-sorbitol, D-fructose, D-mannitol, proline, and alanine. Contact enzyme reaction, gelatin liquefaction, VP test, methyl red test, and starch hydrolysis were positive reactions, while citrate utilization was a negative reaction (Supplementary Table S1). These characteristics were consistent with those of the proximate Bacillus spp.
The partial 16S rDNA, gyrA, and rpoB gene sequences from strain T9 were amplified and submitted to the GenBank database with the gene entry numbers OR241131, OR326857, and OR296710, respectively, to verify the classification status of T9. A BLAST analysis of strain T9 showed that these three sequences had more than 99% homology with B. velezensis in GenBank. Phylogenetic trees based on 16S rDNA, gyrA, and rpoB gene sequences were constructed using MEGA 7.0, and the results showed that the 16S rDNA, gyrA, and rpoB sequences of strain T9 all clustered into a lineage with B. velezensis (Supplementary Figure S3). Based on the morphological, physiological, and biochemical characteristics and sequence analysis, strain T9 was identified as B. velezensis.
Effect of the T9 supernatant on the mycelial growth of Apiospora arundinis
As shown in Figure 2 and Table 2, the 3, 5, and 10% fermentation filtrate significantly inhibited the growth of A. arundinis, with rates of inhibition of 66.54, 73.78, and 82.66%, respectively, on the PDA plates that contained T9 fermentation solution. The rates of inhibition of 1, 3, 5, and 10% fermentation filtrate for the dry weight of A. arundinis were 6.64, 30.62, 76.38, and 99.63%, respectively. The fermentation filtrate of B. velezensis T9 had a significant inhibitory effect on the diameter and amount of growth of the mycelia, but the inhibitory effect of the fermentation filtrate on the amount of growth of A. arundinis was slightly better than that of the mycelial growth rate.
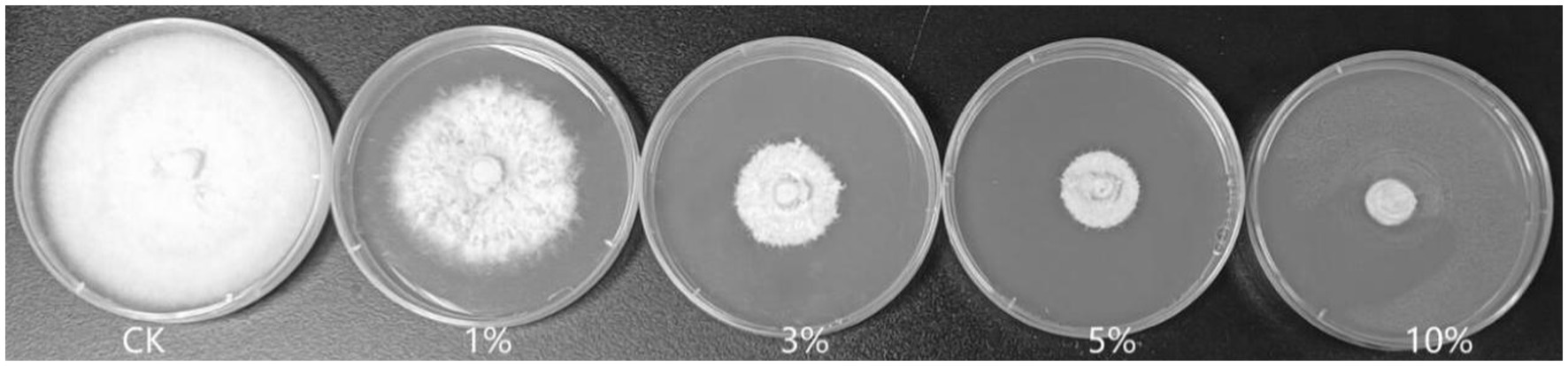
Figure 2. Inhibition test of B. velezensis T9 cell-free supernatant on A. arundinis. Strain T9 suspension was centrifuged at 3500 rpm for 20 min, the supernatant was filtered through a 0.22 μm microporous membrane to obtain the cell-free supernatant, PDA medium that contained 1, 3, 5, and 10% of the T9 cell-free supernatant were inoculated with A. arundinis plugs, with untreated PDA as the control.
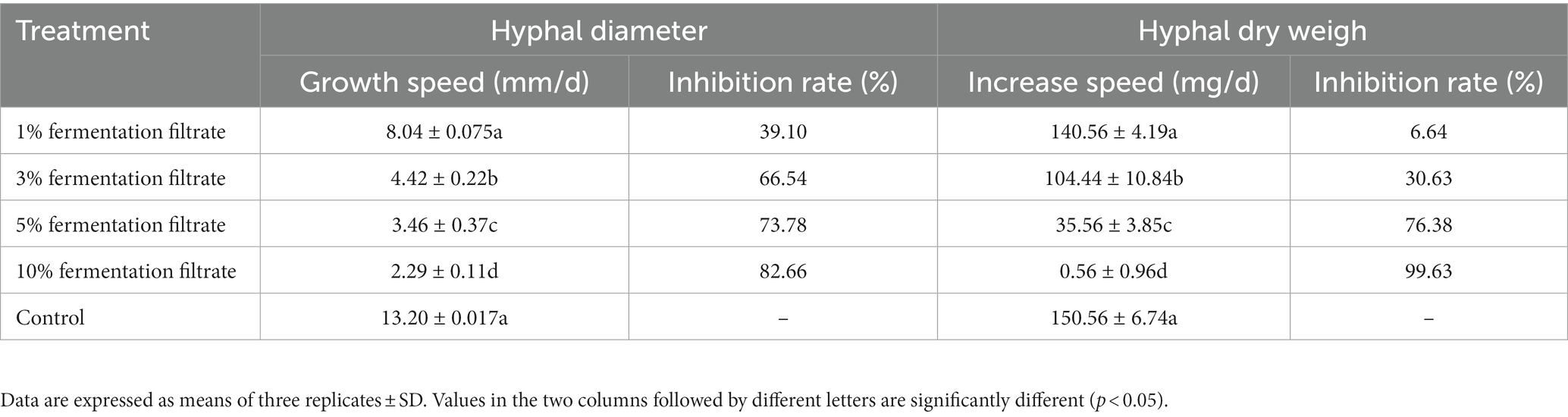
Table 2. Inhibition of cell-free fermentation of B. velenzensis T9 on hyphal growth of A. arundinis.
Effect of the T9 supernatant on the production of 3-NPA by Apiospora arundinis
Table 3 shows the effects of 1, 3, 5%, or 10% of the cell-free supernatant of strain T9 on the production of 3-NPA. With the increase in the amount of supernatant, the effect on the production of 3-NPA became more significant. Compared to the control, 1% of the strain T9 supernatant had little effect on the production of 3-NPA (9.37 μg/mL), and the rate of inhibition by 3-NPA was 21.81%. The production of 3-NPA could not be detected following treatment with 5 and 10% of the supernatant of strain T9.
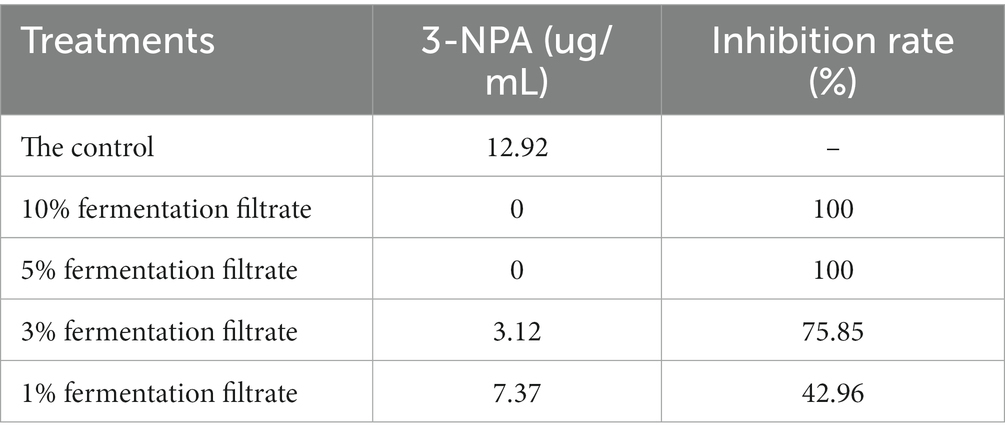
Table 3. 3-NPA production and 3-NPA inhibition ratio as affected by cell-free supernatant of B. velenzensis T9.
Inhibitory effects of the VOCs on Apiospora arundinis
The average colony diameter of A. arundinis exposed to the VOCs produced by B. velezensis T9 was 29.0 mm after 6 d, which was 70.0% smaller than that of the unexposed samples. In addition, the mycelia that were not exposed to the VOCs grew dense, while those of the exposed sample grew extremely poorly. The VOCs produced by B. velezensis T9 significantly inhibited the growth of A. arundinis mycelia in vitro.
Determination of the formation of biofilm by strain T9
The result showed that the LB without strain T9 was clear and transparent; the surface was clean, and no biofilm formed after standing for 72 h. After the incubation of the bacterial solution for 8 h, a thin layer of biofilm formed on the surface of the bacterial solution with an OD570 value of 0.53. After incubation of the bacterial solution for 24 h, a significantly thicker bacterial film was formed on the surface, with an OD570 value of 1.53. This was significantly higher than the amount that formed at 8 h. The bacterial film reached its peak at 48 h, with an OD570 value of 1.86. The thick bacterial film was still maintained at 72 h, with no significant difference from 48 h (Figure 3). Thus, B. velezensis has a strong ability to form biofilms, which could not be degraded in a short time.
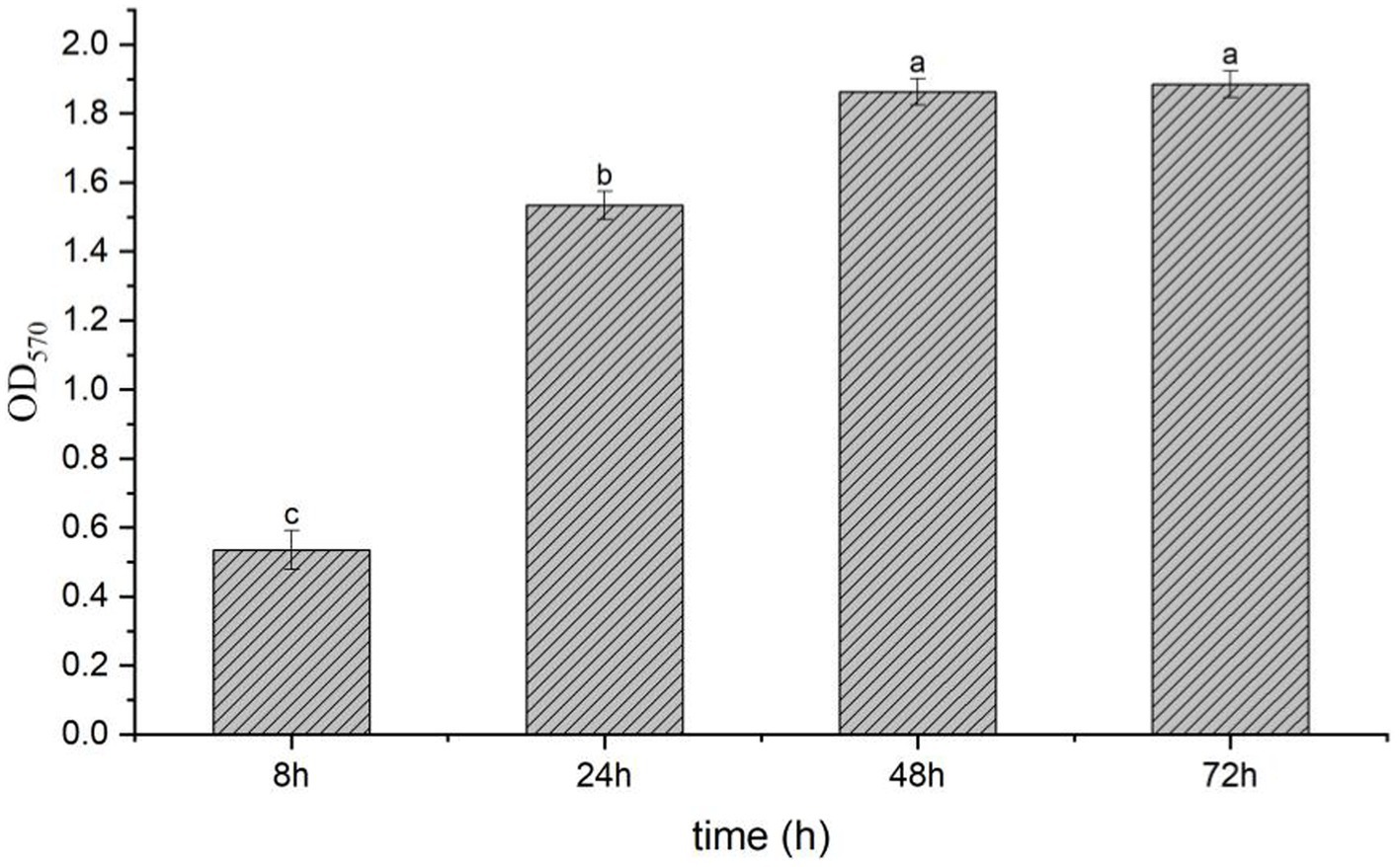
Figure 3. Biofilm formation of B. velezensis T9 using the crystal violet staining method. The absorption value of OD570 were used to represent the yield of biofilm formation by B. velezensis T9. Data were expressed as the mean of three replicates ±SD. Significant differences (p < 0.05) between means are indicated by letters above the histogram bars.
Colonization of Bacillus velezensis T9 in the sugarcane wounds
After inoculation with B. velezensis strain T9, the treated sugarcane stems were cultured in an incubator at 25°C and 90% relative humidity. A total of 3.65 × 105 CFU of strain T9 colonized the sugarcane stems after 3 h (0 d) of inoculation of the sugarcane stems, which rapidly increased to 7.75 × 105 CFU after 1 d. The population of strain T9 colonies reached a small peak with 1.63 × 108 CFU after 2 d. They declined slightly but remained stable after 3 and 4 d of treatment. The total number of colonies reached their highest peak at 4.17 × 108 CFU after 5 d. Subsequently, the number of colonies decreased, and after 10 d of treatment, the number of colonies tended to be stable and maintained 3.50 × 107 CFU, which was still higher than the initial number (Figure 4).
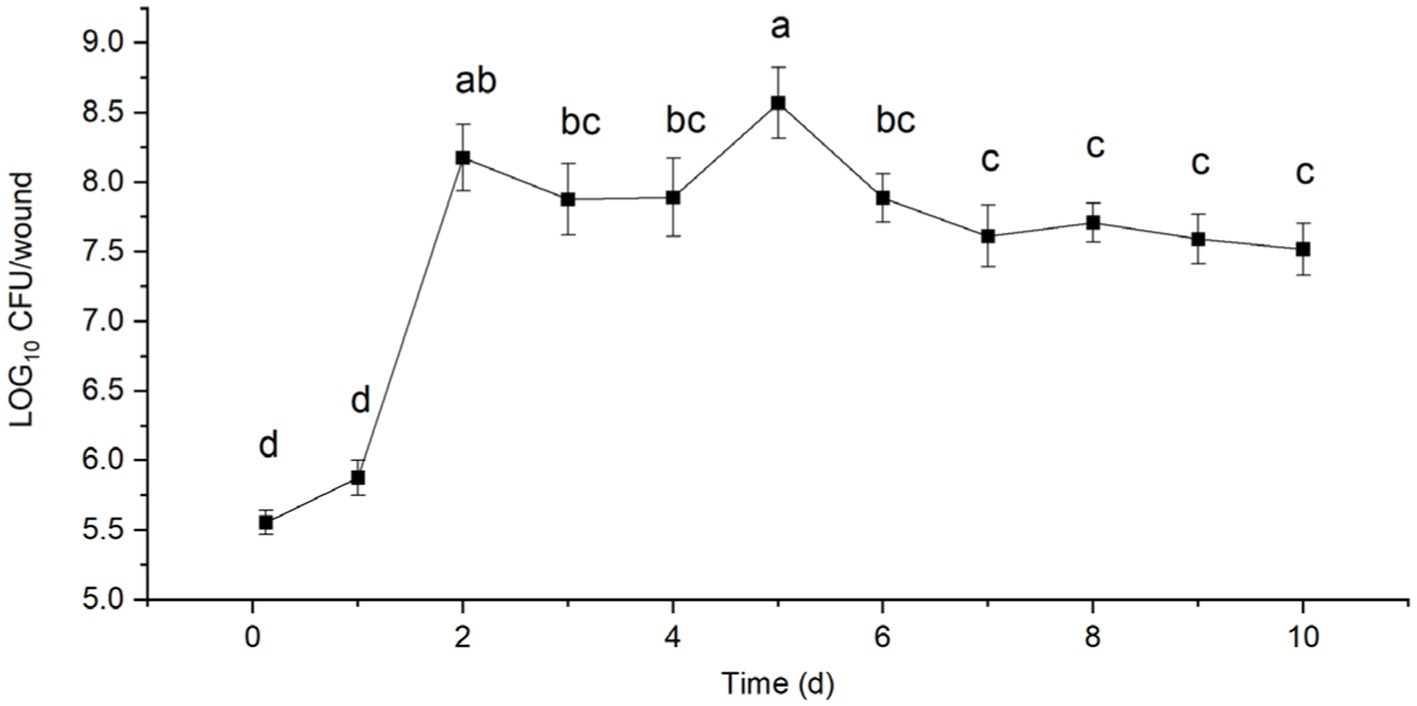
Figure 4. Population dynamics of B. velezensis strain T9 in wounds tissue of sugarcane stems at 25°C for 10 d. Data were expressed as the mean of three replicates ±SD. Significant differences (p < 0.05) between means are indicated by letters above the histogram bars.
Detection of enzyme activity and the secondary metabolites of strain T9
The ability of B. velezensis T9 to produce cell wall lytic enzymes and secondary metabolites was studied to characterize its mechanism of antagonism. This strain formed translucent hydrolysis circles in skim milk and carboxymethyl cellulose (CMC) media, indicating that B. velezensis T9 could produce protease and cellulase, respectively. Additionally, B. velezensis T9 changed the CAS medium from blue to light orange, indicating that T9 was capable of producing siderophores (Figure 5).
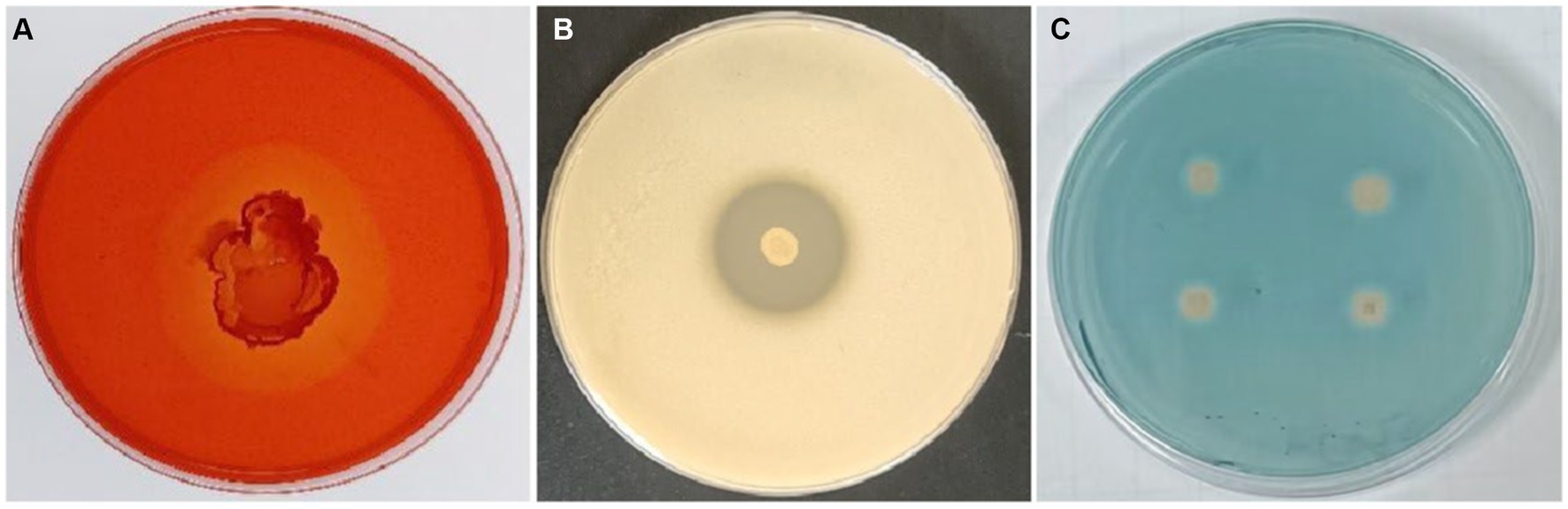
Figure 5. Detection of secretase activity and secondary metabolites in B. velezensis strain T9. (A) Cellulase detection, (B) protease detection, and (C) siderophore detection.
Effect of Bacillus velezensis strain T9 against Apiospora arundinis in sugarcane
The sugarcane stems were treated with a suspension of strain T9 cells or its cell-free supernatant and inoculated with A. arundinis to study the efficacy of strain T9 in controlling Apiospora mold. The wounds were observed on days 5 and 10 (Figure 6A). Compared with the control, the diameters of 5 days-old lesions of sugarcane treated with the T9 cell suspension or its cell-free supernatant decreased by 18.9 and 39.9%, respectively, while the 10 days-old lesion decreased by 45.4 and 51.2%, respectively. The average lesion diameter on the sugarcane stems treated with strain T9 cell suspension or its cell-free supernatant decreased significantly but had no significant difference than treatment with the fungicide prochloraz (Figure 6C). Additionally, as shown in Figure 6B, A. arundinis was not re-isolated from the sugarcane stems treated with T9, culture filtrates, and prochloraz but was re-isolated from the control group. These findings indicated that T9 treatment and its culture filtrates significantly inhibited the effects on Apiospora mold caused by A. arundinis.
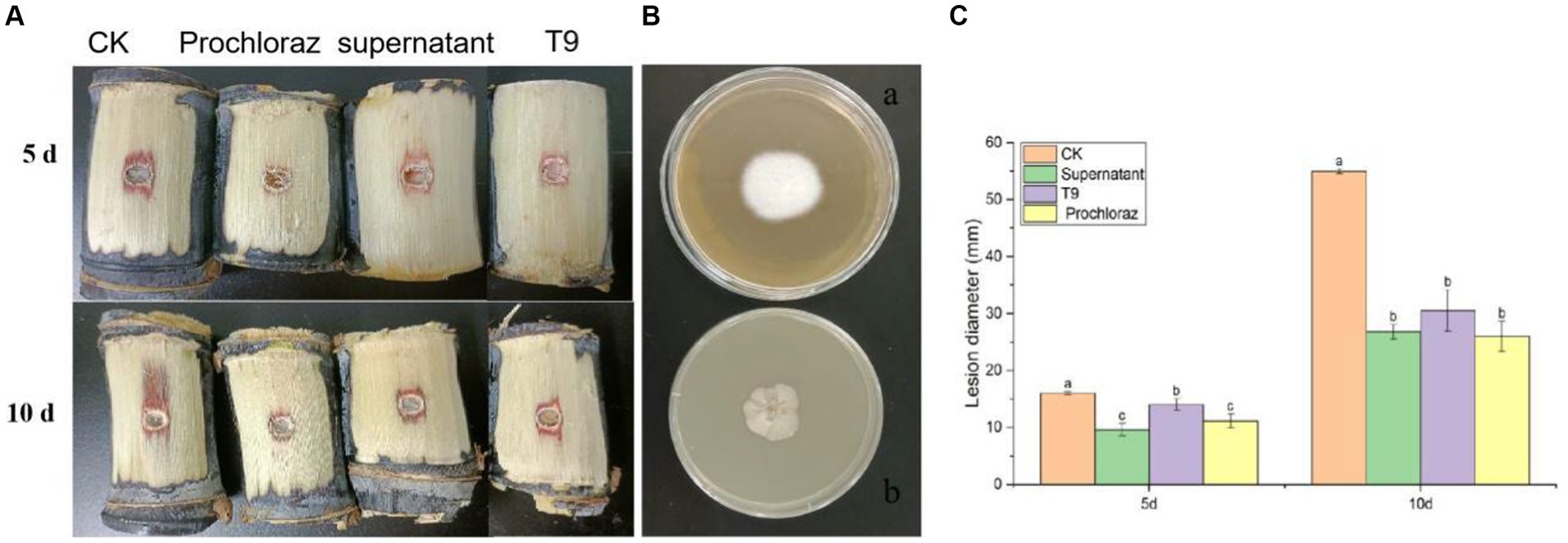
Figure 6. Effect of strain T9 cell suspension and supernatant on sugarcane Apiospora mold disease lesion diameter. (A) Strain T9 cell suspension and supernatant antagonized sugarcane Apiospora mold. Treatment with sterile distilled water or protamine was used as the negative and positive control. (B) The morphological observation of control group (A) and T9 treatment (B). (C) Statistical analysis of lesion diameter. Disease lesion length were measured at 5 and 10 day, respectively. Data were expressed as the mean of three replicates ±SD. Significant differences (p < 0.05) between means are indicated by letters above the histogram bars.
Discussion
The 3-NPA toxin produced by moldy sugarcane spoiled with Apiospora poses a potential threat to human health. Therefore, developing biological agents is critical for biological control. In recent years, biological control agents (BCAs) have attracted increasing attention due to their green and safe characteristics (Wang et al., 2016; Hu et al., 2021). Among them, Bacillus is the most widely used type of biocontrol bacteria, with strong stress resistance, good biosafety, and other outstanding advantages (Lastochkina et al., 2019; Rivas-Garcia et al., 2019; Zhou et al., 2019). However, to our knowledge, there is no report on the use of B. velezensis for the biological control of Apiospora mold on sugarcane caused by A. arundinis. In this study, B. velezensis strain T9 was isolated from the rhizosphere soil of healthy sugarcane and displayed potent biocontrol activity that enabled it to effectively control Apiospora mold caused by A. arundinis on sugarcane. Relevant studies showed that B. velezensis has been considered as a biocontrol agent to inhibite the growth of the major fungal plant pathogens. Myo et al. reported that B. velezensis NKG-2 could be used for bio-control activities against fungal diseases and potential plant growth promotion (Myo et al., 2019).
The cell suspension of strain B. velezensis T9 and its cell-free supernatant significantly inhibited the growth of A. arundinis mycelia in vitro. SEM and TEM showed that B. velezensis T9 had significant inhibitory effects on the mycelia and cells of A. arundinis, which led to disordered growth, atrophy, wrinkles, and distortion of the hyphae and changes in spore morphology, while damaging the cell membrane and inducing protoplasm leakage and eventual cell death. The diameters of the lesions on sugarcane stems treated with B. velezensis T9 cell suspensions and the supernatant of cell-free cultures were significantly lower than those of the control group, which inhibited the growth of A. arundinis and 3-NPA production. This result was consistent with those of previous research. For example, B. subtilis ABS-S14 and its cell-free supernatant had strong antagonistic effects on the hyphal growth of Penicillium digitatum, the causal agent of green mold on citrus (Waewthongrak et al., 2015). B. velezensis strain P2-1 isolated from apple (Malus domestica Borkh.) branches and its cell-free supernatant exhibited strong inhibitory effects on the growth of Botryosphaeria dothidea, which resulted in hyphal deformity (Yuan et al., 2022). Additionally, the biocontrol mechanism of B. velezensis T9 against A. arundinis was studied.
The rapid growth and high population density of antagonists in the wounded tissues could the plants or fruits from pathogens by competing with them for space and nutrients, thus, preventing the ingress of pathogenic microorganisms (Zhang et al., 2008; Liu et al., 2013; Zeriouh et al., 2014). Nunes et al. (2008) reported that the decay of oranges (Citrus sinensis [L.] Osbeck) treated with the bacterium Pantoea agglomerans before refrigeration, i.e., stored at 20°C for 24 h, was more effective with more than a 10-fold increase in P. agglomerans compared to fruits that were immediately refrigerated. In this study, the population of strain T9 colonies reached a peak after 3 d, which was greater than the population at 0 d after 10 d of treatment but retained its ability to colonize at a high density and had a good potential to act as a BCA. Further control experiments showed that the use of strain T9 significantly reduced the degree of damage by sugarcane mold compared with the control group, and its effect at controlling this disease was consistent with that of the imidamide class of chemical pesticides. Thus, this strain has strong potential for application in the field and lays a foundation for the development of BCAs.
In recent years, bacterial VOCs have been shown to play an imperative role in microbial interactions, and bacterial-plant interactions have received much attention. Many studies have reported that B. velezensis can produce VOCs that have strong inhibitory activity on the growth of bacteria and plant pathogens, and the VOCs produced by B. velezensis ZSY-1 significantly inhibited six types of plant pathogenic fungi (Gao et al., 2017). The VOCs produced by B. velezensis inhibited the growth of C. gloeosporioides and controlled anthracnose on mango (Mangifera indica Linn) (Yang et al., 2023). The VOCs produced by B. velezensis T9 could also serve as potential biocontrol agents against fungal diseases.
Biofilms are essential community structures of bacteria and play an important role in the mechanism of antagonism against plant pathogenic fungi (Bogino et al., 2013). The formation of Bacillus biofilm primarily includes adhesion development, maturity, and dispersion dissociation. In this study, T9 formed a thin layer of biofilm at 8 h. The amount of biofilm formed at 24 h increased significantly compared with that 8 h, and the amount of biofilm formed at 72 h increased slightly compared with the 48 h treatment. However, the difference was not significant. This period is the stable period of biofilm formation, which indicated that T9 is effective at forming biofilm and can promote its colonization in sugarcane.
The secretion of extracellular hydrolases is one of the most important biocontrol mechanisms of antagonistic strains of Bacillus (Xu et al., 2016; Subbanna et al., 2018). Dextran, chitin, cellulose, and protein are the primary components of fungal cell walls. It has been shown that chitinase, glucanase, cellulase, and protease play a vital role in controlling plant diseases. In this study, antagonist T9 was found to produce cellulase and protease. Therefore, it was hypothesized that this antagonist can inhibit A. arundinis by acting on the cell wall by changing or degrading it and destroying the integrity of the cell. In addition to increasing the supply of iron to plants and microorganisms, siderophores could inhibit the growth of plant pathogens (Xue et al., 2013).
Conclusion
In summary, B. velezensis strain T9 isolated from the soil of healthy sugarcane exhibited the strongest biocontrol activity against Apiospora mold caused by A. arundinis on postharvest sugarcane during storage. The results showed that the formation of biofilm, strong ability to colonize sugarcane tissue, and the production of volatile organic compounds (VOCs), cellulase, protease might be the primary mode of antagonizing Apiospora mold on postharvest sugarcane. Overall, these findings provide a novel biocontrol agent to prevent and control Apiospora mold caused by A. arundinis on postharvest sugarcane during transportation and storage. To the best of our knowledge, this is the first study that reports the strong antifungal activities of B. velezensis toward A. arundinis.
Data availability statement
The datasets presented in this study can be found in online repositories. The names of the repository/repositories and accession number(s) can be found below: NCBI-OR241131, OR326857, and OR296710.
Author contributions
JL: Writing – original draft, Writing – review & editing. XL: Writing – review & editing. HL: Writing – review & editing. LM: Writing – review & editing. RM: Writing – review & editing. WC: Writing – review & editing. YW: Writing – review & editing. TW: Writing – review & editing. WJ: Writing – review & editing.
Funding
The author(s) declare financial support was received for the research, authorship, and/or publication of this article. This work was supported by the National Natural Science Foundation of China (31860014), Special Project for Basic Scientific Research of the Guangxi Academy of Agricultural Sciences (GXAAS2021JM118, GXAAS2022JM89, GXAAS2023YM119, and GXAAS2021YT137), and Special Project of Science and Technology Vanguard of the Guangxi Academy of Agricultural Sciences (GNKM202316).
Conflict of interest
The authors declare that the research was conducted in the absence of any commercial or financial relationships that could be construed as a potential conflict of interest.
Publisher’s note
All claims expressed in this article are solely those of the authors and do not necessarily represent those of their affiliated organizations, or those of the publisher, the editors and the reviewers. Any product that may be evaluated in this article, or claim that may be made by its manufacturer, is not guaranteed or endorsed by the publisher.
Supplementary material
The Supplementary material for this article can be found online at: https://www.frontiersin.org/articles/10.3389/fmicb.2023.1314887/full#supplementary-material
SUPPLEMENTARY FIGURE S1 | Antifungal activity of strain T9 against A. arundinis. (A) Antifungal activity of strain T9 against A. arundinis. (B) untreated A. arundinis.
SUPPLEMENTARY FIGURE S2 | Colony morphological characteristics of the strain T9. (A) Strain T9 colony morphology. (B) Gram staining of thestrain T9.
SUPPLEMENTARY FIGURE S3 | Neighbor-joining phylogenetic tree of the strain T9. Neighbor-joining phylogenetic tree of the strain T9 were constructed by MEGA7.0 basing on 16 S rDNA (A), gyrA (B), and ropB (C). The numbers at the branches indicate the confifidence level calculated by bootstrap analysis (1000).
References
Birkelund, T., Johansen, R. F., Illum, D. G., Dyrskog, S. E., and Østergaard, J. A. (2021). Fatal 3-Nitropropionic acid poisoning after consuming coconut water. Emerg. Infect. Dis. 27, 278–280. doi: 10.3201/eid2701.202222
Bogino, P. C., De Las Mercedes Oliva, M., Sorroche, F. G., and Giordano, W. (2013). The role of bacterial biofilms and surface components in plant-bacterial associations. Int. J. Mol. Sci. 14, 15838–15859. doi: 10.3390/ijms140815838
Dhansu, P., Ram, B., Singh, A. K., Tomar, S. K., Karuppaiyan, R., and Kumar, R. (2023). Different treatments for sugarcane juice preservation. Foods 12:311. doi: 10.3390/foods12020311
Dong, X. Z., and Cai, M. Y. (2001). Manual for systematic identification of common bacteria. Beijing: Science Press, 364–398
Filippi, M. C. C., Da Silva, G. B., Silva-Lobo, V. L., Côrtes, M. V. C., Moraes, A. J. G., and Prabhu, A. S. (2011). Leaf blast (Magnaporthe oryzae) suppression and growth promotion by rhizobacteria on aerobic rice in Brazil. Biol. Control 58, 160–166. doi: 10.1016/j.biocontrol.2011.04.016
Gao, Z., Zhang, B., Liu, H., Han, J., and Zhang, Y. (2017). Identification of endophytic bacillus velezensis zsy-1 strain and antifungal activity of its volatile compounds against alternaria solani and botrytis cinerea. Biol. Control 105, 27–39. doi: 10.1016/j.biocontrol.2016.11.007
Grzegorczyk, M., Żarowska, B., Restuccia, C., and Cirvilleri, G. (2017). Postharvest biocontrol ability of killer yeasts against Monilinia fructigena and Monilinia fructicola on stone fruit. Food Microbiol. 61, 93–101. doi: 10.1016/j.fm.2016.09.005
Hajnal, E. J., Kos, J., Malachová, A., Steiner, D., Stranska, M., and Krska, R. (2020). Mycotoxins in maize harvested in Serbia in the period 2012–2015. Part 2: non-regulated mycotoxins and other fungal metabolites. Food Chem. 317:126409. doi: 10.1016/j.foodchem.2020.126409
He, F., Zhang, S., Qian, F., and Zhang, C. (1995). Delayed dystonia with striatal CT lucencies induced by a mycotoxin (3-nitropropionic acid). Neurology 45, 2178–2183. doi: 10.1212/WNL.45.12.2178
Holt, J. G., Krieg, N. R., Sneath, P. H. A., Staley, J. T., and Williams, S. T. (1994). Bergey’s manual of determinative bacteriology. Baltimore, MD: Williams and Wilkins Press, 195–256
Hou, Z. Z., Liu, G. H., Song, L. J., Ren, X. R., and Yan, Y. X. (1989). Isolation and toxicity of fungi from moldy sugarcane. Chin. J. Public Health 6, 341–342.
Hu, Y., Li, Y., Yang, X., Li, C., Wang, L., and Feng, J. (2021). Effects of integrated biocontrol on bacterial wilt and rhizosphere bacterial Community of Tobacco. Sci Rep-Uk. 11:2653. doi: 10.1038/s41598-021-82060-3
Hu, W. J., Liang, X. T., Chan, H. M., Wang, Y. H., Liu, X. J., and Luo, X. Y. (1986). Isolation and identification of toxic substance-3-nitropropionic acid from degenerated sugarcane arthrobotrys sp. Chin. J. Prevent. Med. 6, 321–323.
Huang, Y., Sun, C., Guan, X., Lian, S., Li, B., and Wang, C. (2021). Biocontrol efficiency of Meyerozyma guilliermondii Y-1 against apple postharvest decay caused by Botryosphaeria dothidea and the possible mechanisms of action. Int. J. Food Microbiol. 338:108957. doi: 10.1016/j.ijfoodmicro.2020.108957
Kang, X., Wang, L. H., Guo, Y., Arifeen, M. Z. U., and Liu, C. (2019). A comparative transcriptomic and proteomic analysis of Hexaploid Wheat's responses to colonization by bacillus velezensis and Gaeumannomyces graminis, both separately and combined. Mol Plant Microbe 32, 1336–1347. doi: 10.1094/MPMI-03-19-0066-R
Kang, X., Zhang, W., Cai, X., Zhu, T., Xue, Y., and Liu, C. (2018). Bacillus velezensis CC09: a potential vaccine for controlling wheat diseases. Mol. Plant-Microbe Interact 31, 623–632. doi: 10.1094/MPMI-09-17-0227-R
Khalifa, M. W., Rouag, N., and Bouhadida, M. (2022). Evaluation of the antagonistic effect of pseudomonas Rhizobacteria on Fusarium wilt of chickpea. Agriculture 12:429. doi: 10.3390/agriculture12030429
Kumar, S., Stecher, G., and Tamura, K. (2016). MEGA7: molecular evolutionary genetics analysis version 7.0 for bigger datasets. Mol. Biol. Evol. 33, 1870–1874. doi: 10.1093/molbev/msw054
Lastochkina, O., Seififikalhor, M., Aliniaeifard, S., Baymiev, A., and Pusenkova, L. (2019). Bacillus spp.: efficient biotic strategy to control postharvest diseases of fruits and vegetables. Plan. Theory 8:97. doi: 10.3390/plants8040097
Leelasuphakul, W., Hemmanee, P., and Chuenchitt, S. (2008). Growth inhibitory properties of Bacillus subtilis strains and their metabolites against the green mold pathogen (Penicillium digitatum Sacc.) of citrus fruit. Postharvest Biol. Technol. 48, 113–121. doi: 10.1016/j.postharvbio.2007.09.024
Liao, J., Jiang, W. Y., Wu, X. J., He, J., Li, H. L., and Wang, T. S. (2022). First report of apiospora mold on sugarcane in China caused by Apiospora arundinis (Arthrinium arundinis). Plant Dis. 106:1058. doi: 10.1094/PDIS-02-21-0386-PDN
Liu, R., Li, J., Zhang, F., Zheng, D., Chang, Y., Xu, L., et al. (2021). Biocontrol activity of Bacillus velezensis D4 against apple Valsa canker. Biol. Control 163:104760. doi: 10.1016/j.biocontrol.2021.104760
Liu, X. J., and Luo, X. Y. (1984). Etiological study on poisoning of spoiled sugarcane I – analysis of epidemiological and clinical data and toxicity test of poisoned samples. Health research. 13, 24–28.
Liu, X. J., Luo, X. Y., Li, X. F., and Chen, Q. T. (1988). Classification and identification of the pathogen of spoilage sugarcane poisoning. J. Fungi 7, 221–225.
Liu, X. J., Luo, X. Y., Liu, X. M., Li, X. F., and Li, Y. W. (1984). Etiological study on poisoning of spoiled sugarcane II – isolation and toxicity test of pathogenic fungi. Health Res. 13, 28–33.
Liu, J., Sui, Y., Wisniewski, M., Droby, S., and Liu, Y. (2013). Review: utilization of antagonistic yeasts to manage postharvest fungal diseases of fruit. Int. J. Food Microbiol. 167, 153–160. doi: 10.1016/j.ijfoodmicro.2013.09.004
Liu, X. J., Sun, Y. Y., Li, X. F., Liu, J., and Wang, Y. H. (1993). Studies on the prevention of poisoning of spoiled sugarcane II. Studies on the ecology of the pathogen of spoiled sugarcane poisoning. Hygiene Res. 22, 96–98.
Myo, E. M., Liu, B., Ma, J., Shi, L., Jiang, M., and Zhang, K. (2019). Evaluation of Bacillus velezensis NKG-2 for bio-control activities against fungal diseases and potential plant growth promotion. Biol. Control 134, 23–31. doi: 10.1016/j.biocontrol.2019.03.017
Nagpure, A., Choudhary, B., and Gupta, R. K. (2014). Mycolytic enzymes produced by Streptomyces violaceusniger and their role in antagonism towards wood-rotting fungi. J. Basic Microbiol. 54, 397–407. doi: 10.1002/jobm.201200474
Niem, J. M., Billones-Baaijens, R., Stodart, B., and Savocchia, S. (2020). Diversity profiling of grapevine microbial Endosphere and antagonistic potential of Endophytic pseudomonas against grapevine trunk diseases. Front. Microbiol. 11:477. doi: 10.3389/fmicb.2020.00477
Nony, P. A., Scalle, A. C. T., Rountree, R. L., Ye, X., and Binienda, Z. (1999). 3-Nitropropionic acid (3-NPA) produces hypothermia and inhibits histochemical Labeling of succinate dehydrogenase (SDH) in rat brain. Metab. Brain Dis. 14, 83–94. doi: 10.1023/a:1020753629477
Nunes, C., Bajji, M., Stepien, V., Manso, T., and Torres, R. (2008). Development and application of a SCAR marker to monitor and quantify populations of the postharvest biocontrol agent Pantoea agglomerans CPA-2. Postharvest Biol. Technol. 47, 422–428. doi: 10.1016/j.postharvbio.2007.07.016
Parafati, L., Vitale, A., Restuccia, C., and Cirvilleri, G. (2015). Biocontrol ability and action mechanism of food-isolated yeast strains against Botrytis cinerea causing post-harvest bunch rot of table grape. Food Microol. 47, 85–92. doi: 10.1016/j.fm.2014.11.013
Rivas-Garcia, T., Murillo-Amador, B., Nieto-Garibay, A., and Rincon-Enriquez, G. (2019). Enhanced biocontrol of fruit rot on muskmelon by combination treatment with marine Debaryomyces hansenii and Stenotrophomonas rhizophila and their potential modes of action. Postharvest Biol. Technol. 151, 61–67. doi: 10.1016/j.postharvbio.2019.01.013
Shakeel, M., Rais, A., Hassan, M. N., and Hafeez, F. Y. (2015). Root associated bacillus sp improves growth, yield and zinc translocation for basmati rice (Oryza sativa) varieties. Front. Microbiol. 6:1286. doi: 10.3389/fmicb.2015.01286
Souza, S. O., Costa, S. S. L., Brum, B. C. T., Santos, S. H., Garcia, C. A. B., and Araujo, R. G. O. (2019). Determination of nutrients in sugarcane juice using slurry sampling and detection by ICP OES. Food Chem. 273, 57–63. doi: 10.1016/j.foodchem.2018.03.060
Spadaro, D., and Droby, S. (2016). Development of biocontrol products for postharvest diseases of fruit: the importance of elucidating the mechanisms of action of yeast antagonists. Trends Food Sci Tech. 47, 39–49. doi: 10.1016/j.tifs.2015.11.003
Subbanna, A. R. N. S., Rajasekhara, H., Stanley, J., Mishra, K. K., and Pattanayak, A. (2018). Pesticidal prospectives of chitinolytic bacteria in agricultural pest management. Soil Biol. Biochem. 116, 52–66. doi: 10.1016/j.soilbio.2017.09.019
Vos, P., Garrity, G., Jones, D., Krieg, N. R., Ludwig, W., Rainey, F. A., et al. (2011). Bergey’s manual of systematic bacteriology, 3: The Firmicutes. Cham: Springer Science & Business Media
Waewthongrak, W., Pisuchpen, S., and Leelasuphakul, W. (2015). Effect of Bacillus subtilis and chitosan applications on green mold (Penicilium digitatum Sacc.) decay in citrus fruit. Postharvest Biol. Technol. 99, 44–49. doi: 10.1016/j.postharvbio.2014.07.016
Wang, K. L., Deng, Q. Q., Chen, J. W., and Shen, W. K. (2020). Physiological and molecular mechanisms governing the effect of virus-free chewing cane seedlings on yield and quality. Sci. Rep. 10:10306. doi: 10.1038/s41598-020-67344-4
Wang, N. N., Yan, X., Gao, X. N., Niu, H. J., Kang, Z. S., and Huang, L. L. (2016). Purification and characterization of a potential antifungal protein from Bacillus subtilis E1R-J against Valsa Mali. World J Microb Biot. 32, 63–10. doi: 10.1007/s11274-016-2024-5
Wei, D. L., Chang, S. C., Lin, S. C., Doong, M. L., and Jong, S. C. (1994). Production of 3-nitropropionic acid by Arthrinium species. Curr. Microbiol. 28, 1–5. doi: 10.1007/bf01575978
Xu, T., Zhu, T., and Li, S. (2016). β-1, 3-1, 4-glucanase gene from Bacillus velezensis ZJ20 exerts antifungal effect on plant pathogenic fungi. World J. Microb. Biot. 32, 26–3993. doi: 10.1007/s11274-015-1985-0
Xue, L., Xue, Q., Chen, Q., Lin, C., Shen, G., and Zhao, J. (2013). Isolation and evaluation of rhizosphere actinomycetes with potential application for biocontrol of Verticillium wilt of cotton. Crop Prot. 43, 231–240. doi: 10.1016/j.cropro.2012.10.002
Yang, Z., Mm, L., Sp, H., Tang, L., Xp, C., and Tx, G. (2023). Inhibitory effect of volatile bacillus on mango anthrax and its preventive effect on mango anthracnose. Acta Phytopathol. Sin. 1312, 1–17. doi: 10.13926/j.cnki.apps.001312
Yu, X., Ai, C., Xin, L., and Zhou, G. (2011). The siderophore-producing bacterium,Bacillus subtilis CAS15, has a biocontrol effect on Fusarium wilt and promotesthe growth of pepper. Eur. J. Soil Biol. 47, 138–145. doi: 10.1016/j.ejsobi.2010.11.001
Yuan, H., Shi, B., Wang, L., Huang, T., Zhou, Z., and Hou, H. (2022). Isolation and characterization of Bacillus velezensis strain P2-1 for biocontrol of apple postharvest decay caused by Botryosphaeria dothidea. Front. Microbiol. 12:808938. doi: 10.3389/fmicb.2021.808938
Zeriouh, H., de Vicente, A., Pérez-García, A., and Romero, D. (2014). Surfactin triggers biofilm formation of Bacillus subtilis in melon phylloplane and contributes to the biocontrol activity. Environ. Microbiol. 16, 2196–2211. doi: 10.1111/1462-2920.12271
Zhang, H., Ma, L., Wang, L., Jiang, S., Dong, Y., and Zheng, X. (2008). Biocontrol of gray mold decay in peach fruit by integration of antagonistic yeast with salicylic acid and their effects on postharvest quality parameters. Biol. Control 47, 60–65. doi: 10.1016/j.biocontrol.2008.06.012
Zhang, J., Meng, L., Zhang, Y., Sang, L., and Wang, G. (2020). Gapb is involved in biofilm formation dependent on lrgab but not the sini/r system in bacillus cereus 0-9. Front. Microbiol. 11:591926. doi: 10.3389/fmicb.2020.591926
Zhang, S., Zheng, Q., Xu, B., and Liu, J. (2019). Identification of the fungal pathogens of postharvest disease on peach fruits and the control mechanisms of Bacillus subtilis JK-14. Toxins 11:322. doi: 10.3390/toxins11060322
Zhou, M., Li, P., Wu, S., Zhao, P., and Gao, H. (2019). Bacillus subtilis cf-3 volatile organic compounds inhibit monilinia fructicola growth in peach fruit. Front. Microbiol. 10:1804. doi: 10.3389/fmicb.2019.01804
Keywords: Apiospora arundinis, Bacillus velezensis, sugarcane, 3-nitropropionic acid, biological control
Citation: Liao J, Liang X, Li H, Mo L, Mo R, Chen W, Wei Y, Wang T and Jiang W (2023) Biocontrol ability of Bacillus velezensis T9 against Apiospora arundinis causing Apiospora mold on sugarcane. Front. Microbiol. 14:1314887. doi: 10.3389/fmicb.2023.1314887
Edited by:
Moshe Shemesh, Agricultural Research Organization (ARO), IsraelReviewed by:
Tanja Berić, University of Belgrade, SerbiaMonika Singh, Uttaranchal University, India
Copyright © 2023 Liao, Liang, Li, Mo, Mo, Chen, Wei, Wang and Jiang. This is an open-access article distributed under the terms of the Creative Commons Attribution License (CC BY). The use, distribution or reproduction in other forums is permitted, provided the original author(s) and the copyright owner(s) are credited and that the original publication in this journal is cited, in accordance with accepted academic practice. No use, distribution or reproduction is permitted which does not comply with these terms.
*Correspondence: Tianshun Wang, wangts2325@163.com; Wenyan Jiang, duanmuzheng2010@163.com