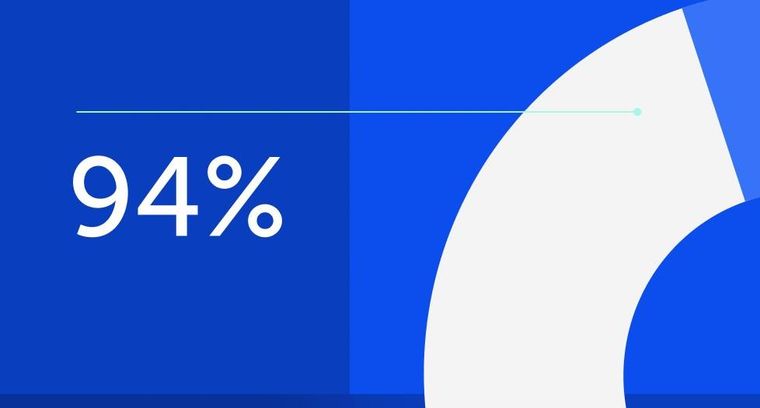
94% of researchers rate our articles as excellent or good
Learn more about the work of our research integrity team to safeguard the quality of each article we publish.
Find out more
ORIGINAL RESEARCH article
Front. Microbiol., 15 January 2024
Sec. Systems Microbiology
Volume 14 - 2023 | https://doi.org/10.3389/fmicb.2023.1306048
This article is part of the Research TopicAnimal Models, Gut Microbiota and Brain DiseasesView all 23 articles
Background: Numerous pertinent investigations have demonstrated a correlation between gut microflora (GM) and the occurrence of dementia. However, a causal connection between GM and dementia and its subtypes has not yet been clarified.
Objective: To explore the causal association between GM and dementia, including its subtypes, a two-sample Mendelian randomization (TSMR) analysis was used.
Methods: Our data comes from the Genome-Wide Association Study (GWAS). The principal approach employed for the Mendelian randomization study was the inverse-variance weighted method, supplemented by four methods: MR-Egger, weighted median, simple mode, and weighted mode. This was followed by Cochrane’s Q test, MR-Egger intercept test, MR-PRESSO global test, and leave-one-out as sensitivity analysis validation.
Results: Twenty-one GMs associated with any dementia, Alzheimer’s disease, vascular dementia, Lewy body dementia, Parkinson’s disease, and dementia under other disease classifications were derived from the analysis, and 21 passed sensitivity tests.
Conclusion: We confirmed the causal relationship between GM and dementia and its subtypes, derived specific flora associated with increased or decreased risk of dementia, and provided new ideas for preventive, diagnostic, and therapeutic interventions for dementia mediated by gut microbiota.
Dementia is a prevalent neurodegenerative disorder clinically distinguished by cognitive impairment and a gradual deterioration in one’s ability to function autonomously (Liu et al., 2019). According to a WHO report (Cheah et al., 2022), dementia has now become the seventh leading cause of death globally, and it is expected that the number of dementia patients worldwide will reach 139 million by 2050. At the same time, the prevention and treatment of dementia bring a substantial economic and healthcare burden to society and countries, and the global investment in dementia will reach 2.8 trillion dollars by 2030 (World Alzheimer Report, 2015). Alzheimer’s disease represents the prevailing form of dementia, comprising approximately 50 to 70% of cases. Other frequently encountered kinds comprise vascular dementia, Lewy body dementia, Parkinson’s disease, and dementia in other diseases as classified elsewhere (Aarsland, 2020; Wilbur, 2023). To date, the underlying mechanisms leading to dementia have not been clarified, and the medical requirements of individuals with dementia are not well met (Zagórska et al., 2023). Therefore, clarification of dementia-related risk factors, and thus dementia prevention, intervention, and care, can significantly help enhance the well-being and survival rates of individuals with dementia (Livingston et al., 2020).
Gut microbiota (GM) generally refers to bacteria in the human gut. It is involved in regulating a wide range of physiological functions in the host organism and protecting the host from pathogenic bacteria (Álvarez et al., 2021; Kuziel and Rakoff-Nahoum, 2022). Increasingly, GM has been found to fulfill an essential role in the nervous system through the brain-gut axis and has even been implicated in neurodegenerative diseases (Cryan et al., 2020; Mitrea et al., 2022). Studies have demonstrated that GM metabolites, molecules, and endotoxins may affect the central nervous system through the bloodstream or the vagus nerve, affecting brain function and cognitive behavior (Chen et al., 2021). This is undoubtedly a complementary approach to diagnosing and treating dementia, and many scholars have endeavored to address neurodegenerative illnesses through the manipulation of gut microbiota (Möhle et al., 2016; Sasmita, 2019). A systematic evaluation based on dementia studies showed that probiotic supplements improved memory in patients with dementia, as well as elevated levels of brain-derived neurotrophic factor (Ruiz-Gonzalez et al., 2021). In addition, some studies have found differences in GM composition between healthy people and people with cognitive impairment or different types of cognitive impairment, attempting to use this information to make a diagnosis of the disease (Guo et al., 2021; Hung et al., 2022). Therefore, clarifying the influence of different flora on dementia is essential for ascertaining new therapeutic targets for dementia and diagnosing dementia using microbial profiles (Liu et al., 2019; Guo et al., 2021; Cuartero et al., 2023).
Mendelian randomization (MR) is a study method that explores causal relationships between exposure factors and outcomes using single nucleotide polymorphisms (SNPs) as instrumental variables (IVs; Burgess et al., 2017), which is consistent with the principle of random allocation of genetic variation during meiosis, avoiding the influence of confounding variables and the potential for reverse causality (Sekula et al., 2016). This work employed GWAS summary statistics of GW taxa associated with dementia and their subtypes for MR analysis in order to evaluate the risk relationship between genetically determined GW taxa and dementia and its subtypes, which provides evidence for existing findings, new research ideas for pathogenesis that has not yet been clarified, and new directions for the early diagnosis, avoidance, and therapy of all types of dementia.
TSMR was employed to analyze the association between GM and dementia (any dementia, Alzheimer’s disease, vascular dementia, Lewy body dementia, Parkinson’s disease, dementia in other diseases classified elsewhere) with regard to causality. The overall design of the study is illustrated in Figure 1. In order to carry out a TSMR study, it is imperative that three fundamental assumptions are satisfied: (1) Strong correlation between IVs and exposure; (2) No correlation between IVs and confounders; (3) IVs can only affect outcomes through exposure (EPIC-InterAct Consortium et al., 2015). IVs that fulfill these three assumptions were included in this MR study (Figure 2). This study followed the most updated guidelines (STROBE-MR; Skrivankova et al., 2021).
The GWAS data for gut microbes were acquired through the MiBioGen consortium1 from genomic statistical research by Kurilshikov et al. of 18,340 individuals of European ethnicity from 11 countries (24 cohorts), and the data contained 211 gut microbes with 122,110 variant loci (Kurilshikov et al., 2021). From this GWAS, we screened IVs of gut bacterial taxa in five ranks.
GWAS statistics for any dementia, Alzheimer’s disease, vascular dementia, Parkinson’s disease, and dementia in other diseases classified elsewhere were derived from the FinnGen study program.2 GWAS statistics for Lewy body dementia were derived from the GWAS Catalog.3 The diagnostic standards for dementia are according to F03 in the ICD-10 criteria, where the GWAS dataset has 16,380,466 variant loci from 5,933 patients and 212,859 controls. The diagnostic standards for Alzheimer’s disease are according to G30.901 of the ICD-10 criteria, which contains 16,380,451 variant loci from 2,191 cases and 209,487 controls. The diagnostic standards for Lewy body dementia are according to G31.805 of the ICD-10 criteria, which contains 7,593,175 variant loci from 2,591 cases and 4,027 controls. The diagnostic standards for vascular dementia are according to F01 of the ICD-10 criteria and contain 16,380,453 variant loci from 98 cases and 211,300 controls. The diagnostic standards for Parkinson’s disease are according to the G20 of the ICD-10 criteria and contain 16,380,459 variant loci from 267 cases and 216,628 controls. The diagnostic standards for dementia in other diseases classified elsewhere are according to F02.8 of the ICD-10 criteria and contain 16,380,450 variant loci from 581 cases and 209,487 controls. In addition, three datasets related to any dementia were added as three validation groups (Table 1).
We screened the relevant IVs according to the following standards: (1) a significant threshold (p < 5 × 10−8) for IVs was associated with exposure and outcome, but the quantity of eligible IVs (exposure) was low, so a more appropriate threshold (p < 1 × 10−5) was used to acquire a larger quantity of IVs (Lv et al., 2021; Zeng et al., 2023); (2) the chain imbalance coefficient r2 < 0.001, distance = 10,000 kb was set to remove the presence of chain imbalance among IVs; (3) to avoid the effects of horizontal pleiotropy, IVs linked to dangerous elements of dementia were eliminated by the utilization of PhenoScanner (Kamat et al., 2019); (4) palindromic SNPs were removed from IVs; and (5) to avoid bias from weak instrumental variables, we removed IVs with F < 10 (Burgess et al., 2011).
In TSMR analysis of gut microbes and dementia (any dementia, Alzheimer’s disease, vascular dementia, Lewy body dementia, Parkinson’s disease, dementia in other diseases classified elsewhere) in causality, the fixed-effects IVW method and the random-effects IVW method were the main methods (Burgess et al., 2013). The choice between the two is determined by the heterogeneity between IVs, if there is heterogeneity in Cochrane’s Q test (p < 0.05), then random-effects IVW method was used, otherwise fixed-effects IVW method or random-effects IVW method was used. Therefore, in this TSMR analysis, We chose the random-effects IVW method as the main method (Greco et al., 2015). In addition, MR-Egger, weighted median, simple mode, and weighted mode can complement IVW (Bowden et al., 2015, 2016), and ORs and 95% confidence intervals were also obtained. A causal relationship between gut microbes and dementia was considered likely if the outcome of one TSMR method was remarkable (p < 0.05; Jin et al., 2023), and the causal relationship was considered reliable if the results of two or more TSMR methods were significant (Ni et al., 2021).
Sensitivity analyses took place to verify the robustness of the findings, and Cochrane’s Q test was used to test for heterogeneity. The IV was considered heterogeneous if p < 0.05. The MR-Egger method’s intercept term indicates horizontal multiplicity in the IVs, and if this intercept term is significantly different from 0, it indicates the presence of horizontal multiplicity (Burgess and Thompson, 2017). MR-PRESSO is also commonly used to test for horizontal multiplicity (Verbanck et al., 2018). Finally, the validation of the data was conducted using the leave-one-out procedure (Xiang et al., 2021). The investigation was carried out utilizing R program (version 4.3.0). The “Two SampleMR” R package4 and “MRPRESSO” R package5 were used for our MR study.
Assuming that there are relevant GMs that can have an effect on dementia and its subtypes in the final findings, we will further conduct a reverse MR analysis to explore the effect of dementia on GMs, with dementia as the exposure and GMs as the outcome to avoid reverse causality interfering with the results of this study.
After screening the above entries, 605 IVs associated with dementia were finally obtained, involving 60 GMs. Detailed information can be found in Supplementary Table 1. All IVs involved had F values greater than 10 (range 16.91–85.37), so there were no weak instrumental variables. These IVs were categorized into five classes: phylum, class, order, family, and genus, comprising two phylum (14 IVs), four classes (40 IVs), nine orders (90 IVs), 13 families (134 IVs), and 32 genera (327 IVs). Because of the inclusionary relationship between gut microbial classifications, there may be a substantial overlap of SNPs and their associated orders contained in various types of enterobacteria.
Causal relationships between the 60 GMs screened and dementia were analyzed using five TSMR methods: IVW, MR-Egger, weighted median, simple mode, and weighted mode (Supplementary Table 2). Potential causal relationships between the 60 GMs and dementia were determined using two TSMR methods, in which six GMs associated with dementia, four GMs associated with Alzheimer’s disease, two GMs associated with vascular dementia, three GMs associated with Lewy body dementia, two GMs associated with Parkinson’s disease, and four GMs associated with other diseases under the classification of dementia-associated GMs, and cross-validation was performed (Table 2; Figure 3). Our attention was directed toward the 21 causal associations that have a relatively steady nature.
Causal relationships were obtained for six related GMs in any dementia using the IVW method, and all six relationships were more stable under IVW and WM cross-validation. Among them, family Desulfovibrionaceae (OR: 1.481, 95% confidence interval (CI): 1.064–2.062, p = 0.020), family Lactobacillaceae (OR: 1.216. CI: 1.040–1.422, p = 0.014), genus Ruminococcusgnavus group (OR: 1.196, CI: 1.033–1.385, p = 0.016), genus Lactobacillus (OR: 1.304, CI: 1.115–1.525, p = 0.001), and order Desulfovibrionales (OR: 1.408, CI: 1.057–1.875, p = 0.019) were related to increased risk of dementia. Genus Defluviitaleaceae UCG011 (OR: 0.735, CI: 0.553–0.977, p = 0.034) was related to a reduced risk of dementia.
Causal relationships were obtained for nine related GMs in Alzheimer’s disease using the IVW method, and four relationships were more stable under IVW and WM cross-validation. Among them, family Desulfovibrionaceae (OR: 1.682, CI: 1.102–2.568, p = 0.016), genus Sellimonas (OR: 1.273, CI: 1.068–1.518, p = 0.007), and order Desulfovibrionales (OR: 1.592, CI: 1.011–2.507, p = 0.045) were associated with increased risk of Alzheimer’s disease. Order Bacillales (OR: 0.738, CI: 0.608–0.896, p = 0.002) was related to a decreased risk of Alzheimer’s disease.
Causal relationships were obtained for 14 relevant GMs in vascular dementia using the IVW method, and two relationships were more stable under IVW and WM cross-validation. Among them, genus Lachnospiraceae NK4A136 group (OR: 0.197, CI: 0.046–0.851, p = 0.030) and order Victivallales (OR: 0.350, CI: 0.125–0.980, p = 0.030) were related to a reduced risk of vascular dementia.
Causal relationships were obtained for nine relevant GMs in Lewy body dementia using the IVW method, and three relationships were more stable under IVW and WM cross-validation. Among them, class Alphaproteobacteria (OR: 1.970, CI: 1.320–2.940, p = 0.001) and order Bacillales (OR: 1.378, CI: 1.116–1.703, p = 0.030) were associated with increased risk of Lewy body dementia. Genus Ruminococcusgnavus group (OR: 0.678, CI: 0.523–0.878, p = 0.003) was related to a reduced risk of Lewy body dementia.
Causal relationships were obtained for 10 related GMs in Parkinson’s disease using the IVW method, and two relationships were more stable under IVW and WM cross-validation. Among them, genus Butyricimonas (OR: 0.314, CI: 0.134–0.737, p = 0.008) and phylum Lentisphaerae (OR: 0.500, CI: 0.255–0.980, p = 0.044) were related to a reduced risk of Parkinson’s disease.
Causal relationships were obtained for 12 relevant GMs in dementia in other diseases classified elsewhere using the IVW method, and four relationships were more stable under IVW and WM cross-validation. Among them, genus Ruminococcusgnavus group (OR: 1.707, CI: 1.125–2.591, p = 0.012) and genus Hungatella (OR: 1.697, CI: 1.026–2.809, p = 0.040) were associated with an increased risk of dementia in other diseases classified elsewhere. Order Burkholderiales (OR: 0.500, CI: 0.250–0.998, p = 0.049) and genus Oscillibacter (OR: 0.538, CI: 0.344–0.841, p = 0.007) were related to decreased risk of dementia in other diseases classified elsewhere.
Finally, we utilized a heat map to causally present the results of the study in the form of various types of GMs and any dementia, Alzheimer’s disease, vascular dementia, Lewy body dementia, Parkinson’s disease, and dementia in other diseases classified elsewhere (Figure 4).
Figure 4. Heatmap of GM causally associated with dementia identified by the IVW method. Red represents risk factors, and blue represents protective factors.
Cochrane’s Q test showed no heterogeneity among the 20 colonies except for the genus Defluviitaleaceae UCG011 (p = 0.031) in dementia, which had a value of p greater than 0.05 (Supplementary Table 3). Genus Defluviitaleaceae UCG011 had p < 0.05 (p = 0.002) in the fixed-effects IVW model, suggesting the presence of causality, and also p < 0.05 (p = 0.034), OR: 0.735, CI: 0.553–0.977 in the random-effects IVW model, and a cause-and-effect link was also present. The MR-Egger regression intercepts for the 21 GMs showed no horizontal pleiotropy, with p-values greater than 0.05 (Supplementary Table 4). The MR-PRESSO Global test value of p > 0.05 also demonstrated no horizontal pleiotropy (Supplementary Table 4). Leave-one-out results showed that phasing out any of the SNPs did not affect the overall results, so this MR analysis has good robustness (Supplementary Figure S1).
Out of the 211 GMs, a total of 50 GMs affected by dementia and its subtypes were finally obtained, including 13 GMs affected by overall dementia, eight GMs by Alzheimer’s disease, five GMs by vascular dementia, seven GMs by Lewy body dementia, nine GMs by Parkinson’s disease, and eight GMs by dementia under the classification of other diseases. Five TSMR methods—IVW, MR-Egger, weighted median, simple mode, and weighted mode—were used to analyze the causal relationships between the different types of dementia and the 50 GMs (Supplementary Table 5). Forest plots were drawn using IVW and WM cross-validation (Figure 5). Upon comparison with the positive MR results, it was found that among the 21 GMs we focused on for causality with dementia, there was only a reverse causality between Lewy body dementia and genus Ruminococcusgnavus group (id: 14376), and no reverse causality was found between the remaining 20 GMs and dementia. Further sensitivity analysis of MR results between Lewy body dementia and genus Ruminococcusgnavus group (Table 3) was performed, and the test showed no heterogeneity or horizontal pleiotropy in this result.
The first validation dataset obtained 121 IVs of GMs associated with dementia involving 10 GMs; the second obtained 77 IVs of GMs associated with dementia involving eight GMs; and the third obtained 82 IVs of GMs associated with dementia involving eight GMs. The MR analysis methodology was consistent with the above studies, and detailed information on the results can be found in Supplementary Table 6. All results passed sensitivity tests. Similarly, a forest plot of the IVW and WM cross-tests was plotted and is shown in Figure 6. Compared to the six GMs associated with the formal group any dementia, five overlapping GMs were in the first validation group and four in the second and third validation groups. Three validation groups had four GMs overlapping with the formal group any dementia, accounting for 66.7% of the formal any dementia group, 40% of the first validation group, and 50% of the second and third validation groups. Therefore, the selected formal group dataset is representative.
Figure 6. Forest plot of causal relationships between GMs and three validation groups under cross-validation.
In this study, by combining MR analysis and sensitivity analysis, 21 GMs were identified as being causally associated with dementia (any dementia, Alzheimer’s disease, vascular dementia, Lewy body dementia, Parkinson’s disease, and dementia in other diseases classified elsewhere). Among them, Desulfovibrionaceae, Lactobacillaceae, Ruminococcusgnavus group, Lactobacillus, Desulfovibrionales, Sellimonas, Bacillales, and Hungatella were positively associated with the risk of outcome disease, and therefore, there may be a risk for the corresponding types of dementia. Defluviitaleaceae UCG011, Bacillales, Lachnospiraceae NK4A136 group, Victivallales, Alphaproteobacteria, Ruminococcusgnavus group, Butyricimonas, Lentisphaerae, Oscillibacter, and Burkholderiales were negatively correlated with the risk of outcome disease, and they may be protective against the corresponding types of dementia.
Understanding the pathogenesis of dementia and the role GM plays in this process is critical to preventing and intervening in dementia. The gut-brain axis is the pathway of communicating among the nervous system and the gastrointestinal tract, which mainly includes the central nervous system (CNS), enteric nervous system (ENS), hypothalamic–pituitary–adrenal axis (HPA), and autonomic nervous system (ANS; Cryan et al., 2019). Moreover, it has been found that GM can influence the pathophysiological processes of diseases such as Alzheimer’s disease and Parkinson’s through ENS (Glinert et al., 2022). For example, it activates the ENS and uses the vagus nerve as a pathway to communicate with the brain (Wang et al., 2020). In addition, GM triggers the progression of a metabolic, inflammatory response that promotes neuroinflammation by engaging in processes that disrupt the blood–brain barrier (BBB), activating astrocytes and microglia, and leading to the deposition of β-amyloid (Aβ), which is now recognized as a significant contributing factor in neurodegenerative diseases (Cryan et al., 2019; Wei et al., 2020; Mou et al., 2022). Relevant scholars have proven that the majority of the variable risk factors for dementia are associated with GM alterations by studying the different variable risk factors for each type of dementia and the different roles of GM for each factor (Cabrera et al., 2021). However, the constitution of GM is subject to the effect of numerous causes, and the diversity of GM may vary due to inconsistencies in gender, ethnicity, and environment.
In our study, we found that order Desulfovibrionales (OR: 1.592, CI: 1.011–2.507, p = 0.045) and family Desulfovibrionaceae (OR: 1.682, CI: 1.102–2.568, p = 0.016) were strongly related to a high risk of Alzheimer’s disease. The results of related experiments showed that Desulfovibrionaceae abundance at the family and genus levels was significantly higher in amyloid precursor protein transgenic mice than in wild mice (Shen et al., 2017). Abnormal production and processing of Aβ and hyperphosphorylation of tau proteins are the molecular signatures of Alzheimer’s disease (He et al., 2020). GM has been shown to reduce Aβ load in patients with Alzheimer’s disease (Li et al., 2019), and related researchers have found that brain Aβ accumulation is negatively correlated with the family Desulfovibrionaceae (Sheng et al., 2022). Tetragonia Tetragonioides Kuntze (TTK) ameliorates memory by decreasing Aβ deposition and modulating GM, with more Desulfovibrionales in the AD-Control group than AD-TTK (Kim et al., 2020). The above studies mentioned the family/order Desulfovibrionales as clinically significant for Alzheimer’s disease. However, the results of the two studies on Aβ deposition in the brain conflicted. In the present study, we found with MR analysis that the family/order Desulfovibrionaceae was associated with an increased risk of developing dementia and Alzheimer’s disease. A growing body of research has been able to demonstrate that altering GMs can attenuate microglia-mediated neuroinflammation and reduce Aβ deposition in the brain, thereby improving cognition (Abraham et al., 2019; Sun et al., 2020; Benichou Haziot and Birak, 2023). Supporting the above findings, we propose that the effect of family/order Desulfovibrionaceae on patients of dementia or Alzheimer’s disease might be related to brain Aβ deposition. However, the exact mechanism of action has not yet been confirmed. Inhibition of patient-specific family/order Desulfovibrionaceae and further study of its pathogenesis based on this may become a new way of intervention to prevent or delay Alzheimer’s disease.
Probiotics are non-pathogenic microorganisms and are beneficial to the organism’s health, with a great capacity to rebuild the microbiota and restore health (Den et al., 2020). Notably, probiotic treatment attenuates age-related learning and memory deficits by reducing microglia activation (Go et al., 2021). Therefore, it has been used as a potential treatment to alleviate psychiatric disorders, including cognitive impairment (CI; Azad et al., 2018). Lactobacillales as a probiotic has been widely used in various CI-related studies, and a study was conducted to induce the expression of brain-derived neurotropic factor (BDNF), inhibit NF-κB activation, and regulate GM in mice to alleviate CI accompanied by systemic inflammation through Lactobacillus griseus (Yun et al., 2023). A systematic evaluation showed increased levels of brain-derived neurotrophic factor, improved inflammatory profile, and cellular biomarker modulation in patients with dementia taking probiotic Lactobacillus (Ruiz-Gonzalez et al., 2021). In addition, the AD-Control group with excessive brain Aβ deposition decreased in the order Lactobacillales (Lactobacillales) compared to the AD-TTK group (Kim et al., 2020). A Mediterranean diet (MeDi) containing very high amounts of Lactobacillales has also been highly effective in preventing Alzheimer’s disease (Trichopoulou and Lagiou, 1997; Walker, 2000; Alfawaz and Aljumah, 2012). It is believed that the evidence that Lactobacillus reduces blood ammonia levels not only offers a connection between Alzheimer’s disease and the MeDi but also lays the groundwork for hyperammonemia and the pharmacology of various neurological disorders (Alfawaz and Aljumah, 2012; Jin et al., 2018). The above research demonstrated the protective function of Lactobacillales in CI from different angles of action. However, our results showed that the family Lactobacillaceae and genus Lactobacillus were weakly correlated with the increased risk of dementia. The reason may be related to sample size, genetics, and research scope.
In addition, our results also showed causal associations with outcomes for probiotics, including Defluvititaleaceae UCG011 associated with dementia, Bacillale associated with Alzheimer’s disease, Ruminococcusgnavus group associated with Lewy body dementia, Lachnospiraceae NK4A136 group and Victivallales strongly associated with vascular dementia, Butyricimonas and Lentisphaerae strongly associated with Parkinson’s disease, and Oscillibacter and Burkholderiales strongly associated with dementia in other diseases classified elsewhere. Some of these results are consistent with existing research findings where Bacillussubtilis was shown to have a protective effect on neurons and behavior in the Caenorhabditis elegans AD model and can help alleviate Alzheimer’s disease (Cogliati et al., 2020). Butyricimona has also been shown to be strongly associated with the reduced hippocampal volume associated with cognitive disorder. Jang hypothesized that acupuncture alleviated inflammation in mice with Parkinson’s disease due to an increase in Butyricimonas (Jang et al., 2020; Liang et al., 2022). Neoagarotetraose (NAT) was shown to modulate GM and thereby attenuate brain damage in mice with Alzheimer’s disease, with a remarkable rise of intestinal bacterial genera (Lactobacillus, Butyricimonas, and Akkermansi) observed after NAT treatment (Li et al., 2023). Our study clarified the beneficial bacterial genera for dementia, Alzheimer’s disease, Parkinson’s disease, and Lewy body dementia. This might be a novel research line for the clinical therapy of various types of dementia.
Short-chain fatty acids (SCFAs), which mainly include acetate, propionate, and butyrate, are metabolites produced by GM. Butyrate in SCFAs has anti-inflammatory effects (Mirzaei et al., 2021) and can improve cognitive function by mediating inflammatory responses and inducing Aβ phagocytosis in microglia (Xie et al., 2023). It has been found that Alzheimer’s disease may occur when butyrate is deficient (Tran et al., 2019). Interestingly, propionate induced higher levels of microglia activation than butyrate (Hou et al., 2021), and this hyperactivated state may reduce their ability to phagocytose Aβ, which may have a differential effect on the disease (Xie et al., 2021). When excessive propionate is ingested, there is an increased risk of developing Alzheimer’s disease (Killingsworth et al., 2021). Ruminococcaceae can promote the production of SCFAs and can be associated with diseases of cognitive dysfunction by affecting the expression of proteins involved in neurotransmission (D’Amato et al., 2020). This study showed that the Ruminococcusgnavus group was associated with a risk of dementia, Lewy body dementia, and dementia in other diseases classified elsewhere. However, its high and low risk of different outcome diseases was inconsistent, and we hypothesized that this might be related to the metabolite SCFAs it produces. The different types and doses of SCFAs might be the influencing factors. In addition, the results of the reverse MR analysis done in this study suggested that elevated levels of Ruminococcusgnavus group were associated with an increased risk of Lewy body dementia. Therefore, the present study provides possible mechanism points of SCFAs for dementia at the microbial level, and its specific role and association need to be further explored.
In addition, this study found a strong risk association between Alphaproteobacteria and Lewy body dementia [OR = 1.97 (95% CI: 1.320–2.940) p = 0.001]. It has been shown that GM is associated with Lewy body dementia, a pathology of dementia characterized by aggregation of α-synuclein, in which the microbe-gut-brain axis plays a vital role through a variety of potential mechanisms (Ryman et al., 2023). However, research into the relationship between Alphaproteobacteria and Lewy body dementia is scarce; Alphaproteobacteria is usually associated with depression, and antidepressants can reduce their abundance (Lukić et al., 2019). Therefore, the conclusion of this study provides suggestions for future research areas with regard to Alphaproteobacteria for the treatment of Lewy body dementia, which may be the key mechanism of its pathogenesis or a potential therapeutic target.
Current research on GM and various types of dementia is both a hot topic and a great challenge at the same time. Since there is no method of preventing, reversing, or eradicating Alzheimer’s disease, medications licensed for the therapy of Alzheimer’s disease have only been able to slow progression to improve symptoms (Breijyeh and Karaman, 2020). Therefore, in terms of GM and dementia, future research should focus on identifying specific GM bacteria with the pathogenesis of dementia. On the one hand, different GM taxa may have diagnostic value for various types of dementia. On the other hand, the risk of dementia can be reduced through the development of new drugs, disease prevention, treatment, and other aspects.
The limitations of this study are as follows: (i) since the number of IVs satisfying the strict threshold (p < 5 × 10−8) was minimal, a relatively loose threshold (p < 1 × 10−5) was used to screen the IVs; (ii) in this study, part of the data for dementia was obtained in 2021 from the FinnGen database version R5, the most recent online data for the IEU data. Nevertheless, there are still limitations regarding the duration of data collection and the quantity of available data. Further supplementation of the results of this study is warranted in the future through the ongoing updating of online data; and (iii) the number of cases of strictly defined as vascular dementia and Parkinson’s disease is relatively low, so a more significant amount of GWAS pooled data is needed for future analysis.
Altogether, we confirmed a causal relationship between GM and dementia and its subtypes based on Mendelian randomization, including family Desulfovibrionaceae (id: 3169), family Lactobacillaceae (id: 1836), genus Ruminococcusgnavus group (id: 14376), genus Defluviitaleaceae UCG011 (id: 11287), genus Lactobacillus (id: 1837), order Desulfovibrionales (id: 3156), family Desulfovibrionaceae (id: 3169), genus Sellimonas (id: 14369), order Bacillales (id: 1674), order Desulfovibrionales (id: 3156), genus Lachnospiraceae NK4A136 group (id:11319), order Victivallales (id: 2254), class Alphaproteobacteria (id: 2379), genus Ruminococcusgnavus group (id: 14376), order Bacillales (id: 1674), genus Butyricimonas (id: 945), phylum Lentisphaerae (id: 2238), genus Ruminococcusgnavus group (id: 14376), genus Hungatella (id: 11306), genus Oscillibacter (id: 2063), and order Burkholderiales (id: 2874). These 21 GMs hold promise as novel markers for the future diagnosis of dementia and its subtypes, as well as new targets for therapy.
The original contributions presented in the study are included in the article/Supplementary material further inquiries can be directed to the corresponding author.
JF: Methodology, Software, Visualization, Writing – original draft, Writing – review & editing. YQ: Formal analysis, Investigation, Visualization, Writing – original draft. LX: Funding acquisition, Project administration, Writing – review & editing. XD: Funding acquisition, Project administration, Resources, Writing – review & editing.
The author(s) declare financial support was received for the research, authorship, and/or publication of this article. This study was supported by the National Key Research and Development Program of China (2022YFC3500501) and Tianjin Science and Technology Program (18PTLCSY00050).
The authors express our sincere gratitude to the FinnGen and GWAS Catalog personnel and volunteers, as well as the MiBioGen consortium, for their invaluable contributions in supplying gut microbiology data.
The authors declare that the research was conducted in the absence of any commercial or financial relationships that could be construed as a potential conflict of interest.
All claims expressed in this article are solely those of the authors and do not necessarily represent those of their affiliated organizations, or those of the publisher, the editors and the reviewers. Any product that may be evaluated in this article, or claim that may be made by its manufacturer, is not guaranteed or endorsed by the publisher.
The Supplementary material for this article can be found online at: https://www.frontiersin.org/articles/10.3389/fmicb.2023.1306048/full#supplementary-material
1. ^https://mibiogen.gcc.rug.nl
3. ^https://www.ebi.ac.uk/gwas/home
Aarsland, D. (2020). Epidemiology and pathophysiology of dementia-related psychosis. J. Clin. Psychiatry 81:AD19038BR1C. doi: 10.4088/JCP.AD19038BR1C
Abraham, D., Feher, J., Scuderi, G. L., Szabo, D., Dobolyi, A., Cservenak, M., et al. (2019). Exercise and probiotics attenuate the development of Alzheimer’s disease in transgenic mice: role of microbiome. Exp. Gerontol. 115, 122–131. doi: 10.1016/j.exger.2018.12.005
Alfawaz, H. A., and Aljumah, A. A. (2012). What improves minimal hepatic encephalopathy: probiotic yogurt, protein restriction or nonabsorbable disaccharides? Saudi J. Gastroenterol. 18, 153–154. doi: 10.4103/1319-3767.96443
Álvarez, J., Fernández Real, J. M., Guarner, F., Gueimonde, M., Rodríguez, J. M., Saenz de Pipaon, M., et al. (2021). Gut microbes and health. Gastroenterol. Hepatol. 44, 519–535. doi: 10.1016/j.gastrohep.2021.01.009
Azad, M. A. K., Sarker, M., Li, T., and Yin, J. (2018). Probiotic species in the modulation of gut microbiota: an overview. Biomed. Res. Int. 2018, 9478630–9478638. doi: 10.1155/2018/9478630
Benichou Haziot, C., and Birak, K. S. (2023). Therapeutic potential of microbiota modulation in Alzheimer’s disease: a review of preclinical studies. J Alzheimers Dis Rep 7, 415–431. doi: 10.3233/ADR-220097
Bowden, J., Davey Smith, G., and Burgess, S. (2015). Mendelian randomization with invalid instruments: effect estimation and bias detection through Egger regression. Int. J. Epidemiol. 44, 512–525. doi: 10.1093/ije/dyv080
Bowden, J., Davey Smith, G., Haycock, P. C., and Burgess, S. (2016). Consistent estimation in Mendelian randomization with some invalid instruments using a weighted median estimator. Genet. Epidemiol. 40, 304–314. doi: 10.1002/gepi.21965
Breijyeh, Z., and Karaman, R. (2020). Comprehensive review on Alzheimer’s disease: causes and treatment. Molecules 25:5789. doi: 10.3390/molecules25245789
Burgess, S., Butterworth, A., and Thompson, S. G. (2013). Mendelian randomization analysis with multiple genetic variants using summarized data. Genet. Epidemiol. 37, 658–665. doi: 10.1002/gepi.21758
Burgess, S., Small, D. S., and Thompson, S. G. (2017). A review of instrumental variable estimators for Mendelian randomization. Stat. Methods Med. Res. 26, 2333–2355. doi: 10.1177/0962280215597579
Burgess, S., and Thompson, S. G. (2017). Interpreting findings from Mendelian randomization using the MR-Egger method. Eur. J. Epidemiol. 32, 377–389. doi: 10.1007/s10654-017-0255-x
Burgess, S., and Thompson, S. G.CRP CHD Genetics Collaboration (2011). Avoiding bias from weak instruments in Mendelian randomization studies. Int. J. Epidemiol. 40, 755–764. doi: 10.1093/ije/dyr036
Cabrera, C., Vicens, P., and Torrente, M. (2021). Modifiable risk factors for dementia: the role of gut microbiota. Curr. Alzheimer Res. 18, 993–1009. doi: 10.2174/1567205018666211215152411
Cheah, W.-T., Hwang, J.-J., Hong, S.-Y., Fu, L.-C., Chang, Y.-L., Chen, T.-F., et al. (2022). A digital screening system for Alzheimer disease based on a neuropsychological test and a convolutional neural network: system development and validation. JMIR Med. Inform. 10:e31106. doi: 10.2196/31106
Chen, Y., Xu, J., and Chen, Y. (2021). Regulation of neurotransmitters by the gut microbiota and effects on cognition in neurological disorders. Nutrients 13:2099. doi: 10.3390/nu13062099
Cogliati, S., Clementi, V., Francisco, M., Crespo, C., Argañaraz, F., and Grau, R. (2020). Bacillus Subtilis delays neurodegeneration and behavioral impairment in the Alzheimer’s disease model Caenorhabditis Elegans. J. Alzheimers Dis. 73, 1035–1052. doi: 10.3233/JAD-190837
Cryan, J. F., O’Riordan, K. J., Cowan, C. S. M., Sandhu, K. V., Bastiaanssen, T. F. S., Boehme, M., et al. (2019). The microbiota-gut-brain Axis. Physiol. Rev. 99, 1877–2013. doi: 10.1152/physrev.00018.2018
Cryan, J. F., O’Riordan, K. J., Sandhu, K., Peterson, V., and Dinan, T. G. (2020). The gut microbiome in neurological disorders. Lancet Neurol. 19, 179–194. doi: 10.1016/S1474-4422(19)30356-4
Cuartero, M. I., García-Culebras, A., Nieto-Vaquero, C., Fraga, E., Torres-López, C., Pradillo, J., et al. (2023). The role of gut microbiota in cerebrovascular disease and related dementia. Br. J. Pharmacol. 16167. doi: 10.1111/bph.16167
D’Amato, A., Di Cesare Mannelli, L., Lucarini, E., Man, A. L., Le Gall, G., Branca, J. J. V., et al. (2020). Faecal microbiota transplant from aged donor mice affects spatial learning and memory via modulating hippocampal synaptic plasticity- and neurotransmission-related proteins in young recipients. Microbiome 8:140. doi: 10.1186/s40168-020-00914-w
Den, H., Dong, X., Chen, M., and Zou, Z. (2020). Efficacy of probiotics on cognition, and biomarkers of inflammation and oxidative stress in adults with Alzheimer’s disease or mild cognitive impairment—a meta-analysis of randomized controlled trials. Aging 12, 4010–4039. doi: 10.18632/aging.102810
EPIC-InterAct ConsortiumBurgess, S., Scott, R. A., Timpson, N. J., Davey Smith, G., and Thompson, S. G. (2015). Using published data in Mendelian randomization: a blueprint for efficient identification of causal risk factors. Eur. J. Epidemiol. 30, 543–552. doi: 10.1007/s10654-015-0011-z
Glinert, A., Turjeman, S., Elliott, E., and Koren, O. (2022). Microbes, metabolites and (synaptic) malleability, oh my! The effect of the microbiome on synaptic plasticity. Biol. Rev. Camb. Philos. Soc. 97, 582–599. doi: 10.1111/brv.12812
Go, J., Chang, D.-H., Ryu, Y.-K., Park, H.-Y., Lee, I.-B., Noh, J.-R., et al. (2021). Human gut microbiota Agathobaculum butyriciproducens improves cognitive impairment in LPS-induced and APP/PS1 mouse models of Alzheimer’s disease. Nutr. Res. 86, 96–108. doi: 10.1016/j.nutres.2020.12.010
Greco, M. F. D., Minelli, C., Sheehan, N. A., and Thompson, J. R. (2015). Detecting pleiotropy in Mendelian randomisation studies with summary data and a continuous outcome: detecting pleiotropy in Mendelian randomisation studies with summary data and a continuous outcome. Stat. Med. 34, 2926–2940. doi: 10.1002/sim.6522
Guo, M., Peng, J., Huang, X., Xiao, L., Huang, F., and Zuo, Z. (2021). Gut microbiome features of Chinese patients newly diagnosed with Alzheimer’s disease or mild cognitive impairment. J. Alzheimers Dis. 80, 299–310. doi: 10.3233/JAD-201040
He, Y., Li, B., Sun, D., and Chen, S. (2020). Gut microbiota: implications in Alzheimer’s disease. J. Clin. Med. 9:2042. doi: 10.3390/jcm9072042
Hou, Y., Li, X., Liu, C., Zhang, M., Zhang, X., Ge, S., et al. (2021). Neuroprotective effects of short-chain fatty acids in MPTP induced mice model of Parkinson’s disease. Exp. Gerontol. 150:111376. doi: 10.1016/j.exger.2021.111376
Hung, C.-C., Chang, C.-C., Huang, C.-W., Nouchi, R., and Cheng, C.-H. (2022). Gut microbiota in patients with Alzheimer’s disease spectrum: a systematic review and meta-analysis. Aging 14, 477–496. doi: 10.18632/aging.203826
Jang, J.-H., Yeom, M.-J., Ahn, S., Oh, J.-Y., Ji, S., Kim, T.-H., et al. (2020). Acupuncture inhibits neuroinflammation and gut microbial dysbiosis in a mouse model of Parkinson’s disease. Brain Behav. Immun. 89, 641–655. doi: 10.1016/j.bbi.2020.08.015
Jin, Q., Ren, F., Dai, D., Sun, N., Qian, Y., and Song, P. (2023). The causality between intestinal flora and allergic diseases: insights from a bi-directional two-sample Mendelian randomization analysis. Front. Immunol. 14:1121273. doi: 10.3389/fimmu.2023.1121273
Jin, Y., Singh, P., Chung, H.-J., and Hong, S.-T. (2018). Blood Ammonia as a possible etiological agent for Alzheimer’s disease. Nutrients 10:564. doi: 10.3390/nu10050564
Kamat, M. A., Blackshaw, J. A., Young, R., Surendran, P., Burgess, S., Danesh, J., et al. (2019). PhenoScanner V2: an expanded tool for searching human genotype–phenotype associations. Bioinformatics 35, 4851–4853. doi: 10.1093/bioinformatics/btz469
Killingsworth, J., Sawmiller, D., and Shytle, R. D. (2021). Propionate and Alzheimer’s disease. Front. Aging Neurosci. 12:580001. doi: 10.3389/fnagi.2020.580001
Kim, D. S., Ko, B.-S., Ryuk, J. A., and Park, S. (2020). Tetragonia tetragonioides protected against memory dysfunction by elevating hippocampal amyloid-β deposition through potentiating insulin signaling and altering gut microbiome composition. Int. J. Mol. Sci. 21:2900. doi: 10.3390/ijms21082900
Kurilshikov, A., Medina-Gomez, C., Bacigalupe, R., Radjabzadeh, D., Wang, J., Demirkan, A., et al. (2021). Large-scale association analyses identify host factors influencing human gut microbiome composition. Nat. Genet. 53, 156–165. doi: 10.1038/s41588-020-00763-1
Kuziel, G. A., and Rakoff-Nahoum, S. (2022). The gut microbiome. Curr. Biol. 32, R257–R264. doi: 10.1016/j.cub.2022.02.023
Li, B., He, Y., Ma, J., Huang, P., Du, J., Cao, L., et al. (2019). Mild cognitive impairment has similar alterations as Alzheimer’s disease in gut microbiota. Alzheimers Dement. 15, 1357–1366. doi: 10.1016/j.jalz.2019.07.002
Li, T., Yang, S., Liu, X., Li, Y., Gu, Z., and Jiang, Z. (2023). Dietary neoagarotetraose extends lifespan and impedes brain aging in mice via regulation of microbiota-gut-brain axis. J. Adv. Res. 52, 119–134. doi: 10.1016/j.jare.2023.04.014
Liang, X., Fu, Y., Cao, W., Wang, Z., Zhang, K., Jiang, Z., et al. (2022). Gut microbiome, cognitive function and brain structure: a multi-omics integration analysis. Transl Neurodegener 11:49. doi: 10.1186/s40035-022-00323-z
Liu, P., Wu, L., Peng, G., Han, Y., Tang, R., Ge, J., et al. (2019). Altered microbiomes distinguish Alzheimer’s disease from amnestic mild cognitive impairment and health in a Chinese cohort. Brain Behav. Immun. 80, 633–643. doi: 10.1016/j.bbi.2019.05.008
Livingston, G., Huntley, J., Sommerlad, A., Ames, D., Ballard, C., Banerjee, S., et al. (2020). Dementia prevention, intervention, and care: 2020 report of the lancet commission. Lancet 396, 413–446. doi: 10.1016/S0140-6736(20)30367-6
Lukić, I., Getselter, D., Ziv, O., Oron, O., Reuveni, E., Koren, O., et al. (2019). Antidepressants affect gut microbiota and Ruminococcus flavefaciens is able to abolish their effects on depressive-like behavior. Transl. Psychiatry 9:133. doi: 10.1038/s41398-019-0466-x
Lv, W., Lin, X., Shen, H., Liu, H., Qiu, X., Li, B., et al. (2021). Human gut microbiome impacts skeletal muscle mass via gut microbial synthesis of the short-chain fatty acid butyrate among healthy menopausal women. J. Cachexia. Sarcopenia Muscle 12, 1860–1870. doi: 10.1002/jcsm.12788
Mirzaei, R., Bouzari, B., Hosseini-Fard, S. R., Mazaheri, M., Ahmadyousefi, Y., Abdi, M., et al. (2021). Role of microbiota-derived short-chain fatty acids in nervous system disorders. Biomed. Pharmacother. 139:111661. doi: 10.1016/j.biopha.2021.111661
Mitrea, L., Nemeş, S.-A., Szabo, K., Teleky, B.-E., and Vodnar, D.-C. (2022). Guts imbalance imbalances the brain: a review of gut microbiota association with neurological and psychiatric disorders. Front. Med. 9:813204. doi: 10.3389/fmed.2022.813204
Möhle, L., Mattei, D., Heimesaat, M. M., Bereswill, S., Fischer, A., Alutis, M., et al. (2016). Ly6C(hi) monocytes provide a link between antibiotic-induced changes in gut microbiota and adult hippocampal neurogenesis. Cell Rep. 15, 1945–1956. doi: 10.1016/j.celrep.2016.04.074
Mou, Y., Du, Y., Zhou, L., Yue, J., Hu, X., Liu, Y., et al. (2022). Gut microbiota Interact with the brain through systemic chronic inflammation: implications on Neuroinflammation, neurodegeneration, and aging. Front. Immunol. 13:796288. doi: 10.3389/fimmu.2022.796288
Ni, J.-J., Xu, Q., Yan, S.-S., Han, B.-X., Zhang, H., Wei, X.-T., et al. (2021). Gut microbiota and psychiatric disorders: a two-sample Mendelian randomization study. Front. Microbiol. 12:737197. doi: 10.3389/fmicb.2021.737197
Ruiz-Gonzalez, C., Roman, P., Rueda-Ruzafa, L., Rodriguez-Arrastia, M., and Cardona, D. (2021). Effects of probiotics supplementation on dementia and cognitive impairment: a systematic review and meta-analysis of preclinical and clinical studies. Prog. Neuropsychopharmacol. Biol. Psychiatry 108:110189. doi: 10.1016/j.pnpbp.2020.110189
Ryman, S., Vakhtin, A. A., Richardson, S. P., and Lin, H. C. (2023). Microbiome-gut-brain dysfunction in prodromal and symptomatic Lewy body diseases. J. Neurol. 270, 746–758. doi: 10.1007/s00415-022-11461-9
Sasmita, A. O. (2019). Modification of the gut microbiome to combat neurodegeneration. Rev. Neurosci. 30, 795–805. doi: 10.1515/revneuro-2019-0005
Sekula, P., Del Greco, M. F., Pattaro, C., and Köttgen, A. (2016). Mendelian randomization as an approach to assess causality using observational data. J. Am. Soc. Nephrol. 27, 3253–3265. doi: 10.1681/ASN.2016010098
Shen, L., Liu, L., and Ji, H.-F. (2017). Alzheimer’s disease histological and behavioral manifestations in transgenic mice correlate with specific gut microbiome state. J. Alzheimers Dis. 56, 385–390. doi: 10.3233/JAD-160884
Sheng, C., Yang, K., He, B., Du, W., Cai, Y., and Han, Y. (2022). Combination of gut microbiota and plasma amyloid-β as a potential index for identifying preclinical Alzheimer’s disease: a cross-sectional analysis from the SILCODE study. Alzheimers Res Therapy 14:35. doi: 10.1186/s13195-022-00977-x
Skrivankova, V. W., Richmond, R. C., Woolf, B. A. R., Yarmolinsky, J., Davies, N. M., Swanson, S. A., et al. (2021). Strengthening the reporting of observational studies in epidemiology using Mendelian randomization: the STROBE-MR statement. JAMA 326, 1614–1621. doi: 10.1001/jama.2021.18236
Sun, J., Xu, J., Yang, B., Chen, K., Kong, Y., Fang, N., et al. (2020). Effect of Clostridium butyricum against microglia-mediated Neuroinflammation in Alzheimer’s disease via regulating gut microbiota and metabolites butyrate. Mol. Nutr. Food Res. 64:e1900636. doi: 10.1002/mnfr.201900636
Tran, T. T. T., Corsini, S., Kellingray, L., Hegarty, C., Le Gall, G., Narbad, A., et al. (2019). APOE genotype influences the gut microbiome structure and function in humans and mice: relevance for Alzheimer’s disease pathophysiology. FASEB J. 33, 8221–8231. doi: 10.1096/fj.201900071R
Trichopoulou, A., and Lagiou, P. (1997). Healthy traditional Mediterranean diet: an expression of culture, history, and lifestyle. Nutr. Rev. 55, 383–389. doi: 10.1111/j.1753-4887.1997.tb01578.x
Verbanck, M., Chen, C.-Y., Neale, B., and Do, R. (2018). Detection of widespread horizontal pleiotropy in causal relationships inferred from Mendelian randomization between complex traits and diseases. Nat. Genet. 50, 693–698. doi: 10.1038/s41588-018-0099-7
Walker, W. A. (2000). Role of nutrients and bacterial colonization in the development of intestinal host defense. J. Pediatr. Gastroenterol. Nutr. 30, S2–S7. doi: 10.1097/00005176-200000002-00002
Wang, S.-Z., Yu, Y.-J., and Adeli, K. (2020). Role of gut microbiota in neuroendocrine regulation of carbohydrate and lipid metabolism via the microbiota-gut-brain-liver Axis. Microorganisms 8:527. doi: 10.3390/microorganisms8040527
Wei, S., Peng, W., Mai, Y., Li, K., Wei, W., Hu, L., et al. (2020). Outer membrane vesicles enhance tau phosphorylation and contribute to cognitive impairment. J. Cell. Physiol. 235, 4843–4855. doi: 10.1002/jcp.29362
World Alzheimer Report (2015), The Global Impact of Dementia: An analysis of prevalence, incidence, cost and trends.
Xiang, K., Wang, P., Xu, Z., Hu, Y.-Q., He, Y.-S., Chen, Y., et al. (2021). Causal effects of gut microbiome on systemic lupus erythematosus: a two-sample Mendelian randomization study. Front. Immunol. 12:667097. doi: 10.3389/fimmu.2021.667097
Xie, J., Bruggeman, A., De Nolf, C., Vandendriessche, C., Van Imschoot, G., Van Wonterghem, E., et al. (2023). Gut microbiota regulates blood-cerebrospinal fluid barrier function and Aβ pathology. EMBO J. 42:e111515. doi: 10.15252/embj.2022111515
Xie, J., Gorlé, N., Vandendriessche, C., Van Imschoot, G., Van Wonterghem, E., Van Cauwenberghe, C., et al. (2021). Low-grade peripheral inflammation affects brain pathology in the AppNL-G-Fmouse model of Alzheimer’s disease. Acta Neuropathol. Commun. 9:163. doi: 10.1186/s40478-021-01253-z
Yun, S.-W., Park, H.-S., Shin, Y.-J., Ma, X., Han, M. J., and Kim, D.-H. (2023). Lactobacillus gasseri NK109 and its supplement alleviate cognitive impairment in mice by modulating NF-κB activation, BDNF expression, and gut microbiota composition. Nutrients 15:790. doi: 10.3390/nu15030790
Zagórska, A., Czopek, A., Fryc, M., Jaromin, A., and Boyd, B. J. (2023). Drug discovery and development targeting dementia. Pharmaceuticals (Basel) 16:151. doi: 10.3390/ph16020151
Keywords: dementia, gut microflora, Mendelian randomization, causality, Alzheimer’s disease
Citation: Fu J, Qin Y, Xiao L and Dai X (2024) Causal relationship between gut microflora and dementia: a Mendelian randomization study. Front. Microbiol. 14:1306048. doi: 10.3389/fmicb.2023.1306048
Received: 03 October 2023; Accepted: 11 December 2023;
Published: 15 January 2024.
Edited by:
Vijaykumar Muley, National Autonomous University of Mexico, MexicoReviewed by:
Sylwia Dziegielewska-Gesiak, Medical University of Silesia, PolandCopyright © 2024 Fu, Qin, Xiao and Dai. This is an open-access article distributed under the terms of the Creative Commons Attribution License (CC BY). The use, distribution or reproduction in other forums is permitted, provided the original author(s) and the copyright owner(s) are credited and that the original publication in this journal is cited, in accordance with accepted academic practice. No use, distribution or reproduction is permitted which does not comply with these terms.
*Correspondence: Xiaoyu Dai, ZHJkYWl4aWFveXVAMTYzLmNvbQ==
†These authors have contributed equally to this work and share first authorship
Disclaimer: All claims expressed in this article are solely those of the authors and do not necessarily represent those of their affiliated organizations, or those of the publisher, the editors and the reviewers. Any product that may be evaluated in this article or claim that may be made by its manufacturer is not guaranteed or endorsed by the publisher.
Research integrity at Frontiers
Learn more about the work of our research integrity team to safeguard the quality of each article we publish.