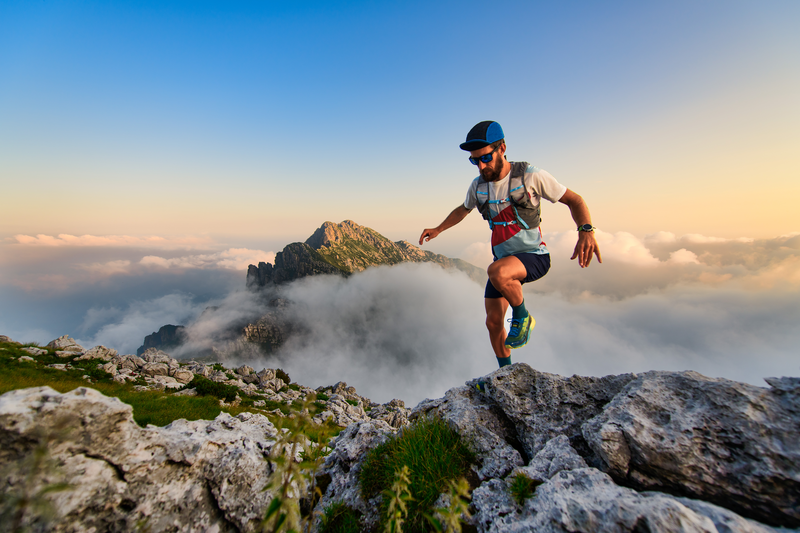
94% of researchers rate our articles as excellent or good
Learn more about the work of our research integrity team to safeguard the quality of each article we publish.
Find out more
ORIGINAL RESEARCH article
Front. Microbiol. , 30 November 2023
Sec. Microorganisms in Vertebrate Digestive Systems
Volume 14 - 2023 | https://doi.org/10.3389/fmicb.2023.1304232
This article is part of the Research Topic Fecal Microbiota Transplants: challenges in translating microbiome research to clinical applications View all 17 articles
Introduction: “Probiotic therapy” to regulate gut microbiota and intervene in intestinal diseases such as inflammatory bowel disease (IBD) has become a research hotspot. Bacteroides acidifaciens, as a new generation of probiotics, has shown beneficial effects on various diseases.
Methods: In this study, we utilized a mouse colitis model induced by dextran sodium sulfate (DSS) to investigate how B. acidifaciens positively affects IBD. We evaluated the effects ofB. acidifaciens, fecal microbiota transplantation, and bacterial extracellular vesicles (EVs) on DSS-induced colitis in mice. We monitored the phenotype of mouse colitis, detected serum inflammatory factors using ELISA, evaluated intestinal mucosal barrier function using Western blotting and tissue staining, evaluated gut microbiota using 16S rRNA sequencing, and analyzed differences in EVs protein composition derived from B. acidifaciens using proteomics to explore how B. acidifaciens has a positive impact on mouse colitis.
Results: We confirmed that B. acidifaciens has a protective effect on colitis, including alleviating the colitis phenotype, reducing inflammatory response, and improving intestinal barrier function, accompanied by an increase in the relative abundance of B. acidifaciens and Ruminococcus callidus but a decrease in the relative abundance of B. fragilis. Further fecal bacterial transplantation or fecal filtrate transplantation confirmed the protective effect of eosinophil-regulated gut microbiota and metabolites on DSS-induced colitis. Finally, we validated that EVs derived from B. acidifaciens contain rich functional proteins that can contribute to the relief of colitis.
Conclusion: Therefore, B. acidifaciens and its derived EVs can alleviate DSS-induced colitis by reducing mucosal damage to colon tissue, reducing inflammatory response, promoting mucosal barrier repair, restoring gut microbiota diversity, and restoring gut microbiota balance in mice. The results of this study provide a theoretical basis for the preclinical application of the new generation of probiotics.
Inflammatory bowel disease (IBD) is a chronic disease with unclear pathogenesis and a repeated course and is mainly divided into Crohn’s disease and ulcerative colitis (Jakubczyk et al., 2020; Santana et al., 2022). The basic characteristics of IBD are abdominal pain and diarrhea, which can be complicated with colon stenosis and obstruction and tissue fibrosis and even induce colon cancer (Ullman and Itzkowitz, 2011). Since 1950, the number of patients in the western world, represented by Europe and America, has gradually increased, and recent epidemiological surveys show that the number of patients in eastern countries has also begun to increase rapidly (Molodecky et al., 2012). IBD has become a global disease. At present, although there are many treatments for IBD, such as hormones, immunosuppressants, biological agents, small molecule drugs and surgical treatments, the therapeutic effects are limited due to the unclear disease mechanism (Rogler et al., 2021).
Although the etiology of IBD is unknown, it is recognized that IBD is influenced by heredity, environment and intestinal microbiota, and it is characterized by a host immune response, intestinal barrier destruction and intestinal microbial community changes (Kudelka et al., 2020). The intestinal microbiota plays an important role in maintaining the intestinal mucosal barrier, stabilizing intestinal immune function and regulating intestinal metabolism (Li et al., 2021). However, intestinal microbiota imbalance or gut microbiota displacement can lead to intestinal inflammation and even tumors (Deng et al., 2022). Studies have shown that compared with healthy people, the ecological imbalance of IBD is characterized by a decrease in bacterial diversity or a change in composition (Franzosa et al., 2018). Therefore, it has become a research hotspot to intervene in intestinal diseases such as IBD by regulating intestinal microbiota. For example, “probiotic therapy,” in which enough live probiotics are supplemented to maintain the health of the host, has been widely used in the clinic and has achieved certain results (Fedorak et al., 2015; Tamaki et al., 2015).
Under physiological conditions, 90% of the intestinal microflora are composed of Firmicutes and Bacteroides (Eckburg et al., 2005). Bacteroides acidifaciens belongs to Bacteroides, a gram-negative anaerobic bacterium and one of the dominant symbiotic bacteria in the intestine (Momose et al., 2011). A study revealed that in a mouse model, B. acidifaciens has a protective effect on concanavalin A-induced liver injury (Wang et al., 2022). Nagahara et al. revealed that B. acidifaciens can increase the level of intestinal immunoglobulin A (IgA), protect the intestine from pathogen infection and relieve IBD (Nakajima et al., 2020). Another study showed that alginate oligosaccharide and its compound supplement may enhance immune function and improve intestinal microecological disorder by increasing the abundance of B. acidifaciens (Yan et al., 2023). Tamas Korcsmaros et al. revealed that bacterial extracellular vesicles (EVs) produced by the intestinal symbiotic bacteria Bacteroides thetaiotaomicron have potential therapeutic value in IBD (Gul et al., 2022). EVs are small, spherical double-layer structures (20∼400 nm) containing proteins, lipids, nucleic acids and some metabolites, which are secreted by gram-negative bacteria and some gram-positive bacteria (Kaparakis-Liaskos and Ferrero, 2015; Toyofuku et al., 2019). EVs are important cell communication carriers that participate in the exchange of biological information between prokaryotes and eukaryotes. EVs can pass through the aseptic mucous layer of the colon through different routes, enter the border intestinal epithelial cells, interact with mucosal immune cells and the intestinal vascular system, and promote its widespread and systemic spread (Chronopoulos and Kalluri, 2020; Liu J. H. et al., 2021; Chen et al., 2022). Previous studies have shown that EVs play an important role in regulating the intestinal microenvironment and host health (Díaz-Garrido et al., 2021; Liu L. et al., 2021). This indicates that bacterial EVs are a possible factor by which the intestinal microbiota alleviates diseases.
In this study, we used B. acidifaciens to interfere with dextran sulfate sodium (DSS)-induced colitis in mice, revealing its anti-inflammatory and barrier protection effects on enteritis mice. The effects of B. acidifaciens-mediated microorganisms and their metabolites on alleviating the symptoms of enteritis mice were confirmed by fecal bacteria transplantation (FMT) and aseptic fecal filtrate transplantation (FFT). Finally, we found that EVs of B. acidifaciens can inhibit inflammatory reactions, repair the intestinal mucosal barrier, and finally alleviate colitis in mice. Through proteomic analysis, it was confirmed that the functional proteins of B. acidifaciens and B. acidifaciens-EVs were different.
This study was approved by the Animal Experiment Ethics Committee of Nanchang University (NCULAE-20221031149).
Bacteroides acidifaciens (BNCC353574, BeNa, Henan, China) was cultured on fastidious anaerobe broth (FAB, Cat# LA4550; Solarbio, Inc., China) or Columbia agar (Cat#HB8511; Qingdao Hope Bio-Technology, Co., Ltd., China) with 5% goat blood (Cat# TX0020; Solarbio, Inc., China) under anaerobic conditions at 37°C for 48–72 h (Wang et al., 2022). The bacterial concentration was calculated by counting the number of CFUs after plating on Columbia agar containing 5% goat blood.
The conditioned medium containing B. acidifaciens was obtained as described above. The conditioned culture of B. acidifaciens was centrifuged at 15,000 × g at 4°C for 20 min and then filtered through a 0.45 μm sterile filter membrane to remove residual bacteria and debris. The filtered supernatant was centrifuged at 20,000 × g at 4°C for 40 min and then filtered through a 0.22 μm sterile filter membrane. The supernatant was transferred to 100 kDa Amicon Ultra15 filters at a concentration of 300–400 times. The concentrated solution was centrifuged at 120,000 × g at 4°C for 2 h to remove the supernatant, washed with sterile PBS, and centrifugation was repeated once to obtain EVs (Ma et al., 2022). As described, EVs were purified by OptiPrep (Cat# D1556, Sigma Aldrich, USA) gradient density centrifugation. Nanoparticle tracking analysis (NTA) method was used to detect the particle size distribution of EVs, and transmission electron microscopy (TEM) was used to observe the morphology of EVs.
In this study, male C57BL/6J mice aged 6–8 weeks and weighing approximately 22–25 g were purchased from Tianqin Biotechnology Co., Ltd (Changsha, Hunan, China). Mice were reared at 22 ± 2°C for 1 week under an alternating light/dark cycle every 12 h. One week later, the mouse colitis model was induced by 2.5% dextran sodium sulfate (molecular mass 36–50 kDa, MP Biologicals, Solon, OH, USA) in a free drink. To study the therapeutic effect of B. acidifaciens on DSS-induced colitis, 24 mice were divided into three groups (n = 8): C group: free access to drinking water and food; M group: 2.5% DSS in a free drink for 7 days and daily oral administration of 0.1 ml saline for 10 days; TBA group: 2.5% DSS in a free drink for 7 days and daily oral administration of 0.1 ml 1 × 109 CFU B. acidifaciens for 10 days.
To determine whether the therapeutic effect of B. acidifaciens on colitis works through metabolites, we performed FMT or FFT on DSS-induced mice (Wu et al., 2020). Twenty-four mice were treated with an antibiotic cocktail [ABX; metronidazole (1 g/L), neomycin sulfate (1 g/L), ampicillin (0.5 g/L), vancomycin (0.5 g/L)] for 7 days before fecal bacteria transplantation and then divided into 4 groups: M-FMT group: 2.5% DSS for 7 days and transplanted with fecal supernatant of the M group for 10 days; M-FFT group: 2.5% DSS for 7 days and transplanted with fecal supernatant filtrate of the M group for 10 days; BA-FMT group: 2.5% DSS for 7 days and transplanted with fecal supernatant of the B. acidifaciens treatment group for 10 days; BA-FFT group: 2.5% DSS for 7 days and transplanted with fecal supernatant filtrate of the B. acidifaciens treatment group for 10 days. After 7 days of ABX administration, 24 mouse fecal samples were anaerobic cultured on a Brain Heart Infusion Broth (BHI; Cat# HB8297-1; Qingdao Hope Bio-Technology, Co., Ltd., China) agar plate for 96 h to ensure the depletion of fecal microbiota. The feces of mice were collected and diluted with PBS (1:10), thoroughly homogenized, centrifuged at 500 × g for 1 min and collected the supernatant for FMT. The supernatant was filtered through a 0.22 μm filter membrane, and the filtrate was collected for FFT.
To study the therapeutic effect of EVs of B. acidifaciens on DSS-induced colitis, 24 mice were divided into 3 groups (n = 8): C group: free access to drinking water and food; M group: 2.5% DSS in a free drink for 7 days and daily oral administration of 0.1 ml saline for 10 days; TBA group: 2.5% DSS in a free drink for 7 days and daily oral administration of 0.1 ml EVs (50 μg/day) for 10 days. The weight of the mice was measured daily throughout the study. The disease activity index (DAI) evaluated the severity of colitis through weight loss, diarrhea of the stool, and the extent of blood in the feces (Ma et al., 2022).
To evaluate the morphological changes in colon tissue in mice, the sample was fixed with 4% paraformaldehyde, embedded in paraffin, cut into 5–6 μm thick sections, rehydrated with xylene and degraded ethanol for 3–5 min, washed with PBS, and then stained with hematoxylin-eosin (H&E). To evaluate the number of goblet cells in the colon, we also stained fixed colon tissue sections with Alcian blue/periodic acid-Schiff staining (AB/PAS). To assess the expression of the colon barrier proteins ZO-1 (Cat# 21773-1-AP; Proteintech Group, Inc.; 1:100, China) and Occludin (Cat# 27260-1-AP; Proteintech Group, Inc.; 1:100, China), the tissue sections were stained with immunofluorescence. To assess the apoptosis of colon tissue cells in mice, fixed colon sections were stained with a terminal deoxynucleotidyl transferase-mediated dUTP nick end labeling (TUNEL) detection kit (Cat# T2196; Solarbio, Inc., China). Finally, the images were visualized by means of microscopy or fluorescence microscopy (Wu Z. et al., 2021).
To evaluate inflammation and oxidative stress in the serum of mice, the levels of the proinflammatory factors interleukin (IL)-1β (Cat# 2M-KMLJM211201m mouse; Camilo Biological, Nanjing, China), IL-6 (Cat# 2M-KMLJM219451m mouse; Camilo Biological, Nanjing, China), and tumor necrosis factor (TNF)-α (Cat# 2M-KMLJM220051m mouse; Camilo Biological, Nanjing, China) were determined according to the manufacturer’s protocol.
Mice colon tissue sample proteins were extracted using cell lysis buffer (Cat# R0020; Solarbio, Inc., China) supplemented with phenylmethanesulfonyl fluoride (PMSF; Cat# P0100; Solarbio, Inc., China) and protease inhibitor cocktail (Cat# HY-K0022; Med Chem Express, Inc., China). A bicinchoninic acid protein assay kit (BCA; Cat# PC0020; Solarbio, Inc., China) was used to determine the protein concentration of the samples. Then, the proteins were detached with 8–10% polyacrylamide resolving gels, transferred to polyvinylidene fluoride membranes (PVDF), and blocked with 5% skim milk for 90 min at RT (Xiao et al., 2023). Then, the membranes were coincubated with the following primary antibodies overnight at 4°C: rabbit anti-Zona occludens protein 1 (ZO-1; Cat# 21773-1-AP; Proteintech Group, Inc.; 1:5,000, China); rabbit anti-Occludin (Cat# 27260-1-AP; Proteintech Group, Inc.; 1:2,000, China); and mouse anti-glyceraldehyde-3-phosphate dehydrogenase (GAPDH; Cat# 60004-1-Ig; Proteintech Group, Inc.; 1:5,000, China). After primary incubation, the membrane was washed with Tris-buffered-saline-Tween-20 (TBST) buffer three times for 10 min each and then incubated with horseradish peroxidase (HRP)-conjugated goat anti-rabbit secondary antibody (Cat#SA00001-2; Proteintech Group; 1: 5,000, China) or goat anti-mouse secondary antibody (Cat#SA00001-1; Proteintech Group; 1: 5,000, China) for 60 min at RT. Finally, the proteins were visualized using a super enhanced chemiluminescence reagent (ECL).
For microbial DNA extraction, mouse feces samples were collected. Bacterial DNA was extracted from feces by the magnetic bead method combined with a genomic DNA kit (Cat# DP712-02; Tiangen Biotech Co., Ltd., China), and the purity and concentration of purified DNA were detected by spectrophotometry at 230 nm (A 230) and 260 nm (A 260). Then, the V4 region of the 16S ribosomal (r)RNA genes was amplified via primers (5’-AYTGGGYDTAAAGNG R-3’; 5’- TACNVGGGTATCTAATCC-3’) in the sample, and the PCR products were sequenced with an Illumina HiSeq 2000 platform (Illumina, Inc., San Diego, CA, USA) (GenBank accession number PRJNA1009232) (Zheng et al., 2021).
Three biological replicate samples of B. acidifaciens (BA1, BA2, and BA3) and B. acidifaciens-derived EVs (EV1, EV2, and EV3) were obtained, and the 4D-label-free quantitative proteomic analysis was performed. The protein extraction of BA and EV was as previously described and quantified using the BCA protein assay kit. Protein digestion by trypsin was performed according to filter-aided sample preparation procedure described by Matthias Mann. LC-MS/MS analysis was performed on a timsTOF Pro mass that was coupled to Nanoelute (Bruker Daltonics). The MS raw data for each sample were combined and searched using the MaxQuant 1.5.3.17 software for identification and quantitation analysis (Chen et al., 2022; Zheng et al., 2023).
The data are represented by the mean standard deviation (SD). GraphPad Prism 9.0 (San Diego, CA, USA) was used for statistical analyses. And statistical analyses of two groups was performed by unpaired two-tailed Student’s t-test, multiple groups were performed by one-way ANOVA followed by Tukey’s multiple comparison test. The adjusted P < 0.05 was considered statistically significant (Zheng et al., 2021).
To observe the relieving effect of B. acidifaciens on colitis in mice, we used 2.5% DSS to induce colitis in mice for 7 days and treated them with B. acidifaciens at the same time for 10 days (Figure 1A). The results showed that compared with the M group, B. acidifaciens significantly alleviated weight loss, reduced the DAI and reversed the shortening of the colon (Figures 1B–D). Histological analysis revealed that inflammatory cells infiltrated the colon, intestinal villi were shortened and thickened, and mucosa was damaged in response to DSS treatment, while the administration of B. acidifaciens alleviated the colonic inflammatory reaction (Figure 1E). To further assess the effect of B. acidifaciens on the systemic inflammatory response, we detected proinflammatory cytokines in the serum of mice. The serum concentrations of IL-1β, IL-6 and TNF-α in B. acidifaciens-treated mice were significantly decreased (Figures 1F–H). To determine the protective effect of B. acidifaciens on the intestinal barrier, we further detected the expression of barrier proteins by western blot and immunofluorescence staining and detected mucin-secreting goblet cells in the colonic epithelium by AB-PAS staining. The results showed that oral administration of B. acidifaciens significantly reversed the decrease in barrier proteins caused by DSS (Figures 1I, J) and maintained better mucus distribution (Figure 1K). Moreover, TUNEL analysis further revealed that DSS significantly increased the frequency of apoptotic cells in the colonic mucosa, but B. acidifaciens largely reversed this trend (Figure 1L).
Figure 1. Bacteroides acidifaciens alleviated DSS-induced colitis in mice. (A) Experimental design. (B) Mouse body weight. (C) DAI score. (D) Colon length. (E) H&E staining and pathological score of colon sections. (F–H) Serum IL-1β, IL-6 and TNF-α levels. (I) The expression of tight junction proteins (ZO-1, Occludin) in mouse colon tissue. (J) Immunofluorescence was used to evaluate the expression of tight junction proteins (ZO-1, Occludin) in colonic sections. (K) Representative images of the Alcian blue-stained inner mucus layer of colonic sections. (L) Representative fluorescent pictures of TUNEL staining of colonic sections. C, control group; M, DSS group; TBA, DSS mice orally administered B. acidifaciens group. C, control group; M, DSS group; TBA, DSS mice orally administered B. acidifaciens group. Scale bar, 100 μm. Data are presented as the mean ± SD. *P < 0.05 versus the C group, # P < 0.05 versus the M group. ns, P > 0.05; */# P < 0.05; **/## P < 0.01; ***/### P < 0.001.
Next, we further explored the influence of B. acidifaciens on the composition of gut microbiota in DSS-treated mice via 16S rRNA gene sequencing. The α-diversity analysis showed that DSS treatment significantly reduced the diversity of the gut microbiota in mice, and the administration of B. acidifaciens promoted its gradual recovery (Figures 2A–C). Principal coordinate analysis (PCoA) confirmed that DSS caused the gut microbiota to be located far away from the C group, while B. acidifaciens caused the TBA and C groups to be close to each other (Figure 2D). In addition, the Venn diagram showed (Figure 2E) that there were 1,399, 801, and 1,102 OTUs in the C, M and TBA groups, and the percentages of common OTUs in each group were 19.30% (270/1,399), 33.71% (270/801) and 24.50% (270/1,102), respectively.
Figure 2. Bacteroides acidifaciens altered gut microbiota diversity in DSS-induced colitis mice. (A) Shannon index. (B) Chao 1 index. (C) Observed species. (D) PCoA of the β diversity index. (E) Venn diagram. (F–H) Relative abundance of the identified fecal microbiota at the (F) phylum, (G) genus, and (H) species levels as per 16S rRNA gene sequencing. (I) Bacteroidetes. (J) Firmicutes. (K) Proteobacteria. (L) Actinobacteria. (M) Bacteroides. (N) Paraprevotella. (O) Parabacteroides. (P) Prevotella. (Q) Streptococcus. (R) B. acidifaciens. (S) Bacteroides fragilis. (T) Ruminococcus callidus. C, control group; M, DSS group; TBA, DSS mice orally administered B. acidifaciens group. Data are presented as the mean ± SD. n = 7. *P < 0.05 versus the C group. ns, P > 0.05; *P < 0.05; **P < 0.01; ***P < 0.001.
Next, we further explored the gut microbiota composition and intestinal disease-related bacteria at the phylum, genus and species levels. At the phylum level, Bacteroidetes, Firmicutes, Proteobacteria, and Actinobacteria were predominant phyla in the fecal microbiota (Figures 2F, I–L). Moreover, the results showed that colitis mice showed a low relative abundance of Bacteroides, and the administration of B. acidifaciens reversed the relative abundance of Bacteroides (Figure 2I). At the genus level, the fecal microbiota was dominated by Bacteroides, Paraprevotella, and Parabacteroides (Figure 2G). Further analysis showed that the administration of B. acidifaciens significantly increased the relative abundance of Bacteroides, Paraprevotella, and Prevotella while decreasing the relative abundance of Parabacteroides and Streptococcus (Figures 2M–Q). The results of species level analysis showed that the administration of B. acidifaciens sharply increased the relative abundance of B. acidifaciens and Ruminococcus callidus but slightly decreased the relative abundance of Bacteroides fragilis (Figures 2H, R–T). However, there was no significant difference in the relative abundance of B. fragilis between the TBA group and the other two groups.
We further performed FMT and FFT to determine the role of gut microbiota and bacterial metabolites in the remission of colitis mediated by B. acidifaciens (Figure 3A). The results showed that compared with the FMT or FFT of the M group mice, the FMT and FFT of the donor mice treated with B. acidifaciens reduced the weight loss and DAI score and shortened the colon length (Figures 3B–D). Histological analysis showed that FMT or FFT of B. acidifaciens-treated donor mice significantly alleviated the infiltration of inflammatory cells into the colon, shortened and thickened villi, and damaged mucosa, thus reducing the histological score (Figure 3E). In addition, the FMT of B. acidifaciens-treated donor mice was helpful to reduce the level of serum inflammation, which was proven by the significantly reduced levels of IL-1β, IL-6 and TNF-α (Figures 3F–H). Furthermore, FMT and FFT of B. acidifaciens-treated donor mice significantly increased the expression of the barrier proteins ZO-1 and Occludin (Figures 3I, J) and maintained the distribution of intestinal mucus (Figure 3K). TUNEL analysis further revealed that FMT or FFT of B. acidifaciens-treated donor mice significantly reduced cell apoptosis in colon mucosa (Figure 3L). Collectively, B. acidifaciens-changed gut microbiota and metabolites were able to alleviate DSS-induced colitis.
Figure 3. Bacteroides acidifaciens changed the gut microbiota, and metabolites contributed to alleviating colitis. (A) Experimental design. (B) Mouse body weight. (C) DAI score. (D) Colon length. (E) H&E staining and pathological score of colon sections. (F–H) Serum IL-1β, IL-6 and TNF-α levels. (I) The expression of tight junction proteins (ZO-1, Occludin) in mouse colon tissue. (J) Immunofluorescence representative images of tight junction proteins (ZO-1, Occludin) in colonic sections. (K) Representative images of the Alcian blue-stained inner mucus layer of colonic sections. (L) Representative fluorescent pictures of TUNEL staining of colonic sections. M-FMT: transplanted with fecal supernatant of the M group; M-FFT: transplanted with fecal supernatant filtrate of the M group; BA-FMT: transplanted with fecal supernatant of the TBA group; BA-FFT: transplanted with fecal supernatant filtrate of the TBA group. Scale bar, 100 μm. Data are presented as the mean ± SD. *P < 0.05 versus the M-FMT group, # P < 0.05 versus the M-FFT group. ns, P > 0.05; */# P < 0.05; **P < 0.01.
Furthermore, we isolated and purified bacterial EVs to explore whether the EVs of B. acidifaciens alleviated DSS-induced colitis (Figure 4A). Transmission electron microscopy of EVs showed saucer-like vesicle morphology (Figure 4B). The NTA results showed that the diameter of the EVs was approximately 129 nm (Figure 4C). Furthermore, we explored whether B. acidifaciens EVs had a therapeutic effect on DSS-induced colitis. Compared with the M group, the administration of B. acidifaciens or its EVs resulted in lower body weight loss, DAI score, and shortened colon length (Figures 4D–F). In addition, we observed that the infiltration of inflammatory cells, tissue damage and histological score in the colon decreased after the administration of B. acidifaciens EVs (Figures 4G, H). Moreover, the levels of IL-1β, IL-6, and TNF-a were significantly reduced in the TBA and TEV groups (Figures 4I–K). Consistently, western blot and immunofluorescence analysis of ZO-1 and Occludin showed that B. acidifaciens or EV treatment weakened the epithelial tight junction protein barrier damaged by DSS (Figures 4L, M). The administration of B. acidifaciens or EVs also significantly enhanced the integrity of the mucus barrier, showing more mucin-positive coverage (Figure 4N). TUNEL analysis revealed that B. acidifaciens or EVs significantly reduced cell apoptosis in the colonic mucosa (Figure 4O). Notably, compared with the treatment of B. acidifaciens, EV administration can significantly alleviate the symptoms of acute colitis.
Figure 4. Bacteroides acidifaciens-EVs had a relieving effect on DSS-induced colitis. (A) Experimental design. (B) Observation of B. acidifaciens-EV morphology by means of TEM. Scale bar, 50 nm. (C) Observation of B. acidifaciens-EV size distribution analyzed by NTA. (D) Mouse body weight. (E) DAI score. (F) Colon length. (G,H) H&E staining and pathological score of colon sections. Scale bar, 100 μm. (I–K) Serum IL-1β, IL-6 and TNF-α levels. (L) The expression of tight junction proteins (ZO-1, Occludin) in mouse colon tissue. (M) Immunofluorescence representative images of tight junction proteins (ZO-1, Occludin) in colonic sections. (N) Representative images of the Alcian blue-stained inner mucus layer of colonic sections. (O) Representative fluorescent pictures of TUNEL staining of colonic sections. C, control group; M, DSS group; TBA, DSS mice orally administered B. acidifaciens group; TEV, DSS mice orally administered B. acidifaciens-EVs group. Data are presented as the mean ± SD. *P < 0.05 versus the C group, # P < 0.05 versus the M group. ns, P > 0.05; */# P < 0.05; **/## P < 0.01; ***/### P < 0.001.
Proteins in B. acidifaciens EVs or B. acidifaciens were identified and quantified using 4D- label-free proteomic analysis. A total of 1,990 proteins were identified in B. acidifaciens-EVs and B. acidifaciens, of which 1,978 were quantified (Figure 5A). Venn diagram analysis showed that there were fewer unique differential proteins identified in EVs compared to B. acidifaciens (Figure 5B and Supplementary Table 1). Furthermore, we found that B. acidifaciens EVs resulted in 277 upregulated differentially expressed proteins (DEPs) and 788 downregulated DEPs (Figure 5C). As shown in Figure 5D, 61.02% of them were from cytoplasmic proteins, and 13.23% of them were annotated as outer membrane proteins. The subcellular localizations of 10.77% of the proteins were periplasmic, and the remaining proteins were from the inner membrane (8.30%) or extracellular (6.68%) (Figure 5D). To further identify biological alterations in B. acidifaciens EVs, the DEPs were classified into three categories based on gene ontology (GO) analysis: biological process (BP), molecular function (MF), cellular component (CC), and the top 20 terms were displayed in Figure 5E. The maximum proportion of DEPs was concentrated in bacterial biological processes, including cellular processes and cellular metabolic processes. Kyoto Encyclopedia of Genes and Genomes (KEGG) pathway enrichment analysis showed that the upregulated proteins in EVs compared with B. acidifaciens were involved in the biosynthesis of various antibiotics, apoptosis, and bacterial chemotaxis. Moreover, EVs downregulated proteins related to biosynthesis and metabolism, including lipopolysaccharide biosynthesis (Figure 5F). Thus, EVs underwent significant changes in protein composition compared to B. acidifaciens, and ultimately altering the biological functions.
Figure 5. Proteomic analysis of B. acidifaciens -EVs and B. acidifaciens. (A) Summary of tandem mass spectrometry (MS/MS) database search results for B. acidifaciens-EVs and B. acidifaciens. (B) Venn diagram. (C) Volcano plot showing the number of proteins differentially expressed between B. acidifaciens-EVs and B. acidifaciens, with a cutoff of P < 0.05 and | fold change| > 2. (D) Subcellular localization pie chart of differentially expressed proteins in B. acidifaciens-EVs and B. acidifaciens. (E) GO classification (biological process, molecular function, and cellular components) of all differentially expressed proteins in B. acidifaciens-EVs and the top 20 enriched GO terms. (F) KEGG pathway enrichment analysis of the differentially expressed proteins in B. acidifaciens-EVs and B. acidifaciens. BA, B. acidifaciens; EV, B. acidifaciens-EVs. n = 3.
In this study, we explored whether B. acidifaciens, a specific intestinal symbiotic bacterium, can alleviate the symptoms of DSS-induced colitis by reducing the inflammatory reaction and repairing the intestinal mucosal barrier. In addition, through fecal transplantation and fecal filtrate transplantation from B. acidifaciens-treated mice, we confirmed that the gut microbiota and metabolites regulated by B. acidifaciens may be the reason the symptoms of colitis are alleviated. Finally, we proved that B. acidifaciens EVs can also alleviate the symptoms of colitis by reducing inflammatory reactions and repairing the intestinal mucosal barrier (Figure 6).
A previous study reported that oral administration of 3% DSS for 5 days can reduce EVs from B. acidifaciens and Akkermansia muciniphila (Paredes-Sabja et al., 2013). Another study revealed that the protective effect of sulfated polysaccharides from Gracilaria lemaneiformis on DSS-induced colitis in mice was closely related to probiotics such as Alistipes and B. acidifaciens (Han et al., 2021). To explore whether oral administration of B. acidifaciens can alleviate colitis, acute colitis in mice was induced by 2.5% DSS and then treated with oral B. acidifaciens. Consistent with previous studies, we confirmed that the therapeutic application of oral B. acidifaciens can alleviate DSS-induced colitis, as shown by the DAI score, colon length and histological damage (Hu et al., 2020; Dong et al., 2022; Sharma et al., 2023). Similarly, oral B. acidifaciens also alleviated colonic inflammation, as exemplified by the decline in inflammatory cytokines such as IL-1β, IL-6 and TNF-α in the serum of DSS-treated mice (Hao et al., 2021; Zhao et al., 2021; Li X. et al., 2022). Furthermore, oral administration of B. acidifaciens alleviated DSS-induced damage to integrity and barrier function, as evidenced by the increased colonic mucus as well as the enhanced tight junctions of the colonic mucosa, in agreement with previous studies (Cui et al., 2021; Wu H. et al., 2021). Moreover, DSS-induced colitis can lead to apoptosis of colon tissue cells, while oral administration of B. acidifaciens reduced this phenomenon (Qu et al., 2017; Li et al., 2019; Kim et al., 2023).
Furthermore, we investigated the differences between the feces of mice orally administered B. acidifaciens (TBA) and the other two groups (C and M). The results of α-diversity analysis showed that the DSS-induced group significantly reduced the microbial diversity of mouse feces, while supplementation with B. acidifaciens reversed this result (Zhang et al., 2019; Huang et al., 2022). PCoA revealed differences in the microbial community structure among the C, M and TBA groups, with the microbial community structure of the TBA group gradually approaching that of the C group. Further analysis of the gut microbiota composition of mice treated with B. acidifaciens showed a significant increase in the abundance of Bacteroides, Paraprevotella, and Prevotella. A previous study revealed a decrease in the metabolites of Bacteroides and SCFAs in IBD patients (Deleu et al., 2021; Guo et al., 2021; Zhang et al., 2022). The Paraprevotella genus has been proven to have anti-inflammatory effects (Renson et al., 2020). Lachnospira and Paraprevotella are symbiotic bacteria that may produce short-chain fatty acids (such as succinic acid or acetic acid) in the gastrointestinal tract (Hattori et al., 2020). In addition, Paraprevotella can accumulate at the healing site of the intestinal mucosa (Feng et al., 2021). Another study reported that the establishment of a UC model can reduce the proportion of Prevotella species, while using drugs to alleviate enteritis can restore their relative abundance (Liu et al., 2020). Specific species of the Prevotella genus can successfully inhibit inflammatory demyelinating disease and multiple sclerosis by inducing Tregs and regulating cytokines (Mangalam et al., 2017; Shahi et al., 2019). Moreover, supplementation with B. acidifaciens also reduced the relative abundance of Parabacteroides and Streptococcus (Li Q. et al., 2022; Wu et al., 2023). Fabio Cominelli et al. reported that Parabacteroides distasonis opportunistically colonizes the gut niche of susceptible CD patients and exerts a depressive effect (Gomez-Nguyen et al., 2021). At the species level, supplementation with B. acidifaciens significantly reduced the relative abundance of B. fragilis. Toshifumi Hibi et al. revealed that B. fragilis was the main bacterial species in the feces of UC patients (Takaishi et al., 2008). In the present study, oral administration of B. acidifaciens significantly increased the relative abundance of Ruminococcus callidus, which was negatively correlated with intestinal symptoms, especially discomfort (Hiel et al., 2019).
Previous studies have reported that disease treatment can be achieved by transplanting functional microflora (FMT) from healthy human feces into the gastrointestinal tract of patients with ulcerative colitis and rebuilding a new gut microbiota (Moayyedi et al., 2015; Sokol et al., 2020). Similarly, transplanting fecal filtrate (FFT) from treatment group mice to enteritis mice can alleviate the colitis phenotype by eliminating ecological imbalances (Wu Z. et al., 2021; Ma et al., 2022). To verify the effects of microbiota and metabolites mediated by B. acidifaciens, we performed FMT and FFT. Consistent with the treatment with B. acidifaciens, BA-FMT was more effective than M-FMT in alleviating the phenotype of colitis in mice, reducing inflammatory reactions and repairing the intestinal mucosal barrier (Dong et al., 2021). In addition, the treatment of FFT in donors with B. acidifaciens also alleviated DSS-induced colitis (Wu Z. et al., 2021). We demonstrated that the gut microbiota and its metabolites induced by B. acidifaciens played a key role in alleviating DSS-induced experimental colitis.
Bacterial EVs are an important bridge for information exchange between gut microbiota and host cells (Toyofuku et al., 2019). EVs selectively carry various molecules (such as nucleic acids and proteins) from their bacteria, which may regulate host signaling pathways and various cellular processes (Toyofuku et al., 2019; Díaz-Garrido et al., 2021). To explore the interaction between B. acidifaciens and the host, we further investigated the role of EVs derived from B. acidifaciens in preventing colitis. As expected, B. acidifaciens and its derived EVs improved colitis symptoms, including colitis phenotype and histological scores (Hao et al., 2021; Tong et al., 2021). Consistent with previous studies, EVs treatment also reduced the expression of proinflammatory genes, such as IL-1β, IL-6, and TNF-α (Hao et al., 2021; Tong et al., 2021). Moreover, EVs enhance the epithelial barrier by regulating the expression of the TJ proteins Occludin and ZO-1, stabilizing the distribution of intestinal mucin proteins, and reducing intestinal tissue cell apoptosis (Kang et al., 2020). In summary, the above results emphasize the crucial role of probiotic-derived EVs in regulating DSS-induced colitis.
Compared to bacteria themselves, EVs derived from bacteria are rich in various functional proteins that can directly act on receptor cells and perform corresponding functions (Brown et al., 2015). Previous studies have shown that compared to Lactobacillus animalis, L. animalis EVs may be able to transfer some pro-angiogenic, pro-osteogenic, and anti-apoptotic proteins to endothelial and bone cells to exert bone protective effects (Chen et al., 2022). Another study reported that signal exchange between the microbiota and host bone can effectively alleviate osteoporosis by transferring Akkermansia muciniphila functional EVs from bacteria to bone cells (Liu J. H. et al., 2021). Therefore, we further utilized proteomics analysis to investigate the differential expression of extracellular vesicular proteins derived from B. acidifaciens. Bacteria have evolved unique ways of killing each other, so many of our most powerful antibiotics are derived from the bacteria themselves (Niehus et al., 2021). Our research findings suggested that B. acidifaciens-EVs have enriched biosynthesis of various antimicrobial-related proteins, which may be their potential mechanism for inhibiting the growth of other bacteria (Domínguez Rubio et al., 2022; Ma et al., 2023). Lipopolysaccharides are components of the outer wall of gram-negative bacterial cells and are endotoxins that can exert their toxic effects by acting on animal cells (Wang and Quinn, 2010). Additionally, we observed that B. acidifaciens-EVs also downregulated biosynthesis- and metabolism-related proteins, including lipopolysaccharide biosynthesis, which may be an indirect mechanism of the action of eosinophils on DSS-induced colitis, which still requires future exploration.
In summary, B. acidifaciens and its derived EVs can alleviate DSS-induced colitis by reducing mucosal damage to colon tissue, reducing inflammatory response, promoting mucosal barrier repair, restoring gut microbiota diversity, and restoring gut microbiota balance in mice. In this study, we clarified the effect of B. acidifaciens on DSS-induced colitis and explored its mechanism of action through its derived EVs. And through proteomics, it was found that there are differences in the proteomics of B. acidifaciens and its EVs. However, how EVs communicates with the host and participates in host regulation may be the key to the treatment of colitis with B. acidifaciens, and further exploration is needed. The results of this study provide a theoretical basis for the preclinical application of the new generation of probiotics.
The datasets presented in this study can be found in online repositories. The names of the repository/repositories and accession number(s) can be found below: NCBI–PRJNA1009232.
The animal study was approved by the Animal Experiment Ethics Committee of Nanchang University (NCULAE-202210311149). The study was conducted in accordance with the local legislation and institutional requirements.
CZ: Methodology, Visualization, Writing – original draft. YZ: Methodology, Software, Visualization, Writing – original draft. JX: Data curation, Methodology, Software, Writing – original draft. ZW: Methodology, Software, Writing – original draft. WenmZ: Methodology, Visualization, Writing – original draft. YP: Methodology, Writing – original draft. WenjZ: Visualization, Writing – original draft. LL: Methodology, Writing – original draft. JL: Conceptualization, Funding acquisition, Writing – original draft. WX: Conceptualization, Funding acquisition, Methodology, Writing – original draft, Writing – review & editing.
The authors declare financial support was received for the research, authorship, and/or publication of this article. This study was supported by the National Natural Science Foundation of China grant 81860435 (WX), The Second Affiliated Hospital of Nanchang University Funding Program 2022efyA01 (WX), Jiangxi Provincial Department of Science and Technology Project 20224ABC03A02 (JL), and The Second Affiliated Hospital of Nanchang University Funding Program 2022efyB03 (JL).
The authors declare that the research was conducted in the absence of any commercial or financial relationships that could be construed as a potential conflict of interest.
All claims expressed in this article are solely those of the authors and do not necessarily represent those of their affiliated organizations, or those of the publisher, the editors and the reviewers. Any product that may be evaluated in this article, or claim that may be made by its manufacturer, is not guaranteed or endorsed by the publisher.
The Supplementary Material for this article can be found online at: https://www.frontiersin.org/articles/10.3389/fmicb.2023.1304232/full#supplementary-material
Brown, L., Wolf, J. M., Prados-Rosales, R., and Casadevall, A. (2015). Through the wall: extracellular vesicles in Gram-positive bacteria, mycobacteria and fungi. Nat. Rev. Microbiol. 13, 620–630. doi: 10.1038/nrmicro3480
Chen, C. Y., Rao, S. S., Yue, T., Tan, Y. J., Yin, H., Chen, L. J., et al. (2022). Glucocorticoid-induced loss of beneficial gut bacterial extracellular vesicles is associated with the pathogenesis of osteonecrosis. Sci. Adv. 8:eabg8335. doi: 10.1126/sciadv.abg8335
Chronopoulos, A., and Kalluri, R. (2020). Emerging role of bacterial extracellular vesicles in cancer. Oncogene 39, 6951–6960. doi: 10.1038/s41388-020-01509-3
Cui, L., Guan, X., Ding, W., Luo, Y., Wang, W., Bu, W., et al. (2021). Scutellaria baicalensis Georgi polysaccharide ameliorates DSS-induced ulcerative colitis by improving intestinal barrier function and modulating gut microbiota. Int. J. Biol. Macromol. 166, 1035–1045. doi: 10.1016/j.ijbiomac.2020.10.259
Deleu, S., Machiels, K., Raes, J., Verbeke, K., and Vermeire, S. (2021). Short chain fatty acids and its producing organisms: an overlooked therapy for IBD? EBioMedicine 66:103293. doi: 10.1016/j.ebiom.2021.103293
Deng, J., Zhao, L., Yuan, X., Li, Y., Shi, J., Zhang, H., et al. (2022). Pre-administration of berberine exerts chemopreventive effects in AOM/DSS-induced colitis-associated carcinogenesis mice via modulating inflammation and intestinal microbiota. Nutrients 14, 726–747. doi: 10.3390/nu14040726
Díaz-Garrido, N., Badia, J., and Baldomà, L. (2021). Microbiota-derived extracellular vesicles in interkingdom communication in the gut. J. Extracell. Vesicles 10:e12161. doi: 10.1002/jev2.12161
Domínguez Rubio, A. P., D’Antoni, C. L., Piuri, M., and Pérez, O. E. (2022). Probiotics, their extracellular vesicles and infectious diseases. Front. Microbiol. 13:864720. doi: 10.3389/fmicb.2022.864720
Dong, F., Xiao, F., Li, X., Li, Y., Wang, X., Yu, G., et al. (2022). Pediococcus pentosaceus CECT 8330 protects DSS-induced colitis and regulates the intestinal microbiota and immune responses in mice. J. Transl. Med. 20, 33–49. doi: 10.1186/s12967-022-03235-8
Dong, S., Zhu, M., Wang, K., Zhao, X., Hu, L., Jing, W., et al. (2021). Dihydromyricetin improves DSS-induced colitis in mice via modulation of fecal-bacteria-related bile acid metabolism. Pharmacol. Res. 171:105767. doi: 10.1016/j.phrs.2021.105767
Eckburg, P. B., Bik, E. M., Bernstein, C. N., Purdom, E., Dethlefsen, L., Sargent, M., et al. (2005). Diversity of the human intestinal microbial flora. Science 308, 1635–1638. doi: 10.1126/science.1110591
Fedorak, R. N., Feagan, B. G., Hotte, N., Leddin, D., Dieleman, L. A., Petrunia, D. M., et al. (2015). The probiotic VSL#3 has anti-inflammatory effects and could reduce endoscopic recurrence after surgery for crohn’s disease. Clin. Gastroenterol. Hepatol. 13, 928–935. doi: 10.1016/j.cgh.2014.10.031
Feng, Z., Peng, S., Wu, Z., Jiao, L., Xu, S., Wu, Y., et al. (2021). Ramulus mori polysaccharide-loaded PLGA nanoparticles and their anti-inflammatory effects in vivo. Int. J. Biol. Macromol. 182, 2024–2036. doi: 10.1016/j.ijbiomac.2021.05.200
Franzosa, E. A., Sirota-Madi, A., Avila-Pacheco, J., Fornelos, N., Haiser, H. J., Reinker, S., et al. (2018). Gut microbiome structure and metabolic activity in inflammatory bowel disease. Nat. Microbiol. 4, 293–305. doi: 10.1038/s41564-018-0306-4
Gomez-Nguyen, A., Basson, A. R., Dark-Fleury, L., Hsu, K., Osme, A., Menghini, P., et al. (2021). Parabacteroides distasonis induces depressive-like behavior in a mouse model of Crohn’s disease. Brain Behav. Immun. 98, 245–250. doi: 10.1016/j.bbi.2021.08.218
Gul, L., Modos, D., Fonseca, S., Madgwick, M., Thomas, J. P., Sudhakar, P., et al. (2022). Extracellular vesicles produced by the human commensal gut bacterium Bacteroides thetaiotaomicron affect host immune pathways in a cell-type specific manner that are altered in inflammatory bowel disease. J. Extracell. Vesicles 11:e12189. doi: 10.1002/jev2.12189
Guo, C., Wang, Y., Zhang, S., Zhang, X., Du, Z., Li, M., et al. (2021). Crataegus pinnatifida polysaccharide alleviates colitis via modulation of gut microbiota and SCFAs metabolism. Int. J. Biol. Macromol. 181, 357–368. doi: 10.1016/j.ijbiomac.2021.03.137
Han, R., Ma, Y., Xiao, J., You, L., Pedisić, S., and Liao, L. (2021). The possible mechanism of the protective effect of a sulfated polysaccharide from Gracilaria Lemaneiformis against colitis induced by dextran sulfate sodium in mice. Food Chem. Toxicol. 149:112001. doi: 10.1016/j.fct.2021.112001
Hao, H., Zhang, X., Tong, L., Liu, Q., Liang, X., Bu, Y., et al. (2021). Effect of extracellular vesicles derived from Lactobacillus plantarum Q7 on gut microbiota and ulcerative colitis in mice. Front. Immunol. 12:777147. doi: 10.3389/fimmu.2021.777147
Hattori, S., Nakamura, M., Yamamura, T., Maeda, K., Sawada, T., Mizutani, Y., et al. (2020). The microbiome can predict mucosal healing in small intestine in patients with Crohn’s disease. J. Gastroenterol. 55, 1138–1149. doi: 10.1007/s00535-020-01728-1
Hiel, S., Bindels, L. B., Pachikian, B. D., Kalala, G., Broers, V., Zamariola, G., et al. (2019). Effects of a diet based on inulin-rich vegetables on gut health and nutritional behavior in healthy humans. Am. J. Clin. Nutr. 109, 1683–1695. doi: 10.1093/ajcn/nqz001
Hu, T., Wang, H., Xiang, C., Mu, J., and Zhao, X. (2020). Preventive effect of Lactobacillus acidophilus XY27 on DSS-induced ulcerative colitis in mice. Drug Design Dev. Ther. 14, 5645–5657. doi: 10.2147/dddt.S284422
Huang, S., Hu, S., Liu, S., Tang, B., Liu, Y., Tang, L., et al. (2022). Lithium carbonate alleviates colon inflammation through modulating gut microbiota and Treg cells in a GPR43-dependent manner. Pharmacol. Res. 175:105992. doi: 10.1016/j.phrs.2021.105992
Jakubczyk, D., Leszczynska, K., and Gorska, S. (2020). The effectiveness of probiotics in the treatment of inflammatory bowel disease (IBD)-a critical review. Nutrients 12:1973. doi: 10.3390/nu12071973
Kang, E. A., Choi, H.-I., Hong, S. W., Kang, S., Jegal, H.-Y., Choi, E. W., et al. (2020). Extracellular vesicles derived from kefir grain Lactobacillus ameliorate intestinal inflammation via regulation of proinflammatory pathway and tight junction integrity. Biomedicines 8, 522–533. doi: 10.3390/biomedicines8110522
Kaparakis-Liaskos, M., and Ferrero, R. L. (2015). Immune modulation by bacterial outer membrane vesicles. Nat. Rev. Immunol. 15, 375–387. doi: 10.1038/nri3837
Kim, K.-Y., Son, J. D., Hwang, S.-J., Lee, J. K., Park, J. Y., Park, K. I., et al. (2023). Fermented glutinous rice extract mitigates DSS-induced ulcerative colitis by alleviating intestinal barrier function and improving gut microbiota and inflammation. Antioxidants 12, 336–351. doi: 10.3390/antiox12020336
Kudelka, M. R., Stowell, S. R., Cummings, R. D., and Neish, A. S. (2020). Intestinal epithelial glycosylation in homeostasis and gut microbiota interactions in IBD. Nat. Rev. Gastroenterol. Hepatol. 17, 597–617. doi: 10.1038/s41575-020-0331-7
Li, G., Lin, J., Zhang, C., Gao, H., Lu, H., Gao, X., et al. (2021). Microbiota metabolite butyrate constrains neutrophil functions and ameliorates mucosal inflammation in inflammatory bowel disease. Gut Microbes 13:e1968257. doi: 10.1080/19490976.2021.1968257
Li, H., Shen, L., Lv, T., Wang, R., Zhang, N., Peng, H., et al. (2019). Salidroside attenuates dextran sulfate sodium-induced colitis in mice via SIRT1/FoxOs signaling pathway. Eur. J. Pharmacol. 861:172591. doi: 10.1016/j.ejphar.2019.172591
Li, Q., Wu, W., Fang, X., Chen, H., Han, Y., Liu, R., et al. (2022). Structural characterization of a polysaccharide from bamboo (Phyllostachys edulis) shoot and its prevention effect on colitis mouse. Food Chem. 387:132807. doi: 10.1016/j.foodchem.2022.132807
Li, X., Wu, X., Wang, Q., Xu, W., Zhao, Q., Xu, N., et al. (2022). Sanguinarine ameliorates DSS induced ulcerative colitis by inhibiting NLRP3 inflammasome activation and modulating intestinal microbiota in C57BL/6 mice. Phytomedicine 104:154321. doi: 10.1016/j.phymed.2022.154321
Liu, B., Piao, X., Niu, W., Zhang, Q., Ma, C., Wu, T., et al. (2020). Kuijieyuan decoction improved intestinal barrier injury of ulcerative colitis by affecting TLR4-dependent PI3K/AKT/NF-κB oxidative and inflammatory signaling and gut microbiota. Front. Pharmacol. 11:1036. doi: 10.3389/fphar.2020.01036
Liu, J. H., Chen, C. Y., Liu, Z. Z., Luo, Z. W., Rao, S. S., Jin, L., et al. (2021). Extracellular vesicles from child gut microbiota enter into bone to preserve bone mass and strength. Adv. Sci. (Weinh) 8:2004831. doi: 10.1002/advs.202004831
Liu, L., Liang, L., Yang, C., Zhou, Y., and Chen, Y. (2021). Extracellular vesicles of Fusobacterium nucleatum compromise intestinal barrier through targeting RIPK1-mediated cell death pathway. Gut Microbes 13:e1902718. doi: 10.1080/19490976.2021.1902718
Ma, L., Lyu, W., Song, Y., Chen, K., Lv, L., Yang, H., et al. (2023). Anti-inflammatory effect of Clostridium butyricum-derived extracellular vesicles in ulcerative colitis: impact on host microRNAs expressions and gut microbiome profiles. Mol. Nutr. Food Res. 67:e2200884. doi: 10.1002/mnfr.202200884
Ma, L., Shen, Q., Lyu, W., Lv, L., Wang, W., Yu, M., et al. (2022). Clostridium butyricum and its derived extracellular vesicles modulate gut homeostasis and ameliorate acute experimental colitis. Microbiol. Spectr. 10, 1–21. doi: 10.1128/spectrum.01368-22
Mangalam, A., Shahi, S. K., Luckey, D., Karau, M., Marietta, E., Luo, N., et al. (2017). Human gut-derived commensal bacteria suppress CNS inflammatory and demyelinating disease. Cell Rep. 20, 1269–1277. doi: 10.1016/j.celrep.2017.07.031
Moayyedi, P., Surette, M. G., Kim, P. T., Libertucci, J., Wolfe, M., Onischi, C., et al. (2015). Fecal microbiota transplantation induces remission in patients with active ulcerative colitis in a randomized controlled trial. Gastroenterology 149, 102–109. doi: 10.1053/j.gastro.2015.04.001
Molodecky, N. A., Soon, I. S., Rabi, D. M., Ghali, W. A., Ferris, M., Chernoff, G., et al. (2012). Increasing incidence and prevalence of the inflammatory bowel diseases with time, based on systematic review. Gastroenterology 142, 46–54. doi: 10.1053/j.gastro.2011.10.001
Momose, Y., Park, S. H., Miyamoto, Y., and Itoh, K. (2011). Design of species-specific oligonucleotide probes for the detection of Bacteroides and Parabacteroides by fluorescence in situ hybridization and their application to the analysis of mouse caecal Bacteroides-Parabacteroides microbiota. J. Appl. Microbiol. 111, 176–184. doi: 10.1111/j.1365-2672.2011.05039.x
Nakajima, A., Sasaki, T., Itoh, K., Kitahara, T., Takema, Y., Hiramatsu, K., et al. (2020). A soluble fiber diet increases Bacteroides fragilis group abundance and immunoglobulin a production in the gut. Appl. Environ. Microbiol. 86:e00405-20. doi: 10.1128/aem.00405-20
Niehus, R., Oliveira, N. M., Li, A., Fletcher, A. G., and Foster, K. R. (2021). The evolution of strategy in bacterial warfare via the regulation of bacteriocins and antibiotics. ELife 10:e69756. doi: 10.7554/eLife.69756
Paredes-Sabja, D., Kang, C.-S., Ban, M., Choi, E.-J., Moon, H.-G., Jeon, J.-S., et al. (2013). Extracellular vesicles derived from gut microbiota, especially Akkermansia muciniphila, protect the progression of dextran sulfate sodium-induced colitis. PLoS One 8:e76520. doi: 10.1371/journal.pone.0076520
Qu, C., Yuan, Z.-W., Yu, X.-T., Huang, Y.-F., Yang, G.-H., Chen, J.-N., et al. (2017). Patchouli alcohol ameliorates dextran sodium sulfate-induced experimental colitis and suppresses tryptophan catabolism. Pharmacol. Res. 121, 70–82. doi: 10.1016/j.phrs.2017.04.017
Renson, A., Mullan Harris, K., Dowd, J. B., Gaydosh, L., McQueen, M. B., Krauter, K. S., et al. (2020). Early signs of gut microbiome aging: biomarkers of inflammation, metabolism, and macromolecular damage in young adulthood. J. Gerontol. A Biol. Sci. Med. Sci. 75, 1258–1266. doi: 10.1093/gerona/glaa122
Rogler, G., Singh, A., Kavanaugh, A., and Rubin, D. T. (2021). Extraintestinal manifestations of inflammatory bowel disease: current concepts, treatment, and implications for disease management. Gastroenterology 161, 1118–1132. doi: 10.1053/j.gastro.2021.07.042
Santana, P. T., Rosas, S. L. B., Ribeiro, B. E., Marinho, Y., and de Souza, H. S. P. (2022). Dysbiosis in inflammatory bowel disease: pathogenic role and potential therapeutic targets. Int. J. Mol. Sci. 23, 3464–3489. doi: 10.3390/ijms23073464
Shahi, S. K., Freedman, S. N., Murra, A. C., Zarei, K., Sompallae, R., Gibson-Corley, K. N., et al. (2019). Prevotella histicola, a human gut commensal, is as potent as COPAXONE(R) in an animal model of multiple sclerosis. Front. Immunol. 10:462. doi: 10.3389/fimmu.2019.00462
Sharma, S., Bhatia, R., Devi, K., Rawat, A., Singh, S., Bhadada, S. K., et al. (2023). A synbiotic combination of Bifidobacterium longum Bif10 and Bifidobacterium breve Bif11, isomaltooligosaccharides and finger millet arabinoxylan prevents dextran sodium sulphate induced ulcerative colitis in mice. Int. J. Biol. Macromol. 231:123326. doi: 10.1016/j.ijbiomac.2023.123326
Sokol, H., Landman, C., Seksik, P., Berard, L., Montil, M., Nion-Larmurier, I., et al. (2020). Fecal microbiota transplantation to maintain remission in Crohn’s disease: a pilot randomized controlled study. Microbiome 8, 12–26. doi: 10.1186/s40168-020-0792-5
Takaishi, H., Matsuki, T., Nakazawa, A., Takada, T., Kado, S., Asahara, T., et al. (2008). Imbalance in intestinal microflora constitution could be involved in the pathogenesis of inflammatory bowel disease. Int. J. Med. Microbiol. 298, 463–472. doi: 10.1016/j.ijmm.2007.07.016
Tamaki, H., Shibatoge, M., and Nakase, H. (2015). Efficacy of probiotic treatment with Bifidobacterium longum 536 for induction of remission in active ulcerative colitis: a randomized, double-blinded, placebo-controlled multicenter trial. J. Crohns Colitis 9, S347–S347. doi: 10.1111/den.12553
Tong, L., Zhang, X., Hao, H., Liu, Q., Zhou, Z., Liang, X., et al. (2021). Lactobacillus rhamnosus GG derived extracellular vesicles modulate gut microbiota and attenuate inflammatory in DSS-induced colitis mice. Nutrients 13, 3319–3335. doi: 10.3390/nu13103319
Toyofuku, M., Nomura, N., and Eberl, L. (2019). Types and origins of bacterial membrane vesicles. Nat. Rev. Microbiol. 17, 13–24. doi: 10.1038/s41579-018-0112-2
Ullman, T. A., and Itzkowitz, S. H. (2011). Intestinal inflammation and cancer. Gastroenterology 140, 1807–1816.e1801. doi: 10.1053/j.gastro.2011.01.057
Wang, H., Wang, Q., Yang, C., Guo, M., Cui, X., Jing, Z., et al. (2022). Bacteroides acidifaciens in the gut plays a protective role against CD95-mediated liver injury. Gut Microbes 14:e2027853. doi: 10.1080/19490976.2022.2027853
Wang, X., and Quinn, P. J. (2010). Lipopolysaccharide: biosynthetic pathway and structure modification. Prog. Lipid Res. 49, 97–107. doi: 10.1016/j.plipres.2009.06.002
Wu, H., Chen, Q.-Y., Wang, W.-Z., Chu, S., Liu, X.-X., Liu, Y.-J., et al. (2021). Compound sophorae decoction enhances intestinal barrier function of dextran sodium sulfate induced colitis via regulating notch signaling pathway in mice. Biomed. Pharmacother. 133:110937. doi: 10.1016/j.biopha.2020.110937
Wu, J., Wei, Z., Cheng, P., Qian, C., Xu, F., Yang, Y., et al. (2020). Rhein modulates host purine metabolism in intestine through gut microbiota and ameliorates experimental colitis. Theranostics 10, 10665–10679. doi: 10.7150/thno.43528
Wu, Y., Ran, L., Yang, Y., Gao, X., Peng, M., Liu, S., et al. (2023). Deferasirox alleviates DSS-induced ulcerative colitis in mice by inhibiting ferroptosis and improving intestinal microbiota. Life Sci. 314:121312. doi: 10.1016/j.lfs.2022.121312
Wu, Z., Huang, S., Li, T., Li, N., Han, D., Zhang, B., et al. (2021). Gut microbiota from green tea polyphenol-dosed mice improves intestinal epithelial homeostasis and ameliorates experimental colitis. Microbiome 9, 184–206. doi: 10.1186/s40168-021-01115-9
Xiao, H., Wei, C., Liu, H., Li, Z., Zheng, C., and Luo, J. (2023). Lentinan alleviates sciatic nerve injury by promoting autophagy to remove myelin fragments. Phytother. Res. 2023, 1–17. doi: 10.1002/ptr.7862
Yan, S., Zhu, Y., Li, L., Qin, S., Yuan, J., Chang, X., et al. (2023). Alginate oligosaccharide ameliorates azithromycin-induced gut microbiota disorder via Bacteroides acidifaciens-FAHFAs and Bacteroides-TCA cycle axes. Food Funct. 14, 427–444. doi: 10.1039/d2fo02812c
Zhang, W., Cheng, C., Han, Q., Chen, Y., Guo, J., Wu, Q., et al. (2019). Flos Abelmoschus manihot extract attenuates DSS-induced colitis by regulating gut microbiota and Th17/Treg balance. Biomed. Pharmacother. 117:109162. doi: 10.1016/j.biopha.2019.109162
Zhang, Z., Zhang, H., Chen, T., Shi, L., Wang, D., and Tang, D. (2022). Regulatory role of short-chain fatty acids in inflammatory bowel disease. Cell Commun. Signal. 20, 64–74. doi: 10.1186/s12964-022-00869-5
Zhao, Y., Luan, H., Jiang, H., Xu, Y., Wu, X., Zhang, Y., et al. (2021). Gegen Qinlian decoction relieved DSS-induced ulcerative colitis in mice by modulating Th17/Treg cell homeostasis via suppressing IL-6/JAK2/STAT3 signaling. Phytomedicine 84:153519. doi: 10.1016/j.phymed.2021.153519
Zheng, C., Chen, T., Lu, J., Wei, K., Tian, H., Liu, W., et al. (2021). Adjuvant treatment and molecular mechanism of probiotic compounds in patients with gastric cancer after gastrectomy. Food Funct. 12, 6294–6308. doi: 10.1039/d1fo01375k
Keywords: inflammatory bowel disease, Bacteroides acidifaciens, extracellular vesicles, gut microbiota, intestinal mucosal barrier
Citation: Zheng C, Zhong Y, Xie J, Wang Z, Zhang W, Pi Y, Zhang W, Liu L, Luo J and Xu W (2023) Bacteroides acidifaciens and its derived extracellular vesicles improve DSS-induced colitis. Front. Microbiol. 14:1304232. doi: 10.3389/fmicb.2023.1304232
Received: 29 September 2023; Accepted: 13 November 2023;
Published: 30 November 2023.
Edited by:
Tetyana Falalyeyeva, Taras Shevchenko National University of Kyiv, UkraineReviewed by:
Yukiko Miyamoto, University of California, San Diego, United StatesCopyright © 2023 Zheng, Zhong, Xie, Wang, Zhang, Pi, Zhang, Liu, Luo and Xu. This is an open-access article distributed under the terms of the Creative Commons Attribution License (CC BY). The use, distribution or reproduction in other forums is permitted, provided the original author(s) and the copyright owner(s) are credited and that the original publication in this journal is cited, in accordance with accepted academic practice. No use, distribution or reproduction is permitted which does not comply with these terms.
*Correspondence: Wei Xu, eHVfd2VpMTExQDEyNi5jb20=
†These authors have contributed equally to this work
Disclaimer: All claims expressed in this article are solely those of the authors and do not necessarily represent those of their affiliated organizations, or those of the publisher, the editors and the reviewers. Any product that may be evaluated in this article or claim that may be made by its manufacturer is not guaranteed or endorsed by the publisher.
Research integrity at Frontiers
Learn more about the work of our research integrity team to safeguard the quality of each article we publish.