- 1National Key Laboratory of Intelligent Tracking and Forecasting for Infectious Diseases, National Institute for Communicable Disease Control and Prevention, Chinese Center for Disease Control and Prevention, Beijing, China
- 2Institute of Health Inspection and Testing, Hubei Provincial Center for Disease Control and Prevention, Wuhan, Hubei, China
- 3Huanggang Municipal Center for Disease Control and Prevention, Huanggang, Hubei, China
In this study, a total of 179 ticks infesting ruminant livestock, including 166 Haemaphysalis longicornis ticks and 13 Rhipicephalus microplus ticks were collected from Yingshan county of Hubei province, China in 2021–2022. PCR testing and sequence analysis revealed that the ticks infected with various species of pathogens including Rickettsia (R. japonica), Anaplasma (A. bovis, A. ovis, A. platys, and Ca. A. boleense), Ehrlichia (E. minasensis and Ehrlichia sp.), Theileria (T. orientalis and T. luwenshuni), and Babesia (B. bigemina). The infection rates of these pathogens were 0.56, 16.76, 7.26, 2.79 and 0.56%. respectively, while only 3 of 13 R. microplus ticks were detected to be infected wth Ehrlichia sp., A. bove., or T. luwenshuni. Our results revealed that a variety of tick-borne pathogens highly carried by these ticks, specially Ha. longicornis. Therefore, it is necessary to make effective control of the ticks and the tick-borne diseases in the County.
1 Introduction
Ticks are hematophagous ectoparasites that feed on terrestrial vertebrates (Kim, 2022). They have a global distribution and are known to be the second most important arthropod vectors of human pathogens after mosquitoes (Mediannikov and Fenollar, 2014; Mansfield et al., 2017; Madison-Antenucci et al., 2020). More than 120 tick species have been found in China. Ha. longicornis is by far the most widely distributed and influential species in the world, exposing over 40% of the nation’s population in various counties. This has enormous implications for public health, given that Ha. longicornis harbors 44 tick-borne pathogens and is a competent vector for severe fever with thrombocytopenia syndrome bunyavirus, which has been associated with a case fatality ratio of 12–50% (Li et al., 2019; El-Alfy et al., 2022). The other tick species that follow in terms of importance are Ixodes persulcatus, Dermacentor nutalli, and Rhipicephalus microplus (R. microplus) (Zhao et al., 2021). Ticks can transmit a wide variety of pathogens to both humans and animals, resulting in significant public health and economic impacts (de la Fuente et al., 2008).
Over the past 30 years, the diversity of tick-borne pathogens have been identified, including viruses, bacteria (especially Rickettsia and Borrelia spp.), protozoa, and helminths. In the mainland of China, 34 tick-borne pathogens have been identified, including eight spotted fever group rickettsiae (SFGR) (R. heilongjiangiensis (He et al., 2023), R. japonica (Li et al., 2019), R. sibirica subsp. mongolotimonae, R. monacensis, R. raoultii, R. slovaca (Tian et al., 2012), Candidatus Rickettsia hebeiii, and Candidatus Rickettsia tarasevichiae [Jia et al., 2013)], four species of genus Ehrlichia [E. chaffeensis (Cao et al., 2000), E. canis (Zhai et al., 2021), Candidatus E. erythraense (Lu et al., 2023) and E. sp. Tibet (Wen et al., 2003)], three species of genus Anaplasma [A. platys, A. capra, and A. phagocytophilum (Li et al., 2015)]; one species of genus Neoehrlichia (Candidatus N. Mikurensis). six species of genus Borrelia, eleven species of genus Babesia, and a severe systemic thrombocytopenia syndrome virus (SFTSV) (Tokarz and Lipkin, 2021). With social change and urbanization, humans, animals, and ticks are increasingly sharing habitats, leading to a higher likelihood of human exposure to ticks (Matos et al., 2022; Jin et al., 2023). These emerging tick-borne infections pose an increasing public health threat in China (Li et al., 2020; el-Alfy et al., 2023).
Yingshan County in Hubei Province is located within the Dabie Mountain Range. The county is mainly characterized by mountainous and forested topography, with a warm, humid climate, abundant rainfall, and distinct seasons, which are often accompanied by natural disasters. This type of climate and terrain provides suitable living and breeding conditions for ticks, increasing the risk of tick-borne diseases. Furthermore, our team previously identified a novel tick-borne pathogen in the Dabie Mountains area of China. Therefore, this study aims to investigate the epidemiology and genetic diversity of bacterial and protozoan pathogens carried by the ticks parasitizing ruminant livestock in Yingshan County, Hubei Province.
2 Materials and methods
2.1 Tick collection and identification
During 2021–2022, this study collected adult ticks in yingshan county (E 115.679, N 30.735) of Hubei Province, China. The ticks infesting ruminant livestock (cattle and goats) were collected. All ticks were morphologically identified according to previous report (Azmat et al., 2018), their species were confirmed by COI gene sequencing assay (Chen et al., 2010).
2.2 DNA extraction
All ticks were initially washed for 15 min each in a sequential manner using 5% bromogeramine solution, 75% alcohol, and PBS (Lu et al., 2023). After air-drying, they were placed in 2 mL centrifuge tubes with steel beads and 200 μL Sucrose-Phosphate-Glutamate (SPG) solution was added. Then, they were homogenized individually using a grinder (Retsch, Germany). Genomic DNA was extracted from each sample using the QIAamp DNA Mini Kit (Qiagen, Hilden, Germany) according to the manufacturer’s instructions and eluted in a final volume of 100 μL (Wen et al., 2003). All DNA samples were stored at −20°C.
2.3 Detection pathogens in ticks
The bacterial pathogens, including Rickettsia spp., Anaplasma spp., Ehrlichia spp., Bartonella spp., Borrelia spp., and Piroplasms. were screened by real-time PCR (qPCR) assays with corresponding primers specific for each pathogen. A Ct cut off value of 35 was used for determining positive samples, which were subsequently subjected to semi-nested or nested PCR specific to each pathogen, the specific primers and amplified fragment sizes are presented in Supplementary Table S1.
For Rickettsia spp., amplification targeted the 16S rRNA (1,200 bp), ompA (500 bp) gltA (900 bp) and groEL gene (1,100 bp). For Anaplasma spp. and Ehrlichia spp., a semi-nested PCR assay targeting a 500 bp region of the 16S rRNA gene was carried out for preliminary typing, followed by genus-specific or species-specific primers targeting 16S rRNA, gltA, or groEL gene for final confirmation. To detect and characterize tick-borne protozoan pathogens, we employed a semi-nested PCR approach using a universal primer set targeting the 18S rRNA gene of Piroplasms (1,400 bp). The corresponding primers for amplification are listed in Supplementary Table S1.
The amplification products were verified through 1.0% agarose gel electrophoresis, and the size of DNA fragments was determined by comparing them with standard molecular size DNA ladders. PCR products with clear target DNA bands were sent to Tianyihuiyuan Biotechnology Company (Beijing, China) for sequencing.
2.4 Phylogenetic data analysis
SeqMan software (DNASTAR, Madison, WI) was utilized to edit and assemble DNA sequences obtained through sequencing, with a specific focus on assembling the 16S rRNA gene from Rickettsia spp., Anaplasma spp., and Ehrlichia spp. by merging two overlapping segments to obtain near full-length gene sequences. The resulting sequences were analyzed and compared with all available sequences in GenBank using the Basic Local Alignment Search Tool (BLAST). A neighbor-joining method was implemented in MEGA 7.0 software to construct a comprehensive phylogenetic tree, and the stability of the tree topology was evaluated by computing bootstrap support values from 1,000 replicates.
3 Results
3.1 Tick sampling and identification
During the period of 2021–2022, a total of 179 ticks were collected from Yingshan County of Hubei province, China (Figure 1). Through meticulous morphological examination and COI sequence analysis, 166 ticks were identified as Rhipicephalus microplus (R. microplus, 92.73%, 166/179) and 13 ticks were identified as Haemaphysalis longicornis (Ha. Longicornis, 7.26%, 13/179). The phylogenetic tree constructed on the basis of COI gene sequences is shown in Figure 2; the COI gene sequences generated in this study were clustered with their respective homologs in two main groups corresponding to two species.
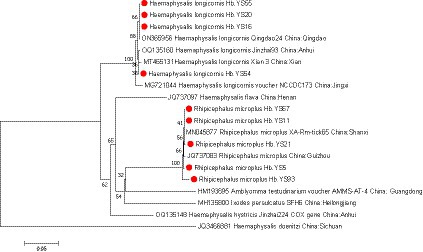
Figure 2. phylogenetic analysis of ticks was conducted based on the nucleotide sequences of COI gene. The resulting tree shows the relationship of Ha. longicornis and R. microplus with other tick species. Sequences obtained in this study are marked with a red dot before their names.
3.2 Pathogen diversity detected in ticks
Various bacterial pathogens, including Rickettsia spp., Anaplasma spp., Ehrlichia spp., and Piroplasms, were detected in ticks through PCR assays, while Borrelia spp. and Bartonella spp. was not detected (Table 1).
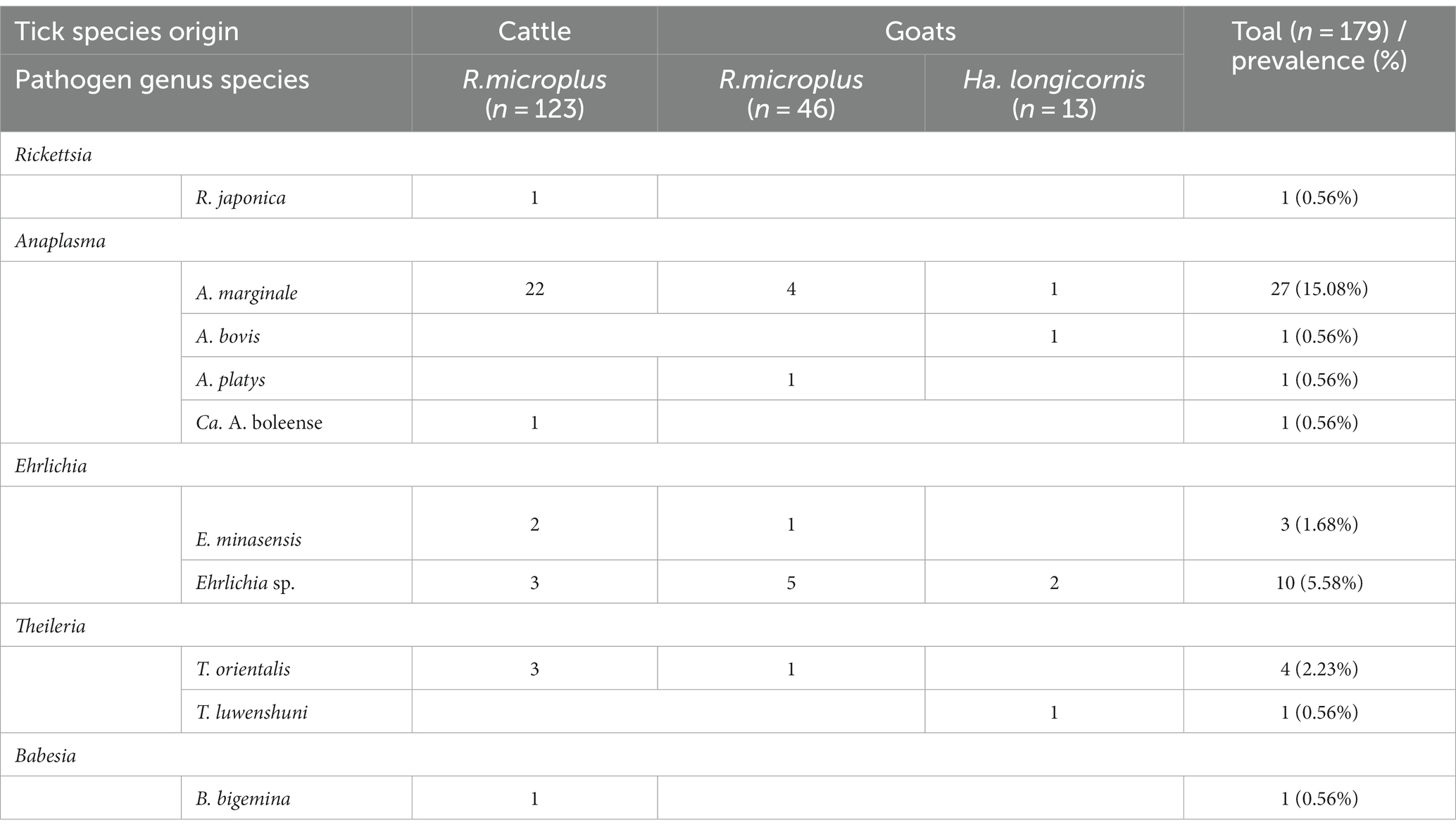
Table 1. Prevalence of tick-borne pathogens in the two ticks collected from Yingshan County of Hubei, China.
A total of four R. microplus ticks were found to carry two or three tick-borne pathogens, accounting for 2.23% of the total. Among them, two ticks (1.12%) were co-infected with A. marginale and Ehrlichia sp.; one tick (0.56%) was co-infected with Ehrlichia sp. and T. orientalis; and one tick (0.56%) carried three different pathogens, namely A. marginale, Ehrlichia sp., and T. orientalis.
The PCR results showed a low infection rate of Rickettsia spp. in the ticks, with only one R. microplus tick positive for R. japonica. The sequences of 16S rRNA, ompA, gltA, and groEL genes obtained from the tick were 99.84 to 100% similar to those of R. japonica strains and 100% identical with that of R. japonica YHM (AP017602) (Figure 3).
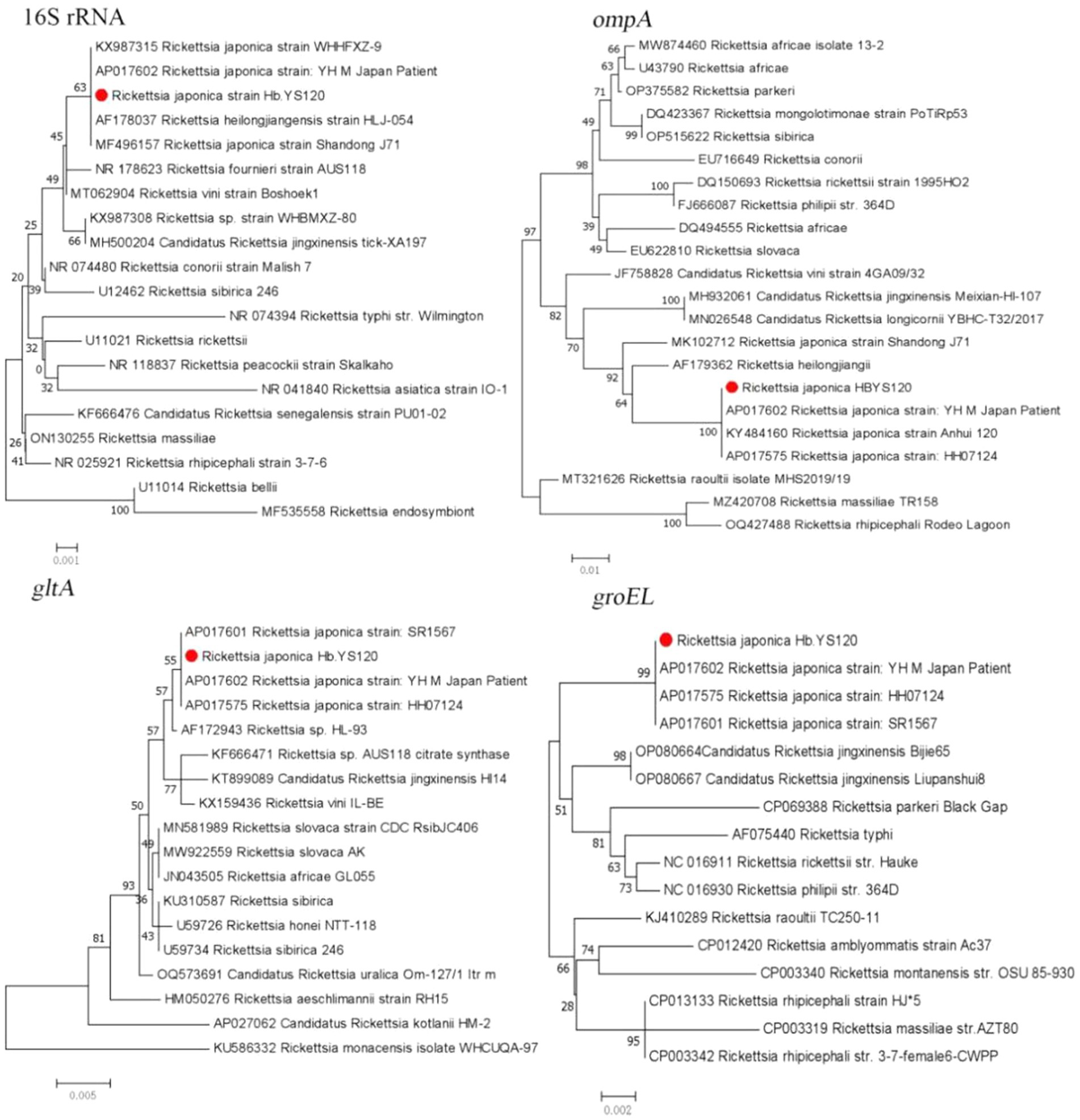
Figure 3. Phylogenetic trees constructed s of Rickettsia strains basis of the nucleotide sequences of 16S rRNA (1,200 bp), ompA (500 bp), gltA (900 bp), and groEL (1,100 bp) genes of Rickettsia strains. Sequences obtained in this study are marked with a red dot before their names.
In this study, four species of the genus Anaplasma (A. bovis, A. ovis, A. platys, and Ca. A. boleense) were detected in the ticks with a positive rate of 16.76% (30/179). A. bovis was only detected in Ha. longicornis, while the other three species were detected in R. microplus. The genetic sequences of the 16S rRNA, gltA, and groEL genes in A. bovis were found to share similarities of 99.76, 100, and 100%, respectively, with those of the previously reported A. bovis strains, one of which was Wangmang-goat-55 strain (MH255935, MH255898, and MH594293) from china. The sequences of 16S rRNA and groEL genes of A. platys detected in the ticks showed similarities of 99.52 and 99.78% to those (KU585997 and KU585930) from the A. platys strain from Wuhan City of Hubei Province, respectively. The sequences of 16S rRNA, gltA, and groEL genes of Ca. A. boleense exhibited similarities of 99.67, 100, and 100%, respectively, with those (KX987332, KX987358, and KX987389) of Ca. A. boleense strain (WHBMXZ-45) detected in Boophilus microplus ticks from Wuhan City of China. For the strains detected in A. marginale, their 16S rRNA, gltA, and groEL sequences showed the highest similarities with those (KX987327, KX987364, KX987395) of WHBMXZ-90-2 strain and those (KX987329, KX987366, KX987397) of WHBMXZ-42-2 strain from A. marginale in Philippines, as well as those (OQ135114, OQ135251, OQ135222) of JZT343 from A. marginale in China. Their similarities ranged from 99.15 to 99.98% for 16S rRNA, 99.68 to 100% for gltA, and 99.44 to 100% for groEL sequences (Figure 4).
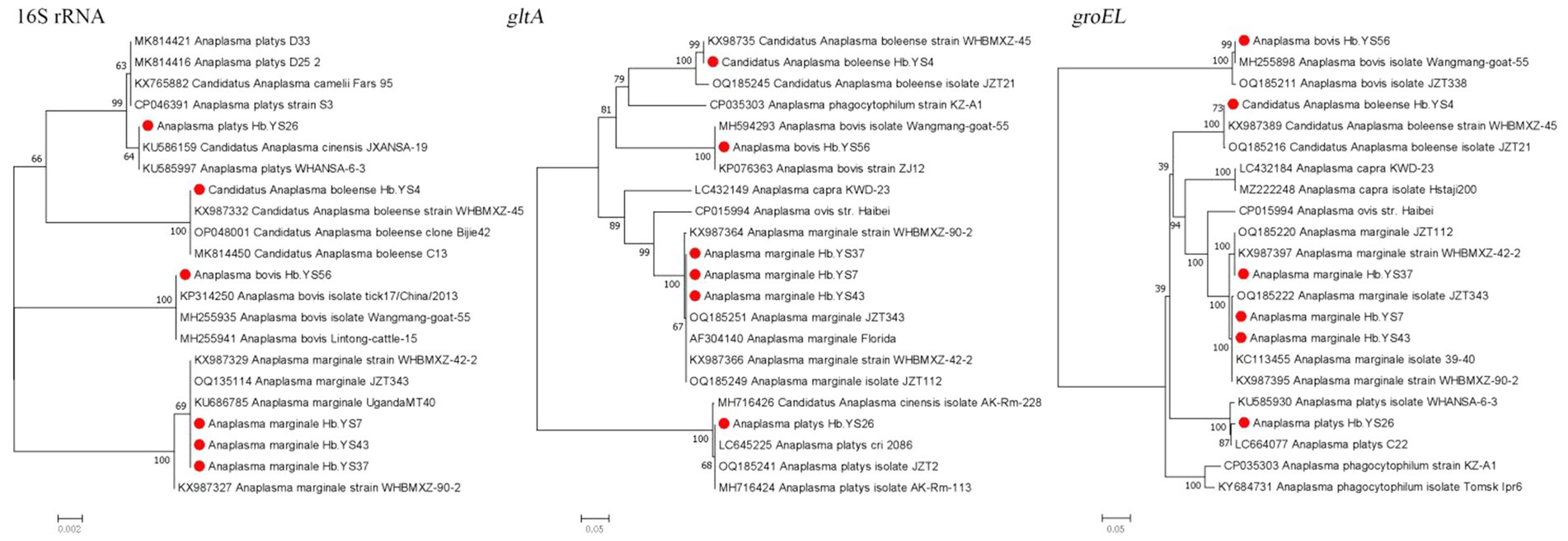
Figure 4. Phylogenetic trees constructed s of Anaplasma strains basis of the nucleotide sequences of 16S rRNA (1,400 bp), gltA and groEL genes of Anaplasma strains. Sequences obtained in this study are marked with a red dot before their names.
This study detected two species (E. minasensis and Ehrlichia sp.) of the genus Ehrlichia. The 16S rRNA, gltA and groEL gene sequences of the E. minasensis detected in the ticks were nearly identical to those (NR148800, JX629807, and JX629806) of E. minasensis UFMG-EV strain isolated from R. microplus ticks in Brazil in 2016. Additionally, the gene sequences were highly similar to those (OQ136683, OQ185261, and OQ185232) of E. minasensis JZT254 strain detected in R. microplus ticks in China in 2021. The Ehrlichia sp. detected in this study were divide into two genotypes based on their 16S rRNA, gltA and groEL gene sequences in the phylogenetic analyses. One genotype shared 100% similarity in 16S rRNA and groEL gene with Ehrlichia sp. Yonaguni138 strain (HQ697588 and HQ697590) from the ticks in Japan. The other genotype showed 99.84% similarity in 16S rRNA gene with the pathogenic Ehrlichia sp. Tibet (AF414399). Additionally, it shared 99.56–99.70 and 99.91% similarity in gltA and groEL gene with those (KX987355 and KX987386) of Ehrlichia sp. WHBMXZ-41 strain isolated from R. microplus ticks from Wuhan city of China, respectively (Figure 5).
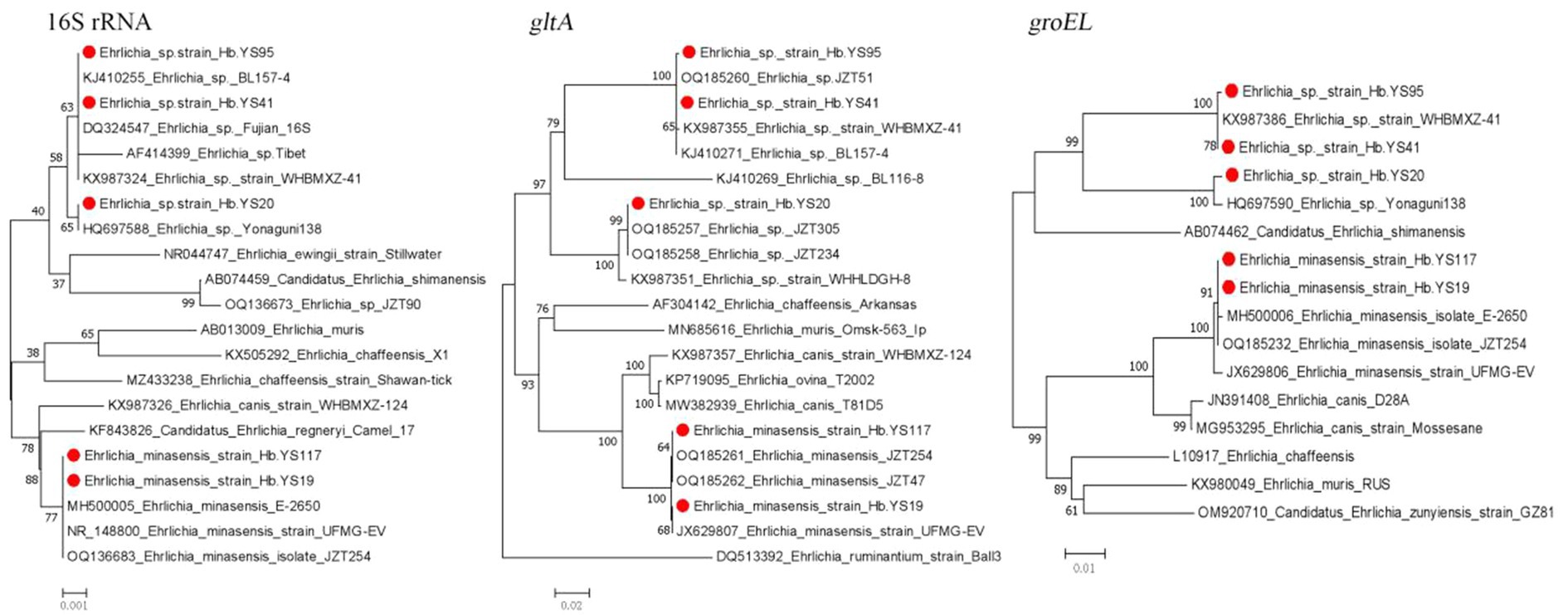
Figure 5. Phylogenetic trees constructed s of Ehrlichia strains basis of the nucleotide sequences of 16S rRNA (1,400 bp), gltA and groEL genes of Ehrlichia strains. Sequences obtained in this study are marked with a red dot before their names.
According to Figure 6, the 18S rRNA gene sequence of T. luwenshuni detected in this study showed high similarity (98.77%) to that of T. luwenshuni T31 strain (MH208628) from R. microplus ticks in China and T. luwenshuni PZG1 strain (LC326006) from goats in Myanmar. In the phylogenetic tree, the 18S rRNA gene sequences of T. orientalis were classified into two genetypes, with 3–7 nucleotide differences between them. Sequencing and BLAST analysis revealed that one genotype exhibited high 18S rRNA gene sequence similarity (97.20%) to T. orientalis Pathein_6 strain (LC576819) from cattle in Myanmar. The other genotype showed a higher similarity (99.79%) to T. orientalis T240 strain (MH20864) from R. microplus ticks in China. Furthermore, the 18S rRNA gene sequence of B. bigemina detected in this study showed 99.10% gene sequence similarity to that (KP710227) of B. bigemina TS103 strain from the cattle in China.
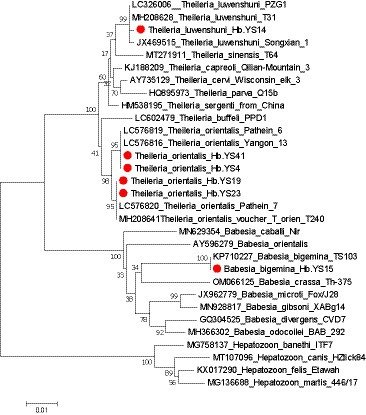
Figure 6. Phylogenetic analysis of the Theileria and Babesia strains based on their 18S rRNA gene sequences (1,400 bp). Sequences obtained in this study are marked with a red dot before their names.
4 Discussion
Our study utilized a molecular approach, incorporating PCR, DNA sequencing and phylogenetic analysis, to investigate the diversity of ticks and tick-borne bacterial and protozoan pathogens. During 2021 and 2022, a total of 179 ticks infesting cattle or sheep were collected from Yingshan County of Hubei Province in China, 166 of which were identified as Ha. Longicornis and only 13 were R. microplus. According to the study reports, Ha. longicornis is predicted to have the widest distribution in Asia, potentially impacting a population of 588 million people across 1,140 counties. And R. microplus holds the third position and is predicted to have the potential to affect a population of 350 million people across 678 counties in Asia (Zhao et al., 2021).
In this study, only one species of genus Rickettsia, R. japonica, was detected in a single specimen of R. microplus, and the sequences of 16S rRNA, ompA, gltA, and groEL genes detected in the tick was showed the highest nucleotide identity with those of R. japonica YHM strain from a patient in Japan (Akter et al., 2017). R. japonica is the causative agent of Japanese spotted fever (JSF) initially found in Japan. So far, human JSF has been found in Japan, South Korea, Philippines, Thailand, and China. From 2014 to 2021, human JSF has been reported at least hundreds of cases in mainland China, including 31 cases from Hubei province, 20 cases from the areas surrounding Jiangxi province, 20 cases from Henan province, and 18 cases from Zhejiang province in China (Li-Juan et al., 2018; Lu et al., 2018; Li et al., 2019; Zhang et al., 2023). The findings demonstrate the presence of R. japonica in ticks in the region, Combining our team’s recent report of the first 5 cases of JSF in Hubei Province, China, including one unfortunate death, this suggests the potentially posing health risks to the local residents (Teng et al., 2023). Therefore, it is imperative to strengthen the prevention and control measures for JSF and raise public awareness about this disease to safeguard public health.
Most of Anaplasma species are considered animal-specific pathogens, making them significant to public health as tick-borne bacteria. In this study, a high diversity of Anaplasma species was identified in the ticks collected from Yingshan County, including A. marginale, Ca. A. boleense, A. bovis, and A. platys. Among them, A. marginale was the most prevalent infectious tick-borned agent, infecting 15.08% of the ticks. A. marginale is known to be a specific pathogen of ruminants, commonly infecting cattle (Kocan et al., 2003), sheep (Yousefi et al., 2017), and goats (Barbosa et al., 2021), indicating its prevalence and potential impact on livestock in Yingshan County. As for Ca. A. boleense, its pathogenicity toward animals or humans is not well studied. Ca. A. boleense was initially identified in ticks from Bole City of Xinjiang Uygur Autonomous Region in China (Kang et al., 2014) and has been detected in mosquitoes and rodents in various provinces of China (Guo et al., 2016). Its wide host range and geographical distribution suggest that Ca. A. boleense merits further investigation. Furthermore, A. platys and A. bovis are considered important zoonotic pathogens. A. platys is widely distributed and can cause infectious cyclic thrombocytopenia in dogs and cats. The first case of A. platys infection in humans was confirmed by DNA sequencing in 2013 (Kang et al., 2014). A. bovis was traditionally considered to only infect cattle and cause bovine ehrlichiosis, which is prevalent in Africa and Asia. However, A. bovis was confirmed as a pathogen causing human infection in China in 2017 (Guo et al., 2016). In this study, we detected A. platys and A. bovis DNA in the ticks from ruminants, suggesting a potential risk of zoonotic transmission of A. bovis from livestock to farmers who are in regular contact with them.
Ehrlichiae are tick-borne bacteria that are responsible for life-threatening emerging human zoonoses and diseases of veterinary importance worldwide, collectively called ehrlichioses (Esemu et al., 2011). E. minasensis has a wide distribution and has been reported in multiple locations around the world, including Canada, Brazil, Pakistan, Malaysia, China, Ethiopia, and the Mediterranean island of Corsica. These findings indirectly suggest that E. minasensis may be transmitted by multiple tick species (Moura de Aguiar et al., 2019). In this study, we detected DNAs of E. minasensis in three R. microplus ticks in Yingshan County, of Hubei Province, China. which expands the distribution of E. minasensis in China and indicates it may transmission among local cattle populations. Further investigations of E. minasensis are warranted to assess the current situation in the region. This study also detected two uncultured Ehrlichia species, one of which is Ehrlichia sp. Yonaguni138 that was initially found in ticks from Japan (Matsumoto et al., 2011). The other species clusters together with Ehrlichia sp. Tibet, a tick-borne pathogen detected in ticks or animal hosts in the phylogenetic tree (Wen et al., 2002).
Piroplasms, including Theileria and Babesia, are apicomplexan parasites and may also be transmitted by ticks, and they are found worldwide infecting both wild and domestic animals. Among these, T. luwenshuni stands out as a highly pathogenic pathogen specifically affecting goats and sheep, leading to significant economic losses for farmers and livestock industries. T. orientalis is the economically predominant pathogen for bovine theileriosis in China, but it usually causes mild or asymptomatic disease (Rizk et al., 2015; Wang et al., 2020). In the context of bovine babesiosis in China, B. bigemina is considered one of the major causative agents. Infections with B. bigemina are characterized by a low level of parasitemia, which may contribute to its persistence infection and pose challenges in diagnosis and control (Niu et al., 2015; He et al., 2021). The impact of piroplasms on livestock production is particularly pronounced in developing countries. These parasites cause considerable morbidity and mortality in affected animals, resulting in reduced yields of meat, milk, and other livestock by-products. Theileria and Babesia infections pose significant challenges to the sustainable growth of the livestock industry, as they not only lead to economic losses but also hinder the overall well-being of animal populations.
5 Conclusion
In summary, we have identified numerous bacterial and protozoan pathogens in R. microplus and Ha. longicornis ticks collected from free-ranging ruminant livestock in Yingshan County of Hubei Province in China. Some of these bacteria have been reported to infect humans. This finding has raised concerns as there is close spatial proximity and contact between humans and tick hosts in Hubei Province, indicating a potential risk of human exposure to these tick-borne pathogens in the region. This is of significant importance for public health and disease prevention. Our research provides valuable references and data support for relevant authorities to develop effective prevention and control measures, and to strengthen surveillance and early warning systems for tick-borne diseases.
Data availability statement
The original contributions presented in the study are publicly available. This data can be found at: https://www.ncbi.nlm.nih.gov/; OR467404-OR467409, OR501452-OR501460, OR508717-OR508728, OR555720-OR555744.
Author contributions
NZ: Writing – original draft. KP: Resources, Writing – review & editing. ZT: Methodology, Writing – original draft. HW: Resources, Writing – review & editing. XZ: Data curation, Methodology, Writing – review & editing. HR: Data curation, Writing – review & editing. LY: Resources, Writing – review & editing. JH: Methodology, Writing – review & editing. KC: Resources, Writing – review & editing. TQ: Conceptualization, Writing – review & editing.
Funding
The author(s) declare financial support was received for the research, authorship, and/or publication of this article. This work was supported by grants from the National Natural Science Foundation of China (grant number 81671985), the Science Foundation for the State Key Laboratory for Infectious Disease Prevention and Control of China (grant numbers 2022SKLID209 and 2019SKLID403), and the Public Health Service Capability Improvement Project of the National Health Commission of the People’s Republic of China (grant number 2100409002).
Conflict of interest
The authors declare that the research was conducted in the absence of any commercial or financial relationships that could be construed as a potential conflict of interest.
Publisher’s note
All claims expressed in this article are solely those of the authors and do not necessarily represent those of their affiliated organizations, or those of the publisher, the editors and the reviewers. Any product that may be evaluated in this article, or claim that may be made by its manufacturer, is not guaranteed or endorsed by the publisher.
Supplementary material
The Supplementary material for this article can be found online at: https://www.frontiersin.org/articles/10.3389/fmicb.2023.1298037/full#supplementary-material
References
Akter, A., Ooka, T., Gotoh, Y., Yamamoto, S., Fujita, H., Terasoma, F., et al. (2017). Extremely low genomic diversity of Rickettsia japonica distributed in Japan. Genome Biol. Evol. 9, 124–133. doi: 10.1093/gbe/evw304
Azmat, M., Ijaz, M., Farooqi, S. H., Ghaffar, A., Ali, A., Masud, A., et al. (2018). Molecular epidemiology, associated risk factors, and phylogenetic analysis of anaplasmosis in camel. Microb. Pathog. 123, 377–384. doi: 10.1016/j.micpath.2018.07.034
Barbosa, I. C., André, M. R., Amaral, R. B. D., Valente, J. D. M., Vasconcelos, P. C., Oliveira, C. J. B., et al. (2021). Anaplasma marginale in goats from a multispecies grazing system in northeastern Brazil. Ticks Tick Borne Dis. 12:101592. doi: 10.1016/j.ttbdis.2020.101592
Cao, W. C., Gao, Y. M., and Zhang, P. H. (2000). Identification Ehrlichia chaffeensis by nested PCR in ticks from southern China. J. Clin. Microbiol. 38, 2778–2780. doi: 10.1128/JCM.38.7.2778-2780.2000
Chen, Z., Yang, X., Bu, F., Yang, X., Yang, X., and Liu, J. (2010). Ticks (acari: ixodoidea: argasidae, ixodidae) of China. Exp. Appl. Acarol. 51, 393–404. doi: 10.1007/s10493-010-9335-2
de la Fuente, J., Estrada-Pena, A., Venzal, J. M., Kocan, K. M., and Sonenshine, D. E. (2008). Overview: ticks as vectors of pathogens that cause disease in humans and animals. Front. Biosci. 13, 6938–6946. doi: 10.2741/3200
El-Alfy, E. S., Abbas, I., Baghdadi, H. B., El-Sayed, S. A. E., Ji, S., and Rizk, M. A. (2022). Molecular epidemiology and species diversity of tick-borne pathogens of animals in Egypt: a systematic review and meta-analysis. Pathogens 11:912. doi: 10.3390/pathogens11080912
el-Alfy, E. S., Abbas, I., Elseadawy, R., Saleh, S., Elmishmishy, B., el-Sayed, S. A. E. S., et al. (2023). Global prevalence and species diversity of tick-borne pathogens in buffaloes worldwide: a systematic review and meta-analysis. Parasit. Vectors 16:115. 2023 Mar 30. doi: 10.1186/s13071-023-05727-y
Esemu, S. N., Ndip, L. M., and Ndip, R. N. (2011). Ehrlichia species, probable emerging human pathogens in sub-Saharan Africa: environmental exacerbation. Rev. Environ. Health 26, 269–279. doi: 10.1515/reveh.2011.034.22435325
Guo, W. P., Tian, J. H., Lin, X. D., Ni, X. B., Chen, X. P., Liao, Y., et al. (2016). Extensive genetic diversity of Rickettsiales bacteria in multiple mosquito species. Sci. Rep. 6:38770. doi: 10.1038/srep38770
He, L., Bastos, R. G., Sun, Y., Hua, G., Guan, G., Zhao, J., et al. (2021). Babesiosis as a potential threat for bovine production in China. Parasit. Vectors 14:460. doi: 10.1186/s13071-021-04948-3
He, M., Zhang, L., Hu, H., Liu, X., Zhang, C., Xin, Y., et al. (2023). Complete genome sequencing and comparative genomic analyses of a new spotted-fever Rickettsia heilongjiangensis strain B8. Emerg. Microbes Infect. 12:2153085. doi: 10.1080/22221751.2022.2153085
Jia, N., Zheng, Y. C., Jiang, J. F., Ma, L., and Cao, W. C. (2013). Human infection with Candidatus Rickettsia tarasevichiae. N. Engl. J. Med. 369, 1178–1180. doi: 10.1056/NEJMc1303004
Jin, X., Liao, J., Chen, Q., Ding, J., Chang, H., Lyu, Y., et al. (2023). Diversity of Rickettsiales bacteria in five species of ticks collected from Jinzhai County, Anhui Province, China in 2021-2022. Front. Microbiol. 14:1141217. doi: 10.3389/fmicb.2023.1141217
Kang, Y. J., Diao, X. N., Zhao, G. Y., Chen, M. H., Xiong, Y., Shi, M., et al. (2014). Extensive diversity of Rickettsiales bacteria in two species of ticks from China and the evolution of the Rickettsiales. BMC Evol. Biol. 14:167. doi: 10.1186/s12862-014-0167-2
Kim, H. K. (2022). Rickettsia-host-tick interactions: knowledge advances and gaps. Infect. Immun. 90:e0062121. doi: 10.1128/iai.00621-21
Kocan, K. M., De La Fuente, J., Guglielmone, A. A., and Meléndez, R. D. (2003). Antigens and alternatives for control of Anaplasma marginale infection in cattle. Clin. Microbiol. Rev. 16, 698–712. doi: 10.1128/cmr.16.4.698-712.2003
Li, Y., Galon, E. M., Guo, Q., Rizk, M. A., Moumouni, P. F. A., Liu, M., et al. (2020). Molecular detection and identification of Babesia spp., Theileria spp., and Anaplasma spp. in sheep from border regions, northwestern China. Front. Vet. Sci. 7:630. doi: 10.3389/fvets.2020.00630
Li, H., Zhang, P. H., du, J., Yang, Z. D., Cui, N., Xing, B., et al. (2019). Rickettsia japonica infections in humans, Xinyang, China, 2014-2017. Emerg. Infect. Dis. 25, 1719–1722. doi: 10.3201/eid2509.171421
Li, H., Zhang, L. K., Li, S. F., Zhang, S. F., Wan, W. W., Zhang, Y. L., et al. (2019). Calcium channel blockers reduce severe fever with thrombocytopenia syndrome virus (SFTSV) related fatality. Cell Res. 29, 739–753. doi: 10.1038/s41422-019-0214-z
Li, H., Zheng, Y. C., and Ma, L. (2015). Human infection with a novel tick-borne Anaplasma species in China: a surveillance study. Lancet Infect. Dis. 15, 663–670. doi: 10.1016/S1473-3099(15)70051-4
Li-Juan, Z., Zhi-Min, X., Si-Min, D., Hui, D., Shan, L., Jing, X., et al. (2018). Endemic status of schistosomiasis in People's Republic of China in 2017. Zhongguo Xue Xi Chong Bing Fang Zhi Za Zhi. 30, 481–488. doi: 10.16250/j.32.1374.2018219
Lu, M., Qin, X. C., Jiang, Y. Z., Guo, Q., Jin, X. J., Teng, Z. Q., et al. (2023). Emergence of ehrlichiosis by a new tick-borne Ehrlichia species in China. Int. J. Infect. Dis. 131, 32–39. doi: 10.1016/j.ijid.2023.03.038
Lu, Q., Yu, J., Yu, L., Zhang, Y., Chen, Y., Lin, M., et al. (2018). Rickettsia japonica infections in humans, Zhejiang Province, China, 2015. Emerg. Infect. Dis. 24, 2077–2079. doi: 10.3201/eid2411.170044
Madison-Antenucci, S., Kramer, L. D., Gebhardt, L. L., and Kauffman, E. (2020). Emerging tick-borne diseases. Clin. Microbiol. Rev. 33, e00083–e00018. doi: 10.1128/CMR.00083-18
Mansfield, K. L., Jizhou, L., Phipps, L. P., and Johnson, N. (2017). Emerging tick-borne viruses in the twenty-first century. Front. Cell. Infect. Microbiol. 7:298. doi: 10.3389/fcimb.2017.00298
Matos, A. L., Curto, P., and Simões, I. (2022). Moonlighting in Rickettsiales: expanding virulence landscape. Trop. Med. Infect. Dis. 7:32. doi: 10.3390/tropicalmed7020032
Matsumoto, K., Takeuchi, T., Yokoyama, N., Katagiri, Y., Ooshiro, M., Zakimi, S., et al. (2011). Detection of the new Ehrlichia species closely related to Ehrlichia ewingii from Haemaphysalis longicornis in Yonaguni Island, Okinawa, Japan. J. Vet. Med. Sci. 73, 1485–1488. doi: 10.1292/jvms.11-0007
Mediannikov, O., and Fenollar, F. (2014). Looking in ticks for human bacterial pathogens. Microb. Pathog. 77, 142–148. doi: 10.1016/j.micpath.2014.09.008
Moura de Aguiar, D., Pessoa Araújo Junior, J., Nakazato, L., Bard, E., Aguilar-Bultet, L., Vorimore, F., et al. (2019). Isolation and characterization of a novel pathogenic strain of Ehrlichia minasensis. Microorganisms 7:528. doi: 10.3390/microorganisms7110528
Niu, Q., Liu, Z., Yu, P., Yang, J., Abdallah, M. O., Guan, G., et al. (2015). Genetic characterization and molecular survey of Babesia bovis, Babesia bigemina and Babesia ovata in cattle, dairy cattle and yaks in China. Parasit. Vectors 8:518. doi: 10.1186/s13071-015-1110-0
Rizk, M. A., el-Sayed, S. A. E. S., Terkawi, M. A., Youssef, M. A., el Said, E. S. E. S., Elsayed, G., et al. (2015). Optimization of a fluorescence-based assay for large-scale drug screening against Babesia and Theileria parasites. PLoS One 10:e0125276. doi: 10.1371/journal.pone.0125276
Teng, Z., Gong, P., Wang, W., Zhao, N., Jin, X., Sun, X., et al. (2023). Clinical forms of Japanese spotted fever from case-series study, Zigui County, Hubei Province, China, 2021. Emerg. Infect. Dis. 29, 202–206. doi: 10.3201/eid2901.220639
Tian, Z. C., Liu, G. Y., Shen, H., Xie, J. R., Luo, J., and Tian, M. Y. (2012). First report on the occurrence of Rickettsia slovaca and Rickettsia raoultii in Dermacentor silvarum in China. Parasit. Vectors 5:19. doi: 10.1186/1756-3305-5-19
Tokarz, R., and Lipkin, W. I. (2021). Discovery and surveillance of tick-borne pathogens. J. Med. Entomol. 58, 1525–1535. doi: 10.1093/jme/tjaa269
Wang, J., Yang, J., Gao, S., Liu, A., Rashid, M., Li, Y., et al. (2020). Rapid detection and differentiation of Theileria annulata, T. Orientalis and T. sinensis using high-resolution melting analysis. Ticks Tick Borne Dis. 11:101312. doi: 10.1016/j.ttbdis.2019.101312
Wen, B., Cao, W., and Pan, H. (2003). Ehrlichiae and ehrlichial diseases in China. Ann. N. Y. Acad. Sci. 990, 45–53. doi: 10.1111/j.1749-6632.2003.tb07335.x
Wen, B., Jian, R., Zhang, Y., and Chen, R. (2002). Simultaneous detection of Anaplasma marginale and a new Ehrlichia species closely related to Ehrlichia chaffeensis by sequence analyses of 16S ribosomal DNA in Boophilus microplus ticks from Tibet. J. Clin. Microbiol. 40, 3286–3290. doi: 10.1128/JCM.40.9.3286-3290.2002
Yousefi, A., Rahbari, S., Shayan, P., Sadeghi-Dehkordi, Z., and Bahonar, A. (2017). Molecular detection of Anaplasma marginale and Anaplasma ovis in sheep and goat in west highland pasture of Iran. Asian Pac. J. Trop. Biomed. 7, 455–459. doi: 10.1016/j.apjtb.2017.01.017
Zhai, J., Wu, Y., Chen, J., Zou, J., Shan, F., Li, W., et al. (2021). Identification of Amblyomma javanense and detection of tick-borne Ehrlichia spp. in confiscated Malayan pangolins. Int. J. Parasitol. Parasites Wildl. 14, 107–116. Published 2021 Jan 30. doi: 10.1016/j.ijppaw.2021.01.008
Zhang, X., Chen, H., Han, D., and Wu, W. (2023). Clinical usefulness of metagenomic next-generation sequencing for Rickettsia and Coxiella burnetii diagnosis. Eur. J. Clin. Microbiol. Infect. Dis. 42, 681–689. doi: 10.1007/s10096-023-04586-w
Keywords: tick, Rickettsia, Ehrlichi, Anaplasma, Piroplasms
Citation: Zhao N, Pan K, Teng Z, Wang H, Zhang X, Ren H, Yi L, He J, Cai K and Qin T (2023) Molecular detection reveals diverse tick-borne bacterial and protozoan pathogens in two tick species from Yingshan County of Hubei Province, China in 2021–2022. Front. Microbiol. 14:1298037. doi: 10.3389/fmicb.2023.1298037
Edited by:
Santosh Pandit, Chalmers University of Technology, SwedenReviewed by:
Mohamed Abdo Rizk, Mansoura University, EgyptQiaocheng Chang, Shantou University, China
Copyright © 2023 Zhao, Pan, Teng, Wang, Zhang, Ren, Yi, He, Cai and Qin. This is an open-access article distributed under the terms of the Creative Commons Attribution License (CC BY). The use, distribution or reproduction in other forums is permitted, provided the original author(s) and the copyright owner(s) are credited and that the original publication in this journal is cited, in accordance with accepted academic practice. No use, distribution or reproduction is permitted which does not comply with these terms.
*Correspondence: Tian Qin, cWludGlhbkBpY2RjLmNu; Kun Cai, Y2tyZWFsQDE2My5jb20=
†These authors have contributed equally to this work