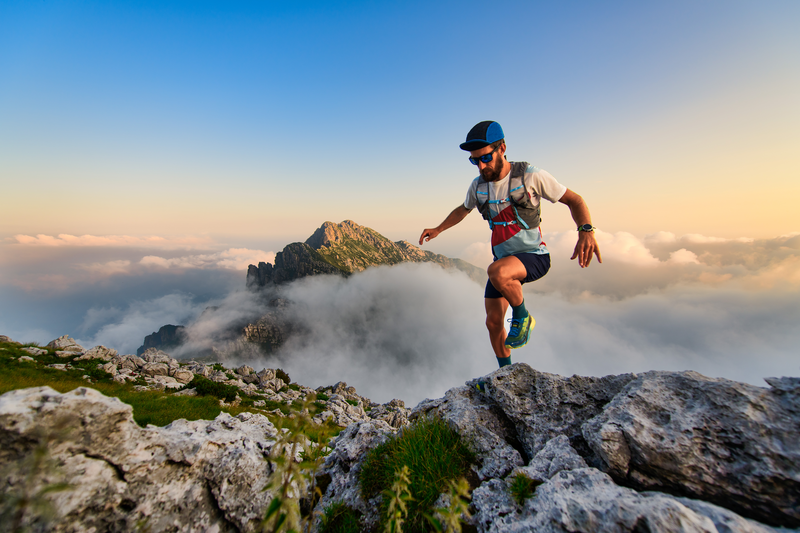
94% of researchers rate our articles as excellent or good
Learn more about the work of our research integrity team to safeguard the quality of each article we publish.
Find out more
ORIGINAL RESEARCH article
Front. Microbiol. , 08 January 2024
Sec. Terrestrial Microbiology
Volume 14 - 2023 | https://doi.org/10.3389/fmicb.2023.1288585
Introduction: The contamination of Trichoderma species causing green mold in substrates poses a significant obstacle to the global production of Lentinula edodes, adversely impacting both yield and quality of fruiting bodies. However, the diversity of Trichoderma species in the contaminated substrates of L. edodes (CSL) in China is not clear. The purpose of this study was to assess the biodiversity of Trichoderma species in CSL, and their interactions with L. edodes.
Methods: A comprehensive two-year investigation of the biodiversity of Trichoderma species in CSL was conducted with 150 samples collected from four provinces of China. Trichoderma strains were isolated and identified based on integrated studies of phenotypic and molecular data. Resistance of L. edodes to the dominant Trichoderma species was evaluated in dual culture in vitro.
Results: A total of 90 isolates were obtained and identified as 14 different Trichoderma species, including six new species named as Trichoderma caespitosus, T. macrochlamydospora, T. notatum, T. pingquanense, T. subvermifimicola, and T. tongzhouense, among which, T. atroviride, T. macrochlamydospora and T. subvermifimicola were identified as dominant species in the CSL. Meanwhile, three known species, namely, T. auriculariae, T. paraviridescens and T. subviride were isolated from CSL for the first time in the world, and T. paratroviride was firstly reported to be associated with L. edodes in China. Notebly, the in vitro evaluation of L. edodes resistance to dominant Trichoderma species showed strains of L. edodes generally possess poor resistance to Trichoderma contamination with L. edodes strain SX8 relatively higher resistant.
Discussion: This study systematically investigated the diversity of Trichoderma species in the contaminated substrate of L. edodes, and a total of 31 species so far have been reported, indicating that green mold contaminated substrates of edible fungi were undoubtedly a biodiversity hotspot of Trichoderma species. Results in this study will provide deeper insight into the genus Trichoderma and lay a strong foundation for scientific management of the Trichoderma contamination in L. edodes cultivation.
Lentinula edodes (Berk.) Pegler is commonly known as “Xianggu” in Chinese, which is the most widely cultivated mushroom species in the world (Yan et al., 2020, 2021). The annual total production and sales rank first, and the total output of L. edodes in 2020 accounted for 29.25% of the total output of edible fungi in China (Zhang et al., 2015; Chinese Edible Fungus A, 2022). Lentinula edodes is distinguished by delicious taste, multiple nutrients, and medicinal properties (Zhao et al., 2020). The cultivation of L. edodes demonstrates inherent characteristics of circularity, high efficiency and ecological compatibility, rendering it a prime avenue for farmers to alleviate poverty and facilitate rural revitalization in China (He, 2019). However, the substrate contamination from Trichoderma spp. is regarded as a significant constraint in the production of L. edodes, resulting in economic losses of 10–20% of the total production (Yan et al., 2019). The investigation into the deleterious effects of Trichoderma spp. on edible fungi commenced relatively late in China. Following the rapid development of edible fungi industry, serious Trichoderma spp. contamination reported after the 1980s, with the most major mold contamination occurred in summer, resulting in entire cultivation batches being discarded (Fu et al., 1981). “Green mold on L. edodes and its chemical control” was formally reported at the National Fungal Lichen Academic Symposium in 1990; then, Trichoderma contamination was officially listed as a disease of L. edodes recorded in history (Wang, 1994). Meanwhile, there is no highly effective fungicide against Trichoderma, which is harmless to L. edodes. The identification and detection of Trichoderma species are essential for the prevention and control of green mold contamination in the production of L. edodes (Lee et al., 2020; Allaga et al., 2021).
The genus Trichoderma Pers. (Ascomycota, Sordariomycetes, Hypocreales) is cosmopolitan and of great diversity with over 441 known species until now (Barrera et al., 2021; Zheng et al., 2021; Cao et al., 2022; Yu et al., 2022). Among them, many species, such as T. atroviride, T. harzianum, and T. pleuroticola, are contaminants associated with the production of edible fungi (Kim et al., 2012). However, the invasiveness and isolation frequencies of Trichoderma spp. are distinct on edible fungi in different regions (Kim et al., 2012; Wang et al., 2016). Furthermore, the recognition of Trichoderma spp. associated with the contamination of edible fungi primarily was previously conducted only by morphological characteristic and ITS sequence analysis. In addition, the samples collected were limit to local regions, which are difficult for systematic understanding of their population structure. There is also a lack of systematic research on the lineage of Trichoderma spp. contaminating on L. edodes in multiple regions (Jiang et al., 1995; Hatvani et al., 2007; Cai and Druzhinina, 2021). Considering the constraints faced by the edible fungi industry, a significant long-term objective for L. edodes breeding is the selection of varieties with superior resistance to Trichoderma contamination under the interaction between Trichoderma species and L. edodes.
To elucidate the Trichoderma spp. present in the CSL in North China, we conducted an in-depth investigation on the microbial community within CSL and identified six novel species belonging to the Harzianum clade, which have not been previously described. Their phylogenetic positions were determined based on sequence analyses of partial translation elongation factor 1-alpha (tef1-α) and partial RNA polymerase second largest subunit (rpb2) genes. Similarities and differences of morphological characters between the new species and their closely related species were presented and compared in detail. Their interaction was also primarily discussed in this study to distinguish the resistance difference of mushroom varieties to Trichoderma.
Cylindrical sticks wrapped in plastic bags are usually used for L. edodes cultivation in the main production areas investigated. Contaminated stick specimens of L. edodes by Trichoderma spp. were separately collected from several edible fungus production bases in seven regions of Beijing, Hebei, Shanxi, and Henan Provinces of China during 2020 to 2022 (Figure 1A). To make the isolation frequency more convincing, contaminated L. edodes sticks were randomly collected. These sticks were kept as intact as possible to avoid secondary contamination due to breakage. Cultures were obtained by aseptically transferring the contaminated substrates of collected stick specimens onto potato dextrose agar (PDA) following the method of Kim et al. (2010) (Supplementary Figure S1). The isolation frequency (%) for Trichoderma species was calculated: the number of isolates per species/total number isolates × 100.
Figure 1. Isolation and identification of Trichoderma spp. (A) Map of isolates collection; (B) isolation frequency of Trichoderma spp.
Colonies with features resembling typical Trichoderma spp. were preliminary screened and then purified with monoconidial isolation method, and single-conidium cultures were stored in 25 vol % glycerol at −80°C (Chomnunti et al., 2014). The strains obtained were deposited in the culture collection of the Beijing Academy of Agricultural and Forestry Sciences, China.
For morphological studies, growth rates and colony characteristics were determined on three different media: potato dextrose agar (PDA; 200 g potato, 18 g dextrose, 18 g agar, 1 L distilled water), cornmeal dextrose agar (CMD; 40 g cornmeal, 20 g glucose, 18 g agar, 1 L distilled water), and synthetic low nutrient agar (SNA; 1 g KH2PO4, 1 g KNO3, 0.5 g MgSO4‧7H2O, 0.5 g KCl, 0.2 g glucose, 0.2 g sucrose, 18 g agar, 1 L distilled water), and cultured at 25, 30, and 35°C in darkness, respectively. Mycelial disks (5 mm diam.) were incubated in Petri dishes (9 cm diam.) with each isolate three replicates. Colony radius was measured after 72 h. The morphological characteristics of colonies, including colony appearance, color, radial, concentric rings formed by spore production, pigmentation, and odor, were recorded (Zheng et al., 2021). Microscopic characters were photographed and measured with Olympus BX51 microscope (Tokyo, Japan) connected to a DP controller digital camera. Microscopic characteristics and micromorphological data were examined on the cultures grown on SNA and PDA for 7 days at 25°C. Descriptions included the data of phialides, conidia, and chlamydospores. New species were described basically following counterparts by Chaverri et al. (2015).
Mycelium of each isolate was cultivated on PDA medium for 7 days at 25°C in darkness; then, genomic DNA was extracted using the Plant Genomic DNA Kit (DP305, TIANGEN Biotech, Beijing, China). The DNA fragments of tef1-α and rpb2 were amplified and sequenced with the primer pairs EF1-728F (Carbone and Kohn, 1999) and TEF1LLErev (Jaklitsch et al., 2005), and fRPB2-5f and fRPB2-7cr (Liu et al., 1999), respectively. Polymerase chain reactions (PCRs) were performed in 25 μL total volumes consisting of 12.5 μL Premix Taq™ (TaKaRa Taq™ Version 2.0 plus dye), 1.0 μL of each primer (10 μM), 1.5 μL DNA, and 9 μL double sterilized water. Cycling parameters were 95°C for 5 min, followed by 30 cycles of 95°C for 1 min, 55°C for 1 min, and 72°C for 2 min, with a final elongation at 72°C for 10 min. The positive amplicons were sequenced by ABI 3730 DNA Sequencer (Applied Biosystems, Bedford, MA, USA) at SinoGenoMax company.
All sequences were assembled and manually adjusted using the DNAStar Seqman program 7.1.0 (DNASTAR. Inc., Madison). Phylogenetic analyses were performed for the identification based on the combined sequences of rpb2 and tef1-α. Sequences generated from this study and those retrieved from GenBank based on previous studies are listed in Supplementary Table S1. The final dataset for analyses comprised 289 sequences representing 149 Trichoderma species, with Nectria berolinensis and N. eustromatica selected as outgroup taxa. Alignments were performed separately for each molecular marker and converted to nexus files with Clustal X 1.83 (Thompson et al., 1997). Then, the rpb2 and tef1-α alignments were manually adjusted and concatenated through BioEdit v.7.0. Ambiguously aligned regions were excluded from phylogenetic analyses. Phylogenetic analyses were performed using both maximum parsimony (MP) and Bayesian tree inference (BI).
Maximum parsimony tree was performed using PAUP 4.0b10. Each iteration performs 1,000 iterations of random sequence addition by heuristic search and subsequent branch-swapping algorithm using tree-bisection-reconnection (TBR) (Swofford, 2002). The topological confidence of the tree was tested by calculating maximum parsimony bootstrap proportion (MPBP). Bootstrap analyses calculated via 1,000 bootstrap replications, each with 10 replicates of random addition of taxa.
Bayesian inference trees were analyzed using MrBayes v. 3.1.2 (Ronquist and Huelsenbeck, 2003). GTR + I + G were estimated as the best-fit model using MrModeltest 2.3 (Nylander, 2004). Metropolis-coupled Markov chain Monte Carlo (MCMCMC) analyses were run using four chains for 6,000,000 generations sampling every 100 generations. The initial 25% tree sample was discarded as burn-in, and Bayesian inference posterior probability (BIPP) was determined from the remaining trees. Trees were visualized in FigTree v1.4.3 (Zhang and Zhuang, 2019).
To evaluate the resistance of L. edodes to two dominant new Trichoderma species, T. macrochlamydospora and T. subvermifimicola, 35 strains of L. edodes were selected for dual-culture experiments on PDA (Supplementary Table S2). Trichoderma and L. edodes strains were first cultured on PDA for 5 and 10 days, respectively; then, mycelial disks (5 mm diameter) of L. edodes were inoculated onto PDA one side of the Petri plates (9 cm diameter). Six days later, Trichoderma mycelial disks of equal size were placed on a fresh PDA plate 50 mm apart from L. edodes. As control, L. edodes alone were placed in Petri dishes without Trichoderma. The plates were incubated in darkness at 25°C. Each assay was repeated three times.
Microscopic features of the hyphal interaction were photographed by DP controller digital camera attached to an Olympus BX51 microscope (Tokyo, Japan) after colonies of Trichoderma and L. edodes contacted with each other in 24–48 h. Status of cultures including growth rate and sporulation of Trichoderma spp. on L. edodes were photographed at the 13th day after inoculation. The inhibitory rate (%) was used to characterize the resistance of L. edodes to Trichoderma spp. as (colony radius of L. edodes in control group – colony radius in treatment group) / (colony radius of L. edodes in control group – 2.5 mm) × 100.
In this study, 90 isolates of L. edodes contaminated with Trichoderma spp. were collected from seven regions of Beijing, Hebei, Shanxi, and Henan provinces during 2020 to 2022. These isolates were then recognized as 14 species, including eight species in the Harzianum clade, two species in the Longibrachiatum clade, and four species in the Viride clade. Twelve strains collected from Beijing belonged to four species, among which T. caespitosus, T. notatum, and T. tongzhouense were new species. The 71 strains from Hebei Province represented 11 species, including three new species as T. macrochlamydospora, T. pingquanense, and T. subvermifimicola. In addition, the isolates collected from Henan province were recognized as three species, and four strains collected from Shanxi province were all recognized as T. macrochlamydospora. The isolation frequency of 14 Trichoderma species was calculated and analyzed. Among them, isolation frequencies of T. atroviride, T. macrochlamydospora, and T. subvermifimicola rank the top three, namely, 15.6, 15.6, and 10.0%, respectively (Figure 1). Three known Trichoderma species, namely, T. auriculariae, T. paraviridescens and T. subviride were isolated from CSL for the first time in the world, and T. paratroviride was firstly reported to be associated with L. edodes in China. The information of the specimens used in this study is shown in Figure 1 and Supplementary Table S3. Interestingly, each stick was usually contaminated by single Trichoderma species and occasionally two Trichoderma species.
The partition homogeneity test (p = 0.01) of rpb2 and tef1-α sequences indicated that the individual partitions were generally congruent (Cunningham, 1997). Phylogenetic positions of Trichoderma species were determined by analyses of the combined rpb2 and tef1-α dataset containing 289 taxa and 2,440 characters, of which 1,184 characters were constant, 275 variable characters were parsimony-uninformative, and 981 were parsimony-informative. Two most-parsimonious trees with the same topology were generated (Figure 2) (tree length = 7,876, CI = 0.2920, HI = 0.7080, RC = 0.2469, and RI = 0.8455). Meanwhile, the BI analysis showed congruence with the topology of the MP analyses.
Figure 2. Maximum parsimony phylogram of investigated Trichoderma species inferred from the combined sequences of rpb2 and tef1-α. MPBP above 50% (left) and BIPP above 90% (right) were indicated at the nodes. New species proposed are indicated in red font, and known species in this study were indicated in blue font. Shorted nodes are marked with crossing lines and indications (×2, ×3, ×4, ×7, ×10) of how many times the node has been shortened.
A total of 289 sequences representing 149 Trichoderma species, including our seven new species and two out-groups, were used to construct the phylogenetic tree. All species formed a strongly supported group (MPBP = 100%), which was generally congruent with the previous studies (Gu et al., 2020).
Phylogenetic analyses revealed that six new species belonged to the Harzianum clade and clustered at the top of the tree. Their phylogenetic relationships were relatively close. Trichoderma tongzhouense and T. pingquanense, and T. subvermifimicola and T. vermifimicola (MPBP = 60%) clustered as two sister groups, respectively. Trichoderma macrochlamydospora, T. caespitosus, and T. notatum formed highly supported terminal branches with statistic values MPBP/BIPP = 100%/100%. In addition, T. auriculariae and T. lentinulae also belonged to the Harzianum clade. Other known species, namely, T. longibrachiatum, T. paraviridescens, T. paratroviride, T. atroviride, T. subviride, and T. citrinoviride, isolated in this study belonged to the Longibrachiatum and Viride clade. Their phylogenetic positions were consistent with previous studies (Gu et al., 2020). Meanwhile, high degree of intraspecies variation for T. paratroviride and T. paraviridescens was accepted.
Trichoderma atroviride P. Karst., Bidr. Känn. Finl. Nat. Folk 51: 363. 1892. Wen, Tao & Chen, Acta Mycol. Sin. 12: 124. 1993.
= Hypocrea atroviridis Dodd, Lieckf. & Samuels, Mycologia 95: 36. 2003.
Materials examined: China, Hebei Province, Pingquan, 1 January 2022, W.T. Qin, X.Q. Wang, JZBQT7Z1, JZBQT7Z2, JZBQT7Z3; ibid., 8 January 2022, W.T. Qin, X.Q. Wang, JZBQT8Z4, JZBQT8Z5, JZBQT8Z6; ibid., 11 February. 2022, W.T. Qin, X.Q. Wang, JZBQT10Z9, JZBQT10Z13; ibid., 8 Mar. 2022, W.T. Qin, X.Q. Wang, JZBQT12Z1, JZBQT12Z2, JZBQT12Z3.
Notes—Trichoderma atroviride is a widely distributed species found in many countries and is critical biological control agents promoting efficient plant growth and improving stress resistance (Skoneczny et al., 2015). In addition, green mold caused by T. atroviride was found to be associated with various edible fungi around the word, such as L. edodes, Pleurotus spp., Agaricus spp., and Ganoderma lingzhi (Kim et al., 2012; Yan et al., 2019). Its main characteristic is the smell of coconut, and subglobose, dark green conidia. Phialides slender, narrow ampulliform, often arcuate toward the end of the branches.
Trichoderma auriculariae Z. J. Cao & W.T. Qin. J. Fungi 8: 7. 2022.
Materials examined: China, Hebei Province, Pingquan, 11 February 2022, W.T. Qin, X.Q. Wang, JZBQT10Z10, JZBQT10Z11.
Notes: Trichoderma auriculariae was originally found in the contaminated substrate of Auricularia auricular (Cao et al., 2022). It was isolated from the CSL for the first time. Trichoderma auriculariae has a typical conical branch of the Harzianum clade, with distinct symmetrical branches on both sides of the principal axis, often with 3–5 phialides whorled at the end of the branch. Notably, T. auriculariae had longer phialides than T. simmonsi and had larger conidia than that of T. vermifimicola and T. xixiacum. Interestingly, characteristics of T. auriculariae varied in its original host isolated from. In this study, strain JZBQT10Z10 isolated from the contaminated substrates of L. edodes grew faster [27–30 mm] and had stronger tolerance to high temperature than that of strain JZBQT1Z7 isolated from the contaminated substrate of A. auricular [5–7 mm] at 35°C (Cao et al., 2022).
Trichoderma caespitosus Z. J. Cao & W.T. Qin, sp. nov. (Figure 3).
Figure 3. Trichoderma caespitosus (JZBQT1Z6) cultures at 25°C after 7 days [(A) on CMD, (B) on SNA, (C) on PDA]; (D,E) conidiation pustules (CMD, 7d); (F–H) conidiophores and phialides (SNA, 7d); (I) chlamydospores (PDA, 7 days); (J) conidia (SNA, 7 days); scale bars: (F,G,H,I) = 10 μm, (J) = 5 μm.
MycoBank: MB849036.
Type: China, Beijing, Tongzhou district, 39°41′51” N, 116°45′1″ E, 26 August 2021, W.T. Qin, Y. Liu, S.X. Wang, (ex-type strain JZBQT1Z6). GenBank accessions: rpb2 = OP832383, tef1-α = OP832398.
Etymology: The specific epithet refers to dense conidiophores of this species.
Description. On CMD after 72 h, colony radius 65–68 mm at 25°C, covering the plate at 30°C, 22–25 mm at 35°C. Colony is round and hyaline, with very few airborne mycelia. A large number of white pustules formed obvious concentric rings along the outer edge of colony after 2 days. Conidiation formed on aerial hyphae and pustules and gradually turn pale green. No diffusing pigment. On PDA after 72 h, colony radius 60–63 mm at 25°C, 63–67 mm at 30°C, 8–10 mm at 35°C. Colony radial, dense, regularly round. Colony is white at 25°C and green at 30°C. Aerial hyphae white and abundant, denser at the colony center. Conidiation effuse in aerial hyphae. No diffusing pigment, no distinct odor. On SNA after 72 h, colony radius 48–52 mm at 25°C, 40–45 mm at 30°C, 12–13 mm at 35°C. Colony hyaline, aerial hyphae lacking. There were more aerial hyphae near the inoculation block, and pustules were formed in the middle circle, which gradually turned light green after 3 days. No diffusing pigment.
Conidiophores pyramidal, with a relatively obvious main axis, often rebranching 1–3 times. The branches are usually at right angles to the main axis, and phialides paired or in whorls of 3–4, rarely solitary at the end of the branches. Phialides ampulliform to lageniform, 4.8–9.9 × 2.1–3.4(−3.7) μm, l/w 1.4–3.1(−3.9), (1.1–)1.3–2.3 μm wide at the base (n = 45). Conidia green, smooth, globose to subglobose, 2.7–3.3 × 2.5–3.3 μm, l/w 1.0–1.2 (n = 30). Chlamydospores common, intercalary or terminal, variable in shape, ellipsoid, globose or oblong, 4.8–8.4 × 4.0–7.7 μm (n = 30).
Additional strains examined: China, Beijing, Tongzhou district, 39°41′51” N, 116°45′1″ E, 26 August 2021, W.T. Qin, Z.J. Cao, JZBQT1Z12.
Notes: The two strains of T. caespitosus formed a separate lineage in relation to T. tongzhouense and T. pingquanense. Their morphology was similar to each other, and their tef1–α sequences were nearly the same. Trichoderma caespitosus possessed 33 bp sequence divergences among 1,061 bp for rpb2 (96.89%) from T. tonzhouense (strain JZBQT1Z1) and T. pingquanense (strain JZBQT7Z10). In addition, T. caespitosus form dense conidiophores pustules on CMD and SNA.
Trichoderma citrinoviride Bissett, Can. J. Bot. 62: 926. 1984.
Materials examined: China, Hebei Province, Pingquan, 11 February. 2022, W.T. Qin, X.Q. Wang, JZBQT10Z1, JZBQT10Z2, JZBQT10Z3, JZBQT10Z4; ibid., 3 March 2022, W.T. Qin, X.Q. Wang, JZBQT11Z5, JZBQT11Z6, JZBQT11Z7, JZBQT11Z8.
Notes: Trichoderma citrinoviride belongs to the clade of Longibrachiatum, which has been reported to be associated with L. edodes and P. ostreatus. It grows in a wide range of temperature. The asexual morphology of T. citrinoviride is similar to that of T. atroviride. According to previous study, the invasion ability of T. citrinoviride to L. edodes was weaker than that of T. atroviride (Park et al., 2005; Kim et al., 2012). This study shows that it is a prevalent species in the CSL in northern China.
Trichoderma lentinulae J. Z. Sun & X.Z. Liu, MycoKeys 73: 116. 2020.
Materials examined: China, Beijing, Haidian district, 3 August 2021, W.T. Qin, Q. Gao, Z. J. Cao, JZBQT0Z1, JZBQT0Z2, JZBQT0Z3, JZBQT0Z4; China, Hebei Province, Pingquan, 11 February. 2022, W.T. Qin, X.Q. Wang, JZBQT10Z5, JZBQT10Z6, JZBQT10Z7, JZBQT10Z8.
Notes: Trichoderma lentinulae is first reported in contaminated fruiting bodies, strains, and plant rhizosphere soils, with shorter phialides than close-related species (Gu et al., 2020). Trichoderma lentinulae is the dominant species contamination in the production of L. edodes in Guizhou, China, and its metabolites and volatile products had obvious inhibitory effects on the mycelial growth of L. edodes (Wang, 2021). In this study, the isolation frequency of T. lentinulae was 8.9%, which was a potential threat to the production of L. edodes in northern China.
Trichoderma longibrachiatum Rifai, Mycol. Pap. 116: 42. 1969.
Materials examined: China, Henan Province, Nanyang, 20 April 2021, W.T. Qin, JZBQL50; China, Hebei Province, Pingquan, 8 January. 2022, W.T. Qin, X.Q. Wang, JZBQT8Z1, JZBQT8Z2, JZBQT8Z3, JZBQT8Z7, JZBQT8Z8, JZBQT8Z9.
Notes: Trichoderma longibrachiatum is a species with wide range of sources, which can be found in soil and marine (Jaklitsch and Voglmayr, 2015; Kim et al., 2020). Studies have shown that T. longibrachiatum is also a common competitor of L. edodes with less invasive to L. edodes than T. harzianum. In addition, the growth rate patterns of T. longibrachiatum are a wide range at optimal temperatures (Kim et al., 2012). Pustules formed concentric circles, and T. longibrachiatum diffused yellow pigment, sometimes with white spots.
Trichoderma macrochlamydospora Z. J. Cao & W.T. Qin, sp. nov. (Figure 4).
Figure 4. Trichoderma macrochlamydospora (JZBQT5Z1) cultures at 25°C after 7 days [(A) on CMD, (B) on SNA, (C) on PDA]; (D,E) conidiation pustules (CMD, 7 days); (F,G) conidiophores, phialides, and conidia (SNA, 7 days); (H–J) chlamydospores (PDA, 7 days); (K) conidia (SNA, 7 days); scale bars: (F,G) = 20 μm, (H–J) = 10 μm, (K) = 5 μm.
MycoBank: MB849037.
Type: China, Shanxi Province, Datong, Guangling, 39°47′22” N, 114°18′8″ E, 3 December 2021, Y. Liu, W.T. Qin. (ex-type strain JZBQT5Z1). GenBank accessions: ITS = ON653399, rpb2 = ON649955, tef1-α = ON649902.
Etymology: The specific epithet refers to the large chlamydospore.
Description: On CMD after 72 h, colony radius 67–70 mm at 25°C, 73–74 mm at 30°C, 13–15 mm at 35°C. Colony hyaline, indistinctly zonate, mycelium loose. Aerial hyphae short, inconspicuous. Many white conidia formed after 3 days of incubation, scant, gradually turning blue-green. Pustules abundant, spreading throughout the colony. No diffusing pigment, no distinct odor. On PDA after 72 h, colony radius 58–62 mm at 25°C, 58–66 mm at 30°C, 8–12 mm at 35°C. Colony white-green to green, distinctly zonate, mycelium dense and radial. Aerial hyphae long, abundant, spreading throughout the colony, forming a loose, floccose mat. Conidiation effuse in the aerial hyphae. No diffusing pigment, odor fruity. On SNA after 72 h, colony radius 54–55 mm at 25°C, 55–58 mm at 30°C, 8–10 mm at 35°C. Colony hyaline, circular, distinctly zonate; mycelia loose. Very small amounts of conidia were observed after 3 days of incubation, starting around the inoculum, first white, turning green. No diffusing pigment, no distinct odor.
Conidiophores pyramidal, with a relatively obvious main axis, often rebranching 1–3 times, side branches paired, more or less symmetrical. Phialides typically formed in whorls of 3–4, rarely solitary or paired, variable in shape and size, ampulliform to lageniform, (4.1–)4.7–11 × (2.0–)2.3–3.8 μm, l/w 1.4–5.5(−6.0), 1.2–2.1 μm wide at the base (n = 50). Conidia green, smooth, globose, 2.5–3.4 × 2.5–3.2 μm, l/w 1.0–1.1 (n = 50). Chlamydospores common, intercalary or terminal, variable in shape, ellipsoid, globose or oblong, 5.0–7.8(−10.8) × 4.7–7.9 μm (n = 25).
Additional strains examined: China, Shanxi Province, Datong, Guangling, 39°47′22” N, 114°18′8″ E, 3 Dec.2021, Y. Liu, W.T. Qin, JZBQT5Z2; China, Hebei Province, Chengde, Fengning, 41°14′49” N, 117°3′34″ E, 19 Dec. 2021, S.X. Wang, W.T. Qin, JZBQT6Z1, JZBQT6Z2, JZBQT6Z3, JZBQT6Z4; ibid., 12 Jan. 2022, S.X. Wang, W.T. Qin, JZBQT9Z1, JZBQT9Z2, JZBQT9Z3, JZBQT9Z4.
Notes: In this study, T. macrochlamydospora is a common species with the isolation frequency 15.6% (14/90), indicating a potential threat to the production of L. edodes in the area investigated. Trichoderma macrochlamydospora shares a common ancestor with T. subvermifimicola, T. vermifimicola, and T. simmonsii, and they were similar in conidiophores, similar size and shape of phialides and conidia. Trichoderma macrochlamydospora is most similar to T. subvermifimicola, except larger chlamydospores (4.0–6.9 × 3.5–6.4 μm). As far as sequence divergences are concerned, 27 bp and 30 bp differences among 1,117 bp (97.58%) and 1,117 bp (97.31%) for rpb2 were detected between T. macrochlamydospora (strain JZBQT5Z1) and its closely related species T. subvermifimicola (strain JZBQT4Z1) and T. vermifimicola (strain HMAS 248255), respectively.
Trichoderma notatum Z. J. Cao & W.T. Qin, sp. nov. (Figure 5).
Figure 5. Trichoderma notatum (JZBQT1Z5) cultures at 25°C after 7 days [(A) on CMD, (B) on SNA, (C) on PDA]; (D,E) conidiation pustules (CMD, 7 days); (F–H) conidiophores, phialides, and conidia (SNA, 7 days); (I) conidia (SNA, 7 days); (J) chlamydospores (PDA, 7 days); scale bars: (F) = 20 μm, (G–J) = 10 μm.
MycoBank: MB849038.
Typification: China, Beijing, Tongzhou district, 39°41′51” N, 116°45′1″ E, 26 August 2021, W.T. Qin, Y. Liu, S.X. Wang, (ex-type strain JZBQT1Z5). GenBank accessions: rpb2 = OP832381, tef1-α = OP832396.
Etymology: The specific epithet refers to special sequence distinct from other species.
Description: On CMD after 72 h, colony radius 60–66 mm at 25°C, covering the plate at 30°C, 34–39 mm at 35°C. Colony hyaline, mycelium loose, more abundant with distance from the original inoculum. Aerial hyphae short, inconspicuous. After 2 days, the margin of the colony formed distinctly zonate, with a large number of white pustules. Conidiation formed on aerial hyphae and pustules, and gradually turn pale green. No diffusing pigment. On PDA after 72 h, colony radius 58–61 mm at 25°C, covering the plate at 30°C, 26–28 mm at 35°C. Colony radial, regularly round. Aerial hyphae white and abundant, flocculent in the middle of the colony. Conidiation effuse in the aerial hyphae after 3 days, first white, then turning green. No diffusing pigment, no distinct odor. On SNA after 72 h, colony radius 53–57 mm at 25°C, 59–62 mm at 30°C, 21–23 mm at 35°C. Colony hyaline, regularly circular, distinctly zonate; mycelium loose. Conidiation noted after 2 days, formed in pustules, first white, turning yellow after 3 days. No diffusing pigment.
Conidiophores pyramidal, with obscure main axis sometimes, often rebranching 2–3 times. The branches are usually at right angles to the main axis, and branch ends are often curved. Phialides formed at the tips of branches most in whorls of 2–4. Phialides ampulliform to lageniform, 4.9–9.9(−11.9) × 2.5–4.3 μm, l/w 1.3–3.3(−3.7), 1.2–2.8 μm wide at the base (n = 35). Conidia green, smooth, globose or subglobose, 2.7–3.6 × 2.5–3.1(−3.5) μm, l/w 1.0–1.2 (n = 40). Chlamydospores common, intercalary or terminal, most globose or oblong, rare ellipsoid, 4.9–9.6 × 4.1–8.3 μm (n = 30).
Additional strains examined: China, Beijing, Tongzhou district, 39°41′51” N, 116°45′1″ E, 26 August 2021, W.T. Qin, Y. Liu, S.X. Wang, JZBQT1Z11.
Notes: Trichoderma notatum was distributed as a separate terminal branch differing from other species. Compared with phylogenetically related species T. simmonsii, the phialides of T. notatum were longer [(4.2–)5.2–6.5(−9.0) μm], and its growth rate was slower at 35°C [25–55 mm] (Chaverri et al., 2015). The sequence similarity of rpb2 and tef1-α between strain JZBQT1Z5 and T. simmonsii (strain S7) was 97.97 and 98.21%, with 23 bp and 22 bp differences among 1,061 bp and 1,231 bp, respectively.
Trichoderma paratroviride Jaklitsch & Voglmayr, Stud. Mycol. 80: 75, 2015. Zhang, Zhang, Chen, Li, Guo & Yang, Shandong Sci. 28: 37. 2015.
Materials examined: China, Shanxi Province, Datong, 3 December 2021, Y. Liu, W.T. Qin, JZBQT5Z4, JZBQT5Z6.
Notes: Trichoderma paratroviride is first discovered on wood and bark of broadleaf trees and shrubs (Jaklitsch and Voglmayr, 2015) and isolated from a monitoring of indoor air in the oak mushroom cultivation houses (Ahn et al., 2018). Furthermore, T. paratroviride might have been considered as a competitor to edible fungi in 2012 (Kim et al., 2012). The strain JZBQT5Z4 employed in this study exhibited distinct characteristics from T. paratroviride isolated in Spain, which manifested as white colonies on PDA (Jaklitsch and Voglmayr, 2015), in contrast to the grayish green and pale yellow colonies observed in our study.
Trichoderma paraviridescens Jaklitsch, Samuels & Voglmayr, Persoonia 31: 128. 2013.
= Hypocrea viridescens Jaklitsch & Samuels, in Jaklitsch, Samuels, Dodd, Lu & Druzhinina, Stud. Mycol. 56: 160. 2006.
Materials examined: China, Henan Province, Nanyang, 20 April 2021, Y. Liu, W.T. Qin, JZBQL50; China, Hebei Province, Pingquan, 1 January 2022, Y. Liu, W.T. Qin, JZBQT7Z6, JZBQT7Z8, JZBQT7Z9; ibid., 5 March 2022, Y. Liu, W.T. Qin, JZBQT11Z1.
Notes: Trichoderma paraviridescens is distributed all over the world. Chinese specimens are often collected from rotten wood, plant rhizosphere soil, plant endogenous, and other substrates (Du, 2019). It was characterized by villous dark red stroma in sexual stage, and radial with colony concentric rings, rapid growth at asexual stage. This study was the first report of T. paraviridescens isolated from the CSL.
Trichoderma pingquanense Z. J. Cao & W.T. Qin, sp. nov. (Figure 6).
Figure 6. Trichoderma pingquanense (JZBQT7Z10) cultures at 25°C after 7 days [(A) on CMD, (B) on SNA, (C) on PDA]; (D,E) conidiation pustules (CMD, 7 days); (F–H) conidiophores, phialides, and conidia (SNA, 7 days); (I) conidia (SNA, 7 days); (J,K) chlamydospores (PDA, 7 days); scale bars: (G,H) = 20 μm, (F,J,K) = 10 μm, (I) = 5 μm.
MycoBank: MB849039.
Type: China, Hebei Province, Pingquan, 41°2′6” N, 118°44′1″ E, 1 Jan. 2022, Y. Liu, S.X. Wang, W.T. Qin (ex-type strain JZBQT7Z10). GenBank accessions: ITS = ON653401, rpb2 = ON649961, tef1-α = ON649908.
Etymology: The specific epithet refers to the type locality.
Description: On CMD after 72 h, colony radius 65–69 mm at 25°C, 72–73 mm at 30°C, 13–16 mm at 35°C. Colony hyaline, indistinctly zonate, mycelium loose. Aerial hyphae short, inconspicuous. Small amount of conidial production noted after 4 days, turning blue-green. Pustules are noted throughout the plate. No diffusing pigment, no distinct odor. On PDA after 72 h, colony radius 62–63 mm at 25°C, 66–72 mm at 30°C, 8–9 mm at 35°C. Colony white to white-green, flocculent, mycelium dense and radial. Aerial hyphae long, conspicuous. Small amount of conidial production noted after 3 days, starting around the original inoculum, effuse in the aerial hyphae. No diffusing pigment, odor fruity. On SNA after 72 h, colony radius 51–52 mm at 25°C, 56–59 mm at 30°C, 5 mm at 35°C. Colony hyaline, regularly circular, distinctly zonate; mycelium loose. Conidial production noted after 4 days, starting around the inoculum, first white, turning dark green, with hairs protruding beyond the surface. No diffusing pigment, no distinct odor.
Conidiophores pyramidal, with a relatively obvious main axis, often rebranching 1–3 times. Side branches solitary or paired, in acute or straight angles with main axis, typically formed in a cruciate whorl of 3–5 phialides. Phialides ampulliform to lageniform, 4.3–8.0 × 2.2–3.1(−3.5) μm, l/w 1.6–3.5, 1.3–1.9 μm wide at the base (n = 50). Conidia green, smooth, globose or subglobose, 2.4–3.5 × 2.1–3.0 μm, l/w 1.0–1.2 (n = 50). Chlamydospores common, intercalary or terminal, variable in shape, ellipsoid, globose or oblong, (4.0–)4.7–8.4 × 3.9–7.5 μm (n = 30).
Additional strains examined: China, Hebei Province, Pingquan, 41°2′6” N, 118°44′1″ E, 1 January 2022, Y. Liu, S.X. Wang, W.T. Qin, JZBQT7Z11, JZBQT7Z12.
Notes: Phylogenetically, Trichoderma pingquanense was sister of T. tongzhouense, and they are similar in tef1-α sequence, while the similarity of rpb2 was 97.14% with 32 bp differences among 1,117 bp. It differed from T. xixiacum by conspicuous chlamydospores. Compared with T. tongzhouense and T. auriculariae, T. pingquanense had narrower phialides and base of phialides [T. tongzhouense phialides 2.4–4.3 μm, base of phialides 1.7–2.9 μm, T. auriculariae phialides 2.7–3.8 μm, base of phialides 1.4–2.7 μm].
Trichoderma subvermifimicola Z. J. Cao & W.T. Qin, sp. nov. (Figure 7).
Figure 7. Trichoderma subvermifimicola (JZBQT4Z1) cultures at 25°C after 7 days [(A) on CMD, (B) on SNA, (C) on PDA]; (D,E) conidiation pustules (CMD, 7 days); (F–H) conidiophores, phialides, and conidia (SNA, 7 days); (I,J) conidia (SNA, 7 days); (K,L) chlamydospores (PDA, 7 days); scale bars: (F,H) = 20 μm, (G,K,L) = 10 μm, (I,J) = 5 μm.
MycoBank: MB849041.
Type: China, Hebei Province, Baoding, Fuping, 38°53′13” N, 114°0′41″ E, 28 November 2021, Y. Liu, W.T. Qin (ex-type strain JZBQT4Z1). GenBank accessions: ITS = ON653398, rpb2 = ON649952, tef1-α = ON649899.
Etymology: The specific epithet refers to the similarity of the fungus to T. vermifimicola.
Description: On CMD after 72 h, colony radius 63–66 mm at 25°C, 68–70 mm at 30°C, and 14–16 mm at 35°C. Colony hyaline, regularly circular, radial. Conidial production noted after 3 days, scant, effuse in aerial hyphae, concentric circles around the inoculum can be noticed. Sometimes diffusing yellow pigment, no distinct odor. On PDA after 72 h, colony radius 60–64 mm at 25°C, 63–70 mm at 30°C, 13–17 mm at 35°C. Colony white, zonate, mycelium dense and radial. Aerial hyphae long, abundant, spreading throughout the colony, forming a loose, floccose mat. Conidiation noted after 3 days, starting around the original inoculum, effuse in the aerial hyphae. Sometimes diffusing yellow pigment, odor fruity. On SNA after 72 h, colony radius 47–53 mm at 25°C, 54–55 mm at 30°C, 5–7 mm at 35°C. Colony hyaline, indistinctly zonate, mycelium loose. Aerial hyphae loose. Conidiation noted after 3 days, forming conspicuous concentric circles. No diffusing pigment, no distinct odor.
Conidiophores typically pyramidal, symmetry, often with a main axis. Side branches in straight angles with main axis, solitary or paired, rebranching 1–3 times. Branches are perpendicular to the main axis. Phialides formed solitary or paired, and often formed in cruciate whorls of 3–4 at the end of the branch, ampulliform to lageniform, often constricted below the tip to form a narrow neck, (4.2–)4.7–9.4 × 2.3–3.6 μm, l/w 1.4–3.9, (1.2–)1.4–2.1 μm wide at the base (n = 50). Conidia green, smooth, globose, sometimes subglobose, 2.7–3.3 × 2.5–3.0 μm, l/w 1.0–1.2(−1.3) (n = 50). Chlamydospores common, intercalary or terminal, ellipsoid, globose, 4.0–6.9 × 3.5–6.4 μm (n = 30).
Additional strains examined: China, Hebei Province, Baoding, Fuping, 38°53′13” N, 114°0′41″ E, 28 November 2021, Y. Liu, W.T. Qin, JZBQT4Z2, JZBQT4Z3, JZBQT4Z4, JZBQT4Z6; China, Hebei Province, Pingquan, 41°2′6” N, 118°44′1″ E, 11 Feb. 2022, W.T. Qin, X.Q. Wang, JZBQT10Z14; ibid., 5 Mar. 2022, Y. Liu, W.T. Qin, JZBQT11Z2, JZBQT11Z3, JZBQT11Z4.
Notes: Trichoderma subvermifimicola is characterized by typically pyramidal and symmetry conidiophores. Phylogenetically, T. subvermifimicola is closely associated with T. vermifimicola. However, they possess 23 bp sequence divergency among 1,206 bp (98.09%) for rpb2. Trichoderma subvermifimicola exclusively forms obvious concentric circles on CMD and differs from T. vermifimicola by larger conidia [(2.0–)2.3–2.6(−3.0) × (1.5–)2.0–2.4(−2.8) μm], and more chlamydospores observed (Gu et al., 2020).
Trichoderma subviride W.T. Qin & W.Y. Zhuang, Scientific Reports 6(27074): 11. 2016.
Material examined: China, Hebei Province, Pingquan, 8 January 2022, W.T. Qin, X.Q. Wang, S.X. Wang, JZBQT8Z10, JZBQT8Z11, JZBQT8Z12.
Notes: Trichoderma subviride was first found on twigs in Henan, China (Qin and Zhuang, 2016), and this is the first report of T. subviride isolated from L. edodes substrates. Trichoderma subviride was featured by pale yellow to yellow green conidiation zones with a squamose surface on PDA (Qin and Zhuang, 2016). Although only three strains among 90 strains investigated were recognized as T. subviride, it was still a potential threat to the production of L. edodes.
Trichoderma tongzhouense Z. J. Cao & W.T. Qin, sp. nov. (Figure 8).
Figure 8. Trichoderma tongzhouense (JZBQT1Z1) cultures at 25°C after 7 days [(A) on CMD, (B) on SNA, (C) on PDA]; (D,E) conidiation pustules (CMD, 7 days); (F–H) conidiophores, phialides, and conidia (SNA, 7 days); (I,J) chlamydospores (PDA, 7 days); (K) conidia (SNA, 7 days); scale bars: (G) = 20 μm, (F,H) = 10 μm, (I–K) = 5 μm.
MycoBank: MB849042.
Type: China, Beijing, Tongzhou district, 39°41′51” N, 116°45′1″ E, 26 August 2021, W.T. Qin, Z.J. Cao, L. Gao, J. Li (ex-type strain JZBQT1Z1). GenBank accessions: ITS = ON653394, rpb2 = ON649945, tef1-α = ON649892.
Etymology: The specific epithet refers to the type locality.
Description: On CMD after 72 h, colony radius 62–70 mm at 25°C, 63–68 mm at 30°C, and 17–20 mm at 35°C. Colony hyaline, circular, weak, mycelium loose. Aerial hyphae inconspicuous, and it was abundant at 30°C. Distinct yellow wheel-like areas appeared at 35°C. Conidia began to appear after 4 days, forming indistinct concentric circles at first, then small pustules spreaded over the entire plate. No diffusing pigment noted, no distinct odor. On PDA after 72 h, colony radius 59–63 mm at 25°C and 62–65 mm 30°C, 13–17 at 35°C. Mycelium white, dense and radial. Aerial hyphae abundant, spreading throughout the colony, forming a loose, floccose mat. Conidia effuse in the aerial hyphae after 3–4 days. No diffusing pigment noted, odor fruity. On SNA after 72 h, colony radius 50–52 mm at 25°C, 54–57 mm at 30°C, 13–15 at 35°C. Colony similar to CMD, mycelium hyaline and smooth, less aerial hyphae. Conidia appeared after 4 days, starting around the inoculum, then turn to green pustules, with the formation of 2–3 concentric rings. No diffusing pigment, no distinct odor.
Conidiophores pyramidal, typically asymmetry, irregularly branched, rebranching 1–3 times. Phialides mostly symmetrically arranged, solitary or divergent in whorls of 2–4(−6), variable in shape and size, ampulliform to lageniform, straight or slightly curved or sinuous, 4.7–13.1(−16.0) × 2.4–4.3 μm, l/w 1.4–4.4(−4.8), 1.7–2.9 μm wide at the base (n = 50). Conidia green, globose or subglobose, sometimes ellipsoidal, smooth, 2.7–3.5(−3.8) × 2.4–3.5 μm, l/w 1.0–1.2(−1.3) (n = 50). After 10 days, chlamydospores were readily visible at PDA, terminal or intercalary, mostly globose or subglobose, ellipsoidal or fusoid, 5.3–9.2 × 5.2–8.7 μm (n = 40).
Additional strains examined: China, Beijing, Tongzhou district, 39°41′51” N, 116°45′1″ E, 26 August 2021, W.T. Qin, Z.J. Cao, L. Gao, J. Li, JZBQT1Z2, JZBQT1Z3, JZBQT1Z4.
Notes: Phylogenetically, T. tongzhouense is sister of T. pingquanense in the Harzianum Clade. Trichoderma tongzhouense possess 32 bp among 1,117 bp rpb2 sequence difference (97.14%) from T. pingquanense (strain JZBQT7Z10). Morphologically, T. tongzhouense is similar to T. pingquanense in characteristics of colonies and microscopic morphologies, but the phialide base of T. tongzhouense is wider than that of T. pingquanense [1.3–1.9 μm]. Differs from T. xixiacum by longer phialides [3.5–7.0 μm] and larger conidia [2.3–2.7 × 2.0–2.6 μm].
The resistance of L. edodes to two Trichoderma species was tested in dual culture. The results showed that both T. macrochlamydospora and T. subvermifimicola showed strong inhibition on L. edodes. The inhibitory rates of T. subvermifimicola against L. edodes ranged from 47.04% (L. edodes strain SX8) to 58.36% (L. edodes strain LHT2). Significance analysis showed that T. macrochlamydospora inhibited slightly on L. edodes strain SX8 and strain SX10 (Supplementary Figure S2). The inhibitory rates for T. macrochlamydospora ranged from 43.27% (L. edodes strain SX8) to 58.41% (L. edodes strain LHT2). Significance analysis showed that T. macrochlamydospora inhibited slightly on L. edodes strain SX8 and strain K21 × Z9 (Supplementary Figure S3).
Trichoderma strains grow approximately faster than L. edodes. At the initial stage of hyphae contacted between Trichoderma and L. edodes, Trichoderma spp. strongly inhibited the growth of hyphae of L. edodes. Trichoderma had also become slow growing, but it was still able to slowly overgrew the territory of L. edodes and sporulate abundantly in most plates in 13 days. Lentinula edodes would secrete more brown substances on most of the plates, and obvious brown antagonistic lines or entire brown colony of L. edodes could be seen on the back of the plates (Figure 9). Simultaneously, both of Trichoderma species showed adhesion or coil growth to hyphae of L. edodes without significant difference (Figure 10). At times, it was observed that the vesicular structure on the mycelium of L. edodes was broken and brown substance flowed out (Figure 10).
Figure 9. Antagonistic ability of L. edodes (left) against Trichoderma strains (right) in dual culture. (A–D) + 1, L. edodes strains only; (E) + 2–5, Trichoderma strains only; [(A–D) + 2, 4] the upper view of the plate in dual culture; [(A–D) + 3, 5] the reverse view of the plate in dual culture.
Figure 10. Hyphal state of L. edodes contacted with Trichoderma spp. (A) production of hyphal coilings by T. subvermifimicola to L. edodes strain SX8; (B) production of hyphal coilings by T. subvermifimicola to L. edodes strain LHT2; (C) L. edodes strain SN602 exuded brown substance; (D) production of hyphal coilings by T. macrochlamydospora to L. edodes strain SX8; (E) production of hyphal coilings by T. macrochlamydospora to L. edodes strain LHT2; (F) L. edodes strain XY exuded brown substance; red arrows indicate hyphae of Trichoderma spp., and black arrows indicate hyphae of L. edodes; scale bars = 100 μm.
Since the artificial cultivation of edible fungi, the serious green mold contamination caused by Trichoderma spp. in various growth stage of edible fungi has always been one of the biggest biological constraints in edible fungus industry. Therefore, from 2020 to 2022, edible fungus sticks contaminated with green mold were collected from four provinces of China. The diversity of Trichoderma species was relatively abundant, with 90 strains belonging to 14 species. Among them, eight species were located in the clade of Harzianum, two species belonged to the Longibrachiatum clade, and four attributed to the Viride clade. The emergence of green mold contaminated substrates of edible fungi undoubtedly constitutes a prominent hotspot for the diversity of Trichoderma species. Six out of the 14 species in the Harzianum clade have been newly established and typified for the first time. Three known Trichoderma species, namely, T. auriculariae, T. paraviridescens and T. subviride were isolated from CSL for the first time in the world, and T. paratroviride was firstly reported to be associated with L. edodes in China. Trichoderma atroviride, T. macrochlamydospora, and T. subvermifimicola were the three most frequently isolated species from the CSL. Consequently, this study lays a foundation for comprehensively understanding Trichoderma spp. in the CSL.
Following the gradual refinement and extensive implementation of DNA-based methodologies, the polyphasic taxonomic approach has been advocated for species delimitation (Druzhinina and Kubicek, 2005; Luecking et al., 2020). With the advancement of Trichoderma classification research, researchers have such a consensus that the morphological identification of Trichoderma with high ambiguity is not suitable for accurate identification of this genus (Chaverri and Samuels, 2003; Jaklitsch et al., 2006). The new species in this study have similar morphological characteristics to some close-related species on the phylogenetic tree. Consequently, phylogenetic analysis became a powerful method to ascertain the placement of ambiguous species, especially for species complexes and cryptic species (Li et al., 2013). According to the species delimitation of Chaverri et al. (2015), most of the new species identified in this study would be considered Trichoderma harzianum species complex. In this study, we provided more examples of the strong biocontrol effect of T. harzianum complex by showing strong inhibition of T. subvermifimicola and T. macrochlamydospora in the dual culture with L. edodes. In addition, Kim et al. (2012) divided isolated strains into two groups of T. atroviride by whether formed a dark green ring pustular band in SNA culture. In this study, we found that T. atroviride JZBQT7Z1 (JZBQT7Z2, JZBQT7Z3) was more likely to form green rings on PDA than T. atroviride JZBQT8Z4 (JZBQT8Z5, JZBQT8Z6), which may also be the result of the accumulation of genetic variations within T. atroviride. Recent studies and the results in this study enriched the species around T. lixii and T. simmonsii in the phylogenetic tree, while the relationships between members were more sophisticated, needing further research (Gu et al., 2020).
Trichoderma spp. play critical roles in biological control of plant diseases through antagonism against plant pathogens. Trichoderma spp. inhabitated in soil, rhizosphere or internal tissues of plant have a variety of mechanisms to protect plants from diseases. One of the most common mechanisms is competition for space and nutrients. In this study, the resistance of L. edodes to Trichoderma spp. was evaluated, and the results indicated that some Trichoderma species also are able to coil around and suck the hyphae of L. edodes to make it swell, deform, or even dissolute. They parasitize competing fungi through the production of volatile organic compounds, lytic enzymes, etc., and also can stimulate the defense mechanisms of plant (Nuangmek et al., 2021; Rajani et al., 2021). Meanwhile, volatile and fermented metabolites of Trichoderma species also have significant inhibitory effect on the growth of mycelia and fruiting bodies of L. edodes (Bruce et al., 1984; Wu et al., 2007). For example, most Trichoderma species can produce secondary metabolites and degrading enzymes that can dissolve host cell walls. Nonetheless, the interaction between edible fungi and pathogenic fungi is still a very complex process (Ma et al., 2021). In this study, the potential of L. edodes varieties with strong resistance to the dominant Trichoderma species on contaminated substrates was screen out. The L. edodes strains showed varying degrees of resistance to the two new Trichoderma species and L. edodes strain SX8 relatively higher resistant. The inhibitory rates of Trichoderma against L. edodes may be influenced by the growth rate of L. edodes mycelium, which should be mentioned. In addition, compared with other edible fungi such as oyster mushroom, almost all varieties of L. edodes generally have poor resistance to Trichoderma spp. (Wu et al., 2007), which were consistent with our results. Unfortunately, no significant morphology characteristics were found to be related to the infection ability of Trichoderma or the resistance of L. edodes. Trichoderma sometimes produced fewer green conidia, such as in dual culture of T. subvermifimicola against L. edodes strain SX10 (Figure 9B2). Moreover, the brown substances secreted by L. edodes were less observed on the back of some plates, such as in dual culture of T. macrochlamydospora and L. edodes strain K21 × Z9 (Figure 9D5). Such differences can exist between different repeats of the same dual culture. However, this phenomenon is difficult to explain in previous studies, which showed that strong resistance of Trichoderma to plant pathogenic fungi was associated with the more abundant sporulation of Trichoderma (Zhang and Zhuang, 2017). The presence of these uncertainties suggests that the inhibitory effect of Trichoderma on L. edodes might not be directly linked to sporulation, and the conidium count of Trichoderma does not seem to have a direct correlation with the brown substance secreted by L. edodes.
This also makes it difficult to distinguish the differences between Trichoderma and different L. edodes varieties through inhibitory rate, brown substance secreted by L. edodes and sporulation by Trichoderma in this study. The investigation of various Trichoderma spp. on the resistance mechanism of edible fungi will contribute to the research on the resistance of edible fungi against Trichoderma (Ma et al., 2019). Considerably, extensive efforts will need to be made to screen and culture-resistant strains of L. edodes against Trichoderma by breeding techniques. Presently, to minimize Trichoderma spp. contamination, it is proposed to examine the Trichoderma occurrence mechanism, including its correlation with mushroom substrate and growing environment. Furthermore, the development of an early monitoring system for the predominant Trichoderma species is crucial, along with the refinement of cultivation techniques and the identification of highly efficient, low-toxic fungicides.
Remarkably green mold contaminated substrates of edible fungi were undoubtedly a hotspot of Trichoderma species diversity. Until now, there are 31 Trichoderma species have been reported to be associated with the CSL (Supplementary Table S4). However, our knowledge of the group in the substrates of edible fungi is far from sufficient compared with other origins, such as rotten wood (Zhu and Zhuang, 2015; Qin and Zhuang, 2016) and soil (Chen and Zhuang, 2017). Comprehensive surveys in unexplored territories or alternative edible fungus hosts are undoubtedly necessary.
To assess the biodiversity of Trichoderma in the CSL in China, 150 samples were collected from four provinces of China during a two-year investigation. A total of 90 isolates were obtained and identified as 14 Trichoderma species, and their phylogenetic positions were determined, among which six Trichoderma species were recognized as new. Three known species, namely, T. auriculariae, T. paraviridescens and T. subviride were isolated from CSL for the first time in the world, and T. paratroviride was firstly reported to be associated with L. edodes in China. Furthermore, this study systematically investigated Trichoderma species in the CSL, and a total of 31 species so far have been reported, indicating that green mold contaminated substrates of edible fungi were undoubtedly a biodiversity hotspot of Trichoderma species. In addition, resistance of L. edodes to the dominant Trichoderma species was evaluated in vitro, and the results showed that strains of L. edodes generally showed poor resistance to Trichoderma contamination with L. edodes strain SX8 relatively higher resistant. Results in this study will provide deeper insight into the genus Trichoderma and lay a strong foundation for scientific management of the Trichoderma contamination in the production of L. edodes.
The data presented in the study are deposited in Mycobank repository (https://www.mycobank.org/), accession number MB849036, MB849037, MB849038, MB849039, MB849041, MB849042.
Z-JC: Data curation, Software, Visualization, Writing – original draft. JZ: Formal analysis, Writing – review & editing. YL: Project administration, Investigation. S-XW: Resources. S-YZ: Supervision. W-TQ: Funding acquisition, Conceptualization, Supervision, Writing – review & editing.
The author(s) declare financial support was received for the research, authorship, and/or publication of this article. This study was financially supported by the Beijing Academy of Agriculture and Forestry Sciences, China (KJCX20220415), the National Natural Science Foundation of China (32002106), and the Beijing Municipal Natural Science Foundation (6232009).
The authors are thankful to Yayong Liu for language refinement, Xinghong Li and Wei Zhang for technical assistance, and all sample collectors for sampling in this study.
The authors declare that the research was conducted in the absence of any commercial or financial relationships that could be construed as a potential conflict of interest.
All claims expressed in this article are solely those of the authors and do not necessarily represent those of their affiliated organizations, or those of the publisher, the editors and the reviewers. Any product that may be evaluated in this article, or claim that may be made by its manufacturer, is not guaranteed or endorsed by the publisher.
The Supplementary material for this article can be found online at: https://www.frontiersin.org/articles/10.3389/fmicb.2023.1288585/full#supplementary-material
Ahn, G. R., Kim, J. E., Kim, J. Y., and Kim, S. H. (2018). Unrecorded fungal species isolated from indoor air in the log bed- and sawdust media-based mushroom cultivation houses. Kor. J. Mycol. 46, 495–503. doi: 10.4489/KJM.20180054
Allaga, H., Zhumakayev, A., Buchner, R., Kocsube, S., Szucs, A., Vagvolgyi, C., et al. (2021). Members of the Trichoderma harzianum species complex with mushroom pathogenic potential. Agronomy-Basel 11:2434. doi: 10.3390/agronomy11122434
Barrera, V. A., Iannone, L., Romero, A. I., and Chaverri, P. (2021). Expanding the Trichoderma harzianum species complex: three new species from argentine natural and cultivated ecosystems. Mycologia 113, 1136–1155. doi: 10.1080/00275514.2021.1947641
Bruce, A., Austin, W. J., and King, B. (1984). Control of growth of Lentinus lepideus by volatiles from Trichoderma. Trans. Br. Mycol. Soc. 82, 423–428. doi: 10.1016/S0007-1536(84)80005-4
Cai, F., and Druzhinina, I. S. (2021). In honor of john bissett: authoritative guidelines on molecular identification of Trichoderma. Fungal Divers. 107, 1–69. doi: 10.1007/s13225-020-00464-4
Cao, Z. J., Qin, W. T., Zhao, J., Liu, Y., Wang, S. X., and Zheng, S. Y. (2022). Three new Trichoderma species in Harzianum clade associated with the contaminated substrates of edible fungi. J. Fungi 8:1154. doi: 10.3390/jof8111154
Carbone, I., and Kohn, L. M. (1999). A method for designing primer sets for speciation studies in filamentous ascomycetes. Mycologia 91, 553–556. doi: 10.2307/3761358
Chaverri, P., Branco-Rocha, F., Jaklitsch, W., Gazis, R., Degenkolb, T., and Samuels, G. J. (2015). Systematics of the Trichoderma harzianum species complex and the re-identification of commercial biocontrol strains. Mycologia 107, 558–590. doi: 10.3852/14-147
Chaverri, P., and Samuels, G. J. (2003). Hypocrea/Trichoderma (ascomycota, hypocreales, hypocreaceae): species with green ascospores. Stud. Mycol. 48, 1–116. doi: 10.1023/B:MYCO.0000003579.48647.16
Chen, K., and Zhuang, W. Y. (2017). Discovery from a large-scaled survey of Trichoderma in soil of China. Sci. Rep. 7:9090. doi: 10.1038/s41598-017-07807-3
Chinese Edible Fungus A (2022). Analysis of the results of the national statistical survey on edible fungi in 2020. Edible Fungi China 41, 85–91. doi: 10.13629/j.cnki.53-1054.2022.01.017
Chomnunti, P., Hongsanan, S., Aguirre-Hudson, B., Tian, Q., Persoh, D., Dhami, M. K., et al. (2014). The sooty moulds. Fungal Divers. 66, 1–36. doi: 10.1007/s13225-014-0278-5
Cunningham, C. W. (1997). Can three incongruence tests predict when data should be combined? Mol. Biol. Evol. 14, 733–740. doi: 10.1093/oxfordjournals.molbev.a025813
Druzhinina, I., and Kubicek, C. P. (2005). Species concepts and biodiversity in Trichoderma and Hypocrea: from aggregate species to species clusters? J Zhejiang Univ Sci B 6, 100–112. doi: 10.1631/jzus.2005.B0100
Du, X. (2019). Study on species diversity and differentiation of Trichoderma in aquatic plants. Master thesis, Yunnan University, Kunming, China.
Fu, Y. C., Hong, J. E., and Gao, Q. J. (1981). Experiments on the control of fungi in cultivated species of Lentinula edodes. Fujian Agric. Sci. Technol. 4:34.
Gu, X., Wang, R., Sun, Q., Wu, B., and Sun, J. Z. (2020). Four new species of Trichoderma in the Harzianum clade from northern China. Mycokeys 73, 109–132. doi: 10.3897/mycokeys.73.51424
Hatvani, L., Antal, Z., Manczinger, L., Szekeres, A., Druzhinina, I. S., Kubicek, C. P., et al. (2007). Green mold diseases of Agaricus and Pleurotus spp. are caused by related but phylogenetically different Trichoderma species. Phytopathology 97, 532–537. doi: 10.1094/PHYTO-97-4-0532
He, Z. D. (2019). Contribution of China’s edible fungi export trade to economic growth. Edible Fungi China 38, 73–76. doi: 10.13629/j.cnki.53-1054.2019.10.022
Jaklitsch, W. M., Komon, M., Kubicek, C. P., and Druzhinina, I. S. (2005). Hypocrea voglmayrii sp. nov. from the Austrian alps represents a new phylogenetic clade in Hypocrea/Trichoderma. Mycologia 97, 1365–1378. doi: 10.3852/mycologia.97.6.1365
Jaklitsch, W. M., Samuels, G. J., Dodd, S. L., Lu, B. S., and Druzhinina, I. S. (2006). Hypocrea rufa/Trichoderma viride: a reassessment, and description of five closely related species with and without warted conidia. Stud. Mycol. 56, 135–177. doi: 10.3114/sim.2006.56.04
Jaklitsch, W. M., and Voglmayr, H. (2015). Biodiversity of Trichoderma (Hypocreaceae) in southern Europe and macaronesia. Stud. Mycol. 80, 1–87. doi: 10.1016/j.simyco.2014.11.001
Jiang, H. F., Wang, F. L., and Tan, Q. (1995). Pathogen species and dominant species of Trichoderma on Lentinula edodes. Acta Agric. Shanghai 11, 85–90.
Kim, K., Heo, Y. M., Jang, S., Lee, H., Kwon, S. L., Park, M. S., et al. (2020). Diversity of Trichoderma spp. in marine environments and their biological potential for sustainable industrial applications. Sustainability 12:4327. doi: 10.3390/su12104327
Kim, C. S., Park, M. S., Kim, S. C., Maekawa, N., and Yu, S. H. (2012). Identification of Trichoderma, a competitor of shiitake mushroom (Lentinula edodes), and competition between Lentinula edodes and Trichoderma species in Korea. Plant Pathol. J. 28, 137–148. doi: 10.5423/PPJ.2012.28.2.137
Kim, J. Y., Yun, Y. H., Hyun, M. W., Kim, M. H., and Kim, S. H. (2010). Identification and characterization of Gliocladium viride isolated from mushroom fly infested oak log beds used for shiitake cultivation. Mycobiology 38, 7–12. doi: 10.4489/MYCO.2010.38.1.007
Lee, S. H., Jung, H. J., Hong, S. B., Choi, J. I., and Ryu, J. S. (2020). Molecular markers for detecting a wide range of Trichoderma spp. that might potentially cause green mold in Pleurotus eryngii. Mycobiology 48, 313–320. doi: 10.1080/12298093.2020.1785754
Li, Q. R., Tan, P., Jiang, Y. L., Hyde, K. D., Mckenzie, E. H. C., Bahkali, A. H., et al. (2013). A novel Trichoderma species isolated from soil in Guizhou, T. Guizhouense. Mycol. Prog. 12, 167–172. doi: 10.1007/s11557-012-0821-2
Liu, Y. J., Whelen, S., and Benjamin, D. H. (1999). Phylogenetic relationships among ascomycetes: evidence from an RNA polymerse II subunit. Mol. Biol. Evol. 16, 1799–1808. doi: 10.1093/oxfordjournals.molbev.a026092
Luecking, R., Aime, M. C., Robbertse, B., Miller, A. N., Ariyawansa, H. A., Aoki, T., et al. (2020). Unambiguous identification of fungi: where do we stand and how accurate and precise is fungal DNA barcoding? Ima Fungus 11:14. doi: 10.1186/s43008-020-00033-z
Ma, X. L., Fan, X. L., Wang, G. Z., Xu, R. P., Yan, L. L., Zhou, Y., et al. (2021). Enhanced expression of thaumatin-like protein gene (letlp1) endows resistance to Trichoderma atroviride in Lentinula edodes. Life Basel 11:863. doi: 10.3390/life11080863
Ma, X. L., Wang, G. Z., Fan, X. L., and Bian, Y. B. (2019). Interaction between edible fungi and Trichoderma spp.: a review. Microbiol. China 46, 184–191. doi: 10.13344/j.microbiol.china.180265
Nuangmek, W., Aiduang, W., Kumla, J., Lumyong, S., and Suwannarach, N. (2021). Evaluation of a newly identified endophytic fungus, Trichoderma phayaoense for plant growth promotion and biological control of gummy stem blight and wilt of muskmelon. Front. Microbiol. 12:634772. doi: 10.3389/fmicb.2021.634772
Nylander, J. A. A. (2004). MrModeltest v2. Program distributed by the author. Evolutionary Biology Centre, Uppsala University.
Park, M. S., Seo, G. S., Bae, K. S., and Yu, S. H. (2005). Characterization of Trichoderma spp. associated with green mold of oyster mushroom by PCR-RFLP and sequence analysis of its regions of rDNA. Plant Pathol. J. 21, 229–236. doi: 10.5423/PPJ.2005.21.3.229
Qin, W. T., and Zhuang, W. Y. (2016). Seven wood-inhabiting new species of the genus Trichoderma (Fungi, Ascomycota) in Viride clade. Sci. Rep. 6:27074. doi: 10.1038/srep30100
Rajani, P., Rajasekaran, C., Vasanthakumari, M. M., Olsson, S. B., Ravikanth, G., and Shaanker, R. U. (2021). Inhibition of plant pathogenic fungi by endophytic Trichoderma spp. through mycoparasitism and volatile organic compounds. Microbiol. Res. 242:126595. doi: 10.1016/j.micres.2020.126595
Ronquist, F., and Huelsenbeck, J. P. (2003). Mrbayes 3: Bayesian phylogenetic inference under mixed models. Bioinformatics 19, 1572–1574. doi: 10.1093/bioinformatics/btg180
Skoneczny, D., Oskiera, M., Szczech, M., and Bartoszewski, G. (2015). Genetic diversity of Trichoderma atroviride strains collected in Poland and identification of loci useful in detection of within-species diversity. Folia Microbiol. 60, 297–307. doi: 10.1007/s12223-015-0385-z
Swofford, D. L. (2002). Paup*. Phylogenetic analysis using parsimony (*and other methods); version 4.0 ; Sinauer Associates: Sunderland, MA.
Thompson, J. D., Gibson, T. J., Plewniak, F., Jeanmougin, F., and Higgins, D. G. (1997). The clustal X windows interface: flexible strategies for multiple sequence alignment aided by quality analysis tools. Nuclc Acids Symposium Series 25, 4876–4882. doi: 10.1093/nar/25.24.4876
Wang, F. L. (1994). Research trends of Trichoderma of both domestic and overseas. Anhui Sci. Technol. 3, 10–11. doi: CNKI:SUN:AHKJ.0.1994-03-004
Wang, Y. (2021). Identification of the pathogen of Lentinus edodes sticks rot and preliminary study on its occurrence. Master thesis, Guizhou University, Guiyang, China
Wang, G. Z., Cao, X. T., Ma, X. L., Guo, M. P., Liu, C. H., Yan, L. L., et al. (2016). Diversity and effect of Trichoderma spp. associated with green mold disease on Lentinula edodes in China. Microbiology 5, 709–718. doi: 10.1002/mbo3.364
Wu, X. J., Zhan, X. Y., and Wu, X. P. (2007). Infection of Trichoderma spp. on edible fungi. Fujian. J. Agric. Sci. 22, 354–359. doi: 10.19303/j.issn.1008-0384.2007.04.005
Yan, D., Gao, Q., Rong, C. B., Liu, Y., Song, S., Yu, Q. Y., et al. (2021). Comparative transcriptome analysis of abnormal cap and healthy fruiting bodies of the edible mushroom Lentinula edodes. Fungal Genet. Biol. 156:103614. doi: 10.1016/j.fgb.2021.103614
Yan, D., Liu, Y., Rong, C. B., Song, S., Zhao, S., Qin, L. M., et al. (2020). Characterization of brown film formed by Lentinula edodes. Fungal Biol. 124, 135–143. doi: 10.1016/j.funbio.2019.12.008
Yan, Y., Zhang, C., Moodley, O., Zhang, L., and Xu, J. Z. (2019). Green mold caused by Trichoderma atroviride on the lingzhi medicinal mushroom, Ganoderma lingzhi (agaricomycetes). Int. J. Med. Mushrooms 21, 515–521. doi: 10.1615/IntJMedMushrooms.2019030352
Yu, S., Wang, R., Liu, H. W., Zhang, X., and Sun, J. Z. (2022). Trichoderma bombaxalis sp. nov., isolated from rhizosphere soils of Lycium barbarum. Phytotaxa 547, 147–157. doi: 10.11646/phytotaxa.547.2.2
Zhang, J. X., Chen, Q., Huang, C. Y., Gao, W., and Qu, J. B. (2015). History, current situation and trend of edible mushroom industry development. Mycosystema 34, 524–540. doi: 10.13346/j.mycosystema.150076
Zhang, Y. B., and Zhuang, W. Y. (2017). First step evaluation of Trichoderma antagonism against plant pathogenic fungi in dual culture. Mycosystema 36, 1251–1259. doi: 10.13346/j.mycosystema.170074
Zhang, Y. B., and Zhuang, W. Y. (2019). Trichoderma bomiense and T. Viridicollare, two new species forming. Mycosystema 38, 11–22. doi: 10.13346/j.mycosystema.180304
Zhao, S., Gao, Q., Rong, C. B., Wang, S. X., Zhao, Z. K., Liu, Y., et al. (2020). Immunomodulatory effects of edible and medicinal mushrooms and their bioactive immunoregulatory products. J. Fungi 6:269. doi: 10.3390/jof6040269
Zheng, H., Qiao, M., Lv, Y. F., Du, X., Zhang, K. Q., and Yu, Z. F. (2021). New species of Trichoderma isolated as endophytes and saprobes from Southwest China. J. Fungi 7:467. doi: 10.3390/jof7060467
Keywords: Hypocreaceae, Trichoderma, green mold, Lentinula edodes, phylogeny, morphology, taxonomy
Citation: Cao Z-J, Zhao J, Liu Y, Wang S-X, Zheng S-Y and Qin W-T (2024) Diversity of Trichoderma species associated with green mold contaminating substrates of Lentinula edodes and their interaction. Front. Microbiol. 14:1288585. doi: 10.3389/fmicb.2023.1288585
Received: 04 September 2023; Accepted: 06 December 2023;
Published: 08 January 2024.
Edited by:
Amit Kumar, Nanjing University of Information Science and Technology, ChinaReviewed by:
Dian-Ming Hu, Jiangxi Agricultural University, ChinaCopyright © 2024 Cao, Zhao, Liu, Wang, Zheng and Qin. This is an open-access article distributed under the terms of the Creative Commons Attribution License (CC BY). The use, distribution or reproduction in other forums is permitted, provided the original author(s) and the copyright owner(s) are credited and that the original publication in this journal is cited, in accordance with accepted academic practice. No use, distribution or reproduction is permitted which does not comply with these terms.
*Correspondence: Wen-Tao Qin, cWlud2VudGFvQGlwcGJhYWZzLmNu
Disclaimer: All claims expressed in this article are solely those of the authors and do not necessarily represent those of their affiliated organizations, or those of the publisher, the editors and the reviewers. Any product that may be evaluated in this article or claim that may be made by its manufacturer is not guaranteed or endorsed by the publisher.
Research integrity at Frontiers
Learn more about the work of our research integrity team to safeguard the quality of each article we publish.