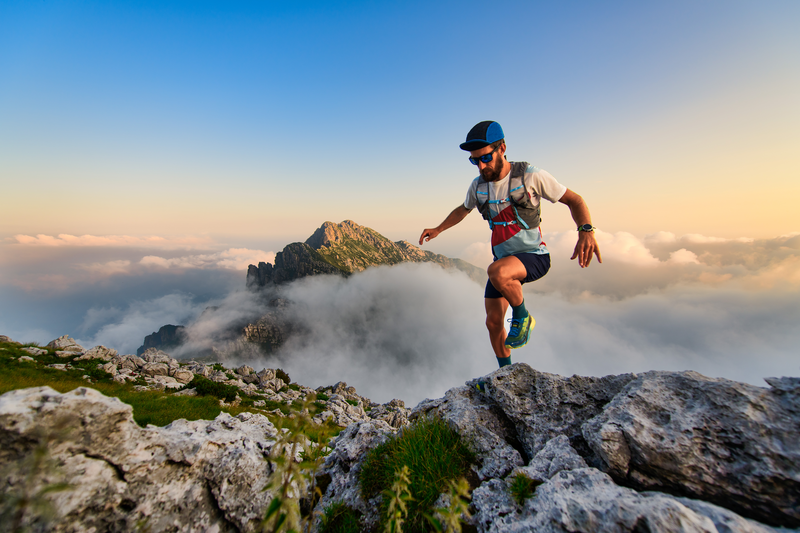
95% of researchers rate our articles as excellent or good
Learn more about the work of our research integrity team to safeguard the quality of each article we publish.
Find out more
REVIEW article
Front. Microbiol. , 04 October 2023
Sec. Microbiotechnology
Volume 14 - 2023 | https://doi.org/10.3389/fmicb.2023.1284369
This article is part of the Research Topic Sustainable Nitrogen Removal in Emerging Pollutant Contaminated Wastewater: Technology, Application and Risk Assessment View all 10 articles
Excessive nitrogen emissions are a major contributor to water pollution, posing a threat not only to the environment but also to human health. Therefore, achieving deep denitrification of wastewater is of significant importance. Traditional biological denitrification methods have some drawbacks, including long processing times, substantial land requirements, high energy consumption, and high investment and operational costs. In contrast, the novel bio-denitrification technology reduces the traditional processing time and lowers operational and maintenance costs while improving denitrification efficiency. This technology falls within the category of environmentally friendly, low-energy deep denitrification methods. This paper introduces several innovative bio-denitrification technologies and their combinations, conducts a comparative analysis of their denitrification efficiency across various wastewater types, and concludes by outlining the future prospects for the development of these novel bio-denitrification technologies.
With the ongoing advancements in industrialization and urbanization, excessive nitrogen emissions from industrial, domestic, and agricultural wastewater have resulted in environmental issues like eutrophication, unpleasant odors, and deterioration of water quality in surface bodies (Qu et al., 2019; Ceulemans et al., 2023; Xia and Yan, 2023). The primary denitrification methods encompass physicochemical and biological approaches. The physicochemical method mainly involves ion exchange, adsorption, chemical precipitation, and redox reactions, often requiring the addition of adsorbents, catalysts, and ion exchangers to achieve nitrogen removal. However, adsorption is sensitive to water quality variations, and adsorbents have a limited lifespan. Ion exchangers can lead to secondary pollution, while the use of catalysts increases treatment expenses (Soldatov et al., 2007; Tarpeh et al., 2017).
In contrast, the bio-denitrification process has gained popularity due to its cost-effectiveness, minimal by-product generation, dependable operation, and environmental compatibility (Zhang et al., 2016). Traditional bio-denitrification technology effectively treats nitrogen-containing wastewater through bio-nitrification (Eq. 1) and bio-denitrification (Eq. 2) processes. However, it necessitates aeration and the addition of organic carbon sources, which not only increase costs but can also contribute to secondary pollution, contradicting the low-carbon paradigm (Sanjrani et al., 2022). Consequently, the research and application of innovative bio-denitrification technologies hold significant importance for environmental preservation, ecological equilibrium, wastewater reclamation, energy conservation, and addressing emerging environmental challenges. This paper will introduce several novel bio-denitrification technologies and their integration processes, assess their denitrification performance, and summarize their research advancements in the field of wastewater denitrification.
Voets et al. (1975) made a pivotal discovery regarding the accumulation of nitrite (NO2−-N) during the nitrification process and introduced the concept of short-cut nitrification denitrification (SCND) technology. The fundamental principle involves the conversion of ammonia nitrogen (NH4+-N) into NO2−-N through controlled reaction parameters (Eq. 1), followed by direct reduction of NO2−-N to nitrogen (N2) through denitrification (Eq. 2; Huang, 2021). This innovation results in significant savings, reducing aeration requirements by 25% and organic carbon source needs by 40% when compared to traditional bio-denitrification processes (Kornaros et al., 2010). The key to SCND technology lies in regulating the nitrification process to stop at the nitrite stage.
It has been demonstrated that the control of reaction temperature, pH, dissolved oxygen (DO) concentration, and sludge age can effectively modulate the growth conditions of ammonia-oxidizing bacteria (AOB) and nitrite-oxidizing bacteria (NOB), thereby facilitating NO2−-N accumulation (Zhu et al., 2008). Currently, SCND technology has found practical applications in NH4+-N removal from landfill leachate (Zhang et al., 2020), sludge digestion liquid (Malamis et al., 2014), and biogas slurry (Chang et al., 2022; Chen et al., 2022), yielding significant results. In a study conducted by Chang et al. (2022) investigating the impact of the carbon-nitrogen ratio (C/N) on biogas slurry treatment within an activated sludge SCND system, it was observed that even with a reduced C/N ratio, NH4+-N and total nitrogen (TN) removal efficiencies could reach approximately 90%.
Anaerobic ammonium oxidation (ANAMMOX) technology involves the direct conversion of NH4+-N to N2 under stringent anaerobic conditions, facilitated by anaerobic ammonia-oxidizing bacteria (AnAOB) utilizing NO2−-N as the electron acceptor (Eq. 5; Kuenen, 2008). Therefore, ANAMMOX is well-suited for treating wastewater containing both NH4+-N and NO2−-N. Notably, electron acceptor NO2−-N can also be generated through short-cut nitrification (Eq. 3), making partial short-range nitrification an ideal precursor process for anaerobic ammonia oxidation (Wang J., 2021). The living conditions for AnAOB require strict anaerobic conditions, which contribute to energy savings by reducing aeration requirements. Moreover, the ANAMMOX process operates as an autotrophic process, eliminating the need for additional organic carbon sources, rendering it particularly suitable for denitrification in wastewater with low C/N ratios (Morales et al., 2015; Chini et al., 2019). Additionally, the stable pH levels maintained during ANAMMOX can preserve alkalinity, while AnAOB exhibits extended generation cycles and generates less sludge, substantially reducing the cost associated with excess sludge treatment and disposal (Jia et al., 2014; Ma et al., 2016).
Simultaneous nitrification and denitrification (SND) technology involves the bio-denitrification process where nitrification and denitrification reactions take place simultaneously in both space and time. SND relies on the generation of an oxygen concentration gradient due to oxygen diffusion limitations, resulting in the formation of hypoxic microenvironments within the core of sludge flocs or within biofilms. These microenvironments support the coexistence of aerobic and hypoxic metabolic activities (Layer et al., 2020). This technology allows nitrification and denitrification reactions to occur in the same spatial domain, significantly reducing the required reactor volume (Third et al., 2005; Masoudi et al., 2018). Additionally, SND imposes minimal demands for aeration and organic carbon, resulting in a reduction of approximately 30% in sludge production compared to traditional bio-denitrification processes (Ma et al., 2017; Zhao et al., 2017). Furthermore, there is no need for sludge reflux, and the alkalinity consumed during the nitrification process can be replenished by the alkalinity generated during denitrification (Ling, 2020). This not only maintains reactor pH stability but also reduces costs and optimizes process operations.
The core of SND technology is the control of DO concentration, which can be categorized into aerobic, low oxygen, and ultra-low DO SND denitrification (Ling, 2020; Xiang, 2020). Temperature, pH, and the C/N ratio are also pivotal factors influencing SND technology (Ling, 2020). It’s worth noting that autotrophic bacteria have a considerably slower growth and reproduction rate in comparison to heterotrophic bacteria. In long-term operation, heterotrophic denitrifying bacteria tend to become the dominant microbial community, reducing the significance of autotrophic nitrifying bacteria and subsequently affecting the denitrification effectiveness of SND (Jia et al., 2020).
Robertson et al. (1985) achieved the successful isolation of a denitrifying Paracoccus bacterium, a heterotrophic microorganism with the unique ability to directly convert ammonia nitrogen in wastewater into gaseous nitrogen. These bacteria possess both heterotrophic aerobic nitrification and heterotrophic aerobic denitrification functions, enabling them to utilize organic matter to convert NH4+-N, NO2¯-N, and nitrate nitrogen (NO3¯-N) into either organic nitrogen or gaseous nitrogen. In recent years, it has become evident that such functional microorganisms are widespread in nature, including species like Paracoccus pantotrophus, Acinetobacter sp., Phytophthora sp., Diaphorobacter sp., Alcaligenes faecalis, among others (Robertson et al., 1985; Xian et al., 2016; Jia et al., 2019). This denitrification method is known as heterotrophic nitrification aerobic denitrification (HN-AD; Robertson and Kuenen, 1990).
The HN-AD process, akin to SND, offers structural integration, reduced footprint, and simplified operational management. Moreover, heterotrophic microorganisms exhibit higher growth rates and denitrification capabilities (Yang Y. et al., 2017; Zhang H. H. et al., 2019; Zhang M. Y. et al., 2019), bestowing HN-AD technology with the advantages of rapid start-up, stable operation, and resilience to oxygen and organic substrates. However, the reaction process requires a higher organic carbon source and aeration rate compared to traditional biological denitrification. Therefore, it is most suitable for denitrification and decarbonization treatment of wastewater with a high C/N ratio, albeit with increase energy consumption and operational costs (Chen et al., 2014; Huang et al., 2021).
Granular sludge is generally considered to be granular activated sludge that gradually forms a stable structure after condensing into flocs by free bacteria (Sarma et al., 2017). There are five theoretical mechanisms for its formation, including the filamentous bacteria hypothesis, selective pressure-driven hypothesis, extracellular polymer hypothesis, microbial self-aggregation hypothesis, and crystal nucleus hypothesis (Li, 2020). Depending on whether microorganisms require oxygen for growth, they can be divided into anaerobic granular sludge and aerobic granular sludge (AGS), with AGS being the more commonly used. Mature AGS typically exhibits an orange-yellow color, a smooth surface, and a spherical or ellipsoidal shape, with particle sizes ranging from 0.5 to 1.5 mm (Moy et al., 2002). The structure of AGS is dense and regular, with strong settling capabilities. Thanks to microorganisms’ inherent self-solidification properties, AGS maintains its structural integrity even under dynamic conditions, forming agglomerated organisms without swelling or negatively affecting water quality (Mota et al., 2014). This characteristic enables AGS to efficiently remove high concentrations of toxic organic substances, nitrogen, and phosphorus (Kishida et al., 2009; He et al., 2020; Chen H. Y. et al., 2023).
In practical applications, the key to this technology lies in the domestication and cultivation of AGS. Factors significantly affecting AGS formation and performance include fluid shear force, COD load, DO, settling time, particle size, temperature, hydraulic retention time, sludge age, and the presence or absence of induced nuclei (Pishgar et al., 2020).
In addition to the aforementioned technologies, novel bio-denitrification technologies also encompass sulfur autotrophic denitrification (Song et al., 2023), iron autotrophic denitrification (Zhao et al., 2022), hydrogen autotrophic denitrification (Dong et al., 2021), and other methods. While these autotrophic bio-denitrification technologies offer numerous advantages over heterotrophic bio-denitrification processes, such as conserving organic carbon sources, being suitable for treating low C/N ratio wastewater, and reducing sludge production, they do present some practical challenges in their application in wastewater treatment.
The research on sulfur autotrophic denitrification technology traces its origins back to the 1970s. Compared to other autotrophic denitrification methods, the reduced sulfides (S0, S2−, Na2S2O3) used as electron donors are cost-effective and readily available, less sensitive to water quality variations, and easy to utilize. Moreover, due to the inclusion of S oxidation and N reduction in the sulfur autotrophic denitrification process, there is significant potential for waste resource utilization (Kosgey et al., 2022). However, the sulfur autotrophic denitrification reaction generates H+, lowers the system’s pH, and produces a substantial amount of environmentally polluting sulfate that must be controlled. High concentrations of sulfides can also impact microbial activity, hinder autotrophic denitrification efficiency, and especially affect the conversion of nitrate to nitrite (Beristain et al., 2006). Some studies have combined sulfur autotrophic denitrification with microbial fuel cell technology to achieve simultaneous organic matter removal and electricity generation. However, the deposition of elemental sulfur can lead to electrode poisoning or scaling, posing challenges to the further advancement of this technology (Lin et al., 2018; Wang and He, 2020).
Iron autotrophic denitrification technology is an autotrophic bio-denitrification method that employs Fe0 or Fe2+ as electron donors (Zhao et al., 2022). Fe (II) is widely distributed in the environment and is cost-effective, but it presents challenges in maintaining system stability for continuous operation. Fe0, on the other hand, offers superior electron-donating potential compared to Fe (II), leading to a significant reduction in the redox potential of denitrification sludge and improved system stability (Zhang Y. H. et al., 2014; Yu Y. et al., 2022). Currently, nano zero-valent iron is a key area of research in the field of iron autotrophic denitrification (Mofradnia et al., 2019). Various factors influence iron autotrophic denitrification, including temperature, pH, and the Fe/N ratio (Johnson et al., 2007; Oshiki et al., 2013; Yu Y. et al., 2022). pH regulation is particularly impactful, although it can increase the operational complexity of the iron autotrophic denitrification process. Moreover, the understanding of the denitrification process mechanism in this technology remains somewhat limited, especially regarding the relationship between its biological and chemical aspects (Yu Y. et al., 2022). Additionally, the microbial population and metabolic pathways in the iron autotrophic denitrification process are not yet fully understood, and further exploration is required to comprehend the reaction mechanism between iron ions and other compounds.
Hydrogen autotrophic denitrification involves the use of hydrogen bacteria to denitrify and remove nitrogen using H2 as an electron donor (Dong et al., 2021). It boasts advantages such as high denitrification efficiency, rapid reaction rates, environmental cleanliness, and the ease of removing residual H2 from water without requiring additional treatment. However, there are safety concerns associated with the flammability and explosiveness of H2 when mixed with air, leading to transportation risks and elevated operation and maintenance costs. Additionally, the low solubility of H2 in water, with only 1.6 mg H2 dissolved per liter of water at 20°C, results in low utilization rates (30–50%). These factors have restricted the widespread adoption of this technology, and current research on hydrogen autotrophic denitrification remains largely in the laboratory stage (Zhang Y. B. et al., 2014).
In recent years, most researchers have coupled new biological denitrification technologies to maximize their individual advantages and compensate for the limitations of independent use. This has resulted in the development of economically and environmentally friendly bio-denitrification technologies that hold great promise. Examples include partial short-cut nitrification ANAMMOX (PN-ANAMMOX; Qiu et al., 2021; Wang Z., 2021; Shang et al., 2023; Yuan et al., 2023), ANAMMOX-SND (Yu Y. et al., 2022), sulfur autotrophic-iron autotrophic denitrification (Yang et al., 2023), iron autotrophic-hydrogen autotrophic denitrification (Liang et al., 2022), and bioelectrochemical denitrification (Huang et al., 2020a; Prarunchaya et al., 2021). In this context, the focus will primarily be on the short-range nitrification anaerobic ammonia oxidation process and the bioelectrochemical system.
PN-ANAMMOX is a novel coupled biological denitrification process discovered at a waste leachate treatment plant in the Mechernich region of Germany (Hippen et al., 1997). It combines the ANAMMOX process with the short-cut nitrification process, often uniting them within a single reactor (Lu et al., 2012; Wang Z., 2021). Short-cut nitrification entails the control of ammonia oxidation to the nitrite stage (Eq. 3), ensuring a continuous and stable supply of nitrite, the essential oxidation substrate for ANAMMOX. The ideal NO2−-N to NH4+-N ratio is recognized as 1.3 (Eq. 3), though practical applications typically achieve a ratio of approximately 1, reflecting the challenge of controlling the short-cut nitrification process (Wang Z., 2021). In comparison to traditional bio-denitrification, short-cut nitrification inherently reduces aeration energy consumption, while AnAOB necessitates strict hypoxia. Consequently, this process can save nearly 60% of the energy demand for aeration compared to traditional bio-denitrification (Zaborowska et al., 2018). The estimated energy consumption for traditional bio-denitrification stands at approximately 2.4 kW·h·kg−1, whereas the energy consumption of the PN-ANAMMOX process is approximately 1.0 kW·h·kg−1 (Figueroa et al., 2012; Liang et al., 2016). Furthermore, both short-cut nitrification and ANAMMOX are autotrophic processes, eliminating the need for additional organic carbon sources, making them highly suitable for denitrifying wastewater with low C/N ratios. Additionally, PN-ANAMMOX significantly reduces sludge production by around 90% (Morales et al., 2015; Zaborowska et al., 2018; Chini et al., 2019).
However, it’s important to note that the initiation and domestication of anaerobic ammonia-oxidizing bacteria are relatively time-consuming. AOB and NOB often coexist, and the removal of NOB from the system is one of the challenges of this process. Moreover, the denitrification efficiency of PN-ANAMMOX is generally influenced by process parameters such as DO concentration, COD concentration, heavy metals, temperature, pH value, and sludge age, contributing to the complexity of its operation (Lotti et al., 2012; Zuo et al., 2020).
Bioelectrochemical systems (BESs) have emerged in recent years as a technology that combines microbiology with electrochemistry to achieve wastewater treatment and energy recovery (Liu and Logan, 2004). BESs rely on electrically active microbial catalytic electrodes, where oxidation reactions occur at the anode and reduction reactions happen at the cathode (Chang et al., 2016; Huang, 2021). Due to its sustainability (Yang W. L. et al., 2017), strong pollutant removal capability (Cao M. J. et al., 2020), and low sludge production (Wang and He, 2020), it has become a new type of low-energy-consumption water treatment technology that has attracted significant attention (Nguyen and Babel, 2022). Currently, many studies have used BESs to enhance various bio-reaction processes, including the removal of pollutants like nitrogen (Cecconet et al., 2020), phosphorus (Elmaadawy et al., 2020), organic matter (Cao et al., 2021), and heavy metals (Cao L. B. et al., 2020) that are challenging to degrade. Some studies have also employed BESs to improve denitrification in low C/N ratio wastewater treatment. The enhancement mechanisms involve the positive effects of bioelectricity on microbial functional enzymes and genes, as well as the direct provision of electrons by bioelectricity to the denitrification process, achieving electro autotrophic denitrification (Huang et al., 2020a, 2022). In addition, for denitrification in wastewater containing high concentrations of hard-to-degrade organic matter, BESs can break down these complex compounds into simpler organic matter, providing an organic carbon source for denitrification (Huang et al., 2020b). However, it’s important to note that BESs are primarily used for medium to low concentration nitrogen loads, and this technology is currently mainly under laboratory research, with the potential to become a mainstream nitrogen removal process in the future.
Section 2.1 introduces several emerging biological denitrification technologies while providing brief explanations of their application scope, advantages, disadvantages, and the factors influencing each technology. Among these aspects, the denitrification performance is a central concern for these innovative denitrification technologies. Table 1 presents the performance data related to nitrogen and carbon removal during the application of some of these novel biological denitrification technologies.
As shown in Table 1, these novel bio-denitrification technologies have been employed in various wastewater treatment processes, including membrane bioreactors (Chang et al., 2022; Chen et al., 2022) and biofilters (Song et al., 2023). Remarkably, these technologies are not limited to synthetic wastewater treatment (Francesca et al., 2021; Chang et al., 2022; Zhao et al., 2022; Jiang et al., 2023; Roumi and Debabrata, 2023; Sun et al., 2023) but have also demonstrated their effectiveness in treating practical wastewater sources such as landfill leachate (Zhang et al., 2020, 2023), biogas slurry (Chen et al., 2022), urban domestic sewage (Dong et al., 2021; Li Y. et al., 2023), secondary effluent from sewage treatment plants (Song et al., 2023), aquaculture wastewater (Chen H. Y. et al., 2023; Guo et al., 2023), and chemical wastewater (Chen H. Y. et al., 2023; Chen P. P. et al., 2023). These novel bio-denitrification technologies exhibit versatility in handling a wide range of nitrogen concentrations, with initial nitrogen loads ranging from as high as 1,600 mgN·L−1 (Chen P. P. et al., 2023) to as low as 11 mgN·L−1 (Sun et al., 2023). Importantly, they consistently achieve robust denitrification effects, with denitrification efficiencies surpassing 70%, and in most cases, approaching 100%.
As shown in Table 1, autotrophic biological denitrification technology is primarily employed for the advanced treatment of low C/N ratio wastewater and has demonstrated significant advantages. Anaerobic ammonia oxidation technology, sulfur autotrophic, hydrogen autotrophic, and iron autotrophic biological denitrification technologies all exhibit treatment efficiencies exceeding 85% when applied to wastewater with a C/N ratio less than 3 (Dong et al., 2021; Zhao et al., 2022; Chen H. Y. et al., 2023; Jiang et al., 2023; Song et al., 2023; Zhang et al., 2023). Even in the study by Zhao et al. (2022), where iron autotrophic biological denitrification technology was employed to treat wastewater with an extremely low C/N ratio of 0.5, a denitrification efficiency of 90% was achieved. The widespread use of these autotrophic bio-denitrification technologies has the potential to effectively reduce chemical costs (related to organic carbon sources) and expenses associated with excess sludge treatment, aligning with the global low-carbon concept that has gained prominence in recent years.
Section 2.2 introduces several novel bio-denitrification coupling technologies, and Table 2 provides data on denitrification and carbon removal performance during the application of selected coupling denitrification technologies. As demonstrated in Table 2, the actual wastewater treated by these new biological denitrification coupling processes includes landfill leachate (Wang Z., 2021), domestic wastewater (Huang et al., 2020a; Prarunchaya et al., 2021; Qiu et al., 2021; Liang et al., 2022; Shang et al., 2023; Yang et al., 2023; Yuan et al., 2023), and chemical wastewater (Wang et al., 2022; Yu D. Y. et al., 2022; Jia et al., 2023), closely resembling the wastewater types addressed by the new biological denitrification technologies in Table 1. PN-ANAMMOX technology has emerged as the most prevalent coupling method in recent years, showcasing its versatility in handling a wide range of nitrogen loads and consistently achieving robust denitrification efficiency exceeding 90% (Qiu et al., 2021; Wang Z., 2021; Shang et al., 2023; Yuan et al., 2023).
The granular sludge technology primarily involves introducing granular sludge into the system, which, while shortening the reactor’s startup time, does not exhibit high adaptability or resistance to sewage impact (Sun et al., 2023). Consequently, researchers have explored its combination with other bio-denitrification technologies to cultivate specific granular sludge for wastewater treatment (Wang et al., 2022). However, the TN removal efficiency of this process has proven to be less than ideal. For instance, in a study by Sun et al. (2023), isolated AGS was applied to treat artificially synthesized urban wastewater, achieving a 95% removal efficiency for ammonia nitrogen, but only a 70% removal efficiency for TN. Similarly, Wang et al. (2022) utilized “heterotrophic nitrification aerobic denitrification” in conjunction with AGS to treat synthetic petroleum wastewater, attaining a 92% removal efficiency for ammonia nitrogen, while the TN removal efficiency reached only 80%. Therefore, whether used independently or in conjunction with other technologies, its TN removal efficiency falls short of that for ammonia nitrogen. Further investigation is needed to better understand the underlying reasons and explore potential enhancement measures.
Transportation safety risks associated with H2 limit the application of hydrogen autotrophic denitrification (Zhang Y. H. et al., 2014). However, BES with external electric fields can produce hydrogen in situ, providing a local source of H2 for hydrogen autotrophic denitrification (Prarunchaya et al., 2021). Nevertheless, the extra electricity needed for this coupled technology increases its operational costs, to some extent, restricting its large-scale practical application. Additionally, sulfur autotrophic denitrification faces challenges such as low pH values and secondary pollution due to sulfate by-products. The sulfur autotrophic coupled with iron autotrophic denitrification system effectively reduces sulfate by-products and exhibits robust denitrification performance (Yang et al., 2023).
The novel bio-denitrification technology represents a promising sewage treatment approach with broad engineering applications. It effectively addresses the challenges associated with traditional denitrification processes, such as lengthy processing, substantial land requirements, high energy consumption, and significant financial investments (Zhang et al., 2022). In comparison to conventional biological denitrification methods, this innovative technology offers superior efficiency and adaptability. It can cater to the treatment requirements of various wastewater types and achieve efficient deep denitrification in low C/N ratio wastewater without the need for additional external carbon sources. Therefore, in light of current domestic and international policies aimed at improving effluent standards in urban sewage treatment plants, the novel bio-denitrification technology emerges as a favorable low-carbon choice.
The novel bio-denitrification technology not only compensates for the limitations of independent use through self-coupling but also pairs effectively with non-biological methods to provide reference parameters that are challenging to regulate in practical engineering. For instance, in treating highly concentrated toxic and hazardous wastewater, physical and chemical techniques such as adsorption, membrane separation, electrochemistry, and oxidation–reduction can be employed as pretreatment. Subsequently, they can be combined with biological denitrification technology for advanced treatment, thereby further expanding the practical application range and improving sewage treatment efficacy. The greater challenge for the future is to explore low-energy, cost-effective technologies while maximizing process coupling to overcome constraints in real-world wastewater scenarios and enable large-scale applications. This development direction aligns with the low-carbon concept and introduces innovative ideas for environmentally friendly denitrification.
Chan-Pacheco et al. (2021) explored the substantial potential of combining new bio-denitrification technologies for the purification and reduction of denitrification gas pollutants, including N2O, CH4, and H2S, which are presently significant greenhouse gases of global concern. This suggests that future research could delve into additional technology coupling approaches for greenhouse gas emission reduction, further contributing to the low-carbon concept.
In the practical application of novel bio-denitrification technologies, the cultivation and regulation of microbial communities are key factors for achieving efficient denitrification. However, challenges can arise, such as unstable environmental conditions or increased operating costs due to the need for adjustments in temperature, pH, and other parameters when cultivating these microorganisms. Therefore, it is worth considering the utilization of novel bio-denitrification technologies within the realm of smart water management, where the integration of automation, computer technology, and other advancements can standardize the domestication and cultivation of microorganisms. Simultaneously, this approach can help reduce the reliance on human resources and propel the advancement of new biological denitrification technology towards broader practical implementation.
This paper reviews the denitrification performance of novel bio-denitrification technologies and their combined applications, analyzes the recent research situation, and summarizes the future development direction and challenges of these technologies. Novel bio-denitrification technology is environmentally friendly and holds vast application prospects, aligning with the sustainable low-carbon development concept. With ongoing technological progress, novel bio-denitrification technologies are expected to expand their presence in various fields, contributing significantly to environmental protection and sustainable development.
SH: Funding acquisition, Investigation, Writing – original draft, Writing – review & editing. YLF: Investigation, Writing – original draft. HMZ: Writing – review & editing. CQW: Writing – review & editing. CLZ: Writing – review & editing. XGL: Writing – review & editing.
The author(s) declare financial support was received for the research, authorship, and/or publication of this article. This research was funded by the National Natural Science Foundation of China (42207159) and Jiangxi Provincial Natural Science Foundation (20232BAB214085).
The authors declare that the research was conducted in the absence of any commercial or financial relationships that could be construed as a potential conflict of interest.
All claims expressed in this article are solely those of the authors and do not necessarily represent those of their affiliated organizations, or those of the publisher, the editors and the reviewers. Any product that may be evaluated in this article, or claim that may be made by its manufacturer, is not guaranteed or endorsed by the publisher.
Beristain, C. R., Reyes, S. A., Pieter, R., Razo, F. E., and Jorge, G. (2006). Sulfide oxidation under chemolithoautotrophic denitrifying conditions. Biotechnol. Bioeng. 95, 1148–1157. doi: 10.1002/bit.21084
Cao, M. J., Feng, Y. J., Wang, N. Y., Li, Y. F., Li, N., Liu, J., et al. (2020). Electrochemical regulation on the metabolism of anode biofilms under persistent exogenous bacteria interference. Electrochim. Acta 340:135922. doi: 10.1016/j.electacta.2020.135922
Cao, L. B., Ma, Y. M., Deng, D. D., Jiang, H. C., Wang, J. X., and Liu, Y. (2020). Electricity production of microbial fuel cells by degrading cellulose coupling with Cr(VI) removal. J. Hazard. Mater. 391:122184. doi: 10.1016/j.jhazmat.2020.122184
Cao, X., Wang, H., Long, X. Z., Nishimura, O., and Li, X. N. (2021). Limitation of voltage reversal in the degradation of azo dye by a stacked double-anode microbial fuel cell and characterization of the microbial community structure. Sci. Total Environ. 754:142454. doi: 10.1016/j.scitotenv.2020.142454
Cecconet, D., Sabba, F., Devecseri, M., Callegari, A., and Capodaglio, A. G. (2020). In situ groundwater remediation with bioelectrochemical systems: a critical review and future perspectives. Environ. Int. 137:105550. doi: 10.1016/j.envint.2020.105550
Ceulemans, T., Verscheure, P., Shadouh, C., Van Acker, K., Devleesschauwer, B., Linard, C., et al. (2023). Environmental degradation and the increasing burden of allergic disease: the need to determine the impact of nitrogen pollution. Front. Allergy 4:1063982. doi: 10.3389/falgy.2023.1063982
Chang, M. D., Liang, B. R., Zhang, K., Wang, Y. Z., Jin, D. T., Zhang, Q. J., et al. (2022). Simultaneous shortcut nitrification and denitrification in a hybrid membrane aerated biofilms reactor (H-MBfR) for nitrogen removal from low COD/N wastewater. Water Res. 211:118027. doi: 10.1016/j.watres.2021.118027
Chang, C., Lu, X. B., Lei, Z. W., Wang, Z. L., and Zhao, C. (2016). 2-Mercaptopyridine as a new leveler for bottom-up filling of micro-vias in copper electroplating. Electrochim. Acta 208, 33–38. doi: 10.1016/j.electacta.2016.04.177
Chan-Pacheco, C. R., Valenzuela, E. I., Cervantes, F. J., and Quijano, G. (2021). Novel biotechnologies for nitrogen removal and their coupling with gas emissions abatement in wastewater treatment facilities. Sci. Total Environ. 797:149228. doi: 10.1016/j.scitotenv.2021.149228
Chen, H. Y., Li, X. K., Liu, G. G., Zhu, J., Ma, X. C., Piao, C. Y., et al. (2023). Decoding the carbon and nitrogen metabolism mechanism in anammox system treating pharmaceutical wastewater with varying COD/N ratios through metagenomic analysis. Chem. Eng. J. 457:141316. doi: 10.1016/j.cej.2023.141316
Chen, M. X., Wang, W. C., Feng, Y., Zhu, X. H., Zhou, H. Z., Tan, Z. L., et al. (2014). Impact resistance of different factors on ammonia removal by heterotrophic nitrification-aerobic denitrification bacterium Aeromonas sp. HN-02. Bioresour. Technol. 167, 456–461. doi: 10.1016/j.biortech.2014.06.001
Chen, Y. W., Wang, H., Gao, X. D., Li, X., Dong, S. Y., Zhou, H. Z., et al. (2022). COD/TN ratios shift the microbial community assembly of a pilot-scale shortcut nitrification-denitrification process for biogas slurry treatment. Environ. Sci. Pollut. Res. 29, 49335–49345. doi: 10.1007/s11356-022-19285-w
Chen, P. P., Zhai, T. R., Zhang, L. J., Zhao, T. T., Xing, Z. L., and Liu, H. (2023). Domestication and pilot-scale culture of mixed bacteria HY-1 capable of heterotrophic nitrification-aerobic denitrification. Bioresour. Technol. 384:129285. doi: 10.1016/j.biortech.2023.129285
Chini, A., Bolsan, A. C., Hollas, C. E., Antes, F. G., Fongaro, G., Treichel, H., et al. (2019). Evaluation of deammonification reactor performance and microrganisms community during treatment of digestate from swine sludge CSTR biodigester. J. Environ. Manag. 246, 19–26. doi: 10.1016/j.jenvman.2019.05.113
Dong, K., Feng, X. H., Wang, W. B., Chen, Y. C., Hu, W., Li, H. X., et al. (2021). Simultaneous partial nitrification and denitrification maintained in membrane bioreactor for nitrogen removal and hydrogen autotrophic denitrification for further treatment. Membranes 11:911. doi: 10.3390/membranes11120911
Elmaadawy, K., Liu, B. C., Hu, J. P., Hou, H. J., and Yang, J. K. (2020). Performance evaluation of microbial fuel cell for landfill leachate treatment: research updates and synergistic effects of hybrid systems. J. Environ. Sci. 96, 1–20. doi: 10.1016/j.jes.2020.05.005
Figueroa, M., Vázquez-Padín, J. R., Mosquera-Corral, A., Campos, J. L., and Méndez, R. (2012). Is the CANON reactor an alternative for nitrogen removal from pre-treated swine slurry? Biochem. Eng. J. 65, 23–29. doi: 10.1016/j.bej.2012.03.008
Francesca, I., Francesco, D. C., Francesco, G., Rudy, G., and Giovanni, E. (2021). Shortcut nitrification-denitrification and biological phosphorus removal in acetate-and ethanol-fed moving bed biofilm reactors under microaerobic/aerobic conditions. Bioresour. Technol. 330:124958. doi: 10.1016/j.biortech.2021.124958
Guo, P., Wang, Q., Ni, L. F., Xu, S. L., Zheng, D. Q., Wang, Y., et al. (2023). Improved simultaneous nitrification-denitrification in fixed-bed baffled bioreactors treating mariculture wastewater: performance and microbial community behaviors. Bioresour. Technol. 385:129468. doi: 10.1016/j.biortech.2023.129468
He, Q. L., Wang, H. T., Chen, L., Gao, S. X., Zhang, W., Song, J. Y., et al. (2020). Elevated salinity deteriorated enhanced biological phosphorus removal in an aerobic granular sludge sequencing batch reactor performing simultaneous nitrification, denitrification and phosphorus removal. J. Hazard. Mater. 390:121782. doi: 10.1016/j.jhazmat.2019.121782
Hippen, A., Rosenwinkel, K. H., Baumgarten, G., and Seyfried, C. F. (1997). Aerobic deammonification: a new experience in the treatment of waste waters. Water Sci. Technol. 35, 111–120. doi: 10.2166/wst.1997.0371
Huang, S. (2021). Approach and mechanisms of enhanced cathode denitrification performance of low COD/N wastewater in the multi-anode microbial fuel cell. Southeast University, Nan Jing, Jiangsu.
Huang, M., Cui, Y., Yang, H., Xu, M., Cui, Y., and Chen, Z. (2023). A halophilic aerobic-heterotrophic strain Halomonas venusta SND-01: nitrogen removal by ammonium assimilation and heterotrophic nitrification-aerobic denitrification. Bioresour. Technol. 374:128758. doi: 10.1016/j.biortech.2023.128758
Huang, S., Lu, Y., Li, X., Lu, Y. Z., Zhu, G., and Hassan, M. (2020b). Tertiary denitrification and organic matter variations of secondary effluent from wastewater treatment plant by the 3D-BER system. Environ. Res. 189:109937. doi: 10.1016/j.envres.2020.109937
Huang, F., Pan, L. Q., He, Z. Y., Zhang, M. Y., and Zhang, M. Z. (2021). Heterotrophic nitrification-aerobic denitrification characteristics and antibiotic resistance of two bacterial consortia from Marinomonas and Halomonas with effective nitrogen removal in mariculture wastewater. J. Environ. Manag. 279:111786. doi: 10.1016/j.jenvman.2020.111786
Huang, S., Zhang, J. R., Wang, C. Q., Zhu, G. C., and Hassan, M. (2022). Weak electric field effect of MFC biocathode on denitrification. J. Environ. Chem. Eng. 10:108596. doi: 10.1016/j.jece.2022.108596
Huang, S., Zhu, G., and Gu, X. (2020a). The relationship between energy production and simultaneous nitrification and denitrification via bioelectric derivation of microbial fuel cells at different anode numbers. Environ. Res. 184:109247. doi: 10.1016/j.envres.2020.109247
Jia, X. F., Fan, H. X., Liang, J. W., Dai, J. H., Sun, Y., and Mai, W. N. (2023). Performance analysis of anaerobic digestion coupled with simultaneous nitrification and denitrification process for treating alcohol precipitation wastewater of Chinese patent medicine. Water 15:1939. doi: 10.3390/w15101939
Jia, F. X., Peng, Y. Z., and Yang, Q. (2014). Competition and synergism between anammox bacteria and other bacteria. Acta Sci. Circumst. 34, 1351–1361. doi: 10.13671/j.hjkxxb.2014.0206
Jia, Y. T., Zhou, M. M., Chen, Y. C., Hu, Y. Y., and Luo, J. (2020). Insight into short-cut of simultaneous nitrification and denitrification process in moving bed biofilm reactor: effects of carbon to nitrogen ratio. Chem. Eng. J. 400:125905. doi: 10.1016/j.cej.2020.125905
Jia, Y. T., Zhou, M. M., Chen, Y. C., Luo, J., and Hu, Y. Y. (2019). Carbon selection for nitrogen degradation pathway by Stenotrophomonas maltophilia: based on the balances of nitrogen, carbon and electron. Bioresour. Technol. 294:122114. doi: 10.1016/j.biortech.2019.122114
Jiang, M. T., Ji, S. H., Wu, R. X., Yang, H., Li, Y. Y., and Liu, J. Y. (2023). Exploiting refractory organic matter for advanced nitrogen removal from mature landfill leachate via anammox in an expanded granular sludge bed reactor. Bioresour. Technol. 371:128594. doi: 10.1016/j.biortech.2023.128594
Johnson, K. J., Ams, D. A., Wedel, A. N., Szymanowski, J. E. S., Weber, D. L., Schneegurt, M. A., et al. (2007). The impact of metabolic state on cd adsorption onto bacterial cells. Geobiology 5, 211–218. doi: 10.1111/j.1472-4669.2007.00111.x
Kishida, N., Tsuneda, S., Kim, J. H., and Sudo, R. (2009). Simultaneous nitrogen and phosphorus removal from high-strength industrial wastewater using aerobic granular sludge. J. Environ. Eng. 135, 153–158. doi: 10.1061/(ASCE)0733-9372(2009)135:3(153)
Kornaros, M., Dokianakis, S. N., and Lyberatos, G. (2010). Partial nitrification/denitrification can be attributed to the slow response of nitrite oxidizing bacteria to periodic anoxic disturbances. Environ. Sci. Technol. 44, 7245–7253. doi: 10.1021/es100564j
Kosgey, K., Zungu, P. V., Bux, F., and Kumari, S. (2022). Biological nitrogen removal from low carbon wastewater. Front. Microbiol. 13:968812. doi: 10.3389/fmicb.2022.968812
Kuenen, J. G. (2008). Anammox bacteria: from discovery to application. Nat. Rev. Microbiol. 6, 320–326. doi: 10.1038/nrmicro1857
Layer, M., Villodres, M. G., Hernandez, A., Reynaert, E., Morgenroth, E., and Derlon, N. (2020). Limited simultaneous nitrification-denitrification (SND) in aerobic granular sludge systems treating municipal wastewater: mechanisms and practical implications. Water Res. X 7:100048. doi: 10.1016/j.wroa.2020.100048
Li, S. (2020). The research on efficiency and mechanism of aerobic granular sludge continuous flow process for nitrogen and phosphorus removal. Harbin Institute of Technology, Harbin, Heilongjiang.
Li, M., Liu, R., Chen, J., Peng, Q., Liu, J., and She, Z. (2023). Effects of pH on simultaneous partial nitrification and denitrification performance. Period. Ocean Univ. China 53, 67–76. doi: 10.16441/j.cnki.hdxb.20220134
Li, Y., Liu, S. J., Lu, L. L., Wang, J. H., Huang, G. R., Chen, F. M., et al. (2023). Non-uniform dissolved oxygen distribution and high sludge concentration enhance simultaneous nitrification and denitrification in a novel air-lifting reactor for municipal wastewater treatment: a pilot-scale study. Bioresour. Technol. 384:129306. doi: 10.1016/j.biortech.2023.129306
Liang, Y. C., Daverey, A., Huang, Y. T., Sung, S. H., and Lin, J. G. (2016). Treatment of semiconductor wastewater using single-stage partial nitrification and anammox in a pilot-scale reactor. J. Taiwan Inst. Chem. Eng. 63, 236–242. doi: 10.1016/j.jtice.2016.02.036
Liang, Y. F., Pan, Z. R., Feng, H. B., Cheng, X. Y., Guo, T., Yan, A. Q., et al. (2022). Biofilm coupled micro-electrolysis of waste iron shavings enhanced iron and hydrogen autotrophic denitrification and phosphate accumulation for wastewater treatment. J. Environ. Chem. Eng. 10:108959. doi: 10.1016/j.jece.2022.108959
Lin, S., Mackey, H. R., Hao, T. W., Guo, G., Loosdrecht, M. C. M. V., and Chen, G. H. (2018). Biological sulfur oxidation in wastewater treatment: a review of emerging opportunities. Water Res. 143, 399–415. doi: 10.1016/j.watres.2018.06.051
Ling, F. (2020). Study on enhancement and kinetics of cathodic simultaneous nitrification and denitrification in microbial fuel cell system. South. Univ. Nan Jing, Jiangsu 186:109505. doi: 10.1016/j.envres.2020.109505
Liu, H., and Logan, B. E. (2004). Electricity generation using an air-cathode single chamber microbial fuel cell in the presence and absence of a proton exchange membrane. Environ. Sci. Technol. 38, 4040–4046. doi: 10.1021/es0499344
Lotti, T., Cordola, M., Kleerebezem, R., Caffaz, S., Lubello, C., and Loosdrechtet, M. C. M. (2012). Inhibition effect of swine wastewater heavy metals and antibiotics on anammox activity. Water Sci. Technol. 66, 1519–1526. doi: 10.2166/wst.2012.344
Lu, H. F., Zheng, P., Ji, Q. X., Zhang, H. T., Ji, J. Y., Wang, L., et al. (2012). The structure, density and settlability of anammox granular sludge in high-rate reactors. Bioresour. Technol. 123, 312–317. doi: 10.1016/j.biortech.2012.07.003
Ma, W. W., Han, Y. X., Ma, W. C., Han, H. J., Zhu, H., Xu, C. Y., et al. (2017). Enhanced nitrogen removal from coal gasification wastewater by simultaneous nitrification and denitrification (SND) in an oxygen-limited aeration sequencing batch biofilm reactor. Bioresour. Technol. 244, 84–91. doi: 10.1016/j.biortech.2017.07.083
Ma, B., Wang, S. Y., Cao, S. B., Miao, Y. Y., Jia, F. X., Du, R., et al. (2016). Biological nitrogen removal from sewage via anammox: recent advances. Bioresour. Technol. 200, 981–990. doi: 10.1016/j.biortech.2015.10.074
Malamis, S., Katsou, E., Fabio, S. D., Bolzonella, D., and Fatone, F. (2014). Biological nutrients removal from the supernatant originating from the anaerobic digestion of the organic fraction of municipal solid waste. Crit. Rev. Biotechnol. 34, 244–257. doi: 10.3109/07388551.2013.791246
Masoudi, S. M. A., Moghaddam, A. H., Sargolzaei, J., Darroudi, A., and Zeynali, V. (2018). Investigation and optimization of the SND-SBR system for organic matter and ammonium nitrogen removal using the central composite design. Environ. Prog. Sustain. Energy 37, 1638–1646. doi: 10.1002/ep.12847
Mofradnia, S. R., Ashouri, R., Tavakoli, Z., Shahmoradi, F., Rashedi, H., Yazdian, F., et al. (2019). Effect of zero-valent iron/starch nanoparticle on nitrate removal using MD simulation. Int. J. Biol. Macromol. Struct. Funct. Interact. 121, 727–733. doi: 10.1016/j.ijbiomac.2018.09.183
Morales, N., Río, Á. V. D., Vázquez-Padín, J. R., Méndez, R., Mosquera-Corral, A., and Campos, J. L. (2015). Integration of the Anammox process to the rejection water and main stream lines of WWTPs. Chemosphere 140, 99–105. doi: 10.1016/j.chemosphere.2015.03.058
Mota, C. R., Head, M. A., Williams, J. C., Eland, L., Cheng, J. J., and Reyes, F. L. D. L. (2014). Structural integrity affects nitrogen removal activity of granules in semi-continuous reactors. Biodegradation 25, 923–934. doi: 10.1007/s10532-014-9712-3
Moy, B., Tay, J., Toh, S., Liu, Y., and Tay, S. (2002). High organic loading influences the physical characteristics of aerobic sludge granules. Lett. Appl. Microbiol. 34, 407–412. doi: 10.1046/j.1472-765X.2002.01108.x
Nguyen, H. D., and Babel, S. (2022). Insights on microbial fuel cells for sustainable biological nitrogen removal from wastewater: a review. Environ. Res. 204:112095. doi: 10.1016/j.envres.2021.112095
Oshiki, M., Ishii, S., Yoshida, K., Fujii, N., Ishiguro, M., Satoh, H., et al. (2013). Nitrate-dependent ferrous iron oxidation by anaerobic ammonium oxidation (znammox) bacteria. Appl. Environ. Microbiol. 79, 4087–4093. doi: 10.1128/AEM.00743-13
Pishgar, R., Dominic, J. A., Tay, J. H., and Chu, A. (2020). Pilot-scale investigation on nutrient removal characteristics of mineral-rich aerobic granular sludge: identification of uncommon mechanisms. Water Res. 168:115151. doi: 10.1016/j.watres.2019.115151
Prarunchaya, P., Orapan, M., Palisa, M., Pakpoom, S., Nga, D. T., Auppatham, N., et al. (2021). Enhancement of nitrate removal under limited organic carbon with hydrogen-driven autotrophic denitrification in low-cost electrode bio-electrochemical reactors. J. Chem. Technol. Biotechnol. 96, 2520–2528. doi: 10.1002/jctb.6788
Qiu, S. J., Liu, J. J., Zhang, L., Zhang, Q., and Peng, Y. Z. (2021). Sludge fermentation liquid addition attained advanced nitrogen removal in low C/N ratio municipal wastewater through short-cut nitrification-denitrification and partial anammox. Front. Environ. Sci. Eng. 15, 107–116. doi: 10.1007/s11783-020-1318-x
Qu, J. H., Wang, H. C., Wang, K. J., Yu, G., Ke, B., Yu, H. Q., et al. (2019). Municipal wastewater treatment in China: development history and future perspectives. Front. Environ. Sci. Eng. 13, 3–9. doi: 10.1007/s11783-019-1172-x
Robertson, L. A., and Kuenen, J. G. (1990). Combined heterotrophic nitrification and aerobic denitrification in Thiosphaera pantotropha and other bacteria. Antonie Van Leeuwenhoek 57, 139–152. doi: 10.1007/BF00403948
Robertson, L. A., Kuenen, J. G., and Kleijntjens, R. (1985). Aerobic denitrification and heterotrophic nitrification by Thiosphaera pantotropha. J. Microbiol. Serol. 51:445. doi: 10.1007/BF02275068
Roumi, B., and Debabrata, M. (2023). Process optimization of a moving bed bioreactor undergoing simultaneous nitrification and denitrification for wastewater in the absence of organic carbon. Environ. Technol., 1–15. doi: 10.1080/09593330.2023.2227388
Sanjrani, M. A., Nafees, A., Sakina, R., and Xue, G. (2022). A review on application of external carbon sources for denitrification for wastewater treatment. Global NEST J. 24, 105–118. doi: 10.30955/gnj.004248
Sarma, S. J., Tay, J. H., and Chu, A. (2017). Finding knowledge gaps in aerobic granulation technology. Trends Biotechnol. 35, 66–78. doi: 10.1016/j.tibtech.2016.07.003
Shang, T. T., Zhu, X. R., Gong, X. F., Guo, J. W., Li, X. Y., Zhang, Q., et al. (2023). Efficient nitrogen removal in a total floc sludge system from domestic wastewater with low C/N: high anammox nitrogen removal contribution driven by endogenous partial denitrification. Bioresour. Technol. 378:128995. doi: 10.1016/j.biortech.2023.128995
Soldatov, V. S., Sokolova, V. I., Medyak, G. V., Shunkevich, A. A., and Akulich, Z. I. (2007). Binary ion exchange equilibria in systems containing NO3−, Cl− and SO42− on fibrous anion exchangers with tetraalkylammomium groups. React. Funct. Polym. 67, 1530–1539. doi: 10.1016/j.reactfunctpolym.2007.07.018
Song, Q., Lin, F., Yu, G., Gao, M., and Zhang, Y. (2023). Pilot study on deep denitrification effect of sulfur autotrophic denitrification filter on secondary effluent. Water Wastewater Eng. 59, 21–25. doi: 10.13789/j.cnki.wwe1964.2022.04.26.0003
Sun, Z., Zhang, J. M., Wang, J., Zhu, H. X., Xiong, J. H., Nong, G. Y., et al. (2023). Direct start-up of aerobic granular sludge system with dewatered sludge granular particles as inoculant. J. Environ. Manag. 326:116540. doi: 10.1016/j.jenvman.2022.116540
Tarpeh, W. A., Udert, K. M., and Nelson, K. L. (2017). Comparing ion exchange adsorbents for nitrogen recovery from source-separated urine. Environ. Sci. Technol. 51, 2373–2381. doi: 10.1021/acs.est.6b05816
Third, K. A., Gibbs, B., Newland, M., and Cord-Ruwisch, R. (2005). Long-term aeration management for improved N-removal via SND in a sequencing batch reactor. Water Res. 39, 3523–3530. doi: 10.1016/j.watres.2005.06.014
Voets, J. P., Vanstaen, H., and Verstraete, W. (1975). Removal of nitrogen from highly nitrogenous wastewaters. Water Pollut. Cont. Federation 47, 394–398.
Wang, J. (2021). The performance and mechanism of completely autotrophic nitrogen removal process based on formic acid aided partial nitrification. South China University of Technology, Guangzhou, Guangdong.
Wang, Z. (2021). Process and mechanism based on anammox treating mature landfill leachate. Harbin Institute of Technology, Harbin, Heilongjiang.
Wang, Z. X., and He, Z. (2020). Demystifying terms for understanding bioelectrochemical systems towards sustainable wastewater treatment. Curr. Opin. Electrochem. 19, 14–19. doi: 10.1016/j.coelec.2019.09.001
Wang, J. J., Huang, B. C., Li, J., and Jin, R. C. (2020). Advances and challenges of sulfur-driven autotrophic denitrification (SDAD) for nitrogen removal. Chin. Chem. Lett. 31, 2567–2574. doi: 10.1016/j.cclet.2020.07.036
Wang, Q. H., Kong, J. W., Liang, J. H., EI-Din, M. G., Zhao, P., Xie, W. Y., et al. (2022). Nitrogen removal intensification of aerobic granular sludge through bioaugmentation with “heterotrophic nitrification-aerobic denitrification” consortium during petroleum wastewater treatment. Bioresour. Technol. 361:127719. doi: 10.1016/j.biortech.2022.127719
Xia, L. L., and Yan, X. Y. (2023). How to feed the world with less nitrogen pollution. Nature 613, 34–35. doi: 10.1038/d41586-022-04490-x
Xian, S. S., Chen, M. X., Xiong, R., and Tan, Z. L. (2016). Research advances of heterotrophic nitrification-aerobic denitrification under different factors. Technol. Water Treat. 42, 1–6. doi: 10.16796/j.cnki.1000-3770.2016.01.001
Xiang, Y. (2020). Nitrogen removal capacity and pathways of the simultaneous nitrification and denitrification process under different oxygen conditions. Chongqing University, Chongqing.
Yang, W. L., Kim, K. Y., Saikaly, P., and Logan, B. E. (2017). The impact of new cathode materials relative to baseline performance of microbial fuel cells all with the same architecture and solution chemistry. Energy Environ. Sci. 10, 1025–1033. doi: 10.1039/C7EE00910K
Yang, Y., Liu, Y. X., Yang, T., and Lv, Y. K. (2017). Characterization of a microbial consortium capable of heterotrophic nitrifying under wide C/N range and its potential application in phenolic and coking wastewater. Biochem. Eng. J. 120, 33–40. doi: 10.1016/j.bej.2016.12.008
Yang, B., Shun, W., Ainur, Z., Igor, V. S., PoHeng, L., and Zhan, X. M. (2023). High-rate iron sulfide and sulfur-coupled autotrophic denitrification system: nutrients removal performance and microbial characterization. Water Res. 231:119619. doi: 10.1016/j.watres.2023.119619
Yu, Y., Liu, N., Liao, Z., Zhao, J., and Zhang, Q. (2022). Research progress of iron-type denitrification removal technology. China Environ. Sci. 42, 83–91. doi: 10.19674/j.cnki.issn1000-6923.2022.0002
Yu, D. Y., Zhang, W. J., Wang, D. Q., and Jin, Y. (2022). Full-scale application of one-stage simultaneous nitrification and denitrification coupled with Anammox process for treating collagen casing wastewater. Int. J. Environ. Res. Public Health 19:5787. doi: 10.3390/ijerph19105787
Yuan, Y., Yongzhi, C., Xinbo, W., Hongzhong, D., Tianxu, Z., Jiao, M., et al. (2023). Enhanced nitrogen removal from rural domestic sewage via partial nitrification-anammox in integrated vertical subsurface flow constructed wetland. Environ. Res. 233:116338. doi: 10.1016/j.envres.2023.116338
Zaborowska, E., Majtacz, J., and Drewnowski, J. (2018). Improving the energy balance in wastewater treatment plants by optimization of aeration control and application of new technologies. Lublin University of Technology, Lublin, Poland, 2018, 317–328.
Zhang, D. W., Chen, L. W., Zhang, S. H., and Zhen, J. (2023). Denitrification performance and microbial community analysis of sulfur autotrophic denitrification filter for low-temperature treatment of landfill leachate. J. Environ. Chem. Eng. 11:109314. doi: 10.1016/j.jece.2023.109314
Zhang, Y. B., Feng, Y. H., Yu, Q. L., Xu, Z. B., and Xie, Q. (2014). Enhanced high-solids anaerobic digestion of waste activated sludge by the addition of scrap iron. Bioresour. Technol. 159, 297–304. doi: 10.1016/j.biortech.2014.02.114
Zhang, Z., Jiang, C., Zhang, W., Xiao, Z., Huang, X., Hua, T., et al. (2020). Short-term nitrification and de-nitrification test for landfill leachate. J. Saf. Environ. 20, 1083–1089. doi: 10.13637/j.issn.1009-6094.2019.0733
Zhang, X. X., Li, A., Szewzyk, U., and Ma, F. (2016). Improvement of biological nitrogen removal with nitrate-dependent Fe(II) oxidation bacterium Aquabacterium parvum B6 in an up-flow bioreactor for wastewater treatment. Bioresour. Technol. 219, 624–631. doi: 10.1016/j.biortech.2016.08.041
Zhang, Y. H., Ma, Z., Zhang, Z. B., and Sun, C. Z. (2014). Research progress in the influencing factors of autohydrogenotrophic biological denitrification for drinking water. Indust. Water Treat. 5, 1–14. doi: 10.12989/mwt.2014.5.1.001
Zhang, M. Y., Pan, L. Q., Huang, F., Gao, S., Su, C., Zhang, M. Z., et al. (2019). Metagenomic analysis of composition, function and cycling processes of microbial community in water, sediment and effluent of Litopenaeus vannamei farming environments under different culture modes. Aquaculture 506, 280–293. doi: 10.1016/j.aquaculture.2019.03.038
Zhang, Q., Xu, X. J., Zhang, R. C., Shao, B., Fan, K. L., Zhao, L., et al. (2022). The mixed/mixotrophic nitrogen removal for the effective and sustainable treatment of wastewater: from treatment process to microbial mechanism. Water Res. 226:119269. doi: 10.1016/j.watres.2022.119269
Zhang, H. H., Zhao, Z. F., Li, S. L., Chen, S. N., Huang, T. L., Li, N., et al. (2019). Nitrogen removal by mix-cultured aerobic denitrifying bacteria isolated by ultrasound: performance, co-occurrence pattern and wastewater treatment. Chem. Eng. J. 372, 26–36. doi: 10.1016/j.cej.2019.04.114
Zhao, J., Feng, L. J., Yang, G. F., Dai, J. C., and Mu, J. (2017). Development of simultaneous nitrification-denitrification (SND) in biofilm reactors with partially coupled a novel biodegradable carrier for nitrogen-rich water purification. Bioresour. Technol. 243, 800–809. doi: 10.1016/j.biortech.2017.06.127
Zhao, L. F., Xue, L. Y., Wang, L., Liu, C., and Li, Y. (2022). Simultaneous heterotrophic and FeS2-based ferrous autotrophic denitrification process for low-C/N ratio wastewater treatment: nitrate removal performance and microbial community analysis. Sci. Total Environ. 829:154682. doi: 10.1016/j.scitotenv.2022.154682
Zhu, G., Peng, Y., and Guo, J. (2008). Biological nitrogen removal with nitrification and denitrification via nitrite pathway. J. Harbin Inst. Tech. 10, 1552–1557. doi: 10.1007/s00253-006-0534-z
Keywords: novel bio-denitrification technology, deep wastewater denitrification, coupling process, denitrification performance, low-carbon
Citation: Huang S, Fu YL, Zhang HM, Wang CQ, Zou CL and Lu XG (2023) Research progress of novel bio-denitrification technology in deep wastewater treatment. Front. Microbiol. 14:1284369. doi: 10.3389/fmicb.2023.1284369
Received: 28 August 2023; Accepted: 20 September 2023;
Published: 04 October 2023.
Edited by:
Huike Dong, Institute of Tibetan Plateau Research (CAS), ChinaReviewed by:
Jingran Zhang, Chinese Academy of Sciences (CAS), ChinaCopyright © 2023 Huang, Fu, Zhang, Wang, Zou and Lu. This is an open-access article distributed under the terms of the Creative Commons Attribution License (CC BY). The use, distribution or reproduction in other forums is permitted, provided the original author(s) and the copyright owner(s) are credited and that the original publication in this journal is cited, in accordance with accepted academic practice. No use, distribution or reproduction is permitted which does not comply with these terms.
*Correspondence: Xiuguo Lu, MzMyNkBlY2p0dS5lZHUuY24=
Disclaimer: All claims expressed in this article are solely those of the authors and do not necessarily represent those of their affiliated organizations, or those of the publisher, the editors and the reviewers. Any product that may be evaluated in this article or claim that may be made by its manufacturer is not guaranteed or endorsed by the publisher.
Research integrity at Frontiers
Learn more about the work of our research integrity team to safeguard the quality of each article we publish.