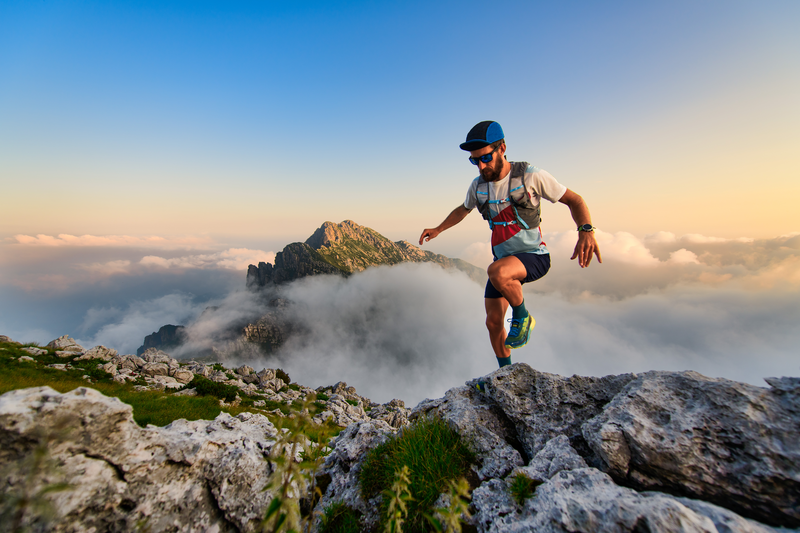
94% of researchers rate our articles as excellent or good
Learn more about the work of our research integrity team to safeguard the quality of each article we publish.
Find out more
ORIGINAL RESEARCH article
Front. Microbiol. , 08 January 2024
Sec. Microbial Symbioses
Volume 14 - 2023 | https://doi.org/10.3389/fmicb.2023.1284276
Introduction: Aspergillus nomiae is known as a pathogenic fungus that infects humans and plants but has never been reported as an entomophagous fungus (EPF) that can provide other functions as an endotype.
Methods: A strain of EPF was isolated and identified from diseased larvae of Spodoptera litura in a soybean field and designated AnS1Gzl-1. Pathogenicity of the strain toward various insect pests was evaluated, especially the ability to colonize plants and induce resistance against phytopathogens and insect pests.
Results: The isolated EPF strain AnS1Gzl-1 was identified as A. nomiae; it showed strong pathogenicity toward five insect pests belonging to Lepidoptera and Hemiptera. Furthermore, the strain inhibited the growth of Sclerotinia sclerotiorum in vitro, a causal agent of soil-borne plant disease. It colonized plants as an endophyte via root irrigation with a high colonization rate of 90%, thereby inducing plant resistance against phytopathogen infection, and disrupting the feeding selectivity of S. litura larvae.
Discussion: This is the first record of a natural infection of A. nomiae on insects. A. nomiae has the potential to be used as a dual biocontrol EPF because of its ability to not only kill a broad spectrum of insect pests directly but also induce resistance against phytopathogens via plant colonization.
The long-term and large-scale use of chemical insecticides not only enhances the physiological resistance of insect pests but also affects the population of important natural enemy species that cause environmental pollution, imbalance of the field community structure, and other negative effects on the ecosystem (Karthi et al., 2018). In the theory of integrated pest management (IPM), entomophagous fungi (EPF) have become one of the most critical tools in the population control of agricultural insect pests and are considered safe alternatives to chemical insecticides (Masoudi et al., 2018), which can directly infect and penetrate the host and cause disease (Arunthirumeni et al., 2023). It is more interesting that EPF can control insect pests by a sustainable effect, due to which they can continue to grow until the hyphae invade all of the tissues and organs and penetrate the host cuticle to produce conidia. After the death of the insect, the conidia can form a new infection cycle by natural transmission and then infect other insect hosts (Conceschi Marcos et al., 2016; Mora Margy et al., 2018).
Currently, more than 1,000 species of EPF have been reported, including commercially applied Metarhizium anisopliae, Beauveria bassiana, Isaria fumosorosea, and Metarhizium rileyi (Kim Jae et al., 2013; Grijalba Erika et al., 2018; Jiang et al., 2019; Malinga Lawrence and Mark, 2021; Wang et al., 2021; Acharya et al., 2022; Faria et al., 2022; Im et al., 2022), as well as literature reported Paecilomyces variotii, Hirsutella citriformis, and Akanthomyces lecanii (Gandarilla-Pacheco et al., 2013; Cortez-Madrigal et al., 2014; Hussain et al., 2018; Naeem et al., 2020). However, the resources of EPF are insufficient due to their host specificity, which could affect their commercial production and application (Wichadakul et al., 2015; Zhang et al., 2020; Islam et al., 2021). Therefore, it is of significance to obtain new EPF strains with high pathogenicity against insect pests for the development of fungal insecticides.
In recent years, EPF strains with multiple control effects on plant disease and insect pests are gradually becoming a research hotspot, which means that the EPF can not only directly kill insects, but also form symbioses with plants through endophytic colonization, via various methods including foliar spraying, seed treatment, root irrigation, and injection (Ownley et al., 2008; Greenfield et al., 2016; Bamisile et al., 2019; Hu and Bidochka, 2021). It is reported that some EPF strains can promote the biomass of plants after colonization (Bamisile et al., 2018; Deb et al., 2022; Altaf et al., 2023), especially having the ability to affect the growth and development of pests and subsequently reducing their survival and reproduction (González-Mas et al., 2021; Altaf et al., 2023). Moreover, the colonization of EPF in plants can inhibit phytopathogen growth and induce plant resistance against infection (Barra-Bucarei et al., 2019; Gange et al., 2019; Canassa et al., 2020).
In the present study, a strain of EPF was isolated from muscardine cadaver larvae of Spodoptera litura, which was collected from a soybean field in Jilin Province, China, and characterized by morphological and molecular methods. Pathogenicity of the EPF isolates toward important Lepidoptera larvae, including S. litura, Chilo suppressalis, and Ostrinia furnacalis, was tested, along with Hemiptera pest adults, Rhopalosiphum padi and Acyrthosiphon pisum. The endophytic colonization ability in soybean plants, the feeding selection effect on S. litura larvae, and the inhibitory effect on the phytopathogenic fungus Sclerotinia sclerotiorum induced by colonization were explored. The findings provide new resources for the development of biopesticides with multiple control measures against plant disease and insect pests.
Muscardine cadaver larvae of S. litura were collected from a soybean field in Gongzhuling City, Jilin Province, China (N43° 50′42″, E124° 82′58″).
Tested insects including Lepidoptera pest larvae S. litura, Chilo suppressalis, and O. furnacalis, as well as Hemiptera pest adults R. padi and A. pisum, which were maintained on regular artificial diets, were provided by Professor Wenjing Xu from the Plant Protection Institute of Jilin Academy of Agricultural Sciences.
Commercialized Jiyu 303 soybean seeds were bred by the Soybean Research Institute of Jilin Academy of Agricultural Sciences.
The S. sclerotiorum strain isolated and characterized from soybean was provided by Professor Jinliang Liu, Jilin University, China.
Muscardine cadaver larvae of S. litura were disinfected with 75% (v/v) anhydrous ethanol for 30 s and with 1% (v/v) sodium hypochlorite for 2 min; rinsed with sterile water three times; the surface water was absorbed with a sterile filter paper; cut into five sections using a sterile scalpel; placed on Potato Dextrose Agar (PDA) medium (Sangon Biotech, Shanghai, China), which contained 1 mL ampicillin per liter at a concentration of 100 μg/mL; and incubated in a constant temperature incubator at 26°C for 5–7 days until mycelium grew. The strain was purified by the single-spore isolation method as described previously (Ghalehgolabbehbahani et al., 2022), and the purified strain was designated AnS1Gz1-1.
Monospores of the purified strain were picked and inoculated on PDA medium, and plates were incubated in a constant temperature incubator at 26°C. When spore mounds were observed with the naked eye, the morphology of conidia and hyphae was observed and recorded under a light microscope LW300-48LT (Siwei Optoelectronic Technology Co., Ltd., Xi’an, China).
Purified monospores were inoculated on the PDA medium for 7 days until sporulation, conidia were collected and suspended in a sterile 0.1% (v/v) Tween-80 solution at a concentration of 1 × 108 conidia /mL, evenly applied to the culture medium, and incubated in a constant temperature incubator at 26°C for 10 days. Fungal bodies were scraped off using a sterile glass slide for DNA extraction according to the description provided by Watanabe et al. (2010). Three genes were employed for molecular identification of the EPF strain according to Hong et al. (2005) by PCR amplification, including the internal transcribed spacer (ITS) gene with primers ITS1 (5′-TCCGTAGGTGAACCTGCG-3′) and ITS4 (5′-TCCTCCGCTTATTGATATGC-3′), a segment of the β-tubulin gene (BenA) with primers βtub1(5′ -AATTGGTGCCGCTTTCTGG-3′) and βtub2 (5′-AGTTGTCGGGACGGAATAG-3′) (Balajee et al., 2005), as well as a segment of the calmodulin gene (CaM) with primers cmd5 (5′-CCGAGTACAAGGAGGCCTTC-3′) and cmd6 (5′-CCGATAGAGGTCATAACGTGG-3′) (submitted separately to GenBank with the accession numbers OR801769, OR801770, and OR801771). The PCR reaction systems (30 μL) included 15 μL of PCR Mix (Nanjing Novozan Biotechnology Co., Ltd.), 11 μL ddH2O, 1.5 μL forward primer, 1.5 μL reverse primer, and 1 μL genomic DNA. Thermal cycling was performed as follows: initial denaturation of 94°C for 3 min, 30 cycles of 94°C for 1 min; 55°C for 30 s, and 72°C for 30 s, respectively; followed by a final extension period of 72°C for 5 min. The PCR products were cloned in a pMD18-T vector (Takara, Dalian, China) and transferred into Escherichia coli DH5ɑ. The plasmid DNA isolated from Luria-Bertani cultures was sequenced in both forward and reverse directions by Sangong Biotech Ltd. (Shanghai, China). The forward and reverse sequencing results were spliced using DANman software (V6.0.3.99). The sequences of the three genes were submitted to GenBank separately to obtain the accession number. The phylogenetic trees were inferred by using the maximum likelihood method with a bootstrap consensus tree inferred from 1,000 replicates (Thompson et al., 2003). The evolutionary distances were computed using the Kimura 2-parameter method. The phylogenetic tree was conducted in MEGA X software (MEGA Inc., Englewood, United States). The tree was rooted to Talaromyces flavus CBS 310.38.
Cultivated conidia on PDA medium were suspended in sterile 0.1% (v/v) Tween-80 solution at a concentration of 1 × 108 conidia /mL, and sterile 0.1% (v/v) Tween-80 served as a control. Uniformly sized second instar larvae of S. litura, C. suppressalis, and O. furnacalis, as well as adults of R. padi and A. pisum, were selected for virulence determination by impregnation assay as described previously (Faria et al., 2022). After inoculation, larvae of S. litura, C. suppressalis, and O. furnacalis were placed in a 24-well culture plate, while R. padi and A. pisum were placed in sterile culture boxes and maintained at 25 ± 1°C with 70 ± 1% relative humidity and a 16 h light:8 h dark photoperiod. Three repetitions were performed with each treatment including 20 insects. Infection and death of insects were assessed every 24 h, as were the external characteristics of infected insects. Insects were considered dead if there was no sign of activity after gently touching the inoculated insect body with sterile forceps. Corrected mortality rates for each treatment group were recorded, and probit linear regression analysis was employed to calculate the median lethal time (LT50) of tested fungi against different insects as described previously (Liu et al., 2022) using the following formula:
Corrected mortality (%) = (mortality of treatment − mortality of control)/(1 − mortality of control) × 100.
Dead insect bodies were removed in a timely manner using sterile tweezers and placed in a sterile culture dish (ɸ = 9 cm) lined with sterile filter paper. Insect bodies were cultivated in a light incubator at 25 ± 1°C with 70 ± 1% relative humidity and a 16 h light: 8 h dark photoperiod. Growth of mycelium and conidia on the surface of insect bodies was observed, and the morphology of mycelium and conidia was examined under a microscope (Leica TCS SP8, Leica Microsystems, Wetzlar, Germany).
The plate confrontation method was employed to assess the in vitro inhibition activity of tested fungi against S. sclerotiorum as described previously (Nzabanita et al., 2022). A 5-mm-diameter fungal block was taken from the edge of the active growing fungal pathogen colony (cultured for 5–7 days on PDA medium) and inoculated in the center of a PDA plate. Two 5-mm-diameter fungal blocks from the AnS1Gzl-1 strain cultured for 5–7 days were inoculated at a distance of 1.5 cm on both sides of the fungal pathogen block on the same line, while the control group was only inoculated with the fungal pathogen block, the plates were cultured at 26°C, observed and photographed at 5 days post-inoculation. Each treatment and control were repeated five times.
Twenty surface-sterilized soybean leaves were selected, 10 leaves were used to inoculate with AnS1Gz1-1 strain, and the others were used as control. The selected soybean leaves were surface-sterilized as described previously (Sui et al., 2020). The trifoliate leaves were removed with sterile scissors and placed on a culture dish with moist filter paper. The moist cotton ball was placed at the petiole for moisture, and 5-mm-diameter fungal blocks of AnS1Gzl-1 strain were used for leaf surface inoculation (avoiding leaf veins), which were collected from the edge of the active growing fungal pathogen colony cultured for 5–7 days on PDA medium. The remaining 10 leaves were inoculated with blocks of culture medium without pathogen inoculation as a control. The fungus-inoculated and medium-inoculated leaves were incubated at 25 ± 1°C with relative humidity maintained above 70% and a 16 h light:8 h dark photoperiod. The symptom of soybean leaves was observed and recorded for 3 days after inoculation.
Healthy soybean plants with consistent growth at the compound leaf stage were selected and irrigated with a conidia suspension (1 × 108 conidia/mL) of strain AnS1Gzl-1. A 0.1 (v/v) Tween-80 solution served as a control. Each treatment included three replicates with 20 seedlings per replicate, and the volume of the root irrigation solution was 20 mL. The colonization rate of strain AnS1Gzl-1 in soybean leaves was assessed by observation of fungal colonies grown from plating surface-sterilized leaf segments on PDA for 14 days as described previously (Sui et al., 2020).
For in vitro disease resistance evaluation, there were four treatment groups. The soybean seedlings were treated by conidia suspension (1 × 108 conidia/mL) of AnS1Gzl-1 strain and 0.1 (v/v) Tween-80 solution via root irrigation, and the colonization seedlings were verified according to the above description. The second healthy soybean trifoliate leaves from top to bottom of seedlings that were positively colonized by AnS1Gzl-1 were removed and inoculated with and without S. sclerotiorum, and the seedlings treated with Tween-80 were also inoculated with and without S. sclerotiorum. Three replicates (20 leaves per replicate) were included for each treatment. Leaf disinfection treatment and phytopathogen inoculation were performed according to the description provided in Section 2.6, in which the strain AnS1Gz1-1 was replaced by S. sclerotiorum (cultured for 5–7 days on PDA medium). The incidence rate of soybean leaves was observed and recorded at 4, 5, 6, and 7 days post-inoculation (dpi); the disease incidence was calculated as follows: incidence rate (%) = (number of incidents/total number of inoculated plants) × 100 (Li et al., 2023). The diameter of disease spots on diseased leaves in each treatment was calculated by the cross method at 4 dpi (Sui et al., 2022).
A sterile plastic culture dish with a diameter of 14 cm was lined with filter paper, an appropriate amount of distilled water was added to keep the filter paper moist, and five pieces of 1 cm × 1 cm soybean leaves colonized or not colonized with fungal strain AnS1Gz1-1, of the same size along the circumference, were spaced evenly on the plate. For the non-selective test, five pieces of colonized and not colonized leaves were placed in different Petri dishes. In both selective and non-selective experiments, 20 s instar larvae of S. litura were inoculated in the center of the filter paper and transferred to a feeding room at 26 ± 1°C with a relative humidity of 60 ± 10% and a 14 h light:10 h dark photoperiod. Both experiments were repeated five times. The number of larvae on each leaf was recorded at 1, 1.5, 2, 2.5, 3, 3.5, 4, 4.5, 5, 5.5, and 6 h after inoculation, and the feeding rate of pests on soybean leaves was calculated using the following formula (Russo et al., 2019):
Feeding rate (%) = (number of insects feeding on soybean leaves/20) × 100.
All experimental data were analyzed using IBM SPSS Statistics 25 software (IBM Corp., Armonk, United States) for one-way analysis of variance (ANOVA; p < 0.05) and plotted using GraphPad Prism 8.0.2 (GraphPad Software Inc., San Diego, United States).
The infected fungal strain from muscardine fungus-infected larvae of S. litura in the soybean field was isolated and purified, designated as AnS1Gz1-1. The fungal strain was cultured on PDA medium with antibiotic, and colonies were yellow (Figures 1A,B). Observation under a light microscope revealed that conidia were single-celled and rough-walled with a diameter of spheres measuring approximately 3–5 μm in size, and hyphae were colorless, separated, and branched (Figure 1C). This morphology matched the description of the fungus given by Zhou et al. (2020).
Figure 1. Biological characteristics of A. nomiae strain AnS1Gzl-1. (A) Colony front on PDA medium after inoculation for 10 days. (B) Colony back on PDA medium after inoculation for 10 days. (C) Conidia and hyphae of the fungal strain.
DNA fragment sequencing results showed that the length of genes ITS, BenA, and CaM of the strain were 586, 433, and 528 bp, respectively. The DNA sequences were submitted to GenBank and assigned the accession numbers OR425151.1, OR450636.1, and OR450635.1, respectively. The DNA sequences by Blastn comparison in Genbank showed that the strain was 99–100% similar to the reported A. nomiae strain. Phylogenetic tree analysis indicated that the strain clustered with the A. nomiae strain CBS 260.88 (Figure 2), which confirmed our morphological identification that the strain belongs to A. nomiae.
Figure 2. Phylogenetic analysis of combined ITS, BenA, and CaM sequence data. Numbers at branches represent bootstrap values from 1,000 replicates in the maximum likelihood method analysis, respectively. The scale represents genetic distance. *Branches support below 50%.
The pathogenicity of strain AnS1Gz1-1 against five important agricultural insect pests was explored. The results showed that AnS1Gz1-1 infection caused muscardine cadavers for all tested insects, whose body surface was covered with clearly visible conidia (Figures 3A–E), the colony and conidia morphologies following reisolation from dead insect bodies were consistent with those of strain AnS1Gz1-1. The EPF strain AnS1Gz1-1 showed high but varying virulence against the five tested insects. The pathogenicity of strain AnS1Gz1-1 toward R. maidis, S. litura, and C. pisum was strongest; the mortality rate of S. litura treated with the fungus was 90% at 5 days post-inoculation, the mortality rate of O. furnacalis treated with the fungus was 80% at 8 days post-inoculation, and the weakest virulence was to C. supperssalis, which mortality rate less than 80% at 9 days post-inoculation (Figure 3F). LT50 was determined to establish the pathogenicity of strain AnS1Gzl-1 against all five insect pests (Table 1), and the lowest LT50 of 2.212 days was against S. litura, while the highest was 5.376 days against C. supperssalis, and virulence against the two Hemiptera insect pests (R. maidis and C. pisum) was relatively high with LT50 values of 2.524 and 3.128 days, respectively.
Figure 3. Virulence evaluation of Aspergillosis nomiae strain AnS1Gzl-1 against insect pests. (A–E) Muscardine cadavers of five kinds of insect pests infected by A. nomiae strain AnS1Gzl-1. (F) Corrected mortality of tested insects following infection by A. nomiae strain AnS1Gzl-1. Different lowercase letters indicate significant differences in corrected death rates of different strains tested by Duncan’s new complex range method (p < 0.05).
After inoculation on PDA medium, colonies of S. sclerotiorum and EPF strain gradually expanded over time, and when they met, an obvious antagonistic circle was observed around EPF colonies, while colony growth of S. sclerotiorum cocultured with EPF strain was lower than when cultured alone (Figure 4). This indicates that A. nomiae could significantly inhibit the growth of S. sclerotiorum in vitro.
Figure 4. Confrontation cultures of Sclerotinia sclerotiorum and Aspergillosis nomiae in vitro at 5 days post-inoculation. The top row corresponds to S. sclerotiorum colonies cultured on medium, and the bottom row corresponds to confrontation cultures of S. sclerotiorum and A. nomiae on PDA medium.
After root irrigation with strain AnS1Gzl-1, leaf pieces of inoculated and non-inoculated plants with EPF were cultured on a PDA medium. On day 4 of cultivation, white fungal mycelium began to grow from the edge of soybean leaf tissue irrigated with the AnS1Gzl-1 strain, which was not observed in controls (Figures 5A,B), indicating that the AnS1Gzl-1 strain had the ability to colonize soybean plants through root irrigation. As shown in Figure 5C, the colonization rate of the fungus in soybean plants was 90%.
Figure 5. Colonization detection of Aspergillosis nomiae strain AnS1Gzl-1 in soybean plants. (A) Blank controls. (B) Inoculated with A. nomiae strain AnS1Gzl-1. (C) Colonization rate (ANOVA, ***p < 0.001).
In the feeding experiment, within 6 h after inoculation, the feeding rate of second instar larvae of S. litura on leaves of the control group was consistently higher than that on leaves of the A. nomiae colonization group in either selective (Figure 6A) or non-selective (Figure 6B) experiments. Endophytic colonization of A. nomiae had a deterrent effect on S. litura larvae feeding.
Figure 6. Feeding selectivity of second instar larvae of Spodoptera litura on Aspergillosis-soybean symbiosis. (A) Feeding rate of leaves with and without A. nomiae colonization in the selective test. (B) Feeding rate of leaves with and without A. nomiae colonization in the non-selective test (ANOVA, *p < 0.05, **p < 0.01, ***p < 0.001).
In vitro assays were performed to determine the resistance of A. nomiae against S. sclerotiorum following endophytic colonization in plants. The results showed that on 3 days post-inoculation of S. sclerotiorum, soybean leaves began to suffer from disease. Over time, the disease incidence rate on leaves was increased in both the A. nomiae colonization group and the control group, but compared with controls, the incidence rate of leaves colonized by A. nomiae was significantly lower. After 7 days post-inoculation, the incidence rate on soybean leaves in the group inoculated with S. sclerotiorum alone was 83.33 ± 5.77%, compared with 60.00 ± 0.00%(p < 0.001, F = 27.500) in the A. nomiae colonization group (Figure 7A).
Figure 7. Disease resistance induction by Aspergillosis nomiae as an endophyte. (A) Incidence rate of different treatments at different times following phytopathogen inoculation. (B) Symptoms of A. nomiae inoculated soybean leaves. (C) Lesion diameter of different treatments on day 4 following phytopathogen inoculation. (D) Symptoms of soybean leaves for different treatments at 4 days after phytopathogen inoculation. Different lowercase letters indicate significant differences in corrected death rates of different strains tested by Duncan’s new complex range method (p < 0.05).
On day 3 post-inoculation, soybean leaves showed no symptoms of pathogen infection in either strain AnS1Gzl-1 (An) or culture groups, which means A. nomiae had no pathogenicity to soybean leaves (Figure 7B). For the assay of induced disease resistance by AnS1Gzl-1 colonization, on day 4 post-inoculation, the diameter of the disease lesions on soybean leaves in the A. nomiae colonization group (An+Ss) was significantly smaller than that in the group inoculated only with the pathogen (Ss). The control and A. nomiae colonization (An) groups exhibited no symptoms, and hence, A. nomiae colonization was not harmful to plants (Figures 7C,D). Symptoms of soybean leaves displayed only partial yellowing around pathogen inoculation sites in the A. nomiae colonized groups (An+Ss), whose lesion diameter on day 4 following phytopathogen inoculation was 11.72 ± 4.36 mm, significantly smaller than that of the phytopathogen inoculation only group (Ss), which was 15.36 ± 2.70 mm (p < 0.001, F = 6.918), while almost the half to whole of leaves displayed yellow color in the group inoculated with pathogen alone (Figure 7D).
EPF strains are important biological control agents for insect pests that cause significant yield loss and quality decline in agriculture and forestry. In previous studies, research on EPF resources mainly focuses on Beauveria spp., Metarhizium spp., and Lecanicillium spp. (Bamisile et al., 2021). Abundant EPF resources can provide more efficient biological control of pests. It is necessary to constantly explore new EPFs with high efficiency and broad spectrum. Aspergillus species are diverse fungi that are widely distributed in nature with multiple biological effects. The biocontrol effect on insect pests of some Aspergillus species has been reported, such as A. flavus, A. nomius, A. fijiensis, and A. oryzae, which could be used to control Locusta migratoria, S. litura, Diaphorina citri, and Dolichoderus thoracicus (Zhang et al., 2015; Karthi et al., 2018; Lin et al., 2021; Yan et al., 2022). It has been reported that A. nomiae is not only morphologically similar to A. flavus, but also can cause fatal rhinofacial infection in humans, but with resistance to antifungal amphotericin B (AMB) (Zhou et al., 2020). Here, as the first record, we isolated and identified a strain of A. nomiae AnS1Gzl-1 that caused muscardine cadaver of S. litura larvae naturally, with relatively high pathogenicity to a variety of agricultural insect pests, and can be used as a new broad-spectrum EPF for insect pest control. Importantly, further research is needed to determine whether the pathogenic mechanism of A. nomiae on insects is the same as that of humans, especially, whether its application as a biological insecticide in the field will pose a risk of infection to humans and animals. Nevertheless, our molecular identification results indicated that A. nomiae AnS1Gzl-1 is more closely related to A. pseudonomiae (a non-pathogenic pathogen) in evolution, while it is more distant from A. flavus. The results are similar to a previous study from Hatmaker et al. (2022). There were only 46 secondary metabolite gene synthesis clusters related to the infection in its genome and it was 16–20 fewer compared with that in the pathogenic A. flavus.
Previous studies have proven that EPF can not only kill insect pests, but also establish obvious biological effects against insect pests via colonization in plants, including toxicity, developmental disorders, and avoidance. For example, endophytic colonization by B. bassiana and M. anisopliae in maize plants affects the fitness of S. frugiperda, and both strains negatively impact the development and fecundity of S. frugiperda (Altaf et al., 2023). Sinno et al. (2021) found that aphids reared on B. bassiana-treated plants for their entire lifespan were negatively affected in terms of survival and fertility in comparison with control cohorts; Mseddi et al. (2022) screened a wild strain of B. bassiana B12 that not only promoted several plant growth parameters after root inoculation, but also displayed toxicity toward A. gossypii following colonization in cotton plants. Zhu et al. (2023) found that B. bassiana-inoculated maize plants can influence the eggs laying selectivity of O. furnacalis females by changing plant volatile profiles; and González-Mas et al. (2021) found that when the cotton was colonized by B. bassiana, they emit a different blend of volatile compounds compared to uncolonized control plants, which can affect the feeding behavior of insect pests A. gossypii, S. frugiperda, and S. littoralis. Here, we have similar results that A. nomiae had the ability of plant colonization and showed a significant repellent effect on S. litura larvae, an important insect pest on soybean, in both selective and non-selective assays. In short, it indicates that A. nomiae not only directly kills insects but also has the potential to achieve ecological control of pests through endogenous colonization.
A. nomiae was also known as a causal agent of plant disease due to its high incidence in Brazil nuts (Bertholletia excelsa H.B.K.) and its strong production of carcinogenic metabolites (Reis et al., 2022). In the present study, we found that A. nomiae strain AnS1Gzl-1 causes no damage to plants by either inoculating it on the surface of soybean leaves or colonizing it in plant tissues through root irrigation. However, A. nomiae strains have been reported to produce both series B and G aflatoxins (Ehrlich et al., 2004), which are notorious for hepatocellular carcinoma development, lung adenocarcinoma, and chronic inflammatory changes (Liu et al., 2015); hence, it is necessary to clarify whether toxins are metabolized by A. nomiae strain AnS1Gzl-1 in its endophytic plants in a future study.
Numerous EPF strains have a plant disease control effect via endogenous colonization (Bamisile et al., 2018; Vega, 2018). Some strains showed no directly antagonistic effects against pathogens in vitro but can improve plant resistance to diseases following endogenous colonization by altering the phyllosphere microbiome of maize after colonization (Chang et al., 2021) or regulating salicylic acid (SA) and jasmonic acid (JA) pathways (Qin et al., 2021; Gupta et al., 2022). Nevertheless, EPF exhibited direct antagonism against pathogens via antibiosis, competition, or parasitism through the production of fungal secondary metabolites (Ownley et al., 2008; Vega et al., 2009). Previous research has found that Aspergillus species could produce various beneficial compounds such as cyclosporine A, asperfuranone, terrrein, itaconic acid, kojic acid, citric acid, and gluconic acid (Sun et al., 2018; Wu et al., 2019; Chigozie et al., 2022), which showed antifungal, antibacterial, and phytotoxic activities, and played a crucial role to antagonize the devastating phytopathogens (Ding et al., 2019). It was found that A. versicolor had the ability to inhibit the destructive phytopathogen Macrophomina phaseolina. In dual culture bioassays, A. versicolor showed potential antagonist activity and reduced the pathogen’s growth by 60% over control, with the mechanism of which secondary metabolites can degrade the DNA of pathogenic fungi (Khan and Javaid, 2022). Herein, A. nomiae strain AnS1Gzl-1 inhibited S. sclerotiorum growth on the PDA medium as evidenced by an obvious antagonistic zone, and it significantly improved resistance of plants against S. sclerotiorum infection on soybean leaves following colonization. Furthermore, it is necessary to clarify whether there are antagonistic products metabolized from A. nomiae that inhibit the growth of phytopathogens in future studies. To the best of our knowledge, there have been few reports on the biocontrol effects against soil-borne diseases and their causal agents induced by endophytic colonization of EPF; only Metarhizium spp. and B. bassiana have been mentioned for the control of Fusarium culmorum, Rhizoctonia spp., and S. sclerotiorum in the literature (Jaber, 2018; Raad et al., 2019; Tomilova et al., 2020; Deb et al., 2023). Here, we found that A. nomiae strain AnS1Gzl-1 has great potential for sclerotinia disease control, although its mechanism of resistance requires further investigation.
In summary, we isolated a strain of A. nomiae from diseased S. litura larvae on soybean in a field, which had broad-spectrum direct insecticidal effects on various insect pests. It is the first record that A. nomiae is an EPF and has potential as a biological insecticide. Another important finding, the strain could indirectly control insect pests and phytopathogen damage via endophytic colonization without damage to host plants like other EPFs.
The datasets presented in this study can be found in online repositories. The names of the repository/repositories and accession number(s) can be found in the article/supplementary material.
ZZ: Conceptualization, Funding acquisition, Writing – original draft. YT: Investigation, Methodology, Validation, Writing – original draft. LS: Formal analysis, Resources, Software, Writing – original draft. YL: Data curation, Methodology, Validation, Writing – original draft. KC: Data curation, Software, Visualization, Writing – original draft. YZ: Data curation, Investigation, Writing – original draft. QL: Conceptualization, Writing – review & editing. WS: Conceptualization, Visualization, Writing – review & editing.
The author(s) declare financial support was received for the research, authorship, and/or publication of this article. This study was supported by the Jilin Agricultural Science and Technology Innovation Project (grant no. CXGC2021ZD008) and the Jilin Project for Outstanding Talents (Teams) for Innovation and Entrepreneurship in Science and Technology (grant no. 20230508011RC).
The authors would like to thank the native English-speaking scientists of Elixigen Company (Huntington Beach, California) for editing our manuscript.
The authors declare that the research was conducted in the absence of any commercial or financial relationships that could be construed as a potential conflict of interest.
All claims expressed in this article are solely those of the authors and do not necessarily represent those of their affiliated organizations, or those of the publisher, the editors and the reviewers. Any product that may be evaluated in this article, or claim that may be made by its manufacturer, is not guaranteed or endorsed by the publisher.
Acharya, R. J. Y., Lee, H. S., Hwang, M. K., Kim, S. Y., Lee, H. Y., Jung, I., et al. (2022). Indentification of entomopathogenic fungus Metarhizium rileyi infested in fall armyworm in the cornfield of Korea, and evaluation of its virulence. Arch. Insect Biochem. Physiol. 111:e21965. doi: 10.1002/arch.21965
Altaf, N. M. I., Ullah, M., Afzal, M., Arshad, S., Ali, M., Rizwan, L. A., et al. (2023). Endophytic colonization by Beauveria bassiana and Metarhizium anisopliae in maize plants affects the fitness of Spodoptera frugiperda (Lepidoptera: Noctuidae). Microorganisms 11:1067. doi: 10.3390/microorganisms11041067
Arunthirumeni, M., Gunasekar, V., and Muthugounder, S. S. (2023). Antifeedant and larvicidal activity of bioactive compounds isolated from entomopathogenic fungi Penicillium sp. for the control of agricultural and medically important insect pest (Spodoptera litura and Culex quinquefasciatus). Parasitol. Int. 92:102688. doi: 10.1016/j.parint.2022.102688
Balajee, S. A., Jennifer, L. G., Edward, H., David, N., and Kieren, A. M. (2005). Aspergillus lentulus sp. nov., a new sibling species of A. fumigatus. Eukaryot. Cell 4, 625–632. doi: 10.1128/EC.4.3.625-632.2005
Bamisile, B. S., Akutse, K. S., Siddiqui, J. A., and Xu, Y. (2021). Model application of entomopathogenic fungi as alternatives to chemical pesticides: prospects, challenges, and insights for next-generation sustainable agriculture. Front. Plant Sci. 12:741804. doi: 10.3389/fpls.2021.741804
Bamisile, B. S., Chandra, K. D., Komivi, S. A., Muhammad, Q., Luis, C. R. A., Wang, F., et al. (2019). Endophytic Beauveria bassiana in foliar-treated citrus limon plants acting as a growth suppressor to three successive generations of Diaphorina citri kuwayama (Hemiptera: Liviidae). Insects 10:176. doi: 10.3390/insects10060176
Bamisile, B. S., Dash, C. K., Akutse, K. S., Keppanan, R., Afolabi, O. G., Hussain, M., et al. (2018). Prospects of endophytic fungal entomopathogens as biocontrol and plant growth promoting agents: an insight on how artificial inoculation methods affect endophytic colonization of host plants. Microbiol. Res. 217, 34–50. doi: 10.1016/j.micres.2018.08.016
Barra-Bucarei, L., France Iglesias, A., Gerding González, M., Silva Aguayo, G., Carrasco-Fernández, J., Castro, J. F., et al. (2019). Antifungal activity of Beauveria bassiana endophyte against Botrytis cinerea in two solanaceae crops. Microorganisms 8:65. doi: 10.3390/microorganisms8010065
Canassa, F., Esteca, F. C. N., Moral, R. A., Meyling, N. V., Klingen, I., and Delalibera, I. (2020). Root inoculation of strawberry with the entomopathogenic fungi Metarhizium robertsii and Beauveria bassiana reduces incidence of the twospotted spider mite and selected insect pests and plant diseases in the field. J. Pest. Sci. 93, 261–274. doi: 10.1007/s10340-019-01147-z
Chang, Y. M., Xia, X. Y., Sui, L., Kang, Q., Lu, Y., Li, L., et al. (2021). Endophytic colonization of entomopathogenic fungi increases plant disease resistance by changing the endophytic bacterial community. J. Basic Microbiol. 61, 1098–1112. doi: 10.1002/jobm.202100494
Chigozie, V. U., Kezie, M. U. O., Ajaegbu, E. E., Okoye, F. B., and Esimone, C. O. (2022). Bioactivities and HPLC analysis of secondary metabolites of a morphologically identified endophytic aspergillus fungus isolated from Mangifera indica. Nat. Prod. Res. 36, 5884–5888. doi: 10.1080/14786419.2021.2021517
Conceschi Marcos, R., Celeste, P. D., Rafael, D. A. M., Clarice, G. B. D., and Italo, D. J. (2016). Transmission potential of the entomopathogenic fungi Isaria fumosorosea and Beauveria bassiana from sporulated cadavers of Diaphorina citri and Toxoptera citricida to uninfected D. citri adults. BioControl 61, 567–577. doi: 10.1007/s10526-016-9733-4
Cortez-Madrigal, H., Sánchez-Saavedra, J. M., Díaz-Godínez, G., and Gustavo, M. A. (2014). Enzymatic activity and pathogenicity of entomopathogenic fungi from central and southeastern Mexico to Diaphorina citri (Hemiptera: Psyllidae). Southwest. Entomol. 39, 491–502. doi: 10.3958/059.039.0310
Deb, L., Dutta, P., Mandal, M. K., and Singh, S. B. (2023). Antimicrobial traits of Beauveria bassiana against Rhizoctonia solani, the causal agent of sheath blight of rice under field conditions. Plant Dis. 107, 1739–1756. doi: 10.1094/pdis-04-22-0806-re
Deb, L., Dutta, P., Tombisana, D. R. K., Thakuria, D., and Majumder, D. (2022). Endophytic Beauveria bassiana can protect the rice plant from sheath blight of rice caused by Rhizoctonia solani and enhance plant growth parameters. Arch. Microbiol. 204:587. doi: 10.1007/s00203-022-03211-2
Ding, L., Xu, P., Li, T., Liao, X., He, S., and Xu, S. (2019). Asperfurandiones a and B, two antifungal furandione analogs from a marine-derived fungus aspergillus versicolor. Nat. Prod. Res. 33, 3404–3408. doi: 10.1080/14786419.2018.1480622
Ehrlich, K. C., Chang, P. K., Yu, J., and Cotty, P. J. (2004). Aflatoxin biosynthesis cluster gene cypA is required for G aflatoxin formation. Appl. Environ. Microbiol. 70, 6518–6524. doi: 10.1128/aem.70.11.6518-6524.2004
Faria, M., Souza, D. A., Sanches, M. M., Schmidt, F. G. V., Oliveira, C. M., Benito, N. P., et al. (2022). Evaluation of key parameters for developing a Metarhizium rileyi-based biopesticide against Spodoptera frugiperda (Lepidoptera: Noctuidae) in maize: laboratory, greenhouse, and field trials. Pest Manag. Sci. 78, 1146–1154. doi: 10.1002/ps.6729
Gandarilla-Pacheco, F. L., Galán-Wong, L. J., López-Arroyo, J. I., Rodríguez-Guerra, R., and Quintero-Zapata, I. (2013). Optimization of pathogenicity tests for selection of native isolates of entomopathogenic fungi isolated from citrusgrowing areas of México on adults of Diaphorina citri Kuwayama (Hemiptera: Liviidae). Fla. Entomol. 96, 187–195. doi: 10.1653/024.096.0125
Gange, A. C., Koricheva, J., Currie, A. F., Jaber, L. R., and Vidal, S. (2019). Meta-analysis of the role of entomopathogenic and unspecialized fungal endophytes as plant bodyguards. New Phytol. 223, 2002–2010. doi: 10.1111/nph.15859
Ghalehgolabbehbahani, A., Sullivan, C. F., Davari, A., Parker, B. L., Razavi, A., and Skinner, M. (2022). Evaluation of the entomopathogenic fungus Metarhizium brunneum and the predatory mite Stratiolaelaps scimitus against Rhizoglyphus robini under laboratory conditions. Exp. Appl. Acarol. 87, 19–29. doi: 10.1007/s10493-022-00719-6
González-Mas, N., Gutiérrez-Sánchez, F., Sánchez-Ortiz, A., Grandi, L., Turlings, T. C. J., Manuel Muñoz-Redondo, J., et al. (2021). Endophytic colonization by the Entomopathogenic fungus Beauveria Bassiana affects plant volatile emissions in the presence or absence of chewing and sap-sucking insects. Front. Plant Sci. 12:660460. doi: 10.3389/fpls.2021.660460
Greenfield, M., Gómez-Jiménez, M. I., Ortiz, V., Vega, F. E., Kramer, M., and Parsa, S. (2016). Beauveria bassiana and Metarhizium anisopliae endophytically colonize cassava roots following soil drench inoculation. Biol. Control. 95, 40–48. doi: 10.1016/j.biocontrol.2016.01.002
Grijalba Erika, P., Carlos, E., Paola, E. C., Martha, L. C., and Laura, F. V. (2018). Metarhizium rileyi biopesticide to control Spodoptera frugiperda: stability and insecticidal activity under glasshouse conditions. Fungal Biol. 122, 1069–1076. doi: 10.1016/j.funbio.2018.08.010
Gupta, R., Keppanan, R., Leibman-Markus, M., Rav-David, D., Elad, Y., Ment, D., et al. (2022). The Entomopathogenic fungi Metarhizium brunneum and Beauveria bassiana promote systemic immunity and confer resistance to a broad range of pests and pathogens in tomato. Phytopathology 112, 784–793. doi: 10.1094/PHYTO-08-21-0343-R
Hatmaker, E. A., Rangel-Grimaldo, M., Raja, H. A., Pourhadi, H., Knowles, S. L., Fuller, K., et al. (2022). Genomic and phenotypic trait variation of the opportunistic human pathogen aspergillus flavus and its close relatives. Microbiol. Spectr. 10:e0306922. doi: 10.1128/spectrum.03069-22
Hong, S. B., Go, S. J., Shin, H. D., Frisvad, J. C., and Samson, R. A. (2005). Polyphasic taxonomy of aspergillus fumigatus and related species. Mycologia 97, 1316–1329. doi: 10.3852/mycologia.97.6.1316
Hu, S., and Bidochka, M. J. (2021). Root colonization by endophytic insect-pathogenic fungi. J. Appl. Microbiol. 130, 570–581. doi: 10.1111/jam.14503
Hussain, M., Komivi, S. A., Yongwen, L., Shiman, C., Wei, H., Jinguan, Z., et al. (2018). Susceptibilities of Candidatus Liberibacter asiaticus-infected and noninfected Diaphorina citri to entomopathogenic fungi and their detoxification enzyme activities under different temperatures. Microbiologyopen 7:e00607. doi: 10.1002/mbo3.607
Im, Y., So-Eun, P., Sue, Y. L., Jong-Cheol, K., and Jae, S. K. (2022). Early-stage defense mechanism of the cotton aphid Aphis gossypii against infection with the insect-killing fungus Beauveria bassiana JEF-544. Front. Immunol. 13:907088. doi: 10.3389/fimmu.2022.907088
Islam, W., Muhammad, A., Asad, S., Hassan, N., Yakubu, S. A., Muhammad, Q., et al. (2021). Insect-fungal-interactions: a detailed review on entomopathogenic fungi pathogenicity to combat insect pests. Microb. Pathog. 159:105122. doi: 10.1016/j.micpath.2021.105122
Jaber, L. R. (2018). Seed inoculation with endophytic fungal entomopathogens promotes plant growth and reduces crown and root rot (CRR) caused by fusarium culmorum in wheat. Planta 248, 1525–1535. doi: 10.1007/s00425-018-2991-x
Jiang, W., Peng, Y., Ye, J., Wen, Y., Liu, G., and Xie, J. (2019). Effects of the Entomopathogenic fungus Metarhizium anisopliae on the mortality and immune response of Locusta migratoria. Insects 11:36. doi: 10.3390/insects11010036
Karthi, S., Vaideki, K., Muthugounder, S. S., Athirstam, P., Annamalai, T., Muthiah, C., et al. (2018). Effect of aspergillus flavus on the mortality and activity of antioxidant enzymes of Spodoptera litura fab. (Lepidoptera: Noctuidae) larvae. Pestic Biochem Phys 149, 54–60. doi: 10.1016/j.pestbp.2018.05.009
Khan, I. H., and Javaid, A. (2022). Antagonistic activity of aspergillus versicolor against Macrophomina phaseolina. Braz. J. Microbiol. 53, 1613–1621. doi: 10.1007/s42770-022-00782-6
Kim Jae, S., Yeon, H. J., Margaret, S., and Bruce, L. P. (2013). An oil-based formulation of Isaria fumosorosea blastospores for management of greenhouse whitefly Trialeurodes vaporariorum (Homoptera: Aleyrodidae). Pest Manag. Sci. 69, 576–581. doi: 10.1002/ps.3497
Li, T., Zhou, J., and Li, J. (2023). Combined effects of temperature and humidity on the interaction between tomato and Botrytis cinerea revealed by integration of histological characteristics and transcriptome sequencing. Hortic. Res. 10:257. doi: 10.1093/hr/uhac257
Lin, W. J., Chiu, M. C., Lin, C. C., Chung, Y. K., and Chou, J. Y. (2021). Efficacy of entomopathogenic fungus aspergillus nomius against Dolichoderus thoracicus. BioControl 66, 463–473. doi: 10.1007/s10526-021-10086-7
Liu, Z., Liu, F. F., Li, H., Zhang, W. T., Wang, Q., Zhang, B. X., et al. (2022). Virulence of the bio-control fungus Purpureocillium lilacinum against Myzus persicae (Hemiptera: Aphididae) and Spodoptera frugiperda (Lepidoptera: Noctuidae). J. Econ. Entomol. 115, 462–473. doi: 10.1093/jee/toab270
Liu, C., Shen, H., Yi, L., Shao, P., Soulika, A. M., Meng, X., et al. (2015). Oral administration of aflatoxin G₁ induces chronic alveolar inflammation associated with lung tumorigenesis. Toxicol. Lett. 232, 547–556. doi: 10.1016/j.toxlet.2014.11.002
Malinga Lawrence, N., and Mark, D. L. (2021). Efficacy of three biopesticides against cotton pests under field conditions in South Africa. Crop Prot. 145:105578. doi: 10.1016/j.cropro.2021.105578
Masoudi, A., Koprowski, J. L., Bhattarai, U. R., and Wang, D. (2018). Elevational distribution and morphological attributes of the entomopathogenic fungi from forests of the Qinling Mountains in China. Appl. Microbiol. Biotechnol. 102, 1483–1499. doi: 10.1007/s00253-017-8651-4
Mora Margy, A. E., Alzimiro, M. C. C., and Marcelo, E. F. (2018). Classification and infection mechanism of entomopathogenic fungi. Arq. Inst. Biol. 97, 2797–2804. doi: 10.1007/s00253-013-4771-7
Mseddi, J., Ben, F. D., and Azzouz, H. (2022). Selection and characterization of thermotolerant Beauveria bassiana isolates and with insecticidal activity against the cotton-melon aphid Aphis gossypii (glover) (Hemiptera: Aphididae). Pest Manag. Sci. 78, 2183–2195. doi: 10.1002/ps.6844
Naeem, A., Shoaib, F., and Muhammad, A. (2020). Biochemical analysis and pathogenicity of entomopathogenic fungi to Diaphorina citri Kuwayama (Hemiptera: Liviidae). Entomol. Res. 50, 245–254. doi: 10.1111/1748-5967.12434
Nzabanita, C., Zhang, L., Zhao, H., Wang, Y., Wang, Y., Sun, M., et al. (2022). Fungal endophyte Epicoccum nigrum 38L1 inhibits in vitro and in vivo the pathogenic fungus fusarium graminearum. Biol. Control 174:105010. doi: 10.1016/j.biocontrol.2022.105010
Ownley, B. H., Griffin, M. R., Klingeman, W. E., Gwinn, K. D., Moulton, J. K., Pereira, R. M., et al. (2008). Beauveria bassiana: endophytic colonization and plant disease control. J. Invertebr. Pathol. 98, 267–270. doi: 10.1016/j.jip.2008.01.010
Qin, X., Zhao, X., Huang, S., Deng, J., Li, X., Luo, Z., et al. (2021). Pest management via endophytic colonization of tobacco seedlings by the insect fungal pathogen Beauveria bassiana. Pest Manag. Sci. 77, 2007–2018. doi: 10.1002/ps.6229,2007,2018
Raad, M., Glare, T. R., Brochero, H., Müller, C., and Rostás, M. (2019). Transcriptional reprogramming of Arabidopsis thaliana defence pathways by the entomopathogen Beauveria bassiana correlates with resistance against a fungal pathogen but not against insects. Front. Microbiol. 10:1481. doi: 10.3389/fmicb.2019.01481
Reis, T. A., Tralamazza, S. M., Coelho, E., Zorzete, P., Fávaro, D. I. T., and Corrêa, B. (2022). Early expression of the aflatoxin gene cluster in Aspergillus nomiae isolated from Brazil nut. Toxicon 209, 36–42. doi: 10.1016/j.toxicon.2022.01.008
Russo, M. L., Scorsetti, A. C., Vianna, M. F., Cabello, M., Ferreri, N., and Pelizza, S. (2019). Endophytic effects of Beauveria bassiana on corn (Zea mays) and its herbivore, Rachiplusia nu (Lepidoptera: Noctuidae). Insects 10:110. doi: 10.3390/insects10040110
Sinno, M., Ranesi, M., Di, L. I., Iacomino, G., Becchimanzi, A., Barra, E., et al. (2021). Selection of endophytic Beauveria bassiana as a dual biocontrol agent of tomato pathogens and pests. Pathogens 10:1242. doi: 10.3390/pathogens10101242
Sui, L., Hui, Z., Wenjing, X., Qinfeng, G., Ling, W., Zhengkun, Z., et al. (2020). Elevated air temperature shifts the interactions between plants and endophytic fungal entomopathogens in an agroecosystem. Fungal Ecol. 47:100940. doi: 10.1016/j.funeco.2020.100940
Sui, L., Lu, Y., Zhu, H., Wan, T., Li, Q., and Zhang, Z. (2022). Endophytic blastospores of Beauveria bassiana provide high resistance against plant disease caused by Botrytis cinerea. Fungal Biol. 126, 528–533. doi: 10.1016/j.funbio.2022.05.007
Sun, Y., Liu, J., Li, L., Gong, C., Wang, S., Yang, F., et al. (2018). New butenolide derivatives from the marine sponge-derived fungus aspergillus terreus. Bioorg. Med. Chem. Lett. 28, 315–318. doi: 10.1016/j.bmcl.2017.12.049
Thompson, J. D., Gibson, T. J., and Higgins, D. G. (2003). Multiple sequence alignment using ClustalW and ClustalX. Curr. Protoc. Bioinformatics Chapter 2:Unit 2.3. doi: 10.1002/0471250953.bi0203s00
Tomilova, O. G., Shaldyaeva, E. M., Kryukova, N. A., Pilipova, Y. V., Schmidt, N. S., Danilov, V. P., et al. (2020). Entomopathogenic fungi decrease Rhizoctonia disease in potato in field conditions. PeerJ 8:e9895. doi: 10.7717/peerj.9895
Vega, F. E. (2018). The use of fungal entomopathogens as endophytes in biological control: a review. Mycologia 110, 4–30. doi: 10.1080/00275514.2017.1418578
Vega, F. E., Goettel, M. S., Blackwell, M., Chandler, D., Jackson, M. A., Keller, S., et al. (2009). Fungal entomopathogens: new insights on their ecology. Fungal Ecol. 2, 149–159. doi: 10.1016/j.funeco.2009.05.001
Wang, L., Wang, J., Zhang, X., Yin, Y., Li, R., Lin, Y., et al. (2021). Pathogenicity of Metarhizium rileyi against Spodoptera litura larvae: Appressorium differentiation, proliferation in hemolymph, immune interaction, and reemergence of mycelium. Fungal Genet. Biol. 150:103508. doi: 10.1016/j.fgb.2020.103508
Watanabe, M., Lee, K., Goto, K., Kumagai, S., Sugita-Konishi, Y., and Hara-Kudo, Y. (2010). Rapid and effective DNA extraction method with bead grinding for a large amount of fungal DNA. J. Food Protect. 73, 1077–1084. doi: 10.4315/0362-028x-73.6.1077
Wichadakul, D., Kobmoo, N., Ingsriswang, S., Tangphatsornruang, S., Chantasingh, D., Luangsa-ard, J. J., et al. (2015). Insights from the genome of Ophiocordyceps polyrhachis-furcata to pathogenicity and host specificity in insect fungi. BMC Genomics 16:881. doi: 10.1186/s12864-015-2101-4
Wu, C. J., Cui, X., Xiong, B., Yang, M. S., Zhang, Y. X., and Liu, X. M. (2019). Terretonin D1, a new meroterpenoid from marine-derived aspergillus terreus ML-44. Nat. Prod. Res. 33, 2262–2265. doi: 10.1080/14786419.2018.1493583
Yan, J., Liu, H., Idrees, A., Chen, F., Lu, H., Ouyang, G., et al. (2022). First record of aspergillus fijiensis as an entomopathogenic fungus against Asian Citrus psyllid, Diaphorina citri Kuwayama (Hemiptera: Liviidae). J. Fungi 8:1222. doi: 10.3390/jof8111222
Zhang, Z. K., Lu, Y., Xu, W. J., Sui, L., Du, Q., Wang, Y., et al. (2020). Influence of genetic diversity of seventeen Beauveria bassiana isolates from different hosts on virulence by comparative genomics. BMC Genomics 21:451. doi: 10.1186/s12864-020-06791-9
Zhang, P., Yinwei, Y., Yuan, S., Youzhi, W., and Long, Z. (2015). First record of aspergillus oryzae (Eurotiales: Trichocomaceae) as an entomopathogenic fungus of the locust, Locusta migratoria (Orthoptera: Acrididae). Biocontrol Sci. Tech. 25, 1285–1298. doi: 10.1080/09583157.2015.1049977
Zhou, Y. B., Li, D. M., Houbraken, J., Sun, T. T., and de Hoog, G. S. (2020). Fatal rhinofacial mycosis due to Aspergillus nomiae: case report and review of published literature. Front. Microbiol. 11:595375. doi: 10.3389/fmicb.2020.595375
Keywords: entomophagous fungus, Aspergillus nomiae , endogenous colonization, plant disease resistance, biological control
Citation: Zhang Z, Tian Y, Sui L, Lu Y, Cheng K, Zhao Y, Li Q and Shi W (2024) First record of Aspergillus nomiae as a broad-spectrum entomopathogenic fungus that provides resistance against phytopathogens and insect pests by colonization of plants. Front. Microbiol. 14:1284276. doi: 10.3389/fmicb.2023.1284276
Received: 28 August 2023; Accepted: 13 December 2023;
Published: 08 January 2024.
Edited by:
Mohamed Hijri, Montreal University, CanadaReviewed by:
Elsherbiny A. Elsherbiny, Mansoura University, EgyptCopyright © 2024 Zhang, Tian, Sui, Lu, Cheng, Zhao, Li and Shi. This is an open-access article distributed under the terms of the Creative Commons Attribution License (CC BY). The use, distribution or reproduction in other forums is permitted, provided the original author(s) and the copyright owner(s) are credited and that the original publication in this journal is cited, in accordance with accepted academic practice. No use, distribution or reproduction is permitted which does not comply with these terms.
*Correspondence: Qiyun Li, cXlsaTEyMjVAMTI2LmNvbQ==; Wangpeng Shi, d3BzaGlAY2F1LmVkdS5jbg==
Disclaimer: All claims expressed in this article are solely those of the authors and do not necessarily represent those of their affiliated organizations, or those of the publisher, the editors and the reviewers. Any product that may be evaluated in this article or claim that may be made by its manufacturer is not guaranteed or endorsed by the publisher.
Research integrity at Frontiers
Learn more about the work of our research integrity team to safeguard the quality of each article we publish.