- 1Department of Biochemistry, Ahmadu Bello University, Zaria, Nigeria
- 2Chemical and Biochemical Sciences-Green Processing Engineering, Mohammed VI Polytechnic University, Ben Guerir, Morocco
- 3African Center of Excellence for Neglected Tropical Diseases and Forensic Biotechnology, Ahmadu Bello University, Zaria, Nigeria
- 4Department of Clinical Laboratory Sciences, College of Applied Medical Sciences, Shaqra University, Alquwayiyah, Saudi Arabia
- 5AgroBioSciences Program, College for Sustainable Agriculture and Environmental Science, Mohammed VI Polytechnic University, Ben Guerir, Morocco
- 6Department of Biochemistry, University of Johannesburgs, Johannesburg, South Africa
Background: African animal trypanosomiasis hinders sustainable livestock productivity in sub-Saharan Africa. About 17 million infected cattle are treated with trypanocides annually but most of the drugs are associated with drawbacks, necessitating the search for a promising chemotherapeutic agent.
Objectives: In this study, the effects of β-sitosterol on Trypanosoma congolense infection were investigated along with its effect on the trans-sialidase gene expressions.
Results: Oral treatment with β-sitosterol at 15 and 30 mg/kg body weight (BW) for 14 days significantly (p < 0.05) reduced parasitemia and ameliorated the parasite-induced anemia. Also, the parasite-induced increase in serum urea level and renal histopathological damage scores in addition to renal hypertrophy was significantly (p < 0.05) reverted following treatment with 30 mg/kg BW β-sitosterol. The compound also significantly (p < 0.05) down-regulated the expression of TconTS1 but not TconTS2, TconTS3, and TconTS4. Correlation analysis between free serum sialic acid with the TconTS1 and TconTS2 gene variants revealed negative correlations in the β-sitosterol-treated groups although they were non-significant (p > 0.05) in the group treated with 15 mg/kg BW β-sitosterol. Similarly, a non-significant negative (p > 0.05) correlation between the biomolecule and the TconTS3 and TconTS4 gene variants was observed in the β-sitosterol-treated groups while positive correlations were observed in the infected untreated control group.
Conclusion: The observed effect of β-sitosterol on T. congolense infection could make the compound a possible template for the design of novel trypanocides.
1. Introduction
As the world intensifies its effort toward searching for an ultimate therapy against diseases such as COVID-19 infection (Weiner et al., 2020), African nations are mainly looking inward to tackle the menace of tropical parasitic diseases such as African Trypanosomiasis (Garchitorena et al., 2017). These diseases not only affect human or animal health but also affect the economic well-being of the continent (Sun and Amon, 2018). One of the most important tropical diseases is African Animal Trypanosomiasis (AAT) caused by parasitic protozoan species including Trypanosoma congolense (T. congolense), Trypanosoma vivax (T. vivax), and Trypanosoma brucei brucei (T. brucei brucei) that are spread across the 37 sub-Saharan African countries where they affect livestock production (Habeeb et al., 2021).
Among the species mentioned, T. congolense has been considered the second most virulent and the most pathogenic species in cattle leading to AAT (Katabazi et al., 2020). After infection, the parasite develops extracellularly and interacts with circulating erythrocytes and vital organs of the infected host thereby causing profound damage at the adhesion sites. Importantly, the interaction imposes systemic oxidative stress that ultimately leads to hemolytic anemia and degenerative changes in the organs (Ibrahim et al., 2016). In fact, the above disease manifestations have been considered as factors resulting in the ultimate death of the infected host whilst anemia has been considered the major and most prominent symptom of the disease (Ibrahim et al., 2016; Saad et al., 2020).
Although multiple pathophysiologic mechanisms have been proposed to be responsible for anemia generation, the involvement of (trans)-sialidase has been reported to be the major etiologic agent (Coustou et al., 2012; Mbaya et al., 2012; Balogun et al., 2014). The T. congolense trans-sialidase (TconTS) has been implicated in the hydrolysis and transfer of host sialic acid for sialylation of the parasite surface molecules (Haynes et al., 2015). The transfer process is essential to the parasite as a survival strategy to escape the host defense mechanism since the sialic acid is abundantly found in the parasite Variant Surface Glycoprotein (VSG) coats responsible for antigenic variation (Quintana et al., 2018). Historically, within the African trypanosomes, trans-sialidases were initially found in procyclic T. brucei brucei with 38% similarities to T. cruzi trans-sialidase with most of the amino acid residues in the active sites conserved (Montagna et al., 2002). Two variants of the enzyme were found in procyclic T. congolense with conserved amino acid residues in the active site (Tiralongo et al., 2003; Montagna et al., 2006). However, further investigation revealed the presence of trans-sialidases in the bloodstream form of T. congolense (Coustou et al., 2012) where four active variants were recognized namely; TconTS1, TconTS2, TconTS3, and TconTS4 (Amaya et al., 2004; Gbem et al., 2013). Distinctively, TconTS1 and TconTS2 have a high trans-sialidase activity ratio while the TconTS3 and TconTS4 exclusively perform sialidase activity. The utilization of these variants to either cleave and/or transfer sialic acid depends on the parasite’s needs at a particular instant. On the other hand, the de-sialylation of the host RBCs leads to erythrophagocytosis and subsequently anemia (Balogun et al., 2014).
With the tremendous negative threats of AAT, exploiting strategies to control the disease is imminent (Samdi et al., 2010). As antigenic variation poses a great challenge in the production of vaccines (Onyilagha and Uzonna, 2019), the available options for disease control primarily centered on vector control or the use of trypanocides (Gimonneau et al., 2018). Since vector control was expensive on a large scale with little success, chemotherapy, therefore, serves as the gold standard approach to manage the disease (Eghianruwa and Oridupa, 2018). Currently, most of the drugs against the disease are limited due to many factors in addition to low therapeutic indices, resistance, and toxicity (Meyer et al., 2016; Assefa and Shibeshi, 2018). For instance, diminazene aceturate as one of the drugs used to treat trypanosomiasis was reported to induce adverse effects on the central nervous system and was associated with several signs of toxicity in the blood and systemic organs, as observed with changes in hematological and biochemical parameters. High doses of the drug eventually lead to mortality (da Silva Oliveira and da Silva, 2022). Moreover, clinical signs such as depression, ataxia, and seizures are associated with diminazene toxicity (Han et al., 2014). Hence, there is a need to develop lead compounds or structural scaffolds that could aid in the discovery of chemotherapeutic agents that could hinder the disease progression.
The propensity of information has confirmed that African medicinal plants possess bioactive compounds with high in vitro antitrypanosomal efficacy (Ibrahim et al., 2014). Specifically, some of the compounds were proven to have a positive effect against T. congolense-induced pathologies even though complete elimination of the parasite from the bloodstream of infected animals was not achieved (Aminu et al., 2017a,b, 2022; Saad et al., 2019, 2020). Consequently, it would be scientifically rewarding to further explore the African antitrypanosomal compounds.β-sitosterol is a phytosterol with a chemical structure similar to cholesterol (Figure 1), but can only be synthesized by plants (Bouic et al., 1999). Several pharmacological activities of the compound such as antioxidant, anticancer, and anti-inflammatory among others have been documented (Paniagua-Pérez et al., 2005). More importantly, the anti-leishmanial efficacy of the compound was reported in addition to in vitro antitrypanosomal activity against T. brucei brucei (Hoet et al., 2007). Although the mechanism of action of β-sitosterol in parasitic infection is not fully understood, the compound has been proposed to potentially disrupt the integrity of the parasite’s lipid bilayer, inhibit some enzyme activities, modulate host immune response, alteration of sterol metabolism and induction of apoptosis in some parasites (Pramanik et al., 2020; David et al., 2021; Kasirzadeh et al., 2021; Elawad et al., 2023; Shokry et al., 2023). Interestingly, our recent investigations employing in vitro kinetic analysis and molecular dynamic simulation studies proved the efficacy of the compound against T. congolense sialidase and phospholipase A2 (Aminu et al., 2023). Considering the wide activity spectrum of the compound and in a bid to further investigate the possible therapeutic effect of β-sitosterol on the trypanosomal infection, we performed an in vivo study to investigate the effect of β-sitosterol on T. congolense-induced pathological damages and the effect of the compound on the expression pattern of the parasite trans-sialidase gene variants. The information generated can improve the current strategies employed in searching for newer chemotherapeutic agents against Nagana.
2. Materials and methods
2.1. Chemicals and reagents
β-sitosterol, fetuin, and N-acetylneuraminic acid were purchased from Sigma Chemical Company (Saint Louis, MO 63103, United States). Assay kits for serum biomarkers (alanine aminotransferases (ALT), aspartate aminotransferases (AST), creatinine, and urea) were purchased from LABKIT (Chemelex, S.A., Barcelona) while RNeasyprotects animal blood and Maxima H minus Reverse Transcriptase (RT) enzyme kits were purchased from Qiagen V. N Company, Hilden, Germany, and Thermo Fisher Scientific Company, United States, respectively. Sodium periodate and thiobarbituric acid (TBA) were obtained from KEM light laboratories PVT Ltd. India. The standard drug, diminazene aceturate (D.A) used in the study was produced by HebelKexing Pharmaceutical Company Limited, China but purchased from a local veterinary clinic.
2.2. Source of trypanosome and experimental animals
The savannah strain of T. congolense used in this study was obtained from the stabilates at the National Institute for Trypanosomiasis and Onchocerciasis Research (NITOR), Kaduna-Nigeria. The parasite was validated using PCR with species-specific ITS-1 primers yielding a band size of 640 base pairs (bp). It was allowed to grow to a peak parasitemia (in donor rats) of about 109 parasite/mL of blood. On the other hand, forty-two (42) apparently healthy Wistar rats (150–200 g) were purchased from the Department of Pharmacology and Therapeutics, Ahmadu Bello University, Zaria, and were acclimatized for 1 week before the commencement of the studies. The animals were housed in plastic cages and provided with commercial rat chow (ECWA Feeds, Jos, Nigeria) and water ad libitum (maintained at room temperature of 25°Cwith 12 h light and dark cycle). The animal’s handling was according to the guidelines of Good Laboratory Practice (GLP) regulations of the World Health Organization while the study was conducted and reported according to the ARRIVE guidelines.1 Ethical approval with the number ABUCAUC/2020/44 was obtained from the Ahmadu Bello University ethical committee before initiating the studies.
2.3. Evaluation of the in vivo antitrypanosomal efficacy of β-sitosterol
After becoming accustomed to the environment, the animals were randomly grouped into six groups containing seven rats each as follows; Normal Control (NC), Infected Control (IC) animals, Infected treated with an intraperitoneal injection of 3.5 mg/kg BW diminazene aceturate (ITDA), Infected but orally treated with 15 (IT15BS) and 30 mg/kg BW (IT30BS) β-sitosterol as well as an uninfected group but orally treated with 30 mg/kg BW (UT30BS) of the β-sitosterol. For the parasite inoculation, the blood from the infected donor rat was collected and diluted with cold physiological saline to make up for the required inoculum of 104 parasite/mL. About 0.4 mL/100 g of the inoculum was used to infect the experimental animals and the establishment of parasites in their bloodstream was monitored. The appearance of the parasite on the 4th-day post-infection (pi)initiated the daily treatment with respective dosages of β-sitosterol and diminazene aceturate which proceeded for 14 days. In the infected animals, daily parasitemia was monitored using the rapid matching counting method (Herbert and Lumsden, 1976; Aminu et al., 2022) while the pre-and post-infection packed cell volumes (PCV) of the experimental animals were determined using the micro-hematocrit method.
2.4. Blood and organ sample collection
At the end of the experiment, the animals’ weights were ascertained and then, were humanely sacrificed under mild anesthesia. Blood samples were collected in both plain and EDTA containers via cardiac puncture. Serum was collected from the blood contained in the plain bottles by centrifuging at 3000 × g for 15 min and was stored at 2°C before further analysis. Immediately, RNALater was added to the EDTA-containing blood (1:4) and was stored at -20°C to preserve the total RNA in the samples whereas the liver, and kidney of animals were washed with normal saline, blotted with filter paper, and then weighed in order to determine their relative organ weight using the formula;
For histopathology, the organs were stored in 10% formalin and stored at 25 ± 2°C prior to analysis.
2.5. Determination of biochemical parameters and free serum sialic acid
The serum activities of aspartate and alanine aminotransferase (AST and ALT) in addition to serum urea and creatinine were determined using LABKIT reagents according to the manufacturer’s protocol. Likewise, free serum sialic acid was determined according to the thiobarbituric acid (TBA) method (Aminoff, 1961; Aminu et al., 2017a), where 100 μL of 25 mM periodate solution was added to serum (100 μL), mixed, and allowed to stand at 37°C for 30 min before the addition of 200 μL of sodium arsenate (2%). The mixture was capped and mixed thoroughly. Thereafter, 2 mL of 0.1 M TBA was added to the mixture and heated at 80°C for 8 min. The mixture was cooled for 5 min before the addition of a 2.5 mL acid-butanol reagent. The absorbance of the butanol layer was measured at 549 nm after centrifuging at 3000 rpm for 5 min. The sialic acid concentration of the samples was determined from a sialic acid standard curve.
2.6. Histopathology of the kidney
The histopathology of the Kidney was conducted by differential staining using hematoxylin and eosin dyes as previously described (Aminu et al., 2022). Briefly, the kidney contained in 10% formalin was dehydrated, cleared, and infiltrated using graded ethanol, xylene, and molten paraffin wax. The kidney was cut into micro-sections and applied on slides which were later stained using hematoxylin and eosin. Thereafter, the slides were viewed by trained personnel who had no idea of the experimental procedure, and the level of kidney damage was quantitatively graded. Tissues without any damage (0%) or with slight damage (1–20%) were scored as <2. Tissues with mild damage (40%) were graded as 2 < 5, while those with moderate damage (60%) were graded as 5 < 25. Tissues with severe damage (> 60%) were graded as >25.
2.7. Isolation, purification and quantification of T. congolense RNA
Total RNA was isolated and purified using RNeasy protect animal blood kits (Eppendorf, Germany) following the manufacturer’s instructions. Briefly, blood was centrifuged and washed with water before suspending in re-suspension buffer and digested with proteinase K in binding buffer followed by the addition of molecular grade ethanol. The samples were centrifuged in RNeasy spin columns and DNases were added. Subsequently, the total RNA was washed with buffer to generate the pure RNA which was eluted with an elution buffer. The eluted RNA was chilled immediately on ice and quantified using a Nanodrop spectrophotometer and its purity was determined.
2.8. Synthesis of complementary DNA (cDNA)
Complementary DNA (cDNA) was synthesized using maxima H minus reverse transcriptase (Thermo Scientific, Hamburg, Germany) strictly following the manufacturer’s instructions as previously described (Aminu et al., 2022). For the synthesis, the kit components were initially thawed, mixed, centrifuged, and then chilled on ice before the addition of the template RNA (100 ng/mL). Thereafter, 1 μL of random hexamer primers (5′ – d (NNNNNN) –3′; N = G, A, T or C), 1 μL of dNTPs and nuclease-free water was added to make up for the required volume. The mixture was gently mixed, centrifuged, and incubated at 37°C for 2 min. Additionally, 5 μL of Maxima cDNA H minus Synthesis Master Mix (5X) was added to make the entire reaction volume of 19.5 μL, and the mixture was centrifuged and incubated at 25°C for 10 min. Further incubation at 50°C for 30 min was done while the reaction was stopped by heating at 85°C for 5 min. The generated cDNA was used in the Real Time Quantitative Polymerase Chain Reaction (RT-Q PCR).
2.9. Real-time quantitative PCR and absolute quantification of T. congolense trans-sialidase gene variants
The qPCR was performed in a 10 μL volume containing 0.2 ng cDNA, 1 μM of each primer, 0.1 μM of each FAM-labeled probe, and 2x Taqman Fast Universal PCR Master Mix (Applied Biosystems). The reaction was performed in a Step-One Plus Real-Time PCR System (Applied Biosystems, United States). The following cycling conditions were employed: 95°C for 30 s, 60°C for 45 s, and 72°C for 60 s. The experiment was done in triplicates. The primers and probe sequences (Chechet, 2015) used in the qPCR reaction are as follows: TconTS1 (Fwd 5′-CTGACGATGGAAAGTCATGG-3′; Rev. 5′-ATCATACGGTAGCCCTGTCC-3′; Probe FAM 5′-TCCGAGGCTGCCCTCACTG-3’ BHQ1), TconTS2 (Fwd 5′-GCCATAACTGTGGAGGGAGT-3′; Rev. 5′-AATCTGTCCAACAAGCCACA-3′; Probe FAM 5′-ATCGCGACCGAATGCGACTG-3′ BHQ1), TconTS3 (Fwd 5′-TTCATCAAGTCGCACTCACA-3′; Rev. 5′-GGATGCCCAACAAAGAAGTT-3′; Probe FAM 5′-CGCCCGCAACCTTCGTATCC-3′ BHQ1), TconTS4 (Fwd 5′-TCGCCGAAAGCAACTATATG-3′; Rev. 5′-AACCCGTCAGCAGCTCTTAT-3′; Probe FAM 5′-TTGTCCCTCAACCGGGAGGC-3′ BHQ1).
2.10. Statistical analysis
Data obtained were expressed as mean ± standard deviation and were analyzed using GraphPad Prism version 5. Except for PCV, the data generated were analyzed using one-way analysis of variance (ANOVA) followed by Dunnet (for parasitemia; IC served as a control) and/or Tukey’s-HSD multiple post hoc tests in order to test for significance. For the PCV, a paired-sample t-test was conducted where differences in pre and post-infection values were determined. In all cases, p values less than 0.05 were considered statistically different. To determine the relationship between the free serum sialic acid and the T. congolense trans-sialidase gene variants, a Pearson correlation was conducted and significance at p < 0.01 was considered.
3. Results
On day 4 p.i, T. congolense appeared in the infected groups indicating successful inoculation and hence, marked the immediate initiation of β-sitosterol treatment (Figure 2). Observably, a progressive increase in the parasite load was observed in the infected control (IC) group throughout the experimental period except on day 8 p.i where a fluctuation was recorded (Figure 2). A similar increase was observed in β-sitosterol treated groups with the infected but treated with 30 mg/kg BW (IT30BS) group exhibiting significant (p < 0.05) parasitemia between day 10–12 pi but it was significantly lowered by day 14 pi compared to the IC group. Although there was an increase in parasite load in the infected-treated with 15 mg/kg BW (IT15BS) group, the value was significantly (p < 0.05) lower than the IC group throughout the experimental period. Noticeably, the parasite completely disappeared in the ITDA group the same day treatment was initiated (Figure 2).
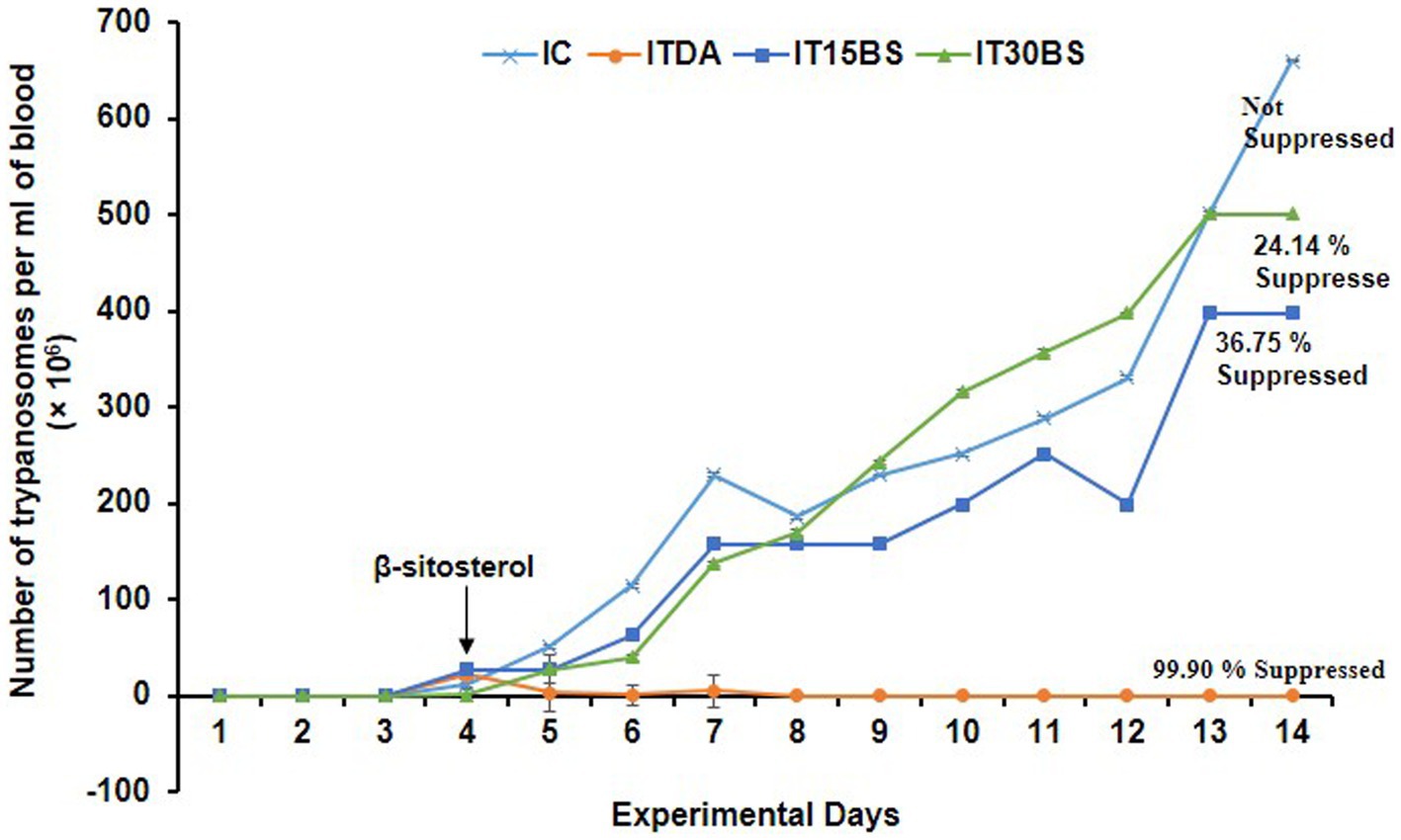
Figure 2. Therapeutic efficacy of oral administration of β-sitosterol on bloodstream T. congolense parasitemia. For parasitemia determination, the data are presented as mean ± standard deviation of seven rats. Dunnett posthoc test was used to analyze the data following one-way ANOVA. IC was used as a control. IC, infected control; IT15ΒS, infected +15 mg/kg BW β-sitosterol, and IT30BS, infected +30 mg/kg BW β-sitosterol; ITDA, infected +3.5 mg/kg BW diminazene aceturate.
In order to determine the therapeutic potential of β-sitosterol on anemia, the packed cell volume (PCV) of the animals was investigated. The final PCV in the IC group significantly (p < 0.05) dropped in relation to their baseline packed cell volume (PCV) value (Table 1). Treatment with β-sitosterol at both 15 and 30 mg/kg BW dosages significantly (p < 0.05) reversed the PCV value of the infected animals. Further analysis of the anemia-ameliorative effect of β-sitosterol showed a % change in PCV of −28.82 and − 33.69% in the IT15BS and IT30BS groups respectively, which were relatively lower than −76.55% observed in the IC group (Table 1).
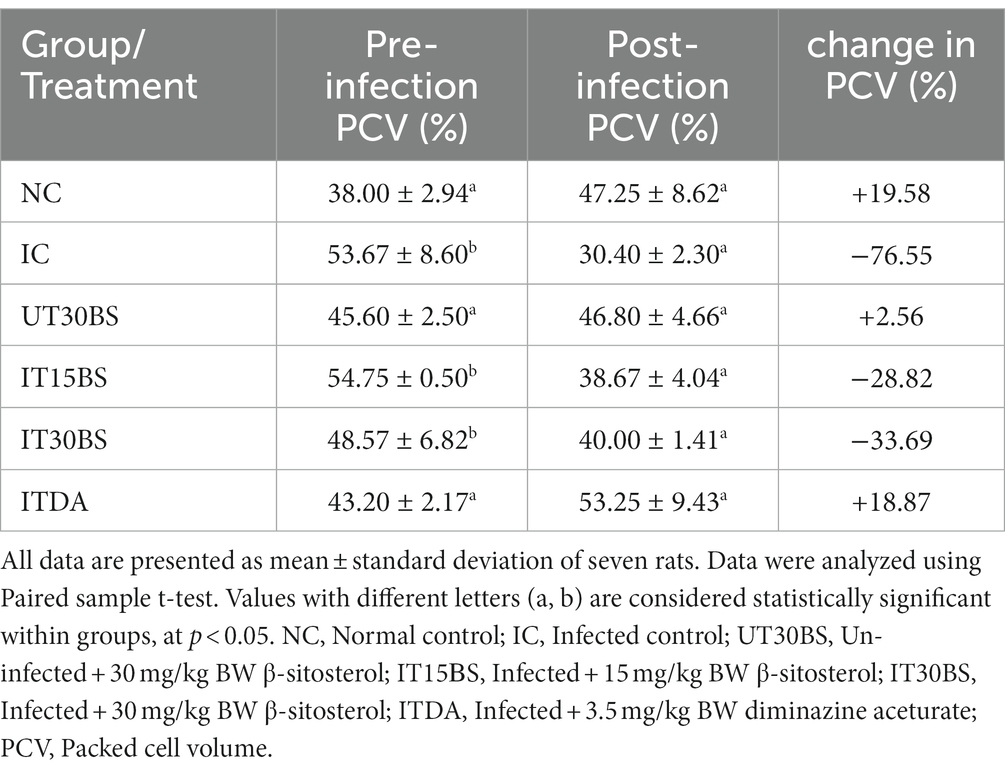
Table 1. Anemia-ameliorative effects of oral administration of β-sitosterol in T. congolense-infected animals.
Trypanosomal infection is accompanied by hepatic and renal damage, and this was prominent in the study. Infection with T. congolense in the IC group was accompanied by significant (p < 0.05) elevation in some biochemical indices of hepatic and renal damage compared with the normal control group (Table 2). The hepatic biomarkers particularly the AST were not affected by the treatment while the compound at both dosages significantly (p < 0.05) reduce the renal damage induced by the parasite in the infected animals (Table 2). Furthermore, investigating the relative hepatic and renal weights of the animals revealed a non-significant (p > 0.05) increase in relative liver weight in the IC group compared with the normal control group (Figure 3). Treatment with both dosages of the β-sitosterol showed a non-significant (p > 0.05) increase in the relative liver weight (Figure 3). With respect to the kidney, a significant (p < 0.05) increase in the weight of kidneys in IC animals was observed compared with the NC group (Figure 3). However, the kidney enlargement was reduced following β-sitosterol administration at both dosages, respectively, (Figure 3).
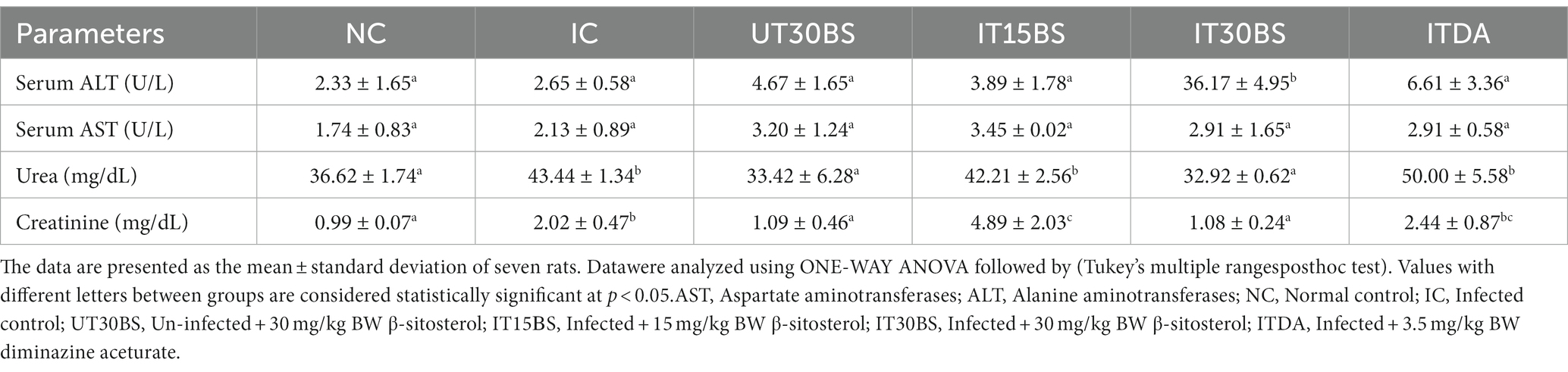
Table 2. Therapeutic efficacy of oral administration of β-sitosterol on biochemical parameters in T. congolense-infected animals.
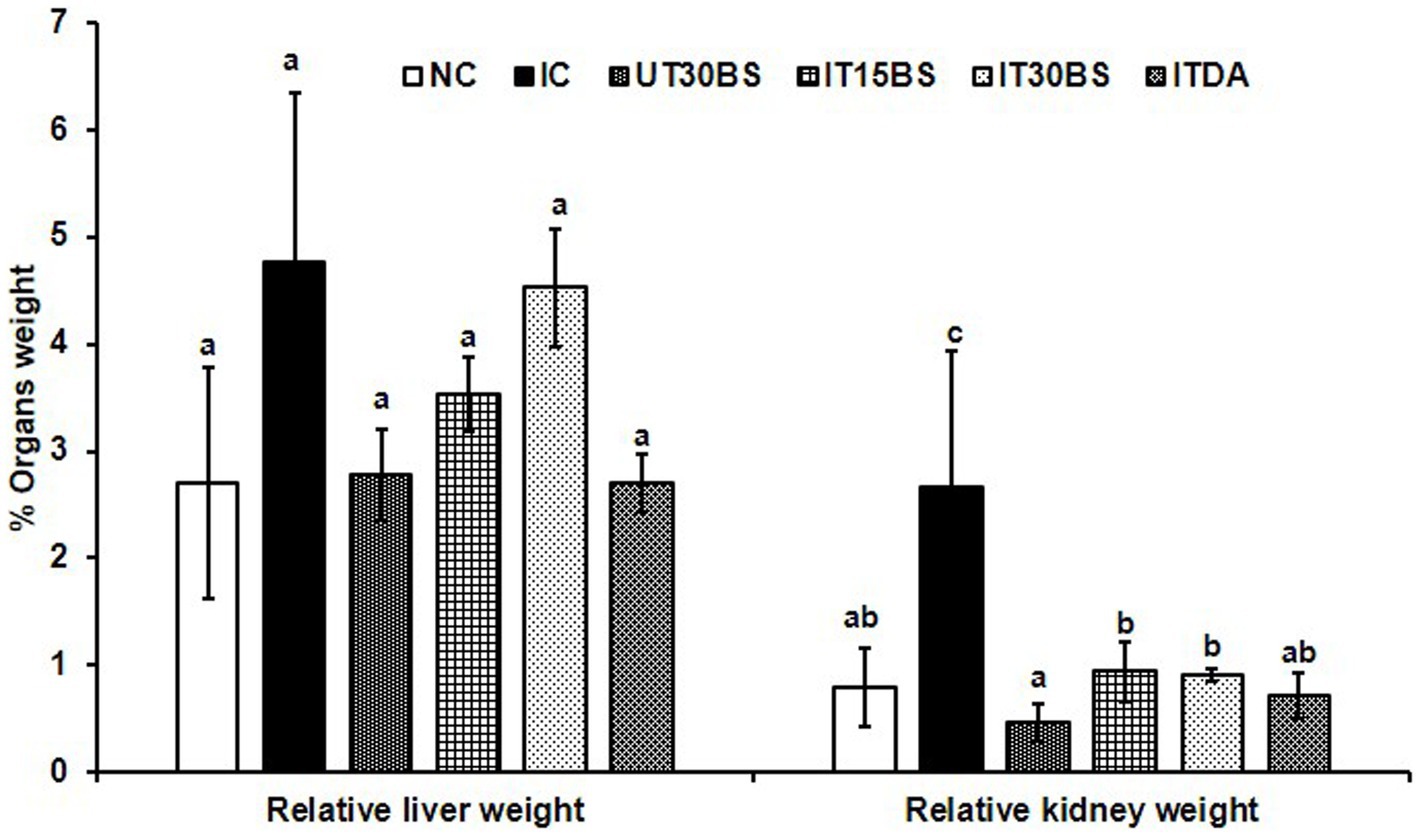
Figure 3. Therapeutic efficacy of oral administration of β-sitosterol on the relative liver and kidney weights in T. congolense-infected animals. Values with different letters between groups are considered statistically significant at p< 0.05. NC, Normal control; IC, infected control; UT30BS, un-infected +30 mg/kg BW β-sitosterol; IT15ΒS, infected +15 mg/kg BW β-sitosterol and IT30BS, infected +30 mg/kg BW β-sitosterol; ITDA, infected +3.5 mg/kg BW diminazene aceturate.
In order to corroborate the effect of the compound on the kidney, the histopathology of the kidney was conducted. Observably, glomerular necrosis (GN) and tubular necrosis (TN) were observed in the IC group (Figure 4). In the treatment and diminazene aceturate-treated groups, tubular necrosis (but not glomerular necrosis) was observed (Figure 4). Also, the quantitative investigation of the total damage revealed a significant (p < 0.05) total damage score in the IC group compared to the normal control group (Figure 5). Treatment with 15 mg/kg and 30 mg/kg BW β-sitosterol significantly (p < 0.05) reduced the kidney total damage score and hence could suggest the pathologic damages induced by the parasite in the kidney were ameliorated by the compound (Figure 5).
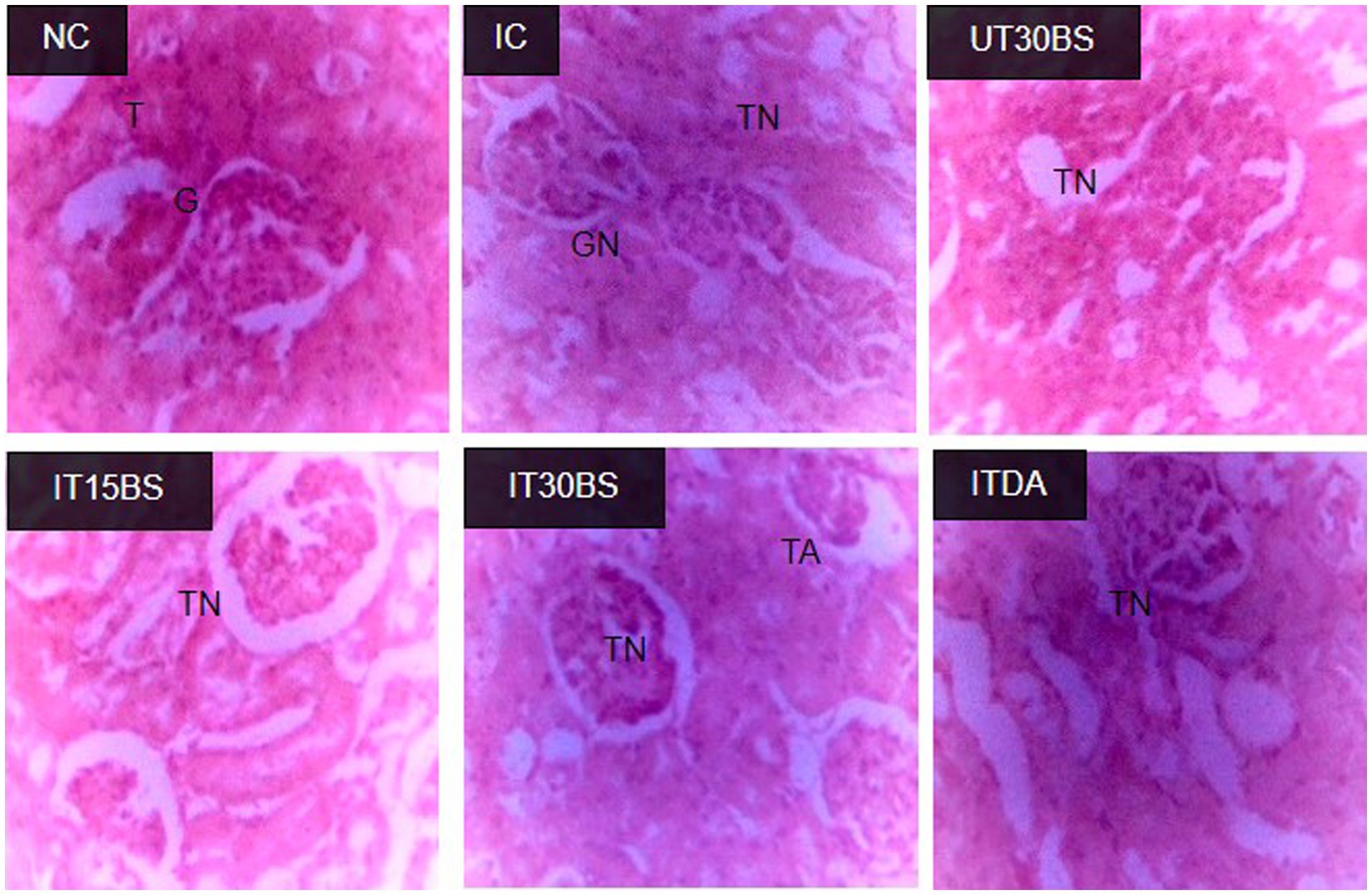
Figure 4. Effect of oral administration of β-sitosterol on the renal histopathological changes observed in T. congolense-infected animals. NC, Normal control; IC, infected control; UT30BS, un-infected +30 mg/kg BW β-sitosterol; IT15ΒS, infected +15 mg/kg BW β-sitosterol and IT30BS, infected +30 mg/kg BW β-sitosterol; ITDA, infected +3.5 mg/kg BW diminazene aceturate. T, Normal Tubules; G, Normal Glomerulus; GN, Glomerular Necrosis; TA, Tubular Adhesion; TN, Tubular Necrosis.
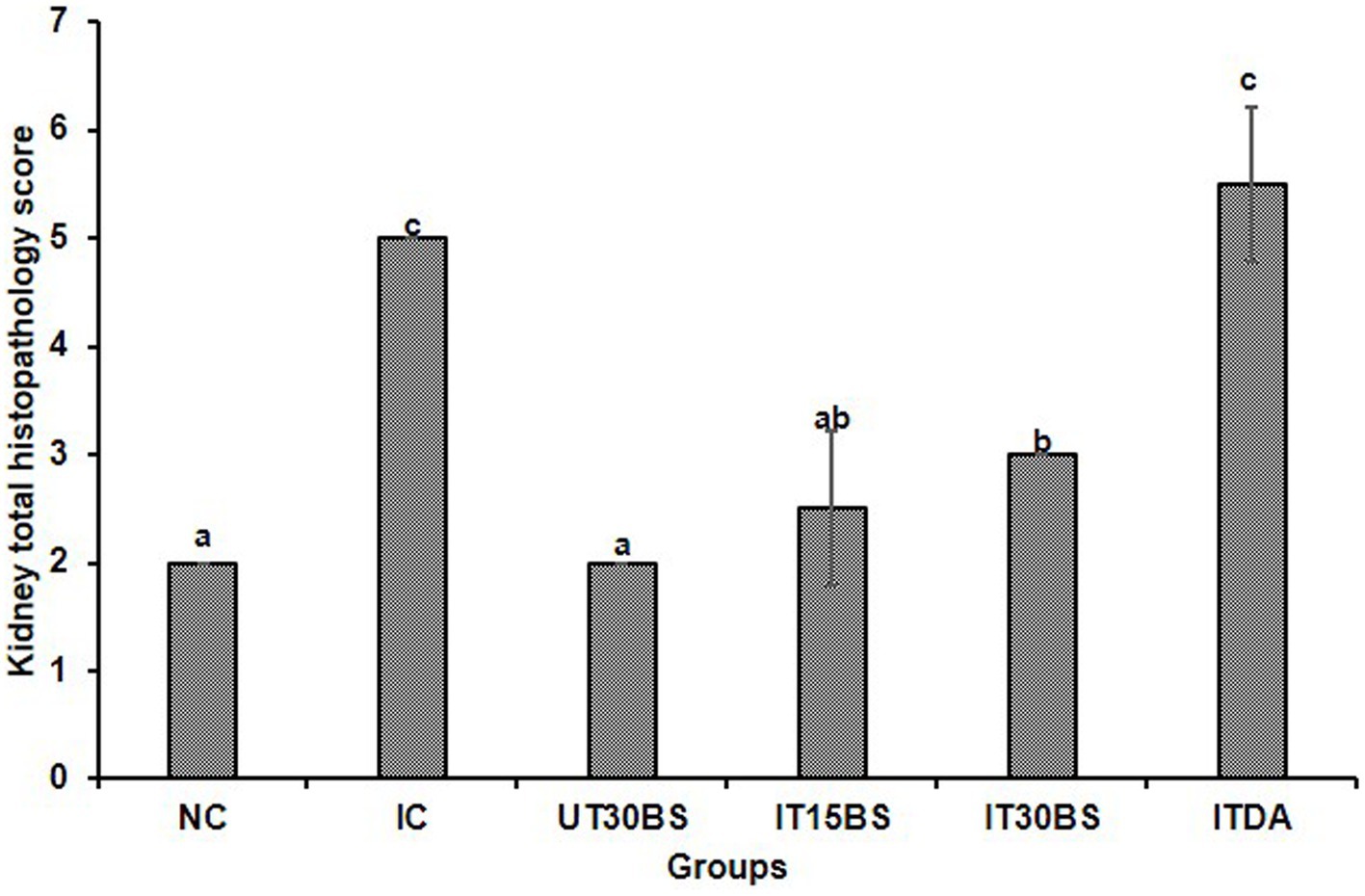
Figure 5. The overall quantitative kidney histopathological scores of T. congolense-infected animals following oral administration of β-sitosterol. The data are presented as the mean ± standard deviation of three rats. Data were analyzed using one-way ANOVA followed by (Tukey’s multiple ranges posthoc test). Values with different letters between groups are considered statistically significant at p < 0.05. NC, Normal control; IC, Infected control, UT30BS, Un-infected +30 mg/kg BW β-sitosterol; IT15ΒS, Infected +15 mg/kg BW β-sitosterol; IT30BS, Infected +30 mg/kg BW β-sitosterol; ITDA, Infected +3.5 mg/kg BW diminazine aceturate.
T. congolense infection is accompanied by the release of sialic acid and the parasite is known to scavenge the sialic acid using its enzyme machinery (particularly, the (trans)-sialidase enzyme). In this study, the amount of free serum sialic acid and the expression of T. congolense trans-sialidase gene variants were investigated in the infected animals (Figure 6A). A significant (p < 0.05) elevation in the free serum sialic acid was observed in the infected control (IC) group (Figure 6A). However, the level of the sialic acid was significantly reduced in animals treated with 30 mg/kg BW β-sitosterol (Figure 6A). With respect to the mRNA level of the trans-sialidase variants, the observed overexpression of TconTS1 in the IC group was significantly (p < 0.05) reduced upon treatment with 15 and 30 mg/kg BW β-sitosterol respectively, while the compound led to a significant (p < 0.05) increase in the expression of TconTS2 variant in the IT30BS group (Figure 6B). In the same vein, expressions of TconTS3 and TconTS4 in the IT15BS and IT30BS were significant (p < 0.05) increased compared with the IC group, indicating that the compound has no effect on the variants (Figure 6B). Pearson correlation between free serum sialic acid with the TconTS1 and TconTS2 gene variants showed strong negative correlations (p < 0.05) in the 30 mg/kg BW β-sitosterol groups (Table 3). Similarly, a negative correlation (p > 0.05) was observed between free serum sialic acid with the TconTS3 and TconTS4, respectively (Table 3). Although the correlations were also negative between the biomolecule and the TconTS gene variants in the 15 mg/kg BW β-sitosterol treated groups, the values were non-significant (p > 0.05) (Table 3). In the IC group, a strong negative correlation (p < 0.05) was observed between free serum sialic acid with TconTS1 and positive correlations were observed between free serum sialic acid with the other gene variants (Table 3). In contrast, positive correlations (p < 0.05) were observed between free serum sialic acids and TconTS1, TconTS3, and TconTS4 gene variants in the ITDA group, although the correlation was negative with respect to TconTS2 (Table 3).
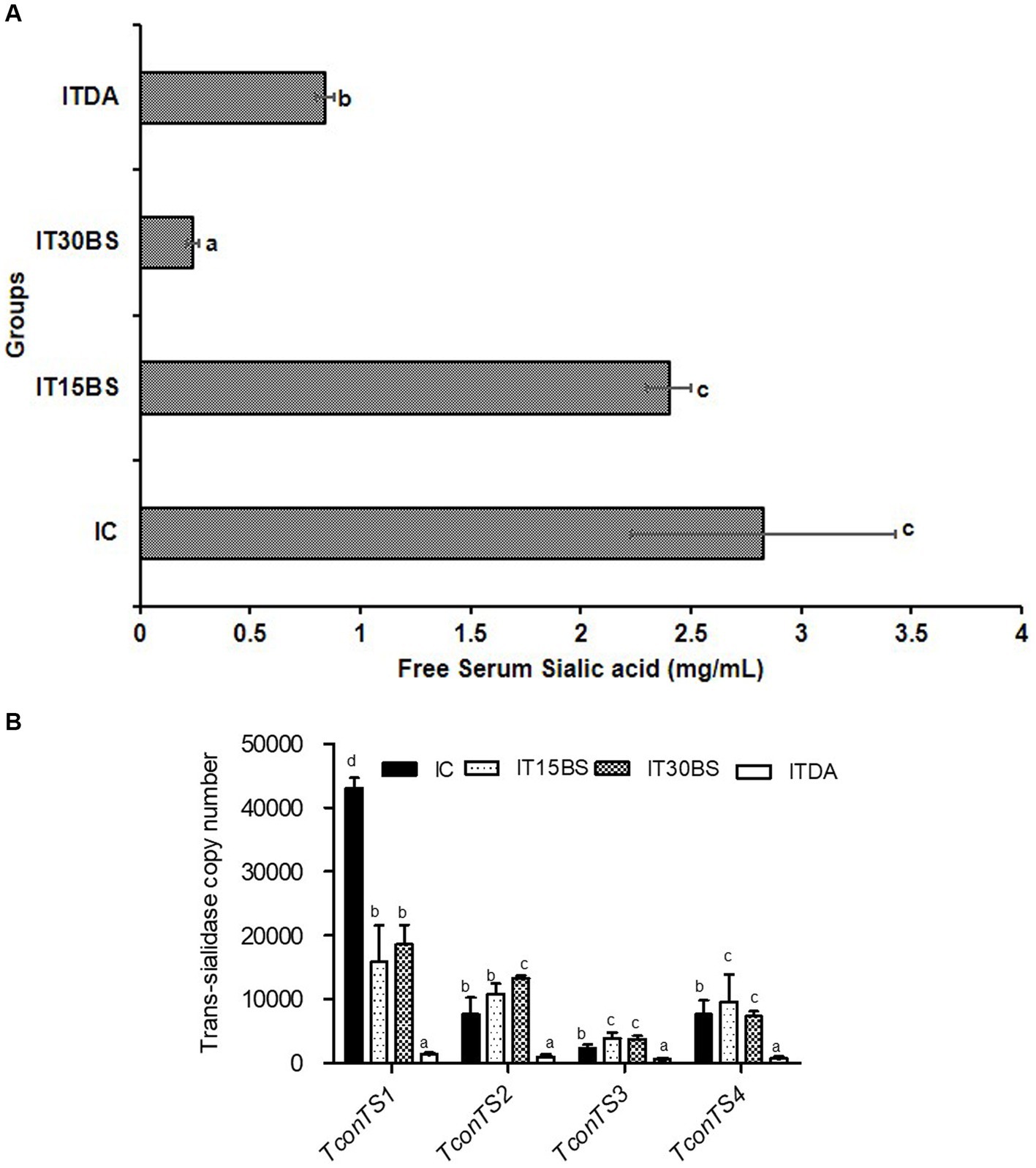
Figure 6. The free serum sialic acid in T. congolense-infected animals (A) and mRNA expression profile of bloodstream T. congolense trans-sialidase gene variants following oral administration of β-sitosterol to T. congolenseinfected animals (B). The data are presented as the mean ± standard deviation of seven rats. Data were analyzed using one-way ANOVA followed by (Tukey’s multiple ranges posthoc test). Values with different letters between groups are considered statistically significant at p < 0.05. NC, Normal control; IC, Infected control; UT30BS, Un-infected +30 mg/kg BW β-sitosterol; IT15ΒS, Infected +15 mg/kg BW β-sitosterol; IT30BS, Infected +30 mg/kg BW β-sitosterol; ITDA, Infected +3.5 mg/kg BW diminazine aceturate.
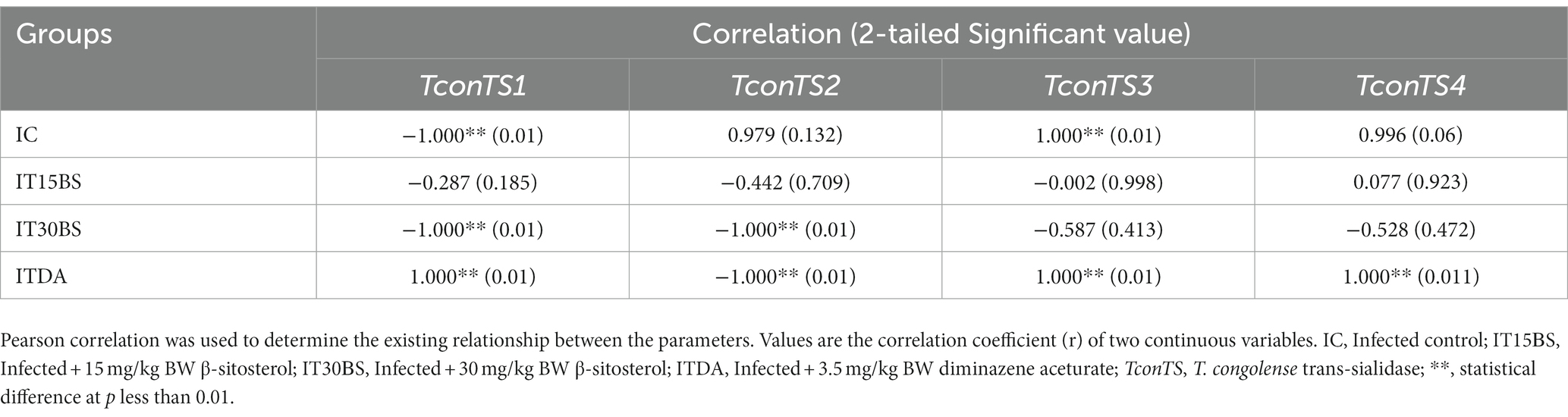
Table 3. Relationship between free serum sialic acid with T. congolense trans-sialidase gene variants in T. congolense-infected animals treated with β-sitosterol.
4. Discussion
Even with the renewed efforts by the scientific communities to control AAT, the disease has continued to pose a great constraint to livestock production in sub-Saharan Africa (Meyer et al., 2016; Habeeb et al., 2021) mainly because the available trypanocides are faced with major drawbacks (Assefa and Shibeshi, 2018). In light of this, the present study demonstrated the therapeutic benefits of β-sitosterol on T. congolense proliferation, anemia amelioration, kidney damage, and the TconTS1 gene expression.
The sustained increase in T. congolense in the IC group throughout the experimental period is a known phenomenon in trypanosomiasis and seemed to be responsible for the major pathologies observed in the infected host (Mugnier et al., 2015). Although treatment with 15 mg/kg BW β-sitosterol manifested a positive effect immediately, the 30 mg/kg BW β-sitosterol becomes effective mainly at the last 2 days of the study. In both cases, the compound appeared to be trypanostatic with parasite suppression below 40%. The low efficacy observed could be related to the high IC50 of the compound recorded against T. brucei brucei (Hoet et al., 2007). The inability of the compound to clear parasites could be due to their low bioavailability as only a small amount of administered phytosterols are known to be absorbed and reach the systemic circulation and their concentration is usually 200 times lower than the concentration of cholesterol under normal nutrition (Ostlund, 2007; Ras et al., 2013). Conversely, β-sitosterol was known to exhibit anthelmintic and anti-leishmanial activities (Vijaya and Yadav, 2014; Majid-Shah et al., 2019), the latter disease type has a similar etiology to trypanosomiasis (Lawyer and Perkins, 2000).
Even with the low efficacy of β-sitosterol towards clearing the T. congolense, the parasite-induced anemia was reverted following treatment with the respective dosages. This could be a remarkable observation since anemia has been reported to be a major symptom associated with the disease (Balogun et al., 2014). Most often, death associated with trypanosomiasis was positively correlated with anemia development (Igbokwe, 2018). Although the treatment reversed anemia, the compound does not maintain the PCV values of the infected animals to their initial status. The inability of the compound to maintain the normal PCV levels of the infected animals could occur due to its inability to completely clear the parasite since a negative correlation between parasitemia and PCV is a known phenomenon during infection (Fidelis-Junior et al., 2016).
In addition to anemia, organ degeneration has been known as another devastating pathologic feature associated with trypanosomiasis (Umar et al., 2009). Observably, damages to the liver and kidney of the T. congolense-infected animals were prominent in our study since an increase in serum hepatic and renal biomarkers was recorded. Organ damage has been associated with oxidative stress imposed by the parasite (Ibrahim et al., 2016). Even with β-sitosterol treatment, the serum aminotransferases were unaffected while the serum urea and creatinine were ameliorated.
Although we have previously reported the anemia-ameliorating effect of β-sitosterol by targeting sialidase and phospholipase A2 (Aminu et al., 2023), the observed compound’s ability to prevent renal hypertrophy could also be considered as an additional factor in reducing anemia. This is because, the concept of renal hypertrophy has been reported as a factor contributing to anemia development during trypanosomiasis (Stijlemans et al., 2018). The 30 mg/kg BW of the compound showed more efficacy than the lower dosage (15 mg/kg BW). Previous administration of a relatively higher dosage of β-sitosterol (20 mg/kg BW) to rats induced with nephrotoxicity showed significant positive changes in renal biochemical and histopathological changes (Sharmila et al., 2016). This could means that, more positive effect might be observed by increasing the dosage. Hence, this could be an important finding as the ability of the compound to maintain renal integrity could be explored in designing promising drug agents.
In addition to the effect of the compound in ameliorating renal hypertrophy, the observed effect of 30 mg/kg BW β-sitosterol towards preventing the release of free serum sialic acid is also an important finding since the cleavage of the sialic acid by trans-sialidases has been implicated in anemia development (Guegan et al., 2013). Supposedly, compounds possessing trypanostatic and anemia-ameliorating potentials could as well, prevent the release of free sialic acid as observed with β-sitosterol (Ajakaiye et al., 2013). Considering the importance of the sialic acid to the parasite, it would be worthwhile to investigate and correlate the expression level of TconTS and the sialic acid in the infected animals (Coustou et al., 2012). To achieve this, absolute quantification of the variants was conducted. This allows an actual measurement of the absolute amount of the variants which was used to correlate with the amount of sialic acid in the animals. Of all the gene variants, it was only the expression of TconTS1 and not TconTS2, TconTS3, and TconTS4 that was reduced after treatment with β-sitosterol. The TconTS1 with TconTS2 were reported to have high trans-sialidase activity and hence, the effect of the compound on the TconTS1 could suggest decreased trans-sialylation of the parasite surface molecules (Gbem et al., 2013) while the increase in the expression of TconTS3 and TconTS4 could suggest enhanced sialic acid hydrolysis (Gbem et al., 2013; Balogun et al., 2014). However, to better understand the significance of these findings, it would be highly appealing to perform relative quantification. With regards to the study objectives, correlating the free serum sialic acid and the variants was performed and the observed positive correlation, especially in IC and ITDA groups, between free serum sialic acid with theTconTS3 and TconTS4 suggests reduced cleavage of sialic acid from host erythrocytes, decreased erythrophagocytosis, and improved survival (Coustou et al., 2012) while the observed negative correlations following β-sitosterol treatment may indicate that the decrease in the expression of TconTS1 gene variant could not be the most important contributor to the anemia-ameliorative effects of the compound.
5. Conclusion
In conclusion, it was observed that β-sitosterol possessed in vivo therapeutic efficacy on bloodstream T. congolense but could not completely clear the parasites from the blood of the infected rats. At the same time, the compound was able to ameliorate anemia and renal damage induced by the parasite as clearly supported by the histopathological investigation. Nonetheless, the compound was also reported to prevent the parasite-induced release of free serum sialic acid and reduce the expression of the TconTS1 gene variant. In order to elucidate more targets, the compound should be further studied by targeting other factors enhancing the progression of the disease. Also, there is a need for structural modification of the compound to improve its antitrypanosomal efficacy, particularly in clearing the parasite.
Data availability statement
The original contributions presented in the study are included in the article/supplementary material, further inquiries can be directed to the corresponding authors.
Ethics statement
The animal study was approved by Ahmadu Bello University ethical committee. The study was conducted in accordance with the local legislation and institutional requirements.
Author contributions
SA: Conceptualization, Formal analysis, Investigation, Methodology, Resources, Writing – original draft. GC: Investigation, Methodology, Resources, Writing – review & editing. SSA: Formal analysis, Funding acquisition, Investigation, Resources, Writing – review & editing. MS: Formal analysis, Funding acquisition, Investigation, Resources, Writing – review & editing. RD: Formal analysis, Funding acquisition, Investigation, Resources, Writing – review & editing. MBS: Formal analysis, Funding acquisition, Investigation, Resources, Writing – review & editing. EO: Formal analysis, Funding acquisition, Investigation, Methodology, Resources, Supervision, Validation, Writing – review & editing. MAI: Conceptualization, Formal analysis, Funding acquisition, Investigation, Methodology, Project administration, Resources, Supervision, Validation, Writing – review & editing.
Funding
The author(s) declare that no financial support was received for the research, authorship, and/or publication of this article.
Acknowledgments
We acknowledged the management of Ahmadu Bello University, Zaria, Nigeria for providing the facilities used in conducting the studies. The TconTS gene expression studies were conducted at the Center for Biomolecular Interactions Bremen (CBIB), Universität Bremen, Germany. MAI is a recipient of the National Research Foundation grant (NRF 2020/SETI/43) from TetFund, Nigeria.
Conflict of interest
The authors declare that the research was conducted in the absence of any commercial or financial relationships that could be construed as a potential conflict of interest.
The author(s) declared that they were an editorial board member of Frontiers, at the time of submission. This had no impact on the peer review process and the final decision.
Publisher’s note
All claims expressed in this article are solely those of the authors and do not necessarily represent those of their affiliated organizations, or those of the publisher, the editors and the reviewers. Any product that may be evaluated in this article, or claim that may be made by its manufacturer, is not guaranteed or endorsed by the publisher.
Footnotes
References
Ajakaiye, M. J. J., Benjamin, R. M., Bizi, M. S., Shuaibu, L. R., Kugu, Y., Mohammad, B. A., et al. (2013). Effects of dietary vitamins C and E oral administration on body temperature, body weight and haematological parameters in wistar rats infected with T. b. Brucei (Federe strain) during the hot rainy season. International research. J. Pharm. Pharmacol. 3, 105–111. doi: 10.14303/irjpp.2013.035
Amaya, M. F., Watts, A. G., Damager, I., Wehenkel, A., Nguyen, T., Buschiazzo, A., et al. (2004). Structural insights into the catalytic mechanism of Trypanosoma cruzi transsialidase. Structure 12, 775–784. doi: 10.1016/j.str.2004.02.036
Aminoff, D. (1961). Methods for the quantitative estimation of N-acetylneuraminic acid and their applications to hydrolysates of sialomucoids. Biochem. J. 81, 384–392. doi: 10.1042/bj0810384
Aminu, S., Danazumi, A. U., Alhafiz, Z. A., Gorna, M. W., and Ibrahim, M. A. (2023). β-Sitosterol could serve as a dual inhibitor of Trypanosoma congolense sialidase and phospholipase A2: in vitro kinetic analyses and molecular dynamic simulations. Mol. Divers. 27, 1645–1660. doi: 10.1007/s11030-022-10517-2
Aminu, S., Ibrahim, M. A., Dada Chechet, G., and Onyike, E. (2022). Chemotherapeutic potentials of β-ionone against Trypanosoma congolense infection: inhibition of parasite proliferation, anemia development, trans-sialidase (TconTS3 and TconTS4) gene expressions, and phospholipase A2. Chem. Biol. Drug Des. 99, 908–922. doi: 10.1111/cbdd.14048
Aminu, R., Ibrahim, M. A., Rahman, M. A., Dash, R., and Umar, I. A. (2017a). Trypano-suppresive effects of ellagic acid and amelioration of the trypanosome-associated pathological features coupled with inhibitory effects on trypanosomal sialidase in vitro and in silico. Phytomedicine 30, 67–73. doi: 10.1016/j.phymed.2017.04.013
Aminu, R., Umar, I. A., Rahman, M. A., and Ibrahim, M. A. (2017b). Stigmasterol retards the proliferation and pathological features of Trypanosoma congolense infection in rats and inhibits trypanosomal sialidase in vitro and in silico. Biomed. Pharmacother. 89, 482–489. doi: 10.1016/j.biopha.2017.02.068
Assefa, S., and Shibeshi, W. (2018). Drug resistance in African animal trypanosomes: a review. Afr. J. Microbiol. Res. 12, 380–386. doi: 10.5897/AJMR2017.8754
Balogun, E. O., Balogun, J. B., Yusuf, S., Inuwa, H. M., Ndams, I. S., Sheridan, P., et al. (2014). Anemia amelioration by lactose infusion during trypanosomosis could be associated with erythrocytes membrane de-galactosylation. Vet. Parasitol. 199, 259–263. doi: 10.1016/j.vetpar.2013.10.013
Bouic, P., Clark, A., Lamprecht, J., Freestone, M., Pool, E., Liebenberg, R., et al. (1999). The effects of B-sitosterol (BSS) and B-sitosterol glucoside (BSSG) mixture on selected immune parameters of marathon runners: inhibition of post marathon immune suppression and inflammation. Int. J. Sports Med. 20, 258–262. doi: 10.1055/s-2007-971127
Chechet, G. D. (2015). Expression pattern of trans-sialidase genes in the life cycle of trypanosoma congolense. Department of Biochemistry, Ahmadu Bello University, Zaria, Nigeria. Ph.D. Dissertation
Coustou, V., Plazolles, N., Guegan, F., and Baltz, T. (2012). Sialidases play a key role ininfection and anaemia in Trypanosoma congolense animal trypanosomiasis. Cell. Microbiol. 14, 431–445. doi: 10.1111/j.1462-5822.2011.01730.x
da Silva Oliveira, G. L., and da Silva, A. P. D. S. C. L. (2022). Evaluation of the non-clinical toxicity of an antiparasitic agent: diminazene aceturate. Drug Chem. Toxicol. 45, 2003–2013. doi: 10.1080/01480545.2021.1894741
David, O. M., Olanlokun, J. O., Owoniyi, B. E., Ayeni, M., Ebenezer, O., and Koorbanally, N. A. (2021). Studies on the mitochondrial, immunological and inflammatory effects of solvent fractions of Diospyros mespiliformis Hochst in plasmodium berghei-infected mice. Sci. Rep. 11:6941. doi: 10.1038/s41598-021-85790-6
Eghianruwa, K. I., and Oridupa, O. A. (2018). Chemotherapeutic control of trypanosomosis - a review of past chemotherapeutic control of trypanosomosis - a review of past measures, current status and future trends, current status and future trends. Veterinarskiarhiv 88, 245–270. doi: 10.24099/vet.arhiv.161115a
Elawad, M. A., Elkhalifa, M. E. M., Hamdoon, A. A. E., Salim, L. H. M., Ahmad, Z., and Ayaz, M. (2023). Natural products derived steroids as potential anti-leishmanial agents; disease prevalence, underlying mechanisms and future perspectives. Steroids 193:109196. doi: 10.1016/j.steroids.2023.109196
Fidelis-Junior, O. L., Sampaio, P. H., Machado, R. Z., André, M. R., Marques, L. C., and Cadioli, F. A. (2016). Evaluation of clinical signs, parasitemia, hematologic and biochemical changes in cattle experimentally infected with Trypanosoma vivax. Rev. Bras. Parasitol. Vet. 25, 69–81. doi: 10.1590/S1984-29612016013
Garchitorena, A., Sokolow, S., Roche, B., Ngonghala, C., Jocque, M., Lund, A., et al. (2017). Disease ecology, health and the environment: a framework to account for ecological and socio-economic drivers in the control of neglected tropical diseases. Philos. Trans. R. Soc. B: Biol. Sci. 372:20160128. doi: 10.1098/rstb.2016.0128
Gbem, T. T., Waespy, M., Hesse, B., Dietz, F., Smith, J., Chechet, G. D., et al. (2013). Biochemical diversity in the Trypanosoma congolense trans-sialidase family. PLoS Negl. Trop. Dis. 7:e2549. doi: 10.1371/journal.pntd.0002549
Gimonneau, G., Rayaisse, J., and Bouyer, J. (2018). “Integrated control of trypanosomosis” in Pests and vector-borne diseases in the livestock industry. eds. G. Claire, J. Bouyer, W. Takken, and R. C. Smallegange, vol. 5 (Wageningen: Wageningen Academic Publishers), 147–174.
Guegan, F., Plazolles, N., Baltz, T., and Coustou, V. (2013). Erythrophagocytosis of desialylated red blood cells is responsible for anaemia during Trypanosoma vivax infection. Cell. Microbiol. 15, 1285–1303. doi: 10.1111/cmi.12123
Habeeb, I. F., Chechet, G. D., and Kwaga, J. K. P. (2021). Molecular identification and prevalence of trypanosomes in cattle distributed within the Jebba axis of the river Niger, Kwara state, Nigeria. Parasites Vect. 14:560. doi: 10.1186/s13071-021-05054-0
Han, D., Yoon, W.-K., and Hyun, C. (2014). Cerebellar encephalopathy from diminazene aceturate (beneril) toxicity in a dog. Korean J. Vet. Res. 54, 193–196. doi: 10.14405/kjvr.2014.54.3.193
Haynes, C. L. F., Ameloot, P., Remaut, H., Callewaert, N., Sterckx, Y. G.-J., and Magez, S. (2015). Production, purification and crystallization of a trans-sialidase from Trypanosoma vivax. Acta Crystallogr. F:Struct. Biol. Commun. 71, 577–585. doi: 10.1107/S2053230X15002496
Herbert, W. J., and Lumsden, W. H. R. (1976). A rapid matching method for estimating the host’s parasitaemia. J. Exp. Parasitol. 40, 427–431. doi: 10.1016/0014-4894(76)90110-7
Hoet, S., Pieters, L., Muccioli, G. G., Habib-Jiwan, J.-L., Opperdoes, F. R., and Quetin-Leclercq, J. (2007). Antitrypanosomal activity of triterpenoids and sterols from the leaves of Strychnos spinosa and related compounds. J. Nat. Prod. 70, 1360–1363. doi: 10.1021/np070038q
Ibrahim, M. A., Isah, M. B., and Abdullahi, A. S. (2016). Antioxidant therapy against trypanosome infections. Curr. Top. Med. Chem. 16, 2233–2244. doi: 10.2174/1568026616666160413125622
Ibrahim, M. A., Mohammed, A., Isah, M. B., and Aliyu, A. B. (2014). Anti-trypanosomal activity of African medicinal plants: a review update. J. Ethnopharmacol. 154, 26–56. doi: 10.1016/j.jep.2014.04.012
Igbokwe, I. O. (2018). Evolving anti-disease strategies from biochemical pathogenesis of African trypanosomiasis. Adv. Cytol. Pathol. 3, 33–39. doi: 10.15406/acp.2018.03.00048
Kasirzadeh, S., Ghahremani, M. H., Setayesh, N., Jeivad, F., Shadboorestan, A., Taheri, A., et al. (2021). β-Sitosterol alters the inflammatory response in CLP rat model of sepsis by modulation of NFκB Signaling. Biomed Res. Int. 2021:5535562. doi: 10.1155/2021/5535562
Katabazi, A., Aliero, A. A., Witto, S. G., Odoki, M., and Musinguzi, S. P. (2020). Prevalence of Trypanosoma congolense and Trypanosoma vivax in Lira District, Uganda. Biomed Res. Int. 2021:7. doi: 10.1155/2021/7284042
Lawyer, P. G., and Perkins, P. V. (2000). “Leishmaniasis and trypanosomiasis” in Medical Entomology. eds. B. F. Eldridge and J. D. Edman (Dordrecht: Springer)
Majid-Shah, S., Ullah, F., Ayaz, M., Sadiq, A., Hussain, S., Ali Shah, A.-H., et al. (2019). β-Sitosterol from Iflogaspicata (Forssk.) as potential anti-leishmanial agent against leishmaniatropica: docking and molecular insights. Steroids 7:208. doi: 10.3390/pr7040208
Mbaya, A., Hussein Kumshe, H., and Nwosu, C. O. (2012). The mechanisms of anaemia in Trypanosomosis: A review, Anemia, Dr. Donald Silverberg (Ed.), ISBN: 978-953-51-0138-3, InTech. Available at: http://www.intechopen.com/books/anemia/the-mechanisms-of-anaemia-in-trypanosomosis-a-review
Meyer, A., Holt, H. R., Selby, R., and Guitian, J. (2016). Past and ongoing tsetse and animal trypanosomiasis control operations in five African countries: a systematic review. PLOS Negl. Trop. Dis. 10:e0005247. doi: 10.1371/journal.pntd.0005247
Montagna, G., Cremona, M. L., Paris, G., Amaya, M. F., Buschiazzo, A., Alzari, P. M., et al. (2002). The trans-sialidase from the African trypanosome Trypanosoma brucei. Eur. J. Biochem. 269, 2941–2950. doi: 10.1046/j.1432-1033.2002.02968.x
Montagna, G. N., Donelson, J. E., and Frasch, A. C. C. (2006). Procyclic Trypanosoma brucei expresses separate sialidase and trans-sialidase enzymes on its surface membrane. J. Biol. Chem. 281, 33949–33958. doi: 10.1074/jbc.M604951200
Mugnier, M. R., Cross, G. A. M., and Papavasiliou, F. N. (2015). The in vivo dynamics of antigenic variation in Trypanosoma brucei. Science 347, 1470–1473. doi: 10.1126/science.aaa4502
Onyilagha, C., and Uzonna, J. E. (2019). Host immune responses and immune evasion strategies in African trypanosomiasis. Front. Immunol. 10, 2738–2750. doi: 10.3389/fimmu.2019.02738
Ostlund, R. E. (2007). Phytosterol, cholesterol absorption, and healthy diets. Lipids 42, 41–45. doi: 10.1007/s11745-006-3001-9
Paniagua-Pérez, R., Madrigal-Bujaidar, E., Reyes-Cadena, S., Molina-Jasso, D., Gallaga, J. P., Silva-Miranda, A., et al. (2005). Genotoxic and cytotoxic studies of beta-sitosterol and pteropodine in mouse. J. Biomed. Biotechnol. 2005, 242–247. doi: 10.1155/JBB.2005.242
Pramanik, P. K., Chakraborti, S., Bagchi, A., and Chakraborti, T. (2020). Bioassay-based Corchoruscapsularis L. leaf-derived β-sitosterol exerts antileishmanial effects against Leishmaniadonovani by targeting trypanothionereductase. Sci. Rep. 10:20440. doi: 10.1038/s41598-020-77066-2
Quintana, J. F., Pino, R. C. D., Yamada, K., and Zhang, N. (2018). Adaptation and therapeutic exploitation of the plasma membrane of African trypanosomes. Genes 2, 368–384. doi: 10.3390/genes9070368
Ras, R. T., Hiemstra, H., Lin, Y., Vermeer, M. A., Duchateau, G. S., and Trautwein, E. A. (2013). Consumption of plant sterol-enriched foods and effects on plasma plant sterol concentrations--a meta-analysis of randomized controlled studies. Atherosclerosis 230, 336–346. doi: 10.1016/j.atherosclerosis08.012
Saad, S. B., Ibrahim, M. A., Jatau, I. D., and Shuaibu, M. N. (2019). Trypanostatic activity of geranylacetone: mitigation of Trypanosoma congolense-associated pathological pertubations and insight into the mechanism of anaemia amelioration using in vitro and in silico models. Exp. Parasitol. 201, 49–56. doi: 10.1016/j.exppara.2019.04.011
Saad, S. B., Ibrahim, M. A., Jatau, I. D., and Shuaibu, M. N. (2020). The therapeutic potential of phytol towards Trypanosoma congolense infection and the inhibitory effects against trypanosomal sialidase. Exp. Parasitol. 216, 107943–107951. doi: 10.1016/j.exppara.2020.107943
Samdi, S. M., Abenga, J. N., Attahir, A., Haruna, M. K., Wayo, B. M., Fajinmi, A. O., et al. (2010). Impact of trypanosomosis on food security in Nigeria: a review. J. Anim. Vet. Adv. 2, 47–50.
Sharmila, R., Sindhu, G., and Arockianathan, P. M. (2016). Nephroprotective effect of β-sitosterol on N-diethylnitrosamine initiated and ferric nitrilotriacetate promoted acute nephrotoxicity in Wistar rats. J. Basic Clin. Physiol. Pharmacol. 27, 473–482. doi: 10.1515/jbcpp-2015-0085
Shokry, S., Hegazy, A., Abbas, A. M., Mostafa, I., Eissa, I. H., Metwaly, A. M., et al. (2023). Phytoestrogen β-sitosterol exhibits potent in vitro antiviral activity against influenza a viruses. Vaccine 11:228. doi: 10.3390/vaccines11020228
Stijlemans, B., De Baetselier, P., Magez, S., Van Ginderachter, J. A., and De Trez, C. (2018). African Trypanosomiasis-Associated Anemia: The Contribution of the Interplay between Parasites and the Mononuclear Phagocyte System. Frontiers in immunology 9, 218. doi: 10.3389/fimmu.2018.00218
Sun, N., and Amon, J. J. (2018). Addressing inequity: neglected tropical diseases and human rights. Health Hum. Rights 20, 11–25.
Tiralongo, E., Martensen, I., Grötzinger, J., Tiralongo, J., and Schauer, R. (2003). Trans-sialidase-like sequences from Trypanosoma congolense conserve most of the critical active site residues found in other trans-sialidases. Biol. Chem. 384, 1203–1213. doi: 10.1515/BC.2003.133
Umar, I. A., Maryoms, N. G., Daikwo, E., Gidado, A., Buratai, L. B., Igbokwe, I. O., et al. (2009). The effects of consumption of Hibiscus sabdariffa calyces on heamatological profile and organ pathological changes in Trypanosoma congolense infected rats. Afr. J. Tradit. Complement. Altern. Med. 6, 585–591.
Vijaya,, and Yadav, A. K. (2014). In vitro anthelmintic assessment of selected phytochemicals against Hymenolepisdiminuta, a zoonotic tapeworm. J. Parasit. Dis. 40, 1082–1086. doi: 10.1007/s12639-014-0560-1
Keywords: anemia, β-sitosterol, organ damage, renal hypertrophy, Trypanosoma congolense, trans-sialidase
Citation: Aminu S, Chechet GD, Alkhalil SS, Sobeh M, Daoud R, Simelane MB, Onyike E and Ibrahim MA (2023) Therapeutic efficacy of β-sitosterol treatment on Trypanosoma congolense infection, anemia development, and trans-sialidase (TconTS1) gene expression. Front. Microbiol. 14:1282257. doi: 10.3389/fmicb.2023.1282257
Edited by:
Hosny El-Adawy, Friedrich Loeffler Institut, GermanyReviewed by:
Sarah Abdo, Kafrelsheikh University, EgyptAman Ullah Khan, University of Veterinary and Animal Sciences, Pakistan
Copyright © 2023 Aminu, Chechet, Alkhalil, Sobeh, Daoud, Simelane, Onyike and Ibrahim. This is an open-access article distributed under the terms of the Creative Commons Attribution License (CC BY). The use, distribution or reproduction in other forums is permitted, provided the original author(s) and the copyright owner(s) are credited and that the original publication in this journal is cited, in accordance with accepted academic practice. No use, distribution or reproduction is permitted which does not comply with these terms.
*Correspondence: Samia S. Alkhalil, salkhalil@su.edu.sa; Mohammed Auwal Ibrahim, mauwalibrahim@gmail.com; maibrahim@abu.edu.ng