- 1Mycotic and Parasitic Agents and Mycobacteria, Robert Koch Institute, Berlin, Germany
- 2ECDC Fellowship Programme, Public Health Microbiology Path (EUPHEM), European Centre for Disease Prevention and Control (ECDC), Stockholm, Sweden
- 3Centre for Biological Threats and Special Pathogens, Highly Pathogenic Microorganisms (ZBS 2), Robert Koch Institute, Berlin, Germany
Global warming has caused an increase in the emergence of Vibrio species in marine and estuarine environments as well as fresh water bodies. Over the past decades, antimicrobial resistance (AMR) has evolved among Vibrio species toward various antibiotics commonly used for the treatment of Vibrio infections. In this study, we assessed virulence and resistance patterns of Vibrio cholerae non-O1/non-O139 strains derived from Germany and other European countries. A total of 63 clinical and 24 environmental Vibrio cholerae non-O1/non-O139 strains, collected between 2011 and 2021, were analyzed. In silico antibiotic resistances were compared with resistance phenotypes according to EUCAST breakpoints. Additionally, genetic relatedness between isolates was assessed by two cgMLST schemes (SeqSphere +, pubMLST). Both cgMLST schemes yielded similar results, indicating high genetic diversity among V. cholerae non-O1/non-O139 isolates. Some isolates were found to be genetically closely related (allelic distance < 20), which suggests an epidemiological link. Thirty-seven virulence genes (VGs) were identified among 87 V. cholerae non-O1/non-O139 isolates, which resulted in 38 virulence profiles (VPs). VPs were similar between clinical and environmental isolates, with the exception of one clinical isolate that displayed a higher abundance of VGs. Also, a cluster of 11 environmental isolates was identified to have the lowest number of VGs. Among all strains, the predominant virulence factors were quorum sensing protein (luxS), repeats-in-toxins (rtxC/rtxD), hemolysin (hlyA) and different type VI secretion systems (T6SS) genes. The genotypic profiles revealed antibiotic resistance genes (ARGs) associated with resistance to beta-lactams, quinolones, macrolides, tetracycline, antifolate, aminoglycosides, fosfomycin, phenicols and sulfonamide. Carbapenemase gene VCC-1 was detected in 10 meropenem-resistant V. cholerae non-O1/non-O139 isolates derived from surface water in Germany. The proportion of resistance among V. cholerae non-O1/non-O139 species isolates against first line treatment (3rd generation cephalosporin, tetracycline and fluoroquinolone) was low. Empirical treatment would likely have been effective for all of the clinical V. cholerae non-O1/non-O139 isolates examined. Nevertheless, carbapenem-resistant isolates have been present in fresh water in Germany and might represent a reservoir for ARGs. Monitoring antimicrobial resistance is crucial for public health authorities to minimize the risks for the human population.
1 Introduction
Vibrio species are aquatic gram-negative bacteria that are found worldwide in fresh and coastal marine waters with moderate salinity. Vibrio cholerae serogroup O1 and O139 strains are well known to be the causative agent of endemic and epidemic cholera. Cholera is occasionally diagnosed in Europe as a travel-associated infection, but not endemic. Pathogenic Vibrio cholerae O1/O139 strains encode two major virulence factors: enterotoxin (cholera toxin CT; product of the ctxA, ctxB genes) and the toxin coregulated pilus (colonization factor TCP: tcpA), both of which enable bacterial cells to adhere and colonize the small intestine. The expression of both virulence factors causes severe diarrhea with the risk of dehydration. The major virulence genes are present in clusters and are encoded on mobile genetic elements integrated into the chromosomes of the toxigenic strains. The CTX genes are coded by part of the genome of a filamentous bacteriophage (CTXΦ), while TCP genes are located within a pathogenicity island (VPI).
There are several species of non-cholera Vibrio, often called “non-toxigenic” due to the lack of CT and TCP virulence genes. These include V. cholerae strains not previously mentioned, and other Vibrio species. However, other virulence factors, previously found in the toxigenic strains, like type III and type VI secretion systems (T3SS, T6SS), mannose-sensitive hemagglutinin pilus (mshA), thermostable hemolysin (hlyA), outer membrane proteins (ompU), cholix toxin (chxA) and repeats-in-toxin (RTX) clusters (Dziejman et al., 2005; Chatterjee et al., 2009; Arteaga et al., 2020) are known to contribute to their pathogenicity. The occurrence of those virulence genes varies between the strains. The clinical manifestation of non-toxigenic Vibrio species includes self-limited acute gastrointestinal infections after consumption of raw or undercooked seafood, wound and soft tissue infections, ear infections, and rarely, septicemia. The majority of infections occur in patients with underlying illnesses (e.g., liver disease, chronic renal failure, diabetes mellitus, and thalassemia major), but also healthy individuals are affected. While almost 80 Vibrio species have been identified, currently 12 of them are potential human pathogens (Baker-Austin et al., 2018). Globally, four Vibrio species (V. cholerae, V. vulnificus, V. alginolyticus, and V. parahaemolyticus) are dominant in human infections (Baker-Austin et al., 2017).
In Europe, infections by non-cholera Vibrio species are rare, however, a rapidly warming marine environment seems to be an important driver for the emergence and proliferation of Vibrio species in the coastal water of the Baltic Sea and North Sea, as Vibrio species multiply particularly strongly in warm water above 20°C (Kirschner et al., 2008; Baker-Austin et al., 2017; Marinello et al., 2017; Brehm et al., 2021). The effects of global warming, in particular an increase in the sea surface temperature, have so far been greater in the Baltic Sea than other coastal seas (Belkin, 2009; Markus Meier et al., 2021). A warming trend in the sea surface temperature contributes to the spread of Vibrio species in marine environments and will lead to an increase in the number of Vibrio infections in vulnerable populations (Vezzulli et al., 2016). However, an increase of Vibrio species abundance is not limited to marine and coastal ecosystems only; inland fresh water and natural and artificial lakes can also be affected (Kirschner et al., 2008; Kokashvili et al., 2015; Rehm et al., 2023).
In recent decades, resistance has evolved in several Vibrio species toward various antibiotics commonly used for the treatment of infections, such as ampicillin, chloramphenicol, tetracycline, streptomycin, kanamycin, trimethoprim, and carbapenems (Bier et al., 2015). Non-toxigenic Vibrio species may act as an environmental reservoir for antibiotic resistance genes (ARGs) which can be acquired through different mechanisms. Chromosomal mutations may contribute to antimicrobial resistance, but acquisition of mobile genetic elements (e.g., plasmids, integrons from closely related strains or other bacterial species) is a key mechanism in multidrug-resistant (MDR) Vibrio species (Verma et al., 2019). Acquisition of the gene cassette in Class 1 integrons through horizonal gene transfer has been previously reported in V. cholerae isolates derived from stool and rectal swabs in South Africa (Dalsgaard et al., 2001). Comparative genomic analyses of MDR and extensively drug-resistant V. cholerae strains isolated from diarrheal samples in India showed that resistance genes are linked to mobile genetic elements and can be easily transferred to other bacterial species (Verma et al., 2019). Another study performed by Borgeaud et al. (2015) on V. cholerae O1 El Tor strains revealed that the virulence genes associated with the type VI secretion system, which is a part of the competence regulation, are involved in DNA uptake by bacterial competitors leading to acquisition of antibiotic resistance or virulence genes. Vibrio species are naturally present in aquatic environments and can contribute to ARG transfer to humans either through direct contact or via food chains. Therefore, in the context of “One Health,” systematically monitoring the aquatic environment and enhancing the surveillance to identify Vibrio species and their antimicrobial resistance is desirable in order to minimize the risk for the human population.
Core genome multilocus sequence typing (cgMLST) is widely used to assess genetic relatedness among bacterial isolates to support outbreak investigation (Pearce et al., 2018) or tracing back transmission chains (Frentrup et al., 2020). The usability of this tool has been reported in comparative genome analysis of Vibrio parahaemolyticus in China (Pang et al., 2020) and North America (Xu et al., 2017). Recently, two cgMLST schemes for Vibrio cholerae have been developed (Liang et al., 2020; Chen et al., 2022) to facilitate the global epidemiology of cholera disease.
Vibrio infections are not always reported across Europe and elsewhere, as they are not classified as notifiable pathogenic agents. This lack of epidemiological data is problematic in the assessment of the overall clinical impact of these infections. In Germany, Vibrio species other than toxigenic V. cholerae strains were not classified as notifiable pathogens before March 2020 (Brehm et al., 2021). Currently, Vibrio infections are reported electronically by clinical laboratories to the Robert Koch Institute (RKI) in Germany. We conducted a study to describe virulence and resistance patterns of Vibrio cholerae non-O1/non-O139 strains isolated in Germany and other European countries. We also disclosed the genetic relatedness between clinical and environmental Vibrio cholerae non-O1/non-O139 isolates using two core genome MLST schemes.
2 Materials and methods
2.1 Study design and strain collection
We conducted a retrospective study on 87 V. cholerae non-O1/non-O139 isolates from clinical (n = 63) and environmental (n = 24) samples, collected from 2011 until 2021. Although non-cholera Vibrio infections were not notifiable in Germany before March 2020, clinical or environmental isolates of Vibrio cholerae and other Vibrio species could be sent to the Department of Highly Pathogenic Microorganisms (ZBS 2) at the Center for Biological Threats and Special Pathogens of the RKI or to the Federal Institute for risk assessment (BfR) in Germany to confirm the diagnosis and for subtyping. This was voluntarily done by several hospitals and diagnostic laboratories in Germany. Therefore, the RKI holds an extensive bacterial strain collection of Vibrio species, which is available for further analyses. We included only V. cholerae non-O1/non-O139 isolates for which it was certain that an infection had occurred within Germany or other European countries in this study. We excluded toxigenic V. cholerae O1/O139. In total, we examined 63 clinical strains, which had resulted in various manifestations of the disease, including gastroenteritis, wound infections, ear infections, or septicemia. We also included 24 environmental isolates derived from various water sources across Germany, different fish species, and an isolate from a dog with diarrhea. Details of the strain characteristics are given in Table 1 and Supplementary Table 1. For each isolate, information about the source and location of isolation (city) was collected, and when known, data on clinical manifestation and region of potential infection (e.g., travel abroad) were added.
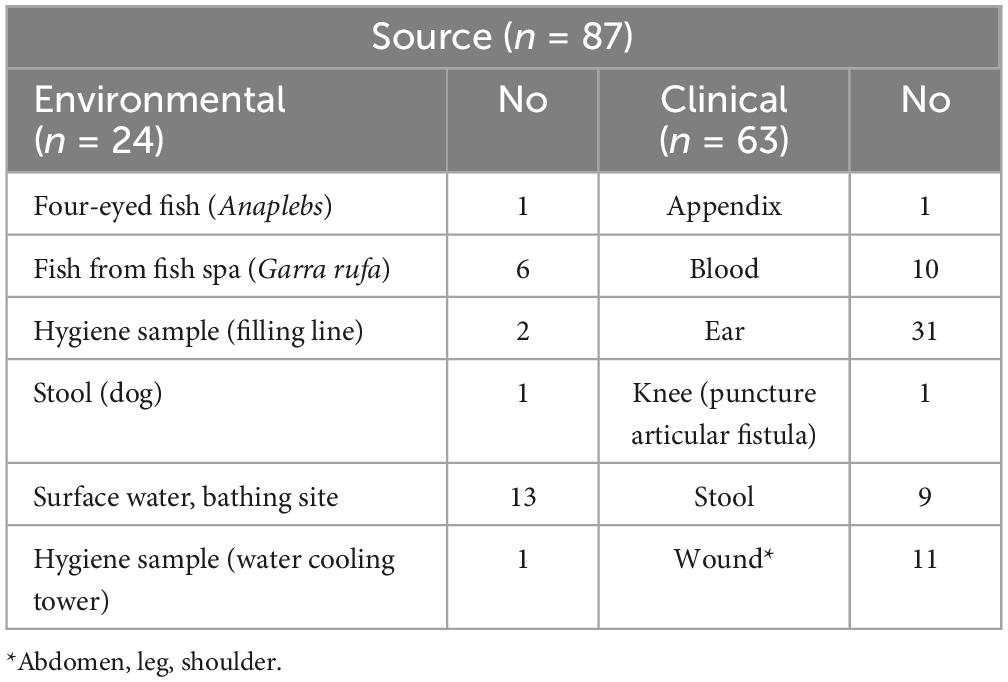
Table 1. The source of Vibrio cholerae non-O1/non-O139 isolates included in the study isolated from environmental and clinical samples from 2011 to 2021.
V. cholerae non-O1/non-O139 isolates from German patients isolated in 2018 and 2019 that were already characterized in the framework of another study (Brehm et al., 2021) were also excluded. Bacterial strains were previously isolated from hospital and clinical laboratories in Germany and Greece. To confirm the identification of V. cholerae non-O1/non-O139 sent to RKI for further analyses, and to differentiate V. cholerae non-O1/non-O139 from closely related Vibrionaceae such as Vibrio parahaemolyticus and Vibrio vulnificus, two independent real-time multiplex PCR assays were performed. The first real-time multiplex PCR (5′ nuclease assay) detected the V. cholerae-specific sequence of the housekeeping gene sodB (superoxide dismutase) and also confirmed the lack of enterotoxin gene ctxA described previously in details (Dupke et al., 2016). The second real-time multiplex PCR was used for the determination of serogroup-specific gene rfb of non-O1/non-O139 V. cholerae strains. This PCR was performed to verify that the isolates were not V. cholerae O1 or O139 strains that had lost the phage-encoded ctx gene before. Primers and probes are described in Supplementary Table 2. PCR conditions were as follows: 50°C for 2 min, 95°C for 10 min, followed by 35 cycles of PCR amplification at 95°C for 15 sec, 55°C for 60 sec.
2.2 Antimicrobial susceptibility testing
Vibrio cholerae non-O1/non-O139 isolates were cultivated on Columbia Sheep Blood agar (CBA, Oxoid, GmbH, Wesel, Germany), and on a thiosulfate–citrate–bile salts–sucrose agar (TCBS, Oxoid) at 37°C overnight. Antimicrobial susceptibility testing (AST) was performed using the disc diffusion and the gradient strip method for doxycycline only with results categorized applying EUCAST (European Committee on Antimicrobial Susceptibility Testing) breakpoints (version 13.0).1 For each bacterial isolate, several morphologically similar colonies were resuspended in 5 ml 0.85% NaCl with a density 0.5 McFarland, streaked onto Muller Hinton agar with tested antibiotics discs/strip and incubated at 36 ± 1°C for 18 ± 2 h. The testing was performed on the following antibiotic discs (Oxoid): piperacillin-tazobactam (36 μg), cefotaxime (5 μg), ceftazidime (10 μg), meropenem (10 μg), ciprofloxacin (5 μg), levofloxacin (5 μg), azithromycin (15 μg), erythromycin (15 μg), tetracycline (30 μg), trimethoprim-sulfamethoxazole (25 μg), and doxycycline [e-test, Liofilchem, Roseto degli Abruzzi (TE), Italy]. Escherichia coli ATCC 25922 and Staphylococcus aureus ATCC 29213 were used as quality control strains.
2.3 DNA extraction and whole genome sequencing
Bacterial DNA was extracted from colony material obtained from overnight cultures on CBA (Oxoid, GmbH, Wesel, Germany) using the Master Pure™ DNA purification Kit (Lucigen, Biozym Scientific GmbH, Hessisch Oldendorf, Germany) following the manufacturer’s instructions. The DNA concentration was assessed on the Qubit®2.0 Fluorometer (Life Technologies, Paisley, UK) using the Qubit dsDNA High Sensitivity (HS) assay kit (Life Technologies, Paisley, UK). For all samples, the DNA concentration ranged from 1.27 to 112 ng/μl. The Nextera XT DNA Sample Preparation Kit (Illumina, San Diego, CA, USA) was used for the library preparation, and the sequencing in paired-end mode (2 × 150 bp) was conducted on the NextSeq and MiSeq instrument (Illumina, San Diego, CA, USA). Prior to genome assembly, raw reads were quality controlled using FastQC (Trivedi et al., 2014); Trimmomatics (Bolger et al., 2014) was used for trimming, cropping and adapter clipping. The reads were down-sampled de novo assembled with SPADES (v3.15.5) (Bankevich et al., 2012). Also, the CLC Main Workbench (Qiagen, Hilden, Germany) was used for genome assembly. All genome sequences were uploaded to the NCBI Short Sequence Read Archive (SRA).2 The BioProject submission ID: PRJNA991120.
2.4 cgMLST target scheme definition
The publicly available, finished genome of V. cholerae O1 biovar El Tor (NCBI Accession: NC_002505.1, NC_002506.1) was selected as a “seed genome.” To determine the cgMLST gene set, a genome-wide gene-by-gene comparison using the cgMLST Target Definer (v. 1.4) function of the SeqSphere + software (v.7.2.3, Ridom GmbH, Münster, Germany) was performed with set parameters as previously described (Appelt et al., 2022). The identified genes were included in a pairwise comparison using BLAST (v.2.2.12) with parameters as follows: word size, 11; mismatch penalty, −1; match reward, 1; gap open costs, 5; gap extension costs, 2 in order to query chromosomes of selected V. cholerae strains. The selected query genomes are publicly available and comprise 13 genomes of V. cholerae strains from various outbreaks with different molecular profiles to cover the entire genetic diversity of V. cholerae (Supplementary Table 3).
The final cgMLST scheme was formed by including all genes of the reference genome that were common in all query genomes (Supplementary Table 4) with a sequence identity of ≥90% and 100% of overlap. Genes that lack a start or stop codon in one of the query genomes, as well as genes that had internal stop codons in more than 20% of the query genomes, were discarded. The final cgMLST scheme consisted of 2,710 target genes (68.4% of the reference genome V. cholerae O1 biovar El Tor str. N16961). The target genes were present in 81.8–97.4% of the examined Vibrio species isolates. The cgMLST assay will be made accessible to the public on www.cgmlst.org as soon as the manuscript is published.
2.5 cgMLST-based analysis
The genetic relationships of 87 V. cholerae non-O1/non-O139 isolates were evaluated by comparing two different cgMLST schemes: (i) the ad hoc cgMLST developed in this study (2,710 loci) and (ii) the publicly available V. cholerae cgMLST [2,457 defined loci (2,317 core loci)] (Liang et al., 2020); (pubMLST3). The Genome Comparator plug-in tool on pubMLST (Jolley and Maiden, 2010) was furthermore used to compare whole genomes of the V. cholerae isolates. For phylogenetic reconstructions minimum spanning trees (MST) analysis were performed and missing values were ignored during pairwise distance calculations. The allelic profiles were visualized with GrapeTree with the MSTree V2 algorithm (Zhou et al., 2018).
2.6 Virulence and genotypic profiles
The virulence and resistance profiles were determined with functions available on the Ridom SeqSphere + platform. To assess virulence genes, the assemblies of Vibrio isolates were compared to sequences stored in the Virulence Factor Database (VFDB) (Chen et al., 2016) with a threshold of >90% sequence identity and 100% coverage. The virulence profiles were then compared to five toxigenic V. cholerae O1 or O139 strains: Vib1 (V. cholerae NIH41, O1 classic) (Schirmeister et al., 2014), Vib3 (V. cholerae HK51, O1 El Tor) (Beutin et al., 1984), Vib4 (V. cholerae 1360, O1 classic) (Gallut and Quiniou, 1970), Vib10 (V. cholerae A-171-2, O1 El Tor) and Vib28 (V. cholerae 186-9, O139 El Tor) (Supplementary Table 1) (SRA BioProject submission ID: PRJNA991120).
The potential in silico antibiotic resistance of bacterial isolates was evaluated using the tool AMRfinder [NCBI AMRfinderPlus v2021-06-01.1; (Feldgarden et al., 2019)] focusing on core AMR proteins. The threshold was set to >90% sequence identity and 100% coverage. For visualization of results, the R software (version 4.1.3) was used (Wickham, 2011).
3 Results
3.1 Antibiotic resistance: antimicrobial susceptibility profile
The summary of the AST results is presented in Table 2. Further details are given in Supplementary Table 5. All of the tested antibiotics are recommended by the EUCAST guideline (v.13.0) for Vibrio species. In general, the majority of the examined V. cholerae non-O1/non-O139 strains (n = 87) were susceptible to beta-lactams (piperacillin-tazobactam, 3rd generation cephalosporin-cefotaxime and ceftazidime, meropenem), quinolones (ciprofloxacin, levofloxacin), macrolides (azithromycin, erythromycin), tetracyclines (tetracycline, doxycycline), and antifolate (trimethoprim-sulfamethoxazole) (Table 2).
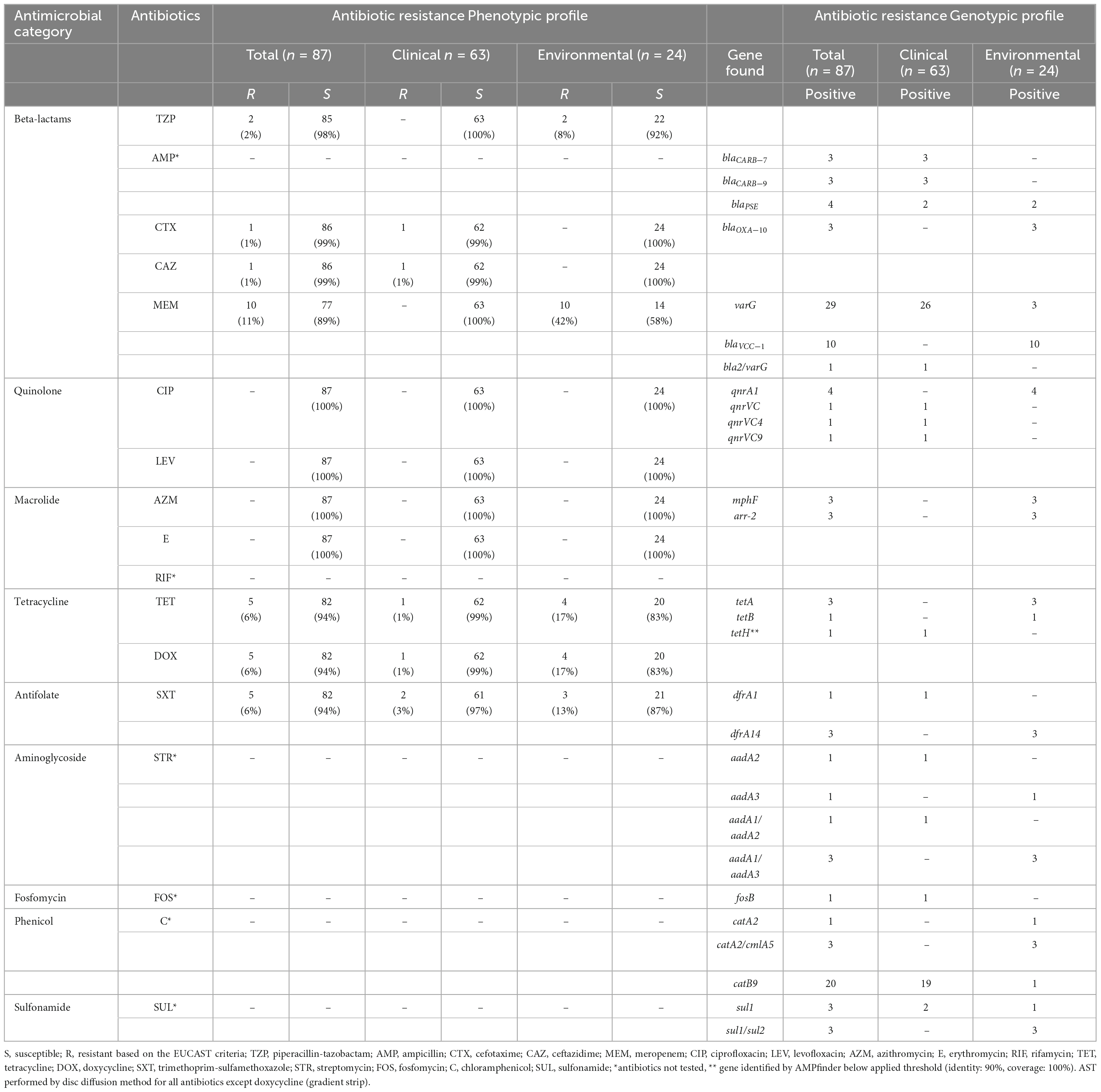
Table 2. Antimicrobial susceptibility testing (AST) and associated antimicrobial resistance determinants found by Illumina sequencing from clinical and environmental Vibrio cholerae non-O1/O139 isolates (n = 87) from Germany and other European countries, isolated from 2011 to 2021.
Based on the AST, 10 out of 24 environmental isolates (42%) were resistant to meropenem, two (8%) environmental isolates were resistant to piperacillin-tazobactam, and one clinical isolate was resistant to cefotaxime and ceftazidime. Five isolates (one clinical and four environmental) were resistant to tetracycline and doxycycline, and two clinical and three environmental isolates were resistant to trimethoprim. All strains were susceptible to ciprofloxacin, levofloxacin, azithromycin and erythromycin. Based on the definition of multidrug resistance (acquired non-susceptibility to at least one agent in three or more antimicrobial categories) (Magiorakos et al., 2012), we detected two environmental isolates out of all examined samples that were resistant to three antibiotic classes (beta-lactam: piperacillin-tazobactam; tetracyclines: tetracycline, doxycycline; and antifolate: trimethoprim-sulfamethoxazole).
3.2 Antibiotic resistance: genotypic profile
Detailed information regarding the genotypic profiles of V. cholerae non-O1/non-O139 isolates are given in Table 2 and Supplementary Table 6. The genotypic profiles disclosed antibiotic resistance genes (ARGs) that confer resistance to beta-lactams, quinolones, macrolides, tetracycline, antifolate, aminoglycosides, fosfomycin, phenicols and sulfonamide (Table 2; Figure 1). Among 87 isolates the sequencing revealed the presence of ARGs in 60 Vibrio isolates examined. Those included beta-lactamase genes belonging to Class A beta-lactamases: blaCARB–7 (n = 3), blaCARB–9 (n = 3), and blaPSE (n = 4); Class D beta-lactamases: blaOXA–10 (n = 3), and metalo-beta-lactamases: varG (n = 29), blaVCC–1 (n = 10) and one bla2/varG. Qnr resistance determinants are associated with low-level resistance or reduced susceptibility to quinolone. Here, despite the detection of several qnr resistance genes, all isolates were phenotypically susceptible to this agent, which could be associated with poor expression, silencing or inactivation of these genes. Genes that encode for resistance to macrolides (mphF) were identified in three environmental isolates, although no erythromycin resistance was observed in these isolates. Tetracycline (tetA/tetB/tetH) genes were detected in all five isolates with phenotypic tetracycline and doxycycline resistance. Trimethoprim (dfrA1/dfrA14) determinants were identified in four out of five isolates also phenotypically resistant to trimethoprim. In this study we only focus on antibiotics that are recommended by EUCAST for the treatment of Vibrio species infections, therefore AST was not performed on aminoglycosides, fosfomycin, rifamycin, chloramphenicol and sulfonamide, however, several associated ARGs were found.
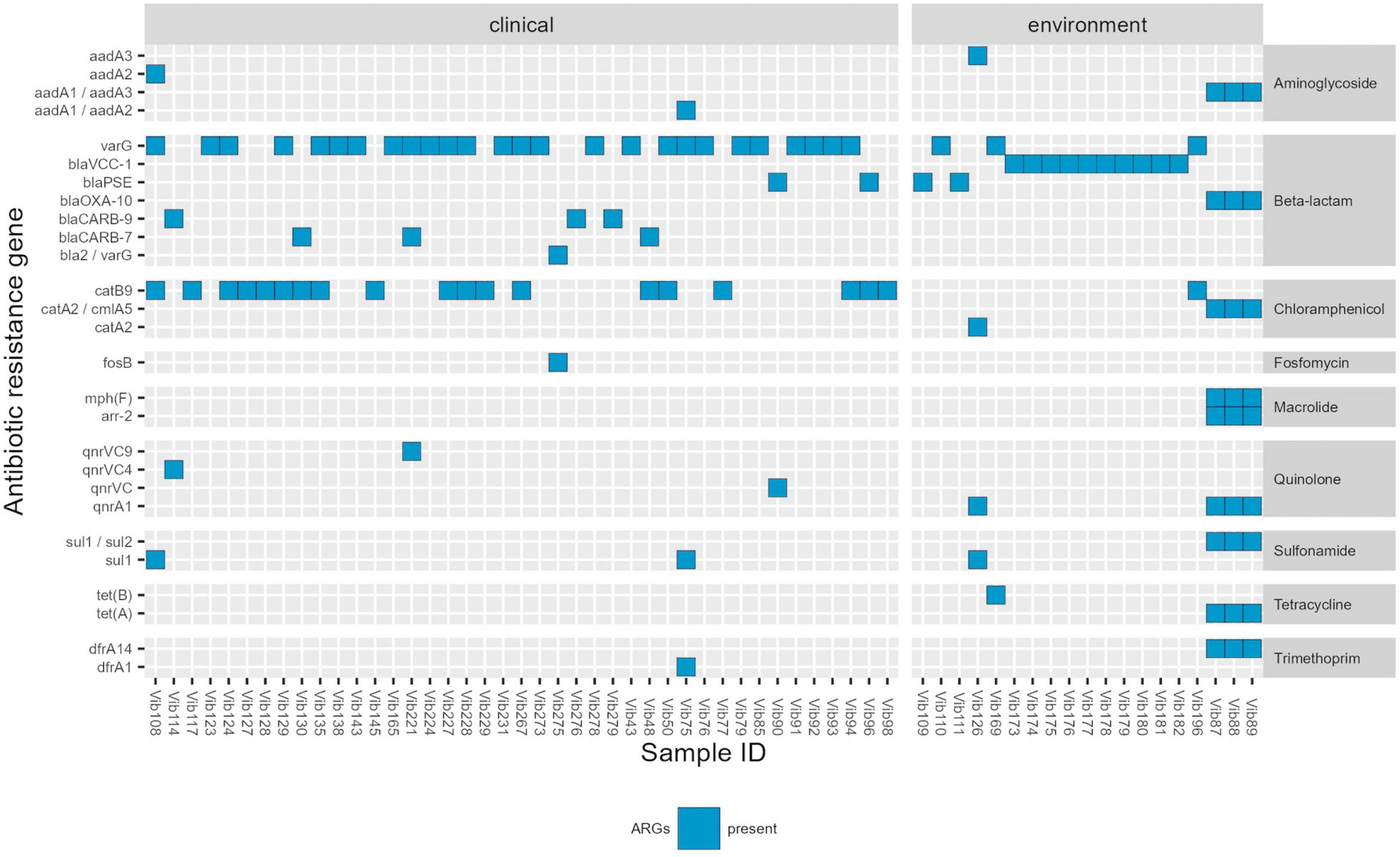
Figure 1. Genotypic profile of Vibrio cholerae non-O1/non-O139 isolates (n = 60) isolated in Germany and other European countries from 2011 to 2021. Legend: ARGs-Antimicrobial resistance genes. Threshold of >90% sequence identity and 100% of coverage against targets was applied.
3.3 Virulence genes profile
Among 87 Vibrio isolates, whole genome sequencing identified 37 virulence genes (VGs). The range of VGs per bacterial isolate was 2–34 with an average of 18 VGs per isolate. In total 38 virulence profiles were observed among all clinical and environmental isolates (Figure 2; Supplementary Table 7). None of the virulence profiles dominated in tested strains, however, one profile was observed more frequently, and it was detected in 8 clinical strains isolated from various sites (5 from ear, 2 from wound and one from blood culture) between 2014 and 2021. This virulence profile comprised the following genes: clpB, cqsA, hcp-2, hly-A, icmF, luxS, rtxC-D, vasA-L, vipA/mglA and vipB/mglB. Generally, virulence profiles were similar between clinical and environmental isolates, except for one clinical-stool isolate (Vib129) with the highest number (34 out of 37) of VGs detected and a cluster of 11 environmental isolates (Vib169, Vib173 to Vib182) with the lowest number of VGs found. The former isolate-Vib129, acquired in Netherlands during a cruise in 2016, possessed additional accessory colonization factors acfB/C/D and a cluster of toxin-coregulated pilus genes tcpA-E/H-J/P/Q which might be linked to toxigenic V. cholerae as possible origin. This isolate could have acquired the cluster of tcp proteins from toxigenic Vibrio isolates or alternatively remove the CTX element from toxigenic TCP positive Vibrio strains. Ten out of the eleven environmental isolates (Vib173-Vib182) with only 4–6 virulence genes were derived from the surface water in North Rhine-Westphalia, Germany (Baldeneysee, dammed river Ruhr) collected in 2018. The remaining Vib169 strain from that cluster, isolated from a fish (Garra rufa), contains only two VGs (cqsA, luxS). None of the tested isolates contained the cholera toxin genes (ctxA/ctxB) and the main virulence factors of the CTX phage such as zot (zonula occludens toxin), accessory cholera enterotoxin (ace), and factors for phage coat proteins (orfU).
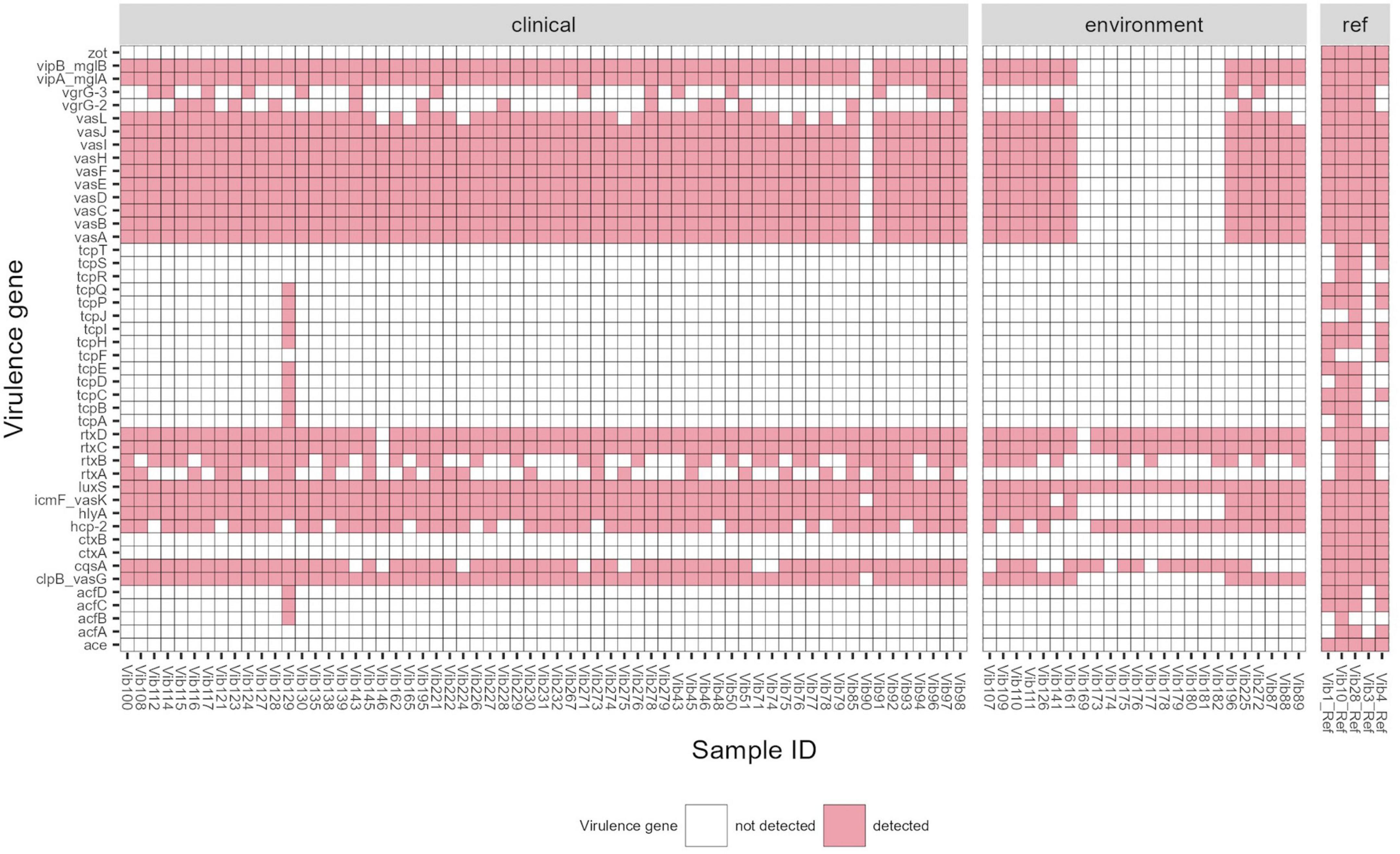
Figure 2. Virulence gene profile of Vibrio cholerae non-O1/non-O139 isolates (n = 87) isolated in Germany and other European countries from 2011 to 2021. Reference strains used for comparison: Vib1 (V. cholerae NIH41, O1 classic), Vib3 (V. cholerae HK51, O1 El Tor), Vib4 (V. cholerae 1360, O1 classic), Vib10 (V. cholerae A-171-2, O1 El Tor) and Vib28 (V. cholerae 186-9, O139 El Tor). Threshold of >90% sequence identity and 100% of coverage against targets was applied.
The prevalence of virulence factors found in clinical and environmental isolates is listed in Table 3. All examined isolates carried the luxS gene which is a part of the Vibrio species quorum sensing system controlling the expression of multiple genes in response to population density (Ye et al., 2008). The predominant virulence factors in V. cholerae non-O1/non-O139 were repeats-in-toxins- rtxC/rtxD (98%) and hemolysin- hlyA (87%), followed by 85–86% of isolates possessing different virulence-associated type VI secretion proteins (T6SS)- vasA-K, clpB, vipA/mglA, vipB/mglB, icmF. Other common virulence factors were hemolysin-coregulated protein- hcp-2 and quorum sensing protein- cqsA (79 and 82%, respectively). Other, less frequently found virulence proteins included rtxA (31%) and rtxB (56%) and proteins associated with T6SS–vgrG-2 (18%) and vgrG-3 (20%). rtxA gene was identified only in clinical isolates (n = 25), and in 7 (28%) out of these 25 isolates were from stool isolates.
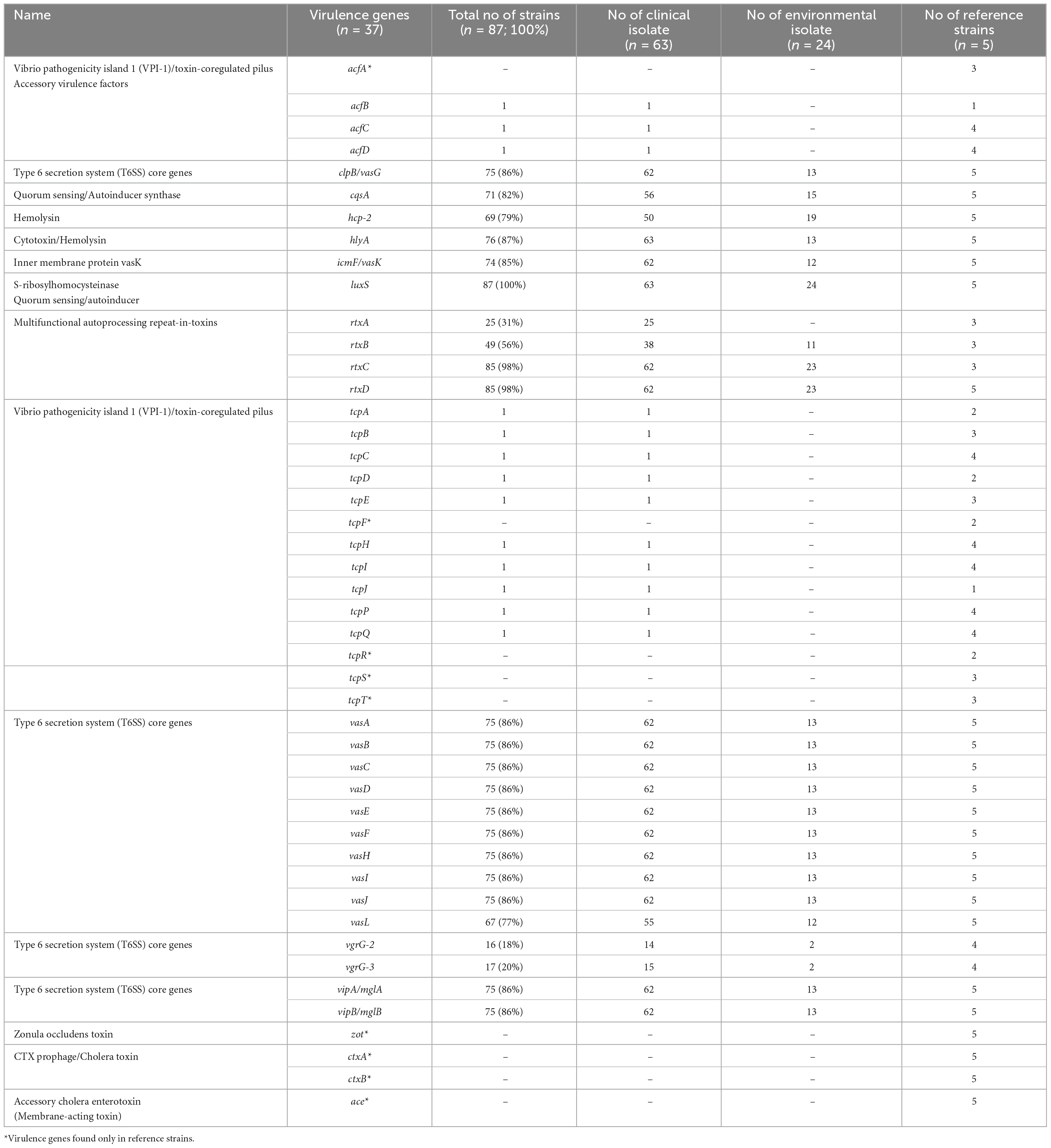
Table 3. Frequency of virulence factors in clinical and environmental Vibrio cholerae non-O1/non-O139 isolates isolated in Germany and other European countries from 2011 to 2021.
3.4 Genetic relatedness between the isolates using cgMLST schemes
Both tested schemes were comparable and revealed identical groupings of closely related isolates with only few allele differences between the two assays. The minimum spanning tree (MST) generated from the ad hoc cgMLST scheme using the Ridom SeqSphere + software is shown in Figure 3. It displayed a high level of genetic diversity between epidemiologically unlinked V. cholerae non-O1/non-O139 isolates with the maximum allelic distances of 2,450 (Supplementary Table 8). The minimum spanning tree (MST) generated from publicly available Vibrio cholerae cgMLST scheme on the pubMLST is presented in Figure 4. The maximum allelic distance among isolates was 2,435 and the distance matrix is shown in Supplementary Table 9. The allelic profiles for ad hoc cgMLST scheme (Ridom SeqSphere +) and pubMLST scheme are given in Supplementary Table 10 and Supplementary Table 11, respectively.
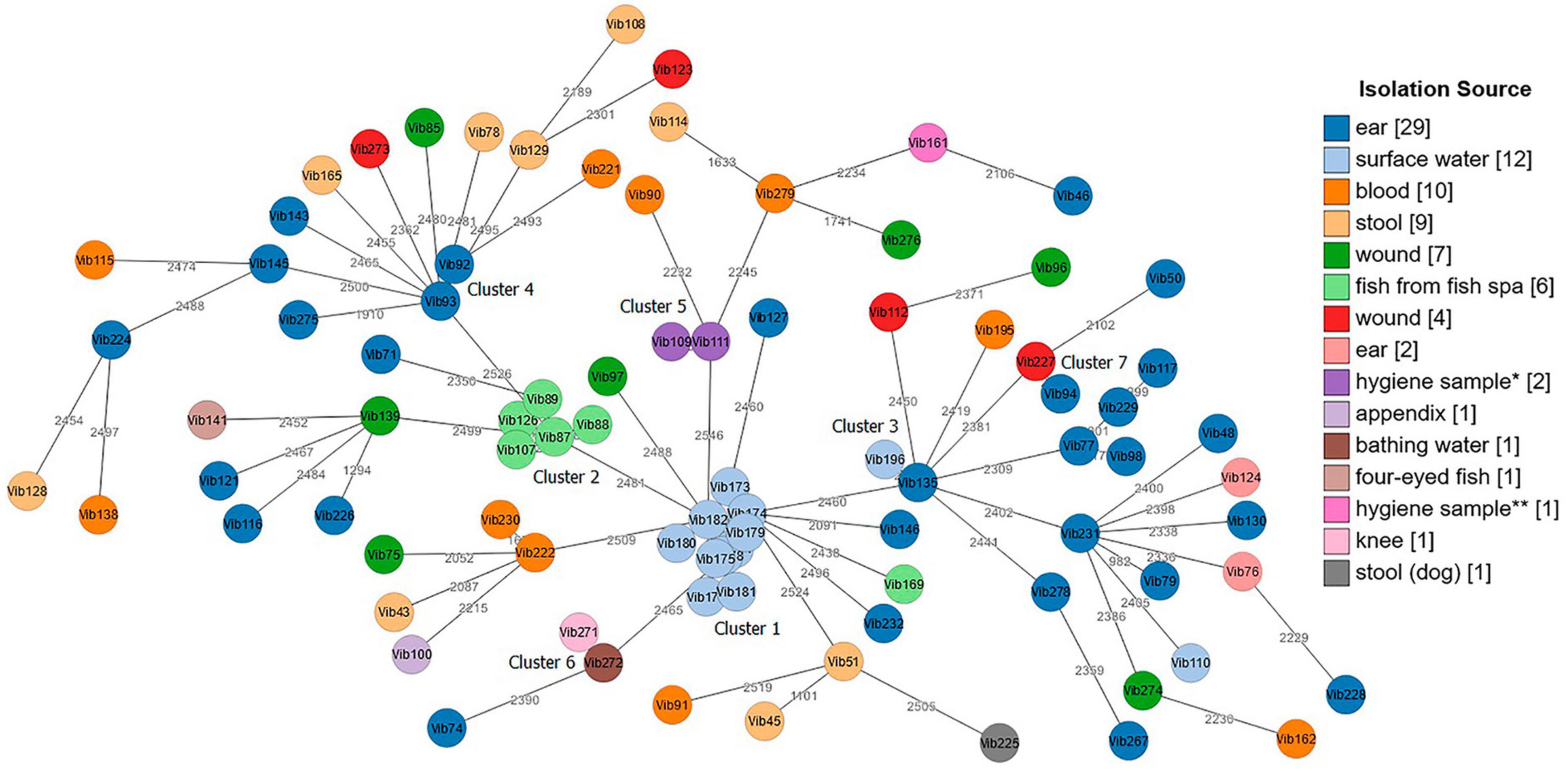
Figure 3. Minimum spanning tree (MST) of Vibrio cholerae non-O1/non-O139 isolates (n = 87) generated from ad hoc cgMLST Ridom SeqSphere + scheme. Distance based on 2,710 core genes V. cholerae N16961 reference strain. Each circle represents one isolate. Numbers between nodes indicate allelic distances between the isolates (maximum allelic distance 2,450). MST cluster distance threshold: 20. Isolates are color-coded based on their isolation source. The visualization of the tree was performed using stand-alone GrapeTree software. *Hygiene sample (filling line); **hygiene sample (water cooling tower).
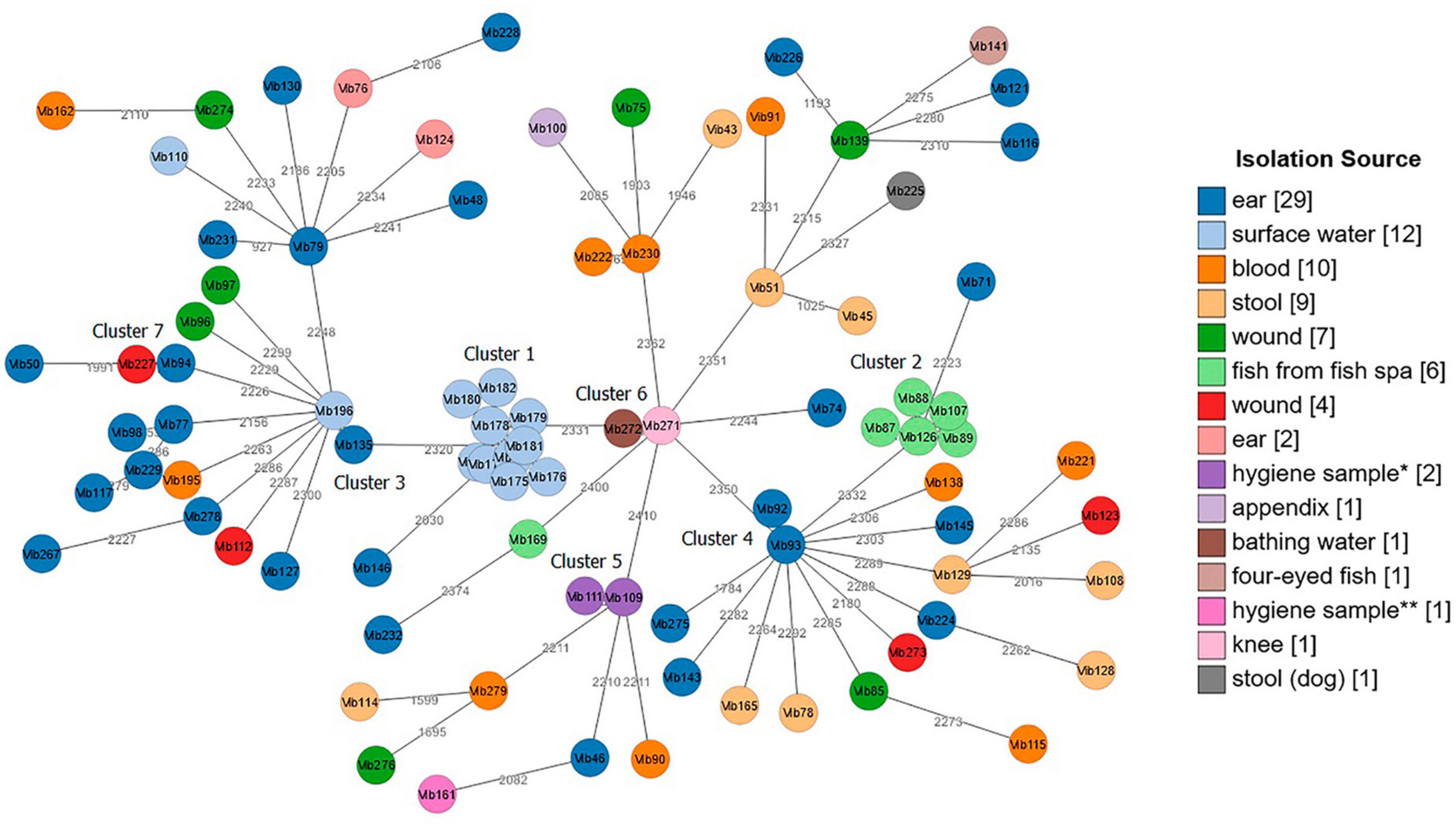
Figure 4. Minimum spanning tree (MST) of Vibrio cholerae non-O1/non-O139 isolates (n = 87) generated from Vibrio cholerae pubMLST scheme. Distance based on 2,457 defined loci. Each circle represents one isolate. Numbers between nodes indicate allelic distances between the isolates (maximum allelic distance 2,435). MST cluster distance threshold: 20. Isolates are color-coded based on their isolation source. The visualization of the tree was performed using stand-alone GrapeTree software. *Hygiene sample (filling line); **hygiene sample (water cooling tower).
Both cgMLST assays identified 7 clusters with closely related Vibrio isolates (allelic distances < 20) suggesting a possible epidemiological linkage (Figures 3, 4). Three out of these 7 clusters (Cluster 1, 2, and 5) derived from various environmental sources. Cluster 4 and Cluster 7 contained samples isolated from clinical sites, and Clusters 3 and 6 included samples collected from both clinical and environmental sources. Cluster 1, consisting of strains Vib173-Vib182 comprised the largest number (n = 10) of closely related isolates with less than 5 (Ridom) and less than 20 (pubMLST) allelic distances. These isolates were collected within 2 days from the same surface (fresh) water (Baldeney See, dammed river Ruhr) in North Rhine-Westphalia, within the context of the 2018 bathing water quality project in Germany (Schmithausen et al., 2019).
Cluster 2, the second largest cluster, contained 5 isolates (Vib87-Vib89 and Vib107, Vib126) with allelic distances less than 10 (Ridom) and less than 20 (pubMLST). Swabs from isolates of this cluster were taken from the mucus membrane of fish (Garra rufa) from a fish spa at different collection dates. Three out of these 5 isolates (Vib87-Vib89) derived from the same sample (2014), but isolates differed phenotypically. Two other isolates (Vib107 and Vib126) were collected from fish of two different spa-pools of the same location, and were collected in 2015 and 2016, respectively. The close genetic relationship of all isolates from Cluster 2 indicates the long-term presence of a single strain in both spa-pools. Another isolate from this spa (Vib169), isolated in 2018, differed in more than 2,000 alleles, which suggests the presence of a second, distantly-related strain at this location.
The remaining five clusters consisted of two isolates each. Cluster 3 comprised strains Vib135 and Vib196. While isolate Vib135 was taken from the ear of a patient with chronic otitis in 2017, isolate Vib196, with a difference of six alleles (Ridom) and 11 alleles (pubMLST), was isolated in 2019 from an environmental source (bathing water). A possible epidemiological linkage exists insofar as the patient lived in close proximity to the bathing area. However, it was not possible to determine whether the patient actually bathed in the water. On the contrary, strain Vib195, isolated from blood culture from another patient who had been shown to have bathed in the same bathing water shortly before the sample from which strain Vib196 was isolated, showed a difference of over 2,000 alleles. Despite the spatial proximity and the temporal connection, an epidemiological linkage could not be confirmed in this case.
Cluster 4 included two closely related isolates Vib92 and Vib93 (one allele difference in Ridom and 3 alleles difference in pubMLST) collected from the same patient’s ear in 2014. Cluster 5 compromised two almost identical environmental hygiene isolates Vib109 and Vib111 (no allele difference in Ridom and two alleles differences in pubMLST). Both isolates were collected from the filling line in 2015 in Belgium but they differed phenotypically. Cluster 6 comprised two strains (Vib271 and Vib272) that were indistinguishable in cgMLST analysis. Vib271 was isolated from the knee synovia of a patient, whereas strain Vib272 was isolated from the bathing site where the patient had previously bathed. In contrast to Cluster 3, a clear epidemiological connection between the patient isolate and the isolate from the bathing site could be demonstrated in this case. Cluster 7 included two clinical isolates, Vib94 and Vib227, isolated from two patients from ear and wound sites with less than 20 (both schemes) allelic differences.
4 Discussion
In Germany, non-cholera Vibrio infections have been reported from coastal waters of the Baltic Sea and North Sea (Boer et al., 2013) with seasonal correlation. Most cases are registered in the warmer months and males are affected more often than females (Brehm et al., 2021). In the framework of a German research project, the VibrioNet4 the Vibrio database (VibrioBase) was created to improve identification of marine environmental Vibrio species (Erler et al., 2015) and to investigate vibriosis among clinical sources across Europe. Several studies in Germany revealed the genotypic and phenotypic patterns of non-toxigenic Vibrio species among clinical and environmental isolates (Schirmeister et al., 2014; Bier et al., 2015; Hammerl et al., 2017; Schwartz et al., 2019; Brehm et al., 2021). The study published by Hammerl et al. (2017) showed that carbapenemase-producing non-O1/non-O139 V. cholerae isolates are present in aquatic environments in Germany and represent environmental reservoir of carbapenem resistance. To get an insight into molecular epidemiology of Vibrio species isolates available in Germany, we conducted a retrospective study to disclose virulence and resistance patterns of Vibrio cholerae non-O1/non-O139 isolates derived from Germany and other European countries from 2011 to 2021. We also investigated the genetic relatedness between clinical and environmental V. cholerae non-O1/non-O139 isolates using two cgMLST schemes.
4.1 Antimicrobial susceptibility profile
A recent guideline in Germany recommended tetracycline (doxycycline) and 3rd generation cephalosporin (ceftriaxone) for skin and soft tissue infections associated with exposure to water that is contaminated by Vibrio species (Sunderkotter et al., 2019). These antibiotic agents have been also successfully used in the treatment of bacteremia caused by non-toxigenic Vibrio species (Marek et al., 2013; Lan et al., 2014). Moreover, treatment with piperacillin/tazobactam (Kadkhoda et al., 2012), azithromycin (Marinello et al., 2017), fluoroquinolone (Chowdhury et al., 2016), ampicillin (Feghali and Adib, 2011), and meropenem (Torp-Pedersen et al., 2012; Lu et al., 2014) has been well described in non-O1/non-O139 Vibrio cholerae infections. While researchers pay attention to antimicrobial resistance patterns in toxigenic Vibrio cholerae, such data is rarely published for non-toxigenic Vibrio isolates.
In our study, the proportion of resistance among clinical and environmental isolates against antimicrobial agents recommended as first line treatment for Vibrio infections were low meaning that empirical treatment would likely have been effective. Similar observations have been reported in German studies (Bier et al., 2015; Brehm et al., 2021) and also in other countries (Ceccarelli et al., 2015; Ottaviani et al., 2018). We noted the highest resistance rate among 10 environmental isolates against beta-lactams, specifically meropenem (42%). These 10 out of the 24 tested environmental strains were collected within 2 days from bathing waters (Baldeneysee, dammed river Ruhr) in North Rhine-Westphalia, Germany as part of the screening project to seek antibiotic-resistant intestinal bacteria (Escherichia coli, other Coliforms and Enterococci) and antibiotic residues (Schmithausen et al., 2019). The investigation resulted in an unexpected outcome when non-toxigenic Vibrio species were detected. Among all examined isolates we reported two environmental MDR non-toxigenic Vibrio species isolates that revealed resistance to three antibiotic classes: beta-lactam (piperacillin-tazobactam), tetracyclines (tetracycline, doxycycline) and antifolate (trimethoprim-sulfamethoxazole). These two isolates were collected from the same source (routine testing of Garra rufa fish from fish tank). Garra rufa is a freshwater fish naturally inhabiting river basins in central Eurasia. They are widely used in cosmetic treatment, in particular for pedicures and body spa to remove dead skin. Identification of Vibrio species from this fish species was previously published (Verner-Jeffreys et al., 2012), however, the antimicrobial resistance pattern was not tested. In contrast to our outcome, the study performed by Bier et al. (2015) in Germany on a broad collection of clinical, environmental and sea food, non-O1/O-139 Vibrio isolates did not reveal any multidrug-resistant strains. Our finding suggests that MDR Vibrio strains are present in environmental sources, and might contribute to transmission of antibiotic resistance from fish or water in a fish spa to vulnerable clients.
4.2 Antimicrobial resistance genes profile
Genotypic profiling disclosed antibiotic resistance genes that mediate resistance to several antibiotic classes, however, not all were confirmed by phenotypic profiling. In our study, we detected several beta-lactamase genes both in clinical and environmental isolates. These included carbenicillin-hydrolyzing beta-lactams: blaCARB–7, blaCARB–9 and blaPSE whose presence has already been described in non-toxigenic Vibrio isolates (Lepuschitz et al., 2019; Brehm et al., 2021; Adesiyan et al., 2022) suggesting activity against amino- and carboxy-penicillin (Lepuschitz et al., 2019), but susceptibility testing was not performed on these antibiotic agents. Most antibiotic resistance determinants are encoded by gene cassettes of Class 1 integrons which compromise two conserved segments (5′-CS and 3′-CS) broken down by variable region (Stokes and Hall, 1989). However, both blaCARB–7 andblaCARB–9 are located in the super integron (VCR island) of the V. cholerae genome (Melano et al., 2002; Petroni et al., 2004). Sequence analysis of three environmental isolates (Vib87, Vib88, Vib89), collected from fish (Garra rufa), revealed the presence of blaOXA–10 and various other resistance determinants that encode for trimethoprim (dfrA14) and tetracycline (tetA), both confirmed by phenotypic profile; sulfonamide resistance (sul1, sul2), quinolone resistance (qnrA1), macrolide resistance (arr-2, mphF) and chloramphenicol resistance (cat2, cmlA5), and aminoglycoside resistance (aadA1, aadA2). The MDR profile disclosed in Vib87 and Vib88 isolates might be associated with the presence of a 7.5 kb plasmid and a very low copy number plasmid of unknown molecular size as well as Class 1 integrons as previously described (Rajpara et al., 2009). The study revealed that the mechanism of multidrug-resistance of Vibrio fluvialis isolates, acquired from patients with acute cholera-like diarrhea in Kolkata, India, is associated with the acquisition of the 7.5 kb pVN84 plasmid originating from Vibrio cholerae O1 in Vietnam indicating the plasmid exchange between two species. The follow up study (Rajpara et al., 2018) on the same MDR V. fluvialis isolate showed a similar genetic profile, as described here, located on the low copy number plasmid that bears multiple resistance genes.
The largest number of Vibrio isolates exhibited genotypic resistance to varG and blavcc–1, which demonstrated activity against penicillin, cephalosporins, and carbapenems (Mangat et al., 2016; Lin et al., 2017). The high prevalence of the varG gene has been demonstrated in a recent study conducted on various Vibrio species isolates collected from human, food, animal and environmental sources in Latin America (Vilela and Falcão, 2021). Carbapenemase VCC-1, which produces non-toxigenic V. cholerae strains, was described for the first time by Canadian researchers in 2016 (Mangat et al., 2016). blavcc–1 was identified in a shrimp imported into Canada for human consumption. A similar finding was published a year later by a German study (Hammerl et al., 2017). They detected blavcc–1 in several non-toxigenic V. cholerae strains collected from different locations on the coastline waters in Germany. Similarly, we detected blavcc–1 in ten meropenem-resistant non-O1/non-O139 V. cholerae isolates acquired from surface water in Germany during routine testing. This finding suggested that V. cholerae strains which produce carbapenemase VCC-1 are continuously present in German waters and might be acquired by seafood or fish inhabiting the aquatic environments, as it was discovered in Canada, entering the human body and developing various clinical symptoms.
The presence of other as-yet unmentioned genetic determinants that confer resistance to aminoglycoside [aph(6)-Id], chloramphenicol (floR, catB9), quinolone (qnrVC), tetracycline (tetB) and trimethoprim (dfrA1) has already been described in Vibrio species (Rahmani et al., 2012; Lepuschitz et al., 2019; Jackel et al., 2020). The fosB gene, which confers resistance to fosfomycin, was identified in one of our clinical isolates collected from an ear location with clinical manifestation as otitis media. Fosfomycin is usually used for the treatment of urinary tract infections caused by Enterobacterales infections (Falagas et al., 2010) and has a moderate activity against Vibrio species (Reparaz and Fernandez, 1977). It is not a common agent in the treatment of Vibrio infections, although it was used in combination with other antibiotics for the treatment of necrotizing fasciitis of the lower leg caused by non-toxigenic Vibrio cholerae (Hirk et al., 2016). To our knowledge, the presence of this determinant in Vibrio species has never yet been reported, and no resistance to fosfomycin has been described.
4.3 Virulence gene profiles and genetic diversity
Two cgMLST schemes have been used to assess genetic relatedness between clinical and environmental isolates. Both cgMLST schemes yielded comparable results, and disclosed high genetic diversity between all tested isolates. Similar findings have been seen in V. cholerae non-O1/non-O139 environmental strains of the Baltic Sea in a German study (Schwartz et al., 2019). Both cgMLST schemes also revealed seven clusters, each compromising closely related isolates with 0–19 (Ridom) and 0–18 (PubMLST) allelic differences. Most of the clusters contained isolates with regional or within-patient pattern, except two isolates in Cluster 7 which derived from different clinical sources and regions. We did not observe a distinct country-wide spread of clonal lineages. For the PubMLST cgMLST assay, a threshold of 7 allelic differences was determined for strains of the same outbreak or with a clear epidemiological linkage (Liang et al., 2020) and up to 40 allelic differences for closely related strains. Based on these cut-offs and by directly comparing the allelic differences of both assays, an approximate cut-off of 3 allelic differences for outbreak strains and a cut-off of 20 for closely related strains can be applied for the ad hoc scheme.
Numerous studies aimed to disclose virulence genes in non-toxigenic Vibrio cholerae non-O1/non-O139 isolates by PCR (Schirmeister et al., 2014; Schwartz et al., 2019); they mainly focused on well-known targets. Here, we investigated virulence gene profiles by a whole-genome sequencing-based approach which delivered a more comprehensive picture than targeted PCR alone. In total, we identified 38 different virulence profiles among all 87 Vibrio isolates. Though we observed genetic diversity among clinical and environmental isolates in general, virulence gene profiles have similar patterns regardless of the source of the isolates. A similar finding was noted in German and Austrian studies (Schwartz et al., 2019; Bhandari et al., 2023), in which virulence factors found in clinical isolates were also present in environmental strains regardless of sequence type. The major virulence factors, ctxAB, zot, ace, and rstA/B/R, were not detected in the examined isolates, while two clusters of toxin-coregulated pilus genes (tcpA-E/H-J/P/Q and acfB/C/D) were identified in one clinical isolate recovered from a stool sample. The presence of these two virulence clusters, especially tcpA and acfB genes, have been reported in both clinical and environmental non-O1/non-O139 V. cholerae strains (Novais et al., 1999; Theophilo et al., 2006; Jagadeeshan et al., 2009). The pilus colonization clusters (TCP) are located on the Vibrio pathogenicity island (VPI-1) and play a role in attachment to the host epithelial cells. They also serve as a receptor for CTX prophage (Krebs and Taylor, 2011). Vibrio cholerae isolates that are negative for ctxAB but positive for tcpA have the highest risk of disease outbreak among the non-toxigenic Vibrio isolates with mild or moderate clinical symptoms (Wang et al., 2020). Some authors suggest that non-toxigenic Vibrio strains with a profile ctxAB –/tcpA+ might be a precursor of toxigenic strains due to the function of the TCP element (Theophilo et al., 2006), and such profile has been observed in our study in one clinical isolate acquired from a stool sample.
Human infections with non-toxigenic V. cholerae mostly manifest as an acute gastroenteritis, ear infections, or severe wound infections. It is important to identify the virulence factors associated with the pathogenesis of these infections. We looked closer into the virulence profile of non-toxigenic Vibrio strains isolated from stool, wound and ear sites. Some of the virulence genes were present in all strains, and some were identified only with a low frequency, e.g., valine-glycine repeat protein G (vgrG-2, vgrG-3) which plays role in competing against the microbial community in the natural environment or in the human gastrointestinal tract (Brooks et al., 2013). A German study revealed that among environmental and clinical strains of non-cholerae Vibrio isolates, the most frequently found virulence genes were toxR, El Tor hlyA, ompU and rtxC (Schwartz et al., 2019). Another study conducted on non-O1/non-O139 V. cholerae environmental isolates in Maryland (Ceccarelli et al., 2015) showed the predominance of hlyA, hap, rtxA, vasA, vasK and vasH genes. Here, we did not observe any toxR and ompU genes, and we did not differentiate classical hlyA gene from El Tor hlyA type. All isolates examined from stool, wound and ear sites in this study showed the presence of virulence genes for T6SS (clpB, vasA-L, vipA-B), quorum sensing genes (luxS), hemolysin gene (hlyA), inner membrane protein (icmF) and repeats-in-toxins genes (rtxC-D), which agreed with previous studies conducted in non-cholera V. cholerae isolates from ducks (Hirsch et al., 2020), clinical sites (Brehm et al., 2021) and environmental source (Shan et al., 2022).
T6SS contains virulence factors that play a role in survival in both the aquatic and host environments by competitive exclusion of bacterial competitors (MacIntyre et al., 2010), promote intestinal colonization in the host indicating intestinal inflammation in vivo (Ma and Mekalanos, 2010) and also foster horizontal gene transfer (Borgeaud et al., 2015). The activity of T6SS is regulated by various mechanisms, such as the expression of hemolysin co-regulated proteins (hcp-2 and hcp-3) controlled by a quorum sensing system (QSS) (Ishikawa et al., 2009). The present of hcp genes was observed in more than half of those isolates (6 out of 9 strains from stool, 24 out of 31 strains from ear and 9 out of 11 from wounds). QSS is bacterial monitoring system involving in various functions, e.g., to control population density in the specific niche, biofilm formation and virulence genes expression. Two genes, luxS and cqsA, are a part of the QSS system, and they were identified in all examined isolates acquired from stool and wound, while cqsA was detected in 81% of the isolates collected from ears. These two virulence genes were also found in environmental non-toxigenic Vibrio strains (Schwartz et al., 2019).
The presence of the hemolysin gene (hlyA), involved in cytotoxicity and apoptosis induction (Kanoktippornchai et al., 2014), and repeats-in-toxin gene cluster (rtxA-D) have been widely observed in non-O1/non-O139 Vibrio isolates collected from the coastal waters of the Baltic Sea and the North Sea (Schwartz et al., 2019) and in other studies (Dutta et al., 2013; Ceccarelli et al., 2015). Here, hlyA and rtxC-D proteins were observed in most of the tested isolates, while other repeats-in-toxins genes, rtxA and rtxB, were less common. The repeats-in-toxin RTX is a group of cytolysins and cytotoxins produced by gram-negative bacteria. RTX proteins are defined by the presence of characteristic repetitions of glycine and aspartate in the toxin protein sequence and by the unique mode of the secretion type I extracellular secretion system (TISS) (Linhartova et al., 2010). A study conducted by Schirmeister et al. (2014) revealed that the rtxA gene occurred only in non-toxigenic Vibrio recovered from diarrhea samples. Similar to our study, the rtxA gene was only identified in clinical isolates (Table 3) and of those, rtxA was observed in 7 out of 9 (77%) clinical stool isolates. To get an insight into those isolates we checked the metadata, however, they turned out to be incomplete. Three patients reported travelling abroad (Greece, Amsterdam and Danube Delta), and in one patient, consumption of crabs in a German city was reported as causing an infection. rtxA encodes multifunctional autoprocessing repeats-in-toxin, and while its role it not entirely clear, it is suspected that it is linked to increased epithelial cell damage in the in vivo model (Satchell, 2007; Lee et al., 2008).
5 Conclusion
The main aim of this study was to perform molecular characterization of non-O1/non-O139 Vibrio cholerae isolates isolated from environmental and clinical samples, including the determination of virulence and antibiotic resistance gene patterns, and to assess whether these isolates were closely genetically related. We found that most of the isolates were susceptible to the antibiotics we tested and recommend that first line treatment for Vibrio infection would likely be effective in clinical isolates if needed. Concern remains over the escalating carbapenemase VCC-1 produced by non-toxigenic V. cholerae strains in aquatic environments. The results of this analysis suggested that carbapenemase-producing non-O1/non-O139 V. cholerae are constantly present in surface water in Germany, and that multidrug-resistant Vibrio isolates are also found in fish spas. Nowadays non-cholera Vibrio species are frequently detected in aquatic environments in Europe, and due to climate change, the abundance of Vibrio species in the aquatic niche might rise; this may lead to an increase in Vibrio species infections in the human population. The virulence profile disclosed that both clinical and environmental isolates possess similar virulence factors. The main virulence genes originally presence in toxigenic Vibrio cholerae were not common in examined isolates, however, some, like the cluster of toxin-coregulated pilus genes, can be sporadically isolated in clinical isolates manifesting with gastrointestinal symptoms. Due to horizontal virulence and resistance gene transfer, systematic monitoring in aquatic environments should be considered as one of the counter-measures to seek antimicrobial resistance and virulence patterns among Vibrio isolates. The genetic diversity among our isolates was high, however, we identified a cluster with identical environmental and clinical isolates, which suggests that direct exposure of water that has been contaminated by Vibrio species may be one of the sources of acquiring the infection. More studies are needed to truly understand the molecular basis of genetic elements involved in pathogenicity of V. cholerae non-O1/non-O139 and how they are transmitted.
Data availability statement
The original contributions presented in the study are publicly available. This data can be found here: https://www.ncbi.nlm.nih.gov/bioproject/; PRJNA991120/.
Author contributions
KS: Conceptualization, Formal analysis, Investigation, Methodology, Validation, Writing—original draft, Writing—review and editing, Data curation, Visualization. HS: Conceptualization, Data curation, Formal analysis, Investigation, Methodology, Validation, Writing—original draft, Writing—review and editing, Resources. SA: Data curation, Methodology, Validation, Writing—review and editing. JM: Writing—review and editing, Formal analysis, Investigation. DJ: Formal analysis, Writing—review and editing, Conceptualization, Methodology, Resources, Supervision, Validation, Writing—original draft. SD: Conceptualization, Formal analysis, Methodology, Resources, Supervision, Validation, Writing—original draft, Writing—review and editing, Investigation, Project administration.
Funding
The author(s) declare financial support was received for the research, authorship, and/or publication of this article. The sequencing was financially covered by the internal funding (9PP RKI). KS was supported financially by the European Centre for Disease Prevention and Control (ECDC) fellowship.
Conflict of interest
The authors declare that the research was conducted in the absence of any commercial or financial relationships that could be construed as a potential conflict of interest.
Publisher’s note
All claims expressed in this article are solely those of the authors and do not necessarily represent those of their affiliated organizations, or those of the publisher, the editors and the reviewers. Any product that may be evaluated in this article, or claim that may be made by its manufacturer, is not guaranteed or endorsed by the publisher.
Author Disclaimer
The views and opinions expressed herein do not state or reflect those of ECDC. ECDC is not responsible for the data and information collation and analysis and cannot be held liable for conclusions or opinions drawn.
Supplementary material
The Supplementary Material for this article can be found online at: https://www.frontiersin.org/articles/10.3389/fmicb.2023.1282135/full#supplementary-material
Footnotes
- ^ www.eucast.org
- ^ www.ncbi.nlm.nih.gov/sra
- ^ https://pubmlst.org/vcholerae/
- ^ http://www.vibrionet.de/
References
Adesiyan, I. M., Bisi-Johnson, M. A., and Okoh, A. I. (2022). Incidence of antibiotic resistance genotypes of Vibrio species recovered from selected freshwaters in Southwest Nigeria. Sci. Rep. 12:18912. doi: 10.1038/s41598-022-23479-0
Appelt, S., Rohleder, A. M., Jacob, D., von Buttlar, H., Georgi, E., Mueller, K., et al. (2022). Genetic diversity and spatial distribution of Burkholderia mallei by core genome-based multilocus sequence typing analysis. PLoS One 17:e0270499. doi: 10.1371/journal.pone.0270499
Arteaga, M., Velasco, J., Rodriguez, S., Vidal, M., Arellano, C., Silva, F., et al. (2020). Genomic characterization of the non-O1/non-O139 Vibrio cholerae strain that caused a gastroenteritis outbreak in Santiago, Chile, 2018. Microb. Genom. 6:e000340. doi: 10.1099/mgen.0.000340
Baker-Austin, C., Oliver, J. D., Alam, M., Ali, A., Waldor, M. K., Qadri, F., et al. (2018). Vibrio spp. infections. Nat. Rev. Dis. Primers 4, 1–19. doi: 10.1038/s41572-018-0005-8
Baker-Austin, C., Trinanes, J., Gonzalez-Escalona, N., and Martinez-Urtaza, J. (2017). Non-cholera Vibrios: The microbial barometer of climate change. Trends Microbiol. 25, 76–84. doi: 10.1016/j.tim.2016.09.008
Bankevich, A., Nurk, S., Antipov, D., Gurevich, A. A., Dvorkin, M., Kulikov, A. S., et al. (2012). SPAdes: A new genome assembly algorithm and its applications to single-cell sequencing. J. Comput. Biol. 19, 455–477. doi: 10.1089/cmb.2012.0021
Belkin, I. M. (2009). Rapid warming of large marine ecosystems. Prog. Oceanogr. 81, 207–213. doi: 10.1016/j.pocean.2009.04.011
Beutin, L., Bode, L., Richter, T., Peltre, G., and Stephan, R. (1984). Rapid visual detection of Escherichia coli and Vibrio cholerae heat-labile enterotoxins by nitrocellulose enzyme-linked immunosorbent assay. J. Clin. Microbiol. 19, 371–375. doi: 10.1128/jcm.19.3.371-375.1984
Bhandari, M., Rathnayake, I. U., Huygens, F., and Jennison, A. V. (2023). Clinical and environmental Vibrio cholerae non-O1, non-O139 strains from Australia have similar virulence and antimicrobial resistance gene profiles. Microbiol. Spectr. 11:e0263122. doi: 10.1128/spectrum.02631-22
Bier, N., Schwartz, K., Guerra, B., and Strauch, E. (2015). Survey on antimicrobial resistance patterns in Vibrio vulnificus and Vibrio cholerae non-O1/non-O139 in Germany reveals carbapenemase-producing Vibrio cholerae in coastal waters. Front. Microbiol. 6:1179. doi: 10.3389/fmicb.2015.01179
Boer, S. I., Heinemeyer, E. A., Luden, K., Erler, R., Gerdts, G., Janssen, F., et al. (2013). Temporal and spatial distribution patterns of potentially pathogenic Vibrio spp. at recreational beaches of the German North Sea. Microb. Ecol. 65, 1052–1067. doi: 10.1007/s00248-013-0221-4
Bolger, A. M., Lohse, M., and Usadel, B. (2014). Trimmomatic: A flexible trimmer for Illumina sequence data. Bioinformatics 30, 2114–2120. doi: 10.1093/bioinformatics/btu170
Borgeaud, S., Metzger, L. C., Scrignari, T., and Blokesch, M. (2015). The type VI secretion system of Vibrio cholerae fosters horizontal gene transfer. Science 347, 63–67. doi: 10.1126/science.1260064
Brehm, T. T., Berneking, L., Martins, M. S., Dupke, S., Jacob, D., Drechsel, O., et al. (2021). Heatwave-associated Vibrio infections in Germany, 2018 and 2019. Euro. Surveill. 26:2002041. doi: 10.2807/1560-7917.ES.2021.26.41.2002041
Brooks, T. M., Unterweger, D., Bachmann, V., Kostiuk, B., and Pukatzki, S. (2013). Lytic activity of the Vibrio cholerae type VI secretion toxin VgrG-3 is inhibited by the antitoxin TsaB. J. Biol. Chem. 288, 7618–7625. doi: 10.1074/jbc.M112.436725
Ceccarelli, D., Chen, A., Hasan, N. A., Rashed, S. M., Huq, A., and Colwell, R. R. (2015). Non-O1/non-O139 Vibrio cholerae carrying multiple virulence factors and V. cholerae O1 in the Chesapeake Bay, Maryland. Appl. Environ. Microbiol. 81, 1909–1918. doi: 10.1128/AEM.03540-14
Chatterjee, S., Ghosh, K., Raychoudhuri, A., Chowdhury, G., Bhattacharya, M. K., and Mukhopadhyay, A. K. (2009). Incidence, virulence factors, and clonality among clinical strains of non-O1, non-O139 Vibrio cholerae isolates from hospitalized diarrheal patients in Kolkata, India. J. Clin. Microbiol. 47, 1087–1095. doi: 10.1128/JCM.02026-08
Chen, L., Zheng, D., Liu, B., Yang, J., and Jin, Q. (2016). VFDB 2016: Hierarchical and refined dataset for big data analysis–10 years on. Nucleic Acids Res. 44, D694–D697. doi: 10.1093/nar/gkv1239
Chen, Y. S., Tu, Y. H., Chen, B. H., Liu, Y. Y., Hong, Y. P., Teng, R. H., et al. (2022). cgMLST@Taiwan: A web service platform for Vibrio cholerae cgMLST profiling and global strain tracking. J. Microbiol. Immunol. Infect. 55, 102–106. doi: 10.1016/j.jmii.2020.12.007
Chowdhury, G., Joshi, S., Bhattacharya, S., Sekar, U., Birajdar, B., Bhattacharyya, A., et al. (2016). Extraintestinal infections caused by non-toxigenic Vibrio cholerae non-O1/non-O139. Front. Microbiol. 7:144. doi: 10.3389/fmicb.2016.00144
Dalsgaard, A., Forslund, A., Sandvang, D., Arntzen, L., and Keddy, K. (2001). Vibrio cholerae O1 outbreak isolates in Mozambique and South Africa in 1998 are multiple-drug resistant, contain the SXT element and the aadA2 gene located on class 1 integrons. J. Antimicrob. Chemother. 48, 827–838. doi: 10.1093/jac/48.6.827
Dupke, S., Akinsinde, K. A., Grunow, R., Iwalokun, B. A., Olukoya, D. K., Oluwadun, A., et al. (2016). Characterization of Vibrio cholerae strains isolated from the Nigerian Cholera outbreak in 2010. J. Clin. Microbiol. 54, 2618–2621. doi: 10.1128/JCM.01467-16
Dutta, D., Chowdhury, G., Pazhani, G. P., Guin, S., Dutta, S., Ghosh, S., et al. (2013). Vibrio cholerae non-O1, non-O139 serogroups and cholera-like diarrhea, Kolkata, India. Emerg. Infect. Dis. 19, 464–467. doi: 10.3201/eid1903.121156
Dziejman, M., Serruto, D., Tam, V. C., Sturtevant, D., Diraphat, P., Faruque, S. M., et al. (2005). Genomic characterization of non-O1, non-O139 Vibrio cholerae reveals genes for a type III secretion system. Proc. Natl. Acad. Sci. U.S.A. 102, 3465–3470. doi: 10.1073/pnas.0409918102
Erler, R., Wichels, A., Heinemeyer, E. A., Hauk, G., Hippelein, M., Reyes, N. T., et al. (2015). VibrioBase: A MALDI-TOF MS database for fast identification of Vibrio spp. that are potentially pathogenic in humans. Syst. Appl. Microbiol. 38, 16–25. doi: 10.1016/j.syapm.2014.10.009
Falagas, M. E., Kastoris, A. C., Kapaskelis, A. M., and Karageorgopoulos, D. E. (2010). Fosfomycin for the treatment of multidrug-resistant, including extended-spectrum beta-lactamase producing, Enterobacteriaceae infections: A systematic review. Lancet Infect. Dis. 10, 43–50. doi: 10.1016/S1473-3099(09)70325-1
Feghali, R., and Adib, S. M. (2011). Two cases of Vibrio cholerae non-O1/non-O139 septicaemia with favourable outcome in Lebanon. East Mediterr. Health J. 17, 722–724.
Feldgarden, M., Brover, V., Haft, D. H., Prasad, A. B., Slotta, D. J., Tolstoy, I., et al. (2019). Validating the AMRFinder tool and resistance gene database by using antimicrobial resistance genotype-phenotype correlations in a collection of isolates. Antimicrob. Agents Chemother. 63, e00483–19. doi: 10.1128/AAC.00483-19
Frentrup, M., Zhou, Z., Steglich, M., Meier-Kolthoff, J. P., Goker, M., Riedel, T., et al. (2020). A publicly accessible database for Clostridioides difficile genome sequences supports tracing of transmission chains and epidemics. Microb. Genom. 6:mgen000410. doi: 10.1099/mgen.0.000410
Gallut, J., and Quiniou, J. (1970). Interactions of classical Vibrio cholerae, V. cholerae biotype E1 Tor, and vibrions NAG. Bull. World Health Organ. 42, 464–466.
Hammerl, J. A., Jackel, C., Bortolaia, V., Schwartz, K., Bier, N., Hendriksen, R. S., et al. (2017). Carbapenemase VCC-1-producing Vibrio cholerae in coastal waters of Germany. Emerg. Infect Dis. 23, 1735–1737. doi: 10.3201/eid2310.161625
Hirk, S., Huhulescu, S., Allerberger, F., Lepuschitz, S., Rehak, S., Weil, S., et al. (2016). Necrotizing fasciitis due to Vibrio cholerae non-O1/non-O139 after exposure to Austrian bathing sites. Wien Klin Wochenschr. 128, 141–145. doi: 10.1007/s00508-015-0944-y
Hirsch, N., Kappe, E., Gangl, A., Schwartz, K., Mayer-Scholl, A., Hammerl, J. A., et al. (2020). Phenotypic and genotypic properties of Vibrio cholerae non-O1, non-O139 isolates recovered from domestic ducks in Germany. Microorganisms 8:1104. doi: 10.3390/microorganisms8081104
Ishikawa, T., Rompikuntal, P. K., Lindmark, B., Milton, D. L., and Wai, S. N. (2009). Quorum sensing regulation of the two hcp alleles in Vibrio cholerae O1 strains. PLoS One 4:e6734. doi: 10.1371/journal.pone.0006734
Jackel, C., Hammerl, J. A., Arslan, H. H., Gollner, C., Vom Ort, N., Taureck, K., et al. (2020). Phenotypic and genotypic characterization of veterinary Vibrio cincinnatiensis isolates. Microorganisms 8:739. doi: 10.3390/microorganisms8050739
Jagadeeshan, S., Kumar, P., Abraham, W. P., and Thomas, S. (2009). Multiresistant Vibrio cholerae non-O1/non-O139 from waters in South India: Resistance patterns and virulence-associated gene profiles. J. Basic Microbiol. 49, 538–544. doi: 10.1002/jobm.200900085
Jolley, K. A., and Maiden, M. C. (2010). BIGSdb: Scalable analysis of bacterial genome variation at the population level. BMC Bioinform. 11:595. doi: 10.1186/1471-2105-11-595
Kadkhoda, K., Adam, H., Gilmour, M. W., and Hammond, G. W. (2012). Nontoxigenic Vibrio cholerae septicemia in an immunocompromised patient. Case Rep. Infect. Dis. 2012:698746. doi: 10.1155/2012/698746
Kanoktippornchai, B., Chomvarin, C., Hahnvajanawong, C., and Nutrawong, T. (2014). Role of hlya-positive Vibrio cholerae non-O1/non-O139 on apoptosis and cytotoxicity in a Chinese hamster ovary cell line. Southeast Asian J. Trop. Med. Public Health 45, 1365–1375.
Kirschner, A. K., Schlesinger, J., Farnleitner, A. H., Hornek, R., Süss, B., Golda, B., et al. (2008). Rapid growth of planktonic Vibrio cholerae non-O1/non-O139 strains in a large alkaline lake in Austria: Dependence on temperature and dissolved organic carbon quality. Appl. Environ. Microbiol. 74, 2004–2015. doi: 10.1128/aem.01739-07
Kokashvili, T., Whitehouse, C. A., Tskhvediani, A., Grim, C. J., Elbakidze, T., Mitaishvili, N., et al. (2015). Occurrence and diversity of clinically important Vibrio species in the aquatic environment of Georgia. Front. Public Health 3:232. doi: 10.3389/fpubh.2015.00232
Krebs, S. J., and Taylor, R. K. (2011). Protection and attachment of Vibrio cholerae mediated by the toxin-coregulated pilus in the infant mouse model. J. Bacteriol. 193, 5260–5270. doi: 10.1128/JB.00378-11
Lan, N. P., Nga, T. V., Yen, N. T., Dung le, T., Tuyen, H. T., and Campbell, J. I, et al. (2014). Two cases of bacteriemia caused by nontoxigenic, non-O1, non-O139 Vibrio cholerae isolates in Ho Chi Minh City, Vietnam. J. Clin. Microbiol. 52, 3819–3821. doi: 10.1128/JCM.01915-14
Lee, B. C., Choi, S. H., and Kim, T. S. (2008). Vibrio vulnificus RTX toxin plays an important role in the apoptotic death of human intestinal epithelial cells exposed to Vibrio vulnificus. Microbes Infect. 10, 1504–1513. doi: 10.1016/j.micinf.2008.09.006
Lepuschitz, S., Baron, S., Larvor, E., Granier, S. A., Pretzer, C., Mach, R. L., et al. (2019). Phenotypic and genotypic antimicrobial resistance traits of Vibrio cholerae non-O1/non-O139 isolated from a large Austrian lake frequently associated with cases of human infection. Front. Microbiol. 10:2600. doi: 10.3389/fmicb.2019.02600
Liang, K. Y. H., Orata, F. D., Islam, M. T., Nasreen, T., Alam, M., Tarr, C. L., et al. (2020). A Vibrio cholerae core genome multilocus sequence typing scheme to facilitate the epidemiological study of cholera. J. Bacteriol. 202, e00086–20. doi: 10.1128/JB.00086-20
Lin, H. V., Massam-Wu, T., Lin, C. P., Wang, Y. A., Shen, Y. C., Lu, W. J., et al. (2017). The Vibrio cholerae var regulon encodes a metallo-beta-lactamase and an antibiotic efflux pump, which are regulated by VarR, a LysR-type transcription factor. PLoS One 12:e0184255. doi: 10.1371/journal.pone.0184255
Linhartova, I., Bumba, L., Masin, J., Basler, M., Osicka, R., Kamanova, J., et al. (2010). RTX proteins: A highly diverse family secreted by a common mechanism. FEMS Microbiol. Rev. 34, 1076–1112. doi: 10.1111/j.1574-6976.2010.00231.x
Lu, B., Zhou, H., Li, D., Li, F., Zhu, F., Cui, Y., et al. (2014). The first case of bacteraemia due to non-O1/non-O139 Vibrio cholerae in a type 2 diabetes mellitus patient in mainland China. Int. J. Infect. Dis. 25, 116–118. doi: 10.1016/j.ijid.2014.04.015
Ma, A. T., and Mekalanos, J. J. (2010). In vivo actin cross-linking induced by Vibrio cholerae type VI secretion system is associated with intestinal inflammation. Proc. Natl. Acad. Sci. U.S.A. 107, 4365–4370. doi: 10.1073/pnas.0915156107
MacIntyre, D. L., Miyata, S. T., Kitaoka, M., and Pukatzki, S. (2010). The Vibrio cholerae type VI secretion system displays antimicrobial properties. Proc Natl Acad Sci. U.S.A. 107, 19520–19524. doi: 10.1073/pnas.1012931107
Magiorakos, A. P., Srinivasan, A., Carey, R. B., Carmeli, Y., Falagas, M. E., Giske, C. G., et al. (2012). Multidrug-resistant, extensively drug-resistant and pandrug-resistant bacteria: An international expert proposal for interim standard definitions for acquired resistance. Clin. Microbiol. Infect. 18, 268–281. doi: 10.1111/j.1469-0691.2011.03570.x
Mangat, C. S., Boyd, D., Janecko, N., Martz, S. L., Desruisseau, A., Carpenter, M., et al. (2016). Characterization of VCC-1, a novel ambler class a carbapenemase from Vibrio cholerae isolated from imported retail shrimp sold in Canada. Antimicrob. Agents Chemother. 60, 1819–1825. doi: 10.1128/AAC.02812-15
Marek, A., Inkster, T., Anderson, E., Jenkins, C., Boyd, J., Kerr, S., et al. (2013). Non-toxigenic Vibrio cholerae bacteraemia: Case report and review of the literature. J. Med. Microbiol. 62, 1357–1359. doi: 10.1099/jmm.0.060400-0
Markus Meier, H. E., Dieterich, C., and Gröger, M. (2021). Natural variability is a large source of uncertainty in future projections of hypoxia in the Baltic Sea. Commun. Earth Environ. 2:50. doi: 10.1038/s43247-021-00115-9
Marinello, S., Marini, G., Parisi, G., Gottardello, L., Rossi, L., Besutti, V., et al. (2017). Vibrio cholerae non-O1, non-O139 bacteraemia associated with pneumonia, Italy 2016. Infection 45, 237–240. doi: 10.1007/s15010-016-0961-4
Melano, R., Petroni, A., Garutti, A., Saka, H. A., Mange, L., Pasteran, F., et al. (2002). New carbenicillin-hydrolyzing beta-lactamase (CARB-7) from Vibrio cholerae non-O1, non-O139 strains encoded by the VCR region of the V. cholerae genome. Antimicrob. Agents Chemother. 46, 2162–2168. doi: 10.1128/AAC.46.7.2162-2168.2002
Novais, R. C., Coelho, A., Salles, C. A., and Vicente, A. C. (1999). Toxin-co-regulated pilus cluster in non-O1, non-toxigenic Vibrio cholerae: Evidence of a third allele of pilin gene. FEMS Microbiol. Lett. 171, 49–55. doi: 10.1111/j.1574-6968.1999.tb13411.x
Ottaviani, D., Medici, L., Talevi, G., Napoleoni, M., Serratore, P., Zavatta, E., et al. (2018). Molecular characterization and drug susceptibility of non-O1/O139 V. cholerae strains of seafood, environmental and clinical origin, Italy. Food Microbiol. 72, 82–88. doi: 10.1016/j.fm.2017.11.011
Pang, R., Li, Y., Chen, M., Zeng, H., Lei, T., Zhang, J., et al. (2020). A database for risk assessment and comparative genomic analysis of foodborne Vibrio parahaemolyticus in China. Sci. Data 7:321. doi: 10.1038/s41597-020-00671-3
Pearce, M. E., Alikhan, N. F., Dallman, T. J., Zhou, Z., Grant, K., and Maiden, M. C. J. (2018). Comparative analysis of core genome MLST and SNP typing within a European Salmonella serovar Enteritidis outbreak. Int. J. Food Microbiol. 274, 1–11. doi: 10.1016/j.ijfoodmicro.2018.02.023
Petroni, A., Melano, R. G., Saka, H. A., Garutti, A., Mange, L., Pasteran, F., et al. (2004). CARB-9, a carbenicillinase encoded in the VCR region of Vibrio cholerae non-O1, non-O139 belongs to a family of cassette-encoded beta-lactamases. Antimicrob. Agents Chemother. 48, 4042–4046. doi: 10.1128/AAC.48.10.4042-4046.2004
Rahmani, F., Fooladi, A. A., Marashi, S. M., and Nourani, M. R. (2012). Drug resistance in Vibrio cholerae strains isolated from clinical specimens. Acta Microbiol. Immunol. Hung. 59, 77–84. doi: 10.1556/AMicr.59.2012.1.8
Rajpara, N., Nair, M., Chowdhury, G., Mukhopadhyay, A. K., Ramamurthy, T., Niyogi, S. K., et al. (2018). Molecular analysis of multidrug resistance in clinical isolates of Shigella spp. from 2001-2010 in Kolkata, India: Role of integrons, plasmids, and topoisomerase mutations. Infect. Drug Resist. 11, 87–102. doi: 10.2147/IDR.S148726
Rajpara, N., Patel, A., Tiwari, N., Bahuguna, J., Antony, A., Choudhury, I., et al. (2009). Mechanism of drug resistance in a clinical isolate of Vibrio fluvialis: Involvement of multiple plasmids and integrons. Int. J. Antimicrob. Agents 34, 220–225. doi: 10.1016/j.ijantimicag.2009.03.020
Rehm, C., Lippert, K., Indra, A., Kolarevic, S., Kracun-Kolarevic, M., Leopold, M., et al. (2023). First report on the occurrence of Vibrio cholerae nonO1/nonO139 in natural and artificial lakes and ponds in Serbia: Evidence for a long-distance transfer of strains and the presence of Vibrio paracholerae. Environ. Microbiol. Rep. 15, 142–152. doi: 10.1111/1758-2229.13136
Reparaz, J., and Fernandez, C. (1977). Sensitivity of Vibrio spp. to fosfomycin. Chemotherapy 23, 58–62. doi: 10.1159/000222027
Satchell, K. J. (2007). MARTX, multifunctional autoprocessing repeats-in-toxin toxins. Infect. Immun. 75, 5079–5084. doi: 10.1128/IAI.00525-07
Schirmeister, F., Dieckmann, R., Bechlars, S., Bier, N., Faruque, S. M., and Strauch, E. (2014). Genetic and phenotypic analysis of Vibrio cholerae non-O1, non-O139 isolated from German and Austrian patients. Eur. J. Clin. Microbiol. Infect. Dis. 33, 767–778. doi: 10.1007/s10096-013-2011-9
Schmithausen, R., Döhla, M., Färber, H., Voigt, A., Sib, E., Exn, M., et al. (2019). Badegewässer-screening untersuchung ausgewählter EG-badegewässer in Nordrhein-Westfalen auf antibiotikaresistente bakterien und antibiotikarückstände. Landesamt für Natur, Umwelt und Verbraucherschutz Nordrhein-Westfalen- Fachbericht 93. Landesamt für Natur, Umwelt und Verbraucherschutz Nordrhein-Westfalen: Recklinghausen.
Schwartz, K., Hammerl, J. A., Gollner, C., and Strauch, E. (2019). Environmental and clinical strains of Vibrio cholerae non-O1, non-O139 from Germany possess similar virulence gene profiles. Front. Microbiol. 10:733. doi: 10.3389/fmicb.2019.00733
Shan, X., Fu, J., Li, X., Peng, X., and Chen, L. (2022). Comparative proteomics and secretomics revealed virulence, and coresistance-related factors in non O1/O139 Vibrio cholerae recovered from 16 species of consumable aquatic animals. J. Proteom. 251:104408. doi: 10.1016/j.jprot.2021.104408
Stokes, H. W., and Hall, R. M. (1989). A novel family of potentially mobile DNA elements encoding site-specific gene-integration functions: Integrons. Mol. Microbiol. 3, 1669–1683. doi: 10.1111/j.1365-2958.1989.tb00153.x
Sunderkotter, C., Becker, K., Eckmann, C., Graninger, W., Kujath, P., and Schofer, H. (2019). S2k guidelines for skin and soft tissue infections excerpts from the S2k guidelines for “calculated initial parenteral treatment of bacterial infections in adults - update 2018”. J. Dtsch Dermatol. Ges. 17, 345–369. doi: 10.1111/ddg.13790
Theophilo, G. N., Rodrigues Ddos, P., Leal, N. C., and Hofer, E. (2006). Distribution of virulence markers in clinical and environmental Vibrio cholerae non-O1/non-O139 strains isolated in Brazil from 1991 to 2000. Rev. Inst. Med. Trop. Sao Paulo 48, 65–70. doi: 10.1590/s0036-46652006000200002
Torp-Pedersen, T., Nielsen, X. C., Olsen, K. E., and Barfod, T. S. (2012). Intracerebral abscess after infection with non-toxigenic Vibrio cholerae. Ugeskr. Laeger. 174, 498–499.
Trivedi, U. H., Cezard, T., Bridgett, S., Montazam, A., Nichols, J., Blaxter, M., et al. (2014). Quality control of next-generation sequencing data without a reference. Front. Genet. 5:111. doi: 10.3389/fgene.2014.00111
Verma, J., Bag, S., Saha, B., Kumar, P., Ghosh, T. S., Dayal, M., et al. (2019). Genomic plasticity associated with antimicrobial resistance in Vibrio cholerae. Proc. Natl. Acad. Sci. U.S.A. 116, 6226–6231. doi: 10.1073/pnas.1900141116
Verner-Jeffreys, D. W., Baker-Austin, C., Pond, M. J., Rimmer, G. S., Kerr, R., Stone, D., et al. (2012). Zoonotic disease pathogens in fish used for pedicure. Emerg. Infect. Dis. 18, 1006–1008. doi: 10.3201/eid1806.111782
Vezzulli, L., Grande, C., Reid, P. C., Helaouet, P., Edwards, M., Hofle, M. G., et al. (2016). Climate influence on Vibrio and associated human diseases during the past half-century in the coastal North Atlantic. Proc Natl Acad Sci. U.S.A. 113, E5062–E5071. doi: 10.1073/pnas.1609157113
Vilela, F. P., and Falcão, J. P. (2021). Analysis of the antimicrobial resistance gene frequency in whole-genome sequenced Vibrio from Latin American countries. J. Med. Microbiol. 70. doi: 10.1099/jmm.0.001428
Wang, H., Yang, C., Sun, Z., Zheng, W., Zhang, W., Yu, H., et al. (2020). Genomic epidemiology of Vibrio cholerae reveals the regional and global spread of two epidemic non-toxigenic lineages. PLoS Negl. Trop. Dis. 14:e0008046. doi: 10.1371/journal.pntd.0008046
Wickham, H. (2011). ggplot2. Wiley Interdiscip. Rev. Comput. Stat. 3, 180–185. doi: 10.1002/wics.147
Xu, F., Gonzalez-Escalona, N., Haendiges, J., Myers, R. A., Ferguson, J., Stiles, T., et al. (2017). Sequence type 631 Vibrio parahaemolyticus, an emerging foodborne pathogen in North America. J. Clin. Microbiol. 55, 645–648. doi: 10.1128/JCM.02162-16
Ye, J., Ma, Y., Liu, Q., Zhao, D. L., Wang, Q. Y., and Zhang, Y. X. (2008). Regulation of Vibrio alginolyticus virulence by the LuxS quorum-sensing system. J. Fish Dis. 31, 161–169. doi: 10.1111/j.1365-2761.2007.00882.x
Keywords: Vibrio cholerae non-O1/non-O139, clinical and environmental isolate, whole genome sequencing, antimicrobial resistance genes, virulence genes, core genome multilocus sequence typing (cgMLST)
Citation: Schmidt K, Scholz HC, Appelt S, Michel J, Jacob D and Dupke S (2023) Virulence and resistance patterns of Vibrio cholerae non-O1/non-O139 acquired in Germany and other European countries. Front. Microbiol. 14:1282135. doi: 10.3389/fmicb.2023.1282135
Received: 23 August 2023; Accepted: 30 October 2023;
Published: 22 November 2023.
Edited by:
Jeffrey H. Withey, Wayne State University, United StatesReviewed by:
Dhrubajyoti Nag, City College of New York (CUNY), United StatesGoutam Chowdhury, National Institute of Cholera and Enteric Diseases, India
Copyright © 2023 Schmidt, Scholz, Appelt, Michel, Jacob and Dupke. This is an open-access article distributed under the terms of the Creative Commons Attribution License (CC BY). The use, distribution or reproduction in other forums is permitted, provided the original author(s) and the copyright owner(s) are credited and that the original publication in this journal is cited, in accordance with accepted academic practice. No use, distribution or reproduction is permitted which does not comply with these terms.
*Correspondence: Susann Dupke, ZHVwa2VzQHJraS5kZQ==