- Molecular Biology Laboratory, School of Biotechnology, Shri Mata Vaishno Devi University, Katra, Jammu and Kashmir, India
In the ever-evolving realm of agriculture, the convoluted interaction between plants and microorganisms have assumed paramount significance. Fungal endophytes, once perceived as mere bystanders within plant tissues, have now emerged as dynamic defenders of plant health. This comprehensive review delves into the captivating world of fungal endophytes and their multifaceted biocontrol mechanisms. Exploring their unique ability to coexist with their plant hosts, fungal endophytes have unlocked a treasure trove of biological weaponry to fend off pathogens and enhance plant resilience. From the synthesis of bioactive secondary metabolites to intricate signaling pathways these silent allies are masters of biological warfare. The world of fungal endophytes is quite fascinating as they engage in a delicate dance with the plant immune system, orchestrating a symphony of defense that challenges traditional notions of plant-pathogen interactions. The journey through the various mechanisms employed by these enigmatic endophytes to combat diseases, will lead to revelational understanding of sustainable agriculture. The review delves into cutting-edge research and promising prospects, shedding light on how fungal endophytes hold the key to biocontrol and the reduction of chemical inputs in agriculture. Their ecological significance, potential for bioprospecting and avenues for future research are also explored. This exploration of the biocontrol mechanisms of fungal endophytes promise not only to enrich our comprehension of plant-microbe relationships but also, to shape the future of sustainable and ecofriendly agricultural practices. In this intricate web of life, fungal endophytes are indeed the unsung heroes, silently guarding our crops and illuminating a path towards a greener, healthier tomorrow.
1 Introduction
In the realm of agriculture and plant health management, the quest for sustainable and environmentally friendly alternatives to conventional methods has never been more pressing. The exponential growth of the global population, along with the ever-escalating demands for food production, places immense pressure on our agricultural systems. In this view, the health of cultivated plants is crucial for many economic sectors because plants not only offer food for the inhabitants, but also critical items like wood, textiles, medicines, and bioenergy, among others. Plant diseases are to blame for production losses that are both large in terms of quantity and quality, costing businesses a lot of money, and occasionally having disastrous social effects (Savary et al., 2019). On a global scale, plant illnesses can result in losses of up to 16%, and studies have already shown that pathogens and, more precisely, conducted cultivations are the targets of losses (Savary et al., 2019). Without a doubt, diseases have the capacity to cause losses, and the severity of those losses may vary depending on the climate, microbes, and the aggressiveness of the disease-causing agent. For ages, numerous methods have been exploited to increase food production, many of which are unsustainable since they pose risks to the environment (Sahu and Mishra, 2021). According to this assumption, reducing these eco-threats and investigating potential endophytic microbes will aid in achieving an ecosystem that is stable and will enable the cultivation of pathogen-free plants for increased crop output. In this context, endophytic fungi have emerged as an intriguing and promising class of microorganisms that hold the potential to revolutionize modern agriculture (Sharma and Singh, 2021). In nature, both plants and microbes live in relationships among themselves, which in turn can affect the overall growth and development of plants. Endophytic microbes are an intriguing collection of organisms that are linked to diverse parts and tissues of plants. The association of these microbes with plants can be facultative or obligate and causes no harm to their host. On the other hand, these endophytes exhibit intricate relationships with their host plants, including antagonism and mutualism (Parker, 1995). One such widely acknowledged interaction of mutualism or symbiosis involves the interaction of medicinal plants and their associated endophytes. These endophytes are known to produce certain secondary metabolites of immense pharmacological importance (Elgorban et al., 2019). Fungal endophytes have gained a lot of attention from taxonomists, mycologists, ecologists, chemists, and evolutionary biologists over the last three decades (Saikkonen, 2007). They actually represent a diverse array of fungi that establish mutualistic relationships within the tissues of plants, all while evading the conventional signs of diseases or distress that are typically associated with pathogenic fungi. This intriguing class of microorganisms has remained hidden allies of the plant kingdom for centuries performing their roles quietly and unobtrusively. However, recent decades have witnessed a surge of research interest in these cryptic microorganisms, revealing their profound influence on plant growth, development, and resistance to abiotic and biotic stresses. The remarkable attribute of these fungi lies in their ability to form mutualistic associations with a wide range of plant species without causing overt symptoms of disease, setting them apart from their pathogenic counterparts (Saikkonen, 2007). Approximately 5% of the estimated million fungal species on earth have been described, which is a very tiny percentage (Panda and Sujogya., 2013). They are being studied due to their ability to boost plant health; for example, in Musa spp., Piriformospora indica has been found to induce resistance against Fusarium oxysporium f. sp. cubense (Cheng et al., 2020). These endophytes can affect the host plant’s biochemistry, physiology, distribution, and ecology, and their persistence has been known for the past 100 years. The effect of the association of endophytic fungi, on plant growth and immunity against pathogens greatly depends on the site of the colonization and the secondary metabolites produced by the endophytes (Yu et al., 2018). Several studies have shown that the presence of microbes in the plant endosphere affects the plant’s response to environmental stimuli and can regulate plant diseases (Zhang et al., 2020). Reports have shown that endophytic fungi have the potential to maintain plant health and can be engineered to integrate into crop breeding (Liu et al., 2018). An interesting fact about Rhizospheric microbes/fungi living in the proximity of root endosphere is that they can modify their morphology rapidly and can colonize the plant tissues, thus becoming endophytic microbe/ fungi (Abedinzadeh et al., 2019). It is interesting to note that data has demonstrated how endophytic fungi can colonize and infiltrate internal plant tissue (endosphere) from their external root environment (rhizosphere), establishing endophytic microbial communities (Vishwas, 2011). When compared to non-symbiotic plants, endophytes can strongly and quickly stimulate and activate the host plant’s stress response (Wu et al., 2018). As already stated above, plant pathogens have always been the first threat to food security in our world, and in most cases, the available tools were insufficient to effectively manage them. Phytophthora infestans, the first ever plant pathogen reported in tomato and other related cultivars, is still responsible for their reduced production. A lot of different hosts and most strains make it harder to use non-host crops and resistant cultivars. The use of chemically synthesized. Pesticides is not always that effective or applicable, both in terms of cost and method of application. On the other hand, security is expected not only in terms of food production but also in quality and overall impact on the environment (Lechenet et al., 2017). Plants can support a microbial community in the rhizosphere and even recruit some in adverse conditions. A fresh perspective on biological control, particularly through endophytes, offers innovative biotechnological methods of managing plant diseases and a unique viewpoint on the relationship between microbes and plants (Tena, 2018). As biocontrol agents, endophytic fungi, during their entire life cycle, protect the host plants from infections as they easily adjust to adverse environmental conditions (Xie et al., 2018). Interaction between endophytes and their host plants improves plant growth and protects the plant from harmful effects, e.g., by helping in developing resistance against pathogens, aiding in resistance mechanisms like phytoremediation, increasing the crop yield, etc. (Saikkonen et al., 2004). In this interaction, both endophytes and hosts benefit as the plant provides protection, nutrition, and shelter to them. Endophytes, on the other hand, assist their hosts by stimulating their growth, development, and adaptation (Killham, 1994). When the plant gets attacked by a disease pathogen, the host defense system gets activated, and protection against disease occurs mainly by minimizing the level of infection as well as masking and reducing the pathogen’s growth (Jia et al., 2016). Endophytes have helped their host plants by enhancing their growth and developing resistance against pathogens in the course of co-existence and evolution. This results in the establishment of special interactions such as mutualism, neutralism, and antagonism between the host plant and its endophytes (Jia et al., 2016). Due to their efficacy as biocontrol agents against several plant infections, the screening of endophytic fungi have recently increased, as reported (Abaya et al., 2021). Endophytic fungi that live in different plant compartments generally encourage plant growth in a variety of direct and indirect ways (Adeleke et al., 2022). In the direct mechanism, endophytes control different plant hormones such as auxins and cytokinins and improve the availability of soil nutrients through nitrogen fixation, siderophore production, phosphorus, and iron solubilization, whereas in the indirect mechanism, endophytes produce various volatile compounds like hydrogen cyanide, enzymes, and antibiotics that cease pathogen activity and promote systemic resistance in plants (Selim et al., 2018). It has been suggested that endophytic ecological occupation and phytoalexin synthesis caused by fungal endophytes may be the primary mechanisms by which plants protect themselves from diseases. This review paper embarks on an exploration of the multifaceted world of endophytic fungi, focusing on their extraordinary biocontrol mechanisms and their potential applications in sustainable agriculture. As the global community seeks innovative solutions to address challenges such as crop diseases, pests, and the adverse effects of climate change, endophytic fungi stand as nature’s hidden allies, offering a treasure trove of mechanisms that can be harnessed to combat these pressing issues. As one traverse the complex landscape of endophytic fungi and their biocontrol mechanisms, it becomes evident that these enigmatic organisms hold the key to a sustainable and resilient agricultural future. This review aims to spark more research and new ideas by shedding light on their many different strategies and the environmental factors that make them successful. This will help us learn more about these hidden allies and how important they are to global food security (Figure 1).
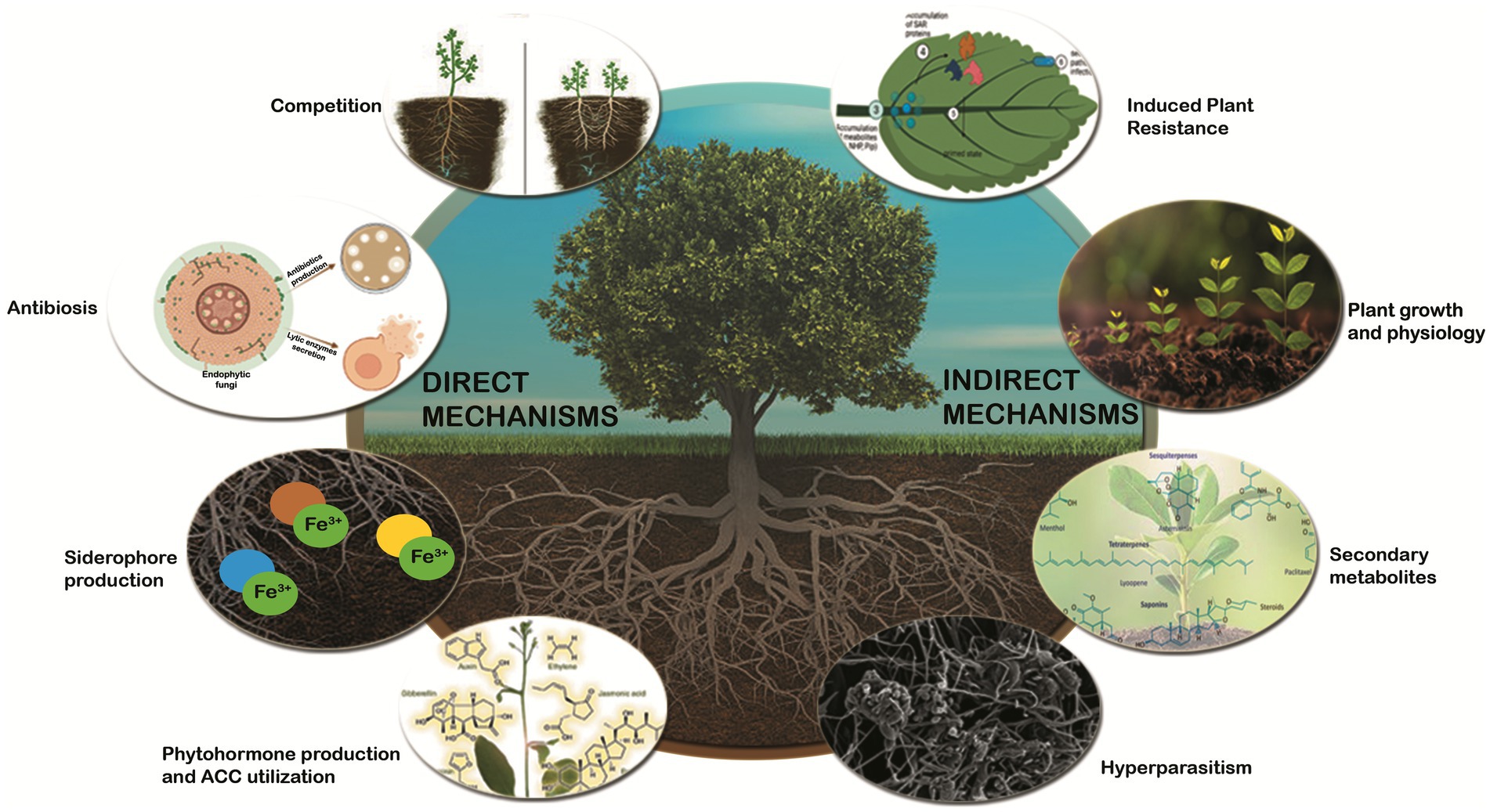
Figure 1. Schematic representation of defense mechanisms used by endophytic fungi for plant protection against phytopathogens.
2 Direct mechanism against plant pathogens
Endophytes have been the subject of recent research, which has shown that they can improve host defense against diseases and lessen the ecological harm that pathogenic microorganisms can cause (Arnold et al., 2003). Most of these investigations involved the assessment of the survival rate of plants in the presence and absence of fungal endophytes or in vitro coculture of fungal endophytes and phytopathogens. Although some studies suggest that endophytes may reduce the effects of plant-pathogen damage, the current understanding of the precise regulation of endophytes, pathogens, and plants is still in the beginning stages (Mejía et al., 2008). The direct mechanism employed by endophytes for the protection of plants against phytopathogens involves direct antagonistic measures such as antibiosis, competitive exclusion of phytopathogens, parasitism and elucidation of pathogen virulence (Köhl et al., 2019). Endophytes can exert antibiosis by secreting allelochemicals like bacteriocins, lipopeptides, biosurfactants, enzymes that break down cell walls, antibiotics, and volatile chemicals that interfere with phytopathogen metabolism and hence stop pathogen development (Raymaekers et al., 2020). Additionally, competition between the endophytes and the pathogens for nutrition and space also plays a role in the reduction of pathogenic infection in plants. Secretion of enzymes like pectinases and chitinases also inhibits the virulence of pathogen by interfering with factors responsible for pathogenicity in phytopathogens (Wang et al., 2022). However, the direct interactions between endophytic fungi and phytopathogens are complicated and species-specific in nature (Gouda et al., 2016).
2.1 Antibiotics production from endophytes
Studies throughout the decades have shown that endophytes are central to plant protection against pathogens (Barkodia et al., 2018). During the direct interaction between endophytes and pathogens, endophytes produce antibiotics to suppress the pathogens and protect the plant from the damage caused by pathogens (Fadiji and Babalola, 2020). Endophytes produce certain secondary metabolites that have antimicrobial properties like antifungal, antibacterial, etc. (Palanichamy et al., 2018). These antimicrobials have a strong inhibitory action on plant pathogens. A single class of endophytes is capable of producing a variety of bioactive agents (antibiotics) such as aromatic compounds, terpenoids, polypeptides, and terpenoids (Balla et al., 2021). Antimicrobials synthesized by some endophytic fungi are listed in Table 1.
2.2 Secretion of lytic enzymes
Lytic enzyme secretions play a significant role in plant protection from pathogens, particularly when it comes to endophytic microorganisms. Most microbes produce lytic enzymes that aid in the hydrolysis of polymers (Tripathi et al., 2008). Endophytic fungi create a symbiotic relationship with plants when they colonize the internal tissues of plants; this interaction is advantageous to both the plant and the fungus. As pathogens attempt to invade the plant, the fungal endophytes parasitize pathogenic hyphae in various ways, including twisting, coiling, perforation of the pathogenic hyphae, and secretion of cell wall-degrading enzymes leading to lysis and inhibition of pathogens (Waghunde et al., 2017). By degrading the cell walls of pathogens, the lytic enzymes limit the ability of the pathogens to invade and propagate within the plant, effectively enhancing the plant’s natural defense mechanisms. This mechanism not only provides immediate protection but also primes the plant’s immune system for induced systemic resistance (ISR), leading to long-lasting and robust defense responses against potential future pathogen attacks (Fadiji and Babalola, 2020; Trivedi et al., 2020). In lieu of chemical pesticides, the use of lytic enzymes produced by endophytic fungi offers a sustainable and environmentally benign method of plant protection, encouraging healthier and more resilient crops. Despite their involvement in mycoparasitism, these lytic enzymes also contribute to cell wall reformation and recycling during active fungal growth, as well as during aging and autolysis (Kumari and Srividhya, 2020). Endophytic fungi have a variety of enzymes such as hemicellulases,1,3-glucanases, chitinases and cellulases, that break down different types of materials (Haran et al., 1996). It has been reported that in Trichoderma species, a number of enzymes are directly involved in the breakdown of the cell wall of pathogenic fungi for the utilization of pathogenic fragments (Rajesh et al., 2016). Mycolytic enzymes like 1,3-glucanase or chitinase produced by Trichoderma asperellum have the potential to degrade the cell walls of phytopathogens. A respective increase in enzymatic activity and transcripts of 1,3, glucanase, and chitinase enzymes in cultures induced by pathogens has been observed in banana wilt affected plantations (Win et al., 2021). Biocontrol of root-knot nematodes by endophytic fungi showed elevated activity of genes encoding enzymes like glucanase and chitinase and downregulation of genes encoding antioxidant enzymes during the stimulation of the immune system of plants in response to pathogens (Molinari and Leonetti, 2019). Although enzymes lack the ability to act as antagonizing agents independently, but they augment the antagonistic activity of other agents and pathways when merged together. Similarly, the pectinase enzyme has also been reported to help reduce pathogenesis in plants (Fadiji and Babalola, 2020).
2.3 Production of phytohormones
Phytohormones of endophytic fungi play a crucial role in plant protection, enhancing defense responses and resistance to pathogens through complex interactions with the plant (Fadiji and Babalola, 2020). The context of plant protection, the secretion of phytohormones by endophytic fungi trigger a sequence of defense mechanisms in the host plant. In response to environmental cues, endophytic fungi that have formed a symbiotic relationship with plants release phytohormones such as auxins, cytokinins, gibberellins, indole acetic acid, and jasmonic acid that act as potent signaling molecules (Baron and Rigobelo, 2022). The two phytohormones, Jasmonic acid (JA) and Salicylic acid (SA) are important defense signaling molecules that regulate the defense responses of plants against disease-causing microbes (Shi et al., 2020). Systemic acquired resistance (SAR) and biotrophic pathogen defense are both impacted by Salicylic acid, which in turn is regulated by the activation of Pathogenesis-related (PR) genes. Localized programmed cell death, a fallout of the hypersensitive response caused by the two phytohormones, jasmonic acid and salicylic acid inhibits the spread of pathogens and protects against stress (Lahlali et al., 2022). These proteins and compounds act as an immediate response to pathogen attack, hindering the progress of invading pathogens and limiting their growth (Rashad et al., 2020). When pattern recognition receptors (PRRs) recognize the pathogens, Jasmonic acid (JA) and Salicylic acid (SA) have the potential to enhance the activity of the enzymes in the phenylpropane pathway of plants resulting in the production of phenolic compounds (such as flavonoids, lignin, coumarins, and tannins), triggering pathogen-associated molecular pattern (PAMP)-triggered immunity (PTI), a robust defense response in plants (Franco-Orozco et al., 2017). Jasmonic acid and ethylene (ET), mainly produced during induced systemic resistance (ISR), are considered important for defense against necrotrophic diseases and beneficial for interactions between plants and pathogens (Li et al., 2019). The phenomenon of JA/ET-dependent systemic resistance has been seen in various plant-associated microorganisms, including Trichoderma asperellum, Penicillium sp., and the endophyte Serendipita indica. But in other pathosystems, S. indica produced resistance without relying on the JA/ET route, while T. asperellum coupled with plants triggered resistance in an SA-dependent manner. This suggests that the roles of phytohormones and their potential interactions are complicated, and the use of a microorganism on the plant is expected to alter the entire hormone profile rather than solely altering the levels of individual hormones. Plant defense signaling pathways have been identified and compounds act as an immediate response to pathogen attack, hindering the progress of invading pathogens and limiting their growth (Rashad et al., 2020). When pattern recognition receptors (PRRs) recognize the pathogens, jasmonic acid (JA) and salicylic acid (SA) have the potential to enhance the activity of the enzymes in the phenylpropane pathway of plants, resulting in the production of phenolic compounds (such as flavonoids, lignin, coumarins, and tannins), triggering pathogen-associated molecular pattern (PAMP)-triggered immunity (PTI), a robust defense response in plants (Bhattacharya et al., 2010). Jasmonic acid and ethylene (ET), mainly produced during induced systemic resistance (ISR), are considered important for defense against necrotrophic diseases and beneficial for interactions between plants and pathogens (Ghozlan et al., 2020). Plant defense signaling pathways have been found to involve ethylene (ET) and abscisic acid (ABA), while auxin, gibberellic acid (GA), cytokinin (CK), brassinosteroids, and peptide hormones may also be involved (Li et al., 2019). Furthermore, ethylene and jasmonic acid also initiate the synthesis of secondary metabolites, including volatile organic compounds (VOCs) and phytoalexins. VOCs can serve as signaling molecules to attract beneficial microorganisms, such as predatory insects or microbes that feed on plant pests, to further enhance plant protection. On the other hand, phytoalexins are antimicrobial compounds that are directly involved in the inhibition of pathogen growth and proliferation within the tissues of plants (Latz et al., 2018). A study conductedal on the functional role of Trichoderma atroviride fungi in controlling the pathogenic activity of Fusarium verticillioides in maize showed the involvement of this endophytic fungi in the synthesis of phytohormones such as salicylic acid, abscisic acid (ABA) and jasmonic acid (Agostini et al., 2019). Similarly, Ren and Dai also found that the interaction between Gilmaniella spp. and endophytic fungi Atractylodes lancea led to increased production of jasmonic acid along -with the production of various volatile antimicrobial compounds such as eudesmol, atractylone, atractylodin and hinesol (Ren and Dai, 2012). Together, ethylene, Jasmonic acid, and salicylic acid create a hormonal response network that is coherent and keeps the plant’s defense mechanisms in place like production of compounds like ethylene and jasmonic acid in response to agents like Penicillium spp., Serendipita indica and Trichoderma asperellum to activate systemic resistance is crucial in avoiding host-pathogen inhabitation or activation of salicylic acid dependent systemic resistance pathway in plants inhabited by Trichoderma asperellum (Latz et al., 2018). These hormonal responses generated between the endophytic fungi and phytopathogens are extremely complicated and involves cross communication and multiple events between plants and the host endophytic fungi (Adeleke et al., 2022; Figure 2).
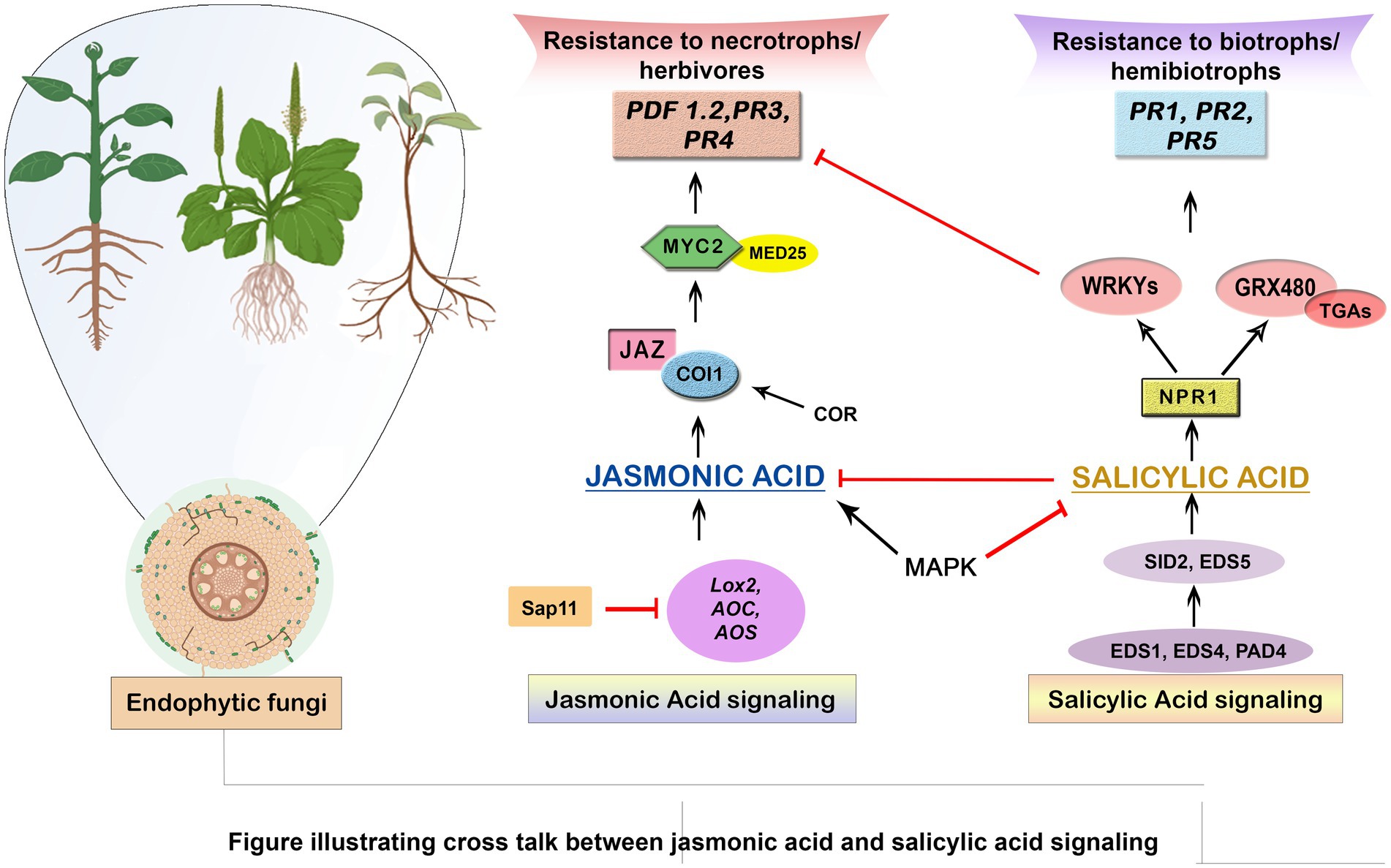
Figure 2. Illustrating cross talk between Jasmonic acid and Salicylic acid signaling. This schematic diagram depicts the intricate interplay between two key plant defense signaling pathways, Jasmonic acid (JA) and Salicylic acid (SA), in response to various biotic and abiotic stressors. The crosstalk between these signaling pathways plays a pivotal role in shaping a plant’s response to different types of threats.
Moreover, phytohormones influence the establishment of induced systemic resistance (ISR) in the plant. Induced Systemic Resistance entails getting the plant’s immune system ready to react more quickly to future pathogen attacks. This priming effect allows the plant to mount a quicker and more robust defense, effectively protecting it from potential pathogen threats (Baron and Rigobelo, 2022). Despite significant advances in research on signal transmission in induced resistance, there is still a lacuna in allocating roles to each hormone in signal transduction, particularly in complex systems. As a result, there is a need to strengthen a plant’s defense systems and use it as a biomarker to detect induced resistance (Latz et al., 2018). Overall, the secretion of phytohormones by endophytic fungi in plant protection is a finely tuned mechanism that involves a network of signaling pathways and defense responses. By modulating the plant’s hormonal balance, endophytic fungi contribute to a heightened state of preparedness against pathogen attacks, leading to improved plant health, resilience, and reduced reliance on chemical pesticides. Harnessing the potential of plant phytohormones from endophytic fungi offers a sustainable and eco-friendly approach to plant protection, benefiting both agricultural productivity and environmental health (Waghunde et al., 2017; Figure 3).
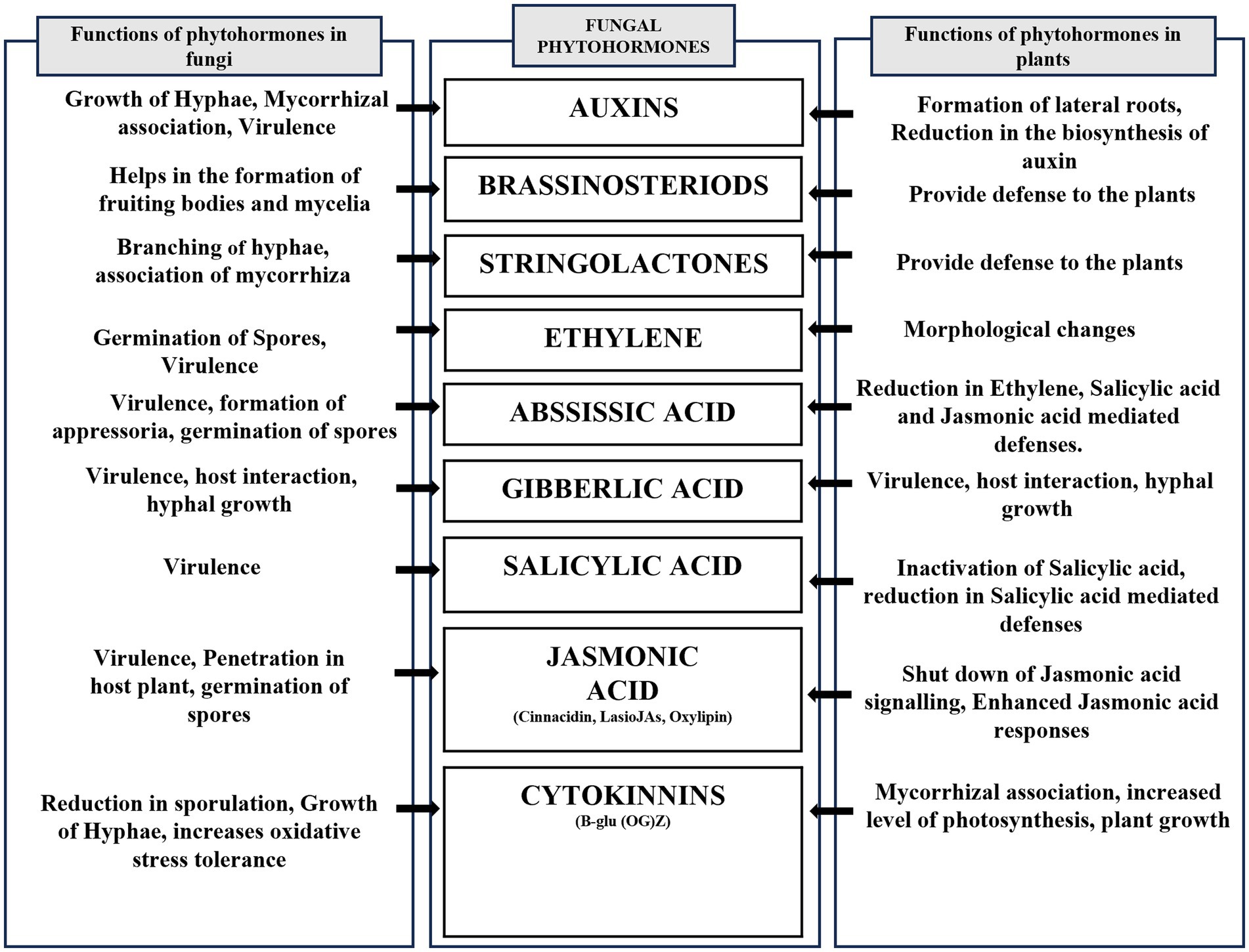
Figure 3. Illustrating phytohormones produced by endophytic fungi. This schematic diagram depicts different phytohormones produced by endophytic fungi and their role both in endophytic fungi and their host.
2.3.1 Volatile and non-volatile compounds of fungal endophytes
The metabolites produced by the endophytic fungi are primarily of two kinds: Volatile compounds and Non-Volatile Compounds (Santra and Banerjee, 2023). Volatile compounds can be defined as low molecular weight hydrophobic organic molecules with a high vapor pressure. Volatile organic compounds (VOC) are the name for the volatile metabolites that endophytic fungi produce. These volatile compounds can easily cross the cell membrane of the plant thus playing a vital role in soil ecosystem. The majority of VOC can be categorized into five different classes namely: Benzenoid compounds, Terpenoids, Amino acid derivatives, Fatty acid derivatives and Phenylpropanoids. These are mostly antibiotic in nature (Kaddes et al., 2019). The non-volatile metabolites produced by endophytic fungi comprise a wide range of chemically different compounds such as peptides, polyketides, steroids, enzymes, alkaloids, amino acids, hormones etc. (Singh and Kumar, 2023). Both VOC and non-volatile metabolites have a wide role in agriculture and help in plant protection against biotic and abiotic stress (Singh and Kumar, 2023). Some of the examples are shown below in Table 2.
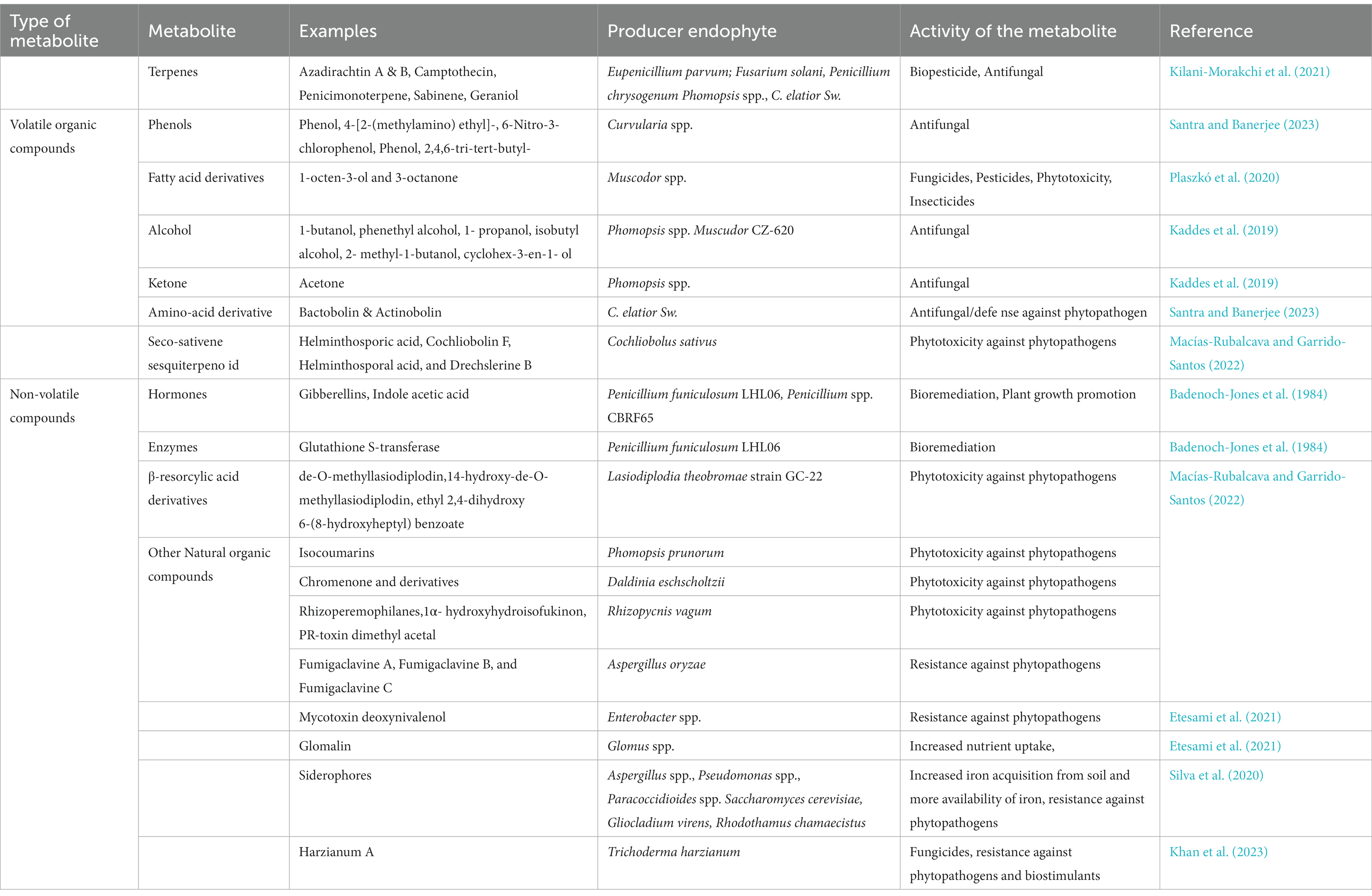
Table 2. List of volatile and non-volatile compounds produced by fungal endophytes and their role in plant protection.
2.3.2 Role of volatile and non-volatile compounds in post-harvest disease management
The role of endophytic bioactive compounds in plant growth, defense, and sustainable agriculture has been well documented in literature (Santra and Banerjee, 2023). The post-harvest loss of crop plants due to pathogens or physiological conditions is high, especially in the case of fruits and vegetables. With the growing resistance of phytopathogens to traditional anti-pathogenic agents like fungicides, pesticides or insecticides etc., and the effects of such substances have shifted the focus towards more sustainable options. The use of alternative approaches such as low temperatures, irradiation, essential oils, salt, antagonistic microorganisms etc. for post-harvest protection of crop plants has been documented (Youssef et al., 2022) The use of endophytic fungi as a biocontrol agent for both pre- and post-harvest protection of crop plants has emerged as a viable alternative for various chemical compounds like pesticides, insecticides, herbicides etc. (Kumar et al., 2021). Fungal endophytes such as members of genera Muscodor, Xylaria, Trichoderma, Fusarium etc. are known to produce a large number of volatile compounds with the potential of post-harvest crop protection (Macías-Rubalcava and Garrido-Santos, 2022). The volatile compounds produced by the endophytic fungi can be applied as fumigation agents, for creating controlled atmospheric storage or as inhibitors of hormones that promotes the ripening of the stored crop. Volatile compounds such as esters, alcohol hydrocarbons, lipids, ketones acids, etc. are more suitable, effective, and ecologically sustainable for the management of post-harvest pathogens due to their long-distance antagonistic action scale, resulting in direct penetration at spatial scales, e.g., volatiles of M. albus (also known as Mycofumigation,), volatiles of Oxyporus latemarginatus, volatiles of Nodulisporium which inhibits 12 different pathogens (Naik, 2018) Similarly, many non-volatile compounds produced by endophytic fungi are exploited for their potential of controlling the post-harvest pathogens. Compounds like ergot alkaloids produced by Clavicipitaceae have antimicrobial and pesticidal properties that are effective against a number of post-harvest pathogens (Florea et al., 2017). Resorcyclic acid lactones produced by Penicillium spp., Zopfiellin produced by Zopfiella spp. Chaetoglobosins are produced by Chaetomium spp. are the examples of non-volatile compounds that possess antimicrobial properties. Other compounds like aflavinines produced by Aspergillus spp., Indole-Diterpenes produced by Clavicipitaceae, Harzianic acid produced by Trichoderma have anti-fungal properties, Peramine produced by Epichloë, Tetramic Acid Derivatives produced by different genus of endophytic fungi posses’ properties such as insecticidal, fungicidal and antimicrobials thus, promoting crop protection post-harvest by providing defense against the phytopathogenic (Song et al., 2021). The role of volatile and non-volatile compound in post-harvest plant protection is paramount. Use of these compounds not only help in post-harvest disease management of the crops but also offer a sustainable agriculture practice. These compounds offer multifaceted solutions that address the complex challenge of controlling pathogens and extending the shelf life of harvested crops.
2.4 Phosphate solubilization
For the growth and development of plants, phosphorus is a crucial nutrient. However, in most soils, phosphorus is present in insoluble forms like phosphate rocks or mineral complexes. This renders it less available to plants, limiting their growth and overall health (Johan et al., 2021). Endophytic fungi have evolved the ability to solubilize phosphorus from these insoluble forms into soluble forms that the plant can readily absorb. This is achieved through the secretion of organic acids, which are powerful chelators capable of breaking down complex phosphate compounds. One of the primary organic acids produced by endophytic fungi is gluconic acid. This acid is particularly effective in dissolving phosphorus compounds in the soil. When the endophytes release gluconic acid into the plant’s rhizosphere, it reacts with the insoluble phosphorus, converting it into soluble phosphate ions. These soluble phosphate ions become more available for uptake by the plant’s root system (Etesami et al., 2021). Phosphate solubilization by endophytic fungi is a crucial process that aids in protecting plants from pathogens. By facilitating phosphorus uptake, endophytic fungi contribute to improved plant health and vigor (Baron and Rigobelo, 2022) and healthy plants are better equipped to defend themselves against pathogens. When a plant is well-nourished with an ample supply of phosphorus, it can allocate more energy and resources toward its defense mechanisms. The mechanisms employed by endophytic fungi for phosphate solubilization can be categorized into acidification, enzyme activity, and ion exchange (Sharma et al., 2013). Phosphate solubilization by acidification involves the secretion of organic acids like citric acid, oxalic acid, malic acid, gluconic acid etc. into the rhizosphere. These organic acids act as chelating agents for the binding of metal ions present in the soil to facilitate the release of bound phosphate, thus making them available for plant uptake. Fungal strains such as Trichoderma viride, Trichoderma harzianum, Trichoderma vixens, and Trichoderma longibrachiatum have been reported for phosphate solubilization by acidification (Elhaissoufi et al., 2022). Many endophytic fungi such as Penicillium and Aspergillus have been shown to produce various enzymes like phosphatases and phytases which are capable of hydrolyzing the organic phosphate compounds present in soil and converting insoluble phosphate into soluble inorganic phosphate (Chaudhary et al., 2022). Other than these two mechanisms, endophytic fungi can also exchange metal cations present in the rhizosphere with phosphates, thus effectively releasing soluble phosphates into the surrounding soil (El Hassan, 2017). Endophytic fungi provide an additional source of phosphorus to the plants by solubilizing phosphate, thus enhancing their nutrient uptake and promoting overall growth and health. This increased nutrient availability makes the plants more resistant to pathogenic attacks in several ways such, as enhanced plant growth, and induced systemic resistance like phosphate-solubilizing endophytic fungi can trigger systemic resistance in plants. They can stimulate the plant’s defense mechanisms and produce various secondary metabolites that act as natural biopesticides, thereby protecting the plant from pathogenic attacks. Overall, phosphate solubilization by endophytic fungi is a multifaceted mechanism that contributes significantly to plant protection against pathogens. These beneficial fungi play a vital role in promoting plant health and crop productivity while reducing the need for chemical fertilizers and pesticides, making them essential components of sustainable agriculture practices.
2.5 Siderophore production
For all eukaryotes and almost all prokaryotes, iron is a crucial nutrient, since it is necessary for metabolic function. Availability of iron in two different oxidation states, i.e., ferric (Fe3+) and ferrous (Fe2+), helps in serving iron both as a cofactor and a catalyst in basic metabolic processes. Despite being one of the metals with the highest abundance on Earth, iron has a low bioavailability due to the fact that iron forms a highly insoluble compound ferric hydroxides in the presence of oxygen (Expert, 1999). Overall, a sufficient supply of iron is necessary for survival. Various species have created controlled systems to maintain homeostasis between sufficient uptake of iron and preventing iron toxicity to avoid cell damage. In plants, animals, and bacteria, iron with high-affinity forms complex with glycoproteins such as lactoferrin or transferrin. Iron is stored intracellularly in ferritin (Arosio and Levi, 2002). Before recent times, little was known about the role of iron in interactions between fungi and their hosts as well as the general regulatory mechanisms that control iron homeostasis. Reductive iron assimilation and Siderophore-mediated iron uptake are the two major systems used by fungi for iron uptake (Oide et al., 2006). Microbes produce siderophores, which are low molecular weight, essentially ferric-specific ligands, as scavenging agents to counteract low iron stress (Sid = Iron, Phores = Bearers; Korat et al., 2001). Except for Lactobacilli, Candida albicans, Cryptococcus neoformans, Saccharomyces cerevisiae all other aerobic and facultative anaerobic microorganisms are known to produce siderophores, which function as iron chelates (Loper and Buyer, 1991). Siderophores are thought to be the result of Fe2+ being simultaneously oxidized to Fe3+ and precipitated as ferric hydroxide as an evolutionary reaction to the presence of O2 in the atmosphere (Winkelmann and Drechsel, 2008). Variety of siderophores produced by fungi have been classified mainly into five major classes (a) fusigens, (b) coprogens, (c) ferrichromes, (d) rhodotorulic acid, and (e) rhizoferrin (Chincholkar et al., 2000). Some of the fungal siderophores are enlisted in Table 3.
The extraordinarily high affinity of siderophores for ferric ions is the most notable characteristic of Siderophores (Askwith et al., 1996). When competing for nutrients in the soil, the ability to exploit siderophores produced by different microbes is a significant selection advantage. The most significant biotechnological importance of siderophores is in the plant’s rhizosphere, where they nourish the plant with iron, act as a first line of defense against parasites that invade the roots, and aid in the removal of hazardous metals from contaminated soil (Matzanke et al., 1987). Studying the opportunistic human pathogen Aspergillus fumigatus led to the initial discovery of the function of fungal siderophores in illness (Sindhu et al., 1997). In vitro removal of iron from transferrin by siderophores was linked to the survival of pathogen in human serum (Hissen et al., 2004). Another example of role of fungal siderophores can be seen in Candida albicans, a non-siderophore producing yeast. Candida albicans requires Ferrichrome-type siderophores along with Arn1p/Sit1p, a siderophore transporter, for invasion and penetration of its host epithelia (Heymann et al., 2002). The discovery that the metabolic byproduct of the biosynthetic pathway involving the NPS6 gene, encoding a non-ribosomal peptide synthase from Cochliobolus heterostrophus, a phytopathogenic fungus, recognized the role of Siderophores in fungal virulence towards plants (Oide et al., 2007). Many studies on NPS6 gene demonstrated its role in virulence to maize against H2O2 hypersensitivity and to be broadly conserved among the filamentous ascomycetes (Lee et al., 2005). Beyond virulence, fungal siderophores also serve to maintain the mutualistic symbiotic relationships between grass and endophytes. The grass symbiont Epichloe festucae uses the NRPS gene sidN to make a new extracellular siderophore that looks like fusarinine-type siderophores. These helpful fungi are never free-living; instead, they are restricted to the intercellular spaces (apoplast) of leaf sheaths and blades, where they do not spread disease. On the other hand, Mycorrhizal fungi frequently create advantageous symbiosis with the roots of terrestrial plant communities, which benefit plant nutrition, including the uptake of micronutrients (Johnson, 2008). Also, these fungi have been found to produce hydroxamate siderophores, and it is believed that siderophore-mediated iron uptake is crucial for the acquisition of iron by the host plant (Haselwandter et al., 2006). Thus, the production of siderophores is a potent strategy employed by various fungal endophytes to grab iron from the environment. Both extracellular and intracellular siderophores play an essential role in numerous fungal-host interactions.
2.6 1-Aminocyclopropane-1-carboxylate utilization
Plant hormones take an active role in symbiotic, defensive, and developmental processes. Ethylene (ET), a gaseous plant hormone that is easily absorbed by plant tissues and has effects even in extremely low quantities, is at the center of these activities (Van de Poel et al., 2015). Some studies have explored the impact of ethylene on the cell division and the finding indicates that ethylene can exert contrasting effects on the cell cycle depending on the specific tissue as well as internal and external stimuli. During the development of the apical hook, ethylene drives cell division in the subepidermal layers, most likely working in conjunction with auxins (Van de Poel et al., 2015). In addition to controlling several aspects of plant growth and development, Ethylene also engages in microbial defense and symbiotic programs which affect overall microbial assembly (Desbrosses and Stougaard, 2011). Moreover, ACC (1-Aminocyclopropane-1-carboxylate) a major precursor of ethylene is a non-proteinogenic α-amino acid that has been reported to play an important role in regulating numerous plant developmental and defense responses. The production of ethylene precursor is mainly regulated at all the major levels (transcription, post-transcription, translation, and post-translation; Lee et al., 2017). The molecule is produced from S’ adenosyl methionine (SAM) in a reaction catalyzed by an enzyme ACC-synthase, releasing MTA (5-methylthioadenosine). Through a series of biochemical reactions, the released MTA is reconverted to methionine to renew the stack of available methionine (Bennett, 2003). Being localized in the cytosol, ACS is one of the members of the Pyridoxal phosphate (PLP) dependent enzymes that uses vitamin B6 as a co-factor for its enzymatic activity (Boller et al., 1979). MACC (malonyl-ACC; Amrhein et al., 1981), GACC (γ-glutamyl-ACC; Martin et al., 1995) and JA-ACC (Jasmonyl-ACC; Staswick and Tiryaki, 2004) are three different conjugates of ACC namely suggesting a complex overall biochemical regulation of ACC pool with eventual effects on the production of ethylene and other developmental and physiological processes. Apart from these conjugates produced in different mechanisms, another distinctive way to metabolize ACC (1-Aminocyclopropane-1-carboxylate) is the deamination of ACC. Initially, ACC deaminase was first reported in bacteria. Many reports suggested that some plant growth-promoting bacteria have the potential to process the plant-based ACC using the ACC deaminase enzyme into ammonia and α-ketoglutarate (Honma and Smmomura, 1978). Being multimeric with an approximate subunit molecular mass of 35-42 kDa (Ullah et al., 2019), ACC deaminase is a sulfhydryl enzyme that requires pyridoxal 5’-phosphate (PLP) for its enzymatic activity (Kushwaha et al., 2020). The cleavage of ACC to ammonia and α-ketoglutarate catalyzed by the enzyme ACC deaminase was discovered in 1978 (Ullah et al., 2019). Following X-ray crystallographic studies, the enzyme ACC deaminase folds into two domains, each of which has an open twisted α/β structure resembling the β-subunit of the tryptophan synthase (Husen et al., 2008). This PLP-dependent enzyme has a low affinity for ACC with a 1.5-15 mM reported Km value (Hontzeas et al., 2004). Many studies have shown that the root exudates contain an explicit amount of ACC that might attract ACC deaminase harboring microorganisms and set up Rhizospheric interaction (Glick et al., 2007). Interaction with the root environment is a must to access the plant-based ACC by the plant growth-promoting microbes containing ACC deaminase enzyme (Payment et al., 2011). Apart from bacterial species, fungi are also exploited for their ACC deaminase activity. Even though the functional principles of the bacterial and fungal ACC deaminase are substantially the same, their structures differ due to sequence variations. Comparison of AcdS gene sequences of various fungal and bacterial strains have shown that approximately 70-90% sequence similarity was shown by the fungal strains with that of bacterial AcdS gene rather than with the fungal gene sequence. Schizosaccharomyces pombe 972 h, Clavispora lusitaniae ATCC 42720, Cyberlindnera saturnus are some of the major examples of fungal strains with higher sequence similarity with bacterial AcdS rather than fungal. It has been hypothesized that horizontal gene transfer is responsible for the transfer of genes from Proteobacteria to the above-mentioned fungal strains (Nascimento et al., 2014) Furthermore, the isolated fungal ACC Deaminase genes similar to Proteobacteria mainly belong to classes Ascomycota and Basidiomycota (Gravel et al., 2007).
2.7 Competition with pathogens
In the rhizosphere and phyllosphere of plants, competitive exclusion, a frequent phenomenon, controls the population of a wide range of species (Sikora et al., 2007). In some circumstances, the biological control of root diseases and nematodes is also a result of competition for nutrients and space. Therefore, when several inoculants are required for efficient pest management, the “first-come, first-serve” aspect of colonization and the idea of survival of the fittest are the major factors that could affect the efficacy of biological control (Backman and Sikora, 2008). Because nutrients in soils are typically in scarce supply and difficult to acquire, competition for resources such as oxygen, nutrients, etc. is the active demand between soil-inhabiting microorganisms including pathogens and non-pathogens, and is a key mechanism for the management of diseases that are carried by soil (Stoytcheva, 2011). Due to competition for scarce nutrients and the fact that starvation is a significant and frequent cause of microbiological death, fungal phytopathogens may be biologically controlled (Eisendle et al., 2004). If the requirements both in terms of nutrients, oxygen, space of endophytic fungi, and the disease-causing pathogens are similar, then only competition will occur among them. Species of Fusarium and Pythium, soil-borne pathogens that infect plants through mycelial contact are more prone to competition than those that invade through infection threads (Van Dijk and Nelson, 2000).
2.7.1 Competition for Iron
Iron is the most abundant element on Earth and an essential nutrient for microorganisms. Though it is the fourth largest mineral found abundantly on our planet it is not readily available to microorganisms. As it gets oxidized easily, it is mainly available in the form of ferric ions that cannot be directly utilized due to its extremely low solubility (Neilands et al., 1987). This in turn leads to competition among microorganisms. One of the biological controls for both bacterial and fungal phytopathogens is the fight for iron nutrition (McMorran et al., 2001). Several studies have indicated that the concentration of iron in the soil is notably lower than the necessary level for microbial growth. Siderophores, known as iron chelators, are low molecular weight molecules that bind to ferric ions dueto their high affinity for iron with Kd values ranging from 10-20 to 10-50 (Castignetti and Smarrelli, 1986). Thus, siderophore-producing microbial strains have a selective advantage over the non-producing strains, including disease-causing microorganisms. This results in the inhibition of the growth of pathogens in their immediate proximity due to iron limitation (O’Sullivan and O’Gara, 1992). It has been found that the rhizosphere biological control agents protect plants from pathogens generally by colonization resulting in the consumption of already available substrates in their surroundings. This makes disease-causing microorganisms or pathogens difficult to grow. Siderophores are produced by a wide range of Plant Growth Promoting Rhizobacteria such as Serratia (Berg et al., 2005), Bacillus (Yu et al., 2011), Pseudomonas (Elad and Baker, 1985) and many more playing major roles in suppressing soil-borne disease-causing pathogens. Based on the ligands, siderophores are mainly classified as carboxylates, phenolates (Catecholates), or hydroxamates (Winkelmann and Drechsel, 2008). Enterobactin, Siderochelin A, Ferrocins, Pyoverdines, Acinetobactin, Cepabactin are among the major examples of bacterial siderophores whereas Ferrichromes, Coprogens, Rhodotorulic Acid, Fusigens and Rhizoferrins are the typical examples of siderophores produces by fungal strains (Winkelmann and Drechsel, 2008). Among these Siderophores, Catecholates are exclusively produced by bacteria but hydroxamate siderophores are produced both by bacteria and fungi (Cox et al., 1981). Many studies revealed that the fungal phytopathogens have a lower affinity for iron as compared to Plant Growth Promoting Rhizobacteria (PGPRs) and thus overpower the fungal phytopathogens for iron (Schippers et al., 2003). In such a case, Pythium and Fusarium species are more prone to competition for iron as they cause infection through mycelial contact, from other microbes associated with soil and plants than those species that germinate directly on plant surfaces (Pal and Gardener, 2006). By analyzing the siderophore mutants, the role of siderophores in biocontrol either alone or in combination with other metabolites such as antibiotics has also been studied (Buysens et al., 1994). Many factors are responsible for affecting the overall capability of siderophores such as properties of soil, type of plant, type of siderophore, type of microbial strain, and type of disease-causing pathogens (Glick and Bashan, 1997). The plant diseases caused by various pathogens such as Fusarium oxysporum, Rhizoctonia solani, Pseudomonas sp., Alternaria sp. etc. are suppressed by siderophores in numerous reports (Sahu and Sindhu, 2011). This overall suggests that to overpower the soil-borne disease-causing pathogens, competition for iron and other mineral ions is one of the major mechanisms, however, siderophores only may not be able to suppress the disease in any case.
2.7.2 Competition for niches
In the biocontrol mechanism of fungal endophytes, competition for niches is how different fungal endophytes fight for space and resources inside a plant host in order to become dominant and use their biocontrol effects against pathogenic fungi. It has long been understood that niche complementarities may play a significant role in a species’ ability to coexist (Backman and Sikora, 2008). The endosphere and the rhizosphere are the complex ecosystems that play a significant role in supporting the overall growth and development of the plants. A wide variety of microorganisms, including fungi, are heavily concentrated in the rhizosphere, the soil environment around plant roots, and the endosphere, which consists of the internal tissues of plants. The two fungal groups—endophytes and plant pathogenic fungi—represent opposing forces in this ecosystem, competing with one another for the little resources and ecological niches available (Oszust et al., 2020). In the endosphere and rhizosphere, competition for resources and space can be fierce, and it is influenced by a number of important variables. First, competition may arise from direct hostility between the two fungal species, which frequently takes the form of mycoparasitism, the direct use of resources, or the synthesis of antimicrobial substances. For instance, the well-known mycoparasitic properties of the endophyte Trichoderma allow it to actively attack and parasitize pathogenic fungi such as Rhizoctonia solani, thereby lessening their detrimental effects on plant health (Howell, 2003). It has also been discovered that fungi in the genus Trichoderma have an ecological niche that is most similar to that of Colletotrichum spp., hence excluding the latter phytopathogenic species (Oszust et al., 2020). Trichoderma spp. is assumed to be tough competitors that drive out slower-growing diseases since they are discovered as being particularly quick colonists (Tyśkiewicz et al., 2022). Based on an in vitro experiment, Oszust et al. (2020) discovered that Trichoderma spp. nutritionally outcompeted Botrytis sp., Verticillium spp., and Phytophthora spp. According to Morandi et al., the non-pathogenic endophyte Clonostachys rosea inhibited the growth and sporulation capability of B. cinerea to regulate it (Morandi et al., 2000). Second, in moderating the conflict between plant diseases and endophytes, plant host selection is essential. Certain plants have the ability to actively attract or encourage the growth of particular endophytes, which benefit them and restrict harmful fungus. In order to give endophytes a competitive edge, this procedure may involve the release of root exudates that specifically favor advantageous fungus. Moreover, the outcome of the rivalry between the two fungal groups can also be determined by their spatial distribution. The vascular system and intercellular gaps are two examples of the particular niches that endophytic fungi frequently occupy within plant tissues (Faeth, 2002). Due to the possibility that they have distinct preferred areas for colonization, this spatial separation can reduce direct competition with plant pathogenic fungus. On the other hand, direct interactions between endophytes and pathogenic fungi are more likely to occur in the rhizosphere, which is the interface between plant roots and soil. Researchers and agricultural scientists are interested in understanding these interactions because they can impact the efficacy of using biocontrol endophytes as a sustainable alternative. By studying the competition for niches among fungal endophytes and their interactions with pathogens and the host plant, they can develop strategies with pathogens and the host plant to enhance the biocontrol potential of specific endophytes, and improve plant disease management practices.
3 Indirect mechanisms of plant protection
To survive in harsh environments like famine, salt stress, and cold, plants use a variety of strategies. Some of the biochemical and morphological changes that can be seen right away are the formation of phytoalexins, the death of cells, and the hypersensitive response. Kiraly et al., found that long-term evolution can lead to both non-specific (generic) and specific (pathogen-specific) resistance (Kiraly et al., 2007). Plants with non-specific resistance can protect themselves from a broad range of diseases, but plants with particular resistance can avoid getting sick from just one or a few infections. Endophytes produce secondary metabolites and have improved resistance, which strengthens the plant’s defense system.
3.1 Induced plant resistance
Several research have examined how plants respond to disease and parasite invasions for more than 20 years, utilizing several categories. Systemic acquired resistance (SAR) and induced systemic resistance (ISR) are the two types of resistance that researchers are most interested in. ISR is regulated by ethylene or jasmonic acid, which is produced by some non-pathogenic rhizobacteria and not connected to the accumulation of pathogenesis-related (PR) proteins. Salicylic acid mediates SAR, which is a result of pathogen infections and is linked to the production of PR proteins (Tripathi et al., 2008). Invading cells are directly lysed by these PR proteins’ many enzymes, like chitinases and 1, 3-glucanases, which also strengthen cell wall borders and increase resistance to cell death and infection (Gao et al., 2010). ISR generated by endophytes has also been associated with increased expression of genes involved in disease. The ISR process does not directly kill the virus or restrict it. Instead, it strengthens the plants’ natural physical or chemical barriers (Van Loon et al., 1998). ISR and SAR frequently have an antagonistic action that controls the signaling at the cellular level. When SA and JA have an antagonistic effect on biotrophic or necro pathogens, and vice versa, there is upstream and downstream signaling between them (Syed Ab Rahman et al., 2018). For instance, Blumeria graminis f. spp. Tritici, which causes powdery mildew in wheat, is controlled by Bacillus subtilis by inducing disease resistance via the SA-dependent signaling pathway (Xia et al., 2022). The non-expression of the pathogenesis-related genes 1 (NPR1 and NPR3/4), which eventually trigger an antagonistic response via SAR through priming and reveal resistance against secondary infections, is crucially regulated by the pathogenesis-related gene 1 (PR1; Ding et al., 2009). By preventing Botrytis cinerea’s spore germination and mycelium growth through the ISR method of resistance, Burkholderia species (BE17 and BE24) shield grapevine from the grey mold disease (Lahlali et al., 2022). Trichoderma spp. AA2 and Pseudomonas fluorescens PFS are the most powerful antagonists of Ralstonia spp., which causes bacterial wilt in tomatoes by generating ISR in the plant.
3.2 Stimulation of plant secondary metabolites
Secondary metabolites are bioactive substances that have an important function in ecological interactions, competition, and defensive signaling (Liu et al., 2018). The production of secondary metabolites via a metabolic exchange, which exhibits a complicated regulatory response, is necessary for the formation of microbial contact. These interactions may be competitive, parasitic, mutualistic, hostile, or mutualistic. The most recent advances in imaging mass spectrometry (IMS) technology have been employed to investigate the diverse roles played by mold metabolites in microbial interactions. The growth of phytopathogens is regulated by the antibacterial and antifungal activities of secondary metabolites. While under biotic stress, plants can create secondary metabolites on their own or in collaboration with other endophytes to manage stress and mount defenses (Ludwig-Müller, 2015). To safeguard plants and enhance crop quality, endophytic secondary metabolites are employed as a biocontrol agent. Whereas microorganisms create homogeneous, high-quality metabolites with the highest efficacy in terms of their biocontrol potential, plants produce bioactive molecules that are inadequate and variable in quality (Lugtenberg et al., 2016). An example of plant secondary metabolite stimulation by endophytic fungi is the production of loline (an alkaloid) in the leaves of fescue grasses by endophytic fungus Neotyphodium spp. and Epichloë spp., which protects the leaves from herbivores. The interaction between endophytic fungus Neotyphodium coenophialum and its host plant is another example of plant secondary metabolite stimulation. Neotyphodium coenophialum protects its host plants from aphids the key carriers of viruses, e.g., protection of Festuca arundinacea against Rhopalosiphum padi also inhibit the spread of viruses (Alam et al., 2021).
3.3 Hyperparasites and predation
Hyperparasites are another means by which endophytes protect their host ecologically. In this approach, recognized pathogens or their zoospores are immediately attacked by endophytes (Tripathi et al., 2008) By twisting and piercing the hyphae of the pathogens and creating lyase, which dissolves the pathogen’s cell wall, endophytic fungi trap the pathogens. This sort of interaction among fungi is frequently seen. Hyper parasitism in bacteria has only occasionally been documented. A predatory bacterium called Bdellovibrio bacteriovorus has the peculiar ability to exploit the cytoplasm of other Gram-negative bacteria as food (McNeely et al., 2017). Over 30 fungal species, including Cladosporium uredinicola against Puccinia violae and Alternaria alternata against Puccinia striiformis f. spp. tritici, reported by Zheng et al. to exhibit hyper parasitism against rust pathogens (Jia et al., 2016). The reduction of plant pathogens through microbial predation is another technique. The majority of endophytes show their predatory traits in nutrient-poor environments. For example, fungal endophytes isolated from Taxillus chinensis produce cell wall-degrading enzymes that promote the dissolution and relaxation of the cell wall between the host and Taxillus chinensis (Wu et al., 2021).
3.4 Fungal endophytes as biocontrol agents
Endophytes are described as microorganisms (bacteria or fungal) that are found living in tissue, plant organs, and seeds of almost all vascular plants (Caruso et al., 2022). The interaction between the endophyte and the host plant during their association has proven to be beneficial rather than harmful for both interacting organisms. These benefits are always based on the interaction between endophytes and the host plants (Kamana et al., 2016). Many novel bioactive compounds like antibiotics, antimycotics, antineoplastics, etc. are endophytic products (Shukla et al., 2014). The agro-industry relies heavily on the use of agrochemicals for controlling phytopathogens. This excessive use of chemicals in the agricultural industry has resulted in the development of resistant phytopathogens. The endophytes play an important in maintaining the health of the host plant (Wu et al., 2021). These endophytes can be exploited as a biocontrol agent in the agro-industry. Biocontrol agents (BCA) are described as living organisms or their products that can fight against plant diseases or pests via direct antagonistic action (Xia et al., 2022). Many endophytes are being exploited as BCA as they are effective in controlling plant diseases and can help attain sustainable agriculture. The endophytic fungi that act as biocontrol agents against different phytopathogens are summarized in Table 4.
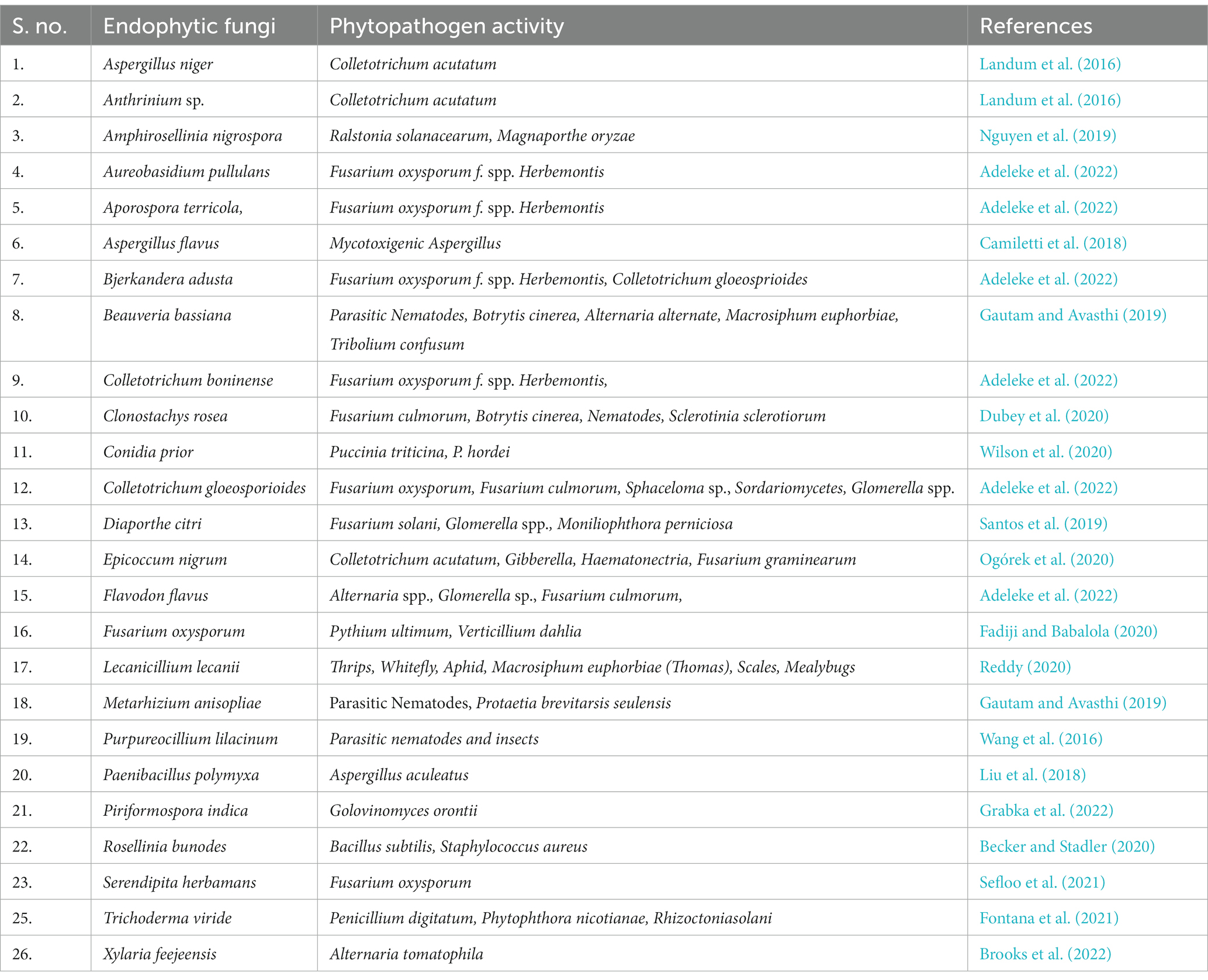
Table 4. Summarized list of endophytic fungi that act as biocontrol agents against various phytopathogens.
3.5 Protection against leaf-cutting ants
Endophytic fungi play a pivotal role in safeguarding plants from the destructive foraging of leaf cutting ants. These mutualistic fungal endophytes, which commonly inhabit plant tissues, establish intricate associations with their host plants. One of their primary mechanisms of protection lies in the production of secondary metabolites, such as alkaloids and mycotoxins, which serve as potent deterrents against herbivores, including leaf-cutting ants (White and Torres, 2010). By secreting these chemical compounds, endophytic fungi effectively shield their host plants, rendering them unpalatable or toxic to the ants. This protective alliance not only benefits the plants by reducing herbivore-induced damage but also highlights the fascinating interplay between plants and their endophytic partners in the intricate web of ecological relationships.
3.5.1 Hypocreales endophytes in coffee plants
Fungal endophytes belonging to the order Hypocreales have been discovered in coffee plants, producing mycotoxins that deter leaf cutting ants. The presence of these endophytes in the coffee plants was demonstrated in a study conducted by Mejia et al. in the year 2008 (Mejía et al., 2008).
3.5.2 Trichoderma species
Certain Trichoderma species are endophytic fungi that have been shown to protect plants from various pests, including leaf-cutting ants. These endophytes can produce antifungal and insecticidal compounds that deter herbivores. Studies like Lo et al. (2015) have highlighted their potential in biocontrol (Yao et al., 2023).
3.5.3 Clavicipitaceous endophytes in grasses
Clavicipitaceous fungal endophytes, such as Neotyphodium and Epichloe species are known to form mutualistic associations with grasses. They can produce alkaloids, like ergot alkaloids, that deter herbivores including leaf-cutting ants (Saikkonen et al., 2004).
These examples highlight the diverse array of fungal endophytes and their crucial role in protecting plants from leaf-cutting ants through various mechanisms, including the production of chemical compounds and mutualistic associations.
4 Conclusive remarks
In light of the aforementioned explanation, endophytic fungi mostly use direct and indirect approaches to combat disease-causing pathogens. The management and treatment of plant diseases are extremely important for sustainable agriculture. In order to prevent adverse impacts on the environment and human health, national rules are becoming harsher when it comes to regulating and authorizing new pesticides. The demand for organic and safe foods is growing daily. It is therefore vital to look for novel control strategies that ensure food safety and lessen these adverse consequences. To keep the harm caused by herbivores or pathogens at manageable levels for farmers, a worldwide strategy that considers all of the aforementioned issues must be devised. In this regard, with emerging applications in agriculture, endophytes present a very intriguing subject of study. Undoubtedly, a variety of endophytic fungi can be exploited for the treatment of various plant diseases and as a substitute for available biocontrol agents against phytopathogens. These fungal endophytes are also a good source of beneficial bioactive substances for plants. However, not much attention has been paid to how important it is for biotechnology that endophytic fungi make beneficial metabolites that help control plant diseases. In recent times, biologically active compound producing and plant growth promoting endophytes have drawn more interest from researchers since they are potential sources of novel drugs and eco-friendly plant protectors. Among all identified possible biocontrol agents, members of the genus Trichoderma have received extensive investigation; however, there are many other comparably potent endophytic fungal families that have not been adequately investigated and explored. These biocontrol organisms have a significant impact on agriculture and the overall environment. Molecular identification of microbes which are being exploited as biocontrol agents and biological characterization of bioactive chemicals produced by them is essential for understanding their antagonistic mechanisms.
5 Future prospects
The future prospects of biocontrol mechanisms involving fungal endophytes in sustainable agriculture appear exceptionally promising. As the world grapples with the growing need for environment friendly and resilient farming practices, fungal endophytes stand out as a valuable ally. These fungi possess the ability to bolster crop health and protect against pests and pathogens, reducing the reliance on chemical pesticides. As the understanding of the intricate interactions between fungal endophytes and plants deepens, can expect the development of specialized, genetically optimized strains that offer even greater efficacy. This could lead to substantial reductions in agriculture’s environmental footprint while simultaneously increasing crop yields and food security. Moreover, the potential of fungal endophytes to enhance soil health and nutrient cycling holds promise for sustainable agriculture in the long term. With ongoing research and innovation, the integration of fungal endophytes into agricultural practices is likely to play a pivotal role in shaping a more sustainable and resilient future for global food production. Future research is required for better understanding of antagonistic behavior of these endophytic fungi to propagate them as environmentally friendly tools for managing phytopathogens naturally.
Author contributions
MB: Writing – original draft. SKa: Writing – original draft. RK: Writing – original draft. SKh: Conceptualization, Writing – original draft, Writing – review & editing. SR: Supervision, Writing – review & editing.
Funding
The author(s) declare that no financial support was received for the research, authorship, and/or publication of this article.
Acknowledgments
We are thankful for all the support from Shri Mata Vaishno Devi University, Katra, J&K, India.
Conflict of interest
The authors declare that the research was conducted in the absence of any commercial or financial relationships that could be construed as a potential conflict of interest.
Publisher’s note
All claims expressed in this article are solely those of the authors and do not necessarily represent those of their affiliated organizations, or those of the publisher, the editors and the reviewers. Any product that may be evaluated in this article, or claim that may be made by its manufacturer, is not guaranteed or endorsed by the publisher.
References
Abaya, A., Xue, A., and Hsiang, T. (2021). Selection and screening of fungal endophytes against wheat pathogens. Biol. Control 154:104511. doi: 10.1016/J.BIOCONTROL.2020.104511
Abedinzadeh, M., Etesami, H., and Alikhani, H. A. (2019). Characterization of rhizosphere and endophytic Bacteria from roots of maize (Zea Mays L.) plant irrigated with wastewater with biotechnological potential in agriculture. Biotechnol. Rep. 21:e00305. doi: 10.1016/J.BTRE.2019.E00305
Adeleke, B. S., Ayilara, M. S., Akinola, S. A., and Babalola, O. O. (2022). Biocontrol mechanisms of endophytic fungi. Egyp. J. Biol. Pest Control. 32:46. doi: 10.1186/s41938-022-00547-1
Agostini, R. B., Postigo, A., Rius, S. P., Rech, G. E., Campos-Bermudez, V. A., and Vargas, W. A. (2019). Long-lasting primed state in maize plants: salicylic acid and steroid signaling pathways as key players in the early activation of immune responses in silks. Mol. Plant-Microbe Interact. 32, 90–106. doi: 10.1094/MPMI-07-18-0208-R/ASSET/IMAGES/LARGE/MPMI-07-18-0208-R_F7.JPEG
Akram, S., Ahmed, A., He, P., He, P., Liu, Y., Wu, Y., et al. (2023). Uniting the role of endophytic fungi against plant pathogens and their interaction. J. Fungi 9:72. doi: 10.3390/jof9010072
Alam, B., Lǐ, J., Gě, Q., Khan, M. A., Gōng, J., Mehmood, S., et al. (2021). Endophytic fungi: from Symbiosis to secondary metabolite communications or vice versa? Front. Plant Sci. 12:791033. doi: 10.3389/fpls.2021.791033
Amrhein, N., Schneebeck, D., Skorupka, H., Tophof, S., and Stöckigt, J. (1981). Identification of a major metabolite of the ethylene precursor 1-aminocyclopropane-1-carboxylic acid in higher plants. Naturwissenschaften 68, 619–620. doi: 10.1007/BF00398617
Arnold, A. E., Mejía, L. C., Kyllo, D., Rojas, E. I., Maynard, Z., Robbins, N., et al. (2003). Fungal endophytes limit pathogen damage in a tropical tree. Proc. Natl. Acad. Sci. 100, 15649–15654. doi: 10.1073/pnas.2533483100
Arosio, P., and Levi, S. (2002). Ferritin, iron homeostasis, and oxidative damage. Free Radic. Biol. Med. 33, 457–463. doi: 10.1016/S0891-5849(02)00842-0
Askwith, C. C., De Silva, D., and Kaplan, J. (1996). Molecular biology of Iron acquisition in Saccharomyces Cerevisiae. Mol. Microbiol. 20, 27–34. doi: 10.1111/j.1365-2958.1996.tb02485.x
Azhari, A., and Supratman, U. (2021). The chemistry and pharmacology of fungal genus Periconia: a review. Sci. Pharm. 89:34. doi: 10.3390/scipharm89030034
Backman, P. A., and Sikora, R. A. (2008). Endophytes: an emerging tool for biological control. Biol. Control 46, 1–3. doi: 10.1016/J.BIOCONTROL.2008.03.009
Badenoch-Jones, J., Summons, R. E., Rolfe, B. G., and Letham, D. S. (1984). Phytohormones, rhizobium mutants, and nodulation in legumes. IV. Auxin metabolites in pea root nodules. J. Plant Growth Regul. 3, 23–39. doi: 10.1007/BF02041989
Balla, A., Silini, A., Cherif-Silini, H., Bouket, A. C., Moser, W. K., Nowakowska, J. A., et al. (2021). The threat of pests and pathogens and the potential for biological control in forest ecosystems. Forests 12:1579. doi: 10.3390/f12111579
Barkodia, M., Joshi, U., Wati, L., and Rami, N. V. (2018). Endophytes: a hidden treasure inside plant. Int. J. Chem. Stud. 6, 1660–1665.
Baron, N. C., and Rigobelo, E. C. (2022). Endophytic fungi: a tool for plant growth promotion and sustainable agriculture. Mycology 13, 39–55. doi: 10.1080/21501203.2021.1945699
Becker, K., and Stadler, M. (2020). Recent progress in biodiversity research on the xylariales and their secondary metabolism. J. Antibiot. 74, 1–23. doi: 10.1038/s41429-020-00376-0
Bennett, J. (2003). Protein phosphorylation in green plant chloroplasts. Annu. Rev. Plant Physiol. Plant Mol. Biol. 42, 281–311. doi: 10.1146/ANNUREV.PP.42.060191.001433
Berg, G., Krechel, A., Ditz, M., Sikora, R. A., Ulrich, A., and Hallmann, J. (2005). Endophytic and ectophytic potato-associated bacterial communities differ in structure and antagonistic function against plant pathogenic fungi. FEMS Microbiol. Ecol. 51, 215–229. doi: 10.1016/j.femsec.2004.08.006
Bhattacharya, A., Sood, P., and Citovsky, V. (2010). The roles of plant phenolics in defence and communication during agrobacterium and rhizobium infection. Mol. Plant Pathol. 11, 705–719. doi: 10.1111/j.1364-3703.2010.00625.x
Boller, T., Herner, R. C., and Kende, H. (1979). Assay for and enzymatic formation of an ethylene precursor, 1-aminocyclopropane-1-carboxylic acid. Planta 145, 293–303. doi: 10.1007/BF00454455
Brooks, S., Klomchit, A., Chimthai, S., Jaidee, W., and Bastian, A. C. (2022). Xylaria feejeensis, SRNE2BP a fungal endophyte with biocontrol properties to control early blight and fusarium wilt disease in tomato and plant growth promotion activity. Curr. Microbiol. 79, 1–15. doi: 10.1007/S00284-022-02803-X/FIGURES/5
Buysens, Saskia, Poppe, Joseph, and Höfte, Monica. (1994). “Role of Siderophores in plant growth stimulation and Antigonism by Pseudomonas Aeruginosa 7NSK2.” Improving plant productivity with rhizosphere Bacteria (MH. Ryder, PM. Stephens, GD. Bowen, Eds.). - Proceedings of the Third International Workshop on Plant Growth-Promoting Rhizobacteria Adelaide, Australia, March 7-11, 139-141. Available at: http://hdl.handle.net/1854/LU-247535.
Camiletti, B. X., Moral, J., Asensio, C. M., Torrico, A. K., Lucini, E. I., de la Paz Giménez-Pecci, M., et al. (2018). Characterization of argentinian endemic Aspergillus flavus isolates and their potential use as biocontrol agents for mycotoxins in maize. Phytopathology 108, 818–828. doi: 10.1094/PHYTO-07-17-0255-R
Caruso, D. J., Palombo, E. A., Moulton, S. E., and Zaferanloo, B. (2022). Exploring the promise of endophytic fungi: a review of novel antimicrobial compounds. Microorganisms 10:1990. doi: 10.3390/microorganisms10101990
Castignetti, D., and Smarrelli, J. (1986). Siderophores, the iron nutrition of plants, and nitrate reductase. FEBS Lett. 209, 147–151. doi: 10.1016/0014-5793(86)81100-0
Chaudhary, P., Agri, U., Chaudhary, A., Kumar, A., and Kumar, G. (2022). Endophytes and their potential in biotic stress management and crop production. Front. Microbiol. 13:933017. doi: 10.3389/FMICB.2022.933017/BIBTEX
Cheng, C., Li, D., Qi, Q., Sun, X., Anue, M. R., David, B. M., et al. (2020). The root endophytic fungus serendipita indica improves resistance of banana to Fusarium oxysporum f. Sp. Cubense tropical race 4. Eur. J. Plant Pathol. 156, 87–100. doi: 10.1007/s10658-019-01863-3
Chincholkar, S B, Chaudhari, B L, Talegaonkar, S K, and Kothari, R M. (2000). “Microbial Iron chelators: a tool for sustainable agriculture.” Biocontrol Potential and Their Exploration in Crop Disease Management 1: 49–70.
Cox, C. D., Rinehart, K. L., Moore, M. L., and Cook, J. C. (1981). Pyochelin: novel structure of an Iron-chelating growth promoter for Pseudomonas Aeruginosa. Proc. Natl. Acad. Sci. 78, 4256–4260. doi: 10.1073/pnas.78.7.4256
Desbrosses, G. J., and Stougaard, J. (2011). Root nodulation: a paradigm for how plant-microbe symbiosis influences host developmental pathways. Cell Host Microbe 10, 348–358. doi: 10.1016/j.chom.2011.09.005
Deshmukh, S. K., Dufossé, L., Chhipa, H., Saxena, S., Mahajan, G. B., and Gupta, M. K. (2022). Fungal endophytes: a potential source of antibacterial compounds. J. Fungi 8:164. doi: 10.3390/jof8020164
Dijk, K.Van, and Nelson, E. B.. (2000). “Fatty acid competition as a mechanism by which Enterobacter Cloacae suppresses Pythium Ultimum sporangium germination and damping-off.” Appl. Environ. Microbiol. 66: 5340–5347. doi: 10.1128/AEM.66.12.5340-5347.2000
Ding, G., Li, Y., Shaobin, F., Liu, S., Wei, J., and Che, Y. (2009). Ambuic acid and torreyanic acid derivatives from the endolichenic fungus Pestalotiopsis Sp. J. Nat. Prod. 72, 182–186. doi: 10.1021/np800733y
Dubey, M., Vélëz, H., Broberg, M., Jensen, D. F., and Karlsson, M. (2020). LysM proteins regulate fungal development and contribute to hyphal protection and biocontrol traits in Clonostachys rosea. Front. Microbiol. 11:520051. doi: 10.3389/FMICB.2020.00679/BIBTEX
Eisendle, M., Oberegger, H., Buttinger, R., Illmer, P., and Haas, H. (2004). Biosynthesis and uptake of siderophores is controlled by the PacC-mediated ambient-PH regulatory system in Aspergillus nidulans. Eukaryot. Cell 3, 561–563. doi: 10.1128/EC.3.2.561-563.2004
Elad, Y., and Baker, R. (1985). Influence of trace amounts of cations and siderophore-producing pseudomonads on chlamydospore germination of Fusarium oxysporum. Phytopathology 75, 1047–1052. doi: 10.1094/Phyto-75-1047
Elgorban, A. M., Bahkali, A. H., Al Farraj, D. A., and Abdel-Wahab, M. A. (2019). Natural products of Alternaria Sp., an endophytic fungus isolated from Salvadora persica from Saudi Arabia. Saudi J. Biol. Sci. 26, 1068–1077. doi: 10.1016/j.sjbs.2018.04.010
Elhaissoufi, W., Ghoulam, C., Barakat, A., Zeroual, Y., and Bargaz, A. (2022). Phosphate bacterial solubilization: a key rhizosphere driving force enabling higher P use efficiency and crop productivity. J. Adv. Res. 38, 13–28. doi: 10.1016/J.JARE.2021.08.014
Etesami, H., Jeong, B. R., and Glick, B. R. (2021). Contribution of arbuscular mycorrhizal Fungi, phosphate–solubilizing bacteria, and silicon to P uptake by plant. Front. Plant Sci. 12:699618. doi: 10.3389/fpls.2021.699618
Expert, D. (1999). Withholding and exchanging Iron: interactions between Erwinia Spp. and their plant hosts. Annu. Rev. Phytopathol. 37, 307–334. doi: 10.1146/annurev.phyto.37.1.307
Fadiji, A. E., and Babalola, O. O. (2020). Elucidating mechanisms of endophytes used in plant protection and other bioactivities with multifunctional prospects. Front. Bioeng. Biotechnol. 8:467. doi: 10.3389/fbioe.2020.00467
Faeth, S. H. (2002). Fungal endophytes: common host plant symbionts but uncommon mutualists. Integr. Comp. Biol. 42, 360–368. doi: 10.1093/icb/42.2.360
Florea, S., Panaccione, D. G., and Schardl, C. L. (2017). Ergot alkaloids of the family clavicipitaceae. Phytopathology 107, 504–518. doi: 10.1094/PHYTO-12-16-0435-RVW
Fontana, D. C., de Paula, S., Torres, A. G., Moura, V. H., de Souza, S., Pascholati, F., et al. (2021). Endophytic fungi: biological control and induced resistance to phytopathogens and abiotic stresses. Pathogens 10:570. doi: 10.3390/pathogens10050570
Franco-Orozco, B., Berepiki, A., Ruiz, O., Gamble, L., Griffe, L. L., Wang, S., et al. (2017). A new proteinaceous pathogen-associated molecular pattern (PAMP) identified in ascomycete fungi induces cell death in solanaceae. New Phytol. 214, 1657–1672. doi: 10.1111/nph.14542
Gao, F.-K., Dai, C.-C., and Liu, X.-Z. (2010). Mechanisms of fungal endophytes in plant protection against pathogens. Afr. J. Microbiol. Res. 4, 1346–1351.
Gautam, Ajay Kumar, and Avasthi, Shubhi. (2019). “Fungal endophytes: potential biocontrol agents in agriculture.” Role of plant growth promoting microorganisms in sustainable agriculture and nanotechnology, January, 241–283.
Ghozlan, M. H., EL-Argawy, E., Tokgöz, S., Lakshman, D. K., Mitra, A., Ghozlan, M. H., et al. (2020). Plant defense against necrotrophic pathogens. Am. J. Plant Sci. 11, 2122–2138. doi: 10.4236/ajps.2020.1112149
Glick, B. R., and Bashan, Y. (1997). Genetic manipulation of plant growth-promoting bacteria to enhance biocontrol of phytopathogens. Biotechnol. Adv. 15, 353–378. doi: 10.1016/S0734-9750(97)00004-9
Glick, Bernard R., Cheng, Zhenyu, Czarny, Jennifer, and Duan, Jin. (2007). “Promotion of plant growth by ACC deaminase-producing soil bacteria.” New perspectives and approaches in plant growth-promoting rhizobacteria research, 329–339.
Gouda, S., Das, G., Sen, S. K., Shin, H. S., and Patra, J. K. (2016). Endophytes: a treasure house of bioactive compounds of medicinal importance. Front. Microbiol. 7:219261. doi: 10.3389/FMICB.2016.01538/BIBTEX
Grabka, R., D’entremont, T. W., Adams, S. J., Walker, A. K., Tanney, J. B., Abbasi, P. A., et al. (2022). Fungal endophytes and their role in agricultural plant protection against pests and pathogens. Plan. Theory 11:384. doi: 10.3390/plants11030384
Gravel, V., Antoun, H., and Tweddell, R. J. (2007). Growth stimulation and fruit yield improvement of greenhouse tomato plants by inoculation with Pseudomonas Putida or Trichoderma atroviride: possible role of indole acetic acid (IAA). Soil Biol. Biochem. 39, 1968–1977. doi: 10.1016/j.soilbio.2007.02.015
Haran, S., Schickler, H., and Warburg, O. (1996). Molecular mechanisms of lytic enzymes involved in the biocontrol activity of 1 Trichoderma Harzianum R-hydrolytic enzymes of Trichoderma harzianum involved in mycoparasitism. Microbiology 142. Baker, 2321–2331. doi: 10.1099/00221287-142-9-2321
Hassan, S. E. D. (2017). Plant growth-promoting activities for bacterial and fungal endophytes isolated from medicinal Plant of Teucrium polium L. J. Adv. Res. 8, 687–695. doi: 10.1016/j.jare.2017.09.001
Haselwandter, K., Passler, V., Reiter, S., Schmid, D. G., Nicholson, G., Hentschel, P., et al. (2006). Basidiochrome - a novel siderophore of the orchidaceous mycorrhizal fungi Ceratobasidium and Rhizoctonia Spp. Biometals 19, 335–343. doi: 10.1007/s10534-006-6986-x
Heymann, P., Gerads, M., Schaller, M., Dromer, F., Winkelmann, G., and Ernst, J. F. (2002). The siderophore Iron transporter of Candida Albicans (Sit1p/Arn1p) mediates uptake of ferrichrome-type siderophores and is required for epithelial invasion. Infect. Immun. 70, 5246–5255. doi: 10.1128/IAI.70.9.5246-5255.2002
Hissen, A. H. T., Chow, J. M. T., Pinto, L. J., and Moore, M. M. (2004). Survival of Aspergillus Fumigatus in serum involves removal of Iron from transferrin: the role of siderophores. Infect. Immun. 72, 1402–1408. doi: 10.1128/IAI.72.3.1402-1408.2004
Honma, M., and Smmomura, T. (1978). Metabolism of 1-Aminocyclopropane-1-carboxylic acid. Agric. Biol. Chem. 42, 1825–1831. doi: 10.1080/00021369.1978.10863261
Hontzeas, N., Zoidakis, J., Glick, B. R., and Abu-Omar, M. M. (2004). Expression and characterization of 1-aminocyclopropane-1-carboxylate deaminase from the rhizobacterium Pseudomonas Putida UW4: a key enzyme in bacterial plant growth promotion. Biochim. Biophysica Acta Prot. Proteomics 1703, 11–19. doi: 10.1016/j.bbapap.2004.09.015
Howell, C. R. (2003). Mechanisms employed by Trichoderma species in the biological control of plant diseases: the history and evolution of current concepts. Plant Dis. 87, 4–10. doi: 10.1094/PDIS.2003.87.1.4
Husen, E., Wahyudi, A. T., Suwanto, A., and Saraswati, R. (2008). Prospective use of 1-aminocyclopropane-1-carboxylate deaminase-producing bacteria for plant growth promotion and defense against biotic and abiotic stresses in peat-soil-agriculture. Microbiol Indonesia 2:2. doi: 10.5454/MI.2.3.2
Jia, M., Chen, L., Xin, H. L., Zheng, C. J., Rahman, K., Han, T., et al. (2016). A friendly relationship between endophytic fungi and medicinal plants: a systematic review. Front. Microbiol. 7:179220. doi: 10.3389/FMICB.2016.00906/BIBTEX
Johan, P. D., Ahmed, O. H., Omar, L., and Hasbullah, N. A. (2021). Phosphorus transformation in soils following co-application of charcoal and wood ash. Agronomy 11:2010. doi: 10.3390/agronomy11102010
Johnson, L. (2008). Iron and Siderophores in fungal–host interactions. Mycol. Res. 112, 170–183. doi: 10.1016/j.mycres.2007.11.012
Kaddes, A., Fauconnier, M.-L., Sassi, K., Nasraoui, B., and Jijakli, M.-H. (2019). Endophytic fungal volatile compounds as solution for sustainable agriculture. Molecules 24:1065. doi: 10.3390/molecules24061065
Kamana, S., Hemalatha, K., Chandanavineela, K., Kalyani, P., and Hemalatha, V. (2016). Endophytic fungi: as source of bioactive compound. World J. Pharm. Pharm. Sci. 5, 1026–1040.
Khan, R., Mujeebur, Z. H., Ahamad, F., and Haniph Shah, M. (2023). “Nematode problems in Rice and their sustainable management” in Nematode diseases of crops and their sustainable management. eds. M. Rahman Khan and M. Quintanilla (Academic Press, Cambridge, Massachusetts, United States: Elsevier), 133–166.
Kilani-Morakchi, S., Morakchi-Goudjil, H., and Sifi, K. (2021). Azadirachtin-based insecticide: overview, risk assessments, and future directions. Front Agron. 3:676208. doi: 10.3389/fagro.2021.676208
Killham, K. (1994). Soil ecology. Oxford Academic, Oxford, United Kingdom: Cambridge University Press.
Kiraly, L., Barna, B., and Király, Z. (2007). Plant resistance to pathogen infection: forms and mechanisms of innate and acquired resistance. J. Phytopathol. 155, 385–396.
Köhl, J., Kolnaar, R., and Ravensberg, W. J. (2019). Mode of action of microbial biological control agents against plant diseases: relevance beyond efficacy. Front. Plant Sci. 10:454982. doi: 10.3389/FPLS.2019.00845/BIBTEX
Korat, K., Dave, B. P., and Dube, H. C. (2001). Detection and chemical characterization of siderophores produced by certain fungi. Indian J. Microbiol. 41, 87–92.
Kumar, Ajay, Zhimo, Yeka, Biasi, Antonio, Salim, Shoshana, Feygenberg, Oleg, Wisniewski, Michael, et al. (2021). “Endophytic microbiome in the Carposphere and its importance in fruit physiology and pathology.” 73–88.
Kumari, N., and Srividhya, S. (2020). “Secondary metabolites and lytic tool box of Trichoderma and their role in plant health” in Molecular aspects of plant beneficial microbes in agriculture. eds. V. Sharma, R. Salwan, and L. K. Tawfeeq Al-Ani (Amsterdam: Elsevier), 305–320.
Kushwaha, P., Kashyap, P. L., Bhardwaj, A. K., Kuppusamy, P., Srivastava, A. K., and Tiwari, R. K. (2020). Bacterial endophyte mediated plant tolerance to salinity: growth responses and mechanisms of action. World J. Microbiol. Biotechnol. 36, 1–16. doi: 10.1007/S11274-020-2804-9/FIGURES/2
Lahlali, R., Ezrari, S., Radouane, N., Kenfaoui, J., Esmaeel, Q., El Hamss, H., et al. (2022). Biological control of plant pathogens: a global perspective. Microorganisms. MDPI 10:596. doi: 10.3390/microorganisms10030596
Landum, M. C., do Rosário Félix, M., Alho, J., Garcia, R., Cabrita, M. J., Rei, F., et al. (2016). Antagonistic activity of fungi of Olea Europaea L. against Colletotrichum acutatum. Microbiol. Res. 183, 100–108. doi: 10.1016/J.MICRES.2015.12.001
Latz, M. A. C., Jensen, B., Collinge, D. B., and Jørgensen, H. J. L. (2018). Endophytic fungi as biocontrol agents: elucidating mechanisms in disease suppression. Plant Eco. Diversity 11, 555–567. doi: 10.1080/17550874.2018.1534146
Lechenet, M., Dessaint, F., Py, G., Makowski, D., and Munier-Jolain, N. (2017). Reducing pesticide use while preserving crop productivity and profitability on arable farms. Nat. Plants 3, 1–6. doi: 10.1038/nplants.2017.8
Lee, H. Y., Chen, Y. C., Kieber, J. J., and Yoon, G. M. (2017). Regulation of the turnover of ACC synthases by phytohormones and heterodimerization in Arabidopsis. Plant J. 91, 491–504. doi: 10.1111/tpj.13585
Lee, B. N., Kroken, S., Chou, D. Y. T., Barbara Robbertse, O. C., Yoder,, and Turgeon, G. (2005). Functional analysis of all nonribosomal peptide synthetases in cochliobolus heterostrophus reveals a factor, NPS6, involved in virulence and resistance to oxidative stress. Eukaryot. Cell 4, 545–555. doi: 10.1128/EC.4.3.545-555.2005
Li, N., Han, X., Feng, D., Yuan, D., and Huang, L. J. (2019). Signaling crosstalk between salicylic acid and ethylene/jasmonate in plant defense: do we understand what they are whispering? Int. J. Mol. Sci. 20:671. doi: 10.3390/ijms20030671
Liu, Y., Bai, F., Li, T., and Yan, H. (2018). An endophytic strain of genus Paenibacillus isolated from the fruits of noni (Morinda Citrifolia L.) has antagonistic activity against a Noni’s pathogenic strain of genus aspergillus. Microb. Pathog. 125, 158–163. doi: 10.1016/J.MICPATH.2018.09.018
Lo, C-T, Nelson, E. B., and Harman, G. E. (2015). Improved biocontrol efficacy of Trichoderma harzianum 1295-22 for foliar phases of turf diseases by use of spray applications. Plant Dis. 81, 1132–1138.
Loper, J. E., and Buyer, J. S. (1991). Siderophores in microbial interactions on plant surfaces. Mol. Plant-Microbe Interact. 4, 5–13. doi: 10.1094/MPMI-4-005
Ludwig-Müller, J. (2015). Plants and endophytes: equal Partners in secondary metabolite production? Biotechnol. Lett. 37, 1325–1334. doi: 10.1007/s10529-015-1814-4
Lugtenberg, B. J. J., Caradus, J. R., and Johnson, L. J. (2016). Fungal endophytes for sustainable crop production. FEMS Microbiol. Ecol. 92:fiw194. doi: 10.1093/femsec/fiw194
Macías-Rubalcava, M. L., and Garrido-Santos, M. Y. (2022). Phytotoxic compounds from endophytic fungi. Appl. Microbiol. Biotechnol. 106, 931–950. doi: 10.1007/s00253-022-11773-w
Martin, M. N., Cohen, J. D., and Saftner, R. A. (1995). A new 1-aminocyclopropane-1-carboxylic acid-conjugating activity in tomato fruit. Plant Physiol. 109, 917–926. doi: 10.1104/pp.109.3.917
Matzanke, B. F., Bill, E., Trautwein, A. X., and Winkelmann, G. (1987). Role of siderophores in Iron storage in spores of Neurospora crassa and Aspergillus ochraceus. J. Bacteriol. 169, 5873–5876. doi: 10.1128/jb.169.12.5873-5876.1987
McMorran, B. J., Shantha Kumara, H. M. C., Sullivan, K., and Lamont, I. L. (2001). Involvement of a transformylase enzyme in siderophore synthesis in Pseudomonas Aeruginosa. Microbiology 147, 1517–1524. doi: 10.1099/00221287-147-6-1517
McNeely, D., Chanyi, R. M., Dooley, J. S., Moore, J. E., and Koval, S. F. (2017). Biocontrol of Burkholderia cepacia complex bacteria and bacterial phytopathogens by Bdellovibrio bacteriovorus. Can. J. Microbiol. 63, 350–358.
Mejía, L. C., Rojas, E. I., Maynard, Z., Sunshine Van Bael, A., Arnold, E., Hebbar, P., et al. (2008). Endophytic fungi as biocontrol agents of Theobroma Cacao pathogens. Biol. Control 46, 4–14. doi: 10.1016/j.biocontrol.2008.01.012
Molinari, S., and Leonetti, P. (2019). Bio-control agents activate plant immune response and prime susceptible tomato against root-knot nematodes. PLoS One 14:e0213230. doi: 10.1371/journal.pone.0213230
Morandi, M. A. B., Sutton, J. C., and Maffia, L. A. (2000). Effects of host and microbial factors on development of Clonostachys rosea and control of Botrytis cinerea in rose. Eur. J. Plant Pathol. 106, 439–448. doi: 10.1023/A:1008738513748
Naik, B. S. (2018). Volatile hydrocarbons from endophytic fungi and their efficacy in fuel production and disease control. Egyp. J. Biol. Pest Control 28:69. doi: 10.1186/s41938-018-0072-x
Nascimento, F. X., Rossi, M. J., Soares, C. R. F. S., McConkey, B. J., and Glick, B. R. (2014). New insights into 1-aminocyclopropane-1-carboxylate (ACC) deaminase phylogeny, evolution and ecological significance. PLoS One 9:e99168. doi: 10.1371/JOURNAL.PONE.0099168
Neilands, J B, Konopka, K, Schwyn, B, Coy, M, Francis, R T, Paw, B H, et al. (1987). “Comparative biochemistry of microbial Iron assimilation, Iron transport in microbes, plants and animals (G. Winkelmann, D. Helmvan Der, and JB Neilands, Eds.).” VCH, Weinheim, Germany.
Nguyen, H. T., Kim, S., Yu, N. H., Park, A. R., Yoon, H., Bae, C. H., et al. (2019). Antimicrobial activities of an oxygenated cyclohexanone derivative isolated from Amphirosellinia nigrospora JS-1675 against various plant pathogenic bacteria and fungi. J. Appl. Microbiol. 126, 894–904. doi: 10.1111/jam.14138
O’Sullivan, D. J., and O’Gara, F. (1992). Traits of fluorescent Pseudomonas Spp. involved in suppression of plant root pathogens. Microbiol. Rev. 56, 662–676. doi: 10.1128/mr.56.4.662-676.1992
Ogórek, R., Przywara, K., Piecuch, A., Cal, M., Lejman, A., and Matkowski, K. (2020). Plant–fungal interactions: a case study of Epicoccoum Nigrum link. Plan. Theory 9:1691. doi: 10.3390/plants9121691
Oide, S., Krasnoff, S. B., Gibson, D. M., and Gillian Turgeon, B. (2007). Intracellular Siderophores are essential for ascomycete sexual development in heterothallic Cochliobolus heterostrophus and homothallic Gibberella zeae. Eukaryot. Cell 6, 1339–1353. doi: 10.1128/EC.00111-07
Oide, S., Moeder, W., Krasnoff, S., Gibson, D., Haas, H., Yoshioka, K., et al. (2006). NPS6, encoding a nonribosomal peptide synthetase involved in siderophore-mediated iron metabolism, is a conserved virulence determinant of plant pathogenic. Plant Cell 18, 2836–2853. doi: 10.1105/tpc.106.045633
Oszust, K., Cybulska, J., and Frąc, M. (2020). How do Trichoderma genus Fungi Win a nutritional competition battle against soft fruit pathogens? A report on niche overlap nutritional potentiates. Int. J. Mol. Sci. 21:4235. doi: 10.3390/ijms21124235
Pal, K. K., and Gardener, B McSpadden. (2006). “Biological control of plant pathogens.” The Plant Health Instructor.
Palanichamy, P., Krishnamoorthy, G., Kannan, S., and Marudhamuthu, M. (2018). Bioactive potential of secondary metabolites derived from medicinal plant endophytes. Egyp. J. Basic Appl. Sci. 5, 303–312. doi: 10.1016/J.EJBAS.2018.07.002
Panda, K., and Sujogya,. (2013). Endophytic fungi with great promises: a review bio-prospecting of wild mushrooms view project insights into new strategies to combat biofilms view project. J. Adv. Pharm. Educ. Res. 3, 152–170.
Parker, M. A. (1995). Plant fitness variation caused by different mutualist genotypes. Ecology 76, 1525–1535. doi: 10.2307/1938154
Payment, P., Berte, A., Prévost, M., Ménard, B., and Barbeau, B. (2011). Occurrence of pathogenic microorganisms in the Saint Lawrence River (Canada) and comparison of health risks for populations using it as their source of drinking water. Can. J. Microbiol. 46, 565–576. doi: 10.1139/W00-022
Plaszkó, T., Szűcs, Z., Kállai, Z., Csoma, H., Vasas, G., and Gonda, S. (2020). Volatile organic compounds (VOCs) of endophytic Fungi growing on extracts of the host, horseradish (Armoracia Rusticana). Meta 10:451. doi: 10.3390/metabo10110451
Rahman, S. A., Farhana, S., Singh, E., Pieterse, C. M. J., and Schenk, P. M. (2018). Emerging microbial biocontrol strategies for plant pathogens. Plant Sci. 267, 102–111. doi: 10.1016/J.PLANTSCI.2017.11.012
Rajesh, R. W., Shelake Rahul, M., and Ambalal, N. S. (2016). Trichoderma: a significant fungus for agriculture and environment. Afr. J. Agric. Res. 11, 1952–1965. doi: 10.5897/AJAR2015.10584
Rani, R., Sharma, D., Chaturvedi, M., and Yadav, J. P. (2017). Antibacterial activity of twenty different endophytic fungi isolated from Calotropis procera and time kill assay. Clin. Microbiol. Open Access. 6:1000280. doi: 10.4172/2327-5073.1000280
Rashad, Y., Aseel, D., and Hammad, S. (2020). Phenolic compounds against fungal and viral plant diseases. Plant Phenolics Sustain. Agric. 1, 201–219. doi: 10.1007/978-981-15-4890-1_9
Raymaekers, K., Ponet, L., Holtappels, D., Berckmans, B., and Cammue, B. P. A. (2020). Screening for novel biocontrol agents applicable in plant disease management – a review. Biol. Control 144:104240. doi: 10.1016/J.BIOCONTROL.2020.104240
Reddy, S. G. E. (2020). Lecanicillium Spp. for the management of aphids, whiteflies, thrips, scales and mealy bugs. London, UK: IntechOpen.
Ren, C. G., and Dai, C. C. (2012). Jasmonic acid is involved in the signaling pathway for fungal endophyte-induced volatile oil accumulation of Atractylodes lancea plantlets. BMC Plant Biol. 12, 1–11. doi: 10.1186/1471-2229-12-128/FIGURES/5
Sahu, P. K., and Mishra, S. (2021). Effect of hybridization on endophytes: the Endo-microbiome dynamics. Symbiosis 84, 369–377. doi: 10.1007/s13199-021-00760-w
Sahu, G. K., and Sindhu, S. S. (2011). Disease control and plant growth promotion of green gram by siderophore producing Pseudomonas Sp. Res. J. Microbiol. 6, 735–749. doi: 10.3923/jm.2011.735.749
Saikkonen, K. (2007). Forest structure and fungal endophytes. Fungal Biol. Rev. 21, 67–74. doi: 10.1016/j.fbr.2007.05.001
Saikkonen, K., Wäli, P., Helander, M., and Faeth, S. H. (2004). Evolution of endophyte-plant symbioses. Trends Plant Sci. 9, 275–280. doi: 10.1016/j.tplants.2004.04.005
Santos, C. M. D., Ribeiro, A. D. S., Garcia, A., Polli, A. D., Polonio, J. C., Azevedo, J. L., et al. (2019). Enzymatic and antagonist activity of endophytic fungi from Sapindus Saponaria L. (sapindaceae). Acta Biol. Colombiana 24, 322–330. doi: 10.15446/abc.v24n2.74717
Santra, H. K., and Banerjee, D. (2023). Antifungal activity of volatile and non-volatile metabolites of endophytes of Chloranthus Elatior sw. Front. Plant Sci. 14:1156323. doi: 10.3389/fpls.2023.1156323
Savary, S., Willocquet, L., Pethybridge, S. J., Esker, P., Mcroberts, N., and Nelson, A. (2019). The global burden of pathogens and pests on major food crops. Nature 3, 430–439. doi: 10.1038/s41559-018-0793-y
Saxena, S., Meshram, V., and Kapoor, N. (2015). Muscodor Tigerii Sp. Nov.-volatile antibiotic producing endophytic fungus from the northeastern Himalayas. Ann. Microbiol. 65, 47–57. doi: 10.1007/s13213-014-0834-y
Sayyed, R. Z., Chincholkar, S. B., Reddy, M. S., Gangurde, N. S., and Patel, P. R. (2013). “Siderophore producing PGPR for crop nutrition and Phytopathogen suppression” in Bacteria in agrobiology: Disease management. ed. D. K. Maheshweri (Berlin, Heidelberg: Springer Berlin Heidelberg), 449–471.
Schippers, B., Bakker, A. W., and Bakker, P. A. H. M. (2003). Interactions of deleterious and beneficial rhizosphere microorganisms and the effect of cropping practices. Ann. Rev. 25, 339–358. doi: 10.1146/ANNUREV.PY.25.090187.002011
Schrettl, M., Bignell, E., Kragl, C., Sabiha, Y., Loss, O., Eisendle, M., et al. (2007). Distinct roles for intra- and extracellular Siderophores during Aspergillus Fumigatus infection. PLoS Pathog. 3:e128. doi: 10.1371/journal.ppat.0030128
Sefloo, G., Negar, S. S., and Hage-Ahmed, K. (2021). The bioprotective effect of root endophytic Serendipita Herbamans against fusarium wilt in tomato and its impact on root traits are determined by temperature. Rhizosphere 20:100453. doi: 10.1016/J.RHISPH.2021.100453
Selim, K. A., Elkhateeb, W. A., Tawila, A. M., El-Beih, A. A., Abdel-Rahman, T. M., El-Diwany, A. I., et al. (2018). Antiviral and antioxidant potential of fungal endophytes of Egyptian medicinal plants. Fermentation 4:49. doi: 10.3390/fermentation4030049
Sharma, S. B., Sayyed, R. Z., Trivedi, M. H., and Gobi, T. A. (2013). Phosphate solubilizing microbes: sustainable approach for managing phosphorus deficiency in agricultural soils. Springerplus 2, 1–14. doi: 10.1186/2193-1801-2-587/FIGURES/3
Sharma, Pooja, and Singh, Surendra Pratap. (2021). “Role of the endogenous fungal metabolites in the plant growth improvement and stress tolerance.” Fungi bio-prospects in sustainable agriculture, environment and nano-technology: volume 3: fungal metabolites, functional genomics and nano-technology, January, 381–401.
Shi, X., Qin, T., Liu, H., Wu, M., Li, J., Shi, Y., et al. (2020). Endophytic fungi activated similar defense strategies of Achnatherum sibiricum host to different trophic types of pathogens. Front. Microbiol. 11:1607. doi: 10.3389/fmicb.2020.01607
Shukla, S. T., Habbu, P. V., Kulkarni, V. H., Jagadish, K. S., Pandey, A. R., and Sutariya, V. N. (2014). Endophytic microbes: a novel source for biologically/pharmacologically active secondary metabolites. Asian J. Pharmacol. Toxicol. 2, 1–16.
Sikora, R. A., Schäfer, K., and Dababat, A. A. (2007). Modes of action associated with microbially induced in planta suppression of plant-parasitic nematodes. Australas. Plant Pathol. 36, 124–134. doi: 10.1071/AP07008
Silva, M. G., Santana, J., de Curcio, M., Silva-Bailão, G., Lima, R. M., Tomazett, M. V., et al. (2020). Molecular characterization of siderophore biosynthesis in Paracoccidioides Brasiliensis. IMA Fungus 11:11. doi: 10.1186/s43008-020-00035-x
Sindhu, S S, Suneja, S, and Dadarwal, K R. (1997). “Plant growth promoting rhizobacteria and their role in improving crop productivity.” Biotechnological approaches in soil microorganisms for sustainable crop production. 149–191.
Singh, V. K., and Kumar, A. (2023). Secondary metabolites from endophytic fungi: production, methods of analysis, and diverse pharmaceutical potential. Symbiosis 90, 111–125. doi: 10.1007/s13199-023-00925-9
Song, H. C., Qin, D., Liu, H. Y., Dong, J. Y., You, C., and Wang, Y. M. (2021). Resorcylic acid lactones produced by an endophytic Penicillium ochrochloron strain from Kadsura Angustifolia. Planta Med. 87, 225–235. doi: 10.1055/a-1326-2600
Sood, M., Kapoor, D., Kumar, V., Sheteiwy, M. S., Ramakrishnan, M., Landi, M., et al. (2020). Trichoderma: the ‘secrets’ of a multitalented biocontrol agent. Plan. Theory 9:762. doi: 10.3390/plants9060762
Staswick, P. E., and Tiryaki, I. (2004). The Oxylipin signal Jasmonic acid is activated by an enzyme that conjugates it to isoleucine in Arabidopsis. Plant Cell 16, 2117–2127. doi: 10.1105/tpc.104.023549
Stoytcheva, M. (2011). Pesticides in the modern world: Pesticides use and management. London, UK: BoD–Books on Demand.
Talbot, N. J. (2015). Plant immunity: a little help from fungal friends. Curr. Biol. 25, R1074–R1076. doi: 10.1016/j.cub.2015.09.068
Tripathi, S., Kamal, S., Sheramati, I., Oelmuller, R., and Varma, A. (2008). “Mycorrhizal Fungi and other root endophytes as biocontrol agents against root pathogens” in Mycorrhiza: state of the art, genetics and molecular biology, eco-function, biotechnology, eco-physiology, structure and systematics (third edition). ed. A. Varma (Berlin, Heidelberg: Springer), 281–306.
Trivedi, P., Leach, J. E., Tringe, S. G., Sa, T., and Singh, B. K. (2020). Plant–microbiome interactions: from community assembly to plant health. Nat. Rev. Microbiol. 18, 607–621. doi: 10.1038/s41579-020-0412-1
Tyśkiewicz, R., Nowak, A., Ozimek, E., and Jaroszuk-Ściseł, J. (2022). ‘Trichoderma: the current status of its application in agriculture for the biocontrol of fungal phytopathogens and stimulation of plant growth’, J. Mol. Sci. 23:2329. doi: 10.3390/IJMS23042329
Ullah, A., Nisar, M., Ali, H., Hazrat, A., Hayat, K., Keerio, A. A., et al. (2019). Drought tolerance improvement in plants: an endophytic bacterial approach. Appl. Microbiol. Biotechnol. 103, 7385–7397. doi: 10.1007/S00253-019-10045-4
Van de Poel, B., Smet, D., and Van Der Straeten, D. (2015). Ethylene and hormonal cross talk in vegetative growth and development. Plant Physiol. 169, 61–72. doi: 10.1104/pp.15.00724
Van Loon, L. C., Bakker, P. A. H. M., and Pieterse, C. M. J. (1998). “Systemic resistance induced by rhizosphere bacteria.” Annu. Rev. Phytopathol. 36, 453–483. doi: 10.1146/annurev.phyto.36.1.453
Vishwas, Mohite Bhavana. (2011). “A review: Natural products from plant associated endophytic Fungi wound healing agents of plant origin view project Nephroprotective plants view project.” Available at: https://www.researchgate.net/publication/258884981.
Waghunde, Rajesh Ramdas, Shelake, Rahul Mahadev, Shinde, Manisha S., and Hayashi, Hidenori. (2017). “Endophyte microbes: a weapon for plant health management.” 303–25.
Wang, G., Liu, Z., Lin, R., Li, E, Mao, Z., Ling, J., et al. (2016). Biosynthesis of antibiotic leucinostatins in bio-control fungus purpureocillium lilacinum and their inhibition on phytophthora revealed by genome mining. PLOS Pathogens. 12:e1005685. doi: 10.1371/journal.ppat.1005685
Wang, Y. J., Deering, A. J., and Kim, H. J. (2021). Effects of plant age and root damage on internalization of Shiga toxin-producing Escherichia coli in leafy vegetables and herbs. Horticulturae 7:68. doi: 10.3390/horticulturae7040068
Wang, Y., Pruitt, R. N., Nürnberger, T., and Wang, Y. (2022). Evasion of plant immunity by microbial pathogens. Nat. Rev. Microbiol. 20, 449–464. doi: 10.1038/s41579-022-00710-3
Wen, J., Okyere, S. K., Wang, J., Huang, R., Wang, Y., Liu, L., et al. (2023). Endophytic Fungi isolated from Ageratina Adenophora exhibits potential antimicrobial activity against multidrug-resistant Staphylococcus Aureus. Plan. Theory 12:650. doi: 10.3390/plants12030650
White, J. F., and Torres, M. S. (2010). Is plant endophyte-mediated defensive mutualism the result of oxidative stress protection? Physiol. Plant. 138, 440–446. doi: 10.1111/j.1399-3054.2009.01332.x
Wicklow, D. T., and Poling, S. M. (2008). Antimicrobial activity of Pyrrocidines from Acremonium Zeae against endophytes and pathogens of maize. Phytopathology 99, 109–115. doi: 10.1094/PHYTO-99-1-0109
Wilson, A., Cuddy, W. S., Park, R. F., Harm, G. F. S., Priest, M. J., Bailey, J., et al. (2020). Investigating Hyperparasites as potential biological control agents of rust pathogens on cereal crops. Australas. Plant Pathol. 49, 231–238. doi: 10.1007/s13313-020-00695-8
Win, T. T., Bo, B., Malec, P., Khan, S., and Pengcheng, F. (2021). Newly isolated strain of trichoderma asperellum from disease suppressive soil is a potential bio-control agent to suppress fusarium soil borne fungal phytopathogens. J. Plant Pathol. 103, 549–561. doi: 10.1007/s42161-021-00780-x
Winkelmann, G., and Drechsel, H. (2008). “Microbial Siderophores” in Biotechnology, second, completely revised edition, volume 7: Products of secondary metabolism. eds. H. J. Rehm and G. Reed (Hoboken, New Jersey, United States: Wiley), 199–246.
Wu, W., Chen, W., Liu, S., Jianjun, W., Zhu, Y., Qin, L., et al. (2021). Beneficial relationships between endophytic Bacteria and medicinal plants. Front. Plant Sci. 12:646146. doi: 10.3389/FPLS.2021.646146/BIBTEX
Wu, Y. Y., Zhang, T. Y., Zhang, M. Y., Cheng, J., and Zhang, Y. X. (2018). An endophytic Fungi of Ginkgo Biloba L. produces antimicrobial metabolites as potential inhibitors of FtsZ of Staphylococcus Aureus. Fitoterapia 128, 265–271. doi: 10.1016/J.FITOTE.2018.05.033
Xia, Y., Liu, J., Chen, C., Mo, X., Tan, Q., He, Y., et al. (2022). The multifunctions and future prospects of endophytes and their metabolites in plant disease management. Microorganisms 10:1072. doi: 10.3390/microorganisms10051072
Xie, J., Ying Ying, W., Zhang, T. Y., Zhang, M. Y., Peng, F., Lin, B., et al. (2018). New antimicrobial compounds produced by endophytic Penicillium Janthinellum isolated from Panax Notoginseng as potential inhibitors of FtsZ. Fitoterapia 131, 35–43. doi: 10.1016/J.FITOTE.2018.10.006
Yao, X., Guo, H., Zhang, K., Zhao, M., Ruan, J., and Chen, J. (2023). Trichoderma and its role in biological control of plant fungal and nematode disease. Front. Microbiol. 14:1160551. doi: 10.3389/fmicb.2023.1160551
Youssef, K., Ippolito, A., and Roberto, S. R. (2022). Editorial: post-harvest diseases of fruit and vegetable: methods and mechanisms of action. Front. Microbiol. 13:900060. doi: 10.3389/fmicb.2022.900060
Yu, X., Ai, C., Xin, L., and Zhou, G. (2011). The Siderophore-producing bacterium, Bacillus Subtilis CAS15, has a biocontrol effect on fusarium wilt and promotes the growth of pepper. Eur. J. Soil Biol. 47, 138–145. doi: 10.1016/j.ejsobi.2010.11.001
Yu, J., Ying, W., He, Z., Li, M., Zhu, K., and Gao, B. (2018). Diversity and antifungal activity of endophytic fungi associated with Camellia oleifera 46, 85–91. doi: 10.1080/12298093.2018.1454008,
Yuan, W. M., Gentil, G. D., Budde, A. D., and Leong, S. A. (2001). Characterization of the Ustilago Maydis Sid2 gene, encoding a multidomain peptide Synthetase in the Ferrichrome biosynthetic gene cluster. J. Bacteriol. 183, 4040–4051. doi: 10.1128/JB.183.13.4040-4051.2001
Keywords: fungal endophyte, biocontrol mechanism, secondary metabolites, sustainable agriculture, bioactive potential
Citation: Bhardwaj M, Kailoo S, Khan RT, Khan SS and Rasool S (2023) Harnessing fungal endophytes for natural management: a biocontrol perspective. Front. Microbiol. 14:1280258. doi: 10.3389/fmicb.2023.1280258
Edited by:
Mina Salehi, Tarbiat Modares University, IranReviewed by:
Debdulal Banerjee, Vidyasagar University, IndiaNakkeeran S., Tamil Nadu Agricultural University, India
Tariq Mukhtar, Pir Mehr Ali Shah Arid Agriculture University, Pakistan
Copyright © 2023 Bhardwaj, Kailoo, Khan, Khan and Rasool. This is an open-access article distributed under the terms of the Creative Commons Attribution License (CC BY). The use, distribution or reproduction in other forums is permitted, provided the original author(s) and the copyright owner(s) are credited and that the original publication in this journal is cited, in accordance with accepted academic practice. No use, distribution or reproduction is permitted which does not comply with these terms.
*Correspondence: Shafaq Rasool, c2hhZmFxLnJhc29vbEBzbXZkdS5hYy5pbg==
†These authors have contributed equally to this work