- 1Department of Entomology and Plant Pathology, University of Tennessee, Knoxville, TN, United States
- 2School of Mathematical and Natural Sciences, Arizona State University, Glendale, AZ, United States
- 3Department of Biological Sciences, Simon Fraser University, Burnaby, BC, Canada
Medicinal and recreational uses of Cannabis sativa, commonly known as cannabis or hemp, has increased following its legalization in certain regions of the world. Cannabis and hemp plants interact with a community of microbes (i.e., the phytobiome), which can influence various aspects of the host plant. The fungal composition of the C. sativa phytobiome (i.e., mycobiome) currently consists of over 100 species of fungi, which includes phytopathogens, epiphytes, and endophytes, This mycobiome has often been understudied in research aimed at evaluating the safety of cannabis products for humans. Medical research has historically focused instead on substance use and medicinal uses of the plant. Because several components of the mycobiome are reported to produce toxic secondary metabolites (i.e., mycotoxins) that can potentially affect the health of humans and animals and initiate opportunistic infections in immunocompromised patients, there is a need to determine the potential health risks that these contaminants could pose for consumers. This review discusses the mycobiome of cannabis and hemp flowers with a focus on plant-infecting and toxigenic fungi that are most commonly found and are of potential concern (e.g., Aspergillus, Penicillium, Fusarium, and Mucor spp.). We review current regulations for molds and mycotoxins worldwide and review assessment methods including culture-based assays, liquid chromatography, immuno-based technologies, and emerging technologies for these contaminants. We also discuss approaches to reduce fungal contaminants on cannabis and hemp and identify future research needs for contaminant detection, data dissemination, and management approaches. These approaches are designed to yield safer products for all consumers.
1. Introduction
Cannabis sativa is a highly domesticated plant species that has been bred for hundreds of years to develop genotypes (i.e., strains) that are cultivated worldwide for medicinal, therapeutic, and recreational properties, as well as for grain, seed, and fiber [Krane, 2020; United States Department of Agriculture (USDA), 2020; Rafei et al., 2023]. The inflorescence tissues (which are also referred to as flowers or buds) are of significant economic value, since they can be used as dried flowers and as raw materials for cannabinoid extraction [United States Department of Agriculture-Economics, Statistics and Market Information System (USDA ESMIS), 2023]. The legal classification of genotypes depends upon content of Δ9-tetrahydrocannabinol (THC) content in the tissues, with cannabis containing levels greater than 0.3% (by dry weight) and hemp containing less than 0.3% Δ9-THC (Williams and Buchert, 2020). Cannabis and hemp can be used in a variety of ways, including as dried flowers that are smoked, inhaled, or vaped; flowers that have undergone an extraction process for cannabinoids; and formulated concentrates that include capsules, gels, creams, suppositories, and tinctures.
The mycobiome of cannabis and hemp is defined as the totality of fungal communities associated with various parts of the plant. The mycobiome is a subset of the phytobiome, which includes bacteria, phytoplasmas, viruses, and viroids that are beyond the scope of this review (Trivedi et al., 2020; Wei et al., 2021). Mycobiomes have garnered considerable recent interest within the scientific community, because they are present in virtually every plant species (Trivedi et al., 2020). Their interactions with host plants can lead to beneficial, neutral, or detrimental interactions, and conversely plant chemical composition can influence the mycobiome (Ranjith et al., 2021). Fungi that occur on the inflorescence tissues of cannabis and hemp as phytopathogens, surface entities (epiphytes) or as internal endophytes have the potential to produce toxic secondary metabolites (mycotoxins) and a few are pathogenic to humans (see Section 2 below). These fungi pose concerns for human health as they can result in opportunistic infections (i.e., mycoses) and cause toxicological effects. For the purposes of this review, the assemblage of fungi and their toxins from these sources found on cannabis and hemp are referred to as “contaminants.”
Although fungi and mycotoxins are common and well-studied contaminants in many agricultural crop species, they have been generally under-studied in cannabis and hemp. This is partly because human health risk assessment methodologies used to regulate food and pharmaceuticals have yet to become standard for the emerging cannabis and hemp industries. Additionally, the wide range of consumer uses of cannabis and hemp flowers, including for medical use by patients with susceptible conditions, makes it uniquely challenging to assess and manage human health risk of these contaminants. This review will discuss the relative importance of the floral mycobiome of cannabis and hemp emphasizing its potential to impact consumer health. We will assess the potential of cannabis and hemp contaminants to affect consumers of cannabis, discuss regulatory considerations and management practices, and highlight areas for future research.
2. Mycobiome of Cannabis sativa: phytopathogens, endophytes, and epiphytes
Fungal organisms comprising the mycobiome belong to the Kingdom Mycota and are characterized by a number of unique features, including: (i) the ability to grow rapidly on an appropriate substrate; (ii) growth over a wide range of temperatures; (iii) production of enzymes and toxins that destroy plant substrates; (iv) production of prolific numbers of asexual propagules (spores, conidia) that are easily disseminated by air and water; and (v) production of mycotoxins potentially harmful to humans and animals. The presence of fungal organisms in cannabis plants is not unique since many species comprising the floral mycobiome are also abundantly present across a wide range of agricultural crops. From the perspective of consumer health, the destruction of plant tissues and the production of spores and mycotoxins in association with cannabis and hemp plants are deemed of potential concern.
The cannabis and hemp mycobiomes can be subdivided into three components: phytopathogens, endophytes, and epiphytes (Figure 1). They are distinguished by where they are found on the plants and whether they cause visible damage to the plant (Figure 2). Phytopathogens cause visible disease symptoms on these plants, endophytes reside within plant tissues without causing discernable symptoms, while the epiphytes reside and grow on the outside of plant tissues and may or may not cause symptoms. According to United States National Fungus Collections and Fungal Database (NFCFD) (2023), more than 100 species of phytopathogens, epiphytes, and endophytes are associated with cannabis and hemp. The composition of this mycobiome can be influenced by host plant genotype, growth stage of the plant, external environment, and cultural practices used during commercial production (Barnett et al., 2020; Comeau et al., 2020; Punja et al., 2023). In particular, the floral mycobiome is impacted by the host genotype, host chemistry, growing environment, and pre-and post-harvest handling practices (Punja et al., 2023). Thus, the floral mycobiome of cannabis and hemp is a dynamic biological entity that is greatly influenced by plant genotype × environment interactions (Punja et al., 2023). This makes predictions and assessments of fungal population levels challenging, which can further complicate efforts to assess their potential impact on consumer health.
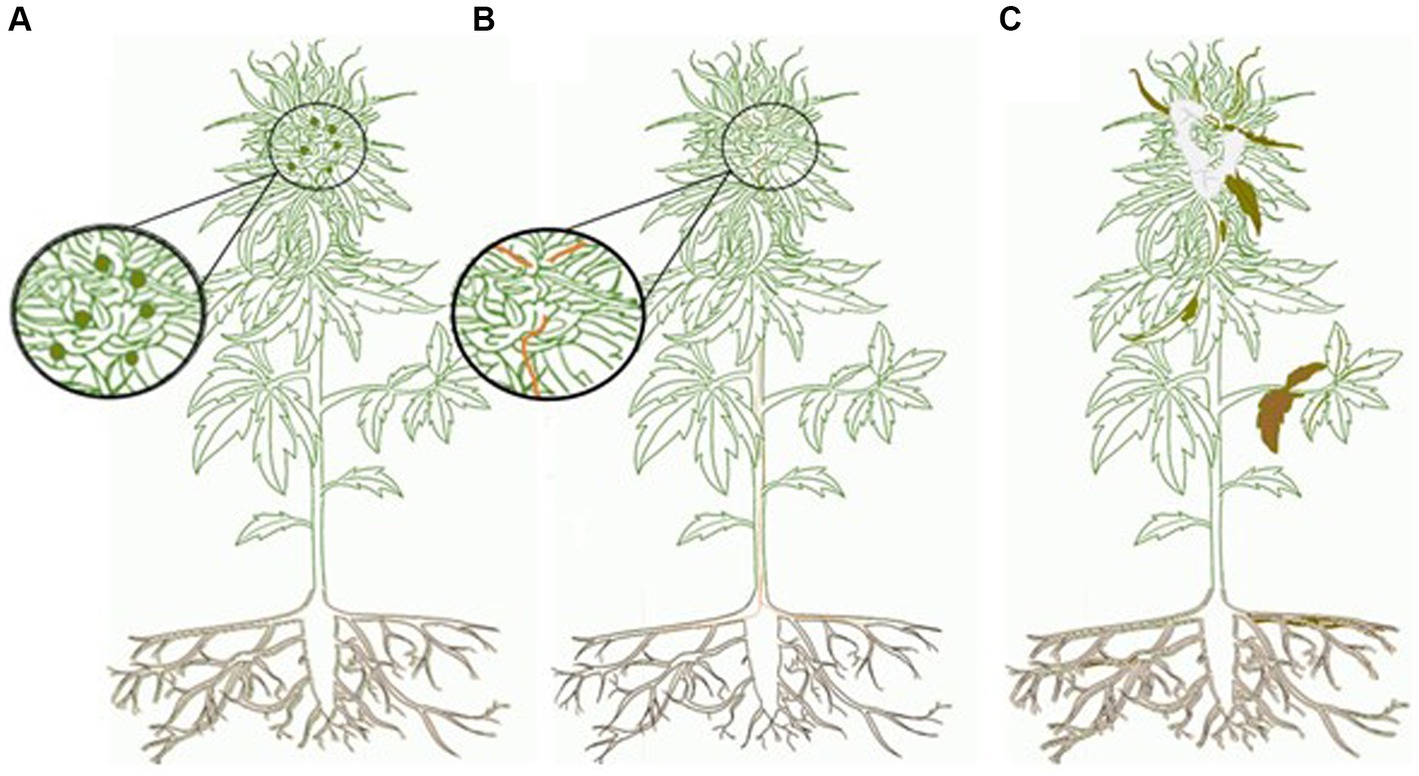
Figure 1. Mycobiome of cannabis and hemp. (A) Epiphyte—asymptomatic relationship with fungal propagules (dark green circles) that colonize only the outside of host plant. Propagules can only be observed with magnification. (B) Endophyte—asymptomatic relationship with fungal propagules (orange) that are found within the host. Endophytes can only be seen with dissection of the host and magnification. They may be beneficial to the plant or with an alteration in host physiology may became pathogenic. (C) Phytopathogen—symptomatic relationship that alters host physiology; signs (pathogen propagules—white) and symptoms (brown) may be seen without magnification. Cannabis graphic created by Maya Albert.
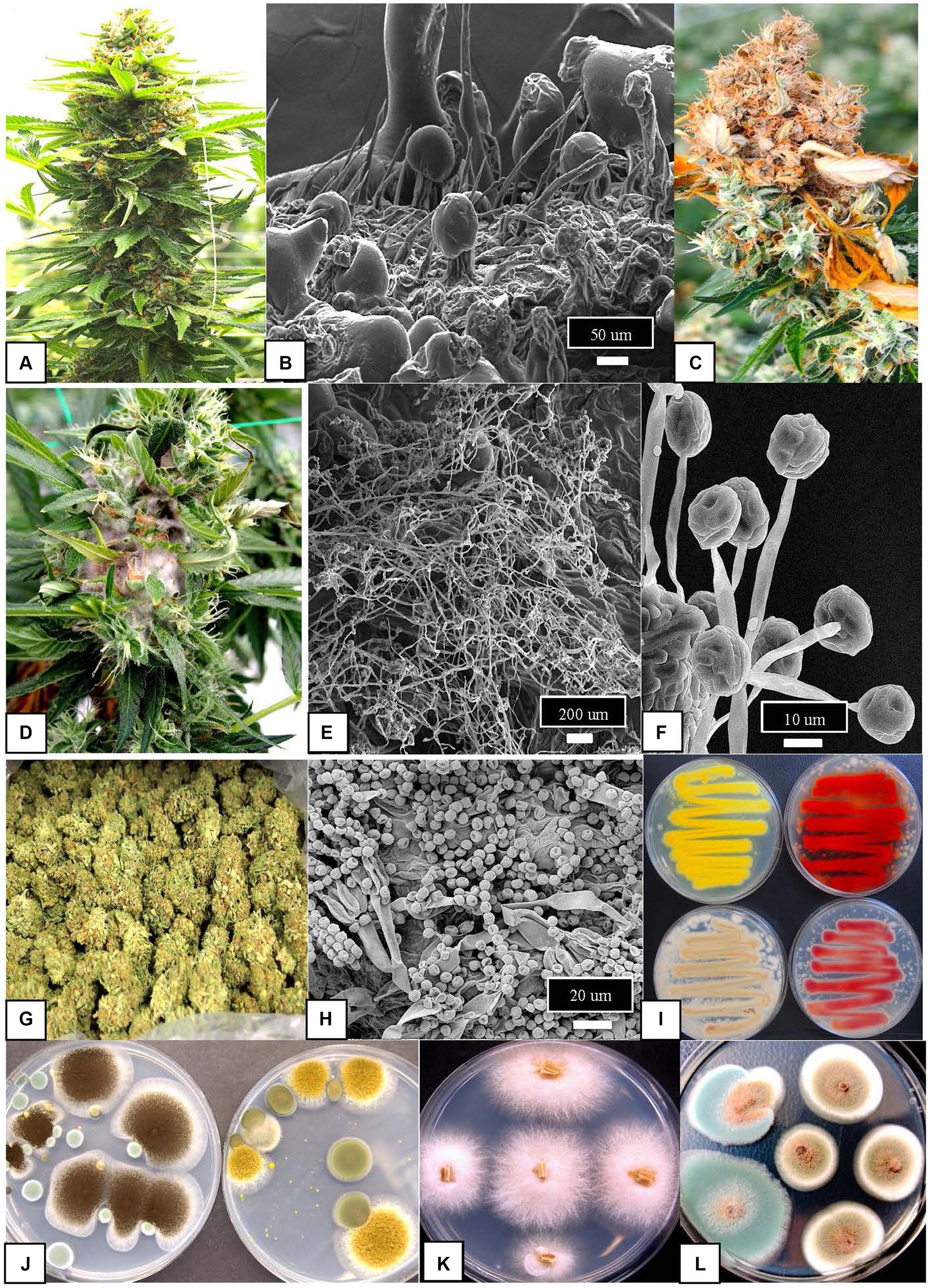
Figure 2. Fungal pathogens, epiphytes, and endophytes affecting cannabis inflorescences. (A) Cannabis inflorescence at maturation showing the large floral structure that is composed of female reproductive organs (pistils) and inflorescence leaves and bracts surrounding them. (B) Scanning electron micrograph of the surface of one of the bracts showing the abundance of glandular trichomes and non-glandular hairs among which fungal populations may reside. (C) Destruction of the inflorescence by the fungal pathogen Botrytis cinerea that causes browning and death of the tissues. (D) Proliferation of mycelium of a Fusarium species within the inflorescence tissues under conditions of high humidity. (E) Scanning electron micrograph showing growth of mycelium of a Trichoderma species on inflorescence tissues following a foliar application made to the plant. (F) Spore bearing structures of a Gliocladium species on inflorescence tissues following a foliar application made to the plant. In (E,F), tissues were collected 5 days following application for observation. (G) Commercially dried cannabis inflorescences prior to packaging. (H) Spores of Penicillium species commonly observed on the surface of cannabis samples as an epiphyte either during or after the drying process. (I) Swabs taken from dried cannabis samples and streaked onto potato dextrose agar shows the diversity of Penicillium species growing on the medium and producing a range of pigments. (J) Growth of two Aspergillus species on potato dextrose agar from swabs taken from dried cannabis samples where they were present as contaminants. On the left dish is A. niger (black) and on the right Aspergillus ochraceus (yellow). (K) Colonies of Fusarium oxysporum emerging from stem pieces of cannabis plants. (L) Colonies of two Penicillium species emerging from sections of stems where they were growing as endophytes.
The floral mycobiome of cannabis and hemp plants discussed above is important for several reasons: (i) it can impact the quality of the final product; (ii) it can have an impact on consumer health; and (ii) it can pose regulatory challenges that can vary depending on the jurisdiction. Examples of fungal species associated with cannabis inflorescences and their relative abundance are summarized in Table 1. A similar study has not been conducted in hemp. In addition, the presence of airborne fungal contaminants during harvesting of cannabis and hemp and those that are present in the cultivation environment can be of potential concern for human health (Martyny et al., 2013; Vanhove et al., 2018; Root et al., 2020; Punja, 2021c; Punja and Scott, 2023). Lastly, a few fungal species of cannabis and hemp can directly impact human health by causing tissue infections, resulting in mycoses for immunocompromised individuals (see Section 3.1). For example, after inhalation of spores, these fungi can enter nasal passages and the lungs where they may cause lung infections, particularly in immunocompromised patients [National Academies of Sciences, Engineering, and Medicine (NASEM), 2017; Benedict et al., 2020].
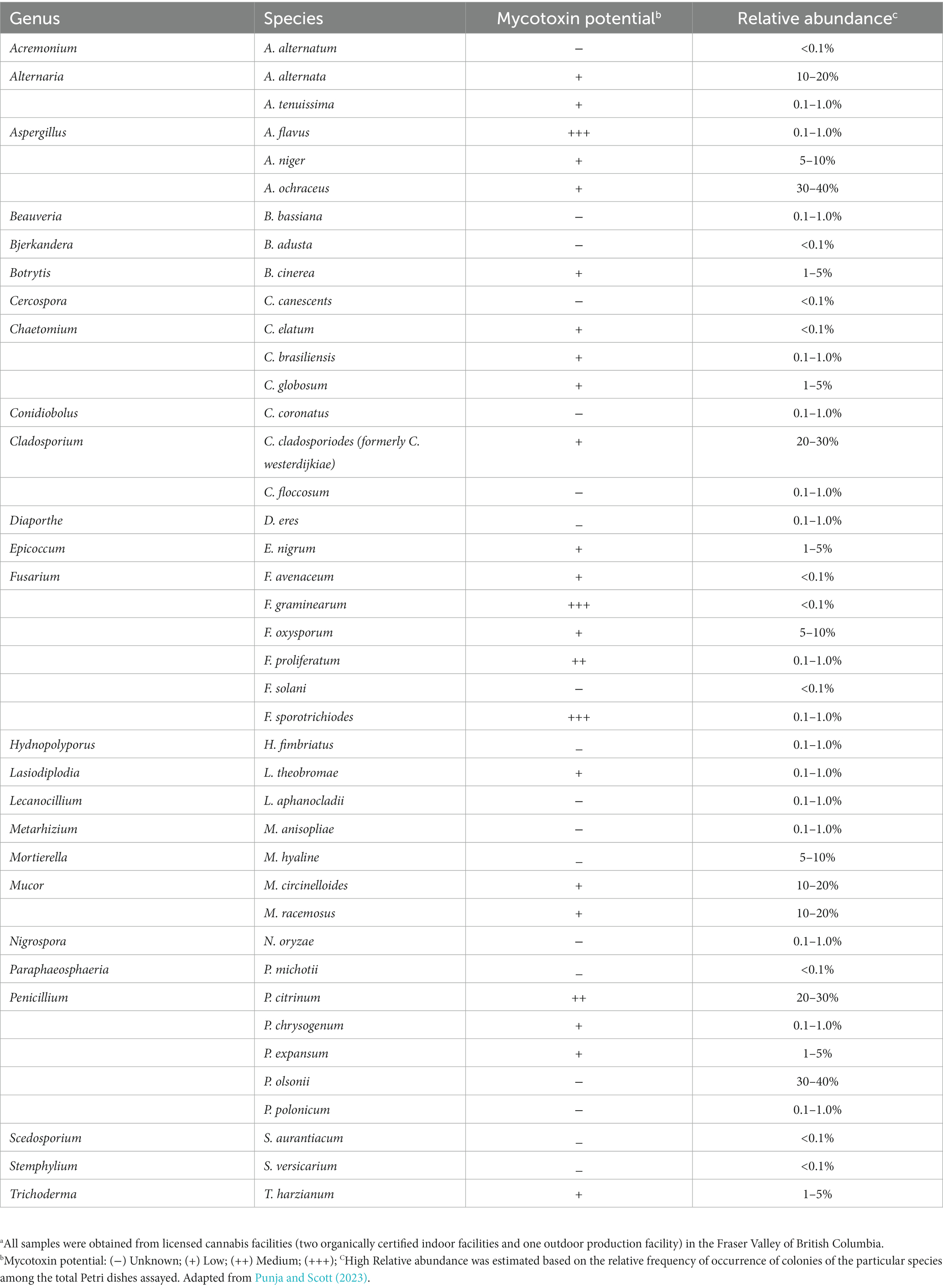
Table 1. The mycobiome of cannabis inflorescences and fungal species relative abundance in samples.a
2.1. Phytopathogens of Cannabis sativa
Just like most agricultural crops, cannabis and hemp plants are grown in cultivation systems that achieve efficiency of production at reduced cost; monoculture conditions are typical for greenhouse or indoor environments or field conditions, depending on the crop. The potential sources and levels of fungal contaminants may vary depending on these environmental factors, but data are lacking on the specific similarities or differences. Hence, the quality of the cannabis and hemp products could be influenced by the specific growing environment. A large number of fungal phytopathogens are known to infect the roots, stems, leaves and inflorescences of cannabis, and hemp plants both indoors and outdoors [Punja, 2021a; Gauthier and Thiessen, 2022; United States National Fungus Collections and Fungal Database (NFCFD), 2023]. In addition, fungi considered to be epiphytes and endophytes which cause no obvious symptoms in plants have been characterized and are common in stems and roots (Figures 1, 2). Furthermore, plant organs (roots, stems, leaves, and flowers) vary in the community structure of their mycobiomes and plant pathogens (Barnett et al., 2020; Comeau et al., 2020). For example, plants grown in soil and soilless media used for indoor production may harbor different endophytic and phytopathogenic fungi (Punja and Scott, 2023). The origins of these fungi can be traced back to the growing medium used, source of plant propagation materials, or are present externally in the ambient environment. These latter populations would constitute one potential source of contaminants on cannabis inflorescences (Punja et al., 2023). Therefore, an understanding of the myriad of potential sources of contaminants on cannabis and hemp inflorescences can have an important bearing on the quality of products derived from them. This information becomes important for consumers and identifying any potential health risks.
2.2. Endophytes of Cannabis sativa
Endophytes in cannabis and hemp can be latent phytopathogens, mutualists, or commensals (Alam et al., 2021). A number of endophytes that are latent pathogens can be present as pre-and post-harvest contaminants of cannabis inflorescences (Table 1). In mutualistic responses in other plants, fungal endophytes may improve plant health by modulating responses to biotic stresses (phytopathogens and pests; Ball et al., 2006; Ownley et al., 2010; Ball et al., 2011) and abiotic factors (Kristy et al., 2022). Endophytes can produce antimicrobial compounds that may render them beneficial in the plant (Taghinasab and Jabaji, 2020; Mishra et al., 2022). There is, however, currently little published data to support these roles in cannabis or hemp plants. Paradoxically, some endophytes may also produce secondary metabolites that are harmful to humans and other animals (i.e., mycotoxins; see Section 3.3). Translocation of these mycotoxins within the plant to distal tissues, including inflorescences, has been demonstrated for other crops (Snigdha et al., 2015; Pecoraro et al., 2018; Jaster-Keller et al., 2023), but to the best of our knowledge, translocation of mycotoxins within cannabis and hemp has not been demonstrated. A secondary metabolite produced by the endophyte, Sarocladium zeae, inhibited biosynthesis of the mycotoxin, fumonsin, by Fusarium verticillioides, a phytopathogen that coexists with S. zea in corn seed (Gao et al., 2020). Therefore, the potential negative impacts of endophytic fungi on human health remain unknown. During post-harvest operations, however, the release of endophytic spores that can colonize the tissues of cannabis inflorescences can lead to potential increases in fungal populations that negatively influence product quality and potentially could impact consumer health (Punja et al., 2019).
2.3. Epiphytes of Cannabis sativa
Cannabis and hemp inflorescences provide unique ecological niches for fungal growth. Temperature and relative humidity conditions are higher within these tissues compared to the ambient environment, which provides a more conducive environment for fungal growth (Punja et al., 2023); however, growth may be restricted by the presence of certain terpenes in these tissues (Ranjith et al., 2021). Epiphytes on cannabis and hemp inflorescences may originate from populations of endophytes that are released from internal tissues, such as stems, during post-harvest processing of inflorescences (Punja et al., 2019). Damaged tissues can also be colonized by fungal spores that are released from harvested tissues or are present in the ambient environment during crop production. Release of nutrient compounds that favor fungal growth has not been established and would depend on the genotype of the plant. These epiphytes can grow and produce spores on damaged inflorescence tissues under favorable ambient conditions that include high temperatures and relative humidity. If sufficient growth is achieved, the potential for mycotoxin production is increased. Spores of epiphytic fungi such as Penicillium and Aspergillus species can also be observed to stick to the surface of the glandular trichome heads (Figure 2), which are produced in abundance on the inflorescence bract tissues of cannabis where terpene concentration are also high. In organic cannabis production facilities, populations of air-borne fungal propagules originating from the soil may be high, and a greater prevalence of species of fungal epiphytes has been reported on cannabis inflorescences than in conventional culture (Punja and Scott, 2023). In addition, fungal spores may be observed stuck to the surface of trichome glands or are embedded in the sticky resin, potentially enhancing these populations (Punja and Scott, 2023). The predominant terpenes found in cannabis inflorescences, namely myrcene, β-caryophyllene, limonene, α-terpinene, and α-pinene (Chacon et al., 2022) may play a role in the community structure of floral mycobiomes. Many of these compounds are shown to be fungistatic and inhibit growth of toxigenic fungi under laboratory conditions (Karpiński, 2020; Cai et al., 2022; Jiang et al., 2023). Antifungal activity of terpene essential oils may also be due to synergistic interactions of compounds. Nanoemulsions composed of terpenes of hops (Humulus lupulus) in which terpene profiles are similar to those in cannabis and hemp, inhibited mycelial growth, spore germination, and mycotoxin production (deoxynivalenol (DON) and its derivatives) by F. graminearum (Jiang et al., 2023). Complex resin composed of terpenes reduced germination of two nonpathogenic species but not that of a phytopathogen (Slinski et al., 2015). These data suggest that terpenes in various cannabis and hemp genotypes and chemotypes may limit development of some epiphytic Aspergillus and Penicillium species. More research is needed to show whether fungistasis can be overcome under conducive environmental conditions. Also, there is presently little information as to whether cannabis or hemp plants can regulate the extent of endophytic or epiphytic colonization of the inflorescence through recruitment or exclusion of microbes. This has potential significance to human health as selection for lower fungal population levels can lead to a higher quality product.
3. Potential health hazards of fungal contaminants in cannabis
3.1. Health impacts for patients with susceptible conditions
Contaminant fungi pose a recognized health risk to patients with susceptible conditions, such as those who are immunocompromised [National Academies of Sciences, Engineering, and Medicine (NASEM), 2017; Figure 3]. These fungi can cause opportunistic infections on skin and lung tissues, which could lead to life-threatening conditions. The exposure route, dosage, and frequency of cannabis and hemp use can determine the potential health effects of fungal contaminants on consumers. In patients, fungal infections were mostly associated with the smoking of cannabis (89%) and were least common for edibles (4%; Levi et al., 2019). Although the heating conditions during smoking and vaping should kill fungal spores, they may be insufficient to render all spores nonviable (Kurup et al., 1983; Szyper-Kravitz et al., 2001). At the same time, these pathogens can gain access to the airway passages as aerosols. For example, species of Mucorales (e.g., Mucor) can be found in cannabis inflorescences (Stone et al., 2019; Punja and Scott, 2023). These fungi release spores into the environment where they remain airborne until they enter the body through inhalation. These fungi can result in opportunistic infections on skin and lung tissues, which can lead to life-threatening conditions (see Section 3.2).
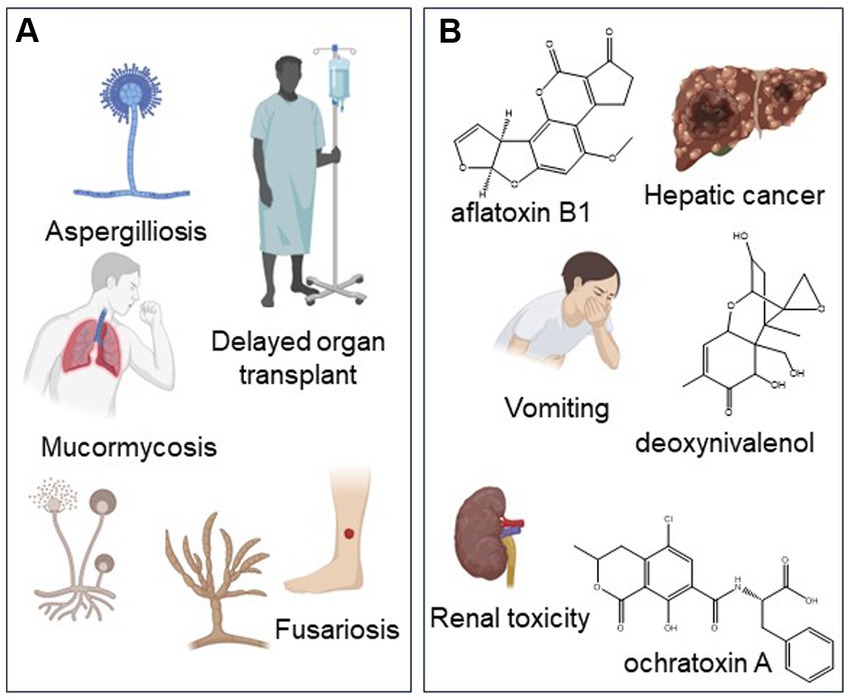
Figure 3. Health risks of fungal and mycotoxin contaminants to cannabis and hemp users. (A) Adverse health effects of fungal pathogens. Aspergillus was the most common organism associated with infection of cannabis users. (B) Adverse health risks of mycotoxins. Created with BioRender.com.
Cancer is another susceptible medical condition that can increase a patients’ risk of opportunistic infection. In 29 out of 30 US states and Washington D.C. that have legalized cannabis for recreational use, cancer is listed as a qualifying condition for medical cannabis use (Jameson et al., 2022). Cancer patients are often treated with chemotherapeutic drugs that result in compromised immunological functions. Consequently, oncologists have expressed concerns over the use of cannabis and cannabis-based products in cancer care, which is administered to improve the appetite of patients and manage symptoms of nausea, vomiting, and pain (Abrams, 2022). Several reports have shown that pulmonary aspergillosis developed in patients with malignancies or other immunocompromised states following smoking of cannabis [National Academies of Sciences, Engineering, and Medicine (NASEM), 2017]. If medical use of cannabis becomes more widely accepted, contaminant fungi represent a potential concern in public health.
Transplant surgeons have expressed concern about the use of cannabis by their patients (Levi et al., 2019; Ryan et al., 2019; Olt et al., 2021). In a study involving 52,689 Medicare kidney transplant recipients, those with post-transplant cannabis dependence or abuse (CDOA) were associated with a higher rate of all-cause graft failure (26.8 vs. 12.6%) and death (10.6 vs. 7.9%) occurring 1–3 years post-transplant as opposed to those without CDOA (Alhamad et al., 2019). Cannabis usage was associated with fungal infections in kidney transplant patients and an increased rate of aspiration pneumonia (4.3 vs. 1.1%) and other forms of pneumonia (18.3 vs. 10.6%; Alhamad et al., 2019; Olt et al., 2021). Aspergillus was the most common organism followed by Mucorales (includes Mucor spp.) responsible for these infections (Levi et al., 2019; Olt et al., 2021). The Canadian Cardiovascular Society/Canadian Cardiac Transplant Network has made a strong recommendation for a 6-month abstinence from cannabis use before heart transplant (Chih et al., 2020). Cannabidiol has been studied as a potential preventative agent to reduce COVID-19 viral load and to suppress cytokine storms related to viremia (Nguyen et al., 2022). COVID 19 patients are also immunocompromised and 20–30% of them get aspergillosis (Kuehn, 2021; Lin et al., 2022; Feys et al., 2023). Given the prevalence of COVID 19 infection, fungal contaminants in cannabis and hemp can be important public health concern in preventative use.
3.2. Fungal pathogens directly affecting humans
A number of studies have examined the allergenic responses to Aspergillus spp. and aspergillosis associated with cannabis use. Trullas et al. (2010) reviewed seven case reports of aspergillosis in cannabis smokers. Cases not reported in Trullas et al. (2010) are summarized in Table 2. Pulmonary aspergillosis is a common form of Aspergillus infection and has been reported in cannabis users with HIV and type 1 diabetes (Remington et al., 2015; Salam and Pozniak, 2017). Aspergillus fumigatus is a common species associated with aspergillosis in cannabis users (Table 2). It was also cultured from the cannabis products used by patients with aspergillosis (Kagen et al., 1983; Kouevidjin et al., 2003).
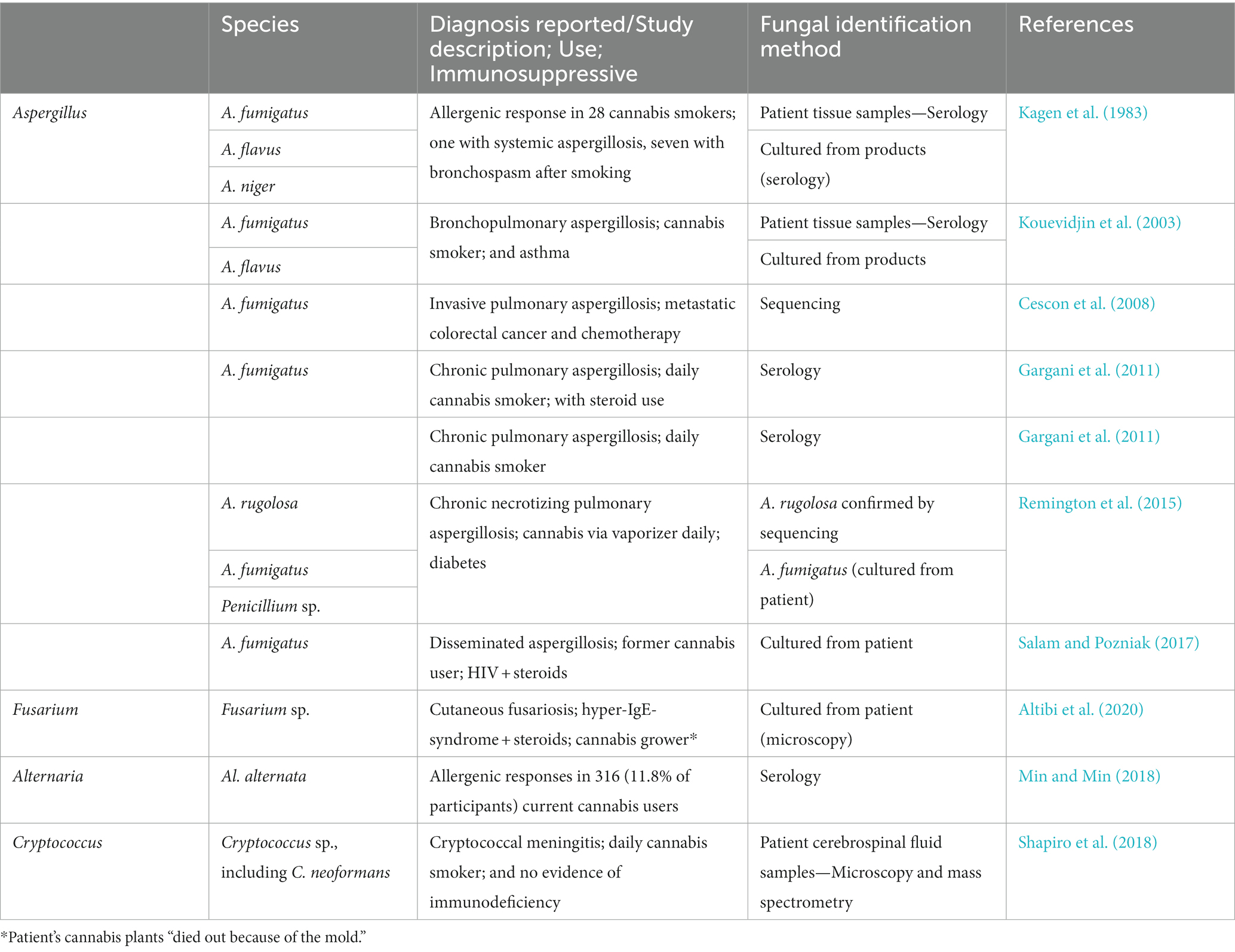
Table 2. Reports of allergenic responses to toxigenic fungi and mycoses associated with cannabis users (excluding those reported in Trullas et al., 2010).
Cannabis has also been reported to contain fungi in the order Mucorales (including Mucor spp.; Stone et al., 2019; Punja and Scott, 2023). These fungi release spores into the environment where they remain airborne and can potentially enter the body through inhalation. Pulmonary mycormycosis was reported seen in patients in a diabetic patient (Stone et al., 2019).
At present, only one published study has examined the association between cannabis use and fungal infection using data collected nationally (Benedict et al., 2020). From the United States insurance records from 53,217 people in 2016, the study demonstrated that cannabis users, particularly those with susceptible conditions, were 3.5 times more likely to develop a fungal infection than non-users. Aspergillosis accounted for 43% of fungal infections among cannabis users, while mucormycosis accounted for 3%. Among the population that was examined, individuals who used cannabis and had fungal infections were generally immunocompromised, a greater proportion were males, and a higher percentage also used tobacco when compared to those that did not use cannabis (Benedict et al., 2020). The source of the infections could not be determined to have originated specifically from cannabis products.
4. Mycotoxins in cannabis and hemp
Among several fungal species reported to be present on cannabis and hemp inflorescences (Table 1), a number can produce mycotoxins when grown on culture medium and potentially in the affected plants (Tables 3–5). Since fungal growth precedes mycotoxin production, generally the greater the growth, the higher the predicted levels of mycotoxins (Ismaiel and Papenbrock, 2015). Fungal growth on any substrate is directly impacted by water activity of the substrate (aw) and most fungi grow best at aw of 0.83–0.99 (Caplan et al., 2022). An important caveat, however, is that presence and recovery of toxigenic fungi from cannabis inflorescences in routine laboratory testing mandated by governmental agencies should not imply that they produce mycotoxins unless the moisture and temperature requirements for extended growth and mycotoxin production are available, which may differ by the type of mycotoxins produced (Milani, 2013). On cannabis inflorescences, visible fungal growth may occur prior to or after harvest (Figure 1). Post-harvest growth commonly leads to rejection of final products due to visible mold contamination and these buds are destroyed (Punja, 2021d). Subsequently, cannabis inflorescences that are improperly dried or stored under humid conditions may be expected to raise concerns for mycotoxin accumulation. If sufficient drying is achieved, the aw (water activity) of cannabis tissues is reduced to <0.7, which prevents the growth of most fungi (Carter, 2019). Because two of the most commonly encountered fungi that can potentially produce mycotoxins in cannabis tissues, namely Penicillium and Aspergillus spp., can survive at aw of 0.62–0.7, this range is suboptimal for fungal growth. Additionally, environmental conditions for gene transcriptional activity for mycotoxin production are narrower than those for fungal growth (Kolawole et al., 2021). Therefore, it is improbable that mycotoxin levels would significantly increase in cannabis tissues maintained at a low aw. The drying phase can be a component of quality assurance that can reduce the concerns for accumulation of mycotoxin produced by epiphytes in cannabis products.
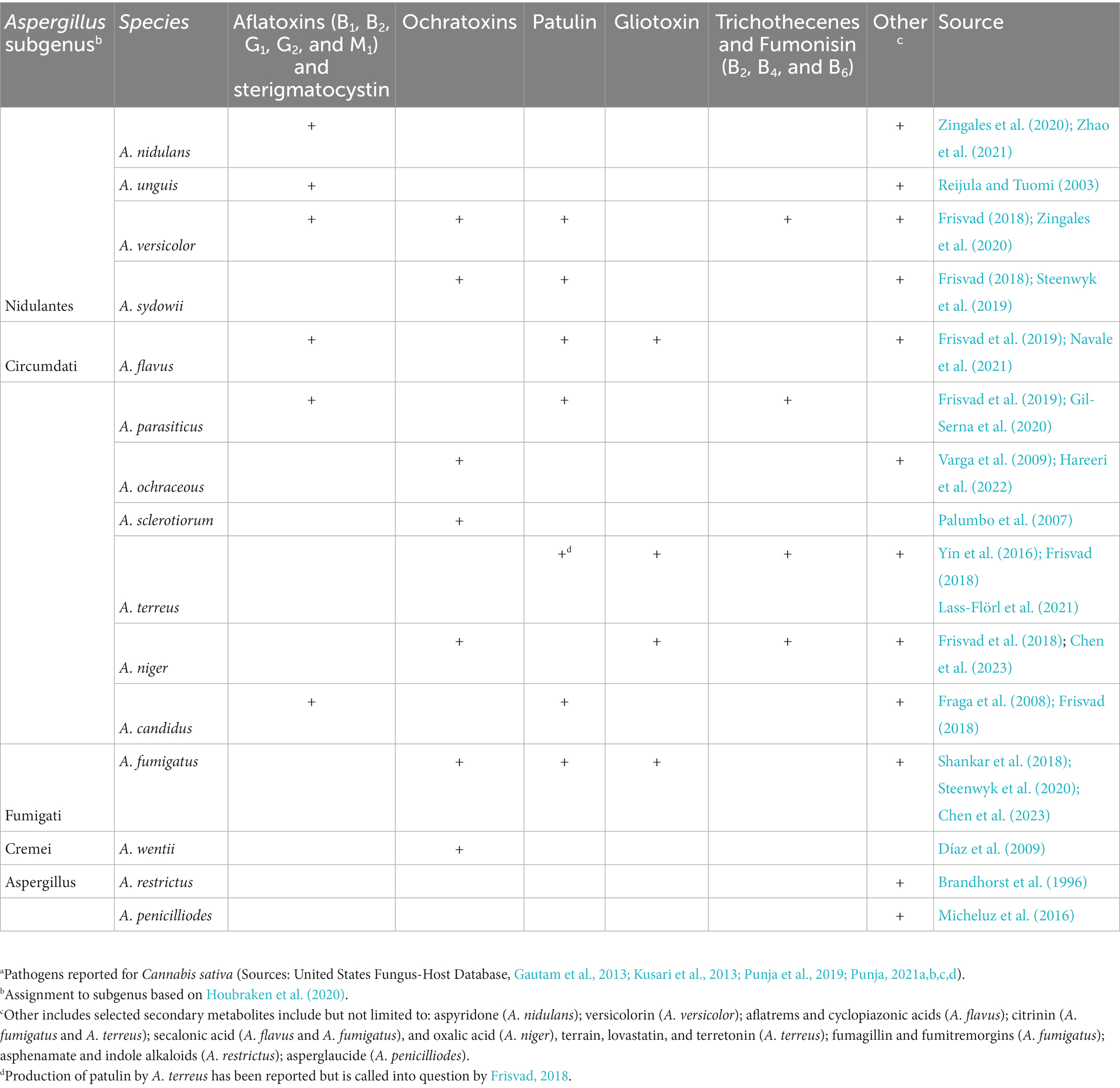
Table 3. Species of Aspergillus that have been reported in Cannabis sativa and mycotoxins produced in vitro.a
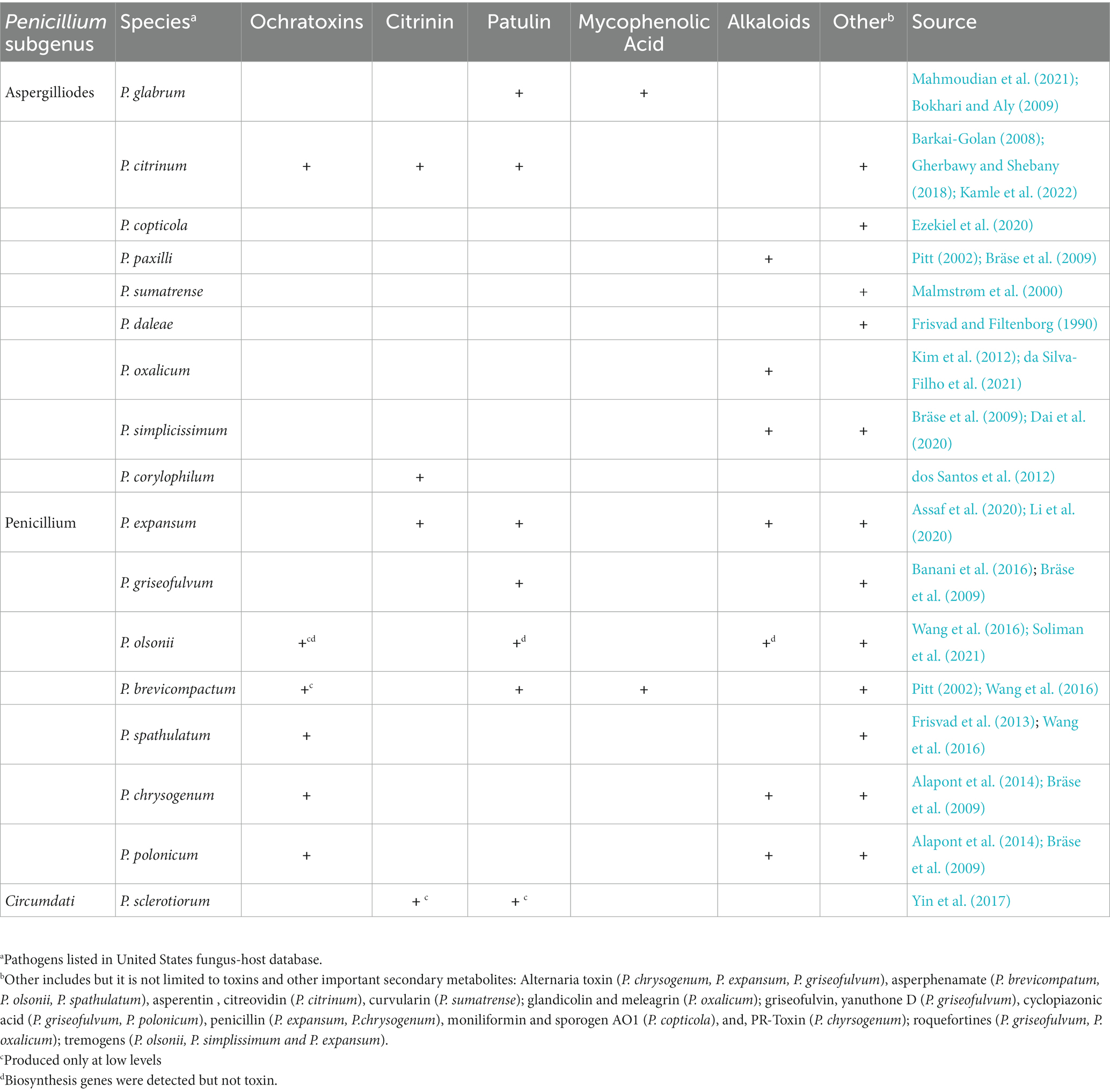
Table 4. Potential for toxigenic Penicillium spp. isolated from Cannabis sativa to synthesize toxic secondary metabolites.
4.1. Mycotoxin-producing fungi
Fungi reported to be present in cannabis and hemp plants (i.e., Penicillium, Aspergillus, and Fusarium spp.) have the genetic potential to produce a range of mycotoxins, including ones recognized as significant problems on other agricultural crops [e.g., aflatoxins, ochratoxin A (OTA), DON, fumonisins (FUM), zearalenone (ZEA), T-2 toxin, HT-2 toxin, citrinin (CIT), and patulin (PAT); Figure 4]. Some mycotoxins such as aflatoxins are produced by a few species, whereas others (e.g., OTA and PAT) are produced by multiple species belonging to multiple genera. The synthesis of mycotoxins involves several metabolic pathways and corresponding enzymes, such as polyketide synthases, nonribosomal peptide synthetase, the mevalonate pathway, or a combination of these. Mycotoxin profile, however, is typically different among genera. For example, Penicillium and Aspergillus are members of the same family, yet they only have a few secondary metabolites in common (Samson et al., 2011), and their mycotoxin profiles are very different from other members of the cannabis floral mycobiome. Other fungi such as Alternaria, Cladosporium, Chaetomium, Mucor, and Trichoderma that can be found in cannabis inflorescences (Table 1) may also produce different cytotoxic and genotoxic mycotoxins such as PAT, alternariol, tenuazonic acid (Ismail et al., 2023), chaetoglobosins (Fogle et al., 2008), viridin, and gliotoxin (Sugui et al., 2007; Bulgari et al., 2020) when adequate fungal growth has been achieved. This list is not exhaustive but is reflective of the diversity of the floral mycobiome from a few recent studies (Punja et al., 2023). Under organic crop production systems, the diversity of fungal species present on cannabis inflorescences can be greater than in conventional production systems (Punja and Scott, 2023). It is important to note that fungi listed in Table 1 are commonly found on a wide range of plant species and, hence, are not unique to cannabis and hemp. They pose a potential concern to cannabis consumers only if they have the potential for mycotoxin production or have been shown to infect individuals as discussed in Section 3.
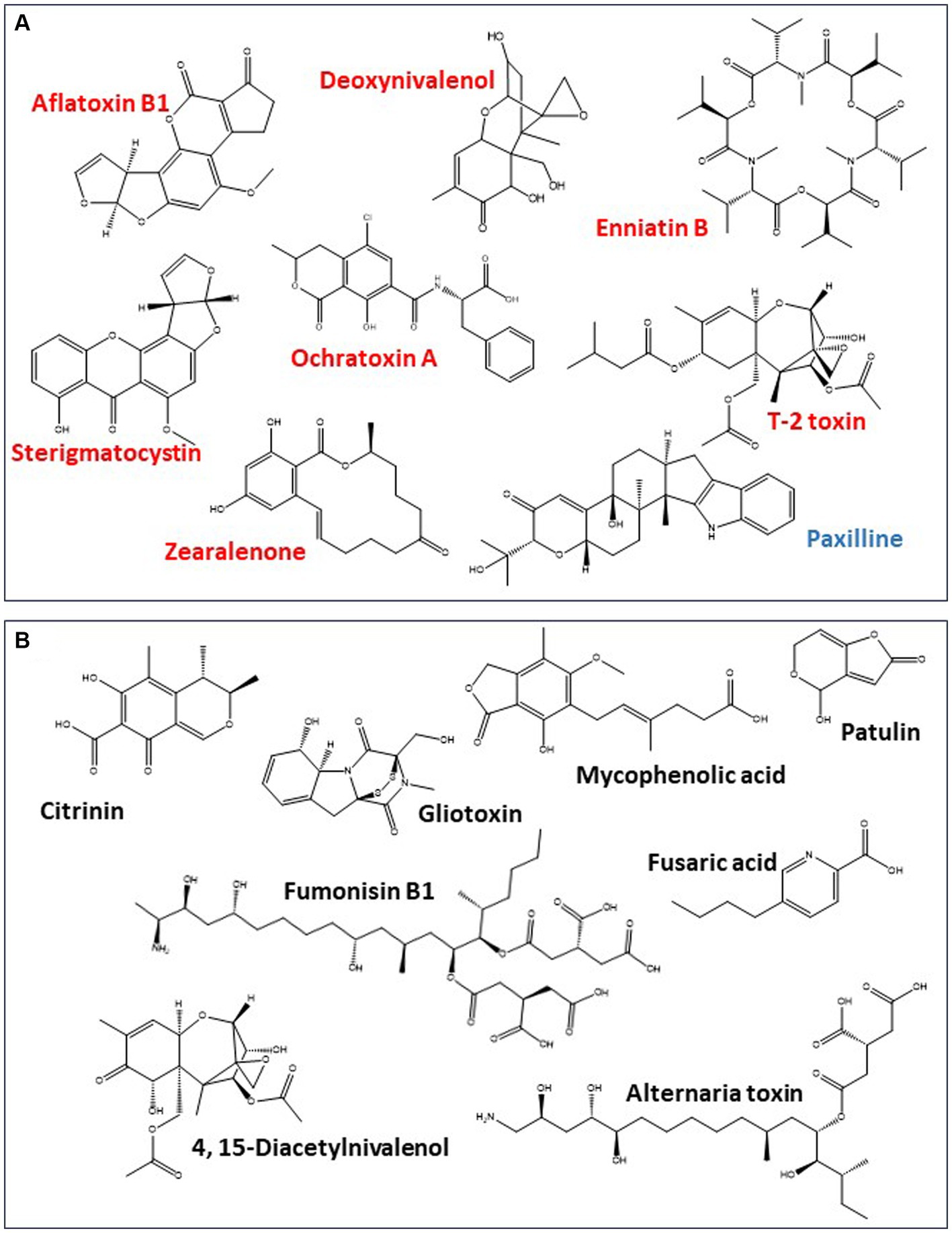
Figure 4. Mycotoxin production potential of toxigenic fungi reported in cannabis mycobiome. (A) Mycotoxins that have been reported in cannabis and hemp products (red) or for which genes for their production have been reported in toxigenic fungi isolated from cannabis products (blue). (B) Other mycotoxins that are known to be produced by toxigenic fungi associated with cannabis and hemp but have not been reported in the crops or their commercial products.
4.2. Aspergillus and Penicillium mycotoxins
There is a paucity of published studies on mycotoxin presence in cannabis-derived products (Table 6). Most of these studies included a limited number of samples (n < 100), with most of them identified as illicit samples. The legalization of cannabis in several countries, including Canada and in numerous US states, should increase the availability of samples for testing for mycotoxin presence moving forward. Species of Aspergillus are known to produce aflatoxins and OTA (Table 3). Aflatoxins B1, B2, G1, and G2 represent some of the most toxic and carcinogenic compounds occurring in nature. Although aflatoxins were produced by A. flavus and A. parasiticus when cultured on sterile herbal cannabis samples (Llewellyn and O'Rear, 1977), they have rarely been identified in compliance testing (Jameson et al., 2022; Table 6). This anomaly may be due to: (1) predominance of atoxigenic isolates in cannabis and hemp production systems (Sweany et al., 2022); (2) environmental conditions during crop production that do not support growth and development of the fungus to the extent where mycotoxins accumulate; (3) terpenes found in the inflorescence that can restrict fungal growth and development (see section 2.2); or (4) degradation of compounds by the plant or its associated microbes can occur. While the presence of potentially toxigenic species of Aspergillus has been reported on cannabis inflorescences (Table 1), there are fewer reports confirming the production of their associated mycotoxins (Table 6). Further research is needed to demonstrate whether the current concern over Aspergillus contamination of cannabis flowers can or should be extrapolated to infer the presence of associated mycotoxins.
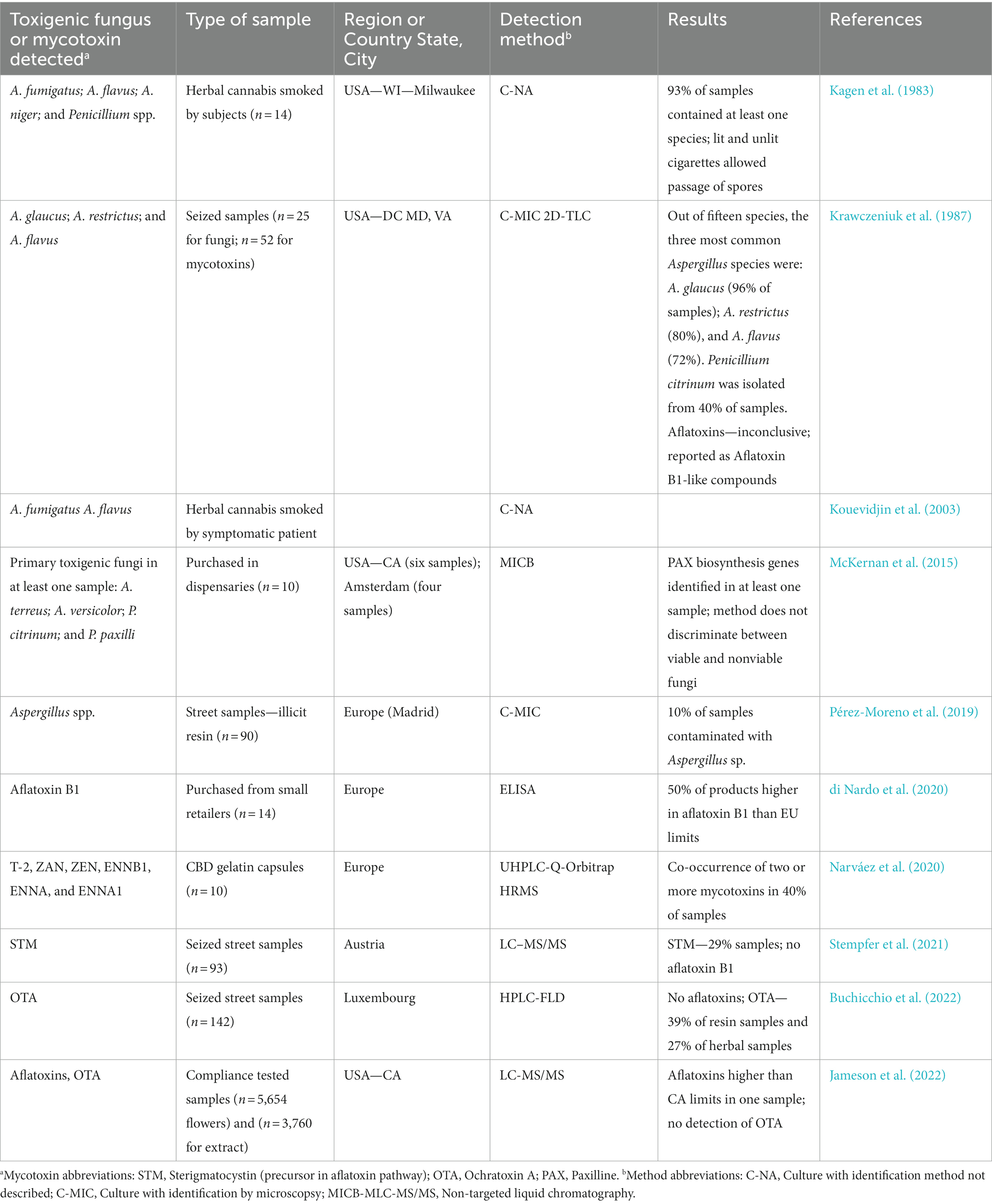
Table 6. Reports of toxigenic fungi in cannabis and cannabis products sold for consumer consumption or consumed by patients with mycoses.
The most frequently recovered toxigenic fungi from cannabis tissues are Penicillium spp. (Punja et al., 2019, 2023; Punja, 2021d). They can produce citrinin, OTA, CIT, and PAT, but only OTA has been found in cannabis samples to date (Tables 4, 6). Exposure of humans to OTA can result in kidney damage (Bui-Klimke and Wu, 2015). One fungal species associated with cannabis, Talaromyces pinophilus, produced OTA in culture, while a closely related strain, T. radicus, did not (Laut et al., 2023). Although no studies have compared mycotoxin levels in legalized and illicit cannabis products, the literature suggests that OTA presence can be of concern in illicit cannabis. In a recent study, OTA was detected in one-third of 142 illicit cannabis samples (Buchicchio et al., 2022). In a second study, OTA was not detected in more than 9,000 flower and extract samples from ~300 legal cannabis producers and manufacturers for compliance testing in California during 2020 and 2021 (Jameson et al., 2022). This study suggests that legalization of cannabis production may be having an intended consequence of improving quality with lower mold contamination, but additional studies are needed to confirm this.
In cannabis and hemp products that are smoked or vaped, aflatoxin B1 is likely to be degraded since it was completely degraded under experimental conditions at 180°C (Raters and Matissek, 2008). Temperatures in marijuana cigarettes can exceed 600°C (Fehr and Kalant, 1972) while coil temperature of three types of vaporizers exceeded 400°C (Oar et al., 2022). For example, OTA levels in dry wheat were reduced by 25% when exposed to 200°C for 6 min and by 44% if exposed for 12 min (Boudra et al., 1995). The degradation of these mycotoxins by heat does not always result in reduced toxicity since some degradation products are as toxic as the parent molecule (Kabak, 2009). Further studies are needed to confirm relationships between modes of product preparation and consumption and mycotoxin, and, in particular, potential for accumulation of mycotoxins in concentrated cannabis and hemp extracts used to prepare consumable items such as infused gummies and candy, or in capsules and gels, warrants further study.
4.3. Fusarium mycotoxins
Production of mycotoxins has been associated with several Fusarium species (Table 5). A number of Fusarium spp. that have been reported to infect cannabis inflorescences can produce an abundance of mycelial growth and mycotoxins under laboratory conditions (Gwinn et al., 2022; Munir et al., 2023). In a recent survey, diseases caused by Fusarium (including Fusarium head blight and Fusarium wilt) were commonly encountered during cannabis and hemp production in the United States (Munir et al., 2023). This may have important implications for cannabis worker safety, as exposure to infected cannabis plants has been linked to cutaneous fusariosis (Altibi et al., 2020). At present, there is a scarcity of analytical studies on Fusarium mycotoxin accumulation in cannabis and hemp inflorescences. There is only one reported study of DON production in hemp (Bergstrom et al., 2019, 2020). In that study, DON was present in the grain and floral tissues of outdoor-grown hemp at concentrations of up to 7 ppm, which was greater than the 1 ppm level established by the U.S. Food and Drug Administration permitted for cereal grains used for food [United States Food and Drug Administration (FDA), 2010]. Fusarium mycotoxins, including trichothecenes, ZEA, ionophores, FUM, and fusaric acid, have both acute and chronic effects in humans (Gruber-Dorninger et al., 2017; Ji et al., 2019). In addition to causing chronic immunosuppression and cancer, trichothecenes such as DON (one of the most common Fusarium mycotoxins, also known as vomitoxin), nivalenol (NIV), and T-2 toxin can cause acute symptoms of toxicosis, including nausea, vomiting, diarrhea, and death (Ji et al., 2019). Translocation of DON from stem base infections to the inflorescences has been reported in barley and wheat plants, which while not showing obvious symptoms in the inflorescences, may contain DON or other mycotoxins (Pecoraro et al., 2018). An analysis of gelatin capsules containing the cannabinoid, cannabidiol (CBD; n = 10) revealed the presence of T-2 toxin in 10% of the samples but the levels were below those allowed for animal food (Narváez et al., 2020). Fusarium mycotoxins are generally considered to be thermally stable and can still be detectable in foods that have undergone thermal processing (Bretz et al., 2006; Kabak, 2009). We are unaware of studies that have discussed the fate of these mycotoxins in combustion. When cannabis or hemp is smoked in a water pipe, DON contamination may be reduced because DON leaches into water at high temperatures; the water acts as filteration for the smoke (Liu et al., 2019; Feizollahi and Roopesh, 2022). However, even though the smoking process can reduce the level of Fusarium mycotoxins, these mycotoxins are absorbed into the bloodstream efficiently and rapidly through inhalation. Further studies are needed to demonstrate if Fusarium mycotoxins could pose potential health risks to consumers, which will be dependent on variables such as the growing environment to which the cannabis and hemp plants are exposed, as well as post-harvest processing and manufacturing and the form of exposure to consumers.
Cannabis hyperemesis syndrome (CHS) is a condition of recurring vomiting that some individuals develop after prolonged cannabis use (Perisetti et al., 2020). In Colorado, most cannabis-related emergency department (ED) visits were for CHS (Wang et al., 2021). The etiology of CHS is largely unknown and it may be triggered by multiple chemicals present in cannabis. Although no studies have linked presence of Fusarium mycotoxins (or their degradation products) in cannabis to CHS, the symptoms of CHS are remarkably similar to the toxicity of DON, NIV, and T-2 toxin, all of which can result in vomiting and partial or complete refusal of food (Bonnet et al., 2012). Additionally, the ingestion of fusaric acid—another common Fusarium mycotoxin that is often found together with DON—can cause hypotension (Gruber-Dorninger et al., 2017). This is consistent with the observation that CHS can be relieved by hot water bathing. Further studies are needed to establish the scale of contamination cannabis and cannabis products by Fusarium spp. and to understand neurotoxicity of potential mixtures of cannabinoids with Fusarium mycotoxins. This is especially important given the presence of several Fusarium species in cannabis and hemp inflorescences, both in indoor and outdoor cultivation (Punja, 2021a, 2021b; Punja and Scott, 2023).
Zearalenone is another prevalent Fusarium mycotoxin that is a major threat to food safety in cereal crops. It was isolated from 60% of CBD capsules (Narváez et al., 2020), but at levels below limits established for food products (Ji et al., 2019). Because of its structural similarity to estrogen, ZEA binds to estrogen receptors and affects reproductive systems of animals. This can potentially add to the adverse health effects associated with cannabis use in a subsegment of the population, which is an increased risk of preterm birth and small-for-gestational-age infants in prenatal exposure (Ryan et al., 2021). In rodent models and human cells, ZEA is immunotoxic, induces toxicity in the liver and kidneys, and is hemotoxic (Choi et al., 2012; Ji et al., 2019). Findings to date suggest that levels of certain mycotoxins associated with Fusarium species infecting growing cannabis or hemp plants pre-harvest may be found in products where extraction and concentration of the mycotoxins can occur alongside the cannabinoids of interest.
4.4. Other potential mycotoxins
The fungi belonging to the Mucorales produce mucoricin, a ricin-like toxin that damages host cells in vitro by inhibiting protein synthesis and is required for pathogenesis (Soliman et al., 2021). Enniatins and beauvericin are cyclodepsipeptides that are often classified as emerging mycotoxins because they are not regulated, and thus, there is little routine testing conducted for them. In animal studies, BEA, ENN, and MON can cause reproductive disruption in both males and females and increased offspring mortality (Chiminelli et al., 2022). In the study on CBD gelatin capsules, one sample contained three enniatins at levels similar to those of ZEA (Narváez et al., 2020). The U.S. EPA and Health Canada have registered a number of biopesticides for cannabis and hemp cultivation that contain Trichoderma spp. as active ingredients [United States Environmental Protection Agency (US EPA), 2023]. These species, including T. harzianum, T. virens, and T. asperellum, can all colonize cannabis plants as an endophyte (Punja and Scott, 2023; Scott and Punja, 2023), and in culture produce toxins, such as viridin and gliotoxin, that could potentially impact consumer health. Gliotoxin is a powerful mycotoxin that inhibits growth of other fungi and causes cytotoxicity by suppressing macrophage immune function and inflammation (Sugui et al., 2007). Additional research is required to establish whether these secondary metabolites are produced in cannabis inflorescence tissues following application of these beneficial fungi for plant pathogen control during the crop production cycle.
5. Contaminant regulation and assessment for cannabis and hemp
ASTM International, AOAC International, and U.S. Pharmacopeia are presently developing standardized methods for quantification of fungal and mycotoxin contaminants in cannabis and hemp, but there is there is a paucity of data available to determine the prevalence of these contaminants and their health impacts to cannabis and hemp consumers worldwide. The regulations currently in place have been largely adopted from the food industry, where fungal and yeast contamination of food products consumed by humans is not uncommon and can result in illnesses and potential fatalities. While the latter has yet to be studied in a statistical robust matter to support human health risk assessment of cannabis and hemp products, regulatory policies and assessment technology are evolving to meet the production and safety needs of the emerging industries.
5.1. Contaminant regulation for cannabis and hemp worldwide
The current regulatory policies for assessing cannabis contaminants in the United States and European Union have been extensively reviewed (Pruyn et al., 2022; Veit, 2023; Jameson et al., 2022). In the United States, the regulation of fungal contaminants in CBD and hemp products falls under the jurisdiction of federal agencies following the passage of the 2018 Farm Bill. However, due to the illegal status of cannabis at the federal level in the United States, the contaminant regulation policies for cannabis and cannabis-related products fall under the jurisdiction of state agencies. Each legalized state therefore has its own regulatory policies and testing programs (Jameson et al., 2022; Pruyn et al., 2022). This creates a dilemma for consumers due to varied potential health exposure risks. For example, regulatory action levels exist for aflatoxins and ochratoxins in some US states, but the levels are highly variable, ranging from a zero-tolerance policy in some states to no action level in others (Jameson et al., 2022). Similarly, the quantification of total yeasts and molds (TYM) and regulatory action levels are highly variable by state. Paradoxically, in jurisdictions where cannabis is still considered illegal but is being sold, there are no regulations to limit fungal contaminants in products. Not surprisingly, a few studies have found that illicit cannabis samples contained more contaminants than legally grown cannabis (Eykelbosh, 2021; Southall, 2022; Gagnon et al., 2023). The literature also suggests that aflatoxins and OTA can be more prevalent in illicit cannabis samples (see Section 4.2). These observations point to the need to harmonize the regulatory action levels for TYM and mycotoxin contaminants across all legalized jurisdictions for cannabis production. It also underlies the importance of supporting legal cannabis and hemp production systems where stringent regulations ensure a higher quality product.
Worldwide, current cannabis contaminant regulations have been directed to address a combination of concerns stemming from production, quality, and safety issues. The European Pharmacopoeia Commission (2023) provides generalized guidelines for aflatoxin and ochratoxin testing, and each member of the European Union has its own interpretation of these requirements. Countries such as Germany and the Netherlands have implemented individual monographs for cannabis (Veit, 2023). A few non-E.U. countries, such as Canada and Israel, also follow the general guidelines for aflatoxin and ochratoxin testing in the European Pharmacopoeia [Israel Ministry of Health—Medical Cannabis Unit (IMC), 2017; Health Canada, 2020]. Canada has an irradiation requirement for the export of cannabis to other countries. In Israel, cannabis is irradiated (beta and gamma) before it can be used for medical purposes. No countries, or any state in the United States, currently require testing for presence of Fusarium spp. or their mycotoxins.
5.2. Methods for assessment of molds and human pathogens
The general guidelines established by AOAC International and United States Pharmacopeia for microbial contamination include testing methods based on isolation on culture media and enumeration of colonies (i.e., total yeast and mold, or TYM) and identification by quantitative PCR (qPCR) analysis, but other methods based on molecular technology are in development (Goldman et al., 2021). Fungi that are known to be pathogens of humans require a qualitative presence/absence analysis. All methods are potentially problematic for regulatory agencies. For example, using culture-based methods to detect Aspergillus spp. can be difficult due to the problems in differentiating human pathogenic and nonpathogenic species. There is currently no requirement to identify the colonies to species level. While it often requires a highly trained mycologist to correctly identify these fungi to species, it is essential in evaluating them for human health risks (Chen et al., 2019). In addition, multiple species of the same genus can infect plants of cannabis and hemp. For example, in Kentucky, Fusarium avenaceum, members of the F. incarnatum-equiseti species complex, F. sporotrichioides, and F. graminearum were all isolated from hemp samples collected during a single cropping season (Smith et al., 2023). This illustrates the importance of species identification from TYM testing.
Currently, TYM quantification is widely used by regulatory agencies such as Health Canada and different state regulatory agencies in the United States. The regulatory action levels for TYM can vary considerably by jurisdiction, ranging from 10,000 colony forming units per gram (cfu/g) to 100,000 cfu/g. Also, there is no standard dilution curve that can be used to measure test reliability because of the inability to ship cannabis samples across state lines. The assessments are usually conducted by certified commercial laboratories using plating assays that homogenize and plate extracts from tissues onto different agar media and enumerate colonies after 3–5 days of incubation. Since the community structure of the mycobiome varies according to season and is impacted by other environmental factors, such as temperature, humidity, and air movement, there is considerable biological variability among samples in TYM content (Punja et al., 2023). Furthermore, the TYM assessments are based on enumeration of all yeasts and molds present in a sample, regardless of whether they have a previous history for concern based on human exposure or have potential for mycotoxin production. For example, cannabis plants that are treated with a registered fungal biological control agents (e.g., Trichoderma spp.) would likely carry over sufficient propagules in the inflorescence tissues that cause it to fail to pass regulatory limits in certain jurisdictions (Punja et al., 2023). The current concept of TYM testing requires further evaluation, and determination of its value to provide information on the fungal species composition to support human health risk assessment is an area that urgently needs assessment.
The variability in the regulatory action levels based on TYM and the inter-laboratory variation of testing results bring into question how cannabis producers, consumers, and health practitioners can reliably interpret what is safe from what is not. More targeted approaches such as testing specifically for certain fungi known to pose a threat to consumers due to potential mycotoxin production (e.g., Aspergillus spp. and Fusarium spp.) would be more informative when compared to an assessment of total fungi and yeasts present. This is consistent with the practices currently used for regulation and monitoring of agricultural food products consumed by humans. If similar TYM testing were to be imposed on these foods, many could fail to pass due to their natural inherent microbiome composition. In fact, a subset of the microbiota of cannabis inflorescences may be providing undetermined benefits based on what is known from other crop plants (Punja et al., 2023).
Several toxigenic Penicillium and Aspergillus species were detected on dispensary-based cannabis flowers, including P. citrinum and P. paxilli, when samples were analyzed by next-generation sequencing but not by culture-based methods (McKernan et al., 2015). Molecular methods such as next-generation sequencing and qPCR, depending on the primer/probe, are far more sensitive and specific than culture-based methods (Sarma et al., 2020). For DNA-based testing, it is recommended that the enrichment of cannabis matrices in fungus-specific media requires at least 48 h for Aspergillus spp. to grow to detectable levels and dilute the concentration of DNA from nonviable organisms. To increase the sensitivity of qPCR methods, a larger sample volume for downstream DNA extraction is highly recommended (Sarma et al., 2020). Molecular methods, however, can lead to false positives because of the cross-reactivity with non-specified Aspergillus species. Since genetic materials from dead organisms are still detectable by qPCR after cannabis irradiation (Frink et al., 2022), assessment of the success of the decontamination process must not solely rely on qPCR and should include culture-based methods unless methods to circumvent these issues are used. These methods include the 48-h enrichment method described above and the use of nucleases that do not penetrate living cell walls or cell membranes but can degrade DNA of nonviable organisms. However, these methods have not been validated on cannabis treated with the decontamination methods currently used in cannabis production. Different forms of decontamination may require different enrichment and PCR techniques to perfectly match plating. Because decontamination techniques have not been certified by AOAC for hemp, no PCR kits are certified to work with decontaminated products. Irradiated products will require enrichments methods similar to those described above for molecular methods to identify only living organisms.
5.3. Methods for assessment of mycotoxins
Analytical methods for testing mycotoxin must be accurate, reproducible, and sensitive, but considerations such as speed and cost are also important if the method is to be adopted for routine testing. In cannabis, the Association of Official Analytical Collaboration (AOAC) International (2021) established target levels for limits of quantification (LOQ) for OTA, aflatoxin B1 and total aflatoxins (aflatoxin B1 + aflatoxin B2 + aflatoxin G1 + aflatoxin G2). In order to be accepted as an AOAC International method, LOQ must be below target levels; however, in some countries (e.g., EU and Australia) regulatory limits are much lower than the LOQs established by Association of Official Analytical Collaboration (AOAC) International (2021). Sample size and sample extraction methods play a pivotal role, and these methods vary for individual laboratories and technologies. Gas chromatography–mass spectrometry is used to monitor mycotoxins in other systems, but there have been no reports on its use in analysis of cannabis or hemp.
5.3.1. Liquid chromatography
Liquid chromatography coupled with MS (LC–MS) is commonly used to detect and identify small molecules in complex matrices, such as cannabis and cannabis-infused products (Table 7). Tandem MS (LC–MS/MS) allows multiresidue methods that detect and quantify both mycotoxins and pesticide contaminants. Typically, these commercial methods target only those specified by AOAC International and does not target other mycotoxins discussed above; however, there are a few studies that have detected contaminants beyond those specified by the AOAC International. Five categories of bioactive compounds were identified (cannabinoids, primary metabolites, secondary metabolites, contaminants, extracted and leached compounds) using technology that coupled a Quick, Easy, Cheap, Effective, Rugged and Safe (QuEChERS) method for sample extraction and separation with identification by non-targeted LC–MS/MS. Contaminants identified by this method included a synthetic cannabinoid, nicotine, 11 drugs, 15 pesticides, and sterigmatocystin, the precursor to aflatoxin biosynthesis, but no aflatoxin was detected (Stempfer et al., 2021). Sixteen mycotoxins (aflatoxins, HT-2, T-2, α-and β-zearalenol, ZEA, beauvericin, and enniatins) were quantified in spiked samples using a QuEChERS method and quantified by ultra-high-performance LC coupled with quadrupole Orbitrap high-resolution MS, but only six mycotoxins were isolated from cannabis-based samples (Narváez et al., 2020; Table 6). To enhance detection, immunoaffinity columns with antibodies specific for aflatoxins and OTA have also been used for cannabis and hemp sample clean-up before LC analysis (Wilcox et al., 2020; Greaves et al., 2021; Buchicchio et al., 2022).
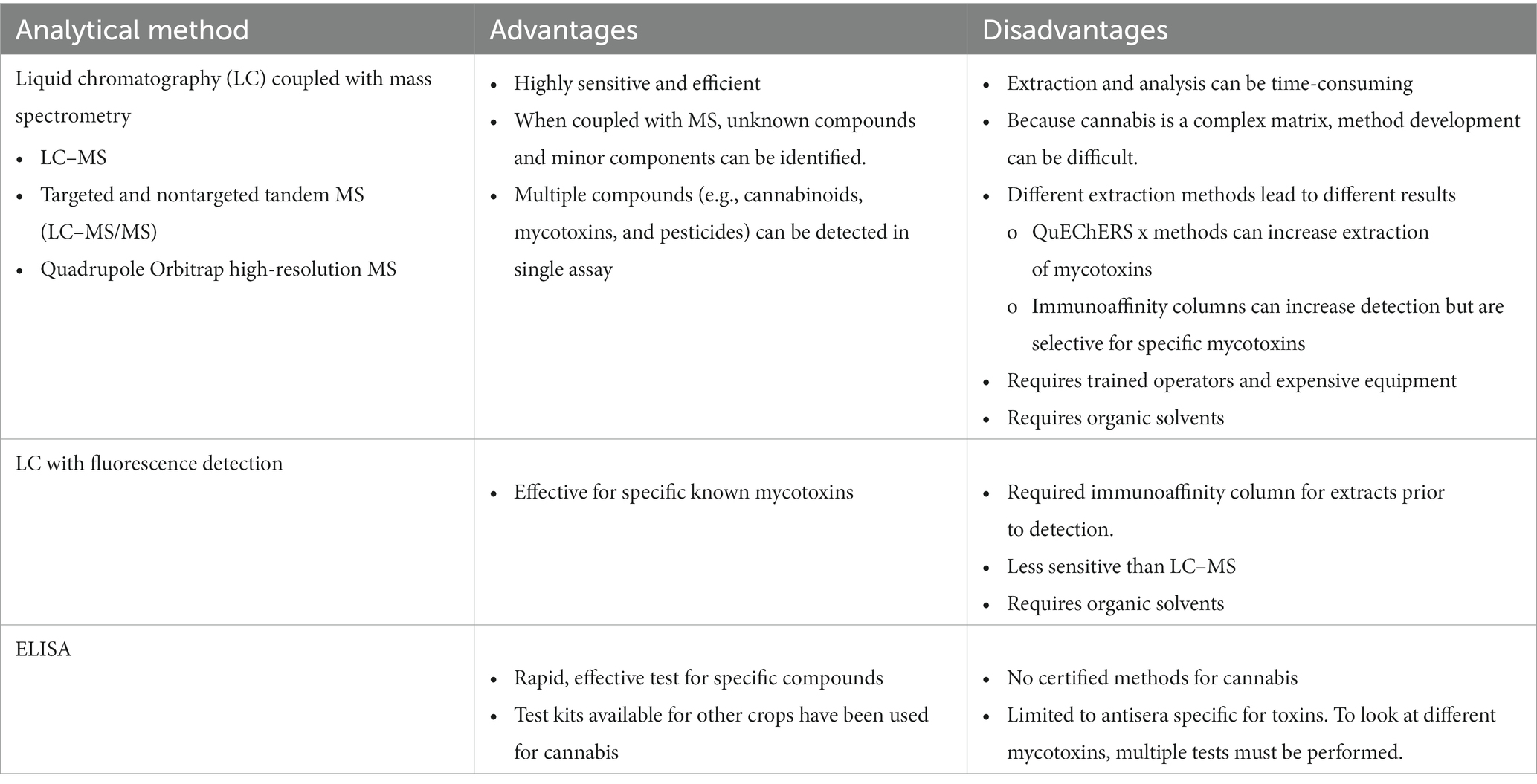
Table 7. Summary of advantages and disadvantages of methods reported for testing of mycotoxins in cannabis and hemp.
5.3.2. Immuno-based technologies
Analysis by LC is time-consuming and requires both trained operators and expensive equipment, so strategies that allow for simpler and more rapid detection of mycotoxins in food samples are desired (Xiong et al., 2022). The most commonly used of these methods is an enzyme-linked immunosorbent assay (ELISA); however, like the immunoaffinity columns above, ELISA is limited by the specificity and availability of mycotoxin-specific antibodies. ELISA is an excellent screening tool that can be used to estimate mycotoxins in other crops and cannabis plants (Bergstrom et al., 2019, 2020). Tests have been certified for rapid (≤30 min) determination of single mycotoxins (aflatoxin, DON, OTA, fumonisin, and ZEA) in grains [United States Federal Grain Inspection Services (FGIS), 2023], but there have been no certifications for use in cannabis or hemp. Dipsticks are rapid tests that can be performed rapidly (usually providing results in 30 min). Most dipstick tests are immuno-based assays that have antibodies immobilized on an analytical membrane and are used to verify mycotoxin content in food and grain. Dipstick tests can detect mycotoxins, either single or a group of compounds, and when portable photometric strip readers are used can support on-site screening (Lattanzio and Nivarlet, 2017). Gold nanoparticles (AuNPs) can be functionalized to recognize and bind to specific target molecules (Ferrari, 2023) and have been used as optical mycotoxin biosensors. A multiplex dipstick test using AuNP technology for detection of Fusarium mycotoxins in oats identified levels of multiple mycotoxins in a rapid on-site protocol, but this technique identified only relative levels of the toxins (e.g., negative, low positive, or positive; Lattanzio and Nivarlet, 2017). AuNPs are routinely used for detection in ELISA testing (Ferrari, 2023).
5.3.3. Other emerging technologies
A DNA-based dipstick technology was developed to identify Fusarium spp. and trichothecene production potential” (Suga et al., 2022). E-nose technology is a rapid and cost-effective diagnostic tool for mycotoxin detection, but development of a universal e-nose is “unrealistic [because] a unique e-nose must be validated for each mycotoxin” (Cheli et al., 2023). In flow-injection analysis mass spectrometry (FIA-MS) chromatographic, chromatography separation steps are omitted. When FIA is coupled with triple quadrupole instruments and high-resolution mass spectrometers, such as time-of-flight (TOF) and Orbitrap instruments, high-throughput quantitative screening of analytes is possible. Rakk et al. (2023) validated FIA-MS methods that provided quantitative analysis of corn and wheat samples for 11 mycotoxins (aflatoxins, DON, fumonisins, HT-2, T-2, OTA, and ZEA) in less than 1 min.
6. Approaches to reduce fungi and mycotoxins on cannabis and hemp
The fungal and yeast species that have been found on cannabis and hemp can be reduced through various practices intended to minimize the incidence of propagules (mostly consisting of spores and/or mycelium) on the tissues or by reducing development under conditions favoring their spread. These practices can be grouped into pre-harvest and post-harvest management and are discussed in more detail below.
6.1. Pre-harvest management
Most strategies aimed at reducing fungal development on inflorescences before harvest are targeted to (i) reduce spread of fungal spores onto inflorescences, (ii) alter environmental conditions by reducing relative humidity in the growing environment, or (iii) prevent spread of spores by workers tending to the plants. For example, enhancing air circulation using fans in the final weeks leading up to harvest significantly reduced the numbers of total yeast and molds in cannabis inflorescences (p = 0.05; Punja et al., 2023). Genotypes of cannabis under cultivation can also affect the levels of fungi found to be present within the inflorescences and thus influence final quality of the product (Punja et al., 2023). Additional research is needed to develop genotypes that would allow cannabis producers to select plants that are less prone to high yeast and mold levels.
Based on observations of significant differences between C. sativa genotypes these differences may be based on chemical interactions with the fungal populations, but more knowledge is needed to determine if naturally occurring chemical compounds in cannabis inflorescences affect fungal development. This knowledge would allow breeders to target chemical profiles for the selection of new chemotypes. Although biological activities have been shown for extracts of C. sativa (summarized by Hourfane et al., 2023), few studies have tested activity against toxigenic fungi. Acetone extracts from C. sativa inflorescences and hashish inhibited both growth of A. flavus and the production of aflatoxin B1 but extracts of leaves and stems did not inhibit the fungus in poisoned food assays (Al Khoury et al., 2021). However, in disk diffusion and agar well diffusion assays, growth of Fusarium spp. and A. niger was inhibited by acetone, chloroform, ethanol, and water extracts of C. sativa leaves (Anjum and Zel-E-Arooj, 2018). For A. niger, inhibition zone in disk diffusion assays ranged from 20.6 mm (chloroform) to 23 mm (ethanol), and for Fusarium spp., inhibition zones ranged from 18.3 mm (chloroform) to 24.3 mm (aqueous). Hot water extracts of callus (CE) derived from leaves that contained alkaloids, terpenoids, and flavonoids, were active against A. flavus, A. fumigatus, A. niger, F. solani, and Mucor in an agar diffusion test. Inhibition zones (IZs) for CE treatments were greatest for A. fumigatus (15 mm) and IZs increased to 20 mm when the fungus was treated with zinc nanoparticles made from CE (CE-ZnONPs); the activity of CE-ZnONPs was greatest against Mucor (IZ = 30 mm), but the fungus was only slightly inhibited by of CE (IZ = 7 mm; Zaka et al., 2021). Growth of F. oxysporum was inhibited (up to 47%) by water extracts of C. sativa leaves (Tapwal et al., 2011). In greenhouse studies, nanoemulsions of the terpene-rich C. sativa by-products from commercial CBD extraction controlled powdery mildew diseases of hemp (Akinrinlola et al., 2022; Fei et al., 2023). These observations indicate that there are opportunities to enhance naturally occurring anti-fungal compounds in C. sativa to reduce levels of TYM.
Another area of research that can reduce potential levels of fungal contaminants is monitoring disease incidence and severity on cannabis and hemp inflorescences as they approach harvest and developing strategies that can reduce their levels pre-harvest. Such remediation approaches reduce the burden for post-harvest interventions that can alter the chemical composition and aroma profile (primarily a function of terpene composition and concentration) of the product. Application of registered microbial products to manage fungal pathogens infecting cannabis inflorescences should be undertaken with the knowledge that they may inadvertently increase total yeast and mold levels (Punja and Ni, 2022; Punja et al., 2023).
6.2. Post-harvest management
After harvesting, the trimming method used can influence the buildup of total yeast and molds in cannabis inflorescences (Punja et al., 2019). Furthermore, duration of drying and final moisture content (water activity) has a significant impact on the levels of fungi present, particularly those like Aspergillus and Penicillium spp. that are tolerant of low moisture levels (Punja et al., 2023). Storage method and duration and temperature can also influence the extent to which the dried inflorescences may build up yeasts and molds. Various post-harvest treatment methods can impact quality of cannabis-derived products with regard to yeast and mold levels. The use of irradiation by e-beam (ß-irradiation) or γ-irradiation is permitted in specific countries such as Canada and Israel and reduces fungal contaminants to zero (Punja, 2021d). These technologies are expensive, and although γ-irradiation did not change terpene profile in cannabis, treatment resulted in overall reduced levels of terpenes (Hazekamp, 2016). In medicinal cannabis, TYM levels of cannabis were reduced (6–4.5 log) when treated with γ-irradiation and by 5-log when treated with cold plasma treatment or e-beam (Jerushalmi et al., 2020). The relative advantages and disadvantages of other methods used for decontamination or sterilization in the food industry [e.g., heat; high pressure; chemical, filtration; extraction; non-ionizing and ionizing irradiation; photonic decontamination (i.e., X-ray); and cold plasma] for use in cannabis industries have been reviewed by Dhillon et al. (2022). In cases where cannabis use is destined for medical use by patients with immunocompromising conditions (e.g., cancer, AIDS, and diabetes), the use of these forms of irradiation may be worthy of consideration to ensure that fungal contaminants are reduced to negligible levels. The enhanced safety of these products should correspondingly reduce the incidence of various forms of fungal infections as reported in Section 3.
6.3. Reducing mycotoxins in cannabis tissues
After mycotoxins are produced either in the intact plant or the harvested inflorescences, there are no proven technologies to degrade or detoxify them, but cold plasma treatments have been reported to degrade mycotoxins in other systems (Doshi and Šerá, 2023); hence, cannabis products that have been sterilized or produced from sterilized materials may still contain mycotoxins. Byproducts formed by alteration of mycotoxins by varied mechanisms [opening of ring structure (aflatoxin B1, DON), closure of a ring structure (OTA), and oxidation, hydroxylation, and methylation (ZEA, FUM B1)] are usually but not always less harmful. A notable exception is the formation 𝛼-zearalanone, a more toxic compound, from ZEA (Liu et al., 2020). Flavonoids and phenolics in water extracts of plants have been implicated as active degrading agents of aflatoxins, but it is likely that higher molecular weight compounds, possibly enzymes, are involved (Loi et al., 2020). Essential oils of plants that contain fungistatic or fungicidal terpenes can potentially reduce mycotoxin content in cannabis products (Ranjith et al., 2021). For example, when the essential oil of Heliotropium bacciferum containing 32.9% a-pinene and 9.4% β-myrcene was mixed with aflatoxin B1, 82.6% of the mycotoxin was degraded (Al-Harrasi et al., 2021). Biocontrol agents may also affect mycotoxin levels. In co-culture studies, some antagonistic Trichoderma strains were able to glycosylate DON or convert ZEA into its hydroxylated derivative, β-zearalenol (Modrzewska et al., 2022). Therefore, there are opportunities for further research into decontamination of cannabis or hemp products should Fusarium mycotoxins become a regulatory issue.
7. Future directions for research
Current knowledge of the extent of fungal contamination on cannabis and hemp inflorescences relies on publicly available data from published independent studies and cannabis compliance tests. The latter is only available in a few legalized jurisdictions where such data are publicly disclosed [California Bureau of Cannabis Control (CBCC), 2018; Li et al., 2023]. Therefore, essential information on the incidence and frequency of several fungal species of concern is unavailable. In addition, most compliance tests currently rely on culture-based isolation (e.g., TYM) that provide no information on which specific fungal contaminants are present. While aflatoxins and OTA are regulated in many legalized jurisdictions, there are no US states or any country that currently regulate Fusarium spp. and their mycotoxins. As such, the incidence of Fusarium contamination is currently unknown in cannabis and hemp products, in contrast to cereal grains and many other agricultural commodities worldwide. In a recent survey of diagnostic professionals in the United States, infection by Fusarium spp. on cannabis or hemp in most areas of the country, except for the Great Plains region (Munir et al., 2023); this is likely due to the low numbers of samples received from this region. Similarly Canadian production areas have reported f Fusarium occurrence on cannabis plants and inflorescences during commercial cultivation (Punja et al., 2019, 2021, 2023; Punja, 2021a,b,c; Punja and Ni, 2022). Continued surveillance for the prevalence of Fusarium species and other phytopathogens that could impact the health of cannabis consumers, particularly those with immunocompromised systems, should be undertaken in production areas annually.
While LC–MS–MS methods are available to detect multiple mycotoxins together with pesticide residues in cannabis and hemp flowers (see Section 5.3), most methods used in cannabis or hemp compliance laboratories are largely based on existing regulatory action levels. Hence, Fusarium mycotoxins are not being included in compliance testing. Further research is needed to develop commercial analytical methods that would allow data collection to determine to what extent these potentially widespread but unregulated mycotoxins occur in cannabis and hemp products. In addition, large-scale international and national collaborations are needed to collect and disseminate information on fungal contaminants found in cannabis and hemp, similar to existing efforts in other agricultural commodities (e.g., NFCFD) for surveillance purposes. These data should be made available in the peer-reviewed literature.
8. Conclusion
A review of the current literature indicates there are potential human health risks of fungal and mycotoxin contaminants in cannabis and hemp flowers, particularly for the subset of the population that is immunocompromised. Further research is needed to understand contamination prevalence, inform regulatory policies, and advance management practices. Several studies have linked cannabis use to increased risk of fungal infection in immunocompromised patients. Current regulations have addressed the potential for harm from Aspergillus species, yet few reports have confirmed whether mycotoxin production is widespread or is rare in occurrence in cannabis products. While there are many reports of cannabis and hemp diseases caused by Fusarium species, there are no regulations currently in place for assessing mycotoxin levels. Additional research is needed to survey the extent of both Aspergillus and Fusarium species occurrence and potential mycotoxin presence.
A major hurdle faced by cannabis and hemp industries is addressing the disconnect between production-related issues and human safety issues. The current regulatory requirement for enumerating TYM present in cannabis and hemp tissues using plating on culture media does not allow for a direct interpretation as to potential health risks to consumers. The huge variation between United States jurisdictions in the acceptable levels of TYM, ranging from 100 to 100,000 cfu/g illustrates the difficulty in implementation of human health risk assessment. A consistent and widely adopted protocol for sampling, analysis, and interpretation of TYM results is essential to provide a consistent quality assessment for consumers and health practitioners. We suggest consideration of exclusion of non-harmful fungal and yeast species from a priori testing and instead encourage the adoption of species testing targeting a smaller range of fungi with potential to cause harm. The current reporting of “everything that is present” in cannabis samples may not serve the cannabis industry well moving forward since other potential harmful fungi such as Fusarium and Penicillium can be under-represented in the overall TYM counts. We recommend testing for the presence of Aspergillus, Penicillium, Fusarium, and Mucor as priorities, with an adjustment to adding other species if they are discovered to have potential harm. Additionally, multi-residue analytical methods (i.e., LC–MS–MS) used by most compliance testing laboratories should be further developed to enable the detection of Fusarium mycotoxins together with regulated contaminants.
One possible solution to reduce potential harm to medical users of cannabis from toxigenic fungi is to develop a two-tier system that distinguishes products intended for medical and recreational use. The products for medical use should have a unified national standard that includes mandatory irradiation to eliminate fungal contaminants and protect immunocompromised patients. The products for recreational use would have a higher tolerance for TYM (e.g., 50,000 cfu/g). The latter would still require mandatory testing for the presence of Aspergillus and Fusarium to provide assurances to consumers that they pose no harm from fungal contaminants. A zero-tolerance policy seems untenable for a plant-derived product exposed to many variables in the cultivation environment. There are many fungal species present in cannabis inflorescences that are not deemed to be of concern to human health. Rather, regulatory agencies could impose a limit of 10 cfu/g for species of concern such as Aspergillus and Fusarium, which may be sufficient to provide assurances to consumers while reducing the burden on an industry that already experiences significant regulation.
Author contributions
KG: Conceptualization, Data curation, Investigation, Project administration, Visualization, Writing – original draft, Writing – review & editing. ML: Conceptualization, Investigation, Resources, Supervision, Writing – original draft, Writing – review & editing. AS: Investigation, Resources, Writing – review & editing. ZP: Conceptualization, Resources, Writing – original draft, Writing – review & editing.
Funding
The author(s) declare financial support was received for the research, authorship, and/or publication of this article. This work was partially supported by the National Institute of Food and Agriculture, United States Department of Agriculture uner TEN00573. Biopesticide potential in controlling pathogens and pests of hemp awarded to Kimberly Gwinn.
Acknowledgments
The authors would like to thank Nita Davidson for reviewing the manuscript.
Conflict of interest
The authors declare that the research was conducted in the absence of any commercial or financial relationships that could be construed as a potential conflict of interest.
Publisher’s note
All claims expressed in this article are solely those of the authors and do not necessarily represent those of their affiliated organizations, or those of the publisher, the editors and the reviewers. Any product that may be evaluated in this article, or claim that may be made by its manufacturer, is not guaranteed or endorsed by the publisher.
References
Abrams, D. I. (2022). Cannabis, cannabinoids, and cannabis-based medicines in cancer care. Integr. Cancer Ther. 21:15347354221081772. doi: 10.1177/15347354221081772
Akinrinlola, R. J., Gwinn, K. D., Wang, T., and Hansen, Z. R. (2022). Numerous fungicides suppress hemp powdery mildew in the greenhouse in Tennessee, 2021. Available at: https://www.plantmanagementnetwork-org.utk.idm.oclc.org/pub/trial/PDMR/reports/2022/V049.pdf (Accessed August 2, 2023).
Al Khoury, A., Sleiman, R., Atoui, A., Hindieh, P., Maroun, R. G., Bailly, J. D., et al. (2021). Antifungal and anti-aflatoxigenic properties of organs of Cannabis sativa L.: relation to phenolic content and antioxidant capacities. Arch. Microbiol. 203, 4485–4492. doi: 10.1007/s00203-021-02444-x
Alam, B., Lî, J., Gě, Q., Khan, M. A., Gōng, J., Mehmood, S., et al. (2021). Endophytic fungi: from symbiosis to secondary metabolite communications or vice versa? Front. Plant Sci. 12:791033. doi: 10.3389/fpls.2021.791033
Alapont, C., López-Mendoza, M. C., Gil, J. V., and Martínez-Culebras, P. V. (2014). Mycobiota and toxigenic Penicillium species on two Spanish dry-cured ham manufacturing plants. Food Addit. Contam. A Chem. Anal. Control Expo. Risk Assess. 31, 93–104. doi: 10.1080/19440049.2013.849007
Alhamad, T., Koraishy, F. M., Lam, N. N., Katari, S., Naik, A. S., Schnitzler, M. A., et al. (2019). Cannabis dependence or abuse in kidney transplantation: implications for posttransplant outcomes. Transplantation 103, 2373–2382. doi: 10.1097/TP.0000000000002599
Al-Harrasi, M. M. A., Al-Sadi, A. M., Al-Sabahi, J. N., Al-Farsi, K., Waly, M. I., and Velazhahan, R. (2021). Essential oils of Heliotropium bacciferum, Ocimum dhofarense and Zataria multiflora exhibit aflatoxin B1 detoxification potential. All Life 14, 989–996. doi: 10.1080/26895293.2021.1991006
Altibi, A. M., Seth, R., Battisha, A., and Kak, V. (2020). Cutaneous fusariosis in a patient with Job’s (hyper-IgE) syndrome. Case Rep. Infect. Dis. 2020:3091806. doi: 10.1155/2020/3091806
Anjum, M., and Zel-E-Arooj, A.S., P. Rehman, and Khadim, J. (2018). Evaluation of antimicrobial activity and ethnobotanical study of Cannabis sativa L. Pure Appl. Biol. 7, 706–713. doi: 10.19045/bspab.2018.70088
Assaf, C. E. H., Zetina-Serrano, C., Tahtah, N., Khoury, A. E., Atoui, A., Oswald, I. P., et al. (2020). Regulation of secondary metabolism in the Penicillium genus. Int. J. Mol. Sci. 21:9462. doi: 10.3390/ijms21249462
Association of Official Analytical Collaboration (AOAC) International (2021). Standard method performance requirements (SMPRs®) for quantitative analysis of mycotoxins in cannabis biomass and cannabis-derived products. Available at: https://www.aoac.org/wp-content/uploads/2021/12/SMPR-2021_010.pdf (Accessed August 2, 2023).
Ball, O. J. -P., Coudron, T. A., Tapper, B. A., Davies, E., Trently, D. J., Bush, L. P., et al. (2006). Importance of host plant species, Neotyphodium endophyte isolate, and alkaloids on feeding by Spodoptera frugiperda (Lepidoptera: Noctuidae) larvae. J. Econ. Entomol. 99, 1462–1473. doi: 10.1093/jee/99.4.1462
Ball, O. J. -P., Gwinn, K. D., Pless, C. D., and Popay, A. J. (2011). Endophyte isolate and host grass effects on Chaetocnema pulicaria (Coleoptera: Chrysomelidae) feeding. J. Econ. Entomol. 104, 665–672. doi: 10.1603/EC10262
Banani, H., Marcet-Houben, M., Ballester, A. R., Abbruscato, P., González-Candelas, L., Gabaldón, T., et al. (2016). Genome sequencing and secondary metabolism of the postharvest pathogen Penicillium griseofulvum. BMC Genomics 17:19. doi: 10.1186/s12864-015-2347-x
Barkai-Golan, R. (2008). “Penicillium mycotoxins” in Mycotoxins in Fruits and Vegetables. eds. R. Barkai and N. Paster (San Diego, CA: Academic Press), 153–183.
Barnett, S. E., Cala, A. R., Hansen, J. L., Crawford, J., Viands, D. R., Smart, L. B., et al. (2020). Evaluating the microbiome of hemp. Phytobiomes J. 4, 351–363. doi: 10.1094/PBIOMES-06-20-0046-R
Benedict, K., Thompson, G. R. 3rd, and Jackson, B. R. (2020). Cannabis use and fungal infections in a commercially insured population, United States, 2016. Emerg. Infect. Dis. 26, 1308–1310. doi: 10.3201/eid2606.191570
Bergstrom, G., Starr, J., and Myers, K. (2020). Diseases affecting hemp in New York. Cornell Hemp. Available at: https://bpb-us-e1.wpmucdn.com/blogs.cornell.edu/dist/a/7491/files/2019/08/2019-Hemp-Diseases-Field-Day-Handout8.9.19.pdf (Accessed August 2, 2023).
Bergstrom, G., Starr, J., Myers, K., and Cummings, J. (2019). “An early view of diseases affecting hemp in New York” in Science of hemp: Production and management (University of Kentucky College of agriculture food and environment SR-112). Available at: https://plantpathology.ca.uky.edu/files/sr112.pdf (Accessed October 11, 2021).
Bokhari, F., and Aly, M. M. (2009). Patulin production by Penicillium glabrum isolated from Coffea arabica L. and the activities of some natural antifungal and antimycotoxin plants. Egypt. J. Microbiol. 44, 47–59. doi: 10.21608/EJM.2009.285
Bonnet, M. S., Roux, J., Mounien, L., Dallaporta, M., and Troadec, J. D. (2012). Advances in deoxynivalenol toxicity mechanisms: the brain as a target. Toxins 4, 1120–1138. doi: 10.3390/toxins4111120
Boudra, H., Le Bars, P., and Le Bars, J. (1995). Thermostability of ochratoxin a in wheat under two moisture conditions. Appl. Environ. Microbiol. 61, 1156–1158. doi: 10.1128/aem.61.3.1156-1158.1995
Brandhorst, T., Dowd, P. F., and Kenealy, W. R. (1996). The ribosome-inactivating protein restrictocin deters insect feeding on Aspergillus restrictus. Microbiology 142, 1551–1556. doi: 10.1099/13500872-142-6-1551
Bräse, S., Encinas, A., Keck, J., and Nising, C. F. (2009). Chemistry and biology of mycotoxins and related fungal metabolites. Chem. Rev. 109, 3903–3990. doi: 10.1021/cr050001f
Bretz, M., Beyer, M., Cramer, B., Knecht, A., and Humpf, H. U. (2006). Thermal degradation of the fusarium mycotoxin deoxynivalenol. J. Agric. Food Chem. 54, 6445–6451.
Buchicchio, L., Asselborn, L., Schneider, S., van Nieuwenhuyse, A., Moris, G., and Schummer, C. (2022). Investigation of aflatoxin and ochratoxin a contamination of seized cannabis and cannabis resin samples. Mycotoxin Res. 38, 71–78. doi: 10.1007/s12550-022-00449-z
Bui-Klimke, T. R., and Wu, F. (2015). Ochratoxin a and human health risk: a review of the evidence. Crit. Rev. Food Sci. Nutr. 55, 1860–1869. doi: 10.1080/10408398.2012.724480
Bulgari, D., Fiorini, L., Gianoncelli, A., Bertuzzi, M., and Gobbi, E. (2020). Enlightening gliotoxin biological system in agriculturally relevant Trichoderma spp. Front. Microbiol. 11:200. doi: 10.3389/fmicb.2020.00200
Cai, J., Yan, R., Shi, J., Chen, J., Long, M., Wu, W., et al. (2022). Antifungal and mycotoxin detoxification ability of essential oils: a review. Phytother. Res. 36, 62–72. doi: 10.1002/ptr.7281
California Bureau of Cannabis Control (CBCC) (2018). Bureau of cannabis control announces weekly reports. Available at: https://cannabis.ca.gov/2018/10/bureau-of-cannabis-control-announces-weekly-reports/ (Accessed August 7, 2023).
Caplan, D., Matzneller, P., and Gutierrez, D. (2022). “Harvest and post-harvest” in Handbook of Cannabis Production in Controlled Environments. ed. Y. Zheng (Boca Raton, FL: CRC Press), 291–310.
Carter, B. P. (2019). The what, why and how of water activity in cannabis. Cannabis Sci. Tech. 2, 30–35.
Cescon, D. W., Page, A. V., Richardson, S., Moore, M. J., Boerner, S., and Gold, W. L. (2008). Invasive pulmonary aspergillosis associated with marijuana use in a man with colorectal cancer. J. Clin. Oncol. 26, 2214–2215. doi: 10.1200/JCO.2007.15.2777
Chacon, F. T., Raup-Konsavage, W. M., Vrana, K. E., and Kellogg, J. J. (2022). Secondary terpenes in Cannabis sativa L.: synthesis and synergy. Biomedicine 10:3142. doi: 10.3390/biomedicines10123142
Cheli, F., Ottoboni, M., Fumagalli, F., Mazzoleni, S., Ferrari, L., and Pinotti, L. (2023). E-nose technology for mycotoxin detection in feed: ready for a real context in field application or still an emerging technology? Toxins 15:146. doi: 10.3390/toxins15020146
Chen, J., Chen, Y., Zhu, Q., and Wan, J. (2023). Ochratoxin a contamination and related high-yield toxin strains in Guizhou dried red chilies. Food Control 145:109438. doi: 10.1016/j.foodcont.2022.109438
Chen, S. C. A., Meyer, W., Sorrell, T. C., Halliday, C. L., Landry, M. L., McAdam, A. J., et al. (2019). Manual of Clinical Microbiology 12th Edn. Washington, DC: ASM Press
Chih, S., McDonald, M., Dipchand, A., Kim, D., Ducharme, A., Kaan, A., et al. (2020). Canadian cardiovascular society/Canadian cardiac transplant network position statement on heart transplantation: patient eligibility, selection, and post-transplantation care. Can. J. Cardiol. 36, 335–356. doi: 10.1016/j.cjca.2019.12.025
Chiminelli, I., Spicer, L. J., Maylem, E. R. S., and Caloni, F. (2022). Emerging mycotoxins and reproductive effects in animals: a short review. J. Appl. Toxicol. 42, 1901–1909. doi: 10.1002/jat.4311
Choi, B. K., Cho, J. H., Jeong, S. H., Shin, H. S., Son, S. W., Yeo, Y. K., et al. (2012). Zearalenone affects immune-related parameters in lymphoid organs and serum of rats vaccinated with porcine parvovirus vaccine. Toxicol. Res. 28, 279–288. doi: 10.5487/TR.2012.28.4.279
Comeau, D., Novinscak, A., Joly, D. L., and Filion, M. (2020). Spatio-temporal and cultivar-dependent variations in the cannabis microbiome. Front. Microbiol. 11:491. doi: 10.3389/fmicb.2020.00491
da Silva-Filho, F., de Souza, M., Rezende, G., da Silva, F., da Cruz, J., da Silva, G., et al. (2021). Screening of alkaloid-producing endophytic Penicillium strains from amazon medicinal plants by electrospray ionization mass spectrometry (ESI-MS) and principal component analysis (PCA). J. Braz. Chem. Soc. 32, 1832–1839. doi: 10.21577/0103-5053.20210074
Dai, C., Chen, C., Guan, D., Chen, H., Wang, F., Wang, W., et al. (2020). Pesimquinolones produced by Penicillium simplicissimum and their inhibitory activity on nitric oxide production. Phytochemistry 174:112327. doi: 10.1016/j.phytochem.2020.112327
Dhillon, S. G., Hukkeri, S., Nightingale, D., and Callaway, J. (2022). Evaluation of different techniques to decontaminate medical cannabis and their effect on cannabinoid content. ACS Agric. Sci. Technol. 2, 1126–1133. doi: 10.1021/acsagscitech.2c00231
Díaz, G. A., Torres, R., Vega, M., and Latorre, B. A. (2009). Ochratoxigenic Aspergillus species on grapes from Chilean vineyards and Aspergillus threshold levels on grapes. Int. J. Food Microbiol. 133, 195–199. doi: 10.1016/j.ijfoodmicro.2009.04.018
dos Santos, C. M. C., da Costa, G. L., and Figueroa-Villar, J. D. (2012). Identification of citrinin as the defence metabolite of Penicillium corylophilum stressed with the antagonist fungus Beauveria bassiana. Nat. Prod. Res. 26:2316–2311. doi: 10.1080/14786419.2012.668690
Doshi, P., and Šerá, B. (2023). Role of non-thermal plasma in Fusarium inactivation and mycotoxin decontamination. Plan. Theory 12:627. doi: 10.3390/plants12030627
European Pharmacopoeia Commission (2023). European Pharmacopoeia 11th Edn. France: Council of Europe
Eykelbosh, A. (2021). Unregulated cannabis: risky production practices raise concern for consumers. Available at: https://ncceh.ca/content/blog/unregulated-cannabis-risky-production-practices-raise-concern-consumers (Accessed August 2, 2023).
Ezekiel, C. N., Oyedele, O. A., Kraak, B., Ayeni, K. I., Sulyok, M., Houbraken, J., et al. (2020). Fungal diversity and mycotoxins in low moisture content ready-to-eat foods in Nigeria. Front. Microbiol. 11:615. doi: 10.3389/fmicb.2020.00615
Fehr, K. O., and Kalant, H. (1972). Analysis of cannabis smoke obtained under different combustion conditions. Can. J. Physiol. Pharmacol. 50, 761–767. doi: 10.1139/y72-111
Fei, T., Gwinn, K. D., Leyva-Gutierrez, F. M. A., and Wang, T. (2023). Nanoemulsions of terpene by-products from cannabidiol production have promising insecticidal effect on Callosobruchus maculatus. Heliyon 9:e15101. doi: 10.1016/j.heliyon.2023.e15101
Feizollahi, E., and Roopesh, M. S. (2022). Mechanisms of deoxynivalenol (DON) degradation during different treatments: a review. Crit. Rev. Food Sci. Nutr. 62, 5903–5924. doi: 10.1080/10408398.2021.1895056
Ferrari, E. (2023). Gold nanoparticle-based plasmonic biosensors. Bios 13:411. doi: 10.3390/bios13030411
Feys, S., Lagrou, K., Lauwers, H. M., Haenen, K., Jacobs, C., Brusselmans, M., et al. (2023). High burden of COVID-19-associated pulmonary aspergillosis (CAPA) in severely immunocompromised patients requiring mechanical ventilation. Clin. Infect. Dis. 11:ciad546. doi: 10.1093/cid/ciad546
Fogle, M. R., Douglas, D. R., Jumper, C. A., and Straus, D. C. (2008). Growth and mycotoxin production by Chaetomium globosum is favored in a neutral pH. Int. J. Mol. Sci. 9, 2357–2365. doi: 10.3390/ijms9122357
Fraga, M. E., Direito, G. M., Gatti, M. J., Moraes, A. M. L., Cavaglieri, L. R., and Dalcero, A. M. (2008). Revaluation of aflatoxin production by Aspergillus candidus and Eurotium chevalieri isolated from poultry feed in Brazil. Rev. Bras. Med. Vet. 30, 86–90.
Frink, S., Marjanovic, O., Tran, P., Wang, Y., Guo, W., Encarnacion, N., et al. (2022). Use of X-ray irradiation for inactivation of Aspergillus in cannabis flower. PLoS One 17:e0277649. doi: 10.1371/journal.pone.0277649
Frisvad, J. C. (2018). A critical review of producers of small lactone mycotoxins: patulin, penicillic acid and moniliformin. World Mycotoxin J. 11, 73–100. doi: 10.3920/WMJ2017.2294
Frisvad, J. C., and Filtenborg, O. (1990). “Revision of Penicillium subgenus Furcatum based on secondary metabolites and conventional characters” in Modern Concepts in Penicillium and Aspergillus Classification. eds. R. A. Samson and J. I. Pitt (Boston, MA: Springer), 159–172.
Frisvad, J. C., Houbraken, J., Popma, S., and Samson, R. A. (2013). Two new Penicillium species Penicillium buchwaldii and Penicillium spathulatum, producing the anticancer compound asperphenamate. FEMS Microbiol. Lett. 339, 77–92. doi: 10.1111/1574-6968.12054
Frisvad, J. C., Hubka, V., Ezekiel, C. N., Hong, S. B., Nováková, A., Chen, A. J., et al. (2019). Taxonomy of Aspergillus section Flavi and their production of aflatoxins, ochratoxins and other mycotoxins. Stud. Mycol. 93, 1–63. doi: 10.1016/j.simyco.2018.06.001
Frisvad, J. C., Møller, L. L. H., Larsen, T. O., Kumar, R., and Arnau, J. (2018). Safety of the fungal workhorses of industrial biotechnology: update on the mycotoxin and secondary metabolite potential of Aspergillus niger, Aspergillus oryzae, and Trichoderma reesei. Appl. Microbiol. Biotechnol. 102, 9481–9515. doi: 10.1007/s00253-018-9354-1
Gagnon, M., McRitchie, T., Montsion, K., Tully, J., Blais, M., Snider, N., et al. (2023). High levels of pesticides found in illicit cannabis inflorescence compared to licensed samples in Canadian study using expanded 327 pesticides multiresidue method. J. Cannabis Res. 5:34. doi: 10.1186/s42238-023-00200-0
Gao, M., Glenn, A. E., Gu, X., Mitchell, T. R., Satterlee, T., Duke, M. V., et al. (2020). Pyrrocidine, a molecular off switch for fumonisin biosynthesis. PLoS Pathog. 16:e1008595. doi: 10.1371/journal.ppat.1008595
Gargani, Y., Bishop, P., and Denning, D. W. (2011). Too many mouldy joints—marijuana and chronic pulmonary aspergillosis. Mediter. J. Hematol. Infect. Dis. 3:e2011005. doi: 10.4084/mjhid.2011.005
Gautam, A. K., Kant, M., and Thakur, Y. (2013). Isolation of endophytic fungi from Cannabis sativa and study their antifungal potential. Arch. Phytopathol. Pflanzenschutz. 46, 627–635. doi: 10.1080/03235408.2012.749696
Gauthier, N. W., and Thiessen, L. D. (Ed.). (2022). Compendium of Cannabis Diseases. Minnesota: APS Publications
Gherbawy, Y., and Shebany, Y. (2018). Mycobiota, total aflatoxins and ochratoxin a of cardamom pods. Food Sci. Technol. 24, 87–96. doi: 10.3136/fstr.24.87
Gil-Serna, J., Vázquez, C., and Patiño, B. (2020). Genetic regulation of aflatoxin, ochratoxin a, trichothecene, and fumonisin biosynthesis: a review. Int. Microbiol. 23, 89–96. doi: 10.1007/s10123-019-00084-2
Goldman, S., Bramante, J., Vrdoljak, G., Guo, W., Wang, Y., Marjanoviccaps, O., et al. (2021). The analytical landscape of cannabis compliance testing. J. Liq. Chromatogr. Relat. 44, 403–420. doi: 10.1080/10826076.2021.1996390
Greaves, A., Maddison, K., Doran, M., Lin, S., and Geiling, B. (2021). Single-laboratory validation of an immunoaffinity column cleanup LC method for the analysis of aflatoxins and ochratoxin a in cannabis plant material, resins, vapes, isolates, and edible products. J. AOAC Int. 104, 1264–1271. doi: 10.1093/jaoacint/qsab057
Gruber-Dorninger, C., Novak, B., Nagl, V., and Berthiller, F. (2017). Emerging mycotoxins: beyond traditionally determined food contaminants. J. Agric. Food Chem. 65, 7052–7070. doi: 10.1021/acs.jafc.6b03413
Gwinn, K. D., Hansen, Z., Kelly, H., and Ownley, B. H. (2022). Diseases of Cannabis sativa caused by diverse Fusarium species. Front. Agron. 3:796062. doi: 10.3389/fagro.2021.796062
Hareeri, R. H., Aldurdunji, M. M., Abdallah, H. M., Alqarni, A. A., Mohamed, S. G. A., Mohamed, G. A., et al. (2022). Aspergillus ochraceus: metabolites, bioactivities, biosynthesis, and biotechnological potential. Molecules 27:6759. doi: 10.3390/molecules27196759
Hazekamp, A. (2016). Evaluating the effects of gamma-irradiation for decontamination of medicinal cannabis. Front. Pharmacol. 7:108. doi: 10.3389/fphar.2016.00108
Health Canada (2020). Food and drug regulations, Ottawa. Available at: https://laws-lois.justice.gc.ca/eng/regulations/c.r.c.,_c._870/index.html (Accessed August 2, 2023).
Houbraken, J., Kocsubé, S., Visagie, C. M., Yilmaz, N., Wang, X. C., Meijer, M., et al. (2020). Classification of Aspergillus, Penicillium, Talaromyces and related genera (Eurotiales): an overview of families, genera, subgenera, sections, series and species. Stud. Mycol. 95, 5–169. doi: 10.1016/j.simyco.2020.05.002
Hourfane, S., Mechqoq, H., Bekkali, A. Y., Rocha, J. M., and El Aouad, N. (2023). A comprehensive review on Cannabis sativa ethnobotany, phytochemistry, molecular docking and biological activities. Plan. Theory 12:1245. doi: 10.3390/plants12061245
Ismaiel, A. A., and Papenbrock, J. (2015). Mycotoxins: producing fungi and mechanisms of phytotoxicity. Agriculture 5, 492–537. doi: 10.3390/agriculture5030492
Ismail, A. M., Elshewy, E. S., El-Ganainy, S. M., Magistà, D., Hamouda, A. F., Alhudaib, K. A., et al. (2023). Mycotoxins from tomato pathogenic Alternaria alternata and their combined cytotoxic effects on human cell lines and male albino rats. J. Fungi 9:282. doi: 10.3390/jof9030282
Israel Ministry of Health—Medical Cannabis Unit (IMC) (2017) SOP 152, IMC-GMP production of cannabis for medical use. Available at: https://www.gov.il/BlobFolder/policy/mmk152-2016/he/files_circulars_mmk_mmk152_2016.pdf (Accessed August 2, 2023).
Jameson, L. E., Conrow, K. D., Pinkhasova, D. V., Boulanger, H. L., Ha, H., Jourabchian, N., et al. (2022). Comparison of state-level regulations for cannabis contaminants and implications for public health. Environ. Health Perspect. 130:97001. doi: 10.1289/EHP11206
Jaster-Keller, J., Müller, M. E. H., El-Khatib, A. H., Lorenz, N., Bahlmann, A., Mülow-Stollin, U., et al. (2023). Root uptake and metabolization of Alternaria toxins by winter wheat plants using a hydroponic system. Mycotoxin Res. 39, 109–126. doi: 10.1007/s12550-023-00477-3
Jerushalmi, S., Maymon, M., Dombrovsky, A., and Freeman, S. (2020). Fungal pathogens affecting the production and quality of medical cannabis in Israel. Plan. Theory 9:882. doi: 10.3390/plants9070882
Ji, F., He, D., Olaniran, A. O., Mokoena, M. P., Xu, J., and Shi, J. (2019). Occurrence, toxicity, production and detection of fusarium mycotoxin: a review. Food Prod. Process Nutr 1:6. doi: 10.1186/s43014-019-0007-2
Jiang, H., Zhong, S., Schwarz, P., Chen, B., and Rao, J. (2023). Antifungal activity, mycotoxin inhibitory efficacy, and mode of action of hop essential oil nanoemulsion against fusarium graminearum. Food Chem. 400:134016. doi: 10.1016/j.foodchem.2022.134016
Kabak, B. (2009). The fate of mycotoxins during thermal food processing. J. Sci. Food Agric. 89, 549–554. doi: 10.1002/jsfa.3491
Kagen, S. L., Kurup, V. P., Sohnle, P. G., and Fink, J. N. (1983). Marijuana smoking and fungal sensitization. J. Allergy Clin. Immunol. 71, 389–393. doi: 10.1016/0091-6749(83)90067-2
Kamle, M., Mahato, D. K., Gupta, A., Pandhi, S., Sharma, N., Sharma, B., et al. (2022). Citrinin mycotoxin contamination in food and feed: impact on agriculture, human health, and detection and management strategies. Toxins 14:85. doi: 10.3390/toxins14020085
Karpiński, T. M. (2020). Essential oils of Lamiaceae family plants as antifungals. Biomol. Ther. 10:103. doi: 10.3390/biom10010103
Kim, H. Y., Park, H. M., and Lee, C. H. (2012). Mass spectrometry-based chemotaxonomic classification of Penicillium species (P. echinulatum, P. expansum, P. solitum, and P. oxalicum) and its correlation with antioxidant activity. J. Microbiol. Methods 90, 327–335. doi: 10.1016/j.mimet.2012.06.006
Kolawole, O., Meneely, J., Petchkongkaew, A., and Elliott, C. (2021). A review of mycotoxin biosynthetic pathways: associated genes and their expressions under the influence of climatic factors. Fungal Biol. Rev. 37, 8–26. doi: 10.1016/j.fbr.2021.04.003
Kouevidjin, G., Mazieres, J., Fayas, S., and Didier, A. (2003). Aspergillose bronchopulmonaire allergique aggravée par la consommation de cannabis. Rev. Francaise D. Allergol. Et D. Immunol. Clin. 43, 192–194. doi: 10.1016/S0335-7457(03)00050-9
Krane, K. (2020). Cannabis legalization is key to economic recovery, much like ending alcohol prohibition helped us out of the great depression. Forbe. Available at: https://www.forbes.com/sites/kriskrane/2020/05/26/cannabis-legalization-is-key-to-economic-recovery-much-like-ending-alcohol-prohibition-helped-us-out-of-the-great-depression/?sh=617cd2ad3241 (Accessed August 3, 2023).
Krawczeniuk, A. S., O’Rear, C. E., Mislivec, P. B., Bruce, V. R., Trucksess, M. W., and Llewellyn, G. C. (1987). “Evaluating illicit marihuana for aflatoxins and toxigenic fungi” in Biodeterioration Research 1. eds. G. C. Llewellyn and C. E. O’Rear (Boston, MA: Springer), 149–164.
Kristy, B., Carrell, A. A., Johnston, E., Klingeman, D., Gwinn, K. D., Syring, K. C., et al. (2022). Chronic drought differentially alters the belowground microbiome of drought tolerant and drought susceptible genotypes of Populus trichocarpa. Phytobiomes 6, 317–330. doi: 10.1094/PBIOMES-12-21-0076-R
Kuehn, B. M. (2021). Aspergillosis is common among COVID-19 patients in the ICU. JAMA 326:1573. doi: 10.1001/jama.2021.17973
Kurup, V. P., Resnick, A., Kagen, S. L., Cohen, S. H., and Fink, J. N. (1983). Allergenic fungi and actinomycetes in smoking materials and their health implications. Mycopathologia 82, 61–64. doi: 10.1007/BF00436948
Kusari, P., Kusari, S., Spiteller, M., and Kayser, O. (2013). Endophytic fungi harbored in Cannabis sativa L.: diversity and potential as biocontrol agents against host plant-specific phytopathogens. Fungal Divers. 60, 137–151. doi: 10.1007/s13225-012-0216-3
Lass-Flörl, C., Dietl, A. M., Kontoyiannis, D. P., and Brock, M. (2021). Aspergillus terreus species complex. Clin. Microbiol. Rev. 34:e0031120. doi: 10.1128/CMR.00311-20
Lattanzio, V. M., and Nivarlet, N. (2017). Multiplex dipstick immunoassay for semiquantitative determination of fusarium mycotoxins in oat. Methods Mol. Biol. 1536, 137–142. doi: 10.1007/978-1-4939-6682-0_10
Laut, S., Poapolathep, S., Piasai, O., Sommai, S., Boonyuen, N., Giorgi, M., et al. (2023). Storage fungi and mycotoxins associated with rice samples commercialized in Thailand. Foods 12:487. doi: 10.3390/foods12030487
Levi, M. E., Montague, B. T., Thurstone, C., Kumar, D., Huprikar, S. S., and Kotton, C. N. (2019). Marijuana use in transplantation: a call for clarity. Clin. Transpl. 33:e13456. doi: 10.1111/ctr.13456
Li, B., Chen, Y., Zhang, Z., Qin, G., Chen, T., and Tian, S. (2020). Molecular basis and regulation of pathogenicity and patulin biosynthesis in Penicillium expansum. Compr. Rev. Food Sci. Food Saf. 19, 3416–3438. doi: 10.1111/1541-4337.12612
Li, L., Chmura, S. E., Judd, C. D., and Duffy, B. C. (2023). “New York perspectives of medical cannabis laboratory analysis” in Medicinal Usage of Cannabis and Cannabinoids. eds. V. R. Preedy, V. B. Patel, and C. R. Martin (New York: Academic Press), 77–89.
Lin, K., Chang, J. Y., and Zou, G. (2022). A case of COVID-19-associated pulmonary aspergillosis. Chest 162, A585–A586. doi: 10.1016/j.chest.2022.08.456
Liu, Y., Galani Yamdeu, J. H., Gong, Y. Y., and Orfila, C. (2020). A review of postharvest approaches to reduce fungal and mycotoxin contamination of foods. Compr. Rev. Food Sci. Food Saf. 19, 1521–1560. doi: 10.1111/1541-4337.12562
Liu, Y., Li, M., Bian, K., Guan, E., Liu, Y., and Lu, Y. (2019). Reduction of deoxynivalenol in wheat with superheated steam and its effects on wheat quality. Toxins 11:414. doi: 10.3390/toxins11070414
Llewellyn, G. C., and O'Rear, C. E. (1977). Examination of fungal growth and aflatoxin production on marihuana. Mycopathologia 62, 109–112. doi: 10.1007/BF01259400
Loi, M., Paciolla, C., Logrieco, A. F., and Mulè, G. (2020). Plant bioactive compounds in pre-and postharvest management for aflatoxins reduction. Front. Microbiol. 11:243. doi: 10.3389/fmicb.2020.00243
Mahmoudian, F., Sharifirad, A., Yakhchali, B., Ansari, S., and Fatemi, S. S. (2021). Production of mycophenolic acid by a newly isolated indigenous Penicillium glabrum. Curr. Microbiol. 78, 2420–2428. doi: 10.1007/s00284-021-02509-6
Malmstrøm, J., Christophersen, C., and Frisvad, J. C. (2000). Secondary metabolites characteristic of Penicillium citrinum, Penicillium steckii and related species. Phytochemistry 54, 301–309. doi: 10.1016/s0031-9422(00)00106-0
Martyny, J. W., Serrano, K. A., Schaeffer, J. W., and Van Dyke, M. V. (2013). Potential exposures associated with indoor marijuana growing operations. J. Occup. Environ. Hyg. 10, 622–639. doi: 10.1080/15459624.2013.831986
McKernan, K., Spangler, J., Zhang, L., Tadigotla, V., Helbert, Y., Foss, T., et al. (2015). Cannabis microbiome sequencing reveals several mycotoxic fungi native to dispensary grade cannabis flowers. F1000Res 4:1422. doi: 10.12688/f1000research.7507.2
Micheluz, A., Sulyok, M., Manente, S., Krska, R., Varese, G. C., and Ravgnan, G. (2016). Fungal secondary metabolite analysis applied to cultural heritage: the case of a contaminated library in Venice. World Mycotoxin J. 9, 397–407. doi: 10.3920/WMJ2015.1958
Milani, J. M. (2013). Ecological conditions affecting mycotoxin production in cereals: a review. Vet. Med. 58, 405–411. doi: 10.17221/6979-VETMED
Min, J. Y., and Min, K. B. (2018). Marijuana use is associated with hypersensitivity to multiple allergens in US adults. Drug Alcohol Depend. 182, 74–77. doi: 10.1016/j.drugalcdep.2017.09.039
Mishra, S., Priyanka,, and Sharma, S. (2022). Metabolomic insights into endophyte-derived bioactive compounds. Front. Microbiol. 13:835931. doi: 10.3389/fmicb.2022.835931
Modrzewska, M., Błaszczyk, L., Stępień, Ł., Urbaniak, M., Waśkiewicz, A., Yoshinari, T., et al. (2022). Trichoderma versus Fusarium-inhibition of pathogen growth and mycotoxin biosynthesis. Molecules 27:8146. doi: 10.3390/molecules27238146
Munir, M., Leonberger, K., Kesheimer, K. A., Bolt, M., Zuefle, M., Aronson, E., et al. (2023). Occurrence and distribution of common diseases and pests of US cannabis: a survey. Plant Health Prog. doi: 10.1094/PHP-01-23-0004-S
Nardo, F. D., Cavalera, S., Baggiani, C., Chiarello, M., Pazzi, M., and Anfossi, L. (2020). Enzyme immunoassay for measuring aflatoxin B1 in legal cannabis. Toxins 12:265. doi: 10.3390/toxins12040265
Narváez, A., Rodríguez-Carrasco, Y., Castaldo, L., Izzo, L., and Ritieni, A. (2020). Ultra-high-performance liquid chromatography coupled with quadrupole orbitrap high-resolution mass spectrometry for multi-residue analysis of mycotoxins and pesticides in botanical nutraceuticals. Toxins 12:114. doi: 10.3390/toxins12020114
National Academies of Sciences, Engineering, and Medicine (NASEM) (2017). The Health Effects of Cannabis and Cannabinoids: The Current State of Evidence and Recommendations for Research. Washington, DC: The National Academies Press
Navale, V., Vamkudoth, K. R., Ajmera, S., and Dhuri, V. (2021). Aspergillus derived mycotoxins in food and the environment: prevalence, detection, and toxicity. Toxicol. Rep. 8, 1008–1030. doi: 10.1016/j.toxrep.2021.04.013
Nguyen, L. C., Yang, D., Nicolaescu, V., Best, T. J., Gula, H., Saxena, D., et al. (2022). Cannabidiol inhibits SARS-CoV-2 replication through induction of the host ER stress and innate immune responses. Sci. Adv. 8:eabi6110. doi: 10.1126/sciadv.abi6110
Oar, M. A., Savage, C. H., Rufer, E. S., Rucker, R. P., and Guzman, J. A. (2022). Thermography of cannabis extract vaporization cartridge heating coils in temperature and voltage-controlled systems during a simulated human puff. PLoS One 17:e0262265. doi: 10.1371/journal.pone.0262265
O'Donnell, K., McCormick, S. P., Busman, M., Proctor, R. H., Ward, T. J., Doehring, G., et al. (2018). Toxigenic Fusarium species: identity and mycotoxicology revisited. Mycologia 110, 1058–1080. doi: 10.1080/00275514.2018.1519773
Olt, C., Faulkenberg, K. D., and Hsich, E. M. (2021). The growing dilemma of legalized cannabis and heart transplantation. J. Heart Lung Transplant. 40, 863–871. doi: 10.1016/j.healun.2021.03.024
Ownley, B. H., Gwinn, K. D., and Vega, F. E. (2010). Endophytic fungal entomopathogens with activity against plant pathogens: ecology and evolution. BioControl 55, 113–128. doi: 10.1007/s10526-009-9241-x
Palumbo, J. D., O'Keeffe, T. L., and Mahoney, N. E. (2007). Inhibition of ochratoxin a production and growth of Aspergillus species by phenolic antioxidant compounds. Mycopathologia 164, 241–248. doi: 10.1007/s11046-007-9057-0
Pecoraro, F., Giannini, M., Beccari, G., Covarelli, L., Filippini, G., Pisi, A., et al. (2018). Comparative studies about fungal colonization and deoxynivalenol translocation in barley plants inoculated at the base with Fusarium graminearum, Fusarium culmorum and Fusarium pseudograminearum. AFSci. 27, 74–83. doi: 10.23986/afsci.67704
Pérez-Moreno, M., Pérez-Lloret, P., González-Soriano, J., and Santos-Álvarez, I. (2019). Cannabis resin in the region of Madrid: adulteration and contamination. Forensic Sci. Int. 298, 34–38. doi: 10.1016/j.forsciint.2019.02.049
Perisetti, A., Gajendran, M., Dasari, C. S., Bansal, P., Aziz, M., Inamdar, S., et al. (2020). Cannabis hyperemesis syndrome: an update on the pathophysiology and management. Ann. Gastroenterol. 33, 571–578. doi: 10.20524/aog.2020.0528
Pitt, J. I. (2002). Biology and ecology of toxigenic Penicillium species. Adv. Exp. Med. Biol. 504, 29–41. doi: 10.1007/978-1-4615-0629-4_4
Pruyn, S. A., Wang, Q., Wu, C. G., and Taylor, C. L. (2022). Quality standards in state programs permitting cannabis for medical uses. Cannabis Cannabinoid Res. 7, 728–735. doi: 10.1089/can.2021.0164
Punja, Z. K. (2021a). Emerging diseases of Cannabis sativa and sustainable management. Pest Manag. Sci. 77, 3857–3870. doi: 10.1002/ps.6307
Punja, Z. K. (2021b). First report of Fusarium proliferatum causing crown and stem rot, and pith necrosis, in cannabis (Cannabis sativa L., marijuana) plants. Can. J. Plant Pathol. 43, 236–255. doi: 10.1080/07060661.2020.1793222
Punja, Z. K. (2021c). Epidemiology of fusarium oxysporum causing root and crown rot of cannabis (Cannabis sativa L., marijuana) plants in commercial greenhouse production. Can. J. Plant Pathol. 43, 216–235. doi: 10.1080/07060661.2020.1788165
Punja, Z. K. (2021d). The diverse mycoflora present on dried cannabis (Cannabis sativa L., marijuana) inflorescences in commercial production. Can. J. Plant Pathol. 43, 88–100. doi: 10.1080/07060661.2020.1758959
Punja, Z. K., Collyer, D., Scott, C., Lung, S., Holmes, J., and Sutton, D. (2019). Pathogens and molds affecting production and quality of Cannabis sativa L. Front. Plant Sci. 10:1120. doi: 10.3389/fpls.2019.01120
Punja, Z. K., Li, N., and Roberts, A. J. (2021). The Fusarium solani species complex infecting cannabis (Cannabis sativa L., marijuana) plants and a first report of fusarium (Cylindrocarpon) lichenicola causing root and crown rot. Can. J. Plant Pathol. 43, 567–581. doi: 10.1080/07060661.2020.1866672
Punja, Z. K., and Ni, L. (2022). The bud rot pathogens infecting cannabis (Cannabis sativa L., marijuana) inflorescences: symptomology, species identification, pathogenicity and biological control. Can. J. Plant Pathol. 43, 827–854. doi: 10.1080/07060661.2021.1936650
Punja, Z. K., Ni, L., Lung, S., and Buirs, L. (2023). Total yeast and mold levels in high THC-containing cannabis (Cannabis sativa L.) inflorescences are influenced by genotype, environment, and pre-and post-harvest handling practices. Front. Microbiol. 14:1192035. doi: 10.3389/fmicb.2023.1192035
Punja, Z. K., and Scott, C. S. (2023). Organically grown cannabis (Cannabis sativa L.) plants contain a diverse range of culturable epiphytic and endophytic fungi in inflorescences and stem tissues. Botany 101, 255–269. doi: 10.1139/cjb-2022-0116
Rafei, P., Englund, A., Lorenzetti, V., Elkholy, H., Potenza, M. N., and Baldacchino, A. M. (2023). Transcultural aspects of cannabis use: a descriptive overview of cannabis use across cultures. Curr. Addict. Rep. 10, 458–471. doi: 10.1007/s40429-023-00500-8
Rakk, D., Kukolya, J., Škrbić, B. D., Vágvölgyi, C., Varga, M., and Szekeres, A. (2023). Advantages of multiplexing ability of the orbitrap mass analyzer in the multi-mycotoxin analysis. Toxins 15:134. doi: 10.3390/toxins15020134
Ranjith, A., Srilatha, C. M., Lekshmi, P. C., and Rameshbabu, N. (2021). Antiaflatoxigenic potential of essential oils of spices—a review. World Mycotoxin J. 14, 463–475. doi: 10.3920/WMJ2020.2636
Raters, M., and Matissek, R. (2008). Thermal stability of aflatoxin B1 and ochratoxin a. Mycotoxin Res. 24, 130–134. doi: 10.1007/BF03032339
Reijula, K., and Tuomi, T. (2003). Mycotoxins of aspergilli: exposure and health effects. Front. Biosci. 8, s232–s235. doi: 10.2741/978
Remington, T. L., Fuller, F., and Chiu, I. (2015). Chronic necrotizing pulmonary aspergillosis in a patient with diabetes and marijuana use. CMAJ 87, 1305–1308. doi: 10.1503/cmaj.141412
Root, K. S., Magzamen, S., Sharp, J. L., Reynolds, S. J., Van Dyke, M., and Schaeffer, J. W. (2020). Application of the environmental relative moldiness index in indoor marijuana grow operations. Ann. Work Expo. Health 64, 728–744. doi: 10.1093/annweh/wxaa071
Ryan, K. S., Bash, J. C., Hanna, C. B., Hedges, J. C., and Lo, J. O. (2021). Effects of marijuana on reproductive health: preconception and gestational effects. Curr. Opin. Endocrinol. Diabetes Obes. 28, 558–565. doi: 10.1097/MED.0000000000000686
Ryan, J. E., Noeder, M., Burke, C., Stubblefield, S. C., Sulieman, S., and Miller, E. G. (2019). Denying renal transplantation to an adolescent medical cannabis user: an ethical case study. Pediatr. Transplant. 23:e13467. doi: 10.1111/petr.13467
Salam, A. P., and Pozniak, A. L. (2017). Disseminated aspergillosis in an HIV-positive cannabis user taking steroid treatment. Lancet Infect. Dis. 17:882. doi: 10.1016/S1473-3099(17)30438-3
Samson, R. A., Yilmaz, N., Houbraken, J., Spierenburg, H., Seifert, K. A., Peterson, S. W., et al. (2011). Phylogeny and nomenclature of the genus Talaromyces and taxa accommodated in Penicillium subgenus Biverticillium. Stud. Mycol. 70, 159–183. doi: 10.3114/sim.2011.70.04
Sarma, N. D., Waye, A., ElSohl, M. A., Brown, P. N., Elzinga, S., Johnson, H. E., et al. (2020). Cannabis inflorescence for medical purposes: USP considerations for quality attributes. J. Nat. Prod. 83, 1334–1351. doi: 10.1021/acs.jnatprod.9b01200
Scott, C., and Punja, Z. K. (2023). Biological control of Fusarium oxysporum causing damping-off and Pythium myriotylum causing root and crown rot on cannabis (Cannabis sativa L.) plants. Can. J. Plant Pathol. 45, 238–252. doi: 10.1080/07060661.2023.2172082
Shankar, J., Tiwari, S., Shishodia, S. K., Gangwar, M., Hoda, S., Thakur, R., et al. (2018). Molecular insights into development and virulence determinants of aspergilli: a proteomic perspective. Front. Cell. Infect. Microbiol. 8:180. doi: 10.3389/fcimb.2018.00180
Shapiro, B. B., Hedrick, R., Vanle, B. C., Becker, C. A., Nguyen, C., Underhill, D. M., et al. (2018). Cryptococcal meningitis in a daily cannabis smoker without evidence of immunodeficiency. BMJ Case Rep. 2018:bcr2017221435. doi: 10.1136/bcr-2017-221435
Slinski, S. L., Zakharov, F., and Gordon, T. R. (2015). The effect of resin and monoterpenes on spore germination and growth in Fusarium circinatum. Phytopathology 105, 119–125. doi: 10.1094/PHYTO-02-14-0027-R
Smith, H., Szarka, D., Dixon, E., Adedokun, O., Munir, M., Ricciardi, M., et al. (2023). Emerging Fusarium spp. causing head blight on hemp in Kentucky. Plant Health Prog. 24, 132–134. doi: 10.1094/PHP-09-22-0089-SC
Snigdha, M., Hariprasad, P., and Venkateswaran, G. (2015). Transport via xylem and accumulation of aflatoxin in seeds of groundnut plant. Chemosphere 119, 524–529. doi: 10.1016/j.chemosphere.2014.07.033
Soliman, S. S. M., Baldin, C., Gu, Y., Singh, S., Gebremariam, T., Swidergall, M., et al. (2021). Mucoricin is a ricin-like toxin that is critical for the pathogenesis of mucormycosis. Nat Microbiol. 6, 313–326. doi: 10.1038/s41564-020-00837-0
Southall, A (2022). What’s in New York’s illicit cannabis: germs, toxins and metals. Available at: https://www.nytimes.com/2022/12/01/nyregion/cannabis-bacteria-pesticides-illegal-dispensary.html (Accessed August 2, 2023).
Steenwyk, J. L., Mead, M. E., Knowles, S. L., Raja, H. A., Roberts, C. D., Bader, O., et al. (2020). Variation among biosynthetic gene clusters, secondary metabolite profiles, and cards of virulence across Aspergillus species. Genetics 216, 481–497. doi: 10.1534/genetics.120.303549
Steenwyk, J. L., Shen, X. X., Lind, A. L., Goldman, G. H., and Rokas, A. (2019). A robust phylogenomic time tree for biotechnologically and medically important fungi in the genera Aspergillus and Penicillium. MBio 10, e00925–e01019. doi: 10.1128/mBio.00925-19
Stempfer, M., Reinstadler, V., Lang, A., and Oberacher, H. (2021). Analysis of cannabis seizures by non-targeted liquid chromatography-tandem mass spectrometry. J. Pharm. Biomed. Anal. 205:114313. doi: 10.1016/j.jpba.2021.114313
Stone, T., Henkle, J., and Prakash, V. (2019). Pulmonary mucormycosis associated with medical marijuana use. Respir. Med. Case. Rep. 26, 176–179. doi: 10.1016/j.rmcr.2019.01.008
Suga, H., Hayashi, M., Kushiro, M., Miyano, N., Inoue, H., Nakajima, K., et al. (2022). A novel medium for isolating two Japanese species in the Fusarium graminearum species complex and a dipstick DNA chromatography assay for species identification and trichothecene typing. J. Fungi 8:1048. doi: 10.3390/jof8101048
Sugui, J. A., Pardo, J., Chang, Y. C., Zarember, K. A., Nardone, G., Galvez, E. M., et al. (2007). Gliotoxin is a virulence factor of Aspergillus fumigatus: gliP deletion attenuates virulence in mice immunosuppressed with hydrocortisone. Eukaryot. Cell 6, 1562–1569. doi: 10.1128/EC.00141-07
Sweany, R. R., Breunig, M., Opoku, J., Clay, K., Spatafora, J. W., Drott, M. T., et al. (2022). Why do plant-pathogenic fungi produce mycotoxins? Potential roles for mycotoxins in the plant ecosystem. Phytopathology 112, 2044–2051. doi: 10.1094/PHYTO-02-22-0053-SYM
Szyper-Kravitz, M., Lang, R., Manor, Y., and Lahav, M. (2001). Early invasive pulmonary aspergillosis in a leukemia patient linked to Aspergillus contaminated marijuana smoking. Leuk. Lymphoma 42, 1433–1437. doi: 10.3109/10428190109097776
Taghinasab, M., and Jabaji, S. (2020). Cannabis microbiome and the role of endophytes in modulating the production of secondary metabolites: an overview. Microorganisms 8:355. doi: 10.3390/microorganisms8030355
Tapwal, A., Nisha, G. S., Gautam, N., and Kumar, R. (2011). In vitro antifungal potency of plant extracts against five phytopathogens. Braz. Arch. Biol. Technol. 54, 1093–1098. doi: 10.1590/S1516-89132011000600003
Trivedi, P., Leach, J. E., Tringe, S. G., Sa, T., and Singh, B. K. (2020). Plant-microbiome interactions: from community assembly to plant health. Nat. Rev. Microbiol. 18, 607–621. doi: 10.1038/s41579-020-0412-1
Trullas, J. -C., Bisbe, J., and Miro, J. M. (2010). “Aspergillosis in drug addicts” in Aspergillosis: From Diagnosis to Prevention. ed. A. C. Pasqualotto (Berlin, DE: Springer Science+Business Media), 545–558.
United States Department of Agriculture (USDA) (2020). Economic viability of industrial hemp in the United States: a review of state pilot programs. Available at: https://www.ers.usda.gov/webdocs/publications/95930/eib-217.pdf?v=4476.8 (Accessed August 3, 2023).
United States Department of Agriculture-Economics, Statistics and Market Information System (USDA ESMIS) (2023). National hemp report. Available at: https://downloads.usda.library.cornell.edu/usda-esmis/files/gf06h2430/76538f824/w9506f61g/hempan23.pdf (Accessed May 1, 2023).
United States Environmental Protection Agency (US EPA) (2023). Available at: https://www.epa.gov/pesticide-registration/pesticide-products-registered-use-hemp (Accessed August 2, 2023).
United States Federal Grain Inspection Services (FGIS) (2023). FGIS approved mycotoxin rapid test kits. Available at: https://www.ams.usda.gov/sites/default/files/media/FGISApprovedMycotoxinRapidTestKits.pdf (Accessed July 19, 2023).
United States Food and Drug Administration (FDA) (2010). Guidance for industry and FDA: advisory levels for deoxynivalenol (DON) in finished wheat products for human consumption and grains and grain by-products used for animal feed. Available at: https://www.fda.gov/regulatory-information/search-fda-guidance-documents/guidance-industry-and-fda-advisory-levels-deoxynivalenol-don-finished-wheat-products-human (Accessed August 6, 2023).
United States National Fungus Collections and Fungal Database (NFCFD) (2023). Available at: https://data.nal.usda.gov/dataset/united-states-national-fungus-collections-fungus-host-dataset (Accessed August 8, 2023).
United States Pharmacopeia (USP) (2019). General chapter <561> articles of botanical origin. Available at: https://hmc.usp.org/system/files/general-chapters/GC-Pdfs_2023/561%20Articles%20of%20Botanical%20Origin.pdf (Accessed May 1, 2023).
Vanhove, W., Cuypers, E., Bonneure, A. J., Gotink, J., Stassen, M., Tytgat, J., et al. (2018). The health risks of Belgian illicit indoor cannabis plantations. J. Forensic Sci. 63, 1783–1789. doi: 10.1111/1556-4029.13788
Varga, J., Frisvad, J. C., and Samson, R. (2009). A reappraisal of fungi producing aflatoxins. World Mycotoxin J. 2, 263–277. doi: 10.3920/WMJ2008.1094
Veit, M. (2023). Quality requirements for medicinal cannabis and respective products in the European Union—status quo. Planta Med. 89, 808–823. doi: 10.1055/a-1808-9708
Wang, G. S., Buttorff, C., Wilks, A., Schwam, D., Tung, G., and Pacula, R. L. (2021). Changes in emergency department encounters for vomiting after cannabis legalization in Colorado. JAMA Netw. Open 4:e2125063. doi: 10.1001/jamanetworkopen.2021.25063
Wang, Y., Wang, L., Liu, F., Wang, Q., Selvaraj, J. N., Xing, F., et al. (2016). Ochratoxin a producing fungi, biosynthetic pathway and regulatory mechanisms. Toxins 8:83. doi: 10.3390/toxins8030083
Wei, G., Ning, K., Zhang, G., Yu, H., Yang, S., Dai, F., et al. (2021). Compartment niche shapes the assembly and network of Cannabis sativa-associated microbiome. Front. Microbiol. 12:714993. doi: 10.3389/fmicb.2021.714993
Wilcox, J., Pazdanska, M., Milligan, C., Chan, D., MacDonald, S. J., and Donnelly, C. (2020). Analysis of aflatoxins and ochratoxin a in cannabis and cannabis products by LC-fluorescence detection using cleanup with either multiantibody immunoaffinity columns or an automated system with in-line reusable immunoaffinity cartridges. J. AOAC Int. 103, 494–503. doi: 10.5740/jaoacint.19-0176
Williams, K., and Buchert, S. (2020). International regulation and control of hemp and cannabinoids. Cayman Chemical News and Announcements. Available at: https://www.caymanchem.com/news/international-regulation-and-control-of-hemp-and-cannabinoids (Accessed August 3, 2023).
Xiong, Y., Li, W., Wen, Q., Xu, D., Ren, J., and Lin, Q. (2022). Aptamer-engineered nanomaterials to aid in mycotoxin determination. Food Control 135:108661. doi: 10.1016/j.foodcont.2021.108661
Yin, Y., Cai, M., Zhou, X., Li, Z., and Zhang, Y. (2016). Polyketides in Aspergillus terreus: biosynthesis pathway discovery and application. Appl. Microbiol. Biotechnol. 100, 7787–7798. doi: 10.1007/s00253-016-7733-z
Yin, G., Zhang, Y., Pennerman, K. K., Wu, G., Hua, S. S. T., Yu, J., et al. (2017). Characterization of blue mold Penicillium species isolated from stored fruits using multiple highly conserved loci. J. Fungi 3:12. doi: 10.3390/jof3010012
Zaka, M., Hashmi, S., Siddiqui, M., Rahman, L., Mushtaq, S., Ali, H., et al. (2021). Callus-mediated biosynthesis of ag and ZnO nanoparticles using aqueous callus extract of Cannabis sativa: their cytotoxic potential and clinical potential against human pathogenic bacteria and fungi. Green Process Synth. 10, 569–584. doi: 10.1515/gps-2021-0057
Zhao, Z., Lou, Y., Shui, Y., Zhang, J., Hu, X., Zhang, L., et al. (2021). Ochratoxigenic fungi in post-fermented tea and inhibitory activities of Bacillus spp. from post-fermented tea on ochratoxigenic fungi. Food Control 126:108050. doi: 10.1016/j.foodcont.2021.108050
Keywords: total yeast and mold, quality control, opportunistic infection, mycotoxins, health risks, cannabis safety
Citation: Gwinn KD, Leung MCK, Stephens AB and Punja ZK (2023) Fungal and mycotoxin contaminants in cannabis and hemp flowers: implications for consumer health and directions for further research. Front. Microbiol. 14:1278189. doi: 10.3389/fmicb.2023.1278189
Edited by:
Jeff Coleman, Auburn University, United StatesReviewed by:
Irene Valenti, University of Milan, ItalyKevin McKernan, Medicinal Genomics Corporation, United States
Copyright © 2023 Gwinn, Leung, Stephens and Punja. This is an open-access article distributed under the terms of the Creative Commons Attribution License (CC BY). The use, distribution or reproduction in other forums is permitted, provided the original author(s) and the copyright owner(s) are credited and that the original publication in this journal is cited, in accordance with accepted academic practice. No use, distribution or reproduction is permitted which does not comply with these terms.
*Correspondence: Kimberly D. Gwinn, kgwinn@utk.edu