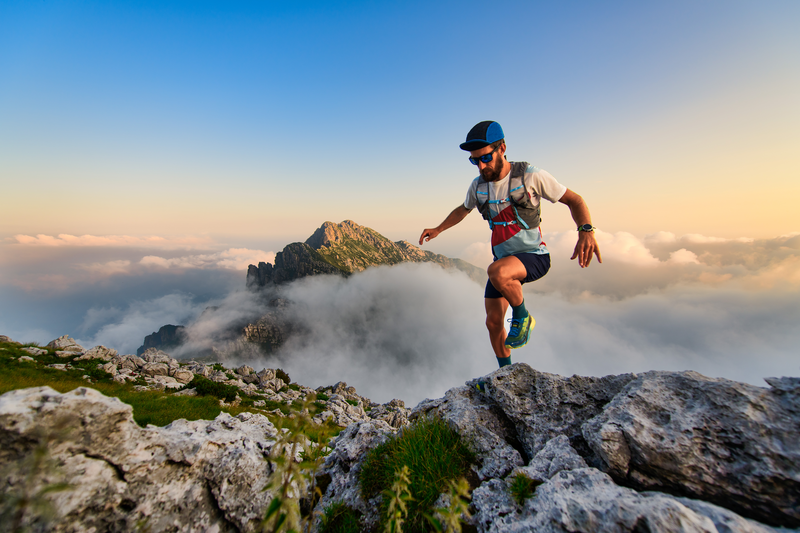
94% of researchers rate our articles as excellent or good
Learn more about the work of our research integrity team to safeguard the quality of each article we publish.
Find out more
ORIGINAL RESEARCH article
Front. Microbiol. , 20 October 2023
Sec. Microbe and Virus Interactions with Plants
Volume 14 - 2023 | https://doi.org/10.3389/fmicb.2023.1276932
Sugarcane mosaic and leaf fleck diseases are significant viral diseases affecting sugarcane crops in India. The use of resistant sugarcane varieties is considered the most economical and effective approach to manage viral diseases, especially in vegetatively propagated crops such as sugarcane. Sugarcane mosaic virus (SCMV) and Sugarcane streak mosaic virus (SCSMV) are the primary pathogens responsible for mosaic disease in sugarcane-growing regions of India. Sugarcane bacilliform virus (SCBV), causing leaf fleck disease, is also often found in mixed infections with mosaic symptoms. The study aimed to identify new sources of resistance by screening sugarcane germplasm for resistance to SCMV, SCSMV, and SCBV. The screening was carried out under high inoculum using the infector row method in both plant and ratoon crops. Out of 129 genotypes tested, only 8 were found to be free of mosaic viruses, indicating a rare occurrence of resistant sources. The study revealed that mosaic disease is widespread, with nearly 95% of tested varieties/genotypes being infected with mosaic viruses. SCMV, SCSMV, and SCBV were detected in 121 out of 129 genotypes using the RT-PCR and PCR assays. Based on their response to the viruses, the tested genotypes were categorized into different resistance grades: highly resistant (grade 1), resistant (grade 2), moderately resistant (grade 3), susceptible (grade 4), and highly susceptible (grade 5). The results of the study provide valuable information about elite resistance resources that can be used for the prevention and control of mosaic disease. These resistant genotypes could also serve as potential donors for mosaic and leaf fleck disease resistance in breeding programs.
Sugarcane is a perennial grass of the genus Saccharum and family Poaceae. It is cultivated as a vital field crop for sugar and bioenergy production and is grown in more than 110 countries worldwide, covering about 26.34 million hectares and yielding approximately 1,859.39 million tons. Approximately 80% of the world’s sugar demand is met by this crop. Sugarcane has had a historical presence in Asian farmlands since ancient times and continues to occupy a significant place in agriculture and the economy. Major sugarcane-producing countries include India, Brazil, China, Thailand, Pakistan, Mexico, Colombia, Indonesia, the Philippines, and the United States. India stands out as the second-largest producer, accounting for approximately 20% of the world’s cane production, covering 5.15 M ha–1 with a production of 405.39 Mt (Food and Agriculture Organization of the United Nations, 2021). The Asian continent, particularly Southeast Asia, being the origin of the crop, is known for its rich genetic diversity. The economic significance of the crop extends beyond sugar to include products like bagasse, crystal white sugar, power, ethanol, and press mud. It is also considered as a potential energy cane/biofuel crop (Matsuoka et al., 2014). Such a potential crop is threatened by viral, fungal, bacterial, and phytoplasmal diseases. Among these, viral diseases are becoming a major concern, leading to varietal degeneration (Viswanathan, 2016). Viral diseases such as mosaic, yellow leaf, and leaf fleck have been reported in sugarcane-growing regions, impacting cane yield and juice quality (Viswanathan et al., 2018).
Sugarcane mosaic disease is primarily caused by the infection of Sugarcane mosaic virus (SCMV), Sugarcane streak mosaic virus (SCSMV), and Sorghum mosaic virus (SrMV). These viruses infect sugarcane plants and lead to the manifestation of mosaic-like symptoms, which involve the development of irregular patterns of light and dark green on the leaves (Lu et al., 2021). Studies have demonstrated that sugarcane mosaic disease in India is attributed to SCMV and SCSMV infections, either alone or in conjunction (Hall et al., 1998; Hema et al., 1999; Viswanathan et al., 2007). Sugarcane mosaic disease, exhibiting typical “mosaic” symptoms (Krishna et al., 2023b), can significantly reduce the sett germination, photosynthetic efficiency, yield, and quality of sugarcane (Costa and Muller, 1982; Smith et al., 1992; Bagyalakshmi et al., 2019). Infected plants are likely to produce fewer stalks and impact the economic value of the harvest. Throughout history, pandemic outbreaks of sugarcane mosaic disease have led to substantial economic losses in the sugarcane industry. These losses have been severe enough to cause financial difficulties and even bankruptcies for sugar companies around the world (Koike and Gillaspie, 1989; Grisham, 2011). Higher yield losses arising due to the severe incidence of this disease led to the discontinuation of several sugarcane cultivars (Singh et al., 1997). Developing sugarcane varieties that are resistant to viruses and rational planting is a key strategy to prevent and control the disease.
Sugarcane streak mosaic virus and SCMV have a broad host range that includes not only sugarcane but also other grass hosts like maize and sorghum, making viruses more adaptable and capable of infecting different plant species (Viswanathan et al., 2018). In India, the incidence of mosaic disease is approximately 100%. This widespread prevalence contributes to a significant yield reduction in elite sugarcane cultivars ranging from 30 to 80% (Singh et al., 2003; Viswanathan and Balamuralikrishnan, 2005; Rao et al., 2006). Mixed infections involving SCMV, SCSMV, and Sugarcane bacilliform virus (SCBV) have been reported in India. These mixed infections can lead to more severe symptoms, such as dwarfing and necrosis, resulting in substantial production losses for susceptible sugarcane cultivars (Hema et al., 2003; Singh et al., 2009). Severe infections of SCBV in susceptible sugarcane cultivars have been associated with biomass production losses of 25–35% (Comstock and Lockhart, 1996; Lockhart and Autrey, 2000). SCMV is transmitted by various aphid species in a non-persistent manner, making it challenging to control the spread of the virus in the field (Hassan et al., 2003). Developing resistant sugarcane varieties can provide a significant resource for minimizing the damage caused by SCMV and related viruses.
Identification and cultivating resistant varieties are the only available options with respect to the management of viral diseases in sugarcane. Although using virus-free planting material lessens the damage that viruses do to plant crops, ratoon crops experience more severe damage as a result of viral transmission through vectors under field conditions. Therefore, in order to control the disease through host resistance, it is crucial to identify resistant sources from the parents and wild germplasm of sugarcane. Host plant resistance is the ultimate tool to manage viral diseases in vegetatively propagated crops like sugarcane. Few researchers have been working on identifying the resistant sources for sugarcane mosaic disease. Recently, Bagyalakshmi and Viswanathan (2021) screened 210 genotypes, with an emphasis on developing a mosaic scoring system. Furthermore, researchers have primarily confined to a few available cultivars, aiming to identify a resistant source for the sugarcane mosaic and leaf fleck diseases. This study examined the germplasm of sugarcane, including commercial hybrid varieties, genotypes, and recently released varieties, for their symptom manifestation under high inoculum pressure for two consecutive years.
A total of 129 sugarcane genotypes were planted in the experimental field of the Division of Plant Pathology at Regional Agricultural Research Station (RARS), Anakapalli, for the evaluation of mosaic and leaf fleck resistance during the period 2021–2022 (plant crop) and 2022–2023 (ratoon crop) crop growing seasons by the infector row method. Entries were planted in two rows of 5 m in length, and 25 three-budded setts were planted in each row. The experiment was laid out in an augmented block design with a susceptible check, 2009A 107, repeated after three entries. Resistance in all the entries was confirmed through RT-PCR and PCR at the formative and maturity stages. Entries that were found to be highly resistant under field conditions in plant crops were planted in a glass house in the next season along with the susceptible check and were inoculated with sugarcane mosaic-infected sap 45 days after planting. Furthermore, resistance was confirmed with RT-PCR and PCR.
The list of 129 sugarcane genotypes evaluated for mosaic and leaf fleck resistance studied in the present study is given in Table 1. The test genotypes were artificially inoculated with the sap of mosaic-infected sugarcane leaves, tested with RT-PCR and PCR assays for the presence of viruses causing mosaic and leaf flecks, and monitored periodically for the manifestation of symptoms typical of the diseases. The types of visual symptoms expressed by the genotypes were recorded from April to November during both crop seasons, namely 2021–2022 and 2022–2023 plant crop and ratoon, respectively. The disease index was recorded in 129 sugarcane genotypes. Throughout the study period, the sugarcane genotypes were consistently monitored for the manifestation of mosaic disease symptoms. The types of visual symptoms displayed by each genotype were carefully recorded and documented. Observations on mosaic incidence were recorded, and percentage incidence was calculated using the below-mentioned formula:
Table 1. List of 129 sugarcane genotypes used for the field evaluation for mosaic and leaf fleck disease resistance during 2021–2022 and 2022–2023 crop growing seasons.
Based on the disease incidence, tested genotypes were categorized according to Li et al. (2018) and mentioned below.
Disease index scale for mosaic disease of sugarcane
Grade | Disease index (%) | Reaction |
1 | 0.00 | Highly resistant (HR) |
2 | 1–10.0 | Resistant (R) |
3 | 10.1–33 | Moderately resistant (MR) |
4 | 33.1–66 | Susceptible (S) |
5 | 66.1–100 | Highly susceptible (HS) |
The genotypes that were found to be highly resistant in plant crops were planted in a glass house and mechanically inoculated with crude sap from RT-PCR-determined SCMV and SCSMV and PCR-determined SCBV-infected sugarcane leaves extracted in 0.1 M phosphate buffer, pH 7.0 (Użarowska et al., 2009).
Total DNA was extracted from the samples collected by following the CTAB technique (Doyle and Doyle, 1987). Leaf samples (each 100 mg), kept at −86°C, were ground to a fine powder using liquid nitrogen in a mortar and pestle. CTAB buffer of about 1 ml was added to the finely powdered samples in a 1.5 ml sterile microcentrifuge tube. The samples were then incubated in a water bath at 65°C for 45 min and allowed to cool to room temperature, followed by centrifugation at 4°C for 10 min at 12,000 rpm. The supernatant was collected in a fresh microcentrifuge tube, followed by the addition of chloroform and isoamyl alcohol (24:1). Following a thorough mixing process, tubes were centrifuged at 4°C for 10 min at 12,000 rpm. Three layers were formed, i.e., upper aqueous phase, middle protein phase, and lower organic phase, among the three upper aqueous phases, which included DNA, and were moved into a fresh microcentrifuge tube, followed by the addition of 0.6 volume of isopropanol, and mixed by gentle inversion for 4–5 times. Later, DNA was allowed to precipitate at 4°C for 30 min. After that, tubes were centrifuged for 10 min at 12,000 rpm to form pellets. The pellet was then centrifuged at 4°C for 10 min at 12,000 rpm with 75% ethanol. The pellet was retained, and the supernatant was discarded. The pellet was then allowed to dissolve in 40 μl of sterile distilled water after being air-dried.
Primers used for PCR amplification
Virus | Region | Synthetic oligonucleotide primers (5′–3′) | References |
SCMV | Coat protein | CCCGAAGCTTGCTGG AACAGTCGATGCAGG | Viswanathan et al., 2010 |
ATCGCGGCCGCTTA GCCAGCTGTGTGTCTCT | |||
SCSMV | Coat protein | GGATCCGGACAAGGAA CGCAGCCAC | Viswanathan et al., 2010 |
AGATCTCGCACGTC GATTTCTGCTGGTG | |||
SCBV | RT/RNase H | GCRCCWGCAGTVTT YCARAGGAAGATG | Krishna et al., 2023a |
CCAYCTGATCTCH GAAGGYTTRTG |
The most conserved RT/RNase H domains and sequences have been widely employed in Badnavirus taxonomy and viral detection. Primers specific to SCBV were used in the PCR reaction to target the RT/RNase H coding region of the genome, which is a region of 794 bp in size. A PCR reaction of 25 μl includes 2 μl of DNA, 2 μl Taq buffer, 2 μl Mgcl2, 2 μl dNTPs, 10 pmol forward and reverse primer, 1.25 units Taq DNA polymerase (Genei, Bangalore), and sterile Milli-Q water. A thermocycler (Mastercycler, Eppendorf) was used to carry out the PCR reaction at an annealing temperature of 59°C for 45 s. The PCR products were separated by electrophoresis and visualized under a gel documentation system (Vilber E: Box, UK).
All the samples collected from 129 sugarcane genotypes at vegetative and grand growth stages were subjected to RNA extraction. In total, 100 mg of fresh sample was powdered using liquid nitrogen to ensure rapid and thorough disruption of cellular structures. The powdered sample is then transferred to a microcentrifuge tube treated with DEPC and mixed with 1 ml of TRI reagent. The sample and TRI reagent mixture are vigorously shaken to ensure proper mixing and then incubated at 4°C. This allows time for the nucleoprotein complexes to dissociate and for RNA to be released from the cells. After incubation, the mixture is centrifuged at a high speed of 12,000 rpm and kept at a low temperature (4°C) for 10 min. The supernatant (liquid phase) containing the RNA was transferred to a new tube, and 200 μl of chloroform was added. The mixture was shaken to create an emulsion and then incubated at room temperature. This step helps to separate different phases within the solution. The mixture was centrifuged again at 12,000 rpm and 4°C for 15 min. This step leads to the separation of the solution into three distinct phases: a lower organic phenolic phase containing proteins, an inter-phase containing DNA, and an upper colorless aqueous phase containing RNA. The upper aqueous phase, which contains the RNA, is carefully transferred to a new tube. Then, 500 μl of isopropanol is added to the RNA-containing solution, causing the RNA to precipitate out of the solution. The mixture is centrifuged again to form a pellet and washed thrice with ethanol. The pellet is air-dried for 10 min to remove any residual ethanol. Then, the RNA pellet is dissolved in 40 μl of molecular-grade water (Chomczynski and Sacchi, 1987; Kumar et al., 2023). The resulting RNA solution was stored at −86°C for further analysis. The quality of the extracted RNA was assessed by running it on a 1.5% agarose gel, which can provide information about the integrity and concentration of the RNA sample.
The first-strand cDNA synthesis kit (Thermo Scientific) was used for the synthesis of cDNA. The reverse transcription was performed in a 10 μl reaction mixture that contained 2 μl 10 mM dNTPs, 4.375 μl ddH2O, 2 μl RT buffer, 0.25 μl Oligod (T), 0.125 μl RT enzyme, 0.25 μl RNase inhibitor, and 2 μl RNA template. The reaction mixture was incubated at 70°C for 5 min, followed by inactivation of the enzyme at 37°C for 90 min, and termination of the reaction at 72°C for 10 min. A PCR reaction volume of 25 μl consisting of 2 μl of cDNA, 2 μl of Taq buffer, 2 μl of Mgcl2, 2 μl of dNTPs, 10 pmol of both forward and reverse primers, 1.25 units of Taq DNA polymerase (Genei, Bangalore), and sterile Milli-Q water makeup to the final volume. Thermocycler (Mastercycler, Eppendorf) was used to carry out the PCR reaction with an annealing temperature of 65°C for 45 s. The PCR products were separated by electrophoresis and visualized under a gel documentation system (Vilber E: Box, UK).
Representative samples of the amplicons from all three viruses were chosen for sequencing. Amplicons were purified and sequenced by Sanger’s dideoxy chain termination method. After obtaining the sequencing data, nucleotide homology searches were performed using the BLASTN algorithm on the NCBI database. The sequences were aligned using the MUSCLE algorithm (Edgar, 2004). A phylogenetic tree was constructed to visualize the evolutionary relationships between the sequences using the MEGA X software (Kumar et al., 2018). The maximum-likelihood (ML) criterion is used, and the neighbor-joining method is applied to build the tree.
Screening of 129 elite sugarcane genotypes for broad-based resistance to mosaic was taken up in both plant crop and ratoon under natural field conditions, along with a susceptible check, 2009A 107, at the experimental field of RARS, Anakapalli. Among the tested (129) genotypes, 8 genotypes (2017A 553, 2017A 416, 2017A 517, 2016A 379, Co7717, Co S 767, Baragua, and Co 13034) were scored as highly resistant (grade 1) with null disease index, eight genotypes (2017A 340, 2016A 381, Co 975, Co 1148, Co Se 95422, BO 91, Co J 64, and Co Lk 15206) were scored as resistant (grade 2), 59 genotypes were scored as moderately resistant (grade 3), 39 genotypes were scored as susceptible (grade 4), and 15 genotypes were scored as highly susceptible (grade 5) to mosaic disease in plant crop (Table 2). The susceptible check, 2009A 107, showed 100% incidence. In the ratoon crop, 8 genotypes showed a highly resistant reaction, 5 genotypes showed a resistant reaction, 55 genotypes showed a moderately resistant reaction, 44 genotypes showed a susceptible reaction, and 17 genotypes showed a highly susceptible reaction (Table 2). The 129 genotypes have shown a wide range in the phenotypic expression of mosaic. The tested genotypes exhibited typical mosaic symptoms between 30 and 250 days after planting. Susceptible genotypes produced variable patterns of mosaic symptoms (Figure 1). The initial symptoms of the disease were characterized by the appearance of chlorotic points with linear distribution in the central portion of the leaf, most commonly at the base, which evolved into typical symptoms such as small chlorotic areas interspersed by green bands throughout the length of the leaf. In the case of highly resistant genotypes, there were no symptoms observed as they were lush green throughout the two cropping seasons. In the case of resistant to moderately resistant genotypes, a mild mosaic of chlorotic patterns to severe mosaic symptoms was observed, whereas in the case of highly susceptible genotypes, systemic yellowing, marginal drying of leaves, and severe stunting were observed. In the case of leaf flecks, under field conditions, the affected genotypes exhibited pronounced flecks, chlorotic stripes, mottling, and stunted growth. The disease started as intense white, isolated flecks on the lamina, followed by the spreading of flecks to the top two to three leaves. Later, flecks became severe, with yellowish discoloration on the lamina followed by complete reddening and early drying of the leaves. In all of the genotypes tested, SCSMV was found to be more prevalent than SCMV and SCBV. The tested genotypes exhibited mosaic symptoms, with disease index ranging from 5.50 to 100 and 6.75 to 100% during the 2021–2022 and 2022–2023 crop growing seasons, respectively. Categorization of sugarcane genotypes into different resistance grades based on field reaction to mosaic under plant and ratoon crop situations (Figures 2, 3). Reddening of mature old leaves is the most commonly observed symptom in the genotype 2009A 107. Mild to intense flecks on old leaves were observed in the cultivar 87A 298. Most of the genotypes, 2003V 46, Co 86032, 81V 48, and 2015A 311, exhibited mild to severe white flecks as an initial symptom in the terminal portion of the leaf; later, these resulted in complete mottling of the leaves.
Table 2. Identification of sugarcane genotypes for their broad-based resistance against mosaic and leaf fleck diseases during 2021–2022 (plant crop) and 2022–2023 (ratoon crop) seasons.
Figure 1. (a) Chlorotic areas alternate with green portions on the basal portion of the young leaf due to SCMV infection; (b) SCMV-infected leaves show yellow blotches throughout the lamina; (c) green inlays alternate with parallel veins due to SCSMV infection; and (d) extensive discoloration of the crop canopy due to SCBV infection.
Figure 2. Mosaic symptoms varied from cultivar to cultivar: (a) chlorotic areas on pale green lamina (87A 298); (b) systemic yellowing and marginal drying of leaf lamina (Co 86032); (c) systemic yellowing, drying, and stunting (2009A 107); (d) pale yellow-green leaves (Co 997); (e) chlorotic streaks expand to large chlorotic patches (2009A 107); (f) chlorotic streaks (93A 145); (g) systemic yellowing and complete drying (2015A 93); and (h) yellow chlorotic areas on green lamina (2018A 166).
Figure 3. Categorization of sugarcane genotypes into different resistance grades based on field reaction to mosaic under plant and ratoon crop situations.
Field resistance of sugarcane genotypes based on visual grading was further confirmed by RT-PCR/PCR detection of SCMV, SCSMV, and SCBV in symptomatic and asymptomatic leaf samples from 129 sugarcane genotypes. The 891, 690, and 794 bp expected fragments of the SCMV and SCSMV coat protein genes and the RT-RNase H region gene were not amplified in the eight samples obtained from sugarcane genotypes, which were graded as highly resistant (grade 1) to all three viruses in the plant crop. These expected amplicons were present in all resistant to highly susceptible tested genotypes rated as grades 2–5 (Figure 4). Genotypes that were scored as highly resistant under field conditions in the plant crop were further tested under greenhouse conditions in the next season by artificial inoculation 45 days after planting. The results of the study clearly showed that all the genotypes rated as grade 1 under field conditions remained highly resistant under greenhouse evaluation except 2017A 340 and 2016A 381.
Figure 4. RT-PCR and PCR amplification of the coat protein gene and RT-RNase H region of (a) SCMV, (b) SCSMV, and (c) SCBV from mosaic and leaf fleck affected sugarcane genotypes, respectively (numbers 1, 2, 3,… indicate positive amplification from mosaic and leaf fleck susceptible genotypes).
The genotypes 2009A 107, 93A 145, 87A 298, 206A 223, 2018 202, 2018A 107, 2017A 497, 2018A 135, Co 997, Khakai, CoC 671, Co 62399, and Co 86032 displayed severe mosaic symptoms and could be quickly identified as diseased even from a few yards away. Younger leaves, often the center leaf, in particular, displayed severe mosaic symptoms such as green streaks and mosaic symptoms throughout the leaf lamina in all tested genotypes. The typical mosaic green inlays/streaks were found to be confined to the proximal part of the leaf lamina in various genotypes, including 2000A 225, 98A 163, 2010A 229, and CoLk 14203, whereas the distal end was devoid of the mosaic symptoms.
At 2–3 months of age, the sugarcane genotypes 2016A 254, 2015A 309, 81A 99, 2006A 223, Co 86032, Co 15024, Co 740, and CoC 671 were reported to exhibit mosaic symptoms; however, when the crop was checked again at sixth and seventh months, there were no such symptoms. The symptoms progressively subsided as the leaves grew older. Similar kinds of gradual disappearing symptoms with the aging of the leaves were observed by Balamuralikrishnan et al. (2003). Therefore, it has been determined that as the crop ages, the mosaic virus titer drops. However, it was found that the majority of the genotypes under study had symptoms throughout their growth phase and did not fully recover from mosaic until the end of the cropping seasons. From the second to the ninth month of crop age, Co se 95422, BO 91, and CoJ 64 were all lush green and free of any evidence of mosaic, but RT-PCR assays showed the presence of virus infection. RT-PCR assays for the genotypes 2017A 340 and 2016A 381 showed the absence of virus infection in plant crops. The same genotypes showed the presence of SCMV and SCSMV infection in RT-PCR assays in ratoon even though they are asymptomatic and may act as carriers of viruses (Figure 4).
The representative nucleotide sequences of SCMV, SCSMV, and SCBV were subjected to BLAST analysis and shared maximum nucleotide homology with most of the Indian isolates. Phylogenetic analysis was performed with the nucleotide sequences of SCMV, SCSMV, and SCBV, along with corresponding sequences available in NCBI. The isolates of SCMV, SCSMV, and SCBV clustered with most of the other Indian and foreign isolates (Figure 5).
Figure 5. Phylogenetic analysis of nucleotide sequences of (A) SCMV, (B) SCSMV, and (C) SCBV along with retrieved sequences from NCBI. The scale bar represents 0.10 and 0.02 substitutions per nucleotide position for SCMV, SCSMV, and SCBV sequences, respectively (the red triangle indicates the current study virus isolate).
Mosaic and leaf fleck diseases of sugarcane are systemic diseases most commonly transmitted through infected seed cane. Bagyalakshmi et al. (2019) reported that mixed infections of SCMV and SCSMV lead to severe degeneration of cv. CoJ 64. Drastic reductions in cane growth and yield due to the mixed infection of mosaic viruses and their association with SCBV in causing varietal degeneration of popular cultivated varieties were reported in India (Singh et al., 2003; Viswanathan and Balamuralikrishnan, 2005; Viswanathan, 2016). Despite the serious prevalence of mosaic and leaf fleck diseases of sugarcane in India, there have not been significant efforts to identify the resistance sources against these diseases. Identifying and developing resistant cultivars is crucial for managing and minimizing the impact of diseases on sugarcane crops.
The most economical, practical, and effective strategy for controlling mosaic and leaf fleck diseases is to identify, develop, and cultivate sugarcane varieties that are resistant to the diseases. Resistance to multiple viruses is crucial because it can provide broader protection against different strains of the disease and reduce the chances of new infections or virus evolution. Breeding disease-resistant cultivars begins with the exploration and use of a genetic resource. Furthermore, systematic studies are required to understand the single or compound infection of viruses to address the resistance to mosaic and leaf fleck in India. In the current study, resistance to SCMV and SCSMV, the two major causes of mosaic disease, and SCBV, the cause of leaf fleck disease, was identified in 129 new elite sugarcane varieties/clones in both plant and ratoon crops. The tested genotypes exhibited typical mosaic symptoms varying with disease index ranging from 5.50 to 100 and 6.75 to 100% in plant and ratoon crops, respectively. Similar results were reported by Bagyalakshmi and Viswanathan (2020), who reported that seven genotypes were found to be free of mosaic viruses out of 210 genotypes tested. Similarly, Li et al. (2018) and Li et al. (2019) reported that 23 genotypes were found to be resistant to or moderately resistant to mosaic among the 71 genotypes tested. Silva et al. (2015) identified that 22 genotypes were found to be resistant to SCMV among 79 sugarcane genotypes, and resistance was confirmed with plate-trapped antibody ELISA. Disease index, as well as symptom expression, was higher in the case of the ratoon crop. Irrespective of the genotype, disease severity increased in ratoon crops. The percentage of mosaic incidence was high in the ratoon crop when compared to the plant crop. The percentage of disease increased in the ratoon crop compared to the plant crop and varied among the genotypes from 0 to 60%. Similar results were reported by Guadie et al. (2019), who stated that the percentage of mosaic disease increase in the ratoon crop ranged from 18 to 83%. Moreover, symptom expression was earlier in sugarcane genotypes in the ratoon crop than in the plant crop. Complete yellowing of leaves and stunting at an early stage were observed in severely infected genotypes in the ratoon crop.
To confirm the presence or absence of the virus, RT-PCR/PCR detection was utilized. Eight genotypes showed tolerance to all three viruses. These findings offer a superior resistance source for the efficient prevention and management of mosaic and leaf fleck diseases, and they could be used as a guide for commercial cultivars. Two viruses, SCMV and SCSMV, are the two major viruses that can cause mosaic disease, and SCSMV has become a major pathogen responsible for mosaic disease in the sugarcane-growing regions of India (Viswanathan and Rao, 2011). Furthermore, the study revealed that plants that appear healthy and show no visible symptoms (asymptomatic plants) are not necessarily free from mosaic and leaf fleck viruses. Despite not showing symptoms, these plants may still carry the viruses, which are in a latent stage. However, these plants serve as hidden sources of the virus and can contribute to the rapid spread of the disease under field conditions. Asymptomatic plants, along with potential vectors, can significantly worsen the incidence of vector-borne plant diseases. The presence of asymptomatic plants and vectors in the same agricultural environment can lead to increased disease transmission. Within just two growing seasons, new clones become infected with the viruses and start showing symptoms of mosaic and leaf fleck diseases with varying degrees of severity.
The resistance mechanism that plants have against viruses can be compromised by several factors. These include the presence of new virus strains, favorable environmental conditions that aid in virus multiplication and infection, the presence of virus-carrying vectors nearby, and more. Geijskes et al. (2004), Parameswari et al. (2013), and Bagyalakshmi et al. (2019) conducted detailed studies on mosaic and leaf fleck viruses, specifically on the whole genome of viruses SCMV, SCSMV, and SCBV, respectively. They found that negative selection pressure and recombination hotspots throughout the genome of both viruses may contribute to the viruses’ ability to successfully infect plants, develop diseases, and adapt to different environmental conditions. The negative selection pressure could help maintain genetic stability in the viral genome. The Hc-Pro (helper component proteinase) gene is known to govern RNA silencing suppressor (RSS) activity in members of the Potyviridae family, which includes mosaic viruses. However, recent research (Bagyalakshmi and Viswanathan, 2020) demonstrated that the P1 gene in SCSMV has RSS activity. This suggests that the P1 gene might play a significant role in suppressing the host’s resistance mechanism against the virus. In certain instances, SCSMV appears to dominate over SCMV and SCBV in sugarcane plants across different states in India. qRT-PCR assays show that SCSMV has a much higher virus titer than SCMV in infected plants (Viswanathan and Karuppaiah, 2010; Bagyalakshmi et al., 2019).
Among the three viruses, SCSMV was found to be the most dominant, with the distribution frequency being as follows: SCSMV > SCBV > SCMV. Infection from a combination of viruses has the potential to be more virulent and harmful than infection from a single virus. According to reports, a crop suffers more damage from a combined SCMV and SrMV infection than from a solo infection with one of these viruses (Xu et al., 2008). The combined infection may also function synergistically to speed up the progression of the disease in elite cultivars and cause sugarcane degeneration (Viswanathan, 2016).
The most practical and economical method for managing any viral disease in a crop that is propagated vegetatively, such as sugarcane, is host-plant resistance. Despite the fact that very few researchers have found the origins of mosaic and leaf fleck resistance to date, the majority of their investigations have only evaluated a small number of genotypes. Contrarily, via our efforts, we were able to identify eight tolerant genotypes with widespread resistance to mosaic and leaf fleck, including 2017A 553, 2017A 416, 2017A 517, 2016A 379, Co7717, Co S 767, Baragua, and Co 13034, which may be used as donors for breeding new varieties that are resistant to the mosaic and leaf fleck viruses. In the current investigation, we also found significant disease susceptibility in sugarcane genotypes and widespread disease occurrence in the majority of genotypes. The prevalence of SCSMV was found to be greater than that of SCMV and SCBV. All three viruses can co-infect, causing severe symptom manifestation and degeneration. The screened germplasms may offer prospective sources of resistance for the development of resistant cultivars to the sugarcane mosaic and leaf fleck diseases.
The datasets presented in this study can be found in online repositories. The names of the repository/repositories and accession number(s) can be found below: https://www.ncbi.nlm.nih.gov/genbank/, OR099818, OR099811, and OR060699.
GVa: Investigation, Writing - original draft. VMK: Supervision. PKV: Conceptualization, Methodology, Resources. BB: Visualization. GVi: Visualization.
The author(s) declare financial support was received for the research, authorship, and/or publication of this article. This study was supported by the institute grants of Acharya N. G. Ranga Agricultural University, and no separate funding has been received for the research work reported in the article.
The study has been undertaken as part of the Ph.D. research program at RARS, Anakapalli. The authors are thankful to the advisory committee for their support. Special thanks to Dr. Kishore Varma, principal scientist, for his guidance and support.
The authors declare that the research was conducted in the absence of any commercial or financial relationships that could be construed as a potential conflict of interest.
All claims expressed in this article are solely those of the authors and do not necessarily represent those of their affiliated organizations, or those of the publisher, the editors and the reviewers. Any product that may be evaluated in this article, or claim that may be made by its manufacturer, is not guaranteed or endorsed by the publisher.
Bagyalakshmi, K., and Viswanathan, R. (2020). Identification of the RNA silencing suppressor activity of Sugarcane streak mosaic virus P1 gene. Virus Dis. 31, 333–340. doi: 10.1007/s13337-020-00618-7
Bagyalakshmi, K., and Viswanathan, R. (2021). Development of a scoring system for sugarcane mosaic disease and genotyping of sugarcane germplasm for mosaic viruses. Sugar Tech. 23, 1105–1117.
Bagyalakshmi, K., Viswanathan, R., and Ravichandran, V. (2019). Impact of the viruses associated with mosaic and yellow leaf disease on varietal degeneration in sugarcane. Phytoparasitica 47, 591–604. doi: 10.1007/s12600-019-00747-w
Balamuralikrishnan, M., Doraisamy, S., Ganapathy, T., and Viswanathan, R. (2003). Sugarcane mosaic virus infection progress in relation to age of sugarcane. Sugar Tech. 5, 21–24.
Chomczynski, P., and Sacchi, N. (1987). Single step method of RNA isolation by acid guanidinium thiocyanate - phenol-chloroform extraction. Analyt. Biochem. 162, 156–159. doi: 10.1006/abio.1987.9999
Comstock, J. C., and Lockhart, B. E. L. (1996). Effect of sugarcane bacilliform virus on biomass production of three sugarcane cultivars. Sugar Cane 4, 12–15. doi: 10.5423/PPJ.OA.08.2018.0156
Costa, A. S., and Muller, G. W. (1982). “General evaluation of the impacts of virus diseases of economic crops on the development of Latin American Countries,” in Proceedings of the Conference on impact of viral diseases in developing Latin American and Caribbean Countries, Rio de Janeiro, 216–130. doi: 10.3390/plants10020233
Doyle, J. J., and Doyle, J. L. (1987). A rapid DNA isolation procedure for small quantities of fresh leaf tissue. Phytochem. Bull. 19, 11–15.
Edgar, R. C. (2004). MUSCLE: Multiple sequence alignment with high accuracy and high throughput. Nucleic Acids Res. 32, 1792–1797. doi: 10.1093/nar/gkh340
Geijskes, R. J., Braithwaite, K. S., Smith, G. R., Dale, J. L., and Harding, R. M. (2004). Sugarcane bacilliform virus encapsidates genome concatamers and does not appear to integrate into the Saccharum officinarum genome. Arch. Virol. 149, 791–798. doi: 10.1007/s00705-003-0260-0
Grisham, M. P. (2011). “Mosaic,” in A guide to sugarcane diseases, eds P. Rott, R. A. Bailey, J. C. Comstock, and B. J. Croft (Montepellier: CIRAD Publication Services), 249–254.
Guadie, D., Knierim, D., Winter, S., Tesfaye, K., and Abraham, A. (2019). Survey for the identification and geographicasl distribution of viruses and virus diseases of maize (Zea mays L.) in Ethiopia. Eur. J. Plant Pathol. 153, 429–439.
Hall, J. S., Adams, B., Parsons, T. J., French, R., Lane, L. C., and Jensen, S. G. (1998). Molecular cloning, sequencing and phylogenetic relationships of a new Potyvirus: Sugarcane streak mosaic virus, and a re-evaluation of the classification of the Potyviridae. Mol. Phylogenet. Evol. 10, 323–332. doi: 10.1006/mpev.1998.0535
Hassan, M., Ghulam, M. S., Waqas, W., and Imanat, Y. (2003). Aphid transmission of sugarcane mosaic virus (SCMV). Pak. J. Agricult. 40, 74–76.
Hema, M., Savithri, H. S., and Sreenivasulu, P. (2003). Comparison of direct binding polymerase chain reaction with recombinant coat protein antibody based dot-blot immunobinding assay and immunocapture-reverse transcription-polymerase chain reaction for the detection of Sugarcanestreak mosaic virus causing mosaic disease of sugarcane in India. Curr. Sci. 85, 1774–1777. doi: 10.1007/s00705-003-0015-y
Hema, M., Venkatramana, M., Savithri, H. S., and Sreenivasulu, P. (1999). Biological, antigenic and genomic relationships among the virus isolates causing mosaic disease of sugarcane in South India. Curr. Sci. 77, 698–702.
Koike, H., and Gillaspie, J. R. (1989). “Mosaic,” in Disease of sugarcane: Major disease, eds C. Ricaud, B. T. Egan, and A. G. Gillaspie (Amsterdam: Elsevier Science Publisher), 301–322.
Krishna, G. V., Kumar, M. K., Varma, P. K., Bhavani, B., and Kumar, G. V. (2023b). Prevalence and detection of viruses associated with mosaic disease of sugarcane in Andhra Pradesh. Biol. Forum Int. J. 15, 731–737.
Krishna, G. V., Kumar, V. M., Varma, P. K., Bhavani, B., and Kumar, G. V. (2023a). Detection of Sugarcane bacilliform virus in diseased sugarcane plants in Andhra Pradesh, India by serological and molecular methods. Braz. J. Microbiol. 54, 1399–1409. doi: 10.1007/s42770-023-01094-z
Kumar, R., Kaundal, P., Tiwari, R. K., Lal, M. K., Kumari, H., Kumar, R., et al. (2023). Development of reverse transcription recombinase polymerase amplification (RT-RPA): A methodology for quick diagnosis of potato leafroll viral disease in potato. Int. J. Mol. Sci. 24:2511. doi: 10.3390/ijms24032511
Kumar, S., Stecher, G., Li, M., Knyaz, C., and Tamura, K. (2018). MEGA X: Molecular evolutionary genetic analysis across computing platforms. Mol. Biol. Evol. 35, 1547–1549. doi: 10.1093/molbev/msy096
Li, W. F., Shan, H. L., Cang, X. Y., Lu, X., Zhang, R. Y., Wang, X. Y., et al. (2019). Identification and evaluation of resistance to Sugarcane streak mosaic virus (SCSMV) and Sorghum mosaic virus (SrMV) in excellent sugarcane innovation germplasms in China. Sugar Tech. 21, 481–485.
Li, W. F., Shan, H. L., Zhang, R. Y., Wang, X. Y., Yang, K., Luo, Z. M., et al. (2018). Identification of resistance to Sugarcane streak mosaic virus (SCSMV) and Sorghum mosaic virus (SrMV) in new elite sugarcane varieties/clones in China. Crop Prot. 110, 77–82.
Lockhart, B. E. L., and Autrey, L. J. C. (2000). “Sugarcane bacilliform virus,” in A guide to sugarcane diseases, eds P. Rott, R. A. Bailey, J. C. Comstock, B. J. Croft, and A. S. Saumtally (Montepellier: CIRAD and ISSCT), 268–272.
Lu, G., Wang, Z., Xu, F., Pan, Y. B., Grisham, M. P., and Xu, L. (2021). Sugarcane mosaic disease: Characteristics, identification and control. Microorganisms 9:1984. doi: 10.3390/microorganisms9091984
Matsuoka, S., Kennedy, A. J., Santos, E. G. D. D., Tomazela, A. L., and Rubio, L. C. S. (2014). Energy cane: Its concept, development, characteristics, and prospects. Adv. Bot. 2014:597275. doi: 10.1155/2014/597275
Parameswari, B., Bagyalakshmi, K., Viswanathan, R., and Chinnaraja, C. (2013). Molecular characterization of Indian Sugarcane streak mosaic virus isolate. Virus Genes 46, 186–189. doi: 10.1007/s11262-012-0827-5
Rao, G. P., Chatenet, M., Girard, J. G., and Rott, P. (2006). Distribution of Sugarcane mosaic and Sugarcane streak mosaic virus in India. Sugar Tech. 8, 79–81.
Silva, M. F., Gonçalves, M. C., Melloni, M. N. G., Perecin, D., Landell, M. G. A., Xavier, M. A., et al. (2015). Screening sugarcane wild accessions for resistance to sugarcane mosaic virus (SCMV). Sugar Tech. 17, 252–257.
Singh, D., Tewari, A. K., Rao, G. P., Karuppaiah, R., Viswanathan, R., Arya, M., et al. (2009). RT-PCR/PCR analysis detected mixed infection of DNA and RNA viruses infecting sugarcane crops in different states of India its phylogenetic relationships to closely related phytoplasmas. Sugar Tech. 11, 373–380.
Singh, S. P., Rao, G. P., Singh, J., and Singh, S. B. (1997). Effect of sugarcane mosaic potyvirus infection on metabolic activity, yield and juice quality. Sugar Cane 5, 19–23.
Singh, V., Sinha, O. K., and Kumar, R. (2003). Progressive decline in yield and quality of sugarcane due to Sugarcane mosaic virus. Indian Phytopathol. 56, 500–502.
Smith, G. R., Ford, R., Frenkel, M. J., Shukla, D. D., and Dale, J. L. (1992). Transient expression of the coat protein of sugarcane mosaic virus in sugarcane protoplasts and expression in Escherichia coli. Arch. Virol. 125, 15–23. doi: 10.1007/bf01309625
Użarowska, A., Dionisio, G., Sarholz, B., Piepho, H. P., Xu, M., Ingvardsen, C. R., et al. (2009). Validation of candidate genes putatively associated with resistance to SCMV and MDMV in maize (Zea mays L.) by expression profiling. BMC Plant Biol. 9:15. doi: 10.1186/1471-2229-9-15
Viswanathan, R. (2016). Varietal degeneration in sugarcane and its management in India. Sugar Tech. 18, 1–7.
Viswanathan, R., and Balamuralikrishnan, M. (2005). Impact of mosaic infection on growth and yield of sugarcane. Sugar Tech. 7, 61–65. doi: 10.1146/annurev-virology-092917-043413
Viswanathan, R., and Karuppaiah, R. (2010). Distribution pattern of RNA viruses causing mosaic symptoms and yellow leaf in Indian sugarcane varieties. Sugar Cane Int. 28, 202–205.
Viswanathan, R., and Rao, G. P. (2011). Disease scenario and management of major sugarcane diseases in India. Sugar Tech. 13, 336–353.
Viswanathan, R., Balamuralikrishnan, M., and Karuppaiah, R. (2007). Sugarcane mosaic in India: A cause of combined infection of sugarcane mosaic virus and sugarcane streak mosaic virus. Sugar Cane Int. 25, 6–14.
Viswanathan, R., Karuppaiah, R., and Balamuralikrishnan, M. (2010). Detection of three major RNA viruses infecting sugarcane by multiplex reverse transcription polymerase chain reaction multiplex- RT-PCR. Austr. Plant Pathol. 39, 79–84.
Viswanathan, R., Parameswari, B., and Nithya, K. (2018). Molecular characterization of sugarcane viruses and their diagnostics. Crop Improv Microb. Biotechnol. 2018, 175–193.
Keywords: mosaic, leaf fleck, resistant sources, SCMV, SCSMV, SCBV, RT-PCR/PCR
Citation: Vamsi Krishna G, Manoj Kumar V, Kishore Varma P, Bhavani B and Vijaya Kumar G (2023) Identification of resistance to Sugarcane mosaic virus, Sugarcane streak mosaic virus, and Sugarcane bacilliform virus in new elite sugarcane accessions in India. Front. Microbiol. 14:1276932. doi: 10.3389/fmicb.2023.1276932
Received: 13 August 2023; Accepted: 03 October 2023;
Published: 20 October 2023.
Edited by:
Ravinder Kumar, Central Potato Research Institute (ICAR), IndiaReviewed by:
Guangyuan Cheng, Fujian Agriculture and Forestry University, ChinaCopyright © 2023 Vamsi Krishna, Manoj Kumar, Kishore Varma, Bhavani and Vijaya Kumar. This is an open-access article distributed under the terms of the Creative Commons Attribution License (CC BY). The use, distribution or reproduction in other forums is permitted, provided the original author(s) and the copyright owner(s) are credited and that the original publication in this journal is cited, in accordance with accepted academic practice. No use, distribution or reproduction is permitted which does not comply with these terms.
*Correspondence: G. Vamsi Krishna, Z3ZrMTUxNEBnbWFpbC5jb20=
Disclaimer: All claims expressed in this article are solely those of the authors and do not necessarily represent those of their affiliated organizations, or those of the publisher, the editors and the reviewers. Any product that may be evaluated in this article or claim that may be made by its manufacturer is not guaranteed or endorsed by the publisher.
Research integrity at Frontiers
Learn more about the work of our research integrity team to safeguard the quality of each article we publish.