- 1College of Life Sciences, Fujian Normal University, Fuzhou, Fujian, China
- 2National Joint Engineering Research Center of Industrial Microbiology and Fermentation Technology, College of Life Sciences, Fujian Normal University, Fuzhou, Fujian, China
Introduction: The objective of this study is to examine the impact of various oyster shell soil conditioners, which are primarily composed of oyster shells, on the growth of tomatoes in acidic soil. Moreover, the aim of this investigation is to analyze the variety and structure of soil bacterial populations in close proximity to tomato roots while also contributing to the understanding of the physical, chemical, and biological mechanisms of oyster shell soil conditioners.
Methods: Tomato plants were grown in acidic red soil in three groups: a control group and a treatment group that used two types of oyster shell soil conditioners, OS (oyster shell powder) and OSF (oyster shell powder with organic microbial fertilizer). A range of soil physicochemical properties were measured to study differences in inter-soil physicochemical parameters and the growth of tomato plantings. In addition, this study utilized the CTAB (Cetyltrimethylammonium Bromide) technique to extract DNA from the soil in order to investigate the effects of oyster shell soil conditioner on the composition and diversity of bacterial populations. Utilizing high-throughput sequencing technologies and diversity index analysis, the composition and diversity of bacterial populations in the soil adjacent to plant roots were then evaluated. Ultimately, correlation analysis was used in this study to explore the relationship between environmental factors and the relative abundance of soil bacteria in the inter-root zone of tomato plants.
Results: The findings indicated that the oyster shell soil conditioners were capable of modifying the physicochemical characteristics of the soil. This was evidenced by significant increases in soil total nitrogen (16.2 and 59.9%), soil total carbon (25.8 and 27.7%), pH (56.9 and 55.8%), and electrical conductivity (377.5 and 311.7%) in the OS and OSF groups, respectively, compared to the control group (p < 0.05). Additionally, data pertaining to tomato seed germination and seedling growth biomass demonstrated that both oyster shell soil conditioners facilitated the germination of tomato seeds and the growth of seedlings in an acidic red clay soil (p < 0.05). On the other hand, the application of two oyster shell soil conditioners resulted in a modest reduction in the diversity of inter-root soil bacteria in tomato plants. Specifically, the group treated with OSF exhibited the most substantial fall in the diversity index, which was 13.6% lower compared to the control group. The investigation carried out on the soil between tomato plant roots yielded findings about the identification of the ten most abundant phyla. These phyla together represented 91.00-97.64% of the overall abundance. In the inter-root soil of tomatoes, a study identified four major phyla, namely Proteobacteria, Bacteroidetes, Acidobacteria, and Actinobacteria, which collectively accounted for up to 85% of the total abundance. At the general level, the relative abundance of Massilia increased by 2.18 and 7.93%, Brevundimonas by 5.43 and 3.01%, and Lysobacter by 3.12 and 7.49% in the OS and OSF groups, respectively, compared to the control group. However, the pathogenic bacteria unidentified_Burkholderiaceae decreased by 5.76 and 5.05%, respectively. The correlation analysis yielded conclusive evidence indicating that, which involved the use of CCA (Canonical Correlation Analysis) graphs and Spearman correlation coefficients, pH exhibited a positive correlation (p < 0.05) with Shewanella and a negative correlation (p < 0.05) with Bradyrhizobium. The relative abundance of Lysobacter and Massilia exhibited a positive correlation with the levels of total soil nitrogen.
Discussion: The utilization of oyster shell soil conditioner on acidic red soil resulted in several positive effects. Firstly, it raised the pH level of the inter-root soil of tomato plants, which is typically acidic. This pH adjustment facilitated the germination of tomato seeds and promoted the growth of seedlings. In addition, the application of oyster shell soil conditioner resulted in changes in the structure of the bacterial community in the inter-root soil, leading to an increase in the relative abundance of Proteobacteria and Bacteroidetes and a decrease in the relative abundance of Acidobacteria. Furthermore, this treatment fostered the proliferation of genera of beneficial bacteria like Massilia, Brevundimonas, and Lysobacter, ultimately enhancing the fertility of the red soil.
1 Introduction
Soils exhibiting a pH level lower than 6.5 are categorized as acidic (Huang, 2000). In typical scenarios, the process of soil acidification occurs gradually over a span of around 100 years (Krug and Frink, 1983). In recent years, there has been a significant increase in the expansion of facility-based agriculture (Shen, 1999). The process of reducing soil acidity has been expedited by the expansion, in conjunction with factors such as limited precipitation and specific environmental conditions prevalent in protected areas. These circumstances include high indoor air temperatures, high humidity, inadequate aeration, and heavy irrigation (Li and Zhang, 2001). Consequently, this has led to the emergence of various soil-related issues (Jiang et al., 2005; Wang et al., 2005; Deng and Zhang, 2006). Each plant species has evolved to thrive within a specific soil pH range, which is considered best for their growth throughout a significant duration of evolutionary history (Gilroy et al., 1989). Soil acidification causes soil surface consolidation, which prevents the soil root system from extending, resulting in a number of phenomena such as a decrease in the number of roots, slow growth, and reduced plant growth (Zeng, 2018), as well as a large loss of effective soil nutrients, resulting in Al3+ enrichment and toxic effects, severely affecting crop yield in the region (Xie, 2019). According to research, soil pH influences the relative number and variety of microorganisms (Zhalnina et al., 2015). The inter-root soil is home to a plethora of microorganisms, including bacteria, fungus, actinomycetes, and protozoa, with bacteria playing an important role in soil fertility, plant growth and development, and nutrient absorption by plant roots (Dotaniya and Meena, 2015; Zhang W. et al., 2019). Inter-rhizosphere bacteria can affect the efficacy of nitrogen, phosphorus, and trace metals, including iron, manganese, zinc, and copper, for plants and soil microbial communities, in addition to the geochemical cycling of these elements. By enhancing the nutritional content of plants, stimulating the production of growth hormones, or serving as a biological agent for disease control, it effectively enhances agricultural yields (Dotaniya and Meena, 2015).
Oysters serve as a crucial marine resource that is utilized by human populations (Southgate and Lucas, 2008; Zhou et al., 2014). Oysters are widely recognized as a highly valuable marine resource in terms of their suitability for human consumption. China is first in terms of oyster production among countries and areas engaged in oyster cultivation, with Korea, Japan, the United States, and France following suit (Zhang, 2014). Throughout an extended period of evolutionary time, each plant species has acquired a distinct and ideal soil pH for its growth. The oyster farming areas in China are all located along the coast, and the main production areas for Chinese oysters are in Fujian, Guangdong, and Shandong, with Fujian having the longest history of oyster farming and currently being the largest oyster farming area in China (Li et al., 2017; Lin et al., 2019). Currently, oysters are predominantly used for their edible component, while the shell, which accounts for 60% of their volume, is underutilized (Zhao et al., 2015). Recent research has demonstrated that oyster shells play a significant role in the improvement of acidic soils. Li (2019) observed that a homemade oyster shell soil conditioner could enhance the acidic soil of Guanxi honeydew, change the compacted soil into a crop-growing state, and raise soil nutrients from class IV to class III. Lee et al. (2008) applied oyster shell powder to the soil and planted cabbage seedlings to study the impact of oyster shell powder on enhancing the physicochemical qualities and crop yield in chalky loam (SiL, pH 6.2) and sandy loam (SL, pH 5.8) soils. They discovered that oyster shell significantly increased the soil pH to 6.9 and 7.4. Strictly established (Yan, 2019) by applying base fertilizer and oyster shell soil conditioner at 2250 and 1500 kg/hm2 to peanuts grown in yellow clay fields, flower production increased by 16.8 and 10.1%, respectively. The soil pH also increased by 0.8 and 0.5, and the soil’s organic, effective phosphorus, alkaline nitrogen, exchangeable calcium, and rapid-efficiency magnesium content were significantly increased. The oyster shell can boost soil organic, rapid phosphorus, and exchange ion concentrations, as well as significantly raise soil microbial carbon and nitrogen content, stimulate soil enzyme activity, and increase crop production (Lee et al., 2008).
In prior studies, our research team has successfully created two soil conditioner products derived from oyster shells. The first product, referred to as OS (Oyster shell powder), is produced by subjecting oyster shell powder to a thermal modification process at a temperature of 700°C and then sieving it through a 300-mesh screen. The second product, known as OSF (Oyster shell powder with organic microbial fertilizer), is a composite material consisting of OS combined with an organic microbial fertilizer.
This study aimed to examine the impact of oyster shell conditioner on tomato growth and the bacterial community in acidic inter-root soil. High-throughput sequencing was employed to analyze the red loam soil samples collected from Wenwu Xuefeng Farm in Minhou, Fujian Province, China. This study holds considerable importance in the comprehensive assessment of the impact of oyster shell conditioner on the bacterial community in acidic inter-root soil. This study provides theoretical support for the application of oyster shell conditioner as a means to improve acidic soil conditions, based on insights from microbiological viewpoints.
2 Research materials and methods
2.1 Overview of the study area
The soil used in this study was collected from Wenwu Xuefeng Farm, located in Minhou County, Fuzhou City, Fujian Province. The geographical coordinates of the farm are 118°51′ 119°25′ E and 25°47′ 26°37′ N. The climate in this region is characterized by a subtropical monsoon climate, with an annual precipitation of 1,673.9 mm and an average annual temperature ranging from 18 to 26°C. The topography exhibits a threshold characteristic, the climatic conditions are of a moderate nature, and the soil composition is notably characterized by a high level of acidity. The soil’s pH level is approximately 4.8. The experimental site is situated within the Qishan campus of Fujian Normal University in Fuzhou City, Fujian Province. Geographically, it is positioned at coordinates 26°03′ N and 119°20′ E. The region has a subtropical monsoon climate characterized by an average annual temperature of 19.6°C and an annual precipitation of 1674 mm.
2.2 Applicators and application methods
The experiment utilized 200 g of red loam soil obtained from Wenwu Xuefeng Farm as the foundational soil. The control group CK did not receive any treatment, while the experimental groups I and II were treated with oyster shell soil conditioner products OS and OSF, respectively. The laboratory independently prepared OS products by subjecting oyster shells to thermal modification at a temperature of 700°C. The resulting shells were subsequently crushed to obtain pure oyster shell powder, which passed through a 300-mesh sieve. The primary constituents of this powder were found to be CaCO3 (90−95%) and CaO (3−5%). OSF products are based on OS products, added to Fujian Hengxiang Fisheries Company’s commercialized organic microbial fertilizer products at 50:1 (w/w). The main components of organic microbial fertilizer are the mass fraction of total nutrients (N+P2O5+K2O) ≥ 4.0% and the number of effective live cells (cfu) ≥ 1 × 108/mL. Mix them thoroughly and place them in a 5 cm tall by 10 cm wide receptacle. Add deionized water to 60−70% of the soil’s normal water content and compact the soil. Then, apply oyster shell soil conditioner at a rate of 1.5 percent of the soil’s weight. Tomato seeds were submerged in water and grown for 24 h. The tomato seeds were immersed in water and cultivated for a duration of 24 h. The seeds were subsequently dispersed evenly across the surface of the soil. Each treatment consisted of 30 One Pine Red Israel Hard Fruited Tomato F1 (Green Bar Seedling Co., Guangzhou) seeds, with three replications being established. After sowing, a layer of dry soil measuring 0.5 cm was used to cover the seeds. Subsequently, a small amount of water was sprayed onto the soil using a watering can. Subsequently, the pots were placed within an incubator set at a temperature of 25°C, and a daily rehydration process was carried out. The experimental groups’ locations were randomly changed at various intervals during the course of the trial.
2.3 Measurement of tomato growth indicators
1. Determination of tomato germination potential, germination rate, and germination index The tomato germination cycle endured 8 days, and the number of seeds that germinated in each experimental group was measured daily, with day 5 being the day of germination potential measurement (Xu, 2019) and day 8 being the day of germination rate measurement; the germination potential, germination rate, and germination index were then calculated. Germination potential (%) = (Number of generates on a specified day/total number of seeds) × 100; Germination rate = (total number of germinations in the germination cycle/total number of seeds) × 100
In the equation, Gt is the number of germination days in the final period of the germination test, and Dt is the number of germination days.
2. determining the height, root length, plant fresh weight, root fresh weight, plant dry weight, and root dry weight of tomato vegetation: Five plants of uniform growth were taken from each treatment on the 30th day of incubation, and their plant height and root length were measured with a ruler; the fresh weight of the plants and the fresh weight of the roots were weighed on a one-in-ten-thousand balance; the plants were separated from the roots with scissors, sterilized at 105°C for 5 min, and then dried at 60°C to a constant weight before the dry weight of the plants and the dry weight of the roots.
2.4 Soil sample collection and processing
Upon the completion of the inquiry, namely on the 30 day, soil samples from the inter-root region were gathered from the OSF, OS, and CK experimental groups. The soil was carefully distributed in a controlled and sterile setting. The tomato seedlings were extracted using sterilized forceps, and the soil adhering to the tomato roots was delicately removed using a sterile soft-bristle brush, ensuring a distance of 1−2 mm from the root surface. Adequate amounts of soil located between the roots were collected into EP tubes. The dirt that remained was isolated from the roots and debris, transferred into a hermetically sealed container, and stored in a refrigeration unit at a temperature of roughly −20°C.
2.5 Soil physicochemical properties determination
In this phase of the investigation, the following experimental instruments were utilized: a portable pH meter (model PHS-3C), a portable conductivity meter (model 2265FS, manufactured in the United States), and a soil carbon and nitrogen analyzer (model Elementar Vario MAX CN, manufactured in Germany) for the determination of soil total nitrogen (TN) and total carbon (TC).
1. Soil water content (WC): a certain mass of fresh soil is weighed, desiccated to a constant weight at 105°C, and then the dry soil mass is weighed and calculated using the formula as follows:
In the equation, Mw is the mass of fresh soil (g); Mt is the mass of dried soil (g); and WC is the water content of the soil (%).
2. Soil pH and electrical conductivity (EC): A sample of soil was removed from the self-sealing bag, air-dried at room temperature, passed through a 2 mm sieve, mixed with deionized water at a ratio of 1:2.5, and stirred for 30 min. After 10 min, the soil pH and EC were measured directly using a portable pH meter (pHS-3C) and conductivity meter (2265FS, USA).
3. Determination of mineral nitrogen: 10.0 g of fresh soil samples were weighed and passed through a 2 mm sieve in a 50 mL centrifuge tube. Revise Su Tao’s literature (Su et al., 2005).
4. The carbon and nitrogen content of the soil was determined by adding a sample of air-dried soil that had been sieved through a 100 mesh sieve to a carbon and nitrogen analyzer. The analyzer was run following the provided instructions. Before determining the sample, it is necessary to make a subtraction of the blank value.
2.6 Analysis of rhizosphere soil bacterial community structure in tomato
2.6.1 Extraction of genomic DNA and PCR amplification
The soil DNA was extracted in accordance with the methodology described by Kamdem et al. (2023). The genomic DNA was isolated from soil samples using the CTAB method, followed by an assessment of its purity and concentration using agarose gel electrophoresis. The DNA extract was diluted to a concentration of 1 nanogram per microliter (ng/μL) and employed as a template for polymerase chain reaction (PCR) amplification. The PCR reaction utilized Phusion® High-Fidelity PCR Master Mix containing GC Buffer and high-performance, high-fidelity enzymes sourced from New England Biolabs. The study done by Zhang H. et al. (2019) examined the application of prokaryotic universal primers 341F and 806R. The primers and sequences utilized for PCR amplification were as described below:
2.6.2 PCR product purification and mixing
Libraries were constructed using Thermofisher’s Ion Plus Fragment Library Kit 48rxns library construction kit and then sequenced using Thermofisher’s Life Ion S5TM after completion of library construction, passing Qubit quantification, and library testing.
2.6.3 Library construction and high-throughput sequencing
This study analyzed the bacterial community composition of tomato inter-rhizosphere soil using high-throughput sequencing. The V3 ∼ V4 regions in the 16S rDNA of the inter-root soil genome of tomatoes grown under CK, OS, and OSF treatments were sequenced and analyzed. In the reference study (Liu et al., 2022), Venn diagrams, PCA plots, Speraman correlation analysis heat maps, and CCA plots were developed based on high-throughput sequencing results to analyze the effects of oyster shell soil amendments on the community structure and diversity of rhizobacteria in acidic tomato soil.
2.7 Data processing and analysis
Using Excel 2016 and SPSS 24 statistical analysis software, the data were analyzed and assembled. To investigate the diversity of species composition in the various treatment groups, all effective tags were clustered into OTUs (operational taxonomic units) with 97% identity, and then the representative sequences of OTUs were annotated with species. The representative sequences for each OTU were then annotated. Spearman correlation was applied to examine the association between bacterial abundance and environmental factors.
3 Results and analysis
3.1 Effect of oyster shell soil conditioner on tomato cultivation
3.1.1 Effect of oyster shell soil conditioner on tomato seed germination
The formulas in the experimental method were used to calculate the germination potential, germination rate, and germination index of tomato seeds. Different oyster shell soil conditioners had varying effects on the germination of tomato seeds, as shown in Table 1. The order of germination potential was OSF > OS > CK, with significant improvements of 24.99% in the OS group and 41.65% in the OSF group over the CK group; the order of germination rate was OSF = OS > CK, with significant improvements of 17.34% in both the OS and OSF groups over the CK group. The order of germination rate was OSF = OS > CK, and the germination rate of both OS and OSF groups was significantly increased by 17.34% relative to the CK group; the order of germination index was OS > OSF > CK, and it was significantly increased by 39.17% in the OS group and 36.86% in the OSF group compared to the CK group. In conclusion, the oyster shell soil conditioner supplemented with oyster shell powder was effective in promoting tomato seed germination in acidic red loam soil, and the OSF group was more effective in promoting germination.
3.1.2 Effect of oyster shell soil conditioner on the development of tomato seedlings
At the end of the 30th day of the experiment, plant height, root length, plant’s fresh weight, root’s fresh weight, plant’s dry weight, and root’s dry weight were measured for each treatment group; the results are shown in Table 2. The biomass data of the OS and OSF groups exhibited statistically significant improvements compared to the CK group, with a significant difference observed (p < 0.05). Moreover, there was no significant difference in biomass between the OSF and OS groups, suggesting that the primary constituent of calcium carbonate in both groups played a significant role in ameliorating soil acidity. Furthermore, the effectiveness of OS and OSF in improving soil conditions was found to be comparable.
3.2 Effect of oyster shell soil conditioner application on the physicochemical properties of tomato inter-root soil
Oyster shell powder is made by grinding the shells of the marine organism oyster, which is alkaline in nature. Oyster shell powder mostly consists of calcium carbonate, a compound known for its excellent adsorption capabilities and ability to raise the soil’s pH level. Moreover, the rise in pH level creates the ideal physicochemical environment for acid-tolerant species to reproduce and metabolize soil organic components, resulting in an augmented biomass of microorganisms and soil respiration (Neale et al., 1997; Lee et al., 2008). Oyster shells are rich in minerals, proteoglycans, and various organic matter, such as sodium, barium, copper, iron, magnesium, manganese, nickel, and several trace elements. Incorporating oyster shell soil conditioner into the soil increases its content, thereby augmenting the availability of ionic minerals to plants. This enhancement facilitates plant growth and effectively improves the soil’s electrical conductivity. Additionally, it has been shown that the application of oyster shell soil conditioner leads to an increase in soil organic matter, rapid phosphorus availability, and exchangeable cation concentrations. Moreover, it has been found to dramatically enhance the levels of soil microbial carbon and nitrogen content, stimulate soil enzyme activity, and ultimately result in higher crop yields.
As shown in Table 3, the different treatment groups had different amounts of total nitrogen (TN), total carbon (TC), pH, electrical conductivity (EC), and water content (WC) in the soil. In comparison to the control group, the implementation of OS and OSF resulted in a substantial increase in soil total nitrogen (p < 0.05), soil total carbon (p < 0.05), soil pH (p < 0.05), and soil electrical conductivity (p < 0.05). In the two treatment groups, the percentage increase in soil total nitrogen was 16.2 and 59.9%, soil total carbon was 25.8 and 27.7%, pH was 56.9 and 55.9%, and conductivity was 377.5 and 311.5%, while soil water content varied. Compared to the control group, the OS group reduced the amount of The OS group experienced a 10.1% decrease in soil water content compared to the control group (p < 0.05), while the OSF group experienced fluctuations compared to the control group that were not significant changes (p > 0.05).
3.3 Effect of oyster shell soil conditioner application on the bacterial diversity of tomato inter-rhizosphere soil
In the field of microbial diversity research, investigators utilize two distinct spatial scales for analysis, namely alpha and beta. Alpha diversity examines the abundance and diversity of microorganisms within a given community through the application of single-sample diversity analysis. The aforementioned investigations encompass a variety of statistically assessed indices that facilitate the assessment of species abundance and diversity within ecological groups. Beta diversity is a measure of the variation in species composition between communities that occupy different habitats along an environmental gradient.
3.3.1 The impact of oyster shell soil conditioner on the quantity and variety of bacteria in the inter-root soil of tomato plants
Table 4 displays the results of the alpha diversity analysis conducted on the bacterial diversity inside the soil next to the roots of tomato plants. The CK, OS, and OSF categories demonstrated a significant number of operational taxonomic units (OTUs), with counts of 1334, 1271, and 1153, respectively. The extent of library coverage for each of the three sample groups was found to be 99.5%. The extensive coverage seen in this study indicates a substantial likelihood of identifying gene sequences within the soil samples. This finding shows that the sequencing depth was adequate and that the resulting sequencing outcomes accurately represent the bacterial population present in the inter-rhizosphere soil of tomatoes.
Based on the findings presented in Table 4, it can be observed that the bacterial community’s diversity experienced a decrease in both the OS and OSF groups when compared to the control group. Specifically, the OS group exhibited a reduction of 4.7%, while the OSF group saw a more substantial decrease of 13.6%. Employing the Chao1 and ACE indices, the number of OUTs in the bacterial community and the diversity of the microbial community in the samples were estimated. Based on the Chao1 and ACE indices, the administration of either OS or OSF decreased the bacterial community’s abundance. OSF significantly reduced bacterial abundance compared to OS.
3.3.2 Effect of oyster shell soil conditioner application on the OTU distribution of inter-root soil bacteria in tomatoes
The utilization of Venn diagrams proves to be a valuable method for evaluating the similarities and distinctions in the composition of operational taxonomic units (OTUs) between the treatment group employing oyster shell soil conditioner and the control group. Additionally, it allows for the analysis of the number of OTUs that are unique to individual samples and those that are shared among numerous samples. The root soil bacteria operational taxonomic unit (OTU) distribution chart for various treatment groups (Figure 1) demonstrates that the CK, OS, and OSF groups harbored 250, 216, and 91 distinct OTUs, respectively. Additionally, it is noteworthy that 1228 OTUs were found to be common among all three groups. The utilization of OS or OSF resulted in the introduction of new species and modifications in the community structure of bacteria residing in the inter-rhizosphere soil of tomato plants, with a more pronounced effect observed in the OS group.
3.3.3 Effect of oyster shell soil conditioner application on bacterial community composition of tomato inter-root soil
3.3.3.1 Soil bacterial colonies in the inter-root zone of tomato: composition analysis
Figure 2 presents the makeup of soil bacterial communities at the phylum level, specifically focusing on the inter-root region. This figure illustrates the variations in population composition across different treatment settings. The relative abundance of the top 10 phyla in soil samples from the CK, OS, and OSF groups varied between 91.00 and 97.64%. The relative abundances of these 10 phyla were: Proteobacteria 55.32 ∼ 67.97%, Bacteroidetes 4.42∼9.22%, Acidobacteria 2.87 ∼ 12.18%, Chloroflexi 1.45 ∼ 5.12%, Actinobacteria 8.92 ∼ 9.46%, Firmicutes 1.98 ∼ 4.03%, unidentified Bacteria 1.27 ∼ 2.29%, Verrucomicrobia 0.67 ∼ 1.35%, and Tenericutes 0.45∼0.70%, and Gemmatimonadetes 0.39 ∼ 0.76%. Proteobacteria, Bacteroidetes, Acidobacteria, and Actinobacteria constituted more than 85% of the total number of samples and were the primary phylum in the three sample categories.
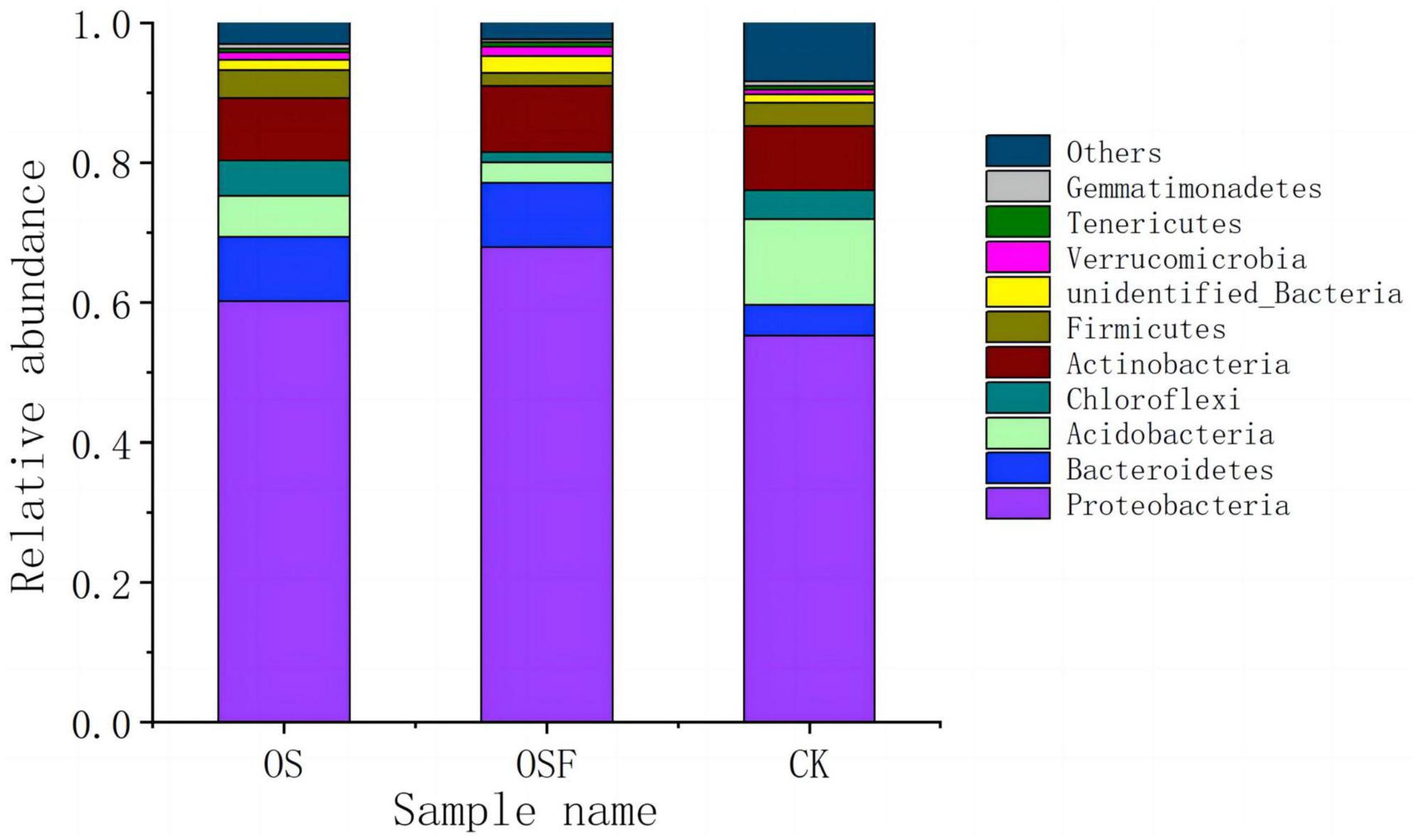
Figure 2. Community composition of bacterial phylum in rhizosphere soil of different treatment groups.
Figure 3 illustrates the horizontal community composition of soil bacterial species across different treatment settings in the root soil. The relative abundance of the top 30 genera in each of the three categories ranged from 45.88 to 61.44% of the total genera. They are: Massilia, Brevundimonas, Lysobacter, unidentified Burkholderiaceae, Sphingomonas, Flavisolibacter, Bradyrhizobium, Bacteroides, Citrobacter, unidentified_Bacteria, unidentified_Lachnospiraceae, Xenophilus, Candidatus_Solibacter, Candidatus_Bacilloplasma, phenylobacterium, Acidothermus, Catellatospora, Methylobacterium, Shewanella, Bosea, Bryobacter, Ramlibacter, Stenotrophomonas, Mucilaginibacter, Filimonas, Opitutus, Cupriavidus, unidentified_Erysipelotrichaceae, Chthoniobacter, Sinomonas. Among them, Massilia, Brevundimonas, Lysobacter, unidentified_Burkholderiaceae, Sphingomonas, Flavisolibacter, and Bradyrhizobium were relatively abundant and were the dominant bacterial genera in tomato inter-rhizosphere soil.
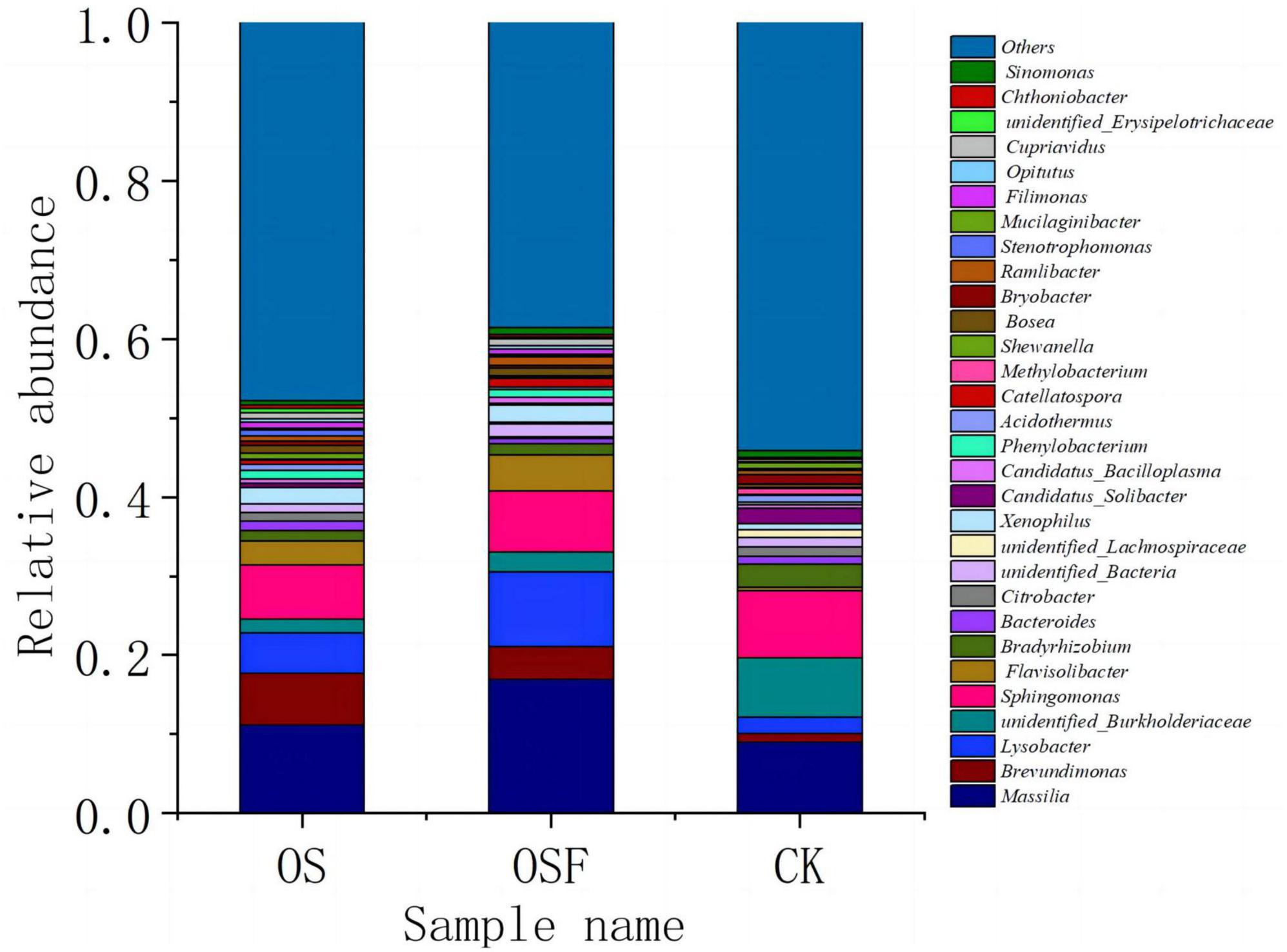
Figure 3. Community composition of bacterial genus in rhizosphere soil of different treatment groups.
3.3.3.2 Effect of application of oyster shell soil amendment on the structure of bacterial genera in the inter-root soil of tomato
At the phylum level, Proteobacteria, Bacteroidetes, and Acidobacteria exhibited greater variability in the OS and OSF groups compared to the control group. Specifically, the relative abundance of Proteobacteria and Bacteroidetes increased, while the relative abundances of Acidobacteria and other phyla decreased. In the OS and OSF groups, the relative abundance of Proteobacteria increased from 55.3 to 60.2 and 68.0%, respectively. Moreover, the relative abundance of Bacteroidetes increased from 4.4 to 9.2%. Conversely, the relative abundance of Acidobacteria decreased from 12.2 to 5.8 and 2.9%, respectively. Additionally, the relative abundance of other phyla decreased from 8.3 to 9.2% in the OS and OSF groups.
At the genus level, the utilization of different oyster shell soil conditioners (OS and OSF) led to a significant alteration in the relative abundance of several bacterial genera compared to the control group. Specifically, there was a marked increase in the relative abundances of the genera Massilia, Brevundimonas, and Lysobacter, while a significant decrease was observed in the relative abundance of unidentified_Burkholderiaceae. The specific alterations were as follows: in the OS and OSF groups, the relative abundance of Massilia increased from 9.00 to 11.18 and 16.93%, respectively; the relative abundance of Brevundimonas increased from 1.13 to 6.56 and 4.14%, respectively; the relative abundance of Lysobacter increased from 2.00 to 5.12 and 9.49%; whereas the relative abundance of unidentified_Burkholderiacea decreased from 7.50 to 1.74 and 2.49%, respectively.
In order to examine the variations in the predominant genera of soil bacteria in the inter-rhizosphere of tomatoes across different treatment groups, we selected the top 35 genera with significant relative abundance. This selection was based on the species annotation and abundance data of all samples at the genus level. The chosen genera were then clustered according to their abundance information and visualized as a heat map (Figure 4). This approach aimed to facilitate the identification of the extent of aggregation among different genera within the distinct treatment groups.
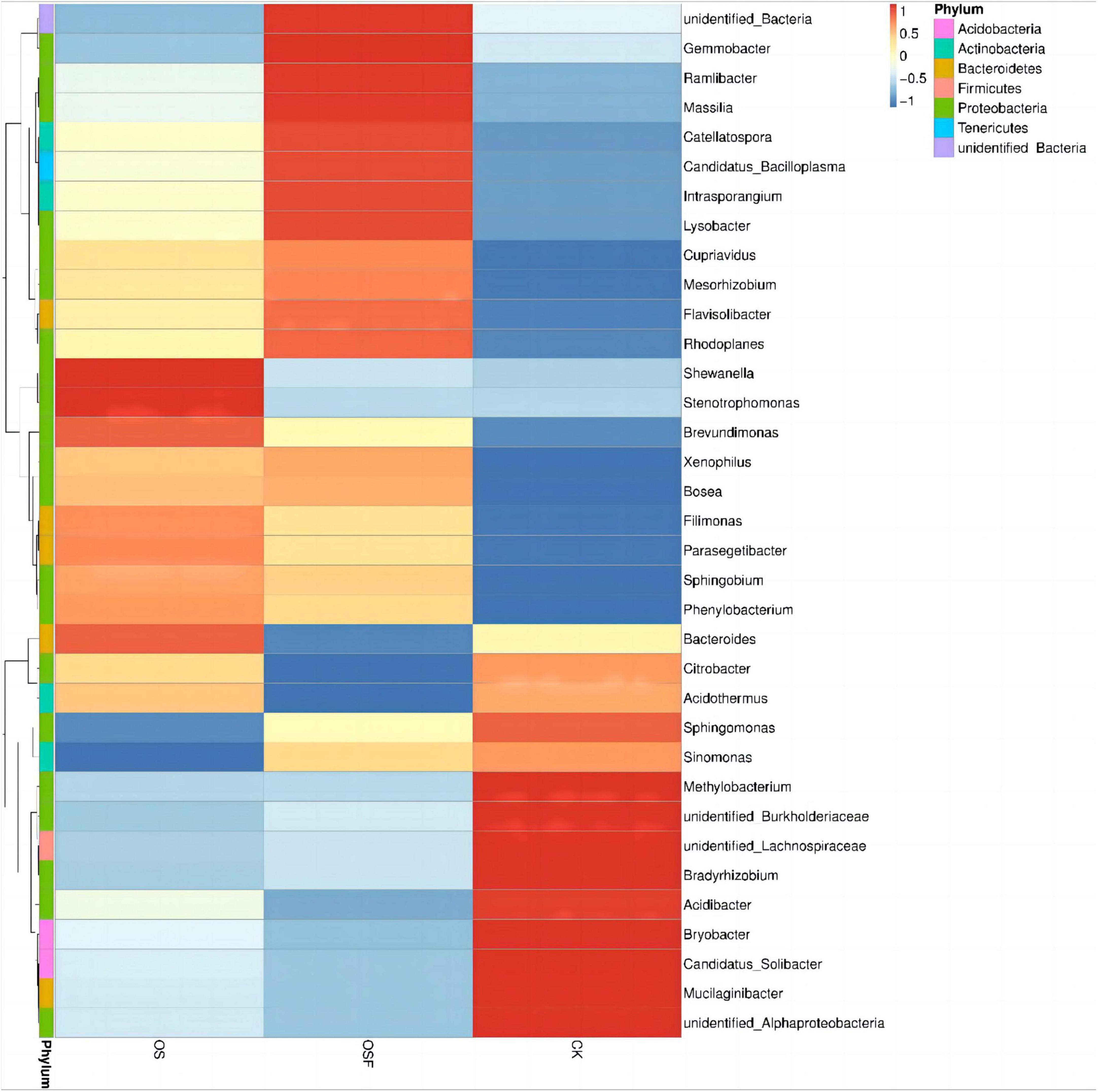
Figure 4. Cluster heat map of bacterial abundance in rhizosphere soil of different treatment groups.
As shown in the heat map (Figure 4), there are differences at the genus level among the three dominant groups, CK, OS, and OSF. Mucilaginibacter, Candidatus_Solibacter, Bryobacter, Acidibacter, Bradyrhizobium, and Methylobacterium are the dominant genera in the CK group; Shewanella and Stenotrophomonas are the dominant genera in the OS group; and Gemmobacter, Ramlibacter, Massilia, Catellatospora, Candidatus_Solibacter, Intrasporangium, and Lysobacter are the dominant genera in the OSF group. It can be observed that the relative abundance of various bacterial genera in distinct treatment groups varied slightly, as did their dominant genera.
The findings above suggest that the implementation of oyster shell soil conditioners, namely OS and OSF, had a considerable impact on the structure of the rhizosphere microbiome. This resulted in a modification of the microbial niche situated between plant roots, which varied in intensity and scope, thus indicating that the effects of OS and OSF on soil composition were distinguishable and independent of one another.
3.3.4 Principal component effects of the application of oyster shell soil conditioner to the inter-root soil bacterial communities of tomato
In order to analyze the differences caused by oyster shell soil amendments on the soil bacterial community structure in the tomato rhizosphere, we performed principal component analysis (PCA) on the soil bacterial community composition of the different treatment groups using PCA analysis in beta diversity analysis; the results of the PCA analysis are shown in the PCA analysis graph (Figure 5).
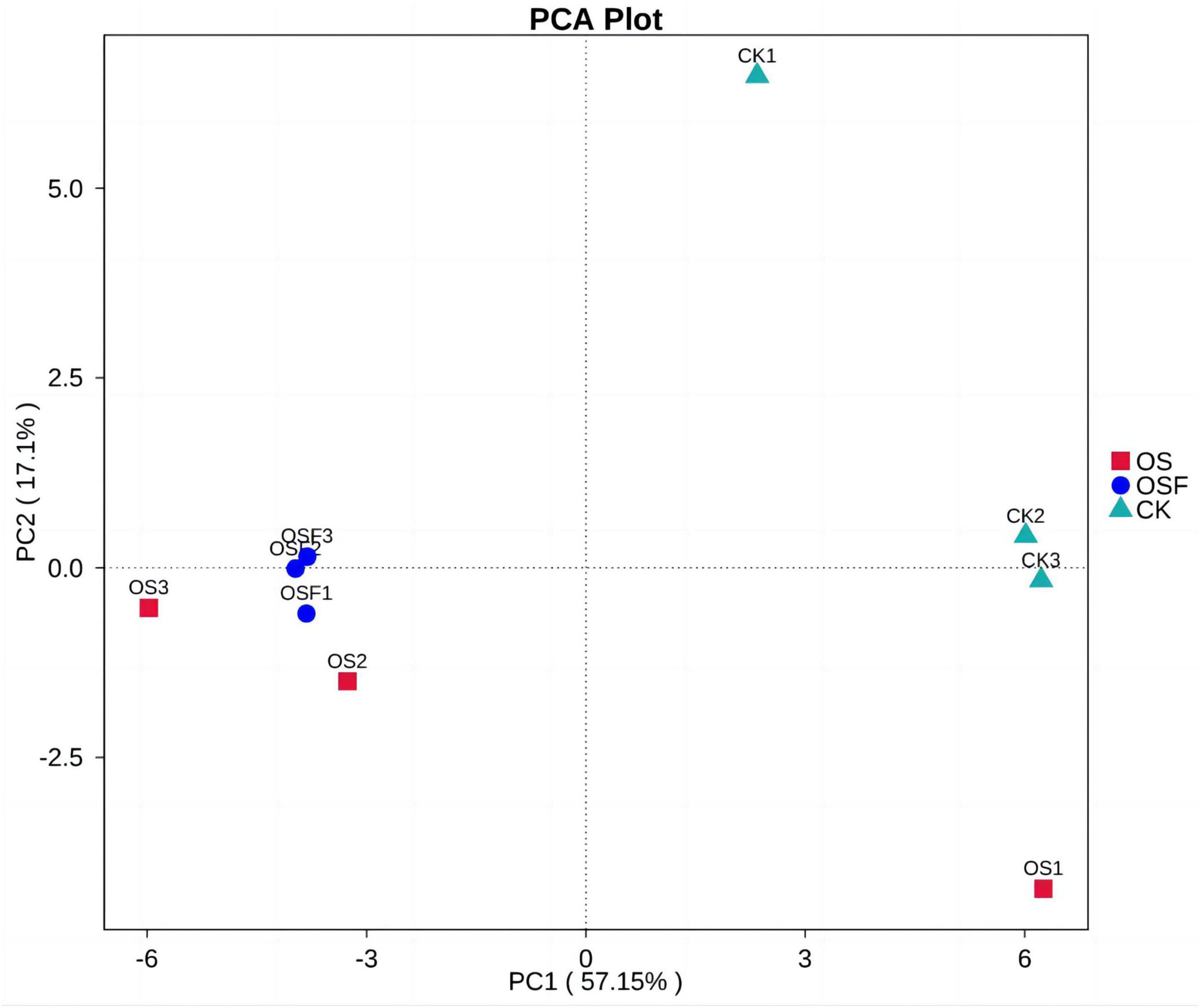
Figure 5. Principal component analysis (PCA) analysis of bacterial community composition in rhizosphere soil of different treatment groups.
The first two principal components (PC1 and PC2) were selected for the analysis of the three groups of tomato inter-root soils. The first principal component made up 57.15% of the difference between samples, and the second principal component made up 17.1% of the difference between samples. This means that these two principal components were the main reasons why the bacterial community structure of tomato inter-root soils was different. The graph depicts the communities of three parallel samples from each treatment group, with the dots representing different colors to indicate the variation in bacterial community structure. Notably, the degree of separation between the dots corresponds to the level of significance of the observed changes. The observed spatial separation between the OS and OSF groups, as measured along the principal component 1 axis (PC1), was found to be larger than that observed for the CK group. Similarly, the spatial separation between the OS and OSF groups along the principal component 2 axis (PC2) was also larger than that observed for the CK group. These findings suggest that both the OS and OSF groups have significantly influenced the composition and structure of bacterial communities.
3.4 Analysis of the correlation between inter-root soil bacterial species diversity and environmental factors in various treatment groups
3.4.1 Spearman correlation analysis
As depicted in the Spearman correlation analysis thermogram (Figure 6), the water content (WC) was positively correlated with unidentified_Alphaproteobacteria, Sinomonas, and Bradyrhizobium (p < 0.05) and negatively correlated with Filimonas (p < 0.05). pH had a significant positive correlation with Shewanella (p < 0.05); a negative correlation with Bradyrhizobium, unidentified_Alphaproteobacteria, and unidentified_Burkholderiaceae (p < 0.05); and a highly significant negative correlation with Methylobacterium (p < 0.01). Electroconductivity (EC) is highly positively correlated with Filimonas, Stenotrophomonas, etc. (p < 0.01), positively correlated with Bosea, etc. (p < 0.05), highly negatively correlated with Bryobacter, etc. (p < 0.01), and negatively correlated with Methylobacterium, etc. (p < 0.05). And for TC and TN, there are also some bacterial properties that are positively (or negatively) related in a very significant way. The aforementioned results indicate that total carbon (TC), total soil nitrogen (TN), electrical impact on thermal conductivity, soil pH, and water content have a great abundance of interracial soil bacteria, with varying effects on distinct bacteria.
3.4.2 CCA analysis
The distance between the points of the CK, OS, and OSF groups was farther in the CCA (Figure 7), indicating that the differences in bacterial community composition among the three groups were obvious. The angles of the control group and WC were acute and obtuse with pH, EC, TN, and TC, indicating that the control group was positively correlated with WC and negatively correlated with pH, EC, TN, and TC; the distribution of the OS group was more dispersed and all were obtuse with WC, indicating that the OS group was negatively correlated with WC, while the angles of the OS group were different with pH, EC, TN, and TC, with large differences; the OSF group was at an obtuse angle to WC and at an acute angle with pH, EC, TN, and TC, indicating that the OSF group was negatively correlated with WC and positively correlated with pH, EC, TN, and TC. Therefore, variations in the structural composition of the inter-rhizosphere soil bacterial community may be related to changes in soil physicochemical factors.
4 Discussion
4.1 Effect of oyster shell soil conditioner application on tomato
In the tomato cultivation experiment, seed germination and seedling growth were enhanced in the OS and OSF groups relative to the CK group. It is possible that the Ca2+ contained in the OS and OSF groups improved the acidic soil environment and stimulated the growth of tomato seedlings. Based on empirical findings, it has been observed that when a tomato plant experiences stress due to adverse conditions, there is a notable elevation in the concentration of Ca2+. This surge in Ca2+ concentration prompts the activation of calcium channels inside the plant, thereby exerting regulatory control over crucial physiological processes and augmenting the plant’s resilience to adverse circumstances (Zhang, 2019). This study examines the impact of utilizing oyster shell soil conditioner on the growth of tomato seedlings in acidic soil. It is hypothesized that one potential mechanism behind this observed growth promotion is the facilitation of Ca2+ channel initiation in tomato plants. However, Ca2+ is an important structural and functional part of plant chloroplasts and is involved in photosynthesis. Adding calcium from outside the plant increases its ability to synthesize food when it is exposed to abiotic stress (Zhang et al., 2014). Therefore, in acidic soils, the application of oyster shell soil conditioner promoted photosynthesis in tomatoes, which in turn promoted the growth of tomatoes. In addition, Ca2+ in soil can interact with P5+ and K+ ions to significantly improve the biological condition and tomato fruit quality during the growth of tomatoes (Xu, 2003). The synergistic effect of Ca2+ and other inorganic salt ions on tomato growth may be another reason why oyster shell soil conditioner promotes tomato growth. Furthermore, a variety of physiological ailments, including navel rot, fruit breaking, and poor coloring, may manifest during the growth of tomatoes in the absence of sufficient calcium (Li et al., 2019; Liu et al., 2019). However, the application of oyster shell soil conditioner can effectively ensure optimal growth conditions, facilitate successful blooming and fruiting, and enhance the overall quality of tomatoes. OS is solely calcium carbonate and contains a small amount of calcium oxide, which is too alkaline and may cause seedling burn if used improperly. OSF, on the other hand, is more suitable for very acidic soils, and the dosage must be calculated and rigorously controlled. When considering all factors, it can be concluded that the oyster shell soil conditioner developed by OSF demonstrated the highest level of effectiveness.
4.2 Effect of oyster shell soil conditioner application on bacterial diversity in acidic inter-root soil of tomato
The diversity of soil microorganisms has become an essential factor in maintaining soil function (Xu et al., 2010; Tan et al., 2014) and an important index for managing and evaluating soil quality (Fernandez et al., 2016; Li, 2018). According to studies, oyster shell powder can modify bacterial abundance and diversity, leading to a rise in bacterial abundance and diversity (Zhang, 2018). In this study, however, OS and OSF altered the relative abundance, diversity, and community structure of tomato soil bacteria, resulting in a slight reduction in bacterial community diversity and a different effect for each. It is hypothesized that this difference is due to the fact that the structure of the tomato inter-rooted bacterial community has not yet reached a stable state during the 30-day cultivation period, and studies have shown that this structure stabilized gradually between 60 and 70 days (Cheng, 2018). It is also possible that OS and OSF altered the soil pH too drastically for too short a period of time, resulting in the mass extinction of the original acid-tolerant bacterial population in the inter-rooted soil, whereas the bacterial community that adapted to the new environment did not recover within a short period of time. Additionally, bacterial diversity and relative abundance were greater in the OSF group than in the OS group, most likely due to the presence of organic microbial fertilizer in the OSF group, which is consistent with previous research (Xiang et al., 2021).
4.3 Effect of oyster shell soil conditioner application on bacterial community structure of tomato acidic inter-root soil
The introduction of oyster shell soil conditioner into acidic soils has the potential to modify the structure of the soil microbial community and facilitate its evolutionary processes (Ji, 2020). Cheng (2018) employed high-throughput sequencing techniques to identify the predominant microbial communities present in the inter-rhizosphere soils of tomatoes. The results revealed that Acidobacteria, Bacteroidetes, Gemmatimonadetes, and Proteobacteria were the primary microbial taxa observed. Proteobacteria constituted the predominant species in the inter-rhizosphere soil of tomatoes, comprising approximately 34.15% of the bacterial population in this particular soil environment. Previous research has demonstrated the substantial contribution of Proteobacteria to soil remediation and the enhancement of nitrogen fertilizer utilization (Zhang et al., 2012; Xu et al., 2017). The phylum identified in this study included the above phylum after the application of oyster shell soil conditioner powder, and the relative abundance of Proteobacteria was the highest, consistent with previous discoveries. In addition, the relative abundance of Bacteroidetes increased significantly in the OS and OSF groups compared to the CK group, indicating that the relative abundance of Bacteroidetes was greater in healthy tomatoes than in diseased tomatoes (Shen et al., 2021), indicating that the application of oyster shell soil conditioner increased the number of dominant Bacteroidetes in tomato inter-root soil and promoted tomato growth. The impact of OSF was marginally more significant compared to OS, presumably due to the inclusion of organic microbial fertilizer in OSF. This component has the ability to assimilate a diverse range of advantageous bacteria, nutrients, and trace elements that facilitate the development of tomatoes. Consequently, this fosters the proliferation and reproductive capabilities of rhizosphere bacteria (Rousk et al., 2010; Wu et al., 2019).
At the taxonomic level of genus, the present study observed that the application of oyster shell soil conditioners OS and OSF resulted in an increase in the relative abundance of Massilia from 9 to 11.18 and 16.93%, respectively. Similarly, the relative abundance of Brevundimonas grew from 1.13 to 6.56 and 4.14% with the application of OS and OSF, respectively. Additionally, the relative abundance of Lysobacter increased from 2 to 5.12 and 9.49% with the application of OS and OSF, respectively.
The principal constituent of oyster shell powder, according to our previous analysis, is calcium carbonate, which alters the soil’s pH when applied to the soil. According to previous studies, changes in soil pH have a cascading effect, i.e., fluctuations in soil pH may trigger changes in a range of soil nutrients, including, but not limited to, microbial-dominated soil nitrogen turnover, soil phosphorus solubility, soil fast-acting potassium content, and soil organic matter. In addition, the pH fluctuations also affect the microbial indicators in the soil (Wang et al., 2013; Reza et al., 2016; Penn and Camberato, 2019; Wu et al., 2023). Therefore, we suppose that the change in soil pH after the application of oyster shell soil conditioner was the main reason for the change in the occupancy structure of bacterial genera in the soil.
At the phylum level, the community structure of tomato inter-rhizosphere soil didn’t change much. However, the genus distribution heat map of tomato inter-rhizosphere soil showed that the genus varied a lot between the applied treatments, and each sample treatment had its own dominant genus. Although the principal components of OS and OSF are identical, the principal dominant genera of the two are distinctive. It’s possible that OSF absorbs related probiotics that have antagonistic or synergistic effects on inter-rhizosphere bacterial populations. It’s also possible that other nutrients or trace elements that OSF absorbs have some effect on inter-rhizosphere bacterial growth that helps it grow. Finally, OSF may cause tomato roots to make more secretions that affect inter-rhizosphere bacterial growth (Zhalnina et al., 2015). When oyster shell soil conditioner was put on tomato inter-rhizosphere soil, it changed the soil’s physical and chemical properties and acted on microorganisms. This caused changes in the species and relative abundance of dominant genera in the soil, which in turn changed the structure and distribution of soil bacterial communities.
4.4 Correlation between the application of oyster shell soil conditioner on microorganisms and environmental factors in acidic tomato inter-root soil
The soil’s microbial activity, abundance, and community structure interact with plant growth and a variety of parameters like carbon and nitrogen content, pH, and electrical conductivity. The number, species, and composition of inter-rooted microorganisms can vary according to environmental factors (Andrew et al., 2012). The principal soil parameter that determines the diversity and biomass of soil microorganisms is pH, which influences the microbial community by modulating the efficacy of soil nutrients (Zhalnina et al., 2015). In this experiment, pH in the experimental group was positively correlated with Shewanella (p < 0.05) and negatively correlated with Bradyrhizobium, unidentified_Alphaproteobacteria, and unidentified_Burkholderiaceae (p < 0.01), showing a highly significant negative correlation with Methylobacterium (p < 0.01). All of the aforementioned genera belong to the Proteobacteria, and it is assumed that the bacteria of the Proteobacteria may be closely related to the regulation of soil pH. This is consistent with previous studies (Cheng, 2018).
4.5 Efficacy of oyster shell soil conditioner application for plant disease control
Previous studies (Ofek et al., 2012; Naqqash et al., 2020; Lin et al., 2021) have demonstrated that Massilia, Lysobacter, and Brevundimonas exhibit beneficial effects on plants, including the induction of resistance mechanisms and promotion of growth. These bacterial species can be classified as helpful bacteria. The Burkholderiaceae, conversely, represent a clearly delineated genus of pathogenic bacteria. Within this genus, many phytopathogenic bacteria are known to induce distinct manifestations of plant diseases, such as rot, wilt, and extensive necrosis.
In this study, the relative abundance of Massilia, Brevundimonas, and Lysobacter increased significantly after the application of oyster shell soil conditioner, while the relative abundance of unidentified_Burkholderiaceae decreased most significantly. As mentioned in the previous paper (Carrión et al., 2018; Gu et al., 2019), the addition of oyster shell soil conditioner to acidic soil will change the community structure of soil microorganisms, and the change in community structure is also important for the control of plant disease. According to previous studies, Massilia plays an important role in soil remediation and amelioration. Feng et al. (2016) found a new Massilia that can produce dimethyl disulfide (DMDS), which can be used as an alternative fumigant to control the high infection levels of soilborne pathogens, nematodes, and weeds. DMDS can also degrade PAH phenanthrene and chloroacetamide-based herbicides, produce heavy metal resistance, and have phosphorus solubilization (Islam et al., 2019; Yang et al., 2019) showed that isolates of Brevundimonas can promote the growth of tomato plants, act as an effective biocontrol agent against tomato wilt, and play an important role in soil toxicity removal (Gu et al., 2019). Lysobacter is not only able to colonize the inter-root zone of many plants but also secretes a variety of antibiotics, extracellular hydrolytic enzymes, and biosurfactants to inhibit the growth of pathogens, thus controlling plant diseases (Ji, 2011), and Lysobacter strains are able to produce a type of antibiotic that has a pronounced antagonistic effect on seedling blight (Hashidoko et al., 1999; Yu et al., 2007). Kobayashi and Yuen (2005) studied enzyme-producing lysogenic bacilli and found a new antibiotic substance with heat stability that altered the morphology of the mycelium by regulating the synthesis pathway of ceramidase to control the disease. In this study, unidentified_Burkholderiaceae showed the most significant decrease in relative abundance. Burkholderia is an important group of plant pathogenic bacteria, including eight different species of phytopathogenic bacteria, and varies according to the specific host plant as well as to a particular occasion and cultivar (Zhang, 2016). Individual species have also been found to be closely related to plant roots (Urakami et al., 1994). After the introduction of oyster shell soil conditioner, we observed significant changes in the relative abundance of the four plant genera mentioned above. Based on examples from previous studies (Shen et al., 2018), microbial abundance and diversity were highly significant and negatively correlated with the incidence of siderophore diseases. Consequently, we deduce that the application of oyster shell soil conditioner led to a steady increase in the presence of beneficial genera, resulting in the suppression of detrimental genera. This was the underlying cause for the observed drop in the overall relative abundance. These data suggest that using oyster shell soil conditioner can improve the ability of tomato plants to fight diseases.
4.6 Main data analysis methods used in the article
The present study utilized various statistical techniques, such as the Shannon index, Spearman correlation, CCA chart, and PAC chart, to examine and interpret the experimental data. The Shannon index was utilized to estimate the diversity of the microbial population in the samples, where higher values corresponded to increased levels of diversity. In the figure depicting the Principal Component Analysis (PCA) analysis (Figure 5), The distance observed on the principal component axis 1 (PC1) between the OS group and the OSF group was significantly greater than that of the CK group. Similarly, the spatial distance between the two groups on the principal component axis 2 (PC2) was also significantly greater than that of the CK group. These findings suggest that both the OS group and the OSF group have altered the composition and structure of the bacterial community. However, the spatial distribution difference between the OS group and the OSF group on the two axes was relatively small, indicating a limited dissimilarity in community composition between these two groups. The disparity in structure is minimal. The observed phenomenon can be attributed to the predominant constituents of OS and OSF, namely calcium carbonate. These constituents primarily influence the structure and diversity of the bacterial population by modulating the pH levels of the soil. Spearman’s correlation analysis was used to figure out how environmental factors, microbial species, and the number of them affected each other. The p-values typically showed the correlation and significance between the two. CCA plots can be used to detect relationships between environmental factors, between samples and colonies, or between two, and to identify the most influential environmental factors on the distribution of samples.
5 Conclusion
The utilization of oyster shell soil conditioner facilitated the germination and development of tomato seedlings, with oyster shell flour (OSF) demonstrating greater overall efficacy compared to oyster shell (OS).
The Shannon index showed that applying OS and OSF to soil decreased the variety of microbes compared to the control group. The OSF group had the biggest drop, at 13.6%.
Between 91.00 and 97.64% of the dirt around tomato plants belonged to the ten most common phyla. A total of 85% of the main groups in the inter-rhizosphere soil of tomatoes were Proteobacteria, Bacteroidetes, Acidobacteria, and Actinobacteria.
When oyster shell soil conditioner was added, the relative abundance of three helpful genera—Massilia, Brevundimonas, and Lysobacter—increased to varying degrees. On the other hand, the relative abundance of the disease-causing Burkholderiaceae dropped by a large amount.
Environmental factors were correlated with the relative abundance of soil bacteria in the inter-root zone of tomatoes. pH was positively correlated with Shewanella (p < 0.05), negatively correlated with Bradyrhizobium, unidentified Alphaproteobacteria, and unidentified_Burkholderiaceae (p < 0.05), and highly significantly negatively correlated with Methylobacterium (p < 0.01).
Data availability statement
The datasets presented in this study can be found in online repositories. The names of the repository/repositories and accession number(s) can be found in the article/supplementary material.
Author contributions
YZ: Writing – original draft, Writing – review & editing. CY: Writing – review & editing, Writing – original draft. YX: Writing – review & editing. TY: Writing – review & editing. SW: Writing – review & editing.
Funding
The author(s) declare financial support was received for the research, authorship, and/or publication of this article. This work was supported by grants from the National Natural Science Foundation of China (Nos. 31670125) and the Fujian Province regional development project (Nos. 2017N3015).
Conflict of interest
The authors declare that the research was conducted in the absence of any commercial or financial relationships that could be construed as a potential conflict of interest.
Publisher’s note
All claims expressed in this article are solely those of the authors and do not necessarily represent those of their affiliated organizations, or those of the publisher, the editors and the reviewers. Any product that may be evaluated in this article, or claim that may be made by its manufacturer, is not guaranteed or endorsed by the publisher.
References
Andrew, D. R., Fitak, R. R., Munguia-Vega, A., Racolta, A., Martinson, V. G., and Dontsova, K. (2012). Abiotic factors shape microbial diversity in Sonoran Desert soils. Appl. Environ. Microbiol. 78, 7527–7537. doi: 10.1128/aem.01459-12
Carrión, V. J., Cordovez, V., Tyc, O., Etalo, D. W., De Bruijn, I., De Jager, V. C., et al. (2018). Involvement of Burkholderiaceae and sulfurous volatiles in disease-suppressive soils. ISME J. 12, 2307–2321. doi: 10.1038/s41396-018-0186-x
Cheng, Z. Q. (2018). Composition structural, diversity, succession and function of the tomato Rhizosphere and Endophytes. Master’s thesis. Fuzhou: Fujian Normal University.
Deng, Y. L., and Zhang, N. M. (2006). Soil pH and organic matter in greenhouse. Ecol. Environ. 15, 367–370. doi: 10.3969/j.issn.1674-5906.2006.02.035
Dotaniya, M., and Meena, V. (2015). Rhizosphere effect on nutrient availability in soil and its uptake by plants: a review. Proc. Natl. Acad. Sci. India B 85, 1–12. doi: 10.1007/s40011-013-0297-0
Feng, G. D., Yang, S. Z., Li, H. P., and Zhu, H. H. (2016). Massilia putida sp. nov., a dimethyl disulfide-producing bacterium isolated from wolfram mine tailing. Int. J. Syst. Evol. Microbiol. 66, 50–55. doi: 10.1099/ijsem.0.000670
Fernandez, A. L., Sheaffer, C. C., Wyse, D. L., Staley, C., Gould, T. J., and Sadowsky, M. J. (2016). Associations between soil bacterial community structure and nutrient cycling functions in long-term organic farm soils following cover crop and organic fertilizer amendment. Sci. Total Environ. 566, 949–959. doi: 10.1016/j.scitotenv.2016.05.073
Gilroy, S., Hughes, W. A., and Trewavas, A. J. (1989). A comparison between quin-2 and aequorin as indicators of cytoplasmic calcium levels in higher plant cell protoplasts. Plant Physiol. 90, 482–491. doi: 10.1104/pp.90.2.482
Gu, X., Liu, Y. W., Wang, X. P., Sun, Q., Wang, R., Hu, F. F., et al. (2019). Microbial remediation of chlorpyrifos by Brevundimonas sp. A1A18 in alkaline soil. Ecol. Environ. 28, 181–189. doi: 10.16258/j.cnki.1674-5906.2019.01.021
Hashidoko, Y., Nakayama, T., Homma, Y., and Tahara, S. (1999). Structure elucidation of xanthobaccin A, a new antibiotic produced from Stenotrophomonas sp. strain SB-K88. Tetrahedron Lett. 40, 2957–2960. doi: 10.1016/S0040-4039(99)00336-6
Huang, C. Y. (2000). Facing the 21st century curriculum materials Edaphology. Beijing: China Agricultre Press.
Islam, A., Kabir, M. S., and Khair, A. (2019). Molecular identification and evaluation of indigenous bacterial isolates for their plant growth promoting and biological control activities against Fusarium wilt pathogen of tomato. Plant Pathol. J. 35, 137–148. doi: 10.5423/PPJ.OA.06.2018.0104
Ji, G. H. (2011). Advances in the study on Lysobacter spp. bacteria and their effects on biological control of plant diseases. J. Yunnan Agric. Univ. 26, 124–130.
Ji, J. Q. (2020). Effect of oyster shell powder on adjusting soil pH and controlling tobacco bacterial wilt. Master’s thesis. Chongqing: Southwest University.
Jiang, Y., Zhang, Y. G., and Liang, W. J. (2005). Influence of greenhouse vegetable cultivation on composition of soil exchangeable base cations. J. Soil Water Conserv. 19, 78–81. doi: 10.3321/j.issn:1009-2242.2005.06.020
Kamdem, C. N., Fogue, P. S., Tiofack, A. A. Z., Mewamba, E. M., Womeni, H. M., Koffi, M., et al. (2023). Assessment of cetyl-trimethyl-ammonium bromide (CTAB) based method for the extraction of soil-transmitted helminth DNAs from stools for molecular dagnostic of soil-transmitted helminthiasis. J. Microbiol. Methods 204:106661. doi: 10.1016/j.mimet.2022.106661
Kobayashi, D. Y., and Yuen, G. Y. (2005). The role of clp-regulated factors in antagonism against Magnaporthe poae and biological control of summer patch disease of Kentucky bluegrass by Lysobacter enzymogenes C3. Can. J. Microbiol. 51, 719–723. doi: 10.1139/w05-056
Krug, E. C., and Frink, C. R. (1983). Acid rain on acid soil: a new perspective. Science 221, 520–525. doi: 10.1126/science.221.4610.520
Lee, C. H., Lee, D. K., Ali, M. A., and Kim, P. J. (2008). Effects of oyster shell on soil chemical and biological properties and cabbage productivity as a liming materials. Waste Manag. 28, 2702–2708. doi: 10.1016/j.wasman.2007.12.005
Li, G. (2018). Application of soil microbial studies to farmland quality evaluation. Acta Pedol. Sin. 55, 543–556. doi: 10.11766/trxb201710310320
Li, H., Li, J., Qin, X., Zeng, Z., Lin, Z., and Li, Q. (2017). The status, problems, and countermeasure of oyster industry in China based on the empirical analyses of Shandong Fujian and Guangdong Guangxi provinces. Mar. Sci. 41, 125–129.
Li, W. Q., and Zhang, M. (2001). Salt contents in soils under plastic greenhouse gardening in China. Pedosphere 11, 359–367. doi: 10.1007/s11769-001-0054-9
Li, X. T., Yang, W. Y., Sun, S. S., Gong, B., and Shi, Q. H. (2019). Effect of exogenous melatonin on alleviating calcium deficiency stress in tomato. Plant Physiol. Commun. 55, 169–176. doi: 10.13592/j.cnki.ppj.2018.0517
Li, Y. Q. (2019). Effect of oyster shell soil conditioner on the quality of Guanxi pomelo. Master’s thesis. Xiamen: Jimei University.
Lin, D., Sun, M. Q., Zhang, K. F., and Lin, R. X. (2019). Analysis on the development situation of Fujian oyster industry. China Fish. 53–57.
Lin, L., Xu, K., Shen, D., Chou, S. H., Gomelsky, M., and Qian, G. (2021). Antifungal weapons of Lysobacter, a mighty biocontrol agent. Environ. Microbiol. 23, 5704–5715. doi: 10.1111/1462-2920.15674
Liu, J. L., Bao, J., Li, J. S., and Gao, Y. M. (2019). Effects of different calcium application on tomato quality, yield and nutrients under roots restriction. Southwest China J. Agric. Sci. 32, 2403–2411. doi: 10.16213/j.cnki.scjas.2019.10.024
Liu, Y., Sun, M., Hou, P., Wang, W., Shen, X., Zhang, L., et al. (2022). Analysis of microbial community structure and volatile compounds in pit mud used for manufacturing Taorong-type Baijiu based on high-throughput sequencing. Sci. Rep. 12:7347. doi: 10.1038/s41598-022-10412-8
Naqqash, T., Imran, A., Hameed, S., Shahid, M., Majeed, A., Iqbal, J., et al. (2020). First report of diazotrophic Brevundimonas spp. as growth enhancer and root colonizer of potato. Sci. Rep. 10:12893. doi: 10.1038/s41598-020-69782-6
Neale, S. P., Shah, Z., and Adams, W. A. (1997). Changes in microbial biomass and nitrogen turnover in acidic organic soils following liming. Soil Biol. Biochem. 29, 1463–1474. doi: 10.1016/s0038-0717(97)00040-0
Ofek, M., Hadar, Y., and Minz, D. (2012). Ecology of root colonizing Massilia (Oxalobacteraceae). PLoS One 7:e40117. doi: 10.1371/journal.pone.0040117
Penn, C., and Camberato, J. (2019). A critical review on soil chemical processes that control how soil pH affects phosphorus availability to plants. Agriculture 9:120. doi: 10.3390/agriculture9060120
Reza, S. K., Baruah, U., Sarkar, D., and Singh, S. K. (2016). Spatial variability of soil properties using geostatistical method: a case study of lower Brahmaputra plains, India. Arab. J. Geosci. 9:446. doi: 10.1007/s12517-016-2474-y
Rousk, J., Bååth, E., Brookes, P. C., Lauber, C. L., Lozupone, C., Caporaso, J. G., et al. (2010). Soil bacterial and fungal communities across a pH gradient in an arable soil. ISME J. 4, 1340–1351. doi: 10.1038/ismej.2010.58
Shen, G., Zhang, S., Liu, X., Jiang, Q., and Ding, W. (2018). Soil acidification amendments change the rhizosphere bacterial community of tobacco in a bacterial wilt affected field. Appl. Microbiol. Biotechnol. 102, 9781–9791. doi: 10.1007/s00253-018-9347-0
Shen, T. X. (1999). Current situation of facility agricultue at home and abroad and development countermeasures of our province. J. Agric. Mech. Res. 5–10.
Shen, Z. Z., Huang, Y., Cao, Y. F., Wang, D. S., Liu, H. J., Li, R., et al. (2021). Comparison of bacterial communities in bulk and rhizosphere soils of healthy and diseased tomato infected by bacterial wilt. Soils 53, 5–12. doi: 10.13758/j.cnki.tr.2021.01.002
Su, T., Si, M., Wang, Z., and Li, S. (2005). Effects of pretreatment, shaking and conserving method and extracting solution on results for soil mineral nitrogen. J. Agro-Environ. Sci. 24, 1238–1242.
Tan, Y., He, Y., and Guo, W. (2014). Research progress on soil microbial diversity based on molecular techniques. J. Cent. South Univ. For. Technol. 34, 1–9. doi: 10.3969/j.issn.1673-923X.2014.10.001
Urakami, T., Ito-Yoshida, C., Araki, H., Kijima, T., Suzuki, K. I., and Komagata, K. (1994). Transfer of Pseudomonas plantarii and Pseudomonas glumae to Burkholderia as Burkholderia spp. and description of Burkholderia vandii sp. nov. Int. J. Syst. Evol. Microbiol. 44, 235–245. doi: 10.1099/00207713-44-2-235
Wang, H., Dong, Y., An, Q., and Sun, H. (2005). Change in pH and salinity of vegetable soil under intensive cultivation—a case study of southern suburbs of Nanjing. Soils 37, 530–533. doi: 10.3321/j.issn:0253-9829.2005.05.011
Wang, L., Du, H., Han, Z., and Zhang, X. (2013). Nitrous oxide emissions from black soils with different pH. J. Environ. Sci. 25, 1071–1076. doi: 10.1016/s1001-0742(12)60129-6
Wu, H. N., Huang, Z. P., and Tang, X. M. (2019). Effects of nitrogen reduction on diversity of soil nitrogen-fixing microbes in peanut rhizosphere. Jiangsu Agric. Sci. 47, 93–97. doi: 10.15889/j.issn.1002-1302.2019.16.019
Wu, Y., Guo, J., Tang, Z., Wang, T., Li, W., Wang, X., et al. (2023). Moso bamboo (Phyllostachys edulis) expansion enhances soil pH and alters soil nutrients and microbial communities. Sci. Total Environ. 912:169346. doi: 10.1016/j.scitotenv.2023.169346
Xiang, F., Li, W., Liu, H., Yin, X., Zeng, Z., and Zhou, L. (2021). On the influence of nitrogen fertilizer reduction on the structure of bacterial community in tea plantation soil. Biotechnol. Bull. 37, 1–8. doi: 10.13560/j.cnki.biotech.bull.1985.2020-1287
Xie, J. W. (2019). Current situation and improvement measures of paddy soil acidification in Dongkou County. China Agric. Technol. Ext. 35, 50–52. doi: 10.3969/j.issn.1002-381X.2019.03.026
Xu, P. X., Han, L. L., He, J. Z., Luo, F., and Zhang, L. M. (2017). Research advance on molecular ecology of asymbiotic nitrogen fixation microbes. J. Appl. Ecol. 28, 3440–3450. doi: 10.13287/j.1001-9332.201710.035
Xu, X. J. (2003). Effects of the phosphorus, Potassium cooperated with Calcium on Calcium Absorption of Tomasto in Greenhous. Master’s thesis. Shanxi: Shanxi Agricultural University.
Xu, X. Y. (2019). Screening of combined initiators for tomato rootstock seeds and study on their effects. Master’s thesis. Nanjing Agricultural University.
Xu, Y., Yu, W., Ma, Q., and Zhou, H. (2010). Assessment of the impact of different fertilization systems on soil microbial ecology. Chin. J. Soil Sci. 41, 1262–1269. doi: 10.1080/00949651003724790
Yan, J. H. (2019). Oyster shell soil conditioner: effects on peanut yield and acidified soil amendment in yellow clayey field. J. Agric. Sci. 9, 17–20.
Yang, E. D., Cui, D. X., and Wang, W. Y. (2019). Research progress on the genus Massilia. Microbiol. China 46, 1537–1548. doi: 10.13344/j.microbiol.china.180573
Yu, F., Zaleta-Rivera, K., Zhu, X., Huffman, J., Millet, J. C., Harris, S. D., et al. (2007). Structure and biosynthesis of heat-stable antifungal factor (HSAF), a broad-spectrum antimycotic with a novel mode of action. Antimicrob. Agents Chem. 51, 64–72. doi: 10.1128/AAC.00931-06
Zeng, B. (2018). Research progress of acidified soil improvement technology. Rural Sci. Technol. 17, 110–111. doi: 10.3969/j.issn.1674-7909.2018.17.067
Zhalnina, K., Dias, R., De Quadros, P. D., Davis-Richardson, A., Camargo, F. A., Clark, I. M., et al. (2015). Soil pH determines microbial diversity and composition in the park grass experiment. Microb. Ecol. 69, 395–406. doi: 10.1007/s00248-014-0530-2
Zhang, G., Liu, Y., Ni, Y., Meng, Z., Lu, T., and Li, T. (2014). Exogenous calcium alleviates low night temperature stress on the photosynthetic apparatus of tomato leaves. PLoS One 9:e97322. doi: 10.1371/journal.pone.0097322
Zhang, H., Wang, L., Li, Y., Wang, P., and Wang, C. (2019). Background nutrients and bacterial community evolution determine 13C-17β-estradiol mineralization in lake sediment microcosms. Sci. Total Environ. 651, 2304–2311. doi: 10.1016/j.scitotenv.2018.10.098
Zhang, J., Lin, X., Liu, W., and Yin, R. (2012). Response of soil microbial community to the bioremediation of soil contaminated with PAHs. Environ. Sci. 33, 2825–2831.
Zhang, K. W. (2019). Identification and stress response analysis of tomato calcium channel OSCA gene family. Master’s thesis. Harbin: Northeast Agricultural University.
Zhang, Q. M. (2016). Study on the DNA barcode detection technology of Burkholderia spp. plant pathogens. Master’s thesis. Nanjing: Nanjing Agricultural University.
Zhang, R. (2014). Public policy research of oyster industry in Guangdong Province. Master’s thesis. Zhanjiang: GuangdongOcean University.
Zhang, S. T. (2018). Study on the mechanism of aluminum ions affecting the occurrence of tobacco bacterial wilt. Master’s thesis. Chongqing: Southwest University.
Zhang, W., Liu, S., Zhang, M., Li, Y., Sheng, K., and Xu, Z. (2019). Phyllostachys edulis (Moso bamboo) rhizosphere increasing soil microbial activity rather than biomass. J. Soils Sediments 19, 2913–2926. doi: 10.1007/s11368-019-02334-2
Zhao, J., Yang, N. D., and Zhou, L. (2015). Review on development and utilization of oyster shell resources. Anhui Agric. Sci. Bull. 21, 79–80. doi: 10.3969/j.issn.1007-7731.2015.21.036
Keywords: oyster shell soil conditioner, acidic red loam soil, tomato, soil physicochemical, bacterial flora structure
Citation: Zheng Y, Yu C, Xiao Y, Ye T and Wang S (2024) The impact of utilizing oyster shell soil conditioner on the growth of tomato plants and the composition of inter-root soil bacterial communities in an acidic soil environment. Front. Microbiol. 14:1276656. doi: 10.3389/fmicb.2023.1276656
Received: 12 August 2023; Accepted: 27 December 2023;
Published: 16 January 2024.
Edited by:
Jian-Wei Guo, Kunming University, ChinaReviewed by:
YingWu Shi, Xinjiang Academy of Agricultural Sciences, ChinaZhanfeng Xia, Tarim University, China
Copyright © 2024 Zheng, Yu, Xiao, Ye and Wang. This is an open-access article distributed under the terms of the Creative Commons Attribution License (CC BY). The use, distribution or reproduction in other forums is permitted, provided the original author(s) and the copyright owner(s) are credited and that the original publication in this journal is cited, in accordance with accepted academic practice. No use, distribution or reproduction is permitted which does not comply with these terms.
*Correspondence: Yi Zheng, eyizheng@fjnu.edu.cn