- 1Dipartimento di Scienze Agroalimentari, Ambientali e Animali, Università degli Studi di Udine, Udine, Italy
- 2enotecUPM, Universidad Politécnica de Madrid, Madrid, Spain
In the current situation, wine areas are affected by several problems in a context of global warming: asymmetric maturities, pH increasing, high alcohol degree and flat wines with low freshness and poor aroma profile. The use of emerging biotechnologies allows to control or manage such problems. Emerging non-Saccharomyces as Lachancea thermotolerans are very useful for controlling pH by the formation of stable lactic acid from sugars with a slight concomitant alcohol reduction. Lower pH improves freshness increasing simultaneously microbiological stability. The use of Hanseniaspora spp. (specially H. vineae and H. opuntiae) or Metschnikowia pulcherrima promotes a better aroma complexity and improves wine sensory profile by the expression of a more complex metabolic pattern and the release of extracellular enzymes. Some of them are also compatible or synergic with the acidification by L. thermotolerans, and M. pulcherrima is an interesting biotool for reductive winemaking and bioprotection. The use of bioprotection is a powerful tool in this context, allowing oxidation control by oxygen depletion, the inhibition of some wild microorganisms, improving the implantation of some starters and limiting SO2. This can be complemented with the use of reductive yeast derivatives with high contents of reducing peptides and relevant compounds such as glutathione that also are interesting to reduce SO2. Finally, the use of emerging non-thermal technologies as Ultra High-Pressure Homogenization (UHPH) and Pulsed Light (PL) increases wine stability by microbial control and inactivation of oxidative enzymes, improving the implantation of emerging non-Saccharomyces and lowering SO2 additions.
Highlights
• Acidifying non-Saccharomyces yeasts: Lachancea thermotolerans.
• Aroma improvement with apiculate yeasts from the genus Hanseniaspora spp.
• Bioprotection, aroma enhancement and biocompatibility by Metschnikowia pulcherrima.
• Yeast polysaccharides and reductive compounds from cell walls and aging on lees. The use of Schizosaccharomyces pombe and Schizosaccharomyces japonicus.
• Control of malolactic fermentation with fumaric acid.
• Emerging non-thermal technologies to improve the implantation of non-Saccharomyces yeasts and to control oxidative enzymes.
Introduction
Global warming is already clearly affecting grape ripening by modifying the composition and affecting polyphenol contents, freshness, acidity, pH, sugars, stability, sensory balance, aroma and color (Jones et al., 2005; Mozell and Thach, 2014; Drappier et al., 2019; Gutiérrez-Gamboa et al., 2021). Additionally, global warming is increasing the water stress in vines due to lower rainfall in many regions especially during the fruit growth and increasing pests and diseases (Jones and Alves, 2012; Sgubin et al., 2022).
The global warming in some regions has produced an improvement in quality but it looks that is reaching its peak (Gambetta and Kurtural, 2021). A global temperature increase of 0.3–1.7°C is expected in the coming years (Drappier et al., 2019), therefore, the undesirable effects of temperature on grape maturity will be worst in the near future. Several viticultural techniques have been proposed as a tool to improve grape maturity in a context of global warming. Some of them can produce a 15-day delay in maturity by better adapting vine physiology and growth cycle to climatic conditions (Gutiérrez-Gamboa et al., 2021). Among them, the following can be considered as quite effective: severe shoot trimming, minimal pruning, leaf removal, late pruning and specially forced regrowth that can delay maturity by up to 2 months (Gutiérrez-Gamboa et al., 2021).
Different physicochemical options can be used to control sugars and acidity levels, such as the use of cation exchange resins (Lasanta et al., 2012) or electrodialysis with bipolar membranes to increase acidity and therefore reduce pH, or membrane technologies (i.e., reverse osmosis or nanofiltration) to reduce sugar content (El Rayess and Mietton-Peuchot, 2016).
But there are also many possibilities to control some of the most important effects of global warming in the winery by using fermentation biotechnologies. Concerning the loss of acidity due to high temperatures and the consequent effect on pH, freshness, and wine stability, a powerful tool is the use of acidifying yeasts that can reduce pH during fermentation by producing organic acids from sugars. Among them, the malic acid producers Saccharomyces cerevisiae (Sc) strains (Yéramian et al., 2007; Vion et al., 2023) and especially the lactic acid producers Lachancea thermotolerans (Lt) have been studied (Comitini et al., 2011; Morata et al., 2018; Porter et al., 2019a). The production of malic acid after a breeding process by selected S. cerevisiae yeasts can be higher than 3 g/L (Vion et al., 2023), with an impact on the sensory perception of the wines. The main issue concerning the production of malic acid is linked to its instability; in addition, it can be metabolized by lactic acid bacteria producing colloidal haze in the bottle. Regarding Lt, it has been reported that one specific strain produced more than 16 g/L of lactic acid (Banilas et al., 2016), but it is easy to find strains able to produce 1–5 g/L under enological conditions (Morata et al., 2022a). The use of Lt also determines a slight reduction in alcohol content (Ciani et al., 2016; Morata et al., 2019a; Hranilovic et al., 2021), being the latter a concomitant problem in warm areas.
Acidifying non-Saccharomyces yeasts: Lachancea thermotolerans
Lachancea thermotolerans (Lt), formerly known as Kluyveromyces thermotolerans or Zygosaccharomyces thermotolerans, is a Saccharomycetaceae yeast that was first described in 1932 (Kurtzman, 2003). It is named Lachancea in honor to Dr. Marc-André Lachance, University of Western Ontario, Canada. The morphology is similar to that of Saccharomyces cerevisiae with an ovoid or spheroidal shape and multilateral budding during asexual reproduction and forms 1–4 ascospores by sexual reproduction (Kurtzman, 2003). Lt has a medium fermentative power reaching 5–9% v/v of ethanol (Morata et al., 2018), so it should be used in coinoculation or sequential fermentation with Sc to complete alcoholic fermentation and produce dry wines. Lt has also been described as a low volatile acidity producer (0.1–0.5 g/L) (Kapsopoulou et al., 2007; Comitini et al., 2011; Aponte and Blaiotta, 2016; Morata et al., 2019a) and moreover it is able to reduce volatile acidity under aerobic conditions (Vilela-Moura et al., 2008), with a moderate formation of ethyl acetate similar to Sc (Morata et al., 2019a). Moreover, even if Lt is sensitive to sulfites (Comitini et al., 2011), some strains can produce effective acidification at 25–75 mg/L of total SO2 (Vaquero et al., 2020), and its growth is affected by low fermentation temperatures (Vaquero et al., 2020).
Its main application in emerging wine biotechnology is acidification and pH control by metabolizing sugars to lactic acid with the use of lactate dehydrogenase enzymes (LDH). Pyruvate can be reduced to lactate by LDH as an alternative pathway to recover NAD+, thus reducing ethanol formation (Hranilovic et al., 2018). Bioproduction of lactic acid in Lt is related to the expression of three lactate dehydrogenase enzymes (LDH) and appears to be unaffected by the expression of alcohol dehydrogenases (ADH) (Sgouros et al., 2020), because of the similar expression of ADH in high and low lactic acid producing strains. LDH2 is up-regulated in high producing strains (Sgouros et al., 2020). Moreover, it has been observed in several strains that LDH2 and LDH3 are organized in tandem and LDH1 is located elsewhere (Gatto et al., 2020). There are also two lactate permeases involved in lactate excretion, JEN1 in a single copy and 2 sequences of ADY2 (Gatto et al., 2020).
The effect of Lt is very powerful, and it is easy to decrease the pH of 0.1–0.5 units, depending on the fermentation time; however, most of the acidification is done at the beginning of fermentation, before day 6 (Morata et al., 2018). Many Lt strains can degrade malate but some of them are also able to produce small amounts of malic acid, up to 0.3 g/L (Hranilovic et al., 2018).
Lt has shown a good ability to control pH in real musts or crushed grapes from several Vitis vinifera L. white (Albariño, Airen, Vilana, Treixadura, Viognier) and red varieties (Tempranillo, Mencía, Merlot, Cabernet sauvignon) (Balikci et al., 2016; Morata et al., 2019a; Binati et al., 2020; Blanco et al., 2020; Sgouros et al., 2020; Vaquero et al., 2020, 2021a; Hranilovic et al., 2021, 2022; Zhang et al., 2023). The pH reduction is variable depending on several factors, but especially on the strain, and usually the reduction can range between 0.3 and 0.5 pH units (Table 1). The concomitant alcohol reduction has ranged from 0.2–0.5% v/v (Table 1). The scale up has been from 0.2–3 L in the laboratory to 60 L-400hL at pilot plant scale (Table 1).
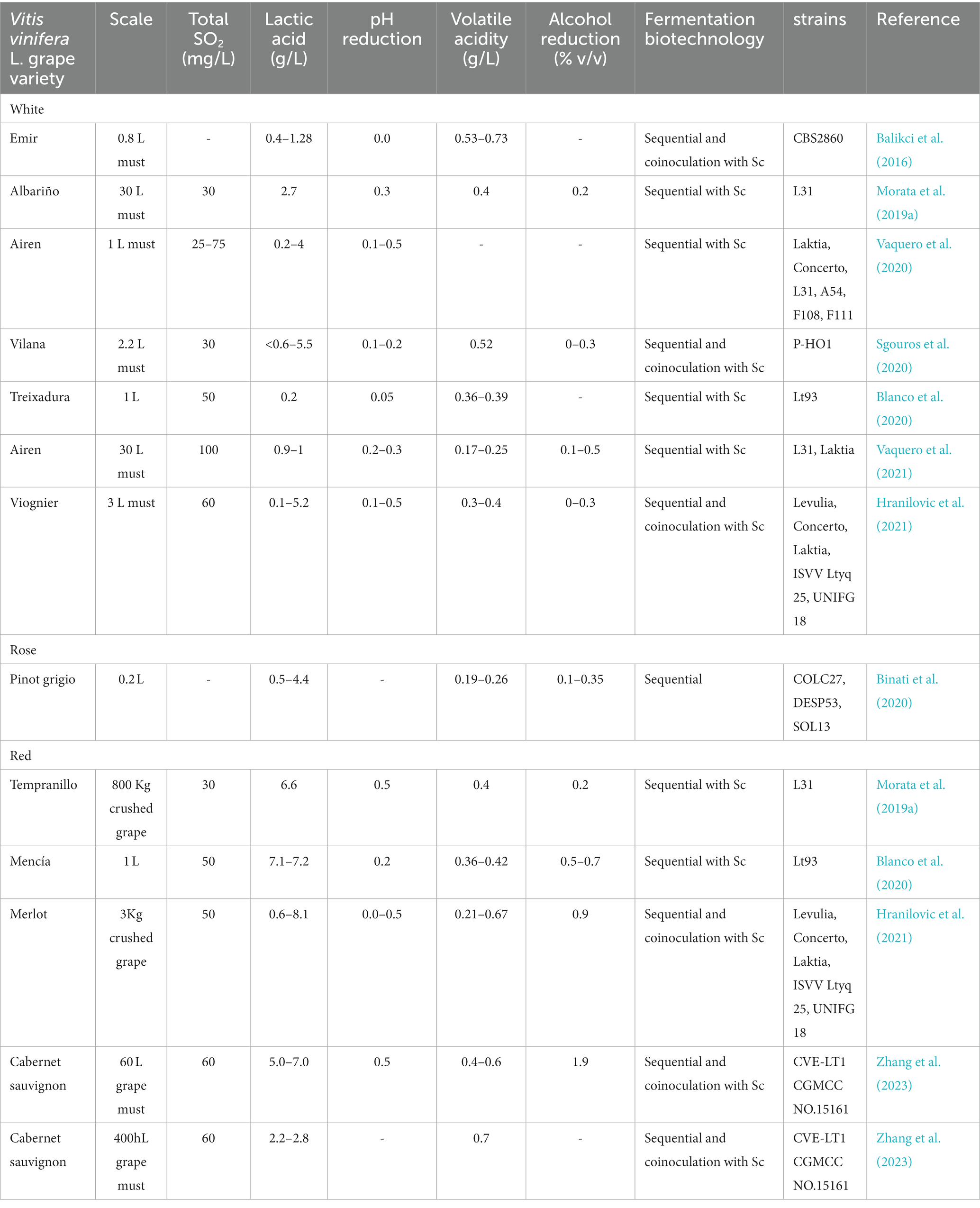
Table 1. Relevant literature results concerning wine acidification by fermentation with Lachancea thermotolerans in sequential fermentation or coinoculation with S. cerevisiae (Sc).
The high formation of lactic acid during fermentation with Lt can also have another positive effect in warm areas that is the by-product inhibitory effect on malolactic fermentation (MLF) (Morata et al., 2020a; Snyder et al., 2021), resulting in the preservation of malic acid that keeps the wines fresher and less flat. A strong inhibitory effect on MLF has been observed when lactic acid levels are 4 g/L or higher (Morata et al., 2020a), and it is even delayed at concentrations above 2 g/L. Moreover, it should be considered that lactic acid concentration is stable and cannot be degraded by microorganisms, so it preserves the wines from undesirable MLF during wine storage, aging on lees or bottle aging in sparkling wines.
The sensory impact of biological acidification by Lt is positive, producing a ‘citric freshness’ in the wines (Morata et al., 2020b), without ‘dairy notes’ that are more typical in MLF due to the formation of carbonyl metabolites such as diacetyl or acetoin. Lt produces these compounds at low concentrations like Saccharomyces cerevisiae.
In terms of aroma contribution, Lt has been described as a moderate producer of higher alcohols, with influence on aroma modulation by the production of floral acetate esters such as 2-phenylethyl acetate (Comitini et al., 2011; Gobbi et al., 2013; Morata et al., 2019a). Lt also produces high amounts of ethyl lactate that can be 30-folds higher than in Sc fermentations (Hranilovic et al., 2021) due to the formation of high levels of lactic acid. Furthermore, some strains are able to release terpenes and thiols as 4MMP and 3MH (Zott et al., 2011) by potential β-glucosidase and carbon-sulfur lyase activities (Rosi et al., 1994; Zott et al., 2011; Porter et al., 2019b). Therefore, even when the main effect of the use of Lt in wines from areas affected by global warming is on the control of pH and alcoholic degree, a positive modulation of aroma can also be obtained by the formation of positive floral and fruity esters or the release of bonded thiols and terpenes. Moreover, the acidification produced by Lt increases the amount of molecular SO2 by pH effect, helping to reduce the overall doses and increasing the effectiveness of its antimicrobial and antioxidant functions (Morata et al., 2021). The selection and industrial scale-up of this species, including production as dry yeast, is well designed and optimized, making Lt a good candidate for industrial production (Morata et al., 2022b).
Aroma improvement with apiculate yeasts from the genus Hanseniaspora spp.
Apiculate yeasts of the genus Hanseniaspora usually predominate on grape skins at maturity and, in the early stages of spontaneous fermentation, they dominate up to an alcohol level of 4–6% v/v (Moreira et al., 2011).
Hanseniaspora spp. have apiculate shape, like lemon, with polar budding (Martin et al., 2018). These yeasts have been traditionally considered as undesired and excluded in the fermentation using SO2, because of their potential effect on volatile acidity and ethyl acetate formation. However, several species, depending on the strain, produce moderate volatile acidity, some of them (e.g., Hanseniaspora vineae – Hv) even lower than Sc (del Fresno et al., 2021a). Additionally, Hanseniaspora spp. have been frequently described as overproducers of floral and fruity acetate esters such as 2-phenylethyl acetate or isoamyl acetate, positively improving flat wines from neutral varieties (Moreira et al., 2011). Moreover, the high glucosidase activity observed in some strains (Testa et al., 2020) may contribute to enhance the varietal characteristics of wines (Lombardi et al., 2018). Several recent reviews have highlighted the usefulness and positive impact of these species in wine technology, including aroma improvement and the effect on body and structure (Martin et al., 2018; van Wyk et al., 2023). Therefore, Hanseniaspora can be very useful for improving flat wines from regions affected by global warming, also having additional interesting applications in biocontrol (van Wyk et al., 2023).
Hv is a very interesting species from an enological point of view and described as a ‘friendly’ yeast (Carrau and Henschke, 2021), because of its ability to intensify the floral and fruity notes in wines by a high acetylation capacity and a highly developed phenylpropanoids pathway (Valera et al., 2021; Carrau et al., 2023), compared to other species such as H. uvarum. It is also convenient to use due to its good fermentative power, easily reaching 8–10% v/v ethanol, and with a production of volatile acidity lower than many Sc strains, often with values below 0.4 g/L (Martin et al., 2018; del Fresno et al., 2021a). Hv produces higher levels of 2-phenylethyl acetate and benzyl alcohol (Viana et al., 2011; Valera et al., 2021). Benzyl alcohol can be synthetized de novo by Hv (Martin et al., 2016) and the average content in several vinifications is 14-folds that obtained by Sc (Carrau et al., 2023). Hv yields high terpene contents in some must fermentations (x3 on average compared to Sc) (del Fresno et al., 2021a). The production of some specific spice compounds such as safranal in Hv above its sensory threshold has also been described (del Fresno et al., 2022). Protective effects on color has also been observed with improved hue parameters that can be representative of a lower oxidation in rose wines (del Fresno et al., 2021b).
Hanseniaspora opuntiae (Ho) is also an apiculate yeast but smaller than Hv (Vaquero et al., 2022). It can promote the release of some terpenes as citronellol (del Fresno et al., 2022; Badura et al., 2023) and has been described as a good producer of floral and fruity acetate esters (Bourbon-Melo et al., 2021; del Fresno et al., 2022), conferring floral notes in wines (Luan et al., 2018), with moderate volatile acidity and low levels of ethyl acetate (del Fresno et al., 2022). However, the fermentative power is lower than in Hv usually reaching 4–6% v/v ethanol depending on strains and fermentation conditions. A good compatibility with Lt has been observed to achieve good acidification as well as suitable release of aromatic esters (Vaquero et al., 2022). When Hv is used together with Lt, the high fermentative performance of Hv strongly decreases the acidification capacity of Lt (Vaquero et al., 2021). As observed for Hv and other Hanseniaspora spp., Ho also produces wines with good body, volume and a softer mouthfeel (Vaquero et al., 2022).
Bioprotection, aroma enhancement, and biocompatibility by Metschnikowia pulcherrima
Metschnikowia pulcherrima (Mp) is a globous or ellipsoidal multipolar budding yeast that evolves to spherical in adult cells due to the accumulation of large amounts of fatty compounds in the vacuole (Morata et al., 2019b). Several reviews have focused on the properties, characteristics and winemaking applications of Mp (Morata et al., 2019b; Sipiczki, 2020). It typically increases the fruity profile in wines and produces a positive sensory impact (Varela et al., 2017, 2021; Binati et al., 2020). In addition, many strains express β-glucosidase and β-lyase activities with remarkable intensity, thus promoting the release of free terpenes and volatile thiols in aromatic varieties (Barbosa et al., 2018). Some strains can be used to reduce the alcohol content of wines (Hranilovic et al., 2020). This species produces moderate or low levels of volatile acidity and H2S (Barbosa et al., 2018). Ethanol tolerance is quite good reaching 3–4%v/v in single fermentation (Barbosa et al., 2018), and viable cells of Mp can be found in the middle-end of alcoholic fermentation.
Mp has been considered an interesting yeast species for bioprotection with an effective antimicrobial effect against some non-Saccharomyces yeasts, but with good compatibility with Sc (Oro et al., 2014; Di Gianvito et al., 2022; Canonico et al., 2023). The antimicrobial and antioxidant activity of this species is mainly based on the production of pulcherrimin (Morata et al., 2019b; Sipiczki, 2020) and the effect on iron chelation. Prefermentative use of Mp has been suggested as an alternative to control microorganisms and to avoid or reduce SO2 (Simonin et al., 2020; Windholtz et al., 2021a,b; Agarbati et al., 2023). A non-negligible production of glutathione (GSH) was also observed for Metschnikowia spp. during the growth phase (Lemos Junior et al., 2021); during sequential fermentation, some strains may increase the final GSH content in wine up to 10 mg/L (Binati et al., 2021), potentially reducing oxidation risks and reducing SO2 requirements.
Mp has shown also very good biocompatibility and synergistic behavior concerning the acidification when used with Lt (Vaquero et al., 2021; Escott et al., 2022), together with a positive sensory impact. Therefore, the simultaneous use of Lt/Mp starters increases the acidification and the low pH promotes a higher proportion of molecular SO2, while producing a natural biocontrol on microbial populations, and improving the aroma profile.
Yeast polysaccharides and reductive compounds from cell walls and aging on lees: the use of Schizosaccharomyces pombe
Yeast derivatives, by-products and yeast lees during aging on lees are being widely used as additives to improve wine quality, by increasing mouthfeel and structure and by softening tannin astringency, but also to stabilize and clarify wines (Morata et al., 2019c; Vejarano, 2020; Rigou et al., 2021). The effect of lees and yeast by-products on the aromatic fraction has also been studied (Comuzzo et al., 2006, 2011; Loira et al., 2013), as well as the absorption of aroma and off-flavors by lees (Chassagne et al., 2005), or the application of lees as drivers of wood aroma has also been observed (Palomero et al., 2015).
To speed and enhance the effect of aging on lees or yeast derivatives, the use of non-Saccharomyces yeasts has been an innovative and powerful tool (Morata et al., 2019c; Vejarano, 2020). Some non-Saccharomyces yeasts such as Schizosaccharomyces pombe (Sp) or Schizosaccharomyces japonicus (Sj) have a special aptitude to release higher cell wall polysaccharide contents in a shorter time and with a positive impact on wine quality (Palomero et al., 2009; Domizio et al., 2017, 2018; Loira et al., 2018b; Portaro et al., 2022). The polysaccharide release capacity of several Sj strains is even higher than that of Sp (Domizio et al., 2017); even if their profiles show similarities, some differences in terms of galactose/mannose ratio have been observed. Both yeast species show interesting properties to be used in aging on lees and in the preparation of yeast derivatives. Polysaccharides of Sj have also produced a positive effect on the control of protein haze in wines (Millarini et al., 2020). The use of these biotechnological products derived from Sc or new species such as Sp or Sj is an interesting tool to improve wine volume and tannin integration in unbalanced grapes from warm areas.
The use of yeast derivatives rich in nitrogen reducing compounds and glutathione (GSH) is another key application of these additives that is especially relevant for reducing the use of SO2 as antioxidant (Rodríguez-Bencomo et al., 2014; Comuzzo et al., 2015; Bahut et al., 2019; Pons-Mercadé et al., 2021; Nioi et al., 2022). Currently, all the yeast derivatives marketed for winemaking use are from Saccharomyces spp. However, besides Sj and Sp (discussed above), other non-Saccharomyces strains might be exploited for this purpose. Different Hanseniaspora yeasts for instance, showed a relevant production of polysaccharides, thiol molecules and GSH during growth and after autolysis, in some cases even higher than certain Saccharomyces strains (Voce et al., 2022). The possibility to use yeast derivatives from non-Saccharomyces yeasts in winemaking is currently under discussion (step 3 out of 7) at the International Organization of Vine and Wine (OIV).1
Control of malolactic fermentation with fumaric acid
Recently, fumaric acid (FA) has been approved by the OIV to control MLF at a maximum dose of 600 mg/L (OIV, 2021), thanks to the inhibition of lactic acid bacteria (LAB), consequently preserving the malic acidity of wines (Cofran and Meyer, 1970; Tchelistcheff et al., 1971; Pilone et al., 1974). The control of LAB protects wines and helps to reduce SO2 levels. Additionally, FA at the allowed dose of 0.6 g/L can lower the pH of 0.05–0.1 units, also depending on the buffering power of the wine (Morata et al., 2023). FA is a stronger acidifier than tartaric acid (Gancel et al., 2022) and currently this additive is under evaluation by OIV also for wine acidification at 2–3 g/L. At the allowed dose, FA has a stronger inhibitory effect, even controlling and stopping an ongoing MLF with 60% malic acid degradation (Morata et al., 2020a). The inhibitory effect against other bacteria has also been published (Barnes and Karatzas, 2020), with an intense effect against acetic acid bacteria. Therefore, FA is a powerful tool to preserve and increase acidity in wines from warm areas. Its use can be complementary to malic acid-producing Sc yeasts or to Lt.
Emerging non-thermal technologies to improve the implantation of non-Saccharomyces yeasts and to control oxidative enzymes
The main drawback of most non-Saccharomyces yeasts is the lower fermentative power and the weaker competitiveness compared to Sc, which makes it necessary to use them in sequential or mixed fermentations, and to facilitate their implantation by must processing. Non-thermal technologies are very interesting to facilitate the implantation of non-Saccharomyces yeasts because of their high antimicrobial effectiveness and the mild effect on the sensory quality of grape and must (Morata et al., 2017). Among them, two techniques are particularly interesting for their efficacy and protective effect on sensory quality: Ultra High Pressure Homogenization (UHPH) and Pulsed Light (PL) (Table 2). Several recent reviews summarize the main features of UHPH (Zamora and Guamis, 2015; Patrignani and Lanciotti, 2016; Comuzzo and Calligaris, 2019; Morata and Guamis, 2020) and PL (Gómez-López et al., 2007; Oms-Oliu et al., 2010; Santamera et al., 2020; Vargas-Ramella et al., 2021).
UHPH involves a continuous pumping of liquid food (grape juice or wine) at pressure higher than 200 MPa (commonly 300 MPa) followed by a depressurization at atmospheric pressure through a special highly resistant valve (Zamora and Guamis, 2015; Morata and Guamis, 2020). In the valve, the fluid is subjected to extreme impact and shear stresses producing nanofragmentation of colloidal structures and microorganisms, down to a size of 300–500 nm (Morata and Guamis, 2020). This breakdown has a very powerful antimicrobial effect. It allows even the spores inactivation (depending on the in-valve temperature), but with a very gentle impact on sensory quality, thanks to the preservation of sensitive molecules such as terpenes (Bañuelos et al., 2020) and anthocyanins (Vaquero et al., 2022) and without formation of thermal markers such as hydroxymethylfurfural (Bañuelos et al., 2020). UHPH also inactivates oxidative enzymes (PPOs) and preserves the antioxidant activity (Loira et al., 2018a; Bañuelos et al., 2020). The highly effective elimination of wild microorganisms allows successful inoculations, even with non-Saccharomyces with low fermentative yield, and, therefore, permits a good expression of their metabolic profile, achieving good acidification and positive sensory impact.
PL entails the application of a high intensity broad spectrum light (200–2,500 nm) rich in UV (200–280 nm) by short duration light flashes (1 μs-0.1 s), typically using xenon lamps (Gómez-López et al., 2007; Santamera et al., 2020; Vargas-Ramella et al., 2021). The main drawback is the penetration depth which is less than 1 mm, but it can be applied on the surface of the grape after destemming to successfully eliminate wild yeasts (Escott et al., 2017). It therefore facilitates the implantation of unconventional yeast starters producing a high sensory impact on wine profile (Escott et al., 2021). In addition, PL is a gentle non-thermal technology (ΔT < 4°C) with protective effect on phenols and antioxidant capacity and low effects on color and anthocyanin degradation (Escott et al., 2017; Chakraborty et al., 2020; Bhagat and Chakraborty, 2022).
Conclusion
The association of emerging biotechnologies such as the use of non-Saccharomyces yeasts in sequential or mixed fermentations and non-thermal technologies to control wild microorganisms and the activity of oxidative enzymes in grapes or must may be a powerful strategy to improve wine quality in warm areas. This can improve the quality of wines from neutral varieties or facilitate the expression of under-ripe grapes. The stability and time persistence of these wines is also improved by the microbial control through emerging non-thermal technologies, the bioprotection and acidification produced by some of the non-Saccharomyces species discussed above, allowing the reduction of SO2 levels. This can also be supported by using antimicrobial additives such as fumaric acid and by the antioxidant properties of yeast derivatives. A new enology for a new climate scenario.
Author contributions
PC: Conceptualization, Writing – original draft, Writing – review & editing. JF: Writing – original draft, Writing – review & editing, Resources. SV: Writing – original draft, Writing – review & editing. IL: Writing – original draft, Writing – review & editing. AM: Writing – original draft, Writing – review & editing, Conceptualization, Funding acquisition, Validation.
Funding
The author(s) declare financial support was received for the research, authorship, and/or publication of this article. Our main funder is the Ministry of Science and Innovation (in Spanish), with the project ENOINNOVAPRESS - PID2021-124250OB-I00. This work was partially supported by the State Program to Promote Scientific-Technical Research and its transfer of the Spanish Ministry of Science and Innovation through the MALTA Consolider Team Research Network (RED2022-134388-T).
Conflict of interest
The authors declare that the research was conducted in the absence of any commercial or financial relationships that could be construed as a potential conflict of interest.
The author(s) declared that they were an editorial board member of Frontiers, at the time of submission. This had no impact on the peer review process and the final decision.
Publisher’s note
All claims expressed in this article are solely those of the authors and do not necessarily represent those of their affiliated organizations, or those of the publisher, the editors and the reviewers. Any product that may be evaluated in this article, or claim that may be made by its manufacturer, is not guaranteed or endorsed by the publisher.
Footnotes
References
Agarbati, A., Canonico, L., Ciani, M., and Comitini, F. (2023). Metschnikowia pulcherrima in cold clarification: biocontrol activity and aroma enhancement in Verdicchio wine. Fermentation 9:302. doi: 10.3390/fermentation9030302
Aponte, M., and Blaiotta, G. (2016). Potential role of yeast strains isolated from grapes in the production of Taurasi DOCG. Front. Microbiol. 7:809. doi: 10.3389/fmicb.2016.00809
Badura, J., Kiene, F., Brezina, S., Fritsch, S., Semmler, H., Rauhut, D., et al. (2023). Aroma profiles of Vitis vinifera L. cv. Gewürztraminer must fermented with co-cultures of Saccharomyces cerevisiae and seven Hanseniaspora spp. Fermentation 9:109. doi: 10.3390/fermentation9020109
Bahut, F., Liu, Y., Romanet, R., Coelho, C., Sieczkowski, N., Alexandre, H., et al. (2019). Metabolic diversity conveyed by the process leading to glutathione accumulation in inactivated dry yeast: a synthetic media study. Food Res. Int. 123, 762–770. doi: 10.1016/j.foodres.2019.06.008
Balikci, E. K., Tanguler, H., Jolly, N. P., and Erten, H. (2016). Influence of Lachancea thermotolerans on cv. Emir wine fermentation. Yeast 33, 313–321. doi: 10.1002/yea.3166
Banilas, G., Sgouros, G., and Nisiotou, A. (2016). Development of microsatellite markers for Lachancea thermotolerans typing and population structure of wine-associated isolates. Microbiol. Res. 193, 1–10. doi: 10.1016/j.micres.2016.08.010
Bañuelos, M. A., Loira, I., Guamis, B., Escott, C., Del Fresno, J. M., Codina-Torrella, I., et al. (2020). White wine processing by UHPH without SO2. Elimination of microbial populations and effect in oxidative enzymes, colloidal stability and sensory quality. Food Chem. 332:127417. doi: 10.1016/j.foodchem.2020.127417
Barbosa, C., Lage, P., Esteves, M., Chambel, L., Mendes-Faia, A., and Mendes-Ferreira, A. (2018). Molecular and phenotypic characterization of Metschnikowia pulcherrima strains from Douro wine region. Fermentation 4:8. doi: 10.3390/fermentation4010008
Barnes, R. H., and Karatzas, K. A. G. (2020). Investigation into the antimicrobial activity of fumarate against listeria monocytogenes and its mode of action under acidic conditions. Int. J. Food Microbiol. 324:108614. doi: 10.1016/j.ijfoodmicro.2020.108614
Bhagat, B., and Chakraborty, S. (2022). Potential of pulsed light treatment to pasteurize pomegranate juice: microbial safety, enzyme inactivation, and phytochemical retention. LWT 159:113215. doi: 10.1016/j.lwt.2022.113215
Binati, R. L., Lemos Junior, W. J. F., Luzzini, G., Slaghenaufi, D., Ugliano, M., and Torriani, S. (2020). Contribution of non-Saccharomyces yeasts to wine volatile and sensory diversity: a study on Lachancea thermotolerans, Metschnikowia spp. and Starmerella bacillaris strains isolated in Italy. Int. J. Food Microbiol. 318:108470. doi: 10.1016/j.ijfoodmicro.2019.108470
Binati, R. L., Lemos Junior, W. J. F., and Torriani, S. (2021). Contribution of non-Saccharomyces yeasts to increase glutathione concentration in wine. Aust. J. Grape Wine Res. 27, 290–294. doi: 10.1111/ajgw.12473
Blanco, P., Rabuñal, E., Neira, N., and Castrillo, D. (2020). Dynamic of Lachancea thermotolerans population in monoculture and mixed fermentations: impact on wine characteristics. Beverages 6:36. doi: 10.3390/beverages6020036
Bourbon-Melo, N., Palma, M., Pinto Rocha, M., Ferreira, A., Bronze, M. R., Elias, H., et al. (2021). Use of Hanseniaspora guilliermondii and Hanseniaspora opuntiae to enhance the aromatic profile of beer in mixed-culture fermentation with Saccharomyces cerevisiae. Food Microbiol. 95:103678. doi: 10.1016/j.fm.2020.103678
Canonico, L., Agarbati, A., Galli, E., Comitini, F., and Ciani, M. (2023). Metschnikowia pulcherrima as biocontrol agent and wine aroma enhancer in combination with a native Saccharomyces cerevisiae. LWT 181:114758. doi: 10.1016/j.lwt.2023.114758
Carrau, F., Dellacassa, E., Boido, E., Medina, K., Valera, M. J., Fariña, L., et al. (2023). Biology and physiology of Hanseniaspora vineae: metabolic diversity and increase flavour complexity for food fermentation. FEMS Yeast Res. 23:foad 010. doi: 10.1093/femsyr/foad010
Carrau, F., and Henschke, P. A. (2021). Hanseniaspora vineae and the concept of friendly yeasts to increase autochthonous wine flavor diversity. Front. Microbiol. 12:702093. doi: 10.3389/fmicb.2021.702093
Chakraborty, S., Ghag, S., Bhalerao, P. P., and Gokhale, J. S. (2020). The potential of pulsed light treatment to produce enzymatically stable Indian gooseberry (Emblica officinal Gaertn.) juice with maximal retention in total phenolics and vitamin C. J. Food Process. Preserv 44:e14932. doi: 10.1111/jfpp.14932
Chassagne, D., Guilloux-Benatier, M., Alexandre, H., and Voilley, A. (2005). Sorption of wine volatile phenols by yeast lees. Food Chem. 91, 39–44. doi: 10.1016/j.foodchem.2004.05.044
Ciani, M., Morales, P., Comitini, F., Tronchoni, J., Canonico, L., Curiel, J. A., et al. (2016). Non-conventional yeast species for lowering ethanol content of wines. Front. Microbiol. 7:642. doi: 10.3389/fmicb.2016.00642
Cofran, D. R., and Meyer, B. J. (1970). The effect of fumaric acid on malo-lactic fermentation. Am. J. Enol. Vitic. 21, 189–192. doi: 10.5344/ajev.1970.21.4.189
Comitini, F., Gobbi, M., Domizio, P., Romani, C., Lencioni, L., Mannazzu, I., et al. (2011). Selected non-Saccharomyces wine yeasts in controlled multistarter fermentations with Saccharomyces cerevisiae. Food Microbiol. 28, 873–882. doi: 10.1016/j.fm.2010.12.001
Comuzzo, P., Battistutta, F., Vendrame, M., Páez, M. S., Graziano, L., and Zironi, R. (2015). Antioxidant properties of different products and additives in white wine. Food Chem. 168, 107–114. doi: 10.1016/j.foodchem.2014.07.028
Comuzzo, P., and Calligaris, S. (2019). Potential applications of high pressure homogenization in winemaking: a review. Beverages 5:56. doi: 10.3390/beverages5030056
Comuzzo, P., Tat, L., Fenzi, D., Brotto, L., Battistutta, F., and Zironi, R. (2011). Interactions between yeast autolysates and volatile compounds in wine and model solution. Food Chem. 127, 473–480. doi: 10.1016/j.foodchem.2011.01.026
Comuzzo, P., Tat, L., Tonizzo, A., and Battistutta, F. (2006). Yeast derivatives (extracts and autolysates) in winemaking: release of volatile compounds and effects on wine aroma volatility. Food Chem. 99, 217–230. doi: 10.1016/j.foodchem.2005.06.049
del Fresno, J. M., Escott, C., Carrau, F., Herbert-Pucheta, J. E., Vaquero, C., González, C., et al. (2022). Improving aroma complexity with Hanseniaspora spp.: terpenes, acetate esters, and Safranal. Fermentation 8:654. doi: 10.3390/fermentation8110654
del Fresno, J. M., Escott, C., Loira, I., Carrau, F., Cuerda, R., Schneider, R., et al. (2021a). The impact of Hanseniaspora vineae fermentation and ageing on lees on the terpenic aromatic profile of white wines of the Albillo variety. Int. J. Mol. Sci. 22:2195. doi: 10.3390/ijms22042195
del Fresno, J. M. D., Loira, I., Escott, C., Carrau, F., González, C., Cuerda, R., et al. (2021b). Application of Hanseniaspora vineae yeast in the production of rosé wines from a blend of Tempranillo and Albillo grapes. Fermentation 7:141. doi: 10.3390/fermentation7030141
Di Gianvito, P., Englezos, V., Rantsiou, K., and Cocolin, L. (2022). Bioprotection strategies in winemaking. Int. J. Food Microbiol. 364:109532. doi: 10.1016/j.ijfoodmicro.2022.109532
Domizio, P., Lencioni, L., Calamai, L., Portaro, L., and Bisson, L. F. (2018). Evaluation of the yeast Schizosaccharomyces japonicus for use in wine production. Am. J. Enol. Vitic. 69, 266–277. doi: 10.5344/ajev.2018.18004
Domizio, P., Liu, Y., Bisson, L. F., and Barile, D. (2017). Cell wall polysaccharides released during the alcoholic fermentation by Schizosaccharomyces pombe and S. japonicus: quantification and characterization. Food Microbiol. 61, 136–149. doi: 10.1016/j.fm.2016.08.010
Drappier, J., Thibon, C., Rabot, A., and Geny-Denis, L. (2019). Relationship between wine composition and temperature: impact on Bordeaux wine typicity in the context of global warming—review. Crit. Rev. Food Sci. Nutr. 59, 14–30. doi: 10.1080/10408398.2017.1355776
El Rayess, Y., and Mietton-Peuchot, M. (2016). Membrane Technologies in Wine Industry: an overview. Crit. Rev. Food Sci. Nutr. 56, 2005–2020. doi: 10.1080/10408398.2013.809566
Escott, C., López, C., Loira, I., González, C., Bañuelos, M. A., Tesfaye, W., et al. (2021). Improvement of must fermentation from late harvest cv. Tempranillo grapes treated with pulsed light. Foods 10:1416. doi: 10.3390/foods10061416
Escott, C., Vaquero, C., del Fresno, J. M., Bañuelos, M. A., Loira, I., Han, S.-Y., et al. (2017). Pulsed light effect in red grape quality and fermentation. Food Bioprocess Technol. 10, 1540–1547. doi: 10.1007/s11947-017-1921-4
Escott, C., Vaquero, C., Loira, I., López, C., González, C., and Morata, A. (2022). Synergetic effect of Metschnikowia pulcherrima and Lachancea thermotolerans in acidification and aroma compounds in Airén wines. Foods 11:3734. doi: 10.3390/foods11223734
Gambetta, G. A., and Kurtural, S. K. (2021). Global warming and wine quality: are we close to the tipping point? OENO One 55:361. doi: 10.20870/oeno-one.2021.353.3.4774
Gancel, A.-L., Payan, C., Koltunova, T., Jourdes, M., Christmann, M., and Teissedre, P.-L. (2022). Solubility, acidifying power and sensory properties of fumaric acid in water, hydro-alcoholic solutions, musts and wines compared to tartaric, malic, lactic and citric acids. OENO One 56, 137–154. doi: 10.20870/oeno-one.2022.56.3.5455
Gatto, V., Binati, R. L., Lemos Junior, W. J. F., Basile, A., Treu, L., de Almeida, O. G. G., et al. (2020). New insights into the variability of lactic acid production in Lachancea thermotolerans at the phenotypic and genomic level. Microbiol. Res. 238:126525. doi: 10.1016/j.micres.2020.126525
Gobbi, M., Comitini, F., Domizio, P., Romani, C., Lencioni, L., Mannazzu, I., et al. (2013). Lachancea thermotolerans and Saccharomyces cerevisiae in simultaneous and sequential co-fermentation: a strategy to enhance acidity and improve the overall quality of wine. Food Microbiol. 33, 271–281. doi: 10.1016/j.fm.2012.10.004
Gómez-López, V. M., Ragaert, P., Debevere, J., and Devlieghere, F. (2007). Pulsed light for food decontamination: a review. Trends Food Sci. Technol. 18, 464–473. doi: 10.1016/j.tifs.2007.03.010
Gutiérrez-Gamboa, G., Zheng, W., and Martínez de Toda, F. (2021). Current viticultural techniques to mitigate the effects of global warming on grape and wine quality: a comprehensive review. Food Res. Int. 139:109946. doi: 10.1016/j.foodres.2020.109946
Hranilovic, A., Albertin, W., Capone, D. L., Gallo, A., Grbin, P. R., Danner, L., et al. (2021). Impact of Lachancea thermotolerans on chemical composition and sensory profiles of merlot wines. Food Chem. 349:129015. doi: 10.1016/j.foodchem.2021.129015
Hranilovic, A., Albertin, W., Capone, D. L., Gallo, A., Grbin, P. R., Danner, L., et al. (2022). Impact of Lachancea thermotolerans on chemical composition and sensory profiles of viognier wines. J. Fungi 8:474. doi: 10.3390/jof8050474
Hranilovic, A., Gambetta, J. M., Jeffery, D. W., Grbin, P. R., and Jiranek, V. (2020). Lower-alcohol wines produced by Metschnikowia pulcherrima and Saccharomyces cerevisiae co-fermentations: the effect of sequential inoculation timing. Int. J. Food Microbiol. 329:108651. doi: 10.1016/j.ijfoodmicro.2020.108651
Hranilovic, A., Gambetta, J. M., Schmidtke, L., Boss, P. K., Grbin, P. R., Masneuf-Pomarede, I., et al. (2018). Oenological traits of Lachancea thermotolerans show signs of domestication and allopatric differentiation. Sci. Rep. 8:14812. doi: 10.1038/s41598-018-33105-7
Jones, G. V., and Alves, F. (2012). Impact of climate change on wine production: a global overview and regional assessment in the Douro Valley of Portugal. Int. J. Glob. Warm. 4, 383–406. doi: 10.1504/IJGW.2012.049448
Jones, G. V., White, M. A., Cooper, O. R., and Storchmann, K. (2005). Climate change and global wine quality. Clim. Chang. 73, 319–343. doi: 10.1007/s10584-005-4704-2
Kapsopoulou, K., Mourtzini, A., Anthoulas, M., and Nerantzis, E. (2007). Biological acidification during grape must fermentation using mixed cultures of Kluyveromyces thermotolerans and Saccharomyces cerevisiae. World J. Microbiol. Biotechnol. 23, 735–739. doi: 10.1007/s11274-006-9283-5
Kurtzman, C. P. (2003). Phylogenetic circumscription of Saccharomyces, Kluyveromyces and other members of the Saccharomycetaceae, and the proposal of the new genera Lachancea, Nakaseomyces, Naumovia, Vanderwaltozyma and Zygotorulaspora. FEMS Yeast Res. 4, 233–245. doi: 10.1016/S1567-1356(03)00175-2
Lasanta, C., Gómez, J., and Caro, I. (2012). “Applications of ion exchangers in alcohol beverage industry” in Ion exchange technology II. eds. D. Inamuddin and M. Luqman (Dordrecht: Springer), 97–107. doi: 10.1007/978-94-007-4026-6-4
Lemos Junior, W. J. F., Binati, R. L., Bersani, N., and Torriani, S. (2021). Investigating the glutathione accumulation by non-conventional wine yeasts in optimized growth conditions and multi-starter fermentations. LWT-Food Sci. Technol. 142:110990. doi: 10.1016/j.lwt.2021.110990
Loira, I., Morata, A., Bañuelos, M. A., Puig-Pujol, A., Guamis, B., González, C., et al. (2018a). Use of ultra-high pressure homogenization processing in winemaking: control of microbial populations in grape musts and effects in sensory quality. Innov. Food Sci. Emerg. Technol. 50, 50–56. doi: 10.1016/j.ifset.2018.10.005
Loira, I., Morata, A., Palomero, F., González, C., and Suárez-Lepe, J. A. (2018b). Schizosaccharomyces pombe: a promising biotechnology for modulating wine composition. Fermentation 4:70. doi: 10.3390/fermentation4030070
Loira, I., Vejarano, R., Morata, A., Ricardo-da-Silva, J. M., Laureano, O., González, M. C., et al. (2013). Effect of Saccharomyces strains on the quality of red wines aged on lees. Food Chem. 139, 1044–1051. doi: 10.1016/j.foodchem.2013.01.020
Lombardi, S. J., Pannella, G., Iorizzo, M., Moreno-Arribas, M. V., Tremonte, P., Succi, M., et al. (2018). Sequential inoculum of Hanseniaspora guilliermondii and Saccharomyces cerevisiae for winemaking Campanino on an industrial scale. World J. Microbiol. Biotechnol. 34, 161–110. doi: 10.1007/s11274-018-2540-6
Luan, Y., Zhang, B.-Q., Duan, C.-Q., and Yan, G.-L. (2018). Effects of different pre-fermentation cold maceration time on aroma compounds of Saccharomyces cerevisiae co-fermentation with Hanseniaspora opuntiae or Pichia kudriavzevii. LWT 92, 177–186. doi: 10.1016/j.lwt.2018.02.004
Martin, V., Giorello, F., Fariña, L., Minteguiaga, M., Salzman, V., Boido, E., et al. (2016). Novo synthesis of benzenoid compounds by the yeast Hanseniaspora vineae increases the flavor diversity of wines. J. Agric. Food Chem. 64, 4574–4583. doi: 10.1021/acs.jafc.5b05442
Martin, V., Valera, M. J., Medina, K., Boido, E., and Carrau, F. (2018). Oenological impact of the Hanseniaspora/Kloeckera yeast genus on wines—a review. Fermentation 4:76. doi: 10.3390/fermentation4030076
Millarini, V., Ignesti, S., Cappelli, S., Ferraro, G., Adessi, A., Zanoni, B., et al. (2020). Protection of wine from protein haze using Schizosaccharomyces japonicus polysaccharides. Foods 9:1407. doi: 10.3390/foods9101407
Morata, A., Adell, E., López, C., Palomero, F., Suárez, E., Pedrero, S., et al. (2023). Use of fumaric acid to inhibit malolactic fermentation in bottled Rioja wines: effect in pH and volatile acidity control. Beverages 9:16. doi: 10.3390/beverages9010016
Morata, A., Arroyo, T., Bañuelos, M. A., Blanco, P., Briones, A., Cantoral, J. M., et al. (2022b). Wine yeast selection in the Iberian Peninsula: Saccharomyces and non-Saccharomyces as drivers of innovation in Spanish and Portuguese wine industries. Crit. Rev. Food Sci. Nutr. 10, 1–29. doi: 10.1080/10408398.2022.2083574
Morata, A., Bañuelos, M. A., López, C., Song, C., Vejarano, R., Loira, I., et al. (2020a). Use of fumaric acid to control pH and inhibit malolactic fermentation in wines. Food Addit. Contam. 37, 228–238. doi: 10.1080/19440049.2019.1684574
Morata, A., Bañuelos, M. A., Vaquero, C., Loira, I., Cuerda, R., Palomero, F., et al. (2019a). Lachancea thermotolerans as a tool to improve pH in red wines from warm regions. Eur. Food Res. Technol. 245, 885–894. doi: 10.1007/s00217-019-03229-9
Morata, A., Escott, C., Bañuelos, M. A., Loira, I., del Fresno, J. M., González, C., et al. (2020b). Contribution of non-Saccharomyces yeasts to wine freshness. Biomol. 10:34. doi: 10.3390/biom10010034
Morata, A., and Guamis, B. (2020). Use of UHPH to obtain juices with better nutritional quality and healthier wines with low levels of SO2. Front. Nutr. 7:598286. doi: 10.3389/fnut.2020.598286
Morata, A., Loira, I., Escott, C., del Fresno, J. M., Bañuelos, M. A., and Suárez-Lepe, J. A. (2019b). Applications of Metschnikowia pulcherrima in wine biotechnology. Fermentation 5:63. doi: 10.3390/fermentation5030063
Morata, A., Loira, I., González, C., Bañuelos, M. A., Cuerda, R., Heras, J. M., et al. (2022a). “Chapter 11- biological acidification by Lachancea thermotolerans” in White wine technology. ed. A. Morata (London, UKElsevier-Academic Press), 131–142.
Morata, A., Loira, I., González, C., and Escott, C. (2021). Non-Saccharomyces as biotools to control the production of off-flavors in wines. Molecules 26:4571. doi: 10.3390/molecules26154571
Morata, A., Loira, I., Tesfaye, W., Bañuelos, M. A., González, C., and Suárez Lepe, J. A. (2018). Lachancea thermotolerans applications in wine technology. Fermentation 4:53. doi: 10.3390/fermentation4030053
Morata, A., Loira, I., Vejarano, R., González, C., Callejo, M. J., and Suárez-Lepe, J. A. (2017). Emerging preservation technologies in grapes for winemaking. Trends Food Sci. Technol. 67, 36–43. doi: 10.1016/j.tifs.2017.06.014
Morata, A., Palomero, F., Loira, I., and Suárez-Lepe, J. A. (2019c). “New trends in aging on lees” in Red wine technology. ed. A. Morata (London, UKAcademic Press), 163–176.
Moreira, N., Pina, C., Mendes, F., Couto, J. A., Hogg, T., and Vasconcelos, I. (2011). Volatile compounds contribution of Hanseniaspora guilliermondii and Hanseniaspora uvarum during red wine vinifications. Food Control 22, 662–667. doi: 10.1016/j.foodcont.2010.07.025
Mozell, M. R., and Thach, L. (2014). The impact of climate change on the global wine industry: challenges & solutions. Wine Econ. Policy 3, 81–89. doi: 10.1016/j.wep.2014.08.001
Nioi, C., Lisanti, M. T., Meunier, F., Redon, P., Massot, A., and Moine, V. (2022). Antioxidant activity of yeast derivatives: evaluation of their application to enhance the oxidative stability of white wine. LWT 171:114116. doi: 10.1016/j.lwt.2022.114116
OIV (2021). RESOLUTION OIV-OENO 581A-2021. Treatment with fumaric acid in wine to inhibit malolactic fermentation. Available at: https://www.oiv.int/public/medias/8084/en-oiv-oeno-581a-2021.pdf
Oms-Oliu, G., Martín-Belloso, O., and Soliva-Fortuny, R. (2010). Pulsed light treatments for food preservation. Rev. Food Bioprocess Technol. 3, 13–23. doi: 10.1007/s11947-008-0147-x
Oro, L., Ciani, M., and Comitini, F. (2014). Antimicrobial activity of Metschnikowia pulcherrima on wine yeasts. J. Appl. Microbiol. 116, 1209–1217. doi: 10.1111/jam.12446
Palomero, F., Bertani, P., Fernández de Simón, B., Cadahía, E., Benito, S., Morata, A., et al. (2015). Wood impregnation of yeast lees for winemaking. Food Chem. 171, 212–223. doi: 10.1016/j.foodchem.2014.08.108
Palomero, F., Morata, A., Benito, S., Calderón, F., and Suárez-Lepe, J. A. (2009). New genera of yeasts for over-lees aging of red wine. Food Chem. 112, 432–441. doi: 10.1016/j.foodchem.2008.05.098
Patrignani, F., and Lanciotti, R. (2016). Applications of high and ultra high pressure homogenization for food safety. Front. Microbiol. 7:1132. doi: 10.3389/fmicb.2016.01132
Pérez-López, A. J., Rodríguez-López, M. I., Burló, F., Carbonell-Barrachina, Á. A., Gabaldón, J. A., and Gómez-López, V. M. (2020). Evaluation of pulsed light to inactivate Brettanomyces bruxellensis in white wine and assessment of its effects on color and aromatic profile. Foods 9:1903. doi: 10.3390/foods9121903
Pilone, G. J., Rankine, B. C., and Pilone, D. A. (1974). Inhibiting malo-lactic fermentation in Australian dry red wines by adding fumaric acid. Am. J. Enol. Vitic. 25, 99–107. doi: 10.5344/ajev.1974.25.2.99
Pons-Mercadé, P., Anguela, S., Giménez, P., Heras, J. M., Sieczkowski, N., Rozès, N., et al. (2021). Measuring the oxygen consumption rate of some inactivated dry yeasts: comparison with other common wine antioxidants. OENO One 55:158. doi: 10.20870/oeno-one.2021.147.2.4618
Portaro, L., Maioli, F., Canuti, V., Picchi, M., Lencioni, L., Mannazzu, I., et al. (2022). Schizosaccharomyces japonicus/Saccharomyces cerevisiae mixed starter cultures: new perspectives for the improvement of Sangiovese aroma, taste, and color stability. LWT 156:113009. doi: 10.1016/j.lwt.2021.113009
Porter, T. J., Divol, B., and Setati, M. E. (2019a). Lachancea yeast species: origin, biochemical characteristics and oenological significance. Food Res. Int. 119, 378–389. doi: 10.1016/j.foodres.2019.02.003
Porter, T. J., Divol, B., and Setati, M. E. (2019b). Investigating the biochemical and fermentation attributes of Lachancea species and strains: deciphering the potential contribution to wine chemical composition. Int. J. Food Microbiol. 290, 273–287. doi: 10.1016/j.ijfoodmicro.2018.10.025
Rigou, P., Mekoue, J., Sieczkowski, N., Doco, T., and Vernhet, A. (2021). Impact of industrial yeast derivative products on the modification of wine aroma compounds and sensorial profile. Rev. Food Chem. 358:129760. doi: 10.1016/j.foodchem.2021.129760
Rodríguez-Bencomo, J. J., Andujar-Ortiz, I., Moreno-Arribas, M. V., Simò, C., Gonzales, J., Chana, A., et al. (2014). Impact of glutathione-enriched inactive dry yeast preparations on the stability of terpenes during model wine aging. J. Agric. Food Chem. 62, 1373–1383. doi: 10.1021/jf402866q
Rosi, I., Vinella, M., and Domizio, P. (1994). Characterization of β-glucosidase activity in yeasts of oenological origin. J. Appl. Bacteriol. 77, 519–527. doi: 10.1111/j.1365-2672.1994.tb04396.x
Santamera, A., Escott, C., Loira, I., del Fresno, J. M., González, C., and Morata, A. (2020). Pulsed light: challenges of a non-thermal sanitation Technology in the Winemaking Industry. Beverages 6:45. doi: 10.3390/beverages6030045
Sgouros, G., Mallouchos, A., Filippousi, M.-E., Banilas, G., and Nisiotou, A. (2020). Molecular characterization and enological potential of a high lactic acid-producing Lachancea thermotolerans vineyard strain. Foods 9:595. doi: 10.3390/foods9050595
Sgubin, G., Swingedouw, D., Mignot, J., Gambetta, G. A., Bois, B., Loukos, H., et al. (2022). Non-linear loss of suitable wine regions over Europe in response to increasing global warming. Glob. Chang. Biol. 29, 808–826. doi: 10.1111/gcb.16493
Simonin, S., Roullier-Gall, C., Ballester, J., Schmitt-Kopplin, P., Quintanilla-Casas, B., Vichi, S., et al. (2020). Bio-protection as an alternative to Sulphites: impact on chemical and microbial characteristics of red wines. Front. Microbiol. 11:1308. doi: 10.3389/fmicb.2020.01308
Sipiczki, M. (2020). Metschnikowia pulcherrima and related pulcherrimin-producing yeasts: fuzzy species boundaries and complex antimicrobial antagonism. Microorganisms 8:1029. doi: 10.3390/microorganisms8071029
Snyder, E. C., Jiranek, V., and Hranilovic, A. (2021). Impact of Lachancea thermotolerans strain and lactic acid concentration on Oenococcus oeni and malolactic fermentation in wine. OENO One 55, 365–380. doi: 10.20870/oeno-one.2021.55.2.4657
Tchelistcheff, A., Peterson, R. G., and Van Gelderen, M. (1971). Control of malolactic fermentation in wine. Am. J. Enol. Vitic. 22, 1–5. doi: 10.5344/ajev.1971.22.1.1
Testa, B., Lombardi, S. J., Iorizzo, M., Letizia, F., Di Martino, C., Di Renzo, M., et al. (2020). Use of strain Hanseniaspora guilliermondii BF1 for winemaking process of white grapes Vitis vinifera cv Fiano. Eur. Food Res. Technol. 246, 549–561. doi: 10.1007/s00217-019-03424-8
Valera, M. J., Olivera, V., Boido, E., Dellacassa, E., and Carrau, F. (2021). Wine aroma characterization of the two main fermentation yeast species of the apiculate genus Hanseniaspora. Fermentation 7:162. doi: 10.3390/fermentation7030162
van Wyk, N., Badura, J., von Wallbrunn, C., and Pretorius, I. S. (2023). Exploring future applications of the apiculate yeast Hanseniaspora. Crit. Rev. Biotechnol., 1–20. doi: 10.1080/07388551.2022.2136565
Vaquero, C., Escott, C., Heras, J. M., Carrau, F., and Morata, A. (2022). Co-inoculations of Lachancea thermotolerans with different Hanseniaspora spp.: acidification, aroma, biocompatibility, and effects of nutrients in wine. Food Res. Int. 161:111891. doi: 10.1016/j.foodres.2022.111891
Vaquero, C., Escott, C., Loira, I., Guamis, B., del Fresno, J. M., Quevedo, J. M., et al. (2022). Cabernet sauvignon red must processing by UHPH to produce wine without SO2: the colloidal structure, microbial and oxidation control, colour protection and sensory quality of the wine. Food Bioprocess Technol. 15, 620–634. doi: 10.1007/s11947-022-02766-8
Vaquero, C., Izquierdo-Cañas, P. M., Mena-Morales, A., Marchante-Cuevas, L., Heras, J. M., and Morata, A. (2021a). Use of Lachancea thermotolerans for biological vs. chemical acidification at pilot-scale in White wines from warm areas. Fermentation 7:193. doi: 10.3390/fermentation7030193
Vaquero, C., Loira, I., Bañuelos, M. A., Heras, J. M., Cuerda, R., and Morata, A. (2020). Industrial performance of several Lachancea thermotolerans strains for pH control in white wines from warm areas. Microorganisms 8:830. doi: 10.3390/microorganisms8060830
Vaquero, C., Loira, I., Heras, J. M., Carrau, F., González, C., and Morata, A. (2021). Biocompatibility in ternary fermentations with Lachancea thermotolerans, other non-Saccharomyces and Saccharomyces cerevisiae to control pH and improve the sensory profile of wines from warm areas. Front. Microbiol. 12:656262. doi: 10.3389/fmicb.2021.656262
Varela, C., Barker, A., Tran, T., Borneman, A., and Curtin, C. (2017). Sensory profile and volatile aroma composition of reduced alcohol merlot wines fermented with Metschnikowia pulcherrima and Saccharomyces uvarum. Int. J. Food Microbiol. 252, 1–9. doi: 10.1016/j.ijfoodmicro.2017.04.002
Varela, C., Bartel, C., Espinase Nandorfy, D. M., Bilogrevic, E., Tran, T., Heinrich, A., et al. (2021). Volatile aroma composition and sensory profile of shiraz and cabernet sauvignon wines produced with novel Metschnikowia pulcherrima yeast starter cultures. Aust. J. Grape Wine Res. 27, 406–418. doi: 10.1111/ajgw.12484
Vargas-Ramella, M., Pateiro, M., Gavahian, M., Franco, D., Zhang, W., Khaneghah, A. M., et al. (2021). Impact of pulsed light processing technology on phenolic compounds of fruits and vegetables. Trends Food Sci. Technol. 115, 1–11. doi: 10.1016/j.tifs.2021.06.037
Vejarano, R. (2020). Non-Saccharomyces in winemaking: source of Mannoproteins, nitrogen, enzymes, and antimicrobial compounds. Fermentation 6:76. doi: 10.3390/fermentation6030076
Viana, F., Belloch, C., Vallés, S., and Manzanares, P. (2011). Monitoring a mixed starter of Hanseniaspora vineae–Saccharomyces cerevisiae in natural must: impact on 2-phenylethyl acetate production. Int. J. Food Microbiol. 151, 235–240. doi: 10.1016/j.ijfoodmicro.2011.09.005
Vilela-Moura, A., Schuller, D., Mendes-Faia, A., and Côrte-Real, M. (2008). Reduction of volatile acidity of wines by selected yeast strains. Appl. Microbiol. Biotechnol. 80, 881–890. doi: 10.1007/s00253-008-1616-x
Vion, C., Muro, M., Bernard, M., Richard, B., Valentine, F., Yeramian, N., et al. (2023). New malic acid producer strains of Saccharomyces cerevisiae for preserving wine acidity during alcoholic fermentation. Food Microbiol. 112:104209. doi: 10.1016/j.fm.2022.104209
Voce, S., Iacumin, L., and Comuzzo, P. (2022). Characterization of non-Saccharomyces yeast strains isolated from grape juice and pomace: production of polysaccharides and antioxidant molecules after growth and autolysis. Fermentation 8, 1–13. doi: 10.3390/fermentation8090450
Windholtz, S., Redon, P., Lacampagne, S., Farris, L., Lytra, G., Cameleyre, M., et al. (2021a). Non-Saccharomyces yeasts as bioprotection in the composition of red wine and in the reduction of sulfur dioxide. LWT 149:111781. doi: 10.1016/j.lwt.2021.111781
Windholtz, S., Vinsonneau, E., Farris, L., Thibon, C., and Masneuf-Pomarède, I. (2021b). () yeast and filamentous Fungi microbial communities in organic red grape juice: effect of vintage, maturity stage, SO2, and bioprotection. Front. Microbiol. 12:748416. doi: 10.3389/fmicb.2021.748416
Yéramian, N., Chaya, C., and Suárez Lepe, J. A. (2007). L-(−)-malic acid production by Saccharomyces spp. during the alcoholic fermentation of wine. J. Agric. Food Chem. 55, 912–919. doi: 10.1021/jf061990w
Zamora, A., and Guamis, B. (2015). Opportunities for ultra-high-pressure homogenization (UHPH) for the food industry. Food Eng. Rev. 7, 130–142. doi: 10.1007/s12393-014-9097-4
Zhang, B., Hu, J., Cheng, C., Xu, Y., Duan, C., and Yan, G. (2023). Effects of native Lachancea thermotolerans combined with Saccharomyces cerevisiae on wine volatile and phenolic profiles in pilot and industrial scale. Food Chem. Adv. 2:100258. doi: 10.1016/j.focha.2023.100258
Keywords: non-Saccharomyces yeasts, Lachancea thermotolerans , Hanseniaspora spp., Metschnikowia pulcherrima , pH control, alcohol reduction, bioprotection, SO2 alternatives
Citation: Comuzzo P, del Fresno JM, Voce S, Loira I and Morata A (2023) Emerging biotechnologies and non-thermal technologies for winemaking in a context of global warming. Front. Microbiol. 14:1273940. doi: 10.3389/fmicb.2023.1273940
Edited by:
Huseyin Erten, Cukurova University, TürkiyeReviewed by:
Neil Paul Jolly, Stellenbosch University, South AfricaEduardo Boido, Universidad de la República, Uruguay
Copyright © 2023 Comuzzo, del Fresno, Voce, Loira and Morata. This is an open-access article distributed under the terms of the Creative Commons Attribution License (CC BY). The use, distribution or reproduction in other forums is permitted, provided the original author(s) and the copyright owner(s) are credited and that the original publication in this journal is cited, in accordance with accepted academic practice. No use, distribution or reproduction is permitted which does not comply with these terms.
*Correspondence: Antonio Morata, YW50b25pby5tb3JhdGFAdXBtLmVz