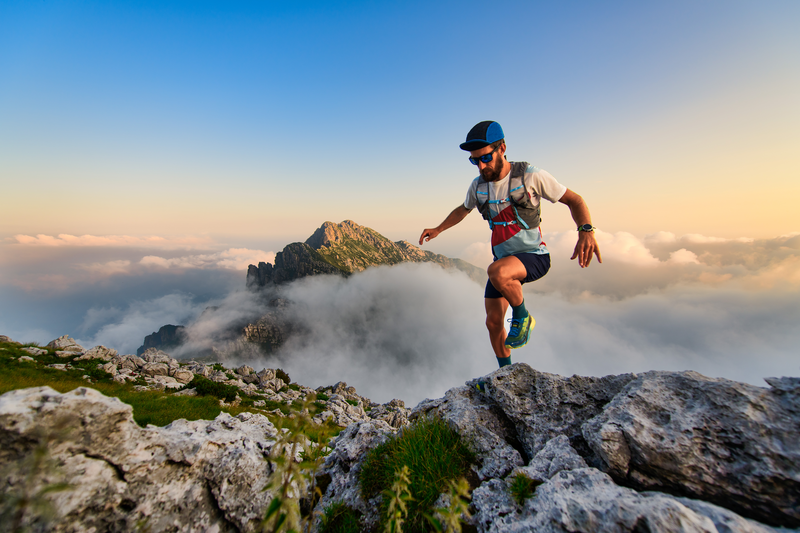
94% of researchers rate our articles as excellent or good
Learn more about the work of our research integrity team to safeguard the quality of each article we publish.
Find out more
ORIGINAL RESEARCH article
Front. Microbiol. , 02 October 2023
Sec. Microorganisms in Vertebrate Digestive Systems
Volume 14 - 2023 | https://doi.org/10.3389/fmicb.2023.1273709
Background: Helicobacter pylori infection and eradication have been reported to cause dysbiosis of the oral microbiota. Probiotics are increasingly being used to maintain the balance of the oral microbiota. We aimed to investigate the effects of H. pylori infection, H. pylori eradication with vonoprazan-amoxicillin dual therapy, and probiotics supplementation on the oral microbiota.
Methods: H. pylori positive patients were randomly assigned to a vonoprazan-amoxicillin regimen plus probiotics (BtT group) or the placebo (PT group) for 14 days. H. pylori negative population served as normal controls. Tongue coating samples were collected from 60 H. pylori positive patients at three time points (before H. pylori eradication, after H. pylori eradication, and at confirmation of H. pylori infection cure) and 20 H. pylori negative subjects. 16S rRNA gene sequencing was used to analyze the oral microbiota.
Results: H. pylori was detected in the oral cavity in positive (34/60), negative (7/20), and eradicated (1/60) subjects using high-throughput sequencing. Compared with normal controls, H. pylori positive patients exhibited higher richness (p = 0.012) and comparable diversity (p = 0.075) of oral microbiota. Beta diversity and KEGG analysis showed oral flora composition and function differences in H. pylori positive and negative subjects. Alpha diversity dramatically decreased after H. pylori eradication and modestly increased with confirmation of H. pylori eradication. Beta diversity and LEfSe analysis revealed distinct structures, and KEGG analysis showed distinct signaling pathways of tongue coating flora at three time points. There was a significant reduction of Firmicutes and Lactobacillus after H. pylori erdication. The PT group and BtT group had identical compositional and functional differences of oral microbiota at three time points.
Conclusion: No substantial link existed between oral and stomach H. pylori, while removing gastric H. pylori helped eliminate oral H. pylori. H. pylori infection and vonoprazan-amoxicillin dual therapy affected oral microbiota diversity, structure, and function. H. pylori eradication demonstrated a suppressive impact on the proliferation of oral pathogens, specifically Firmicutes and Lactobacillus. Nevertheless, probiotics supplementation did not reduce the oral microbial disturbance caused by H. pylori eradication.
Clinical trial registration: https://www.chictr.org.cn/, identifiers CHICTR2200060023.
Helicobacter pylori is widely acknowledged as a pathogenic microorganism capable of causing damage to the stomach and various other physiological systems. The Kyoto Global Consensus (Sugano et al., 2015) recommends that all people infected with H. pylori should receive eradication therapy unless there are competing considerations. The oral cavity is situated proximal to the human gastrointestinal tract and serves as a potential reservoir of H. pylori (Al et al., 2014). Several studies have identified the presence of H. pylori in the oral cavity, specifically in dental plaque, saliva, and other samples (Ansari et al., 2018; Matamala-Valdes et al., 2018). Dysbiosis of the oral microbiota, a significant constituent of the human microbiome, has been observed to contribute to the development of various oral and systemic ailments, such as oral squamous cell carcinoma, diabetes, inflammatory bowel disease, and cardiovascular disease (Peng et al., 2022).
There have been reports indicating that H. pylori infection and subsequent eradication can alter the oral microbiota. The oral microbial community exhibited variations in diversity and composition among persons with H. pylori infection compared to those without infection (Chua et al., 2019; Hu et al., 2022), as well as before and after eradication occurred. H. pylori eradication with a 7-day triple therapy results in an instantaneous decline in the diversity of the oral flora (Jakobsson et al., 2010; Kadota et al., 2019). And this decline is accompanied by a drop in the abundance of oral pathogenic bacteria, as well as Streptococcus and Actinomyces. The restoration of oral flora diversity is observed to occur gradually following the completion of treatment. Ji et al. (2020) suggested that the implementation of quadruple bismuth therapy for H. pylori eradication led to a reduction in the oral flora’s diversity and an augmentation in the abundance of the Fusobacteriota, Leptotrichia, Campylobacter, and Pseucomonas. The study conducted by Hu et al. found that H. pylori eradication with vonoprazan-amoxicillin dual therapy for 7–10 days did not have a significant impact on the diversity of the oral microbiota. However, it was observed that this treatment led to changes in the composition of the flora, characterized by a reduction in the abundance of Firmicutes and Bacteroidota and an increase in the abundance of Proteobacteria (Hu et al., 2022).
Probiotics are described as “live microorganisms which when administered in adequate amounts confer a health benefit on the host” (Hill et al., 2014) and have been gradually proved to maintain the balance of oral microecology (Nguyen et al., 2021). Can probiotics supplementation mitigate oral microbiota disruption caused by H. pylori eradication therapy? To date, no relevant studies have been conducted to ascertain the answer. Therefore, we conducted a randomized, double-blind, placebo-controlled trial to explore the connection between H. pylori infection and eradication and oral microbiota. Additionally, we assessed the impact of probiotics supplementation on oral microbiology.
In our study, H. pylori positive patients were assigned randomly to receive a vonoprazan-amoxicillin regimen together with probiotics or the placebo for 14 days. Tongue coating samples were collected from H. pylori positive patients at three time points (before H. pylori eradication, after H. pylori eradication, and at confirmation of H. pylori infection cure) and 20 H. pylori negative subjects. 16S rRNA gene sequencing was used to evaluate the structure and function of the samples.
The study was designed as a single-center, randomized, double-blind, placebo-controlled, and prospective clinical trial, adhering to the principles outlined in the Declaration of Helsinki, which obtained approval from the Medical Ethics Committee of the Nanjing First Hospital (KY20220314-05) and registered with the Chinese clinical trial registry (CHICTR2200060023).
From December 27, 2021 to June 22, 2022, a cohort of 100 H. pylori positive patients, ranging in age from 18 to 65, diagnosed by 13C-UBT (DOB > 6‰), histology, or culture, were recruited from Nanjing First Hospital in China. The main exclusion criteria were as follows: (1) allergy to the study drug; (2) patients with chronic gastritis with a confirmed peptic ulcer; (3) patients who had previously received H. pylori eradication therapy within 6 months; (4) use of antibiotics, bismuth, and probiotics within 4 weeks or H2 receptor antagonists, proton pump inhibitor (PPI), and potassium competitive acid blockers (P-CAB) within 2 weeks; (5) use of adrenocortical hormones, non-steroidal anti-inflammatory drugs, or anticoagulants; (6) history of esophageal or gastric surgery; (7) pregnant or lactating women; (8) suffer from a serious illness such as liver disease, cardiovascular disease, lung disease, kidney disease, or tumor; (9) alcoholism. Twenty H. pylori negative healthy volunteers (DOB < 4‰ of 13C-UBT) were selected as normal controls who had no history of smoking or alcohol consumption and oral disease.
The biostatisticians programmed the generation of the random allocation sequence for this clinical trial using the SAS 9.4 statistical analysis system and achieved it through the block randomization method with a block length of 4. Subjects meeting the enrollment criteria were randomly assigned to receive the vonoprazan-amoxicillin regimen along with probiotics in the BtT group or the placebo in the PT group for 14 days in a 1:1 ratio. The study medicine was administered to enrolled subjects based on their given randomization number, ensuring that both the subjects and the administering researchers remained blinded.
The BtT group was administered a regimen consisting of amoxicillin 750 mg qid, vonoprazan 20 mg bid, and Bifidobacterium tetravaccine tablets (3 tablets tid) for 14 days. The probiotics contained 0.5 g per tablet. The PT group received the identical vonoprazan-amoxicillin dual therapy in conjunction with the placebo, which was made of starch and had the same weight, appearance, and taste as Bifidobacterium tetravaccine tablets, except that it lacked the active ingredient. Both the probiotics and placebo were provided by Hangzhou Grand Biologic-Pharmaceutical, Inc. And the probiotics or placebo must be taken more than 2 h apart from amoxicillin.
Tongue coating samples were collected from H. pylori positive patients at three time points (before H. pylori eradication (W0), after H. pylori eradication (W2), and at confirmation of H. pylori infection cure (W6)) and H. pylori negative patients. Before the collection process, the oral cavity of each individual was washed with a normal saline solution two to three times. Sterile cotton swabs were utilized to collect samples from a specific region measuring 2 cm × 2 cm located in the central section of the dorsal surface of the tongue. Following 30 s of wiping, the samples were subsequently transferred into sterile test tubes and stored in a refrigerator set at a temperature of −80°C until they were deemed suitable for use.
Total genomic DNA was isolated using the CTAB technique. The concentration and purity of the DNA were evaluated on a 1% agarose gel. Specific primers (341F, CCTAYGGGRBGCASCAG, 806R, GGACTACNGGGTATTAAT) and barcodes were applied to amplify 16S genes from separate locations (V3-V4). All PCR reactions were performed using 15 μL of Phusion® High-Fidelity PCR Master Mix (New England Biolabs), 0.2 μM of forward and reverse primers, and roughly 10 ng of template DNA. The thermal cycle underwent initial denaturation at 98°C for 1 min, followed by denaturation at 98°C for 10 s, annealing at 50°C for 30 s, elongation at 72°C for 30 s, and finally elongation at 72°C for 5 min. The same amount of IX loading buffer (containing SYB green) was mixed with PCR products and detected by electrophoresis on a 2% agarose gel. The PCR products were mixed at an equal density ratio, then purified with the Qiagen Gel Extraction Kit (Qiagen, Germany). Sequencing libraries were generated using the TruSeq® DNA PCR-Free Sample Preparation Kit (Illumina, United States) following the manufacturer’s recommendations, and index codes were added. Finally, 250 bp paired-end reads were acquired by sequencing on the Illumina NovaSeq platform.
First, the technician screened the raw data and discarded any sequences that were shorter than 230 bp, had a quality score of ≤20, contained ambiguous bases, and did not precisely match the primer and barcode tag sequences, and then separated the sequences using sample-specific barcode tags. Then, the high-quality sequences were de-noised using the unoise3 method with usearch11 to produce amplicon sequence variants (ASVs; Edgar, 2016). Finally, all sequences were classified into different taxonomic groups against the SILVA138 database by the BLAST tool.
Qiime (v1.8.0) was used to calculate the richness and diversity indices (Chao1 richness index and Shannon diversity index) based on the ASV information. To compare the membership and structure of microbial communities in different samples, heat maps of the top 20 ASVs were generated using Mothur (Jami et al., 2013). According to the results of taxonomic annotation and relative abundance, PCoA based on bray-curtis distance and PLS-DA were performed using R packages (v3.6.0) to test the similarity between different samples (Wang et al., 2012). LEfSe was used to measure the unique species between H. pylori positive and negative groups, as well as before and after the H. pylori eradication. LDA value >3.0 was considered statistically significant. Microbial function was predicted by using PICRUST2 in the KEGG database.1 Co-occurrence network analysis was performed by the spearman method.
SPSS 25.0 statistical software was used to process the data, and the clinical data were compared by analysis of variance. Continuous data were presented as mean ± standard deviation. Disaggregated information is expressed as frequencies or percentages. Comparisons of measured information among groups were analyzed by one-way ANOVA, and categorical information was analyzed by Chi-squared test. When p < 0.05, the data exhibited statistical significance.
A total of 120 participants were included in the study, consisting of 100 H. pylori positive patients and 20 H. pylori negative individuals. Tongue coating samples were not collected from 40 H. pylori positive patients because of rejection or loss of follow-up. Finally, 60 H. pylori positive patients (referred to as the Hp group) who had undergone successful H. pylori eradication treatment, with 29 patients belonging to the BtT group and 31 patients belonging to the PT group, and 20 H. pylori negative individuals (referred to as the NT group) were selected for oral microbiota analysis (Figure 1).
Figure 1. Flow chart of the study. BtT group: vonoprazan-amoxicillin regimen plus probiotics. PT group: vonoprazan-amoxicillin regimen plus the placebo.
There were no significant differences among the BtT group, PT group, and NT group in demographic characteristics such as age, sex, BMI index, smoking, and alcohol (Supplementary Table S1).
H. pylori was detected in the oral cavity of infected subjects (34/60, 56.7%) and uninfected subjects (7/20, 35%) with no statistical differences (p > 0.05). The detection rate among eradicated subjects was 1.7% (1/60) by high-throught sequencing, significantly lower than the other two groups (p < 0.01).
The Chao1 richness index (Figure 2A) and Shannon diversity index (Figure 2B) were used to assess the alpha diversity of the tongue coating flora. The results indicated that the Hp group exhibited a higher richness compared to the NT group (Chao1 index: 1418.24 vs. 1006.72, p = 0.012). However, there was no statistically significant difference in diversity between the two groups (Shannon index: 4.15 vs. 3.91, p = 0.075). The PCoA analysis, using the bray-curtis distance metric (Figure 2C), revealed significant dissimilarity in community structure between the two groups (p = 0.001). In addition, the results obtained from PLS-DA revealed clear disparities in the taxonomic composition between the two groups, as depicted in Figure 2D.
Figure 2. Diversity of oral microbiota between H. pylori positive and negative subjects. The Hp group exhibited a higher richness compared to the NT group (A), while there was no significant difference in diversity (B). The PCoA analysis using the bray-curtis distance metric (C) and the PLS-DA results (D) revealed significant dissimilarity in community structure between the two groups. Hp: H. pylori positive. NT: H. pylori negative.
At the phylum level, the five dominant bacteria in the Hp group and the NT group both were Bacteroidota, Firmicutes, Proteobacteria, Fusobacteriota, and Actinobacteriota (Figure 3A), together accounting for more than 95% of the total sequences. Table 1 displayed the relative abundance of the predominant phyla (relative abundance ≥1% of the total sequences) in both groups. In comparison to the NT group, the Hp group exhibited a decrease in the relative abundance of Bacteroidota, Firmicutes, and Patescibacteria (35.2% vs. 32.9, 25.0% vs. 22.2%, and 1.8% vs. 1.1%, respectively). Conversely, the Hp group showed an increase in the relative abundance of Proteobacteria, Fusobacteriota, and Actinobacteriota (19.7% vs. 24.2%, 11.5% vs. 14.4%, and 3.9% vs. 4.0%, respectively). However, these differences were not statistically significant (p > 0.05). The only significant difference was observed in Campilobacterota, where the relative abundance was much lower in the Hp group compared to the NT group (0.6% vs. 2.5%, p = 0.00).
Figure 3. Composition of oral microbiota between H. pylori positive and negative subjects. The structure of oral microbiota between the two groups at the phylum level (A) and the genus level (B). Different specific oral bacteria assessed by LEfSe between the two groups (C). Hp: H. pylori positive. NT: H. pylori negative.
Table 1. Comparison of the relative abundance of dominant phyla (relative abundance ≥1% of the total sequences) between H. pylori positive and negative subjects.
As illustrated in Figure 3B, the top ten dominant genera within the NT group were identified as Prevotella, Neisseria, Veillonella, Fusobacterium, Streptococcus, Alloprevotella, Aggregatibacter, Leptotrichia, Campylobacter, and Actinomyces, accounting for 58.6% (top five dominant genera) and 81.7% (top ten dominant genera) of the total sequences. The top ten dominant genera within the Hp group were ranked as follows: Prevotella, Neisseria, Fusobacterium, Aggregatibacter, Veillonella, Streptococcus, Leptotrichia, Lactobacillus, Haemophilus, and Alloprevotella, accounting for 59.9% (top five dominant genera) and 79.0% (top ten dominant genera) of the total sequences. The comparison of the relative abundance of the top ten dominant genera between the two groups, as shown in Table 2, indicated that there were significant differences in the relative abundance of certain bacteria. Specifically, the relative abundance of Veillonella, Alloprevotella, and Campylobacter was significantly lower in the Hp group compared to the NT group (5.8% vs. 9.4, 2.9% vs. 7.6%, and 0.5% vs. 2.5%, respectively, p < 0.05). On the other hand, the relative abundance of Fusobacterium and Haemophilus was significantly higher in the Hp group compared to the NT group (9.9% vs. 7.8% and 3.0% vs. 2.0%, respectively, p < 0.05).
Table 2. Comparison of the relative abundance of the top ten dominant genera between H. pylori positive and negative subjects.
The LEfSe method was employed to identify distinct oral bacterial taxa present in both the Hp group and the NT group (Figure 3C). The potential biomarkers identified in this study encompassed Ruminococcaceae UCG-005, Rothia, Haemophilus, Porphyromonas, Fusobacterium, and Haemophilus of the Hp group, as well as Campilobacterota, Alloprevotella, Veillonella, Campylobacter, Megasphaera, Ralstonia, and Listeria of the NT group.
The results of the KEGG analysis (Supplementary Figure S1) demonstrated notable disparities in signaling pathways between the Hp group and the NT group. H. pylori positive subjects showed enriched signaling pathways associated with cardiovascular disease, bacterial infectious disease, and viral infectious disease. Conversely, the signaling pathway related to lipid metabolism showed a decrease in activity.
We successfully acquired 180 tongue coating samples from 29 subjects in the BtT group and 31 subjects in the PT group at three different time points: W0, W2, and W6. The Chao1 and Shannon indices were utilized to depict the richness and diversity of the two groups, as illustrated in Figures 4A,B,E,F, respectively. In the BtT group, there was a notable drop in the alpha diversity of tongue coating flora at W2 (Chao1 index: 1150.30 vs. 706.00, p = 0.00000051; Shannon index: 4.12 vs. 3.66, p = 0.000037). However, there was a little rise in alpha diversity at W6 (Chao1 index: 706.00 vs. 728.43, p = 0.096; Shannon index: 3.66 vs. 3.78, p = 0.036). The alpha diversity of tongue coating flora in the PT group exhibited a substantial decrease at W0 (Chao1 index: 1265.99 vs. 759.87, p = 0.0000000072; Shannon index: 4.11 vs. 3.66, p = 0.000044) and a slight increase at W6 (Chao1 index: 759.87 vs. 794.55, p = 0.019; Shannon index: 3.66 vs. 3.69, p = 0.48).
Figure 4. Diversity of oral microbiota at W0, W2, and W6 of the BtT group and the PT group. In the BtT group, the richness (A) and diversity (B) of tongue coating flora decreased significantly at W2 and slightly increased at W6. The PCoA analysis based on bray-curtis distance (C) and the PLS-DA results (D) revealed a clear difference in oral microbial structure in the BtT group at W0, W2, and W6. In the PT group, the richness (E) and diversity (F) of tongue coating flora also declined significantly at W0 and somewhat rose at W6. There was a distinct difference in oral microbial structure in the PT group at W0, W2, and W6 by the beta analysis of PCoA based on bray-curtis distance (G) and the PLS-DA results (H). BtT group: vonoprazan-amoxicillin regimen plus probiotics. PT group: vonoprazan-amoxicillin regimen plus the placebo. W0: before H. pylori eradication. W2: after H. pylori eradication. W6: at confirmation of H. pylori infection cure.
The beta analysis of PCoA, based on bray-curtis distance, demonstrated a distinct dissimilarity in the composition of oral microbiota between the two groups at W0, W2, and W6 (Figures 4C,G). According to the PLS-DA results, it was observed that the two groups exhibited distinctive clustering at W0, W2, and W6 (Figures 4D,H).
At the phylum level, the top five dominant bacteria of the BtT group (Figure 5A) and the PT group (Figure 5C) at W0, W2, and W6 all were Bacteroidota, Proteobacteria, Firmicutes, Fusobacteriota, and Actinobacteriota, together accounting for more than 97% of the total sequences. The relative abundance of the top five prevalent phyla at W0, W2, and W6 was compared and presented in Table 3 (BtT group) and Table 4 (PT group), which suggested a considerable drop in the relative abundance of Firmicutes at W2, followed by a slight increase at W6. There were no statistically significant differences in the relative abundance of the other four main phyla.
Figure 5. Composition of oral microbiota at W0, W2, and W6 of the BtT group and the PT group. Composition at the phylum level (A) and at the genus level (B) of the BtT group. Composition at the phylum level (C) and at the genus level (D) of the PT group. The distinct oral microorganisms of the BtT group (E) and the PT group (F) assessed by LEfSe analysis. BtT group: vonoprazan-amoxicillin regimen plus probiotics. PT group: vonoprazan-amoxicillin regimen plus the placebo. W0: before H. pylori eradication. W2: after H. pylori eradication. W6: at confirmation of H. pylori infection cure.
Table 3. Comparison of the relative abundance of the top five dominant phyla at W0, W2, and W6 of the BtT group.
Table 4. Comparison of the relative abundance of the five dominant phyla at W0, W2, and W6 of the PT group.
At the genus level, Prevotella, Neisseria, Fusobacterium, Aggregatibacter, and Veillonella were identified as the five dominant bacteria in both the BtT group (Figure 5B) and the PT group (Figure 5D) at W0, W2, and W6. The relative abundance of the top ten genera at W0, W2, and W6 was compared and presented in Table 5 (BtT group) and Table 6 (PT group). The results indicated that around 50% of the dominant genera exhibited similar levels of abundance throughout all three time points. Nevertheless, there were notable distinctions observed among Leptotrichia, Actinomyces, Lactobacillus, Porphyromonas, and Neisseria. Within the BtT group, there was a marginal rise in the relative abundance of Leptotrichia, Neisseria, and Actinomyces, which subsequently reverted back to the baseline level at W6. Following the therapy, there was a persistent drop in the relative abundance of Lactobacillus. Additionally, the relative abundance of Porphyromonas exhibited a modest decline at W2 but subsequently surpassed the baseline level at W6. In the PT group, the relative abundance of Leptotrichia slightly rose at W2 and decreased to the baseline level at W6. In addition, the abundance of Actinomyces exhibited a progressive increase following the therapy. In contrast, the relative abundance of Lactobacillus exhibited a notable decrease subsequent to the treatment, while the abundance of Neisseria experienced a minor increase at W2, followed by a subsequent decline below the starting level at W6.
Table 5. Comparison of the relative abundance of the ten dominant genera at W0, W2, and W6 of the PT group.
Table 6. Comparison of the relative abundance of the ten dominant genera at W0, W2, and W6 of the PT group.
We further performed co-occurrence network analysis to assess the impact of eradication treatment on oral microbiota interactions at W0 and W2 in the BtT group (Supplementary Figure S2) and in the PT group (Supplementary Figure S3). A negative association was seen between the abundance of Lactobacillus and the presence of Leptotrichia, Neisseria, and Actinomyces in both groups. This finding provided additional support for the previously identified variance in bacterial abundance.
The distinct oral microorganisms screened by LEfSe analysis at W0, W2, and W6 were shown in Figure 5E (BtT group) and Figure 5F (PT group). The potential biomarkers identified in the BtT group encompassed many taxonomic groups, including Firmicutes, Lactobacillus, Bacteroides, Romboutsia, and Rikenellaceae RC9 (at W0). At W2, the biomarkers consisted of Cyanobacteria, Neisseria, Leptotrichia, and Actinomyces. Finally, at W6, the biomarkers included Patescibacteria, Porphyromonas, TM7x, Absconditabacteriales SR1, and Moraxella. The potential biomarkers identified in the PT group were as follows: Firmicutes, Cyanobacteria, Lactobacillus, Bacteroides, Romboutsia, Rikenellaceae_RC9_gut_group, Chloroplast, Granulicatella, Ruminococcaceae UCG-005, Clostridia_UCG − 014, Parabacteroides, Prevotellaceae_Ga6A1_group, Muribaculaceae, Gemella (at W0), and Neisseria, Leptotrichia, Lachnoanaerobaculum (at W2), and Patescibacteria, Actinomyces, Eubacterium_nodatum_group, TM7x (at W6).
The KEGG analysis demonstrated statistically significant variations in the signaling pathways of 16, 12, and 10, respectively, between the time points W0 and W2 (Figure 6A), W2 and W6 (Figure 6B), and W0 and W6 (Figure 6C) in the BtT group. The PT group exhibited variations of 16, 18, and 23 routes at W0 and W2 (Figure 7A), W2 and W6 (Figure 7B), and W0 and W6 (Figure 7C), respectively. The dissimilarities in signaling pathways between W0 and W2 primarily included genetic information processing, environmental information processing, celluar process, human diseases, and metabolism. After H. pylori eradication, there was a decrease in the signaling pathways associated with genetic information processing (including folding and sorting and degradation), environmental information processing (including signal transduction and signaling molecules and interaction), cellular process (including cellular motility and cellular community), and human diseases (including cancer, viral infectious disease, and substance dependence). Conversely, the signaling pathways involved in metabolism (including metabolism of cofactors and vitamins) and human diseases (including drug resistance: antineoplastic, endocrine and metabolic disease, immune disease, and neurodegenerative disease) experienced a decrease. The aforementioned modifications were partially reverted to their original levels. Moreover, notable disparities were seen between 4 signaling pathways in the BtT group (Figure 6D) and 8 signaling pathways in the PT group (Figure 7D) at W0, W2, and W6.
Figure 6. The KEGG analysis before and after H. pylori eradication of the BtT group. There were significant variations in the signaling pathways of 16, 12, and 10, respectively, between the time points W0 and W2 (A), W2 and W6 (B), and W0 and W6 (C) in the BtT group. And there was a significant difference between 4 pathways in the BtT group at W0, W2, and W6 (D). BtT group: vonoprazan-amoxicillin regimen plus probiotics. W0: before H. pylori eradication. W2: after H. pylori eradication. W6: at confirmation of H. pylori infection cure.
Figure 7. The KEGG analysis before and after H. pylori eradication of the PT group. The PT group exhibited variations of 16, 18, and 23 routes at W0 and W2 (A), W2 and W6 (B), and W0 and W6 (C), respectively. And there was a significant difference between 8 pathways in the PT group at W0, W2, and W6 (D). PT group: vonoprazan-amoxicillin regimen plus the placebo. W0: before H. pylori eradication. W2: after H. pylori eradication. W6: at confirmation of H. pylori infection cure.
To our best knowledge, this is the first clinical trial to explore the influence of vonoprazan-amoxicillin dual therapy combined with probiotics for 14 days on the alteration of the oral microbiota. Simultaneously, we investigated the relationship between the existence of oral H. pylori and the status of H. pylori infection in the stomach, as well as the impact of H. pylori infection on the oral microbiota. The novel discoveries were articulated in the following manner.
H. pylori is a gram-negative bacteria that predominantly inhabits in the epithelial and mucus layers of the gastric mucosa and can be transmitted by both the oral-oral and fecal-oral routes, which establishes the oral cavity as a possible reservoir of H. pylori (Al et al., 2014). Previous studies have identified the presence of H. pylori in dental plaque (Mendoza-Cantu et al., 2017), saliva (Seligova et al., 2020), tongue coating (Zhao et al., 2019), and dental pulp (Nomura et al., 2018). Nevertheless, the association between oral H. pylori and gastric H. pylori is still not well understood. According to a study (Yee, 2016), there was a notable disparity in the detection rate of oral H. pylori between H. pylori positive patients and H. pylori negative people (80% vs. 23%), suggesting a potential association between gastric H. pylori and the occurrence of oral H. pylori infection. However, Ji et al. (2020) found no statistical differences in the detection of tongue coating H. pylori among infected, uninfected, and eradicated patients through high-throughput sequencing, which suggested that there is likely no substantial association between oral H. pylori and gastric H. pylori. In our study, we observed a greater incidence of tongue coating H. pylori among H. pylori positive patients compared to H. pylori negative subjects. Nevertheless, the observed disparity did not exhibit statistical significance (p > 0.05). It was worth mentioning that the presence of H. pylori on the tongue coating significantly exhibited a substantial decrease following H. pylori eradication (p < 0.01). The decrease in H. pylori presence may be explained by two factors. Firstly, the use of antibiotics to treat H. pylori in the gastric tract inadvertently leads to the elimination of H. pylori in the oral cavity as well (Bago et al., 2011). Secondly, it is possible that H. pylori in the oral cavity is either temporary or exists as nonviable bacterial fragments. The abundance or scarcity of H. pylori in the oral cavity is significantly impacted by its identified depth and specific location.
The consistency of the results on the impact of H. pylori infection on oral bacteria diversity is not entirely uniform, perhaps due to regional disparities, variances in sample sources, and discrepancies in sample sizes. Multiple studies have demonstrated that there are no significant disparities in the alpha and beta diversity of the oral microbial community between H. pylori positive and negative individuals (Li et al., 2021; Wu et al., 2021). Other research suggested that there are noticeable variations in the beta diversity of the salivary microbiota between H. pylori infected and uninfected subjects (Ji et al., 2020). In their study, Chua et al. (2019) conducted an investigation wherein oral cavity samples were obtained from a total of 24 subjects during daylight hours. The researchers observed that H. pylori positive patients exhibited a notably lower Shannon index compared to H. pylori negative population (p = 0.03). Furthermore, the study revealed the presence of distinct oral microbial community structures between the groups infected with H. pylori and those who were not infected. Otherwise, a study conducted by Hu et al. (2022), demonstrated that H. pylori positive patients had higher richness and diversity and better evenness of oral microbiota than normal controls. Furthermore, a significant distinction in clustering patterns was observed between the two groups (p < 0.05). The results of our study indicate that there was a higher richness and diversity of tongue coating microbiota in H. pylori infected patients compared to healthy controls (p < 0.05 and p > 0.05, respectively). Additionally, there were significant differences in the bacterial composition (p < 0.05). In a comprehensive study conducted by Yamashita and Takeshita (2017), sequencing techniques were employed to examine the composition of the salivary microbial community. The results of this investigation revealed a positive correlation between an elevated abundance of the salivary microbiota and compromised oral health, which suggests a potential impairment of oral health due to H. pylori infection, potentially mediated by an augmentation in the diversity of oral flora.
The study revealed that the two groups under investigation exhibited a constant presence of the five primary phyla, namely Bacteroidetes, Firmicutes, Proteobacteria, Fusobacteria, and Actinomycetes, in a specific order. This finding is in accordance with previous research (Ji et al., 2020; Hu et al., 2022). There were notable differences in the oral microbial makeup between the two groups. The results of the LEfSe analysis revealed that there were discernible taxonomic differences between the two groups, which is consistent with previous research findings (Ji et al., 2020; Hu et al., 2022). However, these findings contradict the results reported by Li et al. (2021). In our study, we observed significant enrichment of Fusobacterium and Haemophilus in H. pylori infected individuals. Fusobacterium is classified as a commensal oral bacterium; however, Fusobacterium nucleatum is hypothesized to be linked to the development of periodontal disease (Signat et al., 2011). Haemophilus is generally regarded as non-pathogenic to humans; nonetheless, there have been documented cases linking Haemophilus parainfluenzae to endocarditis (Revest et al., 2016) and pseudomembranous colitis (Liu Q. et al., 2022). Porphyromonas, together with Fusobacterium and Haemophilus, is recognized as a significant indicator of tongue coating microbiota in H. pylori infected individuals, as shown in our results. Ahn et al. (2012) have suggested that specific microorganisms found in the mouth cavity, such as Porphyromonas and Fusobacteriaceae, have the potential to disturb the microecology within the stomach and contribute to the development of chronic inflammation, which may serve as a potential precursor to gastric cancer. In a study conducted by Hu et al. (2015), notable reductions were observed in the relative prevalence of Porphyromonas, Fusobacterium, and Haemophilus among individuals diagnosed with stomach cancer. In contrast, Stasiewicz and Karpinski (2022) have established a notable correlation between Porphyromonas gingivalis, a widely recognized oral pathogen, and Fusobacterium nucleatum, with the occurrence of oral squamous cell carcinoma. There is a suggestion that infection with H. pylori could potentially influence the colonization of oral bacteria, hence potentially impacting the overall health of the oral cavity.
Through our analysis of the oral microbiota in H. pylori positive patients and healthy controls, we have identified variations in the expression of signaling pathways. H. pylori infection has the potential to influence the gene expression of tongue fur flora, which may establish a connection between H. pylori infection and the development of cardiovascular diseases and metabolic diseases such as lipid metabolism. Prior studies (Chen et al., 2020; Sun et al., 2023) have provided evidence of a correlation between H. pylori infection and metabolic conditions, including dyslipidemia, hypertension, diabetes, and cardiovascular diseases. Nevertheless, the precise mechanism remains uncertain and could potentially be associated with chronic inflammatory responses, endothelial damage, and hyperhomocysteinemia induced by H. pylori infection (Vijayvergiya, 2015). Potential areas of investigation in future research may involve examining the correlation between H. pylori infection and metabolic illnesses, focusing on a microecological perspective.
The precise mechanism underlying the impact of H. pylori infection on oral microbiota composition and metabolism has yet to be fully elucidated. Insufficient research has been conducted on this topic; however, it may be linked to modifications in intragastric acidity, virulence factors, cytokines, and the immunological response of the host. A previous study has shown that the use of PPI can have an impact on oral flora (Mishiro et al., 2018). It is hypothesized that H. pylori infection may lead to the production of significant quantities of urease, an enzyme responsible for the breakdown of urea into carbon dioxide, hence reducing intragastric acidity. On the other hand, the virulence factors associated with H. pylori, including CagA and VacA, have the ability to consistently trigger cellular inflammatory reactions, leading to a reduction in stomach acid output. The modification of gastric acid secretion has the potential to result in a reduction in oral PH, which can have implications for the composition of the oral microbial population. The impact of H. pylori infection on the intestinal flora is mediated by this particular mechanism, as observed in the study conducted by Iino and Shimoyama (2021). Furthermore, existing evidence suggests that the presence of H. pylori infection triggers the activation of cytokines inside the inflamed gastric mucosa. These cytokines, such as TNFα and IL-6, play a crucial role in the development of metabolic diseases (Buzas, 2014). Hence, we propose the presence of a link between H. pylori infection and alterations in the metabolic pathways of the oral microbiota. The association between H. pylori infection and changes in host immunity has been postulated (Cheok et al., 2022). Therefore, any modification in the immune response has the potential to destabilize the oral microbial ecology.
Following the successful H. pylori eradication, we observed a significant decrease in alpha diversity and a notable alteration in the composition of the oral microbiota. These differences did not fully revert to the baseline level at W6. Additionally, notable discrepancies were seen in the signaling pathways of the oral microbiota at W0, W2, and W6. In summary, H. pylori eradication with vonoprazan-amoxicillin regimen has been observed to lower the diversity of tongue coating flora and impact its structure and function. Despite undergoing therapy, the flora exhibited only partial recovery within a limited timeframe, failing to reach the levels observed before eradication. The use of bismuth-containing quadruple therapy, intended for H. pylori eradication, has been demonstrated to result in a reduction in alpha diversity and alterations in the composition of the salivary microbiota (Ji et al., 2020). Jakobsson et al. (2010) observed alterations in oral microbiota for a period of 4 years after H. pylori eradication with a 7-day triple therapy and found that the diversity decreased immediately after eradication, then gradually returned to the original level over time. H. pylori eradication regimen consists mainly of antibiotics and PPI. Previous studies have shown that the use of antibiotics (Abeles et al., 2015) and PPI (Mishiro et al., 2018) may have an impact on oral flora, aligning with the aforementioned discoveries. Alternatively, it seems that the magnitude of the impact is associated with the length of treatment time and amount of antibiotics and PPI administered. Hu et al. (2022) investigated the efficacy of low-dose amoxicillin (2.0 g daily) and high-dose amoxicillin (3.0 g daily) combined with vonoprazan for 7 or 10 days to eradicate H. pylori, then found the salivary flora diversity was not affected and the change in oral microbial community structure was minimal after eradication, which differs from our findings. The observed discrepancy could potentially be attributed to the prolonged length of therapy and the administration of a higher dosage of the medicine in our prescribed regimen.
There was a consistent drop in the relative abundance of Firmicutes and Lactobacillus following H. pylori treatment. The observed decline in the relative abundance of Firmicutes was found to be statistically significant, aligning with the findings reported by Hu et al. (2022). Periapical periodontitis is attributed to the presence of polymicrobial infections, characterized by the coexistence of many bacterial species. Among them, Firmicutes have been identified as the most prevalent and abundant (Korona-Glowniak et al., 2021). At the genus level, there was a notable drop in the relative abundance of Lactobacillus, a bacterium that has been associated with the progression of dental caries (Zhang and Xu, 2022). The eradication of H. pylori has the potential to impede the proliferation of oral infections.
A prior study (Pellicano et al., 2020) has found an association between H. pylori infection and the occurrence of type 2 diabetes mellitus, autoimmune diseases, neuropathy, and metabolism-related diseases. Moreover, some studies have reported the potential improvement of glycemic control in type 2 diabetes mellitus and symptom control in Parkinson’s disease with H. pylori eradication (Pierantozzi et al., 2006; Song et al., 2021). Nevertheless, there is still limited comprehension of the pathophysiology of these disorders, and the precise impact of H. pylori infection and its eradication remains uncertain. Further research is necessary to fully explore these relationships. The findings of our research suggest that the eradication of H. pylori can impact the expression of signaling pathways associated with the aforementioned diseases. This provides an opportunity to investigate the connection between H. pylori and metabolic disorders, immune disorders, and neurodegenerative conditions from a microecological standpoint.
The results of our study indicate that there were noticeable differences in the alpha diversity, compositional structure, and metabolic pathways of the tongue microbiome before and after the eradication of H. pylori. Furthermore, it can be observed that the level of variation was similar in both the groups receiving probiotics and receiving the placebo. This suggests that the simultaneous administration of probiotics did not provide any relief in terms of the disruption to the structure and function of the oral flora. Probiotics are bacteria that provide benefits to the host organism, and numerous studies have demonstrated the efficacy of co-administering probiotics in the eradication of H. pylori for the regulation of gastrointestinal flora (Kakiuchi et al., 2020; Yuan et al., 2021; He et al., 2022). Nevertheless, there remains a divergence of opinions regarding the precise function of probiotics in the context of oral microecology. The literature has demonstrated that probiotics possess the ability to disturb oral pathogenic biofilms and uphold a state of equilibrium within the oral microecology (Nguyen et al., 2021; Radaic et al., 2022). For instance, Bifidobacteria have the ability to impede the proliferation of Porphyromonas gingivalis (Jasberg et al., 2016). In a recent meta-analysis conducted by Liu J. et al. (2022), a total of 11 randomized controlled trials were examined to assess the effects of probiotics on oral flora. The findings of this analysis indicated that there was no significant difference observed in the levels of gingival inflammation and the presence of oral pathogens, such as Porphyromonas gingivalis, Prevotella intermedia, and Fusobacterium nucleatum, between the groups receiving probiotics and those receiving the placebo. Dassi et al. (2018) conducted a study to analyze the impact of probiotics on oral microbiota in healthy volunteers through 16S amplicon sequencing before, during, and after the administration of probiotics. The results showed an increase in the diversity of the salivary microbiota, but the overall structure remained unaltered. To far, there has been a scarcity of research investigating the impact of probiotics on oral flora during the H. pylori eradication. A randomized, placebo-controlled trial (He et al., 2022) investigated the impact of probiotics combined with bismuth quadruple therapy on the oral microbiota. The study revealed that there was no statistically significant disparity in the variety of salivary flora between the groups administered with probiotics and those given the placebo. However, it did notice a more restricted impact on the organization of the microbial community. The probiotics group showed a notable decrease in the prevalence of harmful microorganisms such as Porphyromonas, Actinobacillus, and Prevotella. Nevertheless, the group administered with probiotics in our study did not exhibit a reduced disruption in terms of diversity, structural composition, and function of the tongue flora, and we took into account the following factors: (1) The utilization of dual therapy in this study to eradicate H. pylori, which solely involved the use of one antibiotic, inherently resulted in a minor impact on the composition of oral flora (Hu et al., 2022). (2) The guidelines for the administration of Bifidobacterium bifidum tetrapartum tablets indicate that penicillin hampers the viability of live bacteria. Although, in our study, patients were instructed to take amoxicillin and the probiotics at least 2 h apart. (3) Probiotics gain entry into the gastrointestinal tract via the oral cavity, where the potential for colonization by probiotics may be limited. (4) The oral microbiota is significantly influenced by local oral hygiene practices, and this particular source of variability posed challenges in maintaining control during our clinical study.
In conclusion, the study determined that there was no substantial correlation between oral and stomach H. pylori. However, it was observed that eliminating gastric H. pylori seemed to enhance the elimination of oral H. pylori. H. pylori infection increased the richness of the oral microbiota and altered its composition. Following vonoprazan-amoxicillin eradication therapy, there was a notable reduction in the diversity of tongue flora and subsequent alterations in its composition, which could not be fully restored within a short period. The proliferation of oral pathogens (including Firmicutes and Lactobacillus) exhibited restricted growth subsequent to the H. pylori eradication. Additionally, H. pylori infection and eradication may have an impact on the metabolic pathways expressed in the tongue microbiota. These pathways are associated with various health conditions, such as cardiovascular disease, abnormal lipid metabolism, metabolic diseases, immune disorders, and neurodegenerative pathologies. However, the clinical importance of this phenomenon remains to be elucidated. The addition of probiotics did not result in a reduction of the disturbance to the oral microbiota produced by H. pylori eradication.
Firstly, our study did not consider the impact of diet, geography, and oral health status on oral microbiota. Secondly, this study is single-centered and has a small sample size, which may result in disparities with other studies. Larger studies are warranted to clarify these discrepancies. Additionally, we did not monitor long-term tongue flora changes following H. pylori eradication. In addition, it is unclear what clinical significance the effects of H. pylori infection and eradication have on oral microbiota signaling pathways, as shown in this study. Therefore, future studies should utilize larger sample sizes and longer follow-up times to further investigate the interactions between H. pylori infection, H. pylori eradication, and the oral microbiota.
The datasets analyzed for this study can be found in the National Center for Biotechnology Information (NCBI) (https://www.ncbi.nlm.nih.gov/) with the accession number PRJNA1001062.
The studies involving humans were approved by the Medical Ethics Committee of the Nanjing First Hospital (KY20220314-05). The studies were conducted in accordance with the local legislation and institutional requirements. The participants provided their written informed consent to participate in this study.
RP: Data curation, Formal analysis, Methodology, Writing – original draft. ZZ: Supervision, Writing – review & editing. YQ: Data curation, Writing – original draft. WC: Data curation, Writing – original draft.
The author(s) declare that no financial support was received for the research, authorship, and/or publication of this article.
We are grateful to Nanjing Fengzi Bio-pharmaceutical Technology Co., Ltd. for its assistance in sequencing analysis and charting, as well as to all the participants and medical staff involved in the study at Nanjing First Hospital.
The authors declare that the research was conducted in the absence of any commercial or financial relationships that could be construed as a potential conflict of interest.
All claims expressed in this article are solely those of the authors and do not necessarily represent those of their affiliated organizations, or those of the publisher, the editors and the reviewers. Any product that may be evaluated in this article, or claim that may be made by its manufacturer, is not guaranteed or endorsed by the publisher.
The supplementary material for this article can be found online at: https://www.frontiersin.org/articles/10.3389/fmicb.2023.1273709/full#supplementary-material
Abeles, S. R., Ly, M., Santiago-Rodriguez, T. M., and Pride, D. T. (2015). Effects of long term antibiotic therapy on human oral and fecal viromes. PLoS One 10:e134941. doi: 10.1371/journal.pone.0134941
Ahn, J., Chen, C. Y., and Hayes, R. B. (2012). Oral microbiome and oral and gastrointestinal cancer risk. Cancer Causes Control 23, 399–404. doi: 10.1007/s10552-011-9892-7
Al, S. A., Anand, P. S., Kamath, K. P., Patil, S., Preethanath, R. S., and Anil, S. (2014). Oral cavity as an extragastric reservoir of Helicobacter pylori. Isrn Gastroenterol. 2014:261369. doi: 10.1155/2014/261369
Ansari, S. A., Iqbal, M., Khan, T. A., and Kazmi, S. U. (2018). Association of oral Helicobacter pylori with gastric complications. Life Sci. 205, 125–130. doi: 10.1016/j.lfs.2018.05.026
Bago, I., Bago, J., Plecko, V., Aurer, A., Majstorovic, K., and Budimir, A. (2011). The effectiveness of systemic eradication therapy against oral Helicobacter pylori. J. Oral Pathol. Med. 40, 428–432. doi: 10.1111/j.1600-0714.2010.00989.x
Buzas, G. M. (2014). Metabolic consequences of Helicobacter pylori infection and eradication. World J. Gastroenterol. 20, 5226–5234. doi: 10.3748/wjg.v20.i18.5226
Chen, C., Zhang, C., Wang, X., Zhang, F., Zhang, Z., Ma, P., et al. (2020). Helicobacter pylori infection may increase the severity of nonalcoholic fatty liver disease via promoting liver function damage, glycometabolism, lipid metabolism, inflammatory reaction and metabolic syndrome. Eur. J. Gastroenterol. Hepatol. 32, 857–866. doi: 10.1097/MEG.0000000000001601
Cheok, Y. Y., Tan, G., Lee, C., Abdullah, S., Looi, C. Y., and Wong, W. F. (2022). Innate immunity crosstalk with Helicobacter pylori: pattern recognition receptors and cellular responses. Int. J. Mol. Sci. 23:7561. doi: 10.3390/ijms23147561
Chua, E. G., Chong, J. Y., Lamichhane, B., Webberley, K. M., Marshall, B. J., Wise, M. J., et al. (2019). Gastric Helicobacter pylori infection perturbs human oral microbiota. PeerJ 7:e6336. doi: 10.7717/peerj.6336
Dassi, E., Ferretti, P., Covello, G., Speccher, A., Migazzi, A., Bosco, B., et al. (2018). The short-term impact of probiotics consumption on the oral cavity microbiome. Sci. Rep. 8:10476. doi: 10.1038/s41598-018-28491-x
Edgar, R. C. (2016). Unoise2: Improved Error-Correction for Illumina 16s and Its Amplicon Sequencing. Cold Spring Harbor: Cold Spring Harbor Laboratory Press.
He, C., Xie, Y., Zhu, Y., Zhuang, K., Huo, L., Yu, Y., et al. (2022). Probiotics modulate gastrointestinal microbiota after Helicobacter pylori eradication: a multicenter randomized double-blind placebo-controlled trial. Front. Immunol. 13:1033063. doi: 10.3389/fimmu.2022.1033063
Hill, C., Guarner, F., Reid, G., Gibson, G. R., Merenstein, D. J., Pot, B., et al. (2014). Expert consensus document. The international scientific association for probiotics and prebiotics consensus statement on the scope and appropriate use of the term probiotics. Nat. Rev. Gastroenterol. Hepatol. 11, 506–514. doi: 10.1038/nrgastro.2014.66
Hu, J., Han, S., Chen, Y., and Ji, Z. (2015). Variations of tongue coating microbiota in patients with gastric cancer. Biomed. Res. Int. 2015:173729. doi: 10.1155/2015/173729
Hu, Y., Xu, X., Ouyang, Y. B., He, C., Li, N. S., Xie, C., et al. (2022). Analysis of oral microbiota alterations induced by Helicobacter pylori infection and vonoprazan-amoxicillin dual therapy for Helicobacter pylori eradication. Helicobacter 27:e12923. doi: 10.1111/hel.12923
Iino, C., and Shimoyama, T. (2021). Impact of Helicobacter pylori infection on gut microbiota. World J. Gastroenterol. 27, 6224–6230. doi: 10.3748/wjg.v27.i37.6224
Jakobsson, H. E., Jernberg, C., Andersson, A. F., Sjolund-Karlsson, M., Jansson, J. K., and Engstrand, L. (2010). Short-term antibiotic treatment has differing long-term impacts on the human throat and gut microbiome. PLoS One 5:e9836. doi: 10.1371/journal.pone.0009836
Jami, E., Israel, A., Kotser, A., and Mizrahi, I. (2013). Exploring the bovine rumen bacterial community from birth to adulthood. ISME J. 7, 1069–1079. doi: 10.1038/ismej.2013.2
Jasberg, H., Soderling, E., Endo, A., Beighton, D., and Haukioja, A. (2016). Bifidobacteria inhibit the growth of Porphyromonas gingivalis but not of Streptococcus mutans in an in vitro biofilm model. Eur. J. Oral Sci. 124, 251–258. doi: 10.1111/eos.12266
Ji, Y., Liang, X., and Lu, H. (2020). Analysis of by high-throughput sequencing: Helicobacter pylori infection and salivary microbiome. BMC Oral Health 20:84. doi: 10.1186/s12903-020-01070-1
Kadota, T., Ogaya, Y., Hatakeyama, R., Nomura, R., and Nakano, K. (2019). Comparison of oral flora before and after triple therapy for Helicobacter pylori eradication in patient with gastric disease. Odontology 107, 261–267. doi: 10.1007/s10266-018-0393-y
Kakiuchi, T., Mizoe, A., Yamamoto, K., Imamura, I., Hashiguchi, K., Kawakubo, H., et al. (2020). Effect of probiotics during vonoprazan-containing triple therapy on gut microbiota in Helicobacter pylori infection: a randomized controlled trial. Helicobacter 25:e12690. doi: 10.1111/hel.12690
Korona-Glowniak, I., Piatek, D., Fornal, E., Lukowiak, A., Gerasymchuk, Y., Kedziora, A., et al. (2021). Patterns of oral microbiota in patients with apical periodontitis. J. Clin. Med. 10:2707. doi: 10.3390/jcm10122707
Li, S., Zhang, Y., Yang, Z., Li, J., Li, Y., Li, H., et al. (2021). Helicobacter pylori infection is correlated with the incidence of erosive oral lichen planus and the alteration of the oral microbiome composition. BMC Microbiol. 21:122. doi: 10.1186/s12866-021-02188-0
Liu, Q., Jiang, Z., Teng, Q., and Jiang, M. (2022). Pseudomembranous colitis caused by Haemophilus parainfluenzae. Inflamm. Bowel Dis. 28, e55–e56. doi: 10.1093/ibd/izab293
Liu, J., Liu, Z., Huang, J., and Tao, R. (2022). Effect of probiotics on gingival inflammation and oral microbiota: a meta-analysis. Oral Dis. 28, 1058–1067. doi: 10.1111/odi.13861
Matamala-Valdes, L., Sanchez-Alonzo, K., Parra, C., Saez, K., Aguayo-Reyes, A., and Garcia, A. (2018). Detection of intracellular Helicobacter pylori in candida. Spp from neonate oral swabs. Rev. Assoc. Med. Brassica 64, 928–935. doi: 10.1590/1806-9282.64.10.928
Mendoza-Cantu, A., Urrutia-Baca, V. H., Urbina-Rios, C. S., De la Garza-Ramos, M. A., Garcia-Martinez, M. E., and Torre-Martinez, H. (2017). Prevalence of Helicobacter pylori vaca genotypes and caga gene in dental plaque of asymptomatic mexican children. Biomed. Res. Int. 2017:4923640. doi: 10.1155/2017/4923640
Mishiro, T., Oka, K., Kuroki, Y., Takahashi, M., Tatsumi, K., Saitoh, T., et al. (2018). Oral microbiome alterations of healthy volunteers with proton pump inhibitor. J. Gastroenterol. Hepatol. 33, 1059–1066. doi: 10.1111/jgh.14040
Nguyen, T., Brody, H., Radaic, A., and Kapila, Y. (2021). Probiotics for periodontal health-current molecular findings. Periodontol. 87, 254–267. doi: 10.1111/prd.12382
Nomura, R., Ogaya, Y., Matayoshi, S., Morita, Y., and Nakano, K. (2018). Molecular and clinical analyses of Helicobacter pylori colonization in inflamed dental pulp. BMC Oral Health 18:64. doi: 10.1186/s12903-018-0526-2
Pellicano, R., Ianiro, G., Fagoonee, S., Settanni, C. R., and Gasbarrini, A. (2020). Review: extragastric diseases and Helicobacter pylori. Helicobacter 25:e12741. doi: 10.1111/hel.12741
Peng, X., Cheng, L., You, Y., Tang, C., Ren, B., Li, Y., et al. (2022). Oral microbiota in human systematic diseases. Int. J. Oral Sci. 14:14. doi: 10.1038/s41368-022-00163-7
Pierantozzi, M., Pietroiusti, A., Brusa, L., Galati, S., Stefani, A., Lunardi, G., et al. (2006). Helicobacter pylori eradication and l-dopa absorption in patients with pd and motor fluctuations. Neurology 66, 1824–1829. doi: 10.1212/01.wnl.0000221672.01272.ba
Radaic, A., Brody, H., Contreras, F., Hajfathalian, M., Lucido, L., Kamarajan, P., et al. (2022). Nisin and nisin probiotics disrupt oral pathogenic biofilms and restore their microbiome composition towards healthy control levels in a peri-implantitis setting. Microorganisms 10:1336. doi: 10.3390/microorganisms10071336
Revest, M., Egmann, G., Cattoir, V., and Tattevin, P. (2016). Hacek endocarditis: state-of-the-art. Expert Rev. Anti-Infect. Ther. 14, 523–530. doi: 10.1586/14787210.2016.1164032
Seligova, B., Lukac, L., Babelova, M., Vavrova, S., and Sulo, P. (2020). Diagnostic reliability of nested pcr depends on the primer design and threshold abundance of Helicobacter pylori in biopsy, stool, and saliva samples. Helicobacter 25:e12680. doi: 10.1111/hel.12680
Signat, B., Roques, C., Poulet, P., and Duffaut, D. (2011). Fusobacterium nucleatum in periodontal health and disease. Curr. Issues Mol. Biol. 13, 25–36. doi: 10.21775/cimb.013.025
Song, X., Cai, C., Jin, Q., Chen, X., and Yu, C. (2021). The efficacy of Helicobacter pylori eradication in diabetics and its effect on glycemic control: a systematic review and meta-analysis. Helicobacter 26:e12781. doi: 10.1111/hel.12781
Stasiewicz, M., and Karpinski, T. M. (2022). The oral microbiota and its role in carcinogenesis. Semin. Cancer Biol. 86, 633–642. doi: 10.1016/j.semcancer.2021.11.002
Sugano, K., Tack, J., Kuipers, E. J., Graham, D. Y., el-Omar, E. M., Miura, S., et al. (2015). Kyoto global consensus report on Helicobacter pylori gastritis. Gut 64, 1353–1367. doi: 10.1136/gutjnl-2015-309252
Sun, L., Zheng, H., Qiu, M., Hao, S., Liu, X., Zhu, X., et al. (2023). Helicobacter pylori infection and risk of cardiovascular disease. Helicobacter 28:e12967. doi: 10.1111/hel.12967
Vijayvergiya, R. (2015). Role of Helicobacter pylori infection in pathogenesis of atherosclerosis. World J. Cardiol. 7, 134–143. doi: 10.4330/wjc.v7.i3.134
Wang, Y., Sheng, H. F., He, Y., Wu, J. Y., Jiang, Y. X., Tam, N. F., et al. (2012). Comparison of the levels of bacterial diversity in freshwater, intertidal wetland, and marine sediments by using millions of illumina tags. Appl. Environ. Microbiol. 78, 8264–8271. doi: 10.1128/AEM.01821-12
Wu, Z. F., Zou, K., Xiang, C. J., Jin, Z. J., Ding, H. H., Xu, S., et al. (2021). Helicobacter pylori infection is associated with the co-occurrence of bacteria in the oral cavity and the gastric mucosa. Helicobacter 26:e12786. doi: 10.1111/hel.12786
Yamashita, Y., and Takeshita, T. (2017). The oral microbiome and human health. J. Oral Sci. 59, 201–206. doi: 10.2334/josnusd.16-0856
Yee, J. K. (2016). Helicobacter pylori colonization of the oral cavity: a milestone discovery. World J. Gastroenterol. 22, 641–648. doi: 10.3748/wjg.v22.i2.641
Yuan, Z., Xiao, S., Li, S., Suo, B., Wang, Y., Meng, L., et al. (2021). The impact of Helicobacter pylori infection, eradication therapy, and probiotics intervention on gastric microbiota in young adults. Helicobacter 26:e12848. doi: 10.1111/hel.12848
Zhang, J., and Xu, X. (2022). Research progress in the relationship between lactobacillus and dental caries. Sichuan Da Xue Xue Bao 53, 929–934. doi: 10.12182/20220960103
Zhao, Y., Gao, X., Guo, J., Yu, D., Xiao, Y., Wang, H., et al. (2019). Helicobacter pylori infection alters gastric and tongue coating microbial communities. Helicobacter 24:e12567. doi: 10.1111/hel.12567
Keywords: Helicobacter pylori, eradication, dual therapy, probiotics, oral microbiota
Citation: Peng R, Zhang Z, Qu Y and Chen W (2023) The impact of Helicobacter pylori eradication with vonoprazan-amoxicillin dual therapy combined with probiotics on oral microbiota: a randomized double-blind placebo-controlled trial. Front. Microbiol. 14:1273709. doi: 10.3389/fmicb.2023.1273709
Received: 08 August 2023; Accepted: 11 September 2023;
Published: 02 October 2023.
Edited by:
Edoardo Pasolli, University of Naples Federico II, ItalyReviewed by:
Zhekai Hu, University of Rochester Medical Center, United StatesCopyright © 2023 Peng, Zhang, Qu and Chen. This is an open-access article distributed under the terms of the Creative Commons Attribution License (CC BY). The use, distribution or reproduction in other forums is permitted, provided the original author(s) and the copyright owner(s) are credited and that the original publication in this journal is cited, in accordance with accepted academic practice. No use, distribution or reproduction is permitted which does not comply with these terms.
*Correspondence: Zhenyu Zhang, enp5NjU2NUBzaW5hLmNvbQ==
Disclaimer: All claims expressed in this article are solely those of the authors and do not necessarily represent those of their affiliated organizations, or those of the publisher, the editors and the reviewers. Any product that may be evaluated in this article or claim that may be made by its manufacturer is not guaranteed or endorsed by the publisher.
Research integrity at Frontiers
Learn more about the work of our research integrity team to safeguard the quality of each article we publish.