- College of Animal Science and Technology, Hebei Agricultural University, Baoding, China
The early colonized gut microbiota during the newborn period has been reported to play important roles in the health and immunity of animals; however, whether they can affect the growth performance of suckling lambs is still unclear. In this study, a total of 84 newborn lambs were assigned into LF-1 (top 15%), LF-2 (medium 70%), and LF-3 (bottom 15%) groups according to their average body weight gain at 30 days of age. Fecal samples of lambs (LF) as well as feces (MF), vagina (VAG), colostrum (COL), teat skin (TEAT) samples of ewes, and the air sediment (AIR) in the delivery room were collected 72 h after birth, and then the 16S rRNA gene was sequenced on the Illumina MiSeq platform. The results showed that the early colonized gut microbiota had a significant effect on the growth performance of suckling lambs with alpha and beta diversity (p < 0.05), and we observed that the contribution of early colonized bacteria on the growth performance of lambs increased with age (from BW30 at 25.35% to BW45 at 31.10%; from ADG30 at 33.02% to ADG45 at 39.79% by measuring the relative effects of factors that influence growth performance). The early colonized gut microbiota of suckling lambs with high growth performance was similar to that in VAG, MF, and AIR (p < 0.05). With the RandomForest machine learning algorithm, we detected 11, 11, 6, and 4 bacterial taxa at the genus level that were associated with BW30, BW45, ADG30, and ADG45 of suckling lambs, respectively, and the correlation analysis showed that Butyricicoccus, Ruminococcus_gnavus_group, Ruminococcaceae_Other, and Fusobacterium could significantly affect the growth performance (BW30, BW45, ADG30, and ADG45) of suckling lambs (p < 0.05). In conclusion, the early colonized gut microbiota could significantly affect the growth performance of suckling lambs, and targeting the early colonized gut microbiota might be an alternative strategy to improve the growth performance of suckling lambs.
1. Introduction
The suckling period is the fastest-growing stage of lambs, and it is also the key period for the colonization of gut microbiota in lambs (Lin et al., 2019; Dogra et al., 2021). The disorder of the early colonized gut microbiota in newborn lambs made them vulnerable to various diseases, which led to an increase in mortality and restricted the benefit of lamb raising (Bi et al., 2019; Chen C. et al., 2021; Chen X. et al., 2021; Cristofori et al., 2021). Understanding the effect of the early colonized microbiota in the gut on the growth performance of suckling lambs could provide fundamental concepts for alternative strategies to improve the growth performance of suckling lambs.
Current research indicated that although there have been different views on whether the initiation of microbial colonization begins in utero or after birth (Perez-Munoz et al., 2017), the consensus was that the newborn period is a key period for microbial colonization (Collado et al., 2012). In the suckling period, microorganisms from the mother and the environment were rapidly colonized after birth until a stable microbiota was formed in the gastrointestinal tract after weaning (Jami et al., 2013; Yeoman et al., 2018). Could the early colonized bacteria affect the growth performance of animals was still unclear. Interestingly, some studies have observed some correlations between microbial communities and growth performance (Peled et al., 2016; Noor et al., 2021; Peng et al., 2021). They provided evidence that the yaks with high growth performance had lower microbial richness and higher microbial diversity (Huang et al., 2021), and supplementation of Bacillus subtilis or Lactobacillus rhamnosus in feed could improve the growth performance of calves (Kim et al., 2018; Zhang et al., 2019), while we also observed that rumen fluid transplantation in weaned lambs caused changes in the gut microbial community, average daily feed intake, and average daily gain (ADG) in our previous study (Yin et al., 2021).
Herein, we hypothesized that the early colonized gut microbiota might affect the growth performance of suckling lambs. This study focuses on the effect of the early colonized gut microbiota on the growth performance of suckling lambs, explores the source of early colonized gut microbial colonization, and detects the bacterial taxa that might affect the growth performance of suckling lambs using the RandomForest model. These findings might be helpful in enhancing the growth performance of suckling lambs by targeting the early colonized gut microbiota.
2. Materials and methods
2.1. Ethics statement
The experiments were conducted following the Chinese Animal Welfare Guidelines and experimental protocols. All procedures used in this study were approved by the Animal Care and Use Committee of Hebei Agricultural University (ID: 2020004).
2.2. Experimental design and feeding management
The study was conducted between August and October 2020 at Lanhai Animal Husbandry Co., Ltd. (Zhangjiakou, China). We selected 40 ewes with a similar expected delivery time and achieved 84 newborn Hu lambs.
The delivery rooms were thoroughly cleaned and disinfected 1 week before the start of the experiment. The ewes were moved to the delivery rooms for single-pen feeding 14 days before the expected delivery time. After birth, the umbilical cord of lambs was disinfected with iodophor, the body was wiped with gauze and ear-tagged, and then the litter size and sex were recorded. The lambs were raised in the same pen (1.8 m × 2 m) with the ewes and got free access to the starter and clean water after 7 days of birth.
2.3. Growth performance of suckling lambs
The body weight of lambs was measured within 2 h after birth and before morning feeding at 30 and 45 days of age.
The ADG was calculated as follows:
where ADGi is the average daily gain from birth to ith days of age, BWi is the body weight at ith days of age, and BW0 is the birth weight.
The growth coefficient (GI) of lambs was calculated as:
where GIi is the growth coefficient from birth to ith days of age, BWi is the weight at ith days of age, and BW0 is the birth weight.
The body height, body length, and chest circumference of lambs were measured 30 and 45 days after birth, according to a previous report (Li et al., 2022).
Subsequently, the suckling lambs were divided into LF-1 (top 15% lambs ranked according to the ADG30), LF-2 (medium 70% lambs ranked according to the ADG30), and LF-3 (bottom 15% lambs ranked according to the ADG30) groups.
2.4. Samples collection and measurement
Blood samples of the lambs were collected at 24 h after birth from the jugular vein and transferred into 5 mL coagulation-promoting tubes, and the serum was collected by centrifugation at 3,500 × g for 15 min at room temperature and then frozen at −20°C. The IgG content in the serum was determined using single radial immunodiffusion in the laboratory (Martin et al., 2021). Sheep IgG was purchased from Beijing Biolab Technology Co., Ltd. (Beijing, China) (SP038-10 mg), and rabbit anti-goat IgG antibody was purchased from Beijing Biolab Technology Co., Ltd. (Beijing, China), with a titer of 1:16–32. We classified the lambs into two groups with 24-h serum IgG levels of IgG ≥25 and < 25 mg/mL, according to the criteria for successful passive immunization (Chigerwe et al., 2015). Fecal samples of the lambs were collected from 84 lambs with sterile swabs 72 h after birth and transferred to sterile tubes to be stored at −80°C for subsequent analyses.
For the enrolled ewes in the current experiment, 10 individuals were randomly selected, and vaginal samples were obtained using a sterile swab inserted approximately 8 cm into the vagina and rotated three times. The sterile swab was carefully removed from the vagina, ensuring it did not touch the vulva, and the swab was quickly placed into a 15-ml sterile tube with 10 mL of phosphate-buffered saline (PBS) and stored at −80°C for subsequent testing. Teat skin samples of the ewes were collected with a sterile swab within 1 h after birth before the lambs were breastfed. A sterile swab moistened with saline was used to wipe the teat skin area, and then the tip of the swab was collected and placed into a 5-ml sterile tube and stored at −80°C for subsequent testing. Before colostrum collection at approximately 1 h after birth, teats of the ewes were wiped and cleaned with alcohol containing sterile gauze, and the first 3 mL of colostrum was discarded. Then, 5 mL of colostrum was collected manually, transferred into a sterile tube, and stored at −80°C until testing. Fecal samples of the ewes were collected from the rectum and were transferred into sterile tubes and stored at −80°C for subsequent analyses.
To collect samples of microbiota in air, six sampling points were arranged in the front, middle, and rear sections of the pens, and the samples were collected using the natural sedimentation method following a previous publication (Zhang et al., 2022). Each Petri dish was exposed to air for 30 min, then a sterile swab was used to dip the surface of the Petri dish, transferred into a cryogenic storage tube, and stored at −80°C for testing.
2.5. 16S rRNA gene high-throughput sequencing and analysis
DNA was extracted from collected samples using the PowerSoil DNA Isolation Kit (Allwegene Tech, Beijing, China) according to the manufacturer’s instructions. The concentration and purity of the DNA were determined using a NanoDrop 2000 spectrophotometer (Thermo Fisher Scientific, Inc., United States). The V3–V4 region of the bacterial 16S rRNA gene was amplified with primers 338F (5’-ACTCCTACGGGAGGCAGCAG-3′) and 806R (5’-GGACTACHVGGGTWTCTAAT-3′) on an ABI 9700 PCR instrument (Applied Biosystems, Inc., United States). PCR reactions were performed in a 25-μl mixture containing 30 ng template DNA, 1 μL forward primer (5 μM), 1 μL reverse primer (5 μM), 3 μL BSA (2 ng/μl), 12.5 μL 2× Taq PCR MasterMix, and 7.5 μL ddH2O. The PCR amplification program was 95°C for 5 min, followed by 30 cycles of 94°C for 30 s, 50°C for 30 s, and 72°C for 60 s with a final extension at 72°C for 10 min. PCR products were extracted from 1% agarose gel and purified using the Agencourt AMPure XP (Beckman Coulter, Inc., United States) nucleic acid purification kit. The extracted DNA from fecal samples of 10 lambs was of low quality and had been removed from the experiment, and 74 fecal samples of lambs were subjected to bacterial community detection with high-throughput sequencing.
Deep sequencing of DNA extracts was performed on the Illumina MiSeq platform (Illumina, Inc., United States). The raw reads were split by QIIME (v1.8.0), the sequencing data were filtered and spliced using the Pear software (v0.9.6), and the sequences were filtered to remove chimeras using the Vsearch software (v2.7.1). After OTUs were clustered with high-quality sequences using the Vsearch software (v2.7.1) with a sequence similarity threshold of 97%, the representative sequences were compared with the Silva138 database to obtain the taxonomic information of the species corresponding to each OTU. Diversity indices, including Chao1 and Shannon indices, were calculated using the QIIME software (v1.8.0), and the principal coordinate analysis (PCoA) based on Jaccard distance was performed by the R software (v4.1.2).
2.6. Statistical analysis
Experimental data were analyzed using one-way ANOVA or Student’s t-test of the SPSS software v.22.0 (SPSS Inc., Chicago, IL, United States), and then multiple comparisons were performed using the LSD method. The models between growth performance and microorganisms were constructed using the RandomForest package in the R software (v4.1.2), and signature bacteria were screened by increasing mean squared error (%IncMSE) and cross-validation. Figures were generated with the GraphPad Prism 8.0.2 (GraphPad Software, Inc., San Diego, CA, United States) or R (v4.1.2) software. Data were presented as the mean ± standard deviation (SD), and p < 0.05 was considered a significant difference.
3. Results
3.1. Effect of serum IgG content, birth weight, litter size, and sex on growth performance of suckling lambs
The serum IgG content had no significant effect on the growth performance (BW0, BW30, BW45, ADG30, and ADG45) of suckling lambs (p > 0.05), but the growth performance of suckling lambs could be significantly affected by the birth weight and sex of lambs and the litter size of the ewes (p < 0.05). We observed that the growth performance of suckling lambs with a birth weight > 4 kg was significantly higher than that of lambs with a birth weight of 3–4 kg or < 3 kg (p < 0.05), and the growth performance of single lambs was significantly higher than that of twins and triple lambs (p < 0.05). Male lambs had significantly higher BW0, BW30, and ADG30 than female lambs (p < 0.05) (Table 1). Meanwhile, the effects of these factors on GI30, GI45, and body size parameters were similar to the growth performance indices of BW0, BW30, BW45, ADG30, and ADG45 (Supplementary Tables S1, S2).
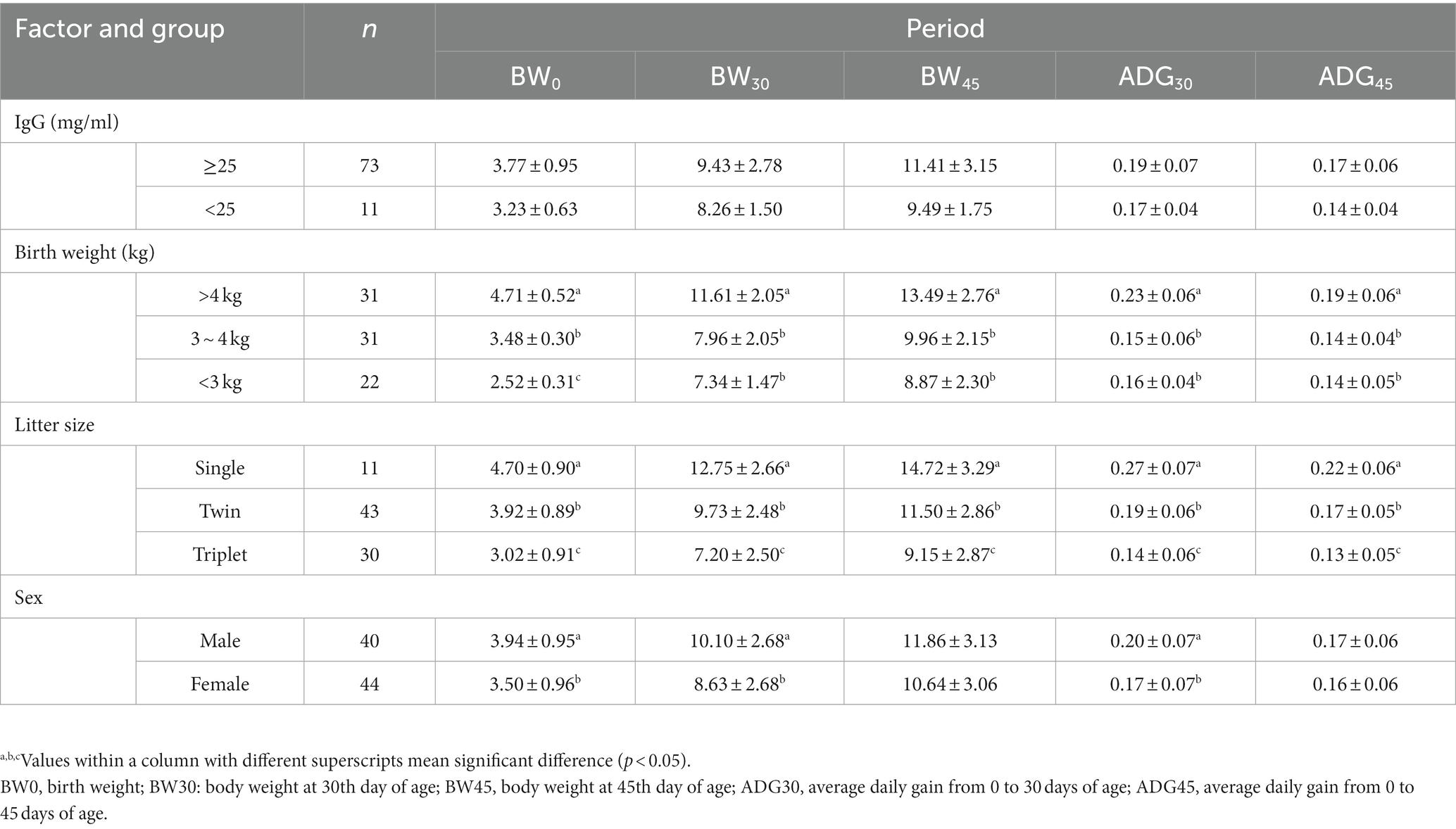
Table 1. Effect of serum IgG content, birth weight, litter size, and sex on growth performance of suckling lambs.
3.2. Comparison of gut microbiota in suckling lambs with different growth performance
We assigned lambs into three groups (LF-1, top 15%; LF-2, medium 70%; LF-3, bottom 15%) based on the average body weight gain 30 days after birth. Our results indicated no significant difference in the Chao1 index between the groups (p > 0.05) (Figure 1A). However, the Shannon index of the LF-1 group was significantly higher than that of the LF-2 and LF-3 groups (p < 0.05) (Figure 1B). A PCoA analysis based on Jaccard distance revealed a significant difference in the gut microbiota composition between the three groups (ANOSIM, R = 0.2, p < 0.05) (Figure 1C), and the within-group distance of the LF-1 group was significantly higher than that of the LF-2 and LF-3 groups (Figure 1D). These results suggested that lambs with different growth performances might have different gut microbiota compositions in early life, and the gut microbiota of suckling lambs with higher growth performance had high inter-individual variability.
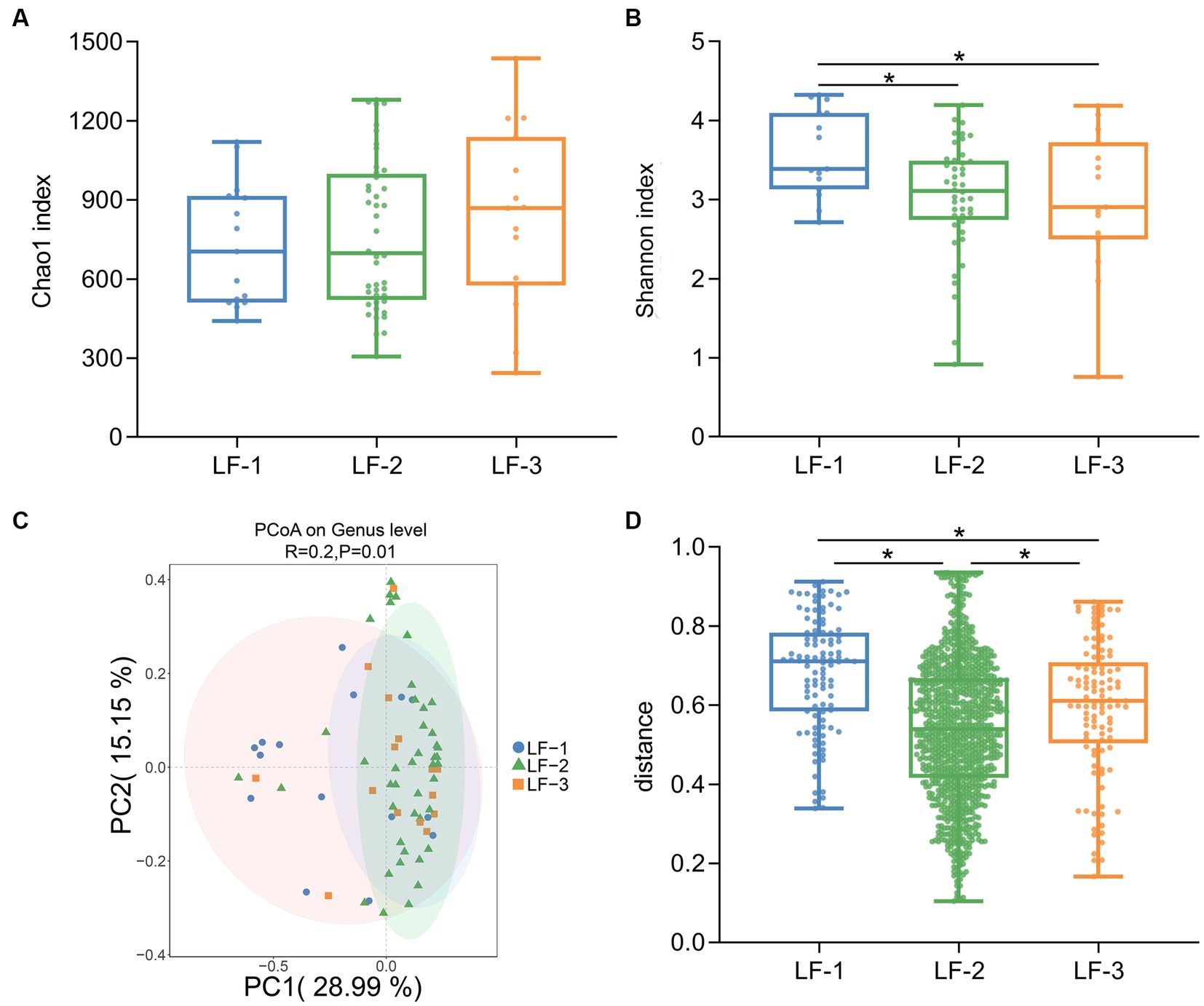
Figure 1. Diversity analysis of the gut bacterial composition of newborn lambs with different growth performance. Chao1 index (A) and Shannon index (B) of gut microbiota among LF-1, LF-2, and LF-3 groups. (C) Principal coordinate analysis (PCoA) of gut microbiota in the suckling lambs on genus level (based on the Jaccard distance). (D) Inner-group distance of gut microbiota in LF-1, LF-2, and LF-3 groups. The suckling lambs were divided into LF-1 (top 15%), LF-2 (medium 70%), and LF-3 (bottom 15%) groups according to ADG30 of lambs. * indicated significant differences among groups (p < 0.05).
3.3. The contribution of multiple factors to the growth performance of suckling lambs
To evaluate the contribution of multiple factors affecting the growth performance of suckling lambs, we fitted a multiple linear regression model with growth performance, with the independent variables including gut microbiota, sex, birth weight, IgG content in the serum of suckling lambs, and litter size of the ewes. We observed that these factors could explain 66.42% of the variation of BW30, 54.33% of the variation of BW45, 43.16% of the variation of ADG30, and 30.73% of the variation of ADG45 for suckling lambs. The gut microbiota, birth weight of lambs, and litter size of ewes have been identified as the top three influencing factors on the growth performance of suckling lambs. Among them, the contribution of early colonized gut microbiota on the growth performance of lambs increased with age (from BW30 at 25.35% to BW45 at 31.10%; from ADG30 at 33.02% to ADG45 at 39.79%) (Figure 2). We also observed a similar result for the GI30 and GI45 indices, and the contribution of gut microbiota to the growth index increased from GI30 with 32.79% to GI45 with 35.94% with age increase (Supplementary Figure S1). These results indicated that the early colonized microbiota played important roles in the growth performance of suckling lambs, and their effect on growth performance might increase with age.
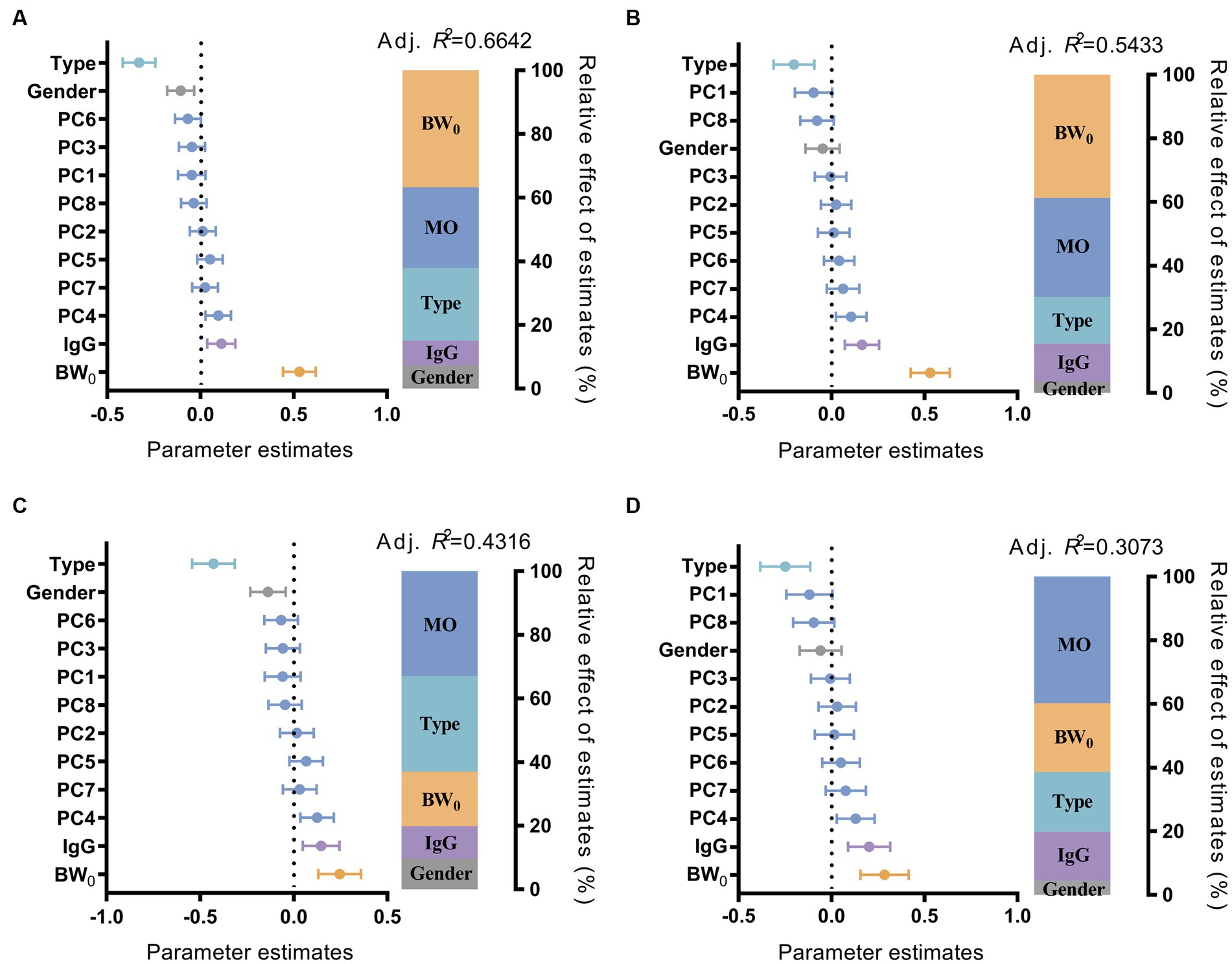
Figure 2. Effect of multiple factors on the growth performance of suckling lambs. Parameter estimates (standardized regression coefficients) of multiple linear regression model on BW30 (A), BW45 (B), ADG30 (C), and ADG45 (D) of suckling lamb. Type: Litter size of ewe; Sex: sex of suckling lambs; IgG: IgG content in 24 h serum of suckling lambs; BW0: birth weight of suckling lambs; MO: gut microbiota of suckling lambs. PC1–8: the 1st to 8th principal components of the principal coordinate analysis, which explained more than 80% of the microbiota difference between the samples.
3.4. Sources of microbiota associated with the growth performance of suckling lambs
We then compared the gut microbiota of suckling lambs and their potential sources. The results of PCoA revealed a significant difference between the gut microbiota composition of lambs and the vaginal microbiota of ewes, the microbiota of air sediment, the microbiota of ewes’ teat skin, the colostrum microbiota, or the fecal microbiota of ewes (ANOSIM, R = 0.928, p < 0.01) (Figure 3A), and the inner-group distances in each source were also significantly different (p < 0.01) (Figure 3B). Furthermore, we calculated the relative distances between the gut microbiota of suckling lambs and the potential sources and found the distance between the gut microbiota of lamb and that of ewes’ teat skin was significantly lower than that of vagina, colostrum, and feces of ewe, as well as the air sediment (p < 0.05) (Figure 3C). In addition, the distance of gut microbiota composition between that of the vagina, air sediment, and feces of ewe for higher growth performance lambs (LF-1 group) was significantly lower than that between lower growth performance lambs (LF-2 and LF-3 groups) (p < 0.05) (Figures 3D–H). These findings indicated that the early colonized gut bacteria of newborn lambs had significant individual differences and mainly came from the teat skin of ewes, but the early colonized microbiota was more similar to the bacterial composition of the vagina and feces of ewes, and the air might be helpful to improve the growth performance of suckling lambs.
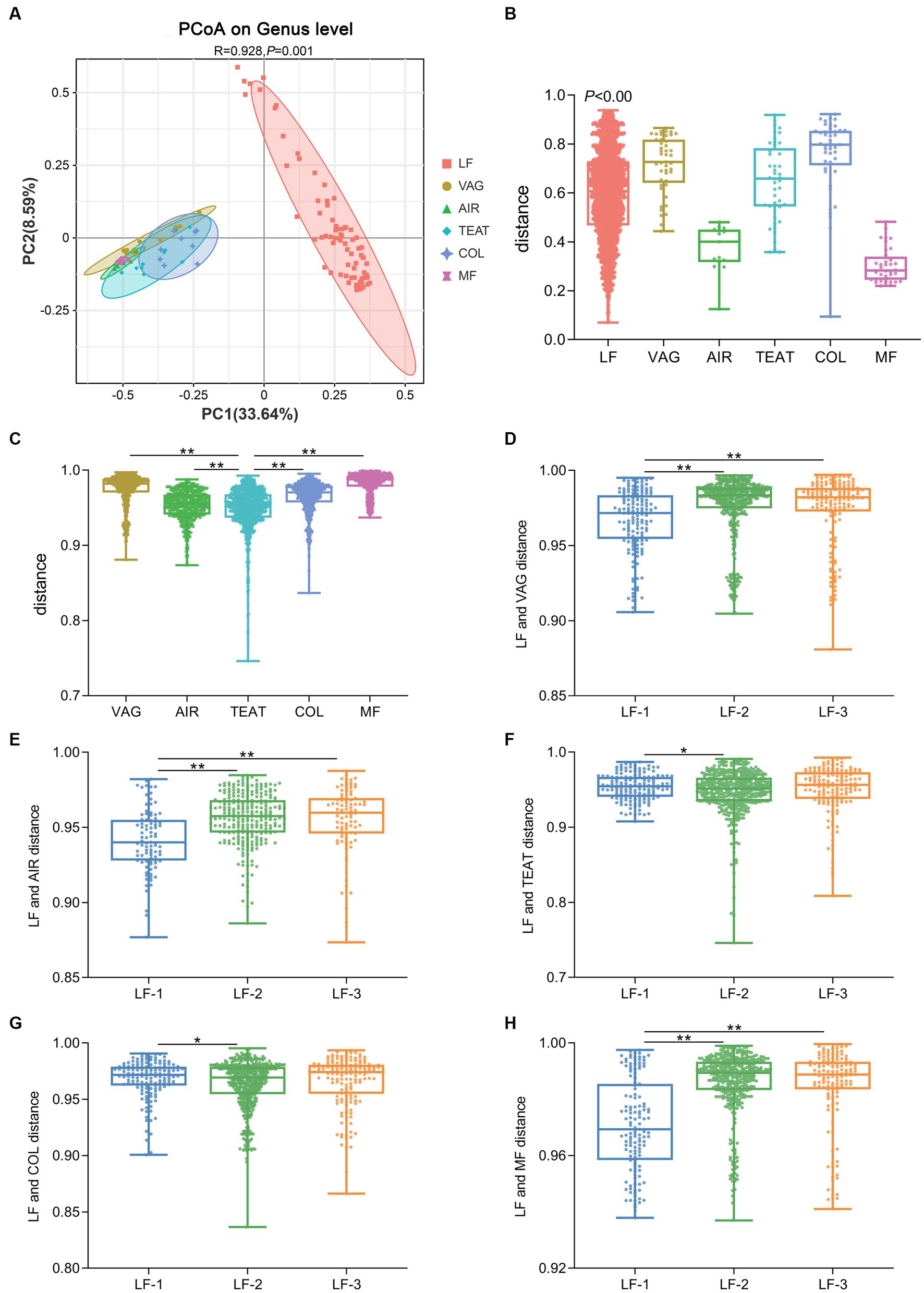
Figure 3. Source of the gut microbiota of suckling lambs. (A) Principal coordinate analysis (PCoA) of microbial communities at the genus level (based on the Jaccard distance). (B) Inner-group distance of microbiota composition in each group using Jaccard distance. (C) Distance between gut microbiota of suckling lambs and their potential sources. (D–H) Distance between gut microbiota of suckling lambs with different growth performances and their potential sources. VAG: vagina of ewes; AIR: air sediment; TEAT: teats skin of ewes; COL: colostrum of ewes; MF: feces of ewes. The suckling lambs were divided into LF-1 (top 15%), LF-2 (medium 70%), and LF-3 (bottom 15%) groups according to ADG30 of lambs. ** indicated significant differences among groups (p < 0.01).
3.5. Signature bacteria associated with growth performance of suckling lambs
To screen the signature bacteria associated with the growth performance of suckling lambs, we used the RandomForest machine learning algorithm with the lowest cross-validation error and detected 11, 11, 6, and 4 bacterial taxa at the genus level that were associated with BW30, BW45, ADG30, and ADG45 of suckling lambs, respectively (Figure 4).
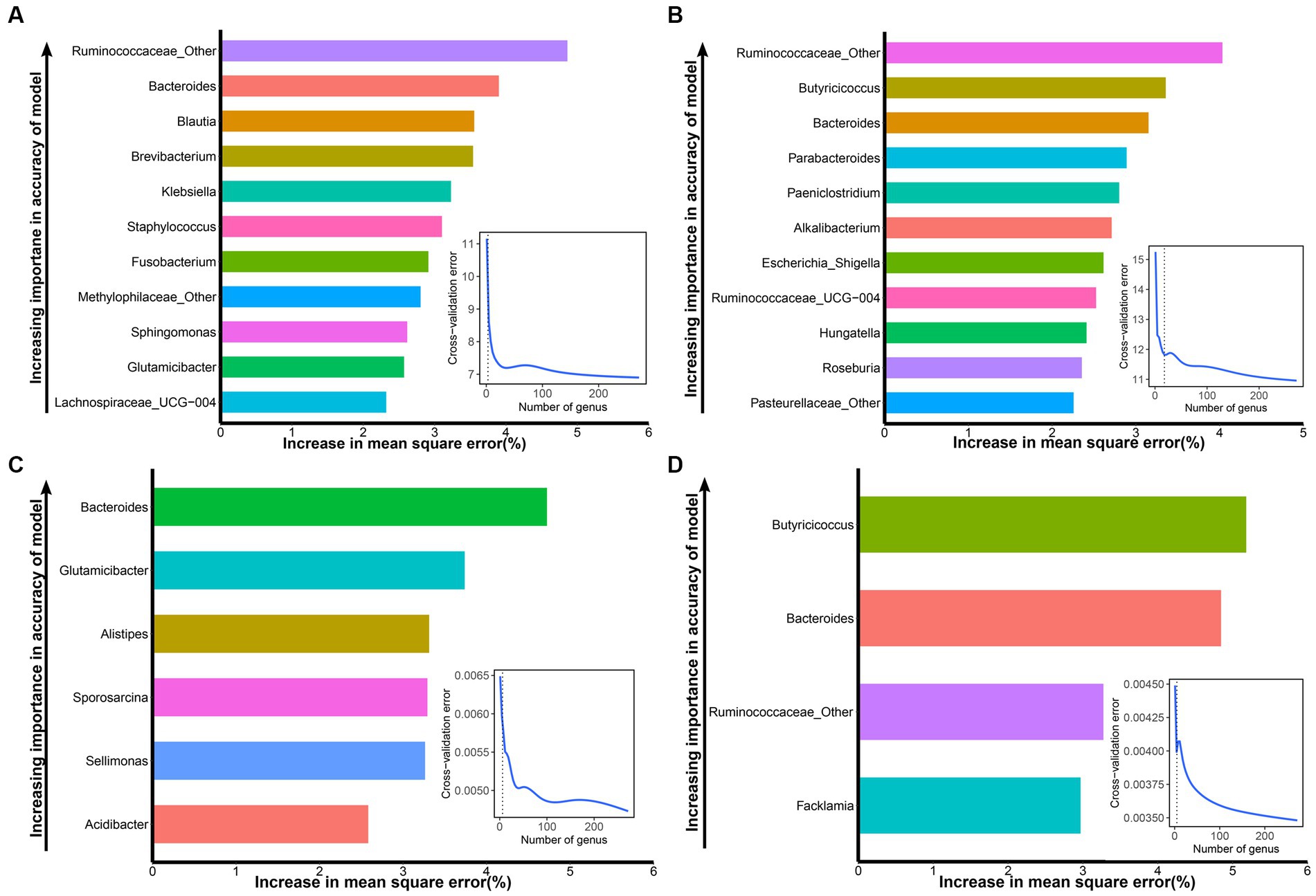
Figure 4. Signature bacteria associated with growth performance (BW30, BW45, ADG30, and ADG45) of suckling lambs. (A) The top 11 signature bacteria associated with BW30 were identified by the RandomForest model. (B) The top 11 signature bacteria associated with BW45 were identified by the RandomForest model. (C) The top six signature bacteria associated with ADG30 were identified by the RandomForest model. (D) The top four microbial markers associated with ADG45 were identified by the RandomForest model. Signature bacteria were ranked in descending order of importance in the RandomForest model. The inset panel represents the 10-fold cross-validation error.
Ranked according to the importance of the index, the signature bacteria associated with BW30 were: Ruminococcaceae_Other, Bacteroides, Blautia, Brevibacterium, Klebsiella, Staphylococcus, Fusobacterium, Methylophilaceae_Other, Sphingomonas, Glutamicibacter, and Lachnospiraceae_UCG-004 (Figure 4A). The signature bacteria associated with BW45 were: Ruminococcaceae_Other, Butyricicoccus, Bacteroides, Parabacteroides, Paeniclostridium, Alkalibacterium, Escherichia_Shigella, Ruminococcaceae_UCG-004, Hungatella, Roseburia, and Pasteurellaceae_Other (Figure 4B). The signature bacteria associated with ADG30 were: Bacteroides, Glutamicibacter, Alistipes, Sporosarcina, Sellimonas, and Acidibacter (Figure 4C). The signature bacteria associated with ADG45 were: Butyricicoccus, Bacteroides, Ruminococcaceae_Other, and Faecalibacterium (Figure 4D). Similarly, the signature bacteria associated with GI30 were: Bacteroides, Ruminococcus_gnavus_group, Faecalibacterium, Fusobacterium, Acidibacter, Atopostipes, Ruminococcus_torques_group, Globicatella, Flavonifractor, and Ruminococcus_2. The signature bacteria associated with GI45 were: Alistipes, Lachnospiraceae_AC2044_group, Microvirga, Ruminococcus_1, and Acidibacter (Supplementary Figure S2).
3.6. Correlation between signature bacteria and growth performance of suckling lambs
To investigate which gut signature bacteria affect the growth performance of suckling lambs, we further assessed their relationship with the Pearson correlation analysis. The results showed that the growth performance was negatively correlated with two signature bacteria and positively correlated with eight signature bacteria. Among them, BW30 was negatively correlated with Staphylococcus (r = −0.27, p < 0.05), and Atopostipes (r = −0.27, p < 0.05), and positively correlated with Lachnospiraceae_UCG-004 (r = 0.39, p < 0.01), Hungatella (r = 0.30, p < 0.05), Butyricicoccus (r = 0.30, p < 0.01), Ruminococcaceae_Other (r = 0.28, p < 0.05), Bacteroides (r = 0.31, p < 0.01), and Fusobacterium (r = 0.35, p < 0.01). ADG30 was negatively correlated with Staphylococcus (r = −0.24, p < 0.05) and Atopostipes (r = −0.24, p < 0.05), and positively correlated with Lachnospiraceae_UCG-004 (r = 0.27, p < 0.01), Hungatella (r = 0.29, p < 0.05), Butyricicoccus (r = 0.29, p < 0.05), Ruminococcaceae_Other (r = 0.28, p < 0.05), Ruminococcus_gnavus_group (r = 0.29, p < 0.01), and Fusobacterium (r = 0.25, p < 0.01). BW45 was negatively correlated with Staphylococcus (r = −0.24, p < 0.05), and positively correlated with Lachnospiraceae_UCG-004 (r = 0.25, p < 0.05), Butyricicoccus (r = 0.42, p < 0.01), Ruminococcaceae_Other (r = 0.29, p < 0.05), Ruminococcus_gnavus_group (r = 0.27, p < 0.05), Bacteroides (r = 0.35, p < 0.01), Fusobacterium (r = 0.32, p < 0.01), and Parabacteroides (r = 0.24, p < 0.05). ADG45 was positively correlated with Butyricicoccus (r = 0.40, p < 0.01), Ruminococcaceae_Other (r = 0.27, p < 0.05), Ruminococcus_gnavus_group (r = 0.27, p < 0.01), Bacteroides (r = 0.31, p < 0.01), Fusobacterium (r = 0.26, p < 0.05), and Parabacteroides (r = 0.25, p < 0.05) (Figure 5). Butyricicoccus, Ruminococcus_gnavus_group, Ruminococcaceae_Other, and Fusobacterium were common microorganisms that affect growth performance, and the relative abundance of Butyricicoccus, Ruminococcus_gnavus_group, and Fusobacterium had a significant difference among the LF-1, LF-2, and LF-3 groups (Supplementary Figure S3). Similarly, we also detected the bacterial taxa related to growth performance at the phylum, class, order, and family level through LEfSe analysis and correlation analysis, which are shown in Supplementary Figure S4.
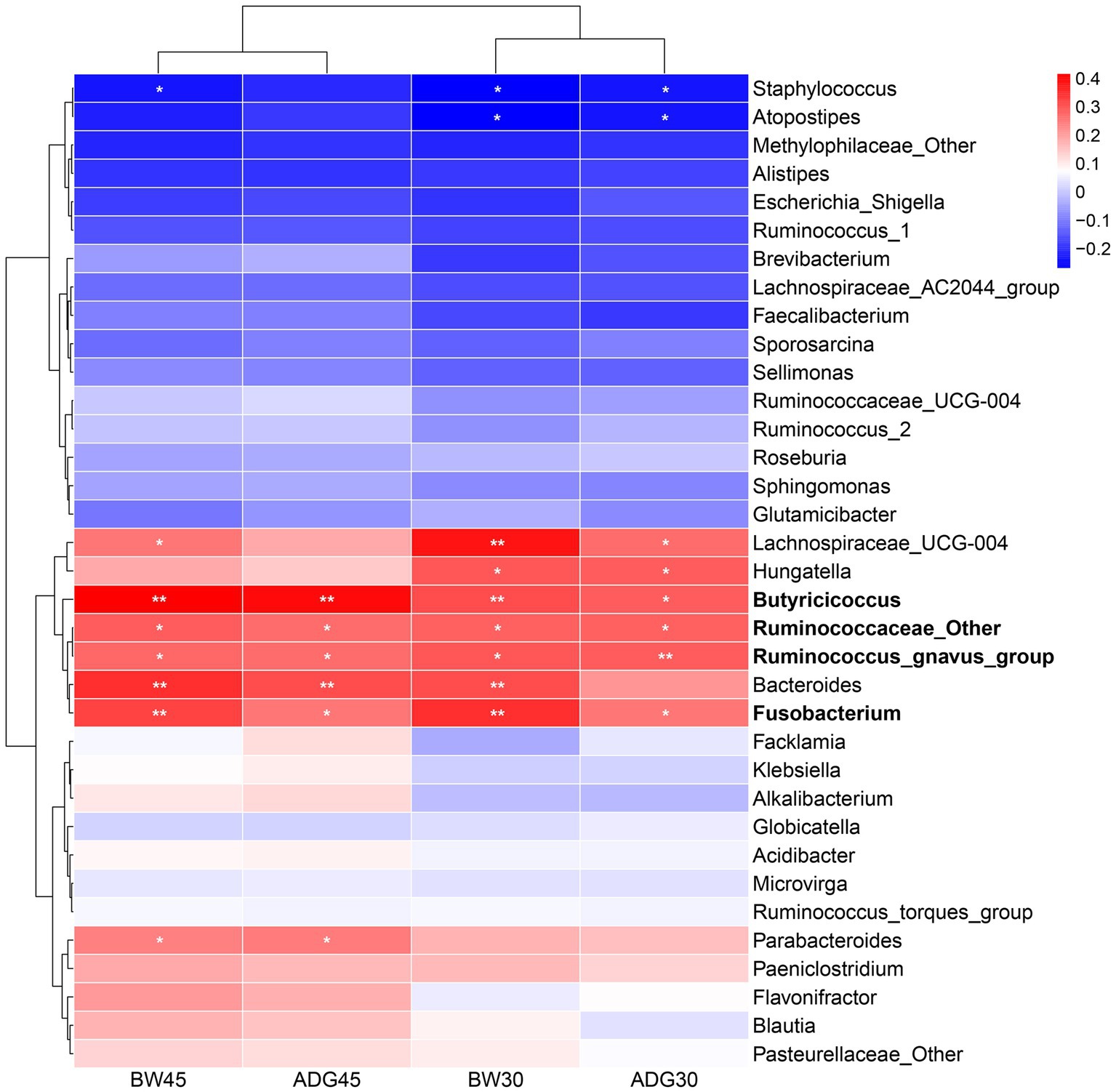
Figure 5. Correlation between signature bacteria and growth performance (BW30, BW45, ADG30, and ADG45) of suckling lambs. *p < 0.05, **p < 0.01.
4. Discussion
4.1. Effect of gut microbiota on growth performance of suckling lambs
Previous studies have shown that various factors can influence the growth performance of suckling lambs, such as serum IgG levels, birth weight, litter size, and sex of lambs; however, these factors could not fully explain the growth performance of suckling lambs (Gokce et al., 2013; Le Dividich et al., 2017; Gokce and Atakisi, 2019; Charneca et al., 2021). Some studies in recent years indicated that the early colonization of gut microbiota could also affect the immunity and health of animals (Tanca et al., 2017; Momo et al., 2023); therefore, we hypothesized that the early colonized bacteria could also affect the growth performance of suckling lambs.
Our findings indicated that the gut microbiota of suckling lambs had high individual variability, lambs with different growth performance might have different gut microbiota composition in early life, and the suckling lambs with higher growth performance had higher Shannon diversity of gut microbiota; this finding was consistent with previous studies in yaks (Petri et al., 2013; Huang et al., 2021) and pigs or calves (Dill-McFarland et al., 2019; Chen C. et al., 2021; Chen X. et al., 2021; Hu et al., 2023), which supported the previous findings that microbial diversity and composition might play a crucial role in growth performance. Furthermore, we observed that the contribution of early colonized bacteria on the growth performance of lambs increased with age (from BW30 at 25.35% to BW45 at 31.10%; from ADG30 at 33.02% to ADG45 at 39.79% by measuring the relative effects of factors that influence growth performance). These unexpected findings suggested that the early colonization gut microbiota might have a long-lasting effect on the growth performance of suckling lambs.
4.2. Sources of gut microbiota on growth performance of suckling lambs
The newborn period is a key period for microbial colonization, and bacteria rapidly colonize the gut of newborn lambs by transferring from the surrounding environment, such as the vagina, teat skin, colostrum, and feces of ewes, as well as the bacteria in air sediment (Arrieta et al., 2015; Koleva et al., 2015; Perez-Munoz et al., 2017). The microbiota formed in the gut of newborn lambs depends on the environment to which they were first exposed. This also supported our previous observations that the gut microbiota of newborn lambs has high individual variability, which raised another question: Would the different sources of microbiota affect the growth performance of suckling lambs?
We calculated the distance between the gut microbiota of lambs and that of the potential sources and found that the gut microbiota of lambs with higher growth performance was more similar to that of the vagina, feces of ewe, and air sediment. Previous studies had found that there were abundant beneficial bacteria such as lactobacillus and bifidobacterial in the vagina, feces, and air sediment, which could enhance immunity, intestinal functions, and maturity of the gut microbiota, thus improving the growth performance of animals in early life (Mikami et al., 2012; Makino et al., 2013; Shin et al., 2015; Ferretti et al., 2018; Quintana et al., 2020; Saturio et al., 2021). Therefore, newborn lambs more frequently contacted with the vagina and feces of ewes as well as the air sediment might be helpful to improve their growth performance.
4.3. Signature bacteria that affect the growth performance of suckling lambs
We identified 35 signature bacteria associated with the growth performance of suckling lambs using the RandomForest model and investigated their correlation with the growth performance.
The results showed that Butyricicoccus, Ruminococcus_gnavus_group, Ruminococcaceae_Other, and Fusobacterium had positive correlations with growth performance (BW30, BW45, ADG30, and ADG45). The possible explanation for this finding might be due to their functions. Butyricicoccus is the major butyric-acid-producing bacterial taxa in the gut, which has demonstrated a new generation of probiotics because butyric acid (the production of Butyricicoccus) is one of the most important nutrients for intestinal epithelial cells, and Butyricicoccus can also reduce the load of pathogenic bacteria in the cecum and ileum (Mahdavi et al., 2021) and prevent inflammation by inhibiting secretion of IL-8 and interferon γ (IFN γ) (Eeckhaut et al., 2013). Both Ruminococcus_gnavus_group and Ruminococcaceae_Other belonged to the Ruminococcaceae at the family level, which played a vital role in digesting nutrients and regulating the metabolism of the host. Some studies also observed that Ruminococcaceae colonized in the gut would benefit animals by keeping the integrity of the gut barrier and preventing diarrhea (Chua et al., 2018; Vojinovic et al., 2019). Some members of the genus Fusobacterium were capable of fermentative metabolism in anaerobic environments to produce organic acids, which might play a positive role in the metabolism of nutrients in the gut (Potrykus et al., 2007; Ma et al., 2022). These signature bacteria detected in the current experiment might provide potential targets for manipulating the gut microbiota in early life to improve the growth performance of suckling lambs.
5. Conclusion
The early colonized gut microbiota could significantly affect the growth performance of suckling lambs, and the contribution of early colonized bacteria to the growth performance of lambs increased with age. Butyricicoccus, Ruminococcus_gnavus_group, Ruminococcaceae_Other, and Fusobacterium were the signature bacteria affecting the growth performance of suckling lambs.
Data availability statement
The datasets presented in this study can be found in online repositories. The names of the repository/repositories and accession number(s) can be found at: https://ngdc.cncb.ac.cn/gsa/. Accession number CRA012031.
Ethics statement
The animal studies were approved by Animal Care and Use Committee of Hebei Agricultural University. The studies were conducted in accordance with the local legislation and institutional requirements. Written informed consent was obtained from the owners for the participation of their animals in this study.
Author contributions
HX: Data curation, Formal analysis, Investigation, Methodology, Writing – original draft. HY: Data curation, Validation, Writing – review & editing. PT: Investigation, Methodology, Writing – original draft. SJ: Conceptualization, Data curation, Funding acquisition, Project administration, Resources, Supervision, Validation, Writing – review & editing. WZ: Formal analysis, Writing – original draft. CL: Formal analysis, Writing – original draft. YZ: Funding acquisition, Resources, Writing – review & editing. YL: Conceptualization, Resources, Supervision, Writing – review & editing.
Funding
The author(s) declare financial support was received for the research, authorship, and/or publication of this article. This study was funded by the Scientific Research Foundation of Hebei Agricultural University (YJ20182), the Hebei Natural Science Foundation (C2022204232), and the China Agriculture Research System of Mutton Sheep (CARS-39).
Acknowledgments
The authors thank Jinhui Li, Lu Wang, and Mengyu Liu for their help in statistical analysis.
Conflict of interest
The authors declare that the research was conducted in the absence of any commercial or financial relationships that could be construed as a potential conflict of interest.
Publisher’s note
All claims expressed in this article are solely those of the authors and do not necessarily represent those of their affiliated organizations, or those of the publisher, the editors and the reviewers. Any product that may be evaluated in this article, or claim that may be made by its manufacturer, is not guaranteed or endorsed by the publisher.
Supplementary material
The Supplementary material for this article can be found online at: https://www.frontiersin.org/articles/10.3389/fmicb.2023.1273444/full#supplementary-material
References
Arrieta, M. C., Stiemsma, L. T., Dimitriu, P. A., Thorson, L., Russell, S., Yurist-Doutsch, S., et al. (2015). Early infancy microbial and metabolic alterations affect risk of childhood asthma. Sci. Transl. Med. 7:307ra152. doi: 10.1126/scitranslmed.aab2271
Bi, Y., Cox, M. S., Zhang, F., Suen, G., Zhang, N., Tu, Y., et al. (2019). Feeding modes shape the acquisition and structure of the initial gut microbiota in newborn lambs. Environ. Microbiol. 21, 2333–2346. doi: 10.1111/1462-2920.14614
Charneca, R., Nunes, J. T., Freitas, A., and Le Dividich, J. (2021). Effect of litter birth weight standardization before first suckling on colostrum intake, passive immunization, pre-weaning survival, and growth of the piglets. Animal 15:100184. doi: 10.1016/j.animal.2021.100184
Chen, C., Fang, S., Wei, H., He, M., Fu, H., Xiong, X., et al. (2021). Prevotella copri increases fat accumulation in pigs fed with formula diets. Microbiome. 9:175. doi: 10.1186/s40168-021-01110-0
Chen, X., Su, X., Li, J., Yang, Y., Wang, P., Yan, F., et al. (2021). Real-time monitoring of ruminal microbiota reveals their roles in dairy goats during subacute ruminal acidosis. Npj Biofilms Microbiomes. 7:45. doi: 10.1038/s41522-021-00215-6
Chigerwe, M., Hagey, J. V., and Aly, S. S. (2015). Determination of neonatal serum immunoglobulin g concentrations associated with mortality during the first 4 months of life in dairy heifer calves. J. Dairy Res. 82, 400–406. doi: 10.1017/S0022029915000503
Chua, H. H., Chou, H. C., Tung, Y. L., Chiang, B. L., Liao, C. C., Liu, H. H., et al. (2018). Intestinal dysbiosis featuring abundance of ruminococcus gnavus associates with allergic diseases in infants. Gastroenterology 154, 154–167. doi: 10.1053/j.gastro.2017.09.006
Collado, M. C., Cernada, M., Bauerl, C., Vento, M., and Perez-Martinez, G. (2012). Microbial ecology and host-microbiota interactions during early life stages. Gut Microbes 3, 352–365. doi: 10.4161/gmic.21215
Cristofori, F., Dargenio, V. N., Dargenio, C., Miniello, V. L., Barone, M., and Francavilla, R. (2021). Anti-inflammatory and immunomodulatory effects of probiotics in gut inflammation: a door to the body. Front. Immunol. 12:578386. doi: 10.3389/fimmu.2021.578386
Dill-McFarland, K. A., Weimer, P. J., Breaker, J. D., and Suen, G. (2019). Diet influences early microbiota development in dairy calves without long-term impacts on milk production. Appl. Environ. Microbiol. 85, e02141–e02118. doi: 10.1128/AEM.02141-18
Dogra, S. K., Kwong, C. C., Wang, D., Sakwinska, O., Colombo, M. S., and Sprenger, N. (2021). Nurturing the early life gut microbiome and immune maturation for long term health. Microorganisms. 9:2110. doi: 10.3390/microorganisms9102110
Eeckhaut, V., Machiels, K., Perrier, C., Romero, C., Maes, S., Flahou, B., et al. (2013). Butyricicoccus pullicaecorum in inflammatory bowel disease. Gut 62, 1745–1752. doi: 10.1136/gutjnl-2012-303611
Ferretti, P., Pasolli, E., Tett, A., Asnicar, F., Gorfer, V., Fedi, S., et al. (2018). Mother-to-infant microbial transmission from different body sites shapes the developing infant gut microbiome. Cell Host Microbe 24, 133–145.e5. doi: 10.1016/j.chom.2018.06.005
Gokce, E., and Atakisi, O. (2019). Interrelationships of serum and colostral igg (passive immunity) with total protein concentrations and health status in lambs. Kafkas Univ. Vet. Fak. 25, 387–396. doi: 10.9775/kvfd.2018.21035
Gokce, E., Atakisi, O., Kirmizigul, A. H., and Erdogan, H. M. (2013). Risk factors associated with passive immunity, health, birth weight and growth performance in lambs: ii. Effects of passive immunity and some risk factors on growth performance during the first 12 weeks of life. Kafkas Univ. Vet. Fak. 19, 619–627. doi: 10.9775/kvfd.2013.8442
Hu, R., Li, S., Diao, H., Huang, C., Yan, J., Wei, X., et al. (2023). The interaction between dietary fiber and gut microbiota, and its effect on pig intestinal health. Front. Immunol. 14:1095740. doi: 10.3389/fimmu.2023.1095740
Huang, C., Ge, F., Yao, X. X., Guo, X., Bao, P. J., Ma, X. M., et al. (2021). Microbiome and metabolomics reveal the effects of different feeding systems on the growth and ruminal development of yaks. Front. Microbiol. 12:682989. doi: 10.3389/fmicb.2021.682989
Jami, E., Israel, A., Kotser, A., and Mizrahi, I. (2013). Exploring the bovine rumen bacterial community from birth to adulthood. ISME J. 7, 1069–1079. doi: 10.1038/ismej.2013.2
Kim, T. I., Lim, D. H., Jang, S. S., Kim, S. B., Park, S. M., Park, J. H., et al. (2018). Effects of supplementing barodon, bacillus subtilis, and ampbio on growth performance, biochemical metabolites, and hormone levels in korean native heifers. Trop. Anim. Health Prod. 50, 1637–1643. doi: 10.1007/s11250-018-1606-7
Koleva, P. T., Kim, J. S., Scott, J. A., and Kozyrskyj, A. L. (2015). Microbial programming of health and disease starts during fetal life. Birth Defects Res. C Embryo Today 105, 265–277. doi: 10.1002/bdrc.21117
Le Dividich, J., Charneca, R., and Thomas, F. (2017). Relationship between birth order, birth weight, colostrum intake, acquisition of passive immunity and pre-weaning mortality of piglets. Span. J. Agric. Res. 15:e0603. doi: 10.5424/sjar/2017152-9921
Li, X., Ding, N., Zhang, Z., Tian, D., Han, B., Liu, D., et al. (2022). Identification of sstr5 gene polymorphisms and their association with growth traits in hulun buir sheep. Front. Genet. 13:831599. doi: 10.3389/fgene.2022.831599
Lin, L. M., Xie, F., Sun, D. M., Liu, J. H., Zhu, W. Y., and Mao, S. Y. (2019). Ruminal microbiome-host crosstalk stimulates the development of the ruminal epithelium in a lamb model. Microbiome. 7:83. doi: 10.1186/s40168-019-0701-y
Ma, C., Azad, M., Tang, W., Zhu, Q., Wang, W., Gao, Q., et al. (2022). Maternal probiotics supplementation improves immune and antioxidant function in suckling piglets via modifying gut microbiota. J. Appl. Microbiol. 133, 515–528. doi: 10.1111/jam.15572
Mahdavi, M., Laforest-Lapointe, I., and Masse, E. (2021). Preventing colorectal cancer through prebiotics. Microorganisms 9:1325. doi: 10.3390/microorganisms9061325
Makino, H., Kushiro, A., Ishikawa, E., Kubota, H., Gawad, A., Sakai, T., et al. (2013). Mother-to-infant transmission of intestinal bifidobacterial strains has an impact on the early development of vaginally delivered infant’s microbiota. PLoS One 8:e78331. doi: 10.1371/journal.pone.0078331
Martin, P., Vinet, A., Denis, C., Grohs, C., Chanteloup, L., Dozias, D., et al. (2021). Determination of immunoglobulin concentrations and genetic parameters for colostrum and calf serum in charolais animals. J. Dairy Sci. 104, 3240–3249. doi: 10.3168/jds.2020-19423
Mikami, K., Kimura, M., and Takahashi, H. (2012). Influence of maternal bifidobacteria on the development of gut bifidobacteria in infants. Pharmaceuticals (Basel) 5, 629–642. doi: 10.3390/ph5060629
Momo, K. B., Otiti, M. I., Ramsteijn, A. S., Sow, D., Faye, B., Heffernan, C., et al. (2023). Modulating the early-life gut microbiota using pro-, pre-, and synbiotics to improve gut health, child development, and growth. Nutr. Rev. 11:nuad050. doi: 10.1093/nutrit/nuad050
Noor, R., Naz, A., Maniha, S. M., Tabassum, N., Tabassum, T., Tabassum, T., et al. (2021). Microorganisms and cardiovascular diseases: importance of gut bacteria. Front Biosci (Landmark Ed). 26, 22–28. doi: 10.52586/4921
Peled, J. U., Jenq, R. R., Holler, E., and van den Brink, M. R. (2016). Role of gut flora after bone marrow transplantation. Nat. Microbiol. 1:16036. doi: 10.1038/nmicrobiol.2016.36
Peng, J., Tang, Y., and Huang, Y. (2021). Gut health: the results of microbial and mucosal immune interactions in pigs. Anim Nutr. 7, 282–294. doi: 10.1016/j.aninu.2021.01.001
Perez-Munoz, M. E., Arrieta, M. C., Ramer-Tait, A. E., and Walter, J. (2017). A critical assessment of the “sterile womb” and “in utero colonization” hypotheses: implications for research on the pioneer infant microbiome. Microbiome. 5:48. doi: 10.1186/s40168-017-0268-4
Petri, R. M., Schwaiger, T., Penner, G. B., Beauchemin, K. A., Forster, R. J., McKinnon, J. J., et al. (2013). Characterization of the core rumen microbiome in cattle during transition from forage to concentrate as well as during and after an acidotic challenge. PLoS One 8:e83424. doi: 10.1371/journal.pone.0083424
Potrykus, J., Mahaney, B., White, R. L., and Bearne, S. L. (2007). Proteomic investigation of glucose metabolism in the butyrate-producing gut anaerobe fusobacterium varium. Proteomics 7, 1839–1853. doi: 10.1002/pmic.200600464
Quintana, A. R., Sesena, S., Garzon, A., and Arias, R. (2020). Factors affecting levels of airborne bacteria in dairy farms: a review. Animals (Basel). 10:526. doi: 10.3390/ani10030526
Saturio, S., Nogacka, A. M., Suarez, M., Fernandez, N., Mantecon, L., Mancabelli, L., et al. (2021). Early-life development of the bifidobacterial community in the infant gut. Int. J. Mol. Sci. 22:3382. doi: 10.3390/ijms22073382
Shin, H., Pei, Z., Martinez, K. N., Rivera-Vinas, J. I., Mendez, K., Cavallin, H., et al. (2015). The first microbial environment of infants born by c-section: the operating room microbes. Microbiome. 3:59. doi: 10.1186/s40168-015-0126-1
Tanca, A., Fraumene, C., Manghina, V., Palomba, A., Abbondio, M., Deligios, M., et al. (2017). Diversity and functions of the sheep faecal microbiota: a multi-omic characterization. Microb. Biotechnol. 10, 541–554. doi: 10.1111/1751-7915.12462
Vojinovic, D., Radjabzadeh, D., Kurilshikov, A., Amin, N., Wijmenga, C., Franke, L., et al. (2019). Relationship between gut microbiota and circulating metabolites in population-based cohorts. Nat. Commun. 10:5813. doi: 10.1038/s41467-019-13721-1
Yeoman, C. J., Ishaq, S. L., Bichi, E., Olivo, S. K., Lowe, J., and Aldridge, B. M. (2018). Biogeographical differences in the influence of maternal microbial sources on the early successional development of the bovine neonatal gastrointestinal tract. Sci. Rep. 8:3197. doi: 10.1038/s41598-018-21440-8
Yin, X., Ji, S., Duan, C., Ju, S., Zhang, Y., Yan, H., et al. (2021). Rumen fluid transplantation affects growth performance of weaned lambs by altering gastrointestinal microbiota, immune function and feed digestibility. Animal 15:100076. doi: 10.1016/j.animal.2020.100076
Zhang, L., Jiang, X., Liu, X., Zhao, X., Liu, S., Li, Y., et al. (2019). Growth, health, rumen fermentation, and bacterial community of Holstein calves fed lactobacillus rhamnosus gg during the preweaning stage1. J. Anim. Sci. 97, 2598–2608. doi: 10.1093/jas/skz126
Keywords: early colonized gut microbiota, suckling lambs, growth performance, RandomForest, gut signature bacteria
Citation: Xiao H, Yan H, Tian P, Ji S, Zhao W, Lu C, Zhang Y and Liu Y (2023) The effect of early colonized gut microbiota on the growth performance of suckling lambs. Front. Microbiol. 14:1273444. doi: 10.3389/fmicb.2023.1273444
Edited by:
Ignacio Badiola, Institute of Agrifood Research and Technology (IRTA), SpainReviewed by:
Ana Griselda Binetti, CONICET Instituto de Lactología Industrial (INLAIN), ArgentinaRashmi Hogarehalli Mallappa, National Dairy Research Institute (ICAR), India
Copyright © 2023 Xiao, Yan, Tian, Ji, Zhao, Lu, Zhang and Liu. This is an open-access article distributed under the terms of the Creative Commons Attribution License (CC BY). The use, distribution or reproduction in other forums is permitted, provided the original author(s) and the copyright owner(s) are credited and that the original publication in this journal is cited, in accordance with accepted academic practice. No use, distribution or reproduction is permitted which does not comply with these terms.
*Correspondence: Shoukun Ji, amlzaG91a3VuQDE2My5jb20=; Yueqin Liu, bGl1eXVlcWluNjZAMTI2LmNvbQ==
†These authors have contributed equally to this work