- 1Key Laboratory of Animal Nutrition and Feed Science in Inner Mongolia Autonomous Region Universities, College of Animal Science, Inner Mongolia Agricultural University, Hohhot, China
- 2Beijing Key Laboratory of Dairy Cattle Nutrition, College of Animal Science and Technology, Beijing University of Agriculture, Beijing, China
The objective of this study was to evaluate the potential modulating effects of Allium mongolicum regel ethanol extract (AME) on rumen fermentation and biohydrogenation (BH) bacteria in vitro. Four Holstein cows were used as donors for the rumen fluid used in this study. In experiment 1, five treatments (supplemented with 0 mg/g, 1 mg/g, 2 mg/g, 3 mg/g, and 4 mg/g of AME based on fermentation substrate, respectively) were conducted to evaluate the effects of different levels of AME on fermentation status in vitro. The results showed that after 24 h of fermentation, MCP was reduced with AME supplementation (p < 0.05), and the multiple combinations of different combinations index (MFAEI) value was the highest with 3 mg/g of AME. In experiment 2, six treatments were constructed which contained: control group (A1); the unsaturated fatty acid (UFA) mixture at 3% concentration (A2); the mixture of A2 and 3 mg/g of AME (A3); 3 mg/g of AME (A4); the UFA mixture at 1.5% concentration (A5); the mixture of A5 and 3 mg/g of AME (A6). The abundance of bacterial species involved in BH was measured to evaluate the potential modulating effect of AME on rumen BH in vitro. Compared with the A1 group, the A3, A4, and A6 groups both showed significant decreases in the abundance of rumen BH microbial flora including Butyrivibrio proteoclasticus, Butyrivibrio fibrisolvens, Ruminococcus albus and Clostridium aminophilum (p < 0.01). The A3 group was less inhibitory than A4 in the abundance of B. proteoclasticus, B. fibrisolvens, and R. albus, and the inhibitory effect of the A6 group was higher than that of A4. In conclusion, the supplementation with 3 mg/g of AME could modulate the rumen fermentation and affect BH key bacteria, which suggests that AME may have the potential to inhibit the rumen BH of dairy cows.
1. Introduction
Plant extracts (PE) have been extensively studied in recent ruminant research due to their significant regulatory effects on rumen fermentation (Cobellis et al., 2016) and rumen biohydrogenation (BH; Durmic et al., 2008). In addition, UFAs are of considerable benefit to human health (Du et al., 2018), and reduced intake of saturated fatty acid (SFA) products may reduce cardiovascular disease risk (Xie et al., 2018). Improving the unsaturated fatty acid (UFA) profile of meat and milk is an important topic in ruminant production. Regulating the rumen fermentation pattern and optimizing the structure of rumen microflora involved in hydrogenation have been considered effective approaches to increase the level of post-ruminal UFAs (Dewhurst and Moloney, 2013). In addition, rumen health is crucial for ensuring high-quality livestock products (Pang et al., 2022). Pan et al. (2014) reported that dietary-supplemented PE facilitates maintaining the homeostasis of the rumen environment and promotes ruminant performance. Griinari et al. (1988) reported that the rumen fermentation may cause milk fat depression, characterized by a decline in rumen pH and a shift in the rumen pattern of VFA (lower acetate and higher propionate proportions).
Partial PE supplementation in diets could modify the UFA composition of meat and milk (Frutos et al., 2020). Dietary inclusion of grapeseed, which contains high levels of phenolic compounds, resulted in greater linoleic acid (LA) and CLA levels in milk (Correddu et al., 2016). This may be related to the regulation effect of PE on rumen fermentation and BH. Diet supplementation with compound phenolic enriched yerba mate extracts resulted in less BH time, which increased the content of polyunsaturated fatty acid (PUFA) deposited in lamb tissues (Pena-Bermudez et al., 2022). Another study pointed out that supplementation of essential oil from anise increased ruminal pH and increased UFA concentrations in the rumen (El-Essawy et al., 2021). Furthermore, A recent study showed that condensed tannins from grapeseed induced slight change in microbial structural lipids, which decreased the level of cis-9, cis-12 C18:2 and increased the levels of cis-9, trans-11 C18:2 and trans-11 C18:1 in vitro (Costa et al., 2017). According to Liu et al. (2022), including Moringa leaf flavonoids in the diet can boost the protein content in milk. Similarly, Zhao et al. (2022a) discovered that adding citrus peel extract to milk can decrease SFA and increase MUFA and CLA due to its high flavonoid content. Santos et al. (2014) also support this finding. Kumar et al. (2014) pointed out that the possibility of PE flavonoids may improve the production performance of ruminants by changes in the rumen microbiota and intermediate nutrient metabolism. Another study showed that Pistacia atlantica gum essential oil decreased the production of propionic acid and increased the relative abundance of Ruminococus flavefaciens (Naseri et al., 2022). Butyrivibrio (Paillard et al., 2007; Choi et al., 2009; Maia et al., 2010; Ramos-Morales et al., 2013), Ruminococcus albus (Nam and Garnsworthy, 2007) and Clostridium aminophilum (Moon et al., 2008) have been considered to play a major role in the rumen fermentation and ruminal BH process. Buccioni et al. (2015) reported that diet supplementation with quebracho tannins decreased the abundance of Butyrivibrio proteoclasticus and Butyrivibrio fibrisolvens and increased the levels of cis-9 trans-11 conjugated linoleic acid (CLA) and vaccenic acid (VA) in milk fat. It has been shown that phenolic compounds suppressed the last step of BH of PUFAs, which was achieved by inhibiting the proliferation and activity of Butyrivibrio proteoclasticus (Vasta et al., 2010).
Allium mongolicum regel (AMR), a typical herb in the genus Allium and the family Liliaceae, grows extensively in the northern and northwestern grasslands and deserts in China (Ding et al., 2021). The extracts of AMR have been shown to regulate the rumen environment and FA metabolism by increasing the concentration of total VFA and propionic acid in the rumen and the contents of UFAs in the longissimus dorsi muscle of lambs (Liu et al., 2019). Recent research reported that C17:0, and C18:0, which correlated with the “mutton flavor” of Small-Tailed Han sheep were decreased by adding AME (Watkins and Frank, 2019; Zhao et al., 2022b). The structure of rumen microflora is closely related to FA deposition in the longissimus dorsi muscle. Diet supplemented with AME changed the rumen fermentation pattern and the structure of rumen microflora (Zhao et al., 2022c), thus influencing the FA metabolism by improving MUFA and PUFA deposition in longissimus dorsi muscle (Zhao et al., 2022b). Existing research has demonstrated that AMR has significantly modulating effects on rumen microbial flora and FA metabolism in mutton sheep, its potential modulating effects on the rumen of dairy cows remain unknown.
Rumen fermentation and fatty acids BH could influence the UFA metabolism in dairy cows and thus regulate milk UFA profile. It is speculated that the AME would regulate the rumen fermentation and key bacteria involved in BH in dairy cows. Thus, this experiment aimed to evaluate the effect of AME on the bacteria involved in the hydrogenation and rumen fermentation of dairy cows in vitro.
2. Materials and methods
2.1. AME preparation
AMR was purchased from the company (Haohai Technology Co., Ltd., Alxa League, China) and was dried at 65°C and then ground (DFT-300, Shanghai Xinnuo Instrument Group CO., LTD, Shanghai, China) to powder form, was passed through an 80-mesh sieve (Shaoxing Shangyu Shengchao Instrument CO., Zhejiang, China).
The dried AMR powder was mixed with petroleum ether (Tianjin Beilian Fine Chemical Development Co., Tianjin, China) at a ratio of 1:5 (wt/vol) and placed on a shaker (KS 501 digital, IKA (Guangzhou) Instrumentation Co., Guangdong, China) set at 100 r/min for 24 h, after which the upper layer of petroleum ether was discarded to obtain the defatted and decolored AMR powder.
After defatting and decolorization, the AMR powder was mixed with 75% ethanol at a ratio of 1:30 (wt/vol), placed in an ultrasonic cell pulverizer (KQ-300DE, Kunshan Ultrasonic Instruments Co., Ltd., Jiangsu, China) and then filtered through a vacuum pump (FUD-2110, Tokyo Rikakikai CO., LTD., Tokyo, Japan) to obtain a filtrate. The filtrate was added to 75% ethanol and mixed at a ratio of 1:30; the above process was repeated, and the resulting filtrate was distilled through a rotary evaporator (65 r/min, 75°C, 15–18 min) to obtain the AME concentrate, which was lyophilized to remove excess water and obtain the AME powder. The main components of AME are flavonoids, organic acids and their derivatives, nucleotides and their derivatives, amino acids, phenol amine, choline, lipids, and others (Zhao et al., 2021).
2.2. Experimental design
The experiment contained two parts:
Experiment 1 was conducted to screen appropriate AME supplementation concentrations for experiment 2. The treatments were supplemented with 0 (control group), 1, 2, 3, and 4 mg of AME per gram of fermentation substrate for simulated diets. Each treatment had three technical replicates.
Experiment 2 contained 6 treatments. The treatments were designed as rumen fluid culture supplemented with (1) none (control group, A1); (2) 3% UFA (mixture of cis-9 C18:1, cis-9,cis-12 C18:2 and cis-9,cis-12,cis-15 C18:3, A2); (3) 3% UFA plus AME (A3); (4) AME (A4); (5) 1.5% UFA (mixture of cis-9 C18:1, cis-9, cis-12 C18:2 and cis-9, cis-12, cis-15 C18:3, A5); and (6) 1.5% UFA plus AME (A6); the supplementation of AME was 3 mg/g.
Each treatment had three technical replicates. The AME supplemental concentration in experiment 2 was determined from experiment 1. The UFA mixture contained the same levels of cis-9 C18:1, cis-9, cis-12 C18:2, and cis-9, cis-12, cis-15 C18:3 and was purchased from Sigma-Aldrich [Sigma Aldrich (Shanghai) Trading Ltd., Shanghai, China].
2.3. Incubation with ruminal fluid in vitro
Experiments were conducted in December 2021. The rumen fluid was obtained from Beiya Halal Slaughterhouse in Hohhot, China (111°63′E, 40°80’N). Four Holstein cows (550 ± 30 kg, two cows for each experiment) were subjected to strict quarantine at the slaughterhouse to ensure healthy body condition. The rumen fluid sample was filtered through 4 layers of gauze and then transported in a preheated flask with CO2 at 40°C to prevent temperature drop before sampling. In experiment 1, rumen fluid was mixed with artificial saliva at a ratio of 1:2 (15 mL,30 mL) and added to the culture flasks that were prefilled with different concentrations of AME and fermentation substrate, for details of each additive, refer to 2.2 (Experimental design). Artificial saliva was constructed according to a previous study (Menke et al., 1979). The composition and nutrient levels of the fermentation substrate are shown in Table 1. Fermentation was performed in an air bath shaker (39°C, 120 r/min). Fermentation lasted 24 h, and the samples were immediately placed on ice at the 24th hour to ensure that fermentation was stopped simultaneously to determine fermentation indicators.
The procedures for acquiring and cultivating rumen fluid in experiment 2 were consistent with experiment 1. The culture flasks that were prefilled with different concentration of UFA or AME and fermentation. This approach ensured the maintenance of standardized experimental conditions throughout the entire study.
2.4. Sample collection and analysis
At 24 h of fermentation in experiment 1 and at 24 h of fermentation in experiment 2, samples were collected to immediately determine pH by using a precalibrated hand-held precision pH meter (PHS-3C, Shanghai Osterol Industrial Co., Shanghai, China). After pH determination, the collected filtrate was placed in a centrifuge, centrifuged at 4000 r/min for 10 min, and stored at −20°C to determine ammonia-N (NH3-N), microbial crude protein (MCP) and volatile fatty acid (VFA) levels. Other samples were stored at room temperature overnight to count the protozoa using a hemocytometer. The rumen fluid of each sample was centrifuged, and the pellet was collected in a 5-mL cryopreservation tube and stored immediately at −80°C until DNA extraction.
The concentration of NH3-N was determined in the filtered solution by the indophenol colorimetric method (Chaney and Marbach, 1962). The VFAs of rumen fluid samples were determined using gas chromatography (GC-2014, SHIMADZU), which was equipped with a hydrogen flame detector and a capillary column (SHIMADZU, Technologies; 60-m long, 0.25-mm diameter; 50-μm film thickness) according to the method described in a previous study with modifications (Guo et al., 2021). The concentration of MCP was determined by the Coomassie brilliant blue method (Broderick and Craig, 1989).
The DNA of rumen bacteria was extracted by an E.Z.N. A® Bacterial DNA Kit (Omega Bio-Tek, Inc., Norcross, GA, United States) according to the instructions from the manufacturer. The final elution volume was 100 μL, and the NDA concentration and purity were measured by a spectrophotometer (NanoDrop 2000c; Thermo Fisher Scientific, DE, United States). The plasmid construction was confirmed, and the primer sequences (Table 2) were designed using primer-BLAST (NCBI). Each sample contained 2 μL of DNA, 0.4 μL 50 × ROX Reference Dye1 (Biolile, Xi’an, China), 0.8 μL of each primer, and 6 μL ddH2O in a final volume of 20 μL. Total DNA extracted from rumen samples was used as a template for PCR amplification. Six species of bacteria involved in hydrogenation were preamplified by qPCR, and two samples failed to amplify. The PCR program included 95°C for 5 min initial denaturation, 1 PCR cycle of 95°C for 30 s, 35 PCR cycles of 58°C for 30 s, and a final extension step at 72°C for 1 min.
The plasmid copy number was calculated using the following formula: plasmid copy number (copies/μL) = [10−9 × plasmid concentration (ng/μL) × 6.02 × 1023]/(plasmid length×660).
The plasmid abundance was calculated from the plasmid concentration determined by a NanoDrop2000 c spectrophotometer.
2.5. Statistical analysis
Data were analyzed by one-way ANOVA using SAS 9.21 software. Duncan’s multiple range test was used for multiple comparisons. The significant differences were indicated by p < 0.05, and a statistically meaningful trend was determined as 0.05 < p < 0.1.
When the combination effect is greater, the reaction is more favorable to the rumen’s fermentation or the component’s use. The multiple combinations of different combinations indexes (MFAEI) is the sum of the single combination effect indexes (SFAEI) of the different combinations (treatments) and can be used to determine the fermentation status of the rumen (Yuan and Wan, 2019). The calculation is as follows.
n: Total number of fermentation time points.
Q: Values of each indicator at each time point for the control group.
T: Values of each indicator at each time point for each experimental group.
R: Mean of the sum of T at each time point.
3. Results
The results are presented in two sections: screening suitable concentrations of AME and additives (Experiment 1), and evaluating changes in BH key bacteria in vitro (Experiment 2).
3.1. AME on rumen fermentation (experiment 1)
The effects of AME on rumen fermentation in vitro are outlined in Table 3. After 24 h of fermentation, the addition of AME did not affect rumen pH, TVFA, acetate, propionate, protozoa, and NH3-N, and MCP was reduced in response to AME supplementation (p < 0.05).
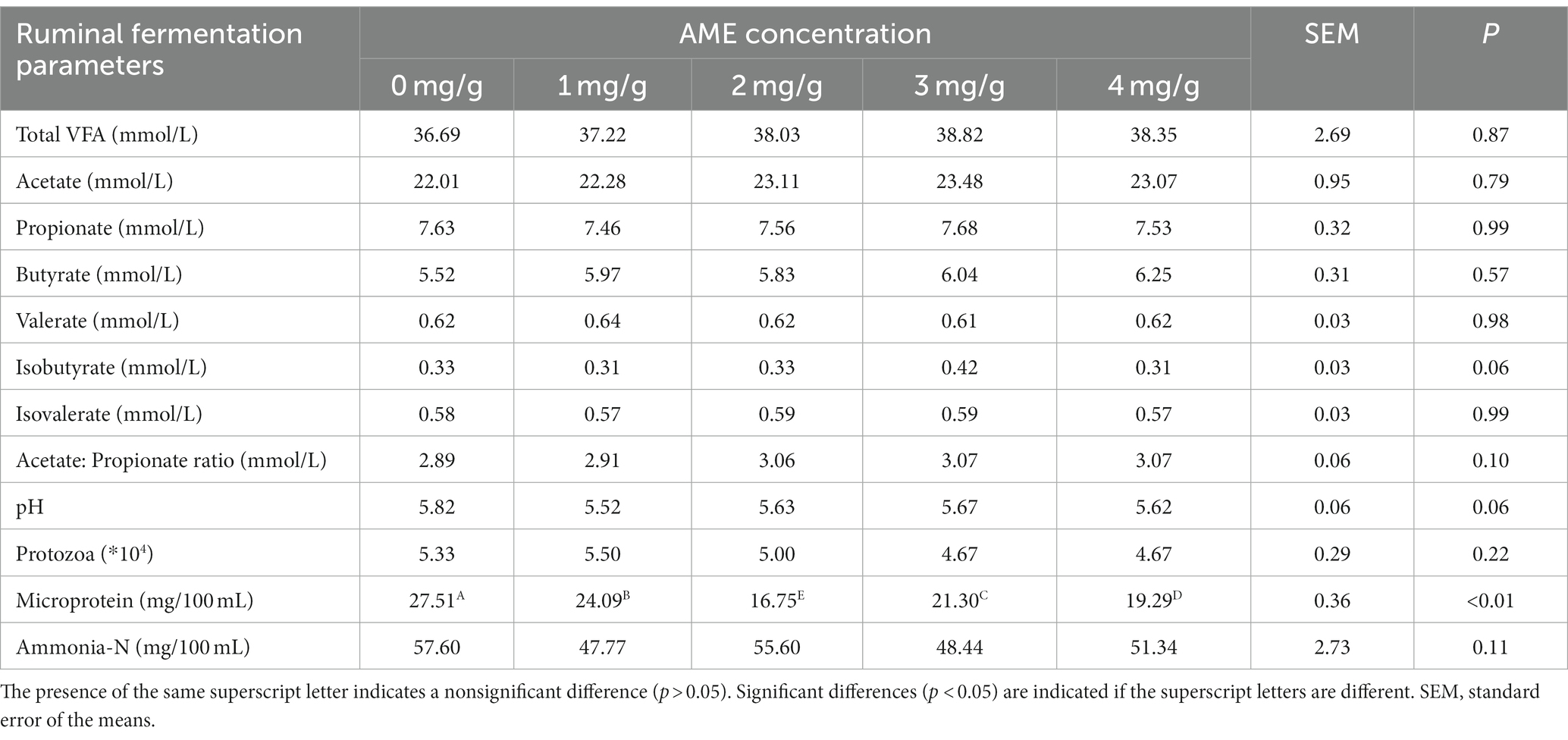
Table 3. Effect of AME at different concentrations on rumen fermentation parameters in experiment 1.
Table 4 shows the effect of AME on rumen fermentation. The MFAEI value under an AME concentration of 3 mg/g was the absolute maximum value of the experiments in this study, and the result shows that the suitable concentration of AME in modulating rumen fermentation was 3 mg/g.
3.2. AME-USFA and AME on rumen fermentation (experiment 2)
The effects of AME and UFA on rumen fermentation in experiment 2 are presented in Table 5. There were no effects of additives on rumen concentrations of acetate and total VFA. Whereas the amount of Propionate in the A4 group was lower than in other groups (p < 0.01), and the proportion of Acetate: Propionate ratio in the A4 group was higher than in other groups (p < 0.01). Significantly lower concentrations were detected in Butyrate acid that was supplemented with both UFA and AME groups (p < 0.01). The pH of A1 was significantly lower than that of the other treatment groups (p < 0.01). The number of protozoa in the A5 and A6 groups was significantly lower than that in the A1, A2, A3, and A4 groups. In addition, the number of protozoa in the A5 group was significantly lower than that in the control group and the other treatment groups (p < 0.05). The highest concentration of MCP was observed in the A3 group (p < 0.01), and the A4 group was significantly lower than the control group and other treatment groups (p < 0.01). The concentrations of NH3-N in the A1, A2, and A4 groups were significantly lower than those in the A3, A5, and A6 groups (p < 0.01).
3.3. AME-USFA on rumen biohydrogenation-related microbial flora (experiment 2)
Supplementation with AME and the AME-UFA mixture significantly affected the bacteria involved in hydrogenation, as shown in Table 6. Compared with the control group, the treatment groups exhibited decreased relative abundances of B. proteoclasticus, R. albus, and B. fibrisolven (p < 0.01; Table 5). The abundance of B. proteoclasticus in A2 was significantly lower than that in A5 (p < 0.01). The A4, A5, and A6 groups showed inhibition of R. albus growth, and R. albus growth in the A6 group was significantly lower than that in the other treatment groups (p < 0.01). Supplementation with AME and UFAs also significantly affected the relative abundances of B. fibrisolvens, and the abundance of B. fibrisolvens was lower than that in the control group in each treatment (p < 0.01). The growth of C. aminophilum was inhibited (p < 0.01) in the AME supplementation group (A4) after fermentation for 24 h.
4. Discussion
4.1. Effects of AME on rumen fermentation
The effects of PE on ruminal fermentation are variable (Naseri et al., 2022). In experiment 1, the concentration of NH3-N in the rumen fluid did not change in the AME supplementation group at 24 h. It has been reported that PE may not affect the concentration of NH3-N (Kozłowska et al., 2021). Moreover, a previous study reported that tannins as feed additives to modulate ruminal fermentation and ruminal BH in dairy ewes had no effects on NH3-N concentration (Toral et al., 2011). The addition of garlic oil to the diet of lactating dairy cows did not affect the concentration of NH3-N (Blanch et al., 2016). Furthermore, the NH3-N concentration in dairy cows did not change when feeding them high or low doses of Origanum vulgare L. extract (Olijhoek et al., 2019). However, a previous study reported that Pistacia atlantica gum essential oil may change the ruminal NH3-N concentration (Naseri et al., 2022). In addition, a high dose of thymol decreased the ruminal concentration of NH3-N, but lower doses did not affect the NH3-N concentration (Castillejos et al., 2006). Vakili et al. (2013) indicated that the effect of thymol on ruminal NH3-N concentration is dose-dependent. Zhao et al. (2022a) pointed out that AME supplementation in lambs did not affect ruminal NH3-N. In addition, although the treatments did not significantly affect the NH3-N concentration, 4 mg/g AME supplementation increased the in vitro MCP concentration after 24 h of fermentation.
A previous study considered protozoan variation to be positively correlated with NH3-N concentration (Spanghero et al., 2022). However, in another study, the type and concentration of the main active compounds in different PEs resulted in inconsistent protozoan population results (Soltan et al., 2018). In the present study, the number of protozoa was decreased by AME supplementation at 24 h. A study by Totakul et al. (2022) suggested that Chaya may directly inhibit the protozoal population by interrupting cell wall synthesis or nucleic acid synthesis. The additional microbial reduction caused by PE may be attributed to the destruction of the cell membrane. A study by Francis et al. (2002) showed that phyto-saponins can form irreversible complexes with cholesterol in the bacterial cell membrane, which causes membrane breakdown and cell death. However, research by Śliwiński et al. (2002) demonstrated that saponin-rich products did not affect rumen fluid protozoal counts. Another study suggested that the type and origin of the PE may play a role in the differential effects against protozoa (Makkar and Becker, 1996).
Some previous studies have found that the effects of PE supplementation on rumen fermentation may be contradictory. It has been reported that flaxseed oil supplementation did not affect VFAs (Ueda et al., 2003). In another major study, Yang et al. (2022) found that adding flaxseed oil did not shift the concentration of VFAs, in the meantime, UFA proportion would change the microbial community’s diversity and abundance. Our study in experiment 2 found that adding AME can significantly decrease the concentration of MCP and increase the concentration of NH3-N, which indicated that AME may regulate rumen fermentation by changing the microflora. Additionally, we noticed that the pH was significantly increased in A4 as compared with the A1 group, which might affect the rumen BH pathways by AME supplementation. Harvatine et al. (2009) opined that typical pathways of rumen BH are altered when rumen pH is low. The low proportion of cis-9, trans-11 CLA, and trans-11 C18:1 at low pH was also observed by Troegeler-Meynadier et al. (2003). Piperova et al. (2002) observed that the low rumen pH resulted in higher trans-10 C18:1 produced and the effluent proportion of trans-10 C18:1 was positively related to BH (Bauman and Griinari, 2003). Results of the present experiment indicate that the pH is an important factor that may affect the BH process. It appears that a substantial correlation exists between AME and pH in relation to rumen BH. A possible hypothesis is that higher pH levels lead to a decrease in hydrogen ions, which are essential for hydrogenation during the process. Alternatively, an increase in pH induced by AME supplementation may potentially affect the hydrogenation process by limiting the availability of hydrogen ions in the rumen. However, additional experiments are needed to verify this hypothesis.
4.2. Effects of AME on rumen biohydrogenation bacteria
PE may have the potential to limit ruminal BH (Guerreiro et al., 2016). Published research suggests that PE causes certain changes in the rumen microflora and beneficial changes in rumen lipid metabolism (Huws et al., 2013). The inhibitory effect of PE on hydrogenation has been reported before. A study by Wood et al. (2010) suggested that PE potentially inhibits BH by acting on microorganisms. Several bacteria involved in BH function were identified: B. fibrisolvens, B. proteoclasticus, and B. crossotus (Palevich et al., 2017). Moreover, other microorganisms may participate in the process of BH, such as members of the B. fibrisolvens group, which convert LA to VA (trans-11-18:1) via cis-9, trans-11-CLA (Ramos-Morales et al., 2016).
Diet supplementation with UFAs may interfere with the BH process (Kamel et al., 2018). A study by Maia et al. (2010) suggested that UFAs play a preventive role in the BH process and disrupt the lipid bilayer structure of bacteria. Studies of other sulfur-containing PEs have also shown inhibitory effects on gram-positive bacteria. Busquet et al. (2005) suggested that the inhibitory effect of garlic oil may be mediated by inhibiting HMG-CoA reductase, which plays a role in the synthesis of isoprenoid ethers, and this process is also achieved by mediating the cell membrane (Busquet et al., 2005). A previous study showed that phenolic compounds have a toxic effect on certain rumen microbes, which contributed to the permeability of membranes and inhibited the enzyme activity of ruminal microorganisms (Jones et al., 1994). Moreover, research has shown that phenolic compounds can trigger endogenous oxidative stress in bacterial cells by inducing ROS formation, which can lead to bacteria cell death (Efenberger-Szmechtyk et al., 2021).
Kim et al. (2015) showed that rumen fluid incubated with PE (Punica granatum, Betula schmidtii, Ginkgo biloba, and Camellia japonica) decreased populations of R. albus, which is a cellulose-digesting species that has been identified as the major hydrogenating bacteria in the rumen. These bacteria benefit fungi by rapidly hydrogenating UFAs (Nam and Garnsworthy, 2007). R. albus belongs to “Type A” bacteria, which can hydrogenate C18:2n-6 and C18:3n-3, with VA being their major end product (Ishlak et al., 2015). In experiment 2, AME was added at 3 mg/g as determined by experiment 1. We observed that the abundance of R. albus was decreased in the AME treatment groups (A3, A4, A6). Interestingly, we noticed variability in the antibacterial rate between groups treated with different concentrations of the AME-UFA mixture. The low-concentration AME-UFA mixture group (A6) exhibited a higher antibacterial rate than the low-concentration UFA group (A5), but the high-concentration UFA mixture group (A2) was not as effective as the high-concentration AME-UFA group (A3). This observation suggests that AME may play an ‘adjuvant’ role in the inhibition of R. albus by supplementing UFAs.
B. proteoclasticus is a proteolytic bacterium in the rumen that produces rumenic acid (RA) together with VA and is the only species to form stearic acid (SA; Wallace et al., 2006). Another study reported that B. proteoclasticus could reduce trans-18:1 FA to SA (Ramos-Morales et al., 2013). Furthermore, several studies have also verified the association between SA and decreased B. proteoclasticus abundance (Zhu et al., 2012). A study by Henderson (1973) noted that Butyribrio sp. was most sensitive to FA. Therefore, the mixed acid used in the present study was greatly toxic to Butyribrio sp. Although the abundance of B. proteoclasticus in the A4 treatment group was not different from that in the A2, A3, A5, and A6 treatment groups, it was still significantly lower than that in the A1. In addition, the antibacterial effect of AME on B. fibrisolvens was observed. B. fibrisolvens are highly prevalent gram-positive bacteria in the rumen (Maia et al., 2010). Studies have shown that B. fibrisolvens considerably contributes to the BH of UFAs in the rumen. In our study, the antimicrobial effect of UFAs at high concentrations (A2) was more pronounced than that of the AME-UFA mixture (A3, A6), and the antimicrobial effect of the AME-FA mixture (A3, A6) was more pronounced than that of the single AME additive (A4). Despite this, the A4 group significantly inhibited (p < 0.01) the growth of B. fibrisolvens, which indicates the inhibitory potential of AME for BH in the rumen.
C. aminophilum is the typical ammonia producer in the rumen (Shen et al., 2017), which is closely related to B. proteoclasticus and involved in BH in the rumen (Moon et al., 2008). Compared to other bacteria in this study, C. aminophilum was more sensitive to the A4 treatment group and low concentrations of UFAs (A5) and AME-UFA mixtures (A3, A6). Compared to the A3, A5, and A6 treatment groups, the A4 treatment group significantly inhibited the growth of C. aminophilum (p < 0.01); however, C. aminophilum does not appear to be largely sensitive to UFAs at high concentrations (A2), and between A1 and A2 groups, there was no significant difference in microbial counts.
AME had a significant inhibitory (p < 0.01) effect on B. proteoclasticus, and the higher concentration AME-UFA mixture group (A3) was less inhibitory than the single AME treatment group (A4). However, the inhibitory effect of the lower concentration AME-UFA mixture (A6) was higher than that of the single AME treatment group (A4), and the underlying mechanism for this phenomenon is not yet clear. Above all, AME reduced the abundance of key BH bacteria. Such impacts may contribute to the production of high-quality livestock products.
5. Conclusion
Supplementation of AME could modulate ruminal fermentation in vitro of dairy cows. The suitable concentration of AME in modulating rumen fermentation is 3 mg/g, which reduces the MCP concentration significantly without compromising total VFA and tends to reduce the concentration of propionate and the population of protozoa. AME and AME with low concentration UFA mixture decreased the abundance of B. proteoclasticus, R. albus, B. fibrisolvens, and C. aminophilum. Based on our results, AME has the potential to inhibit rumen BH and thus may influence the UFA metabolism in dairy cows. However, the results need further in vivo research to confirm.
Data availability statement
The raw data supporting the conclusions of this article will be made available by the authors, without undue reservation.
Ethics statement
The animal studies were approved by Animal Ethics Committee of Inner Mongolia Agricultural University. The studies were conducted in accordance with the local legislation and institutional requirements. Written informed consent was obtained from the owners for the participation of their animals in this study.
Author contributions
XW: Data curation, Investigation, Methodology, Validation, Writing – original draft, Writing – review & editing. CB: Funding acquisition, Investigation, Methodology, Project administration, Software, Supervision, Writing – review & editing. KE: Supervision, Writing – review & editing. AU: Methodology, Project administration, Supervision, Writing – review & editing. QC: Software, Writing – review & editing. CA: Conceptualization, Funding acquisition, Methodology, Project administration, Resources, Supervision, Validation, Writing – review & editing. LJ: Formal analysis, Funding acquisition, Methodology, Project administration, Resources, Supervision, Validation, Writing – review & editing.
Funding
The author(s) declare financial support was received for the research, authorship, and/or publication of this article. This research was funded by the Program for Improving the Scientific Research Ability of Youth Teachers of Inner Mongolia Agricultural University (BR230102), the High-level achievement cultivation project of the College of Animal Science, Inner Mongolia Agricultural University (QT202220), the Open Project Program of Beijing Key Laboratory of Dairy Cow Nutrition (2020019).
Acknowledgments
We sincerely thank the Beijing University of Agriculture for providing funding and experimental instruments.
Conflict of interest
The authors declare that the research was conducted in the absence of any commercial or financial relationships that could be construed as a potential conflict of interest.
Publisher’s note
All claims expressed in this article are solely those of the authors and do not necessarily represent those of their affiliated organizations, or those of the publisher, the editors and the reviewers. Any product that may be evaluated in this article, or claim that may be made by its manufacturer, is not guaranteed or endorsed by the publisher.
References
Bauman, D. E., and Griinari, J. M. (2003). Nutritional regulation of milk fat synthesis. Annu. Rev. Nutr. 23, 203–227. doi: 10.1146/annurev.nutr.23.011702.073408
Blanch, M., Carro, M. D., Ranilla, M. J., Viso, A., Vázquez-Añón, M., and Bach, A. (2016). Influence of a mixture of cinnamaldehyde and garlic oil on rumen fermentation, feeding behavior and performance of lactating dairy cows. Anim. Feed Sci. Technol. 219, 313–323. doi: 10.1016/j.anifeedsci.2016.07.002
Broderick, G., and Craig, W. M. (1989). Metabolism of peptides and amino acids during in vitro protein degradation by mixed rumen organisms 1. J. Dairy Sci. 72, 2540–2548. doi: 10.3168/jds.S0022-0302(89)79394-2
Buccioni, A., Pauselli, M., Viti, C., Minieri, S., Pallara, G., Roscini, V., et al. (2015). Milk fatty acid composition, rumen microbial population, and animal performances in response to diets rich in linoleic acid supplemented with chestnut or quebracho tannins in dairy ewes. J. Dairy Sci. 98, 1145–1156. doi: 10.3168/jds.2014-8651
Busquet, M., Calsamiglia, S., Ferret, A., Cardozo, P. W., and Kamel, C. (2005). Effects of Cinnamaldehyde and garlic oil on rumen microbial fermentation in a dual flow continuous culture. J. Dairy Sci. 88, 2508–2516. doi: 10.3168/jds.S0022-0302(05)72928-3
Castillejos, L., Calsamiglia, S., and Ferret, A. (2006). Effect of essential oil active compounds on rumen microbial fermentation and nutrient flow in in vitro systems. J. Dairy Sci. 89, 2649–2658. doi: 10.3168/jds.S0022-0302(06)72341-4
Chaney, A. L., and Marbach, E. (1962). Modified reagents for determination of urea and Ammonia. Clin. Chem. 8, 130–132. doi: 10.1016/0009-8981(62)90080-3
Choi, N. J., Park, H. G., Kim, J. H., Hwang, H. J., Kwon, K. H., Yoon, J. A., et al. (2009). Characterizations of environmental factors in conjugated linoleic acid production by mixed rumen bacteria. J. Agric. Food Chem. 57, 9263–9267. doi: 10.1016/j.anifeedsci.2005.04.054
Cobellis, G., Yu, Z., Forte, C., Acuti, G., and Trabalza-Marinucci, M. (2016). Dietary supplementation of Rosmarinus officinalis L. leaves in sheep affects the abundance of rumen methanogens and other microbial populations. J. Anim. Sci. Biotechno. 7, 27–28. doi: 10.1186/s40104-016-0086-8
Correddu, F., Gaspa, G., Pulina, G., and Nudda, A. (2016). Grape seed and linseed, alone and in combination, enhance unsaturated fatty acids in the milk of Sarda dairy sheep. J. Dairy Sci. 99, 1725–1735. doi: 10.3168/jds.2015-10108
Costa, M., Alves, S. P., Cabo, A., Guerreiro, O., Stilwell, G., Dentinho, M. T., et al. (2017). Modulation of in vitro rumen biohydrogenation by Cistus ladanifer tannins compared with other tannin sources. J. Sci. Food Agric. 97, 629–635. doi: 10.1002/jsfa.7777
Dewhurst, R., and Moloney, A. (2013). Modification of animal diets for the enrichment of dairy and meat products with omega-3 fatty acids. Food Enrich. Omega-3 Fatty Acids 257-287. doi: 10.1533/9780857098863.3.257
Ding, H., Liu, W., Erdene, K., Du, H., and Ao, C. (2021). Effects of dietary supplementation with Allium mongolicum regel extracts on growth performance, serum metabolites, immune responses, antioxidant status, and meat quality of lambs. Anim Nutr. 7, 530–538. doi: 10.1016/j.aninu.2021.04.001
Du, X., Huang, Q., Guan, Y., Lv, M., He, X., Fang, C., et al. (2018). Caffeine promotes the conversion of palmitic acid to palmitoleic acid by inducing the expression of fat-5 in Caenorhabditis elegans and scd1 in mice. Front. Pharmacol. 9:321. doi: 10.3389/fphar.2018.00321
Durmic, Z., Mcsweeney, C. S., Kemp, G. W., Hutton, P., Wallace, R. J., and Vercoe, P. E. (2008). Australian plants with the potential to inhibit bacteria and processes involved in ruminal biohydrogenation of fatty acids. Anim. Feed Sci. Technol. 145, 271–284. doi: 10.1016/j.anifeedsci.2007.05.052
Efenberger-Szmechtyk, M., Nowak, A., and Czyzowska, A. (2021). Plant extracts rich in polyphenols: antibacterial agents and natural preservatives for meat and meat products. Crit. Rev. Food Sci. Nutr. 61, 149–178. doi: 10.1080/10408398.2020.1722060
El-Essawy, A. M., Anele, U. Y., Abdel-Wahed, A. M., Abdou, A. R., and Khattab, I. M. (2021). Effects of anise, clove, and thyme essential oils supplementation on rumen fermentation, blood metabolites, milk yield, and milk composition in lactating goats. Anim. Feed Sci. Technol. 271:114760. doi: 10.1016/j.anifeedsci.2020.114760
Francis, G., Kerem, Z., Makkar, H. P., and Becker, K. (2002). The biological action of saponins in animal systems: a review. Br. J. Nutr. 88, 587–605. doi: 10.1079/BJN2002725
Frutos, P. H., Natalello, A. G., Luciano, G., Fondevila, M., Priolo, A., and Toral, P. G. (2020). The ability of tannins to modulate ruminal lipid metabolism and milk and meat fatty acid profiles. Anim. Feed Sci. Technol. 269:114623. doi: 10.1016/j.anifeedsci.2020.114623
Griinari, J. M., Dwyer, D. A., McGuire, M. A., Bauman, D. E., Palmquist, D., and Nurmela, K. V. V. (1988). Trans-octadecenoic acids and milk fat depression in lactating dairy cows. J. Dairy Sci. 81, 1251–1261. doi: 10.3168/jds.S0022-0302(98)75686-3
Guerreiro, O., Alves, S. P., Costa, M., Cabo, Â., Duarte, M. F., Jerónimo, E., et al. (2016). Effects of extracts obtained from Cistus ladanifer L. on in vitro rumen biohydrogenation. Anim. Feed Sci. Technol. 219, 304–312. doi: 10.1016/j.anifeedsci.2016.06.024
Guo, T., Guo, T., Cao, Y., Guo, L., Li, F., Li, F., et al. (2021). Changes in the fermentation and bacterial community by artificial saliva pH in RUSITEC system. Front. Nutr. 8:760316. doi: 10.3389/fnut.2021.760316
Harvatine, K. J., Boisclair, Y. R., and Bauman, D. E. (2009). Recent advances in the regulation of milk fat synthesis. Animal 3, 40–54. doi: 10.1017/S1751731108003133
Henderson, C. (1973). The effects of fatty acids on pure cultures of rumen bacteria. J. Agric. Sci. 81, 107–112. doi: 10.1017/S0021859600058378
Huws, S. A., Scott, M. B., Tweed, J. K., and Lee, M. R. (2013). Fatty acid oxidation products ('green odour') released from perennial ryegrass following biotic and abiotic stress, potentially have antimicrobial properties against the rumen microbiota resulting in decreased biohydrogenation. J. Appl. Microbiol. 115, 1081–1090. doi: 10.1111/jam.12314
Ishlak, A., Günal, M., and Abughazaleh, A. A. (2015). The effects of cinnamaldehyde, monensin, and quebracho condensed tannin on rumen fermentation, biohydrogenation, and bacteria in continuous culture system. Anim. Feed Sci. Technol. 207, 31–40. doi: 10.1016/j.anifeedsci.2015.05.023
Jones, G., Mcallister, T., Muir, A., and Cheng, K. J. (1994). Effects of sainfoin (Onobrychis viciifolia Scop.) condensed tannins on growth and proteolysis by four strains of ruminal bacteria. Appl. Environ. Microbiol. 60, 1374–1378. doi: 10.1128/aem.60.4.1374-1378.1994
Kamel, H. E. M., Al-Dobaib, S. N., Salem, A. Z. M., López, S., and Alaba, P. A. (2018). Influence of dietary supplementation with sunflower oil and quebracho tannins on growth performance and meat fatty acid profile of Awassi lambs. Anim. Feed Sci. Technol. 235, 97–104. doi: 10.1016/j.anifeedsci.2017.11.006
Kim, E. T., Guan, L. L., Lee, S. J., Lee, S. M., and Lee, S. S. (2015). Effects of flavonoid-rich Plant extracts on in vitro ruminal Methanogenesis, microbial populations and fermentation characteristics. Asian Australas. J. Anim. Sci. 28, 530–537. doi: 10.5713/ajas.14.0692
Kozłowska, M., Cieślak, A., Jóźwik, A., El-Sherbiny, M., Gogulski, M., Lechniak, D., et al. (2021). Effects of partially replacing grass silage by lucerne silage cultivars in a high-forage diet on ruminal fermentation, methane production, and fatty acid composition in the rumen and milk of dairy cows. Anim. Feed Sci. Technol. 277:114959. doi: 10.1016/j.anifeedsci.2021.114959
Kumar, M., Kumar, V., Roy, D., Kushwaha, R., and Vaiswani, S. (2014). Application of herbal feed additives in animal nutrition-a review. Int. J. Livestock Res. 4, 1–8. doi: 10.5455/ijlr.20141205105218
Liu, W., Ding, H., Erdene, K., Chen, R., Mu, Q., Ao, C., et al. (2019). Effects of flavonoids from Allium mongolicum regel as a dietary additive on meat quality and composition of fatty acids related to flavor in lambs. Can. J. Anim. Sci. 99, 15–23. doi: 10.1139/cjas-2018-0008
Liu, J., Wang, Y., Liu, L., Ma, G., Zhang, Y., and Ren, J. (2022). Effect of Moringa leaf flavonoids on the production performance, immune system, and rumen fermentation of dairy cows. Vet. Med. 9, 917–923. doi: 10.1002/vms3.993
Maia, M. R., Chaudhary, L. C., Bestwick, C. S., Richardson, A. J., Mckain, N., Larson, T. R., et al. (2010). Toxicity of unsaturated fatty acids to the biohydrogenating ruminal bacterium, Butyrivibrio fibrisolvens. BMC Microbiol. 10:52. doi: 10.1186/1471-2180-10-52
Makkar, H., and Becker, K. (1996). Effect of quillaya saponins on in vitro rumen fermentation. In: Saponins used in food and agriculture. Exp. Biol. Med. (Maywood) 405, 387–394. doi: 10.1007/978-1-4613-0413-5_33
Menke, K. H., Raab, L., Salewski, A., Steingass, H., and Schneider, W. (1979). The estimation of the digestibility and metabolizable energy content of ruminant feedingstuffs from the gas production when they are incubated with rumen liquor in vitro. J. Agric. Sci. 93, 217–222. doi: 10.1017/S0021859600086305
Moon, C. D., Pacheco, D. M., Kelly, W. J., Leahy, S. C., Li, D., Kopecny, J., et al. (2008). Reclassification of Clostridium proteoclasticum as Butyrivibrio proteoclasticus comb. nov., a butyrate-producing ruminal bacterium. Int. J. Syst. Evol. Microbiol. 58, 2041–2045. doi: 10.1099/ijs.0.65845-0
Nam, I. S., and Garnsworthy, P. C. (2007). Biohydrogenation of linoleic acid by rumen fungi compared with rumen bacteria. J. Appl. Microbiol. 103, 551–556. doi: 10.1111/j.1365-2672.2007.03317.x
Naseri, V., Kafilzadeh, F., and Jahani-Azizabadi, H. (2022). Effects of Pistacia atlantica gum essential oil on ruminal methanogen, protozoa, selected bacteria species and fermentation characteristics in sheep. Small Rumin. Res. 209:106650. doi: 10.1016/j.smallrumres.2022.106650
Olijhoek, D. W., Hellwing, A. L. F., Grevsen, K., Haveman, L. S., Chowdhury, M. R., Lovendahl, P., et al. (2019). Effect of dried oregano (Origanum vulgare L.) plant material in feed on methane production, rumen fermentation, nutrient digestibility, and milk fatty acid composition in dairy cows. J. Dairy Sci. 102, 9902–9918. doi: 10.3168/jds.2019-16329
Paillard, D., Mckain, N., Chaudhary, L. C., Walker, N. D., Pizette, F., Koppova, I., et al. (2007). Relation between phylogenetic position, lipid metabolism, and butyrate production by different Butyrivibrio-like bacteria from the rumen. Antonie Van Leeuwenhoek 91, 417–422. doi: 10.1007/s10482-006-9121-7
Palevich, N., Kelly, W. J., Leahy, S. C., Altermann, E., Rakonjac, J., and Attwood, G. T. (2017). The complete genome sequence of the rumen bacterium Butyrivibrio hungatei MB2003. Stand. Genomic Sci. 12:72. doi: 10.1186/s40793-017-0285-8
Pan, L., Bu, D., Wang, J., Cheng, J., Sun, X., and Zhou, L. (2014). Effects of Radix Bupleuri extract supplementation on lactation performance and rumen fermentation in heat-stressed lactating Holstein cows. Anim. Feed Sci. Technol. 187, 1–8. doi: 10.1016/j.anifeedsci.2013.09.008
Pang, K., Chai, S., Yang, Y., Wang, X., Liu, S., and Wang, S. (2022). Dietary forage to concentrate ratios impact on yak ruminal microbiota and metabolites. Front. Microbiol. 13:964564. doi: 10.3389/fmicb.2022.964564
Pena-Bermudez, Y. A., Lobo, R. R., De Amorim, T. R., Rojas-Moreno, D. A., Rodriguez-Aguilar, D., Poleti, M. D., et al. (2022). Effect of yerba mate (Ilex paraguariensis) in lamb diets on fatty acid profile, physical and sensory characteristics of the longissimus muscle. Livest. Sci. 265:105095. doi: 10.1016/j.livsci.2022.105095
Piperova, L. S., Sampugna, J., Teter, B. B., Kalscheur, K. F., Yurawecz, M. P., Ku, Y., et al. (2002). Duodenal and milk trans octadecenoic acid and conjugated linoleic acid (CLA) isomers indicate that postabsorptive synthesis is the predominant source of cis-9-containing CLA in lactating dairy cows. J. Nutr. 132, 1235–1241. doi: 10.1093/jn/132.6.1235
Ramos-Morales, E., Martínez-Fernández, G., Abecia, L., Martin-García, A. I., Molina-Alcaide, E., and Yáñez-Ruiz, D. R. (2013). Garlic-derived compounds modify ruminal fatty acid biohydrogenation and induce shifts in the Butyrivibrio community in continuous-culture fermenters. Anim. Feed Sci. Technol. 184, 38–48. doi: 10.1016/j.anifeedsci.2013.05.017
Ramos-Morales, E., Mckain, N., Gawad, R. M. A., Hugo, A., and Wallace, R. J. (2016). Vernonia galamensis and vernolic acid inhibit fatty acid biohydrogenation in vitro. Anim. Feed Sci. Technol. 222, 54–63. doi: 10.1016/j.anifeedsci.2016.10.002
Santos, G. T., Lima, L. S., Schogor, A. L. B., Romero, J. V., De Marchi, F. E., Grande, P. A., et al. (2014). Citrus pulp as a dietary source of antioxidants for lactating Holstein cows fed highly polyunsaturated fatty acid diets. Asian Australas. J. Anim. Sci. 27, 1104–1113. doi: 10.5713/ajas.2013.13836
Shen, J., Liu, Z., Yu, Z., and Zhu, W. (2017). Monensin and Nisin affect rumen fermentation and microbiota differently in vitro. Front. Microbiol. 8:1111. doi: 10.3389/fmicb.2017.01111
Śliwiński, B., Soliva, C. R., Machmüller, A., and Kreuzer, M. (2002). Efficacy of plant extracts rich in secondary constituents to modify rumen fermentation. Anim. Feed Sci. Technol. 101, 101–114. doi: 10.1016/S0377-8401(02)00139-6
Soltan, Y. A., Natel, A. S., Araujo, R. C., Morsy, A. S., and Abdalla, A. L. (2018). Progressive adaptation of sheep to a microencapsulated blend of essential oils: ruminal fermentation, methane emission, nutrient digestibility, and microbial protein synthesis. Anim. Feed Sci. Technol. 237, 8–18. doi: 10.1016/j.anifeedsci.2018.01.004
Spanghero, M., Braidot, M., Fabro, C., and Romanzin, A. (2022). A meta-analysis on the relationship between rumen fermentation parameters and protozoa counts in in vitro batch experiments. Anim. Feed Sci. Technol. 293:115471. doi: 10.1016/j.anifeedsci.2022.115471
Toral, P. G., Hervás, G., Bichi, E., Belenguer, Á., and Frutos, P. (2011). Tannins as feed additives to modulate ruminal biohydrogenation: effects on animal performance, milk fatty acid composition, and ruminal fermentation in dairy ewes fed a diet containing sunflower oil. Anim. Feed Sci. Technol. 164, 199–206. doi: 10.1016/j.anifeedsci.2011.01.011
Totakul, P., Viennasay, B., Sommai, S., Matra, M., Infascelli, F., and Wanapat, M. (2022). Chaya (Cnidoscolus aconitifolius, mill. Johnston) pellet supplementation improved rumen fermentation, milk yield, and milk composition of lactating dairy cows. Livest. Sci. 262:104974. doi: 10.1016/j.livsci.2022.104974
Troegeler-Meynadier, A., Nicot, M. C., Bayourthe, C., Moncoulon, R., and Enjalbert, F. (2003). Effects of pH and concentrations of linoleic and linolenic acids on extent and intermediates of ruminal biohydrogenation in vitro. J. Dairy Sci. 86, 4054–4063. doi: 10.3168/jds.S0022-0302(03)74017-X
Ueda, K., Ferlay, A., Chabrot, J., Loor, J., Chilliard, Y., and Doreau, M. (2003). Effect of linseed oil supplementation on ruminal digestion in dairy cows fed diets with different forage: concentrate ratios. J. Dairy Sci. 86, 3999–4007. doi: 10.3168/jds.S0022-0302(03)74011-9
Vakili, A. R., Khorrami, B., Mesgaran, M. D., and Parand, E. (2013). The effects of thyme and cinnamon essential oils on performance, rumen fermentation, and blood metabolites in Holstein calves consuming high concentrate diet. Asian Australas. J. Anim. Sci. 26, 935–944. doi: 10.5713/ajas.2012.12636
Vasta, V., Yáñez-Ruiz, D. R., Mele, M., Serra, A., Luciano, G., Lanza, M., et al. (2010). Bacterial and protozoal communities and fatty acid profile in the rumen of sheep fed a diet containing added tannins. Appl. Environ. Microbiol. 76, 2549–2555. doi: 10.1128/AEM.02583-09
Wallace, R. J., Chaudhary, L. C., Mckain, N., Mcewan, N. R., Richardson, A. J., Vercoe, P. E., et al. (2006). Clostridium proteoclasticum: a ruminal bacterium that forms stearic acid from linoleic acid. University Press. 265, 195–201. doi: 10.1111/j.1574-6968.2006.00487.x
Watkins, P. J., and Frank, D. (2019). Heptadecanoic acid as an indicator of BCFA content in sheep fat. Meat Sci. 151, 33–35. doi: 10.1016/j.meatsci.2019.01.005
Wood, T. A., Ramos-Morales, E., Mckain, N., Shen, X., Atasoglu, C., and Wallace, R. J. (2010). Chrysanthemum coronarium as a modulator of fatty acid biohydrogenation in the rumen. Anim. Feed Sci. Technol. 161, 28–37. doi: 10.1016/j.anifeedsci.2010.07.016
Xie, Z., Mclean, R., and Marshall, M. (2018). Dietary sodium and other nutrient intakes among patients undergoing hemodialysis in New Zealand. Nutrients 10:502. doi: 10.3390/nu10040502
Yang, Z., Liu, S., Xie, T., Wang, Q., Wang, Z., Yang, H., et al. (2022). Effect of unsaturated fatty acid ratio in vitro on rumen fermentation, methane concentration, and microbial profile. Fermentation. 8:540. doi: 10.3390/fermentation8100540
Yuan, J., and Wan, X. (2019). Multiple-factor associative effects of peanut shell combined with alfalfa and concentrate determined by in vitro gas production method. Czeh J. Anim. Sci. 64, 352–360. doi: 10.17221/94/2019-CJAS
Zhao, Y. X., Khas, E., Ao, C. J., Bao, Z. B., Du, H. X., Fan, Z. J., et al. (2021). Effects of Allium mongolicum regel and its extracts supplementation on the growth performance, carcass parameters and meat quality of sheep. Ital. J. Anim. Sci. 20, 1899–1908. doi: 10.1080/1828051X.2021.1971572
Zhao, Y., Yu, S., Zhao, H., Li, L., Li, Y., and Tu, Y. (2022a). Lipidomic profiling using GC and LC-MS/MS revealed the improved milk quality and lipid composition in dairy cows supplemented with citrus peel extract. Food Res. Int. 161:111767. doi: 10.1016/j.foodres.2022.111767
Zhao, Y., Zhang, Y., Bai, C., Ao, C., Qi, S., Cao, Q., et al. (2022b). Effects of the dietary inclusion of Allium mongolicum regel extract on serum index and meat quality in small-tailed Han sheep. Animals (Basel). 13:110. doi: 10.3390/ani13010110
Zhao, Y., Zhang, Y., Khas, E., Ao, C., and Bai, C. (2022c). Effects of Allium mongolicum regel ethanol extract on three flavor-related rumen branched-chain fatty acids, rumen fermentation, and rumen bacteria in lambs. Front. Microbiol. 13:978057. doi: 10.3389/fmicb.2022.978057
Keywords: Allium mongolicum regel, rumen fermentation, rumen hydrogenation, dairy cows, in vitro
Citation: Wang X, Bai C, Khas Erdene, Umair AM, Cao Q, Ao C and Jiang L (2023) Potential modulating effects of Allium mongolicum regel ethanol extract on rumen fermentation and biohydrogenation bacteria of dairy cows in vitro. Front. Microbiol. 14:1272691. doi: 10.3389/fmicb.2023.1272691
Edited by:
Caihong Hu, Zhejiang University, ChinaCopyright © 2023 Wang, Bai, Khas Erdene, Umair, Cao, Ao and Jiang. This is an open-access article distributed under the terms of the Creative Commons Attribution License (CC BY). The use, distribution or reproduction in other forums is permitted, provided the original author(s) and the copyright owner(s) are credited and that the original publication in this journal is cited, in accordance with accepted academic practice. No use, distribution or reproduction is permitted which does not comply with these terms.
*Correspondence: ChangJin Ao, changjinao@aliyun.com; LinShu Jiang, jls@bua.edu.cn
†These authors have contributed equally to this work