- 1Cigar Fermentation Technology Key Laboratory of China Tobacco (China Tobacco Sichuan Industrial Co., Ltd.), Cigar Technology Innovation Center of China Tobacco, Chengdu, China
- 2Industrial Efficient Utilization of Domestic Cigar Tobacco Key Laboratory of Sichuan Province, Shifang, China
- 3Science Center for Future Foods, Jiangnan University, Wuxi, China
Introduction: Adding a fermentation medium is an effective way to improve the quality of cigar tobacco leaves.
Methods: A novel microbial fermentation medium produced by an edible medicinal fungus, Tremella aurantialba SCT-F3 (CGMCC No.23831) was used to improve the quality of cigar filler leaves (CFLs). Changes in sensory quality, chemical components, volatile flavor compounds (VFCs), and the structure and function of microbes were investigated during the fermentation process.
Results: The sensory quality of CFLs supplemented with the T. aurantialba SCT-F3 fermentation medium significantly improved. Adding the fermentation medium increased the total alkaloid, reducing sugar, total sugar, and 12 VFCs significantly. A total of 31 microbial genera were significantly enriched, which increased the microbial community’s richness and diversity. Microbial functions increased, including nucleotide biosynthesis, amino acid biosynthesis, fatty acid and lipid biosynthesis, nicotine degradation, and nicotinate degradation. During fermentation, the total alkaloid, reducing sugar, and total sugar content decreased. The richness and diversity of the microbial community decreased, whereas bacterial enzyme activity increased. At the end of fermentation, the sensory quality was excellent. The microbial structure gradually stabilized, and functional genes were low. The contents of the four Maillard reaction products and three nicotine degradation products increased significantly. 2-Ethyl-6-methylpyrazine, methylpyrazine, D,L-anatabine, β-nicotyrine, nicotinic degradation products, and total nitrogen were significantly and positively correlated with sensory quality. Methylpyrazine, D,L-anatabine, and β-nicotyrine were negatively correlated with Luteimonas, Mitochondria, Paracoccus, Stemphylium, and Stenotrophomonas.
Conclusion: This research provides not only a new microbial fermentation medium that utilizes edible and medicinal fungi to improve the quality of fermented CFLs, but also new ideas for the development and application of other edible medicinal fungi to improve the quality of cigar tobacco leaves.
1. Introduction
A cigar is a tobacco product made from fermented tobacco leaves and comprises three parts: a wrapper, binder, and filler. The wrapper determines the cigar’s appearance, and the binder fixes the position of the filler. As the most important component accounts for approximately 75% of the cigar’s weight, filler determines the style of the cigar. Fillers, rich in aroma and flavor, are essential for rolling superior-quality cigars. Fermentation is an important process to improve the quality of cigar filler leaves (CFLs). There are two fermentation methods for cigar tobacco leaves: spontaneous fermentation and artificial fermentation. When spontaneous fermentation using only microorganisms in the natural environment cannot meet the quality requirements of the product, fermentation media need to be artificially added to significantly improve the fermentation quality (China Tobacco Sichuan Industrial Co., Ltd., 2021). Traditional fermentation media include not only plant extracts, Fritillaria cirrhosa tincture, coffee tincture, cocoa tincture, holly gum, jujube juice, and cane sugar, but also fermented products, such as red rice (rice fermented by Monascus), sweet rice (rice fermented by Rhizopus), rice wine, and loquat wine (Jin, 1988; Cai et al., 2022; Hu et al., 2022; Zong et al., 2023). Novel fermentation media, strains such as Bacillus cereus, B. pumilus, Acinetobacter, and Candida, and enzymes such as proteases, amylases, cellulases, and pectinases, have been shown to improve the quality of CFLs (Li et al., 2012; Zhang et al., 2020a,b; Zheng et al., 2022a,b; Jia et al., 2023).
Tremella aurantialba SCT-F3 was a colloidal edible medicinal fungus isolated from Naematelia aurantialba (Bandoni & M. Zang) Millanes & Wedin. The fermentation broth of T. aurantialba was found to be rich in polysaccharides (Zhu et al., 2011; Deng et al., 2016; Sun et al., 2020), cellulose, hemicellulose, amylase, pectinase, protease, and lignin degradation enzymes (Yang et al., 2021). Polysaccharides are strong hydrophilic compounds with excellent moisture adsorption and retention capacities, which can improve tobacco’s moisture stability and ultimately improve tobacco production quality (Lin et al., 2020). In addition, the fermentation broth of T. aurantialba SCT-F3 has a complex aroma. It produces volatile aromatic components, including ethyl acetate, isobutanol, isoamylol, 2-methylbutan-1-ol, methyl isovalerate, furfural, methyl furan-3-carboxylate, 2-ethylhexanol, methyl benzoate, ethyl benzoate, methyl cinnamate, and globulol (Supplementary Table S1). We speculated that the fermentation broth of T. aurantialba may be a new fermentation medium that could improve the quality of ordinary CFLs.
In this study, we added the fermentation broth of T. aurantialba into CFLs to improve the quality. After fermentation, changes in sensory quality, chemical components, volatile flavor compounds (VFCs), and the structure and function of microbes were investigated, and the contributions of chemical components and VFCs to sensory quality and correlation analysis of the predominant microbes and VFCs were analyzed.
2. Materials and methods
2.1. Strain
Tremella aurantialba SCT-F3 (CGMCC No.23831) was isolated from Naematelia aurantialba (Bandoni & M. Zang) Millanes & Wedin and deposited at the China General Microbiological Culture Collection Center.
2.2. CFL fermentation
The fermentation medium was potato glucose broth containing 6 g/L potato extract powder and 20 g/L glucose and was autoclaved at 121°C for 15 min. T. aurantialba SCT-F3 was inoculated by sterile loops in 250-mL flasks with 75 mL fermentation medium and cultured at 150 rpm/28°C for 5 days. The fermented potato glucose broth was centrifuged (5,000 × g for 10 min) to remove mycelium pellets, and the supernatant was used as the fermentation medium. Unfermented potato glucose broth was used as the unfermented medium. Dexue No.1 from the original Chinese tobacco drying strips was used in this study. CFLs were loosened and spread flat on the table by hand. Mixed the medium and pure water in a ratio of 1:3 (v/v), then the mixture was evenly sprayed using an electronic sprayer on the surface of the leaves with 20% inoculation amount (v/g). After 2 h, the medium and water were absorbed entirely by the CFLs. The CFLs were transferred to an oak barrel for fermentation. The oak barrels were placed in the fermentation room, the temperature of the fermentation room was controlled at 37°C, the relative humidity of the fermentation room was controlled at 75%, and the fermentation was carried out for 40 days. CFLs added with T. aurantialba SCT-F3 fermented medium were sampled at 0, 20, and 40 days and marked as J0, J20, and J40; CFLs added with unfermented potato glucose broth fermented were sampled at 0, 20, and 40 days and marked as P0, P20, and P40; uninoculated CFLs were marked as Control. Samples were taken from the four corners and central position of the oak barrel, and the five positions of the CLTs were ground and mixed for testing. All samples were mixed well, transferred into sterile bags, and stored at −20°C until further analysis. All experiments were performed in triplicates.
2.3. Sensory evaluation
The fermented CFLs were stemmed and dried to 15 ± 2% moisture content. The samples were hand-rolled to a length of 110 mm and a circumference of 47 mm, then placed in a temperature humidity chamber at a temperature of 20 ± 2°C and humidity of 62 ± 3% to balance the samples’ moisture to 13 ± 1%. Five assessors from the staff trained by the China National Tobacco Quality Supervision & Test Center evaluated the sensory quality of all samples to recognize the aroma, smoke, aftertaste, combustibility, and comfort level. The samples were identified and scored according to 0–9 points, ranging from weak to strong.
2.4. Chemical components analysis
The chemical components of total alkaloids, total nitrogen, reducing sugars, and total sugars in the CFLs were determined according to the continuous flow (potassium thiocyanate) method of the Tobacco Industry Standard for the determination of total alkaloids (YC/T 468–2013), total nitrogen (YC/T 161–2002), and water-soluble sugar (YC/T 159–2019) (Hu et al., 2022).
2.5. VFC analysis
VFCs in CFLs were assayed using headspace solid-phase microextraction coupled with gas chromatography–mass spectrometry (HS-SPME-GC–MS) as previously described (Zheng et al., 2022a,b). A 50/30 μm DVB/CAR/PMDS fiber (Supelco Inc., United States) was used for aroma extraction. The CFLs were pulverized using a grinder, and the powder (1.5 g) was placed in a 10–mL glass vial. The sample was extracted at 60°C for 30 min, and the fiber was inserted into the injection port of the GC device at 250°C for 1 min to desorb the analytes. The sample was analyzed on a DB-5MS column (60 m × 0.25 mm × 0.25 μm, Agilent Technologies, United States). The GC oven temperature was maintained at 40°C for 2 min, followed by an increase of 10°C/min to 250°C and then held for 5 min. Helium (purity: 99.999%) was used as the carrier gas at a constant flow of 1 mL/min. The mass spectrometer conditions were: EI voltage, 70 eV; ion source temperature, 300°C; mass range, m/z 35–350. The identification of volatile compounds in the extracts was based on a comparison of the mass spectra and retention times of the individual compounds with those of standard compounds deposited in the National Institute of Standards and Technology database in the MS device (SI and RSI ≥800). Peak areas were compared with those of the internal standard for quantification.
2.6. Microbial community analysis
Samples (5.0 g) were suspended in 250 mL sterile phosphate buffer saline and shaken for 2 h at 200 rpm, after which the supernatant was centrifuged (10,000 × g for 30 min). The total genomic DNA of each sample was extracted using an EZNA® Soil DNA Kit (Omega, United States) according to the manufacturer’s instructions. The bacterial genomic DNA were amplified and sequenced using primers 515F and 907R for 16S rRNA genes (Parada et al., 2016). The fungal genomic DNA was amplified using primers ITS1F and ITS2R for internal transcribed spacer (ITS) genes (Usyk et al., 2017). Amplicons were pooled in equal amounts and sequenced using a 2 × 300 paired-end configuration and an Illumina MiSeq sequencing system (Illumina, United States). Operational taxonomic units (OTUs) of qualified sequences were identified using the clustering program VSEARCH version 1.9.6 and the SILVA version 132 database (Rognes et al., 2016) with 97% similarity. Alpha diversity, including the Chao1, Shannon, and Simpon values, was analyzed using QIIME version 1.9.1 (Caporaso et al., 2010). Phylogenetic Investigation of Communities by Reconstruction of Unobserved States (PICRUSt2) and Fungi Functional Guild (FUNGuild) were used to predict metagenomic functions based on normalized OTU tables (Douglas et al., 2019; Xie et al., 2021).
2.7. Statistical analysis
All experiments were repeated at least thrice. SPSS version 19 software (SPSS Inc., United States) was used to carry out a one-way analysis of variance and Duncan’s multiple comparison test (p < 0.05). Heat maps and cluster analyses were performed using R version 4.0.0. Galaxy 1 was used for the linear discriminant analysis of effect size (LEfSe) to assess significant differences in CFLs with different treatments. SIMCA-P version 13.0 software (Umetrics, Sweden) enabled a partial least-squares (PLS) analysis of the contributions of conventional chemical constituents and VFCs to the sensory evaluation. Additionally, to determine the correlation between representative microbes and core VFCs based on Spearman’s correlation coefficients (p < 0.05), network analysis was performed using the Gephi software.
3. Results
3.1. Results of sensory evaluation
Seven CFLs were evaluated and scored according to the cigar evaluation criteria. The detailed evaluation scores for each sample are shown in Figure 1. The scores of the fermented CFLs were higher than those of the control, and as fermentation progressed, the quality of the CFLs improved gradually. Except for combustibility, other qualities were significantly improved (p < 0.05) in those with T. aurantialba SCT-F3 fermentation medium (J0, J20, and J40) compared to those in potato glucose broth (P0, P20, and P40). Specifically, aroma mellow, richness, maturity, and sweetness increased; irritation decreased, and balance sense improved.
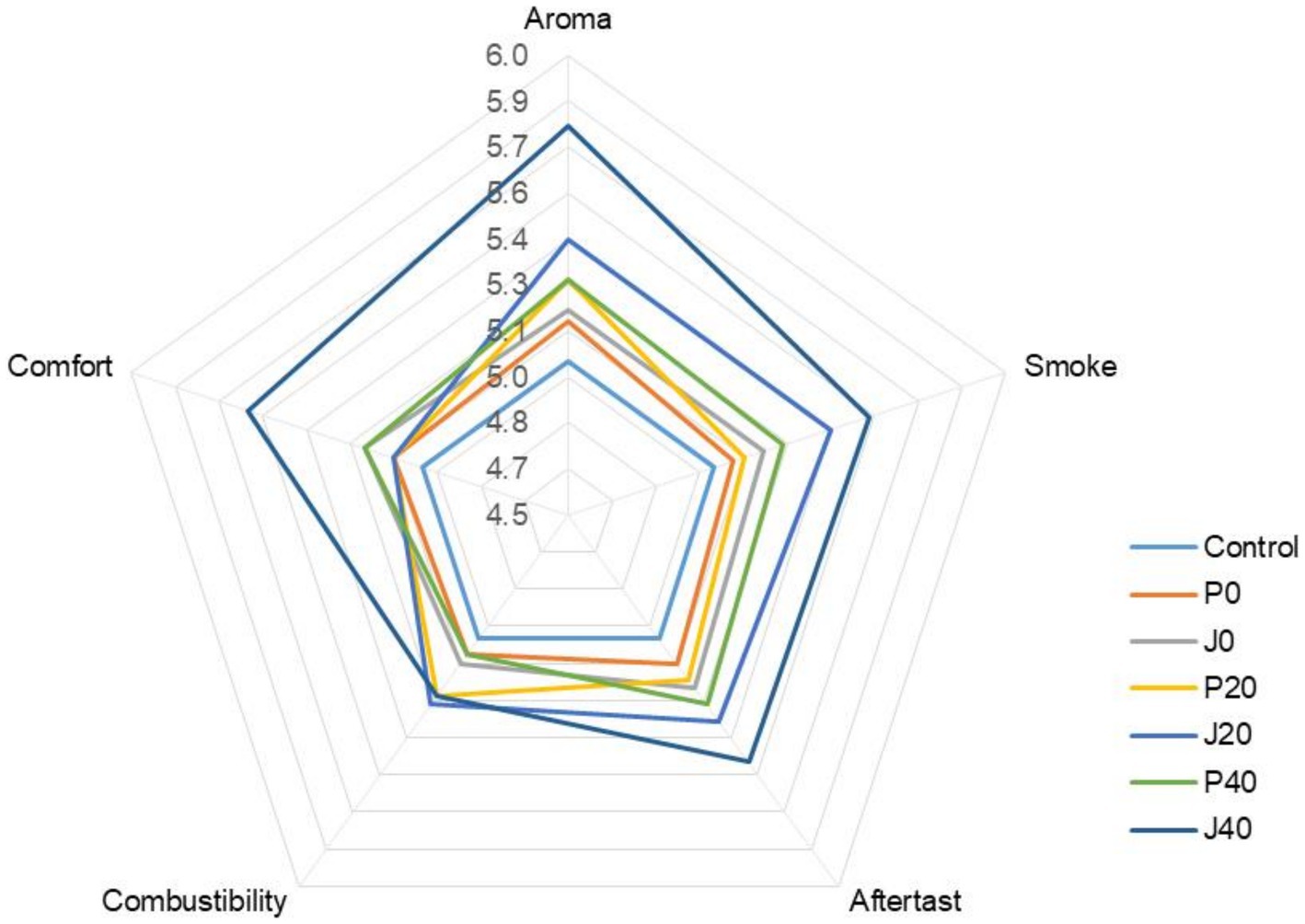
Figure 1. Plot of the sensory score of cigar filler leaves (CFLs). J0, J20, and J40 denote CFLs added with T. aurantialba SCT-F3 fermented medium at 0, 20, and 40 days; P0, P20, and P40 denote CFLs added with unfermented potato glucose broth fermented at 0, 20, and 40 days; Control denotes uninoculated CFLs.
3.2. Profiles of chemical component
During fermentation, the contents of total alkaloid, total nitrogen, total sugar and reducing sugar fluctuated from 1.8–2.8%, 4.3–5.4%, 0.2–1.7% and 0.2–1.4%, respectively. The total alkaloid, reducing sugar, and total sugar contents significantly increased (p < 0.05) in samples supplemented with fermentation media on day 0 (F0 and P0), as shown in Figure 2. They then decreased to their lowest levels on day 20 (J20 and P20) and gradually increased (J40 and P40). The total nitrogen content at 20 and 40 days (J20, P20, J40, and P40) was higher than that on day 0 (J0 and P0) and in control.
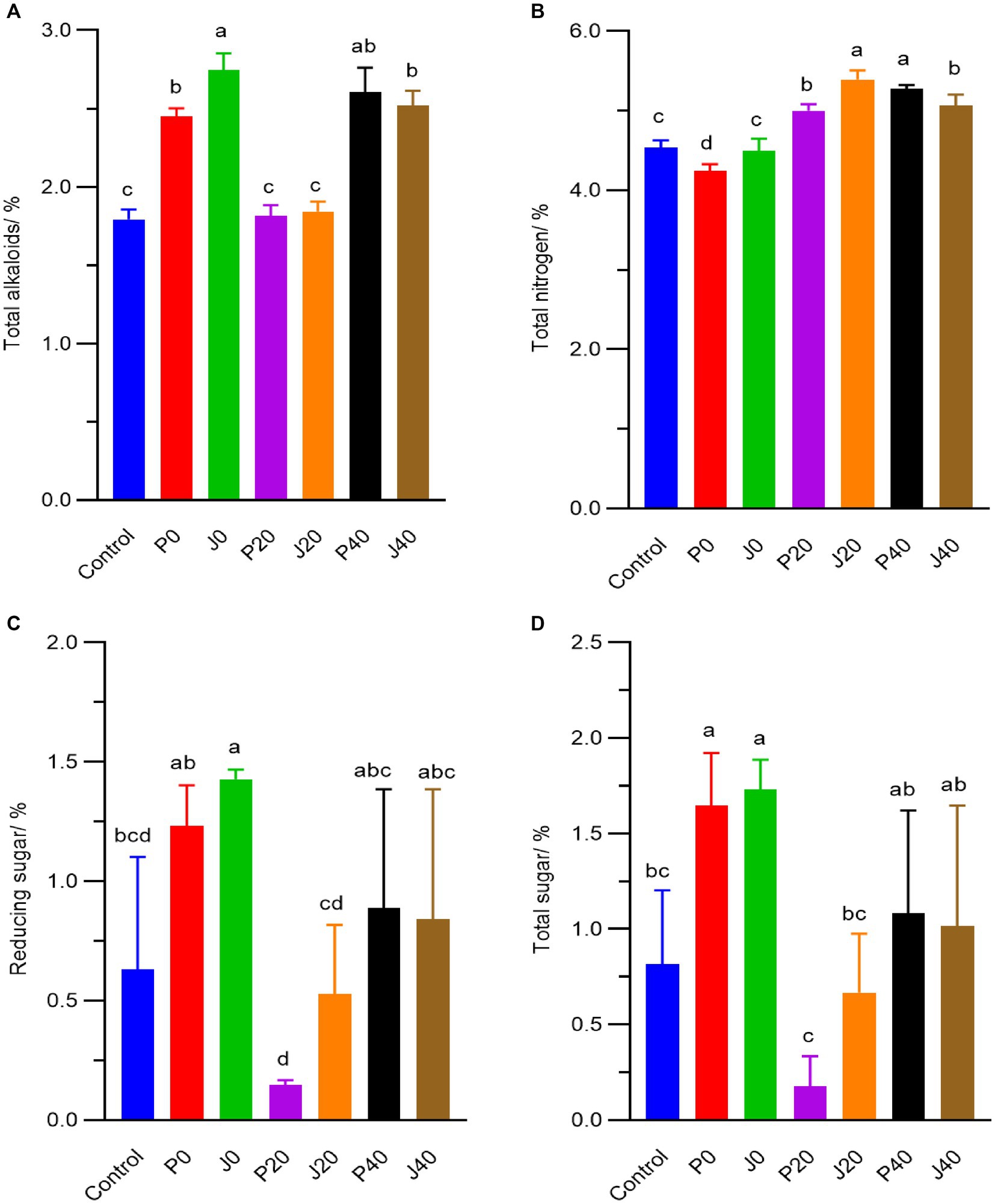
Figure 2. Contents of (A) total alkaloids, (B) total nitrogen, (C) reducing sugar, (D) total sugar of CFLs.
3.3. Profiles of VFCs
In total, 188 volatile components were detected in the CFLs, including 32 ketones, 20 aldehydes, 11 alcohols, and 10 esters. Furthermore, 34 VFCs (Figure 3), five aromatic amino acid degradation products, six plastochrome degradation products, one cembrane degradation product, 10 Maillard reaction products, and four nicotine degradation products were selected for further analysis. These compounds have been reported to have different aromas that may play important roles in the aroma profiles of cigars. The contents of aromatic amino acid degradation products, plastochrome degradation products, and cembrane degradation product, decreases gradually with fermentation, whereas four Maillard reaction products and 3 nicotine degradation products increased.
Cluster analysis divided the unfermented (control, P0, and J0) and fermented (J20, P20, J40, and P40) CFLs into two clusters, and the fermented CFLs were subdivided into two groups: P20 and J20, P40 and J40. The addition of fermentation medium caused a decrease in eight VFCs and an increase in 14 VFCs. Compared to the control, the 12 components of J0 increased. Styrene and 2-methyl-propanal levels peaked at J20. The vast majority of the VFCs changed after fermentation. Compared to the control, 15 VFCs in J40 decreased, 13 VFCs increased, 18 VFCs in P40 decreased, and 11 VFCs increased. Specifically, 2,3-butanedione, methylpyrazine, 2,6-dimethylpyrazine, 2-ethyl-6-methylpyrazine, β-nicotyrine, D,L-anatabine, cotinine, and ethyl acetate were enriched on day 40.
3.4. Contributions of chemical components and VFCs to sensory
PLS was performed on scaled values of chemical constituents and VFCs as X-variables and on scaled values of total sensory evaluation scores as Y-variables for correlations. Two significant principal components of the total variance in the data matrix were extracted. The R2X (cum), R2Y (cum), and Q2 (cum) were 0.522, 0.808, and 0.701, respectively, which meant that 52.2% variation was due to these two components, with a total of 80.8% dummy Y-variables per class and 70.1% overall cross-validated R2 for these two components. The data indicated that the PLS model was suitable for this study. The distributions of the samples in the first and second components of the statistical analysis are shown in Figure 4. Groups J0 and P0 are located on the right side of the plot, J20 and P20 on the lower left, and J40 and P40 on the upper left. Table 1 shows that 14 variables with a VIP higher than 1.0 were important in the first and second most significant principal components. 2-ethyl-6-methylpyrazine, methylpyrazine, D,L-anatabine, β-nicotyrine, nicotinic degradation products, and total nitrogen were significantly positively correlated with the total sensory evaluation scores. In contrast, 1-phenylethanol, geranylacetone, megastigmatonone, D-solanone, 6-methyl-3,5-heptadiene-2-one, Maillard reaction products, 1,2-dimethoxybenzene, and γ-caprolactone were significantly negatively correlated with the total sensory evaluation scores.
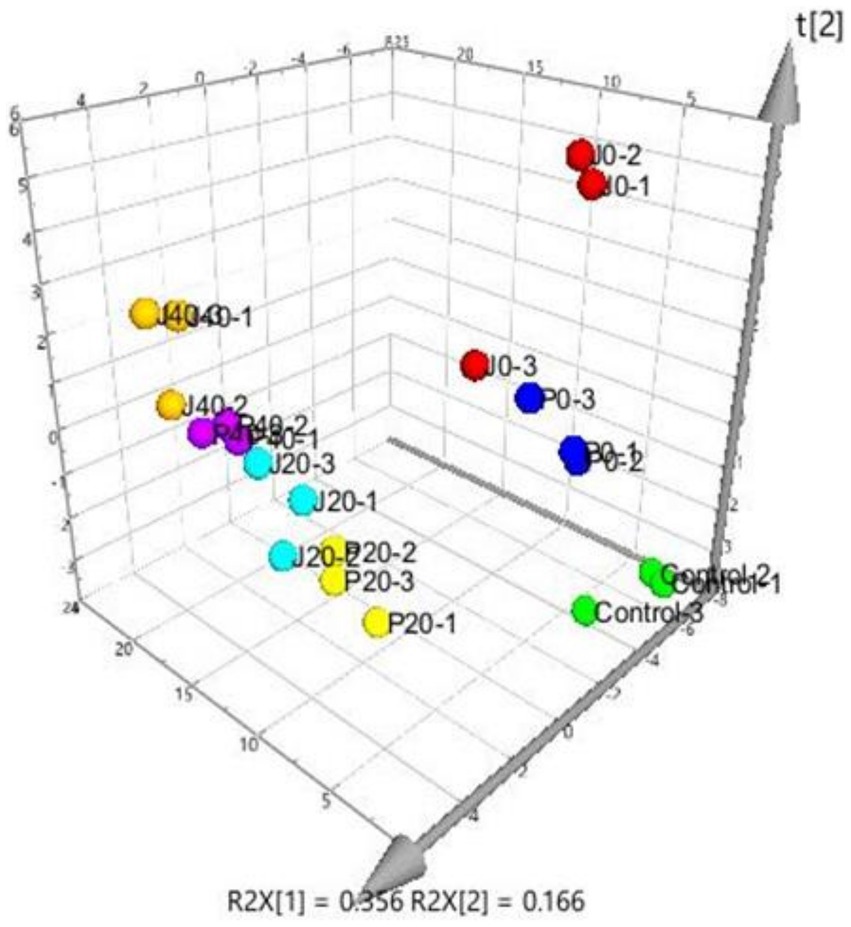
Figure 4. Score scatter three-dimensional plots of the partial least-squares regression (PLS). PLS of various CFLs is represented as a two-dimensional representation of the scores (t[1] and t[2]) on the first and second PLS components.
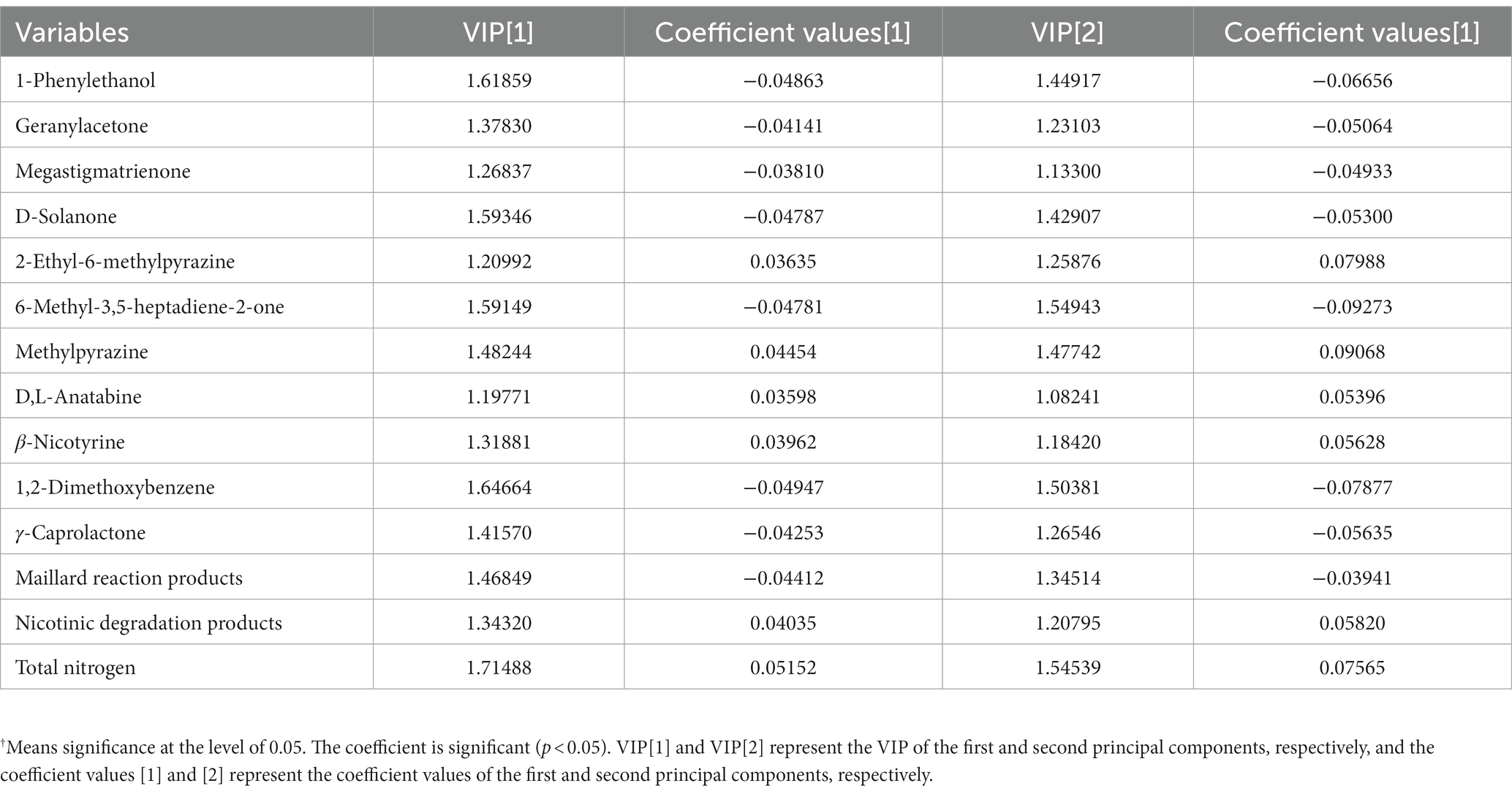
Table 1. VIP values and correlation coefficients between variables and total score of sensory evaluation†.
3.5. Overview of microbial community
Figure 5A shows alpha diversity indices, including the Chao1, Shannon, and Simpson indices, of bacterial and fungal communities in CFLs. The Chao1, Shannon, and Simpson values of the bacterial communities were higher than those of the fungal communities, indicating that the richness and diversity of the bacterial communities were generally higher than those of the fungal communities. The Chao1 values of J0 were higher than those of others, indicating that the richness of the bacterial and fungal communities increased after adding the T. aurantialba SCT-F3 fermentation medium. The Shannon and Simpson values of microbes in CFLs with medium without fermentation (P0 and J0) were higher than those in the other samples, indicating that adding fermentation medium increased the diversity of the microbial community. As fermentation progressed, fungal alpha diversity indices decreased.
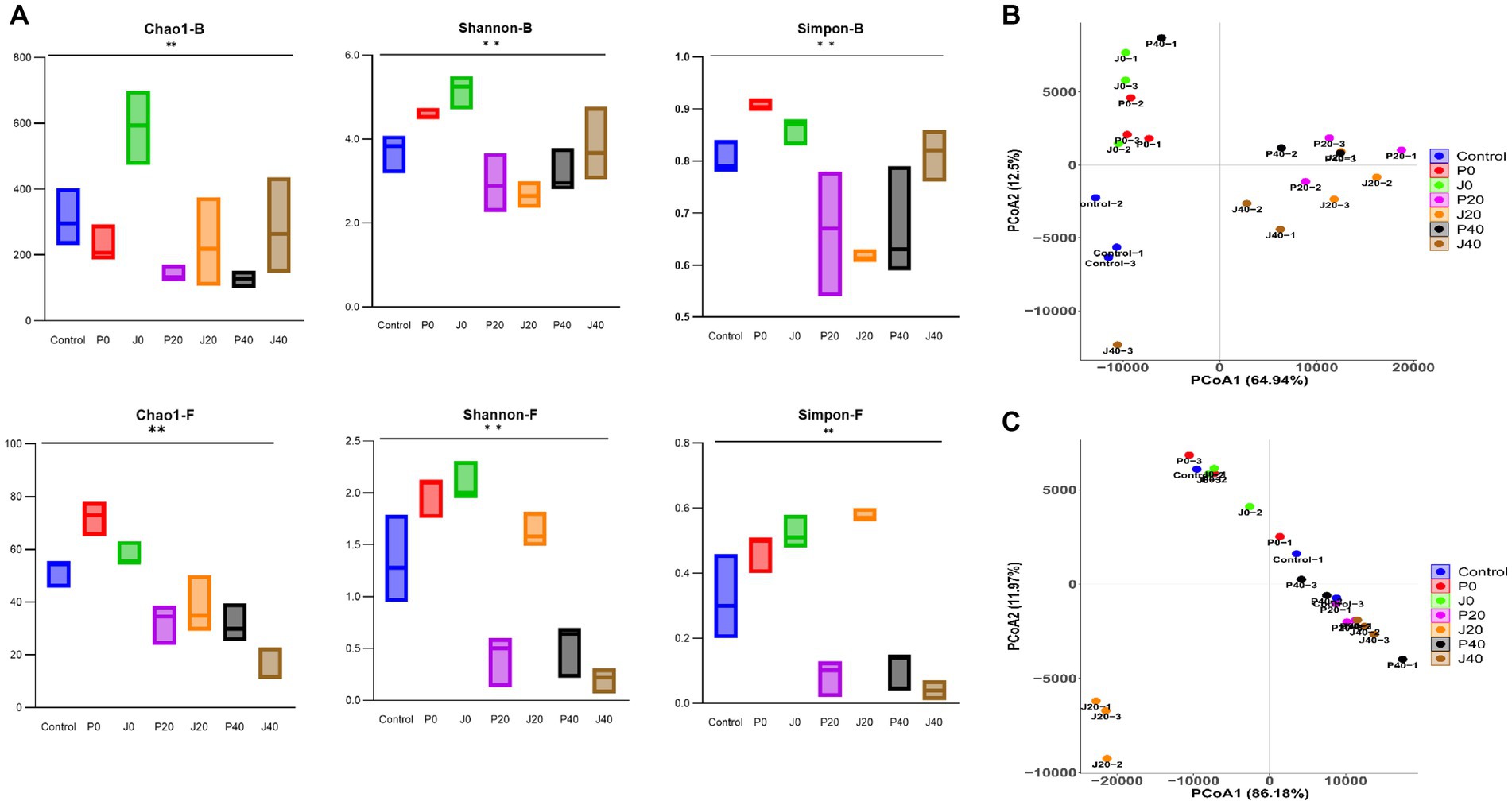
Figure 5. Diversity of microbial communities in CFLs. Alpha diversity was determined based on the Chao1, Shannon, and Simpson indices (A). Bacterial beta diversity (B) and fungal beta diversity (C) were measured by weighted UniFrac distance. **means significance at the level of 0.01.
Unconstrained principal coordinate analysis of weighted UniFrac distance revealed that the microbiota of CFLs with different treatments explained 77.4% of the bacterial variance (Figure 5B) and 98.1% of the fungal variance (Figure 5C). The addition of the fermentation medium altered the structure of the bacterial and fungal communities. The fungal community structure of sample J20 significantly differed from the other samples.
3.6. Changes in the structure of microbial flora
The abundance of bacterial and fungal taxa is shown in Figure 6. The dominant bacterial phyla were Bacillota and Pseudomonadota, and the dominant fungal phyla were Ascomycota and Basidiomycota (Figures 6A,B). Pseudomonadota and Ascomycota of unfermented samples (control, P0, and J0) were significantly higher than those of the fermented samples. The dominant bacterial genera, with relative abundances higher than 5.0% in at least one sample, were Staphylococcus, Burkholderia-Caballeronia-Paraburkholderia, unclassified Enterobacteriaceae, Pantoea, Aerococcus, Pseudomonas, and unclassified Burkholderiaceae, which represented between 44.6 and 96.1% of the total abundance in each CFL sample (Figure 6C). The relative abundance of Staphylococcus decreased significantly at P0 and J0. The dominant fungal genera were Aspergillus and Alternari (Figure 6D). The relative abundance of Aspergillus and Alternari significantly decreased in P0 and J0, whereas the relative abundance of Stemphylium showed the opposite trend.
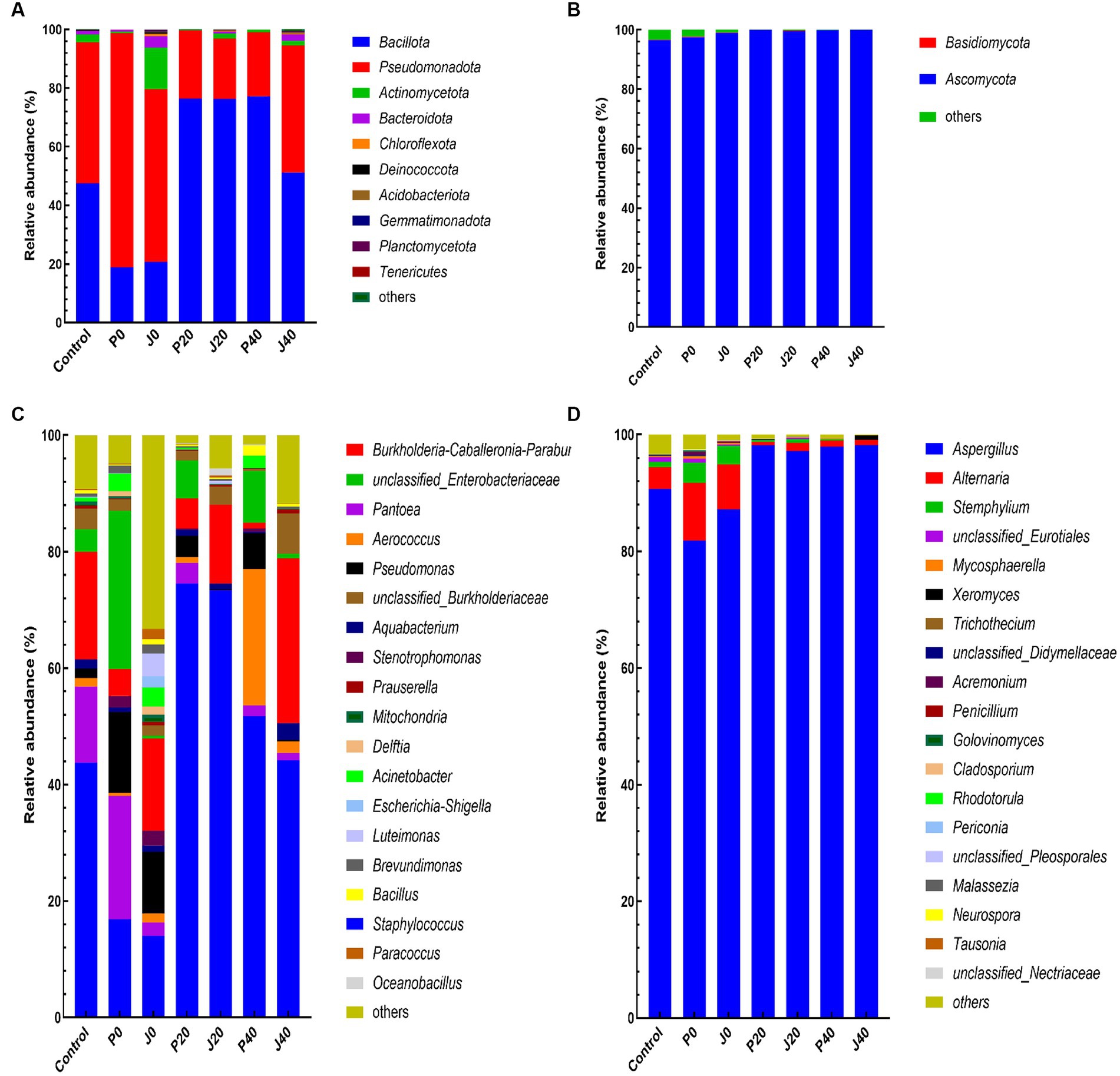
Figure 6. Plot of the phylum and genus level relative abundances for the bacterial and fungal communities in CFLs. Bacterial communities at the phylum (A) and genus levels (C), and fungal communities at the phylum (B) and genus levels (D).
LEfSe analyses revealed significant differences below the phylum level to explore the different microbes among the CFLs under different treatments (Figure 7). The circles from innermost to the outermost represent bacterial and fungal classifications from the phylum to genus levels, and the corresponding colors in every group denote bacterial and fungal taxa with a significant difference. Notably, 85 different bacteria appeared at the LDA threshold of 2, judging by statistically significant differences (p < 0.05), which consisted of two phyla, four classes, 10 orders, 22 families, and 47 genera (Figure 7A). In detail, 30 genera were significantly enriched in inoculated T. aurantialba SCT-F3 CFLs without fermented (J0), such as Escherichia-Shigella, Luteimonas, and Brachybacterium. Four genera were significantly enriched in P0: Brevundimonas, Clostridium sensu stricto1, Variovorax, and Moraxellaaceae. Two genera were significantly enriched in J20, including Oceanobacillus and Curvibacter. Eight genera were significantly enriched in J40, such as Aquabacterium, Burkholderia-Caballeronia-Paraburkholderia, and Prauserella. There were 12 fungi of two classes, one order, four families, and five genera (Figure 7B). Colletotrichum and Symmetrospora were significantly enriched in J0 and P0, Sagenomella was enriched in J20, and Aspergillus in J40.
3.7. Changes in microbial metabolic pathways
Function predictions derived from the microbial community data were obtained using PICRUSt for bacterial functional genes and FUNGuild for fungal functional genes to analyze microbial function (Figures 8A,B). The abundance of functional gene sequences in fungi was much lower than in bacteria. The functions of bacteria and fungi in CFLs include nucleotide biosynthesis, amino acid biosynthesis, fatty acid and lipid biosynthesis, and fermentation. The abundance of many bacterial metabolic pathways increased significantly after fermentation, whereas fungal metabolic pathways showed the opposite trend. For samples with T. aurantialba SCT-F3 fermentation medium (J0, J20, and J40), the abundance of functional genes increased, peaked on the 20th day of fermentation, and then gradually decreased. The relative abundance of functional bacterial genes at P40 increased significantly. There were 65 functional genes up-regulated and 11 down-regulated in samples inoculated with T. aurantialba SCT-F3 (J0, J20, and J40) compared with samples inoculated with potato glucose broth (P0, P20, and P40) (Table 2 and Figure 8C). The abundance of functional genes related to amino acid metabolism, fermentation, and fatty acid degradation increased after adding the microbial medium.
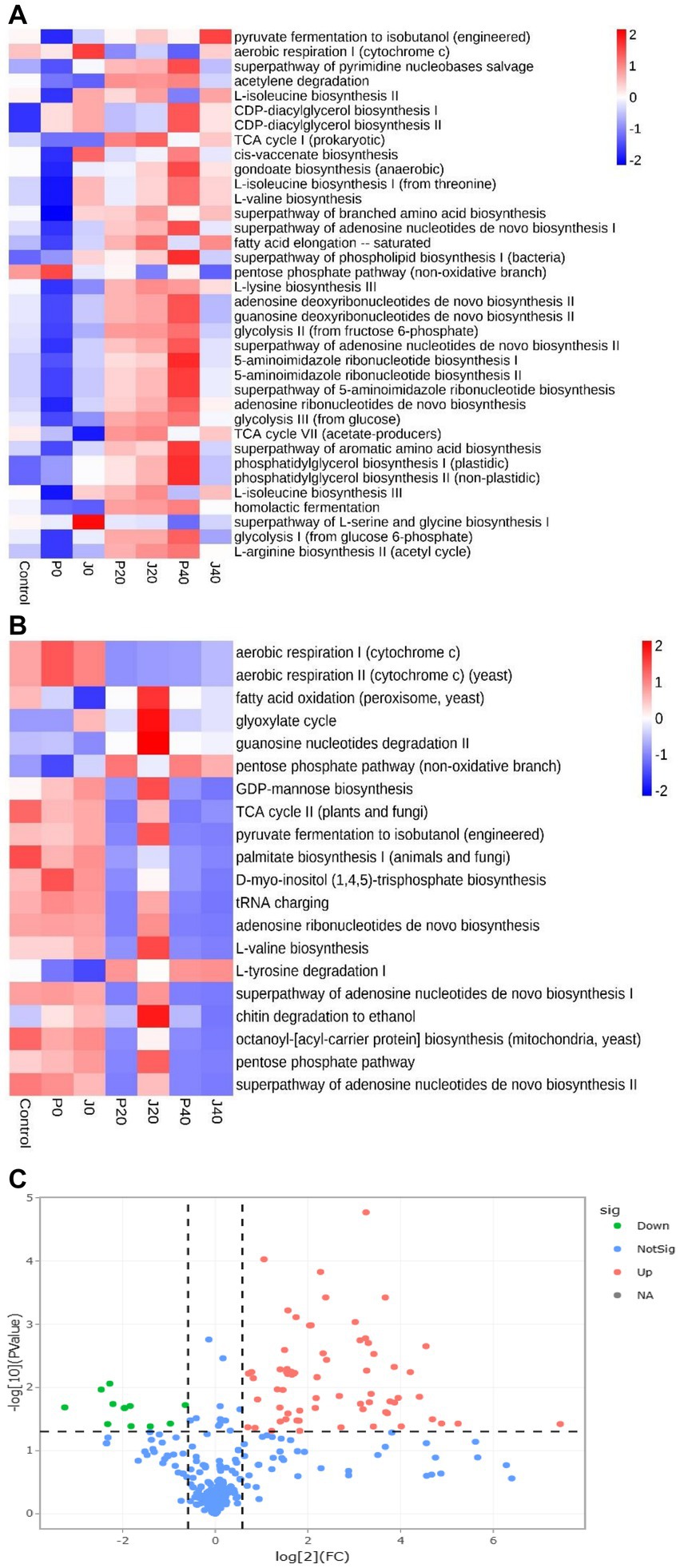
Figure 8. The relative abundance of bacterial metabolic pathways in CFLs. Function prediction of the bacteria (A) and fungi (B) in CFLs. Volcano plot of the numbers of differentially expressed genes between the two groups (C). Red represents the up-regulated, and green represents the down-regulated genes in group A. Blue represents that these genes had no differential expression between the two groups.
Furthermore, a metabolic network, including nicotine degradation (M00810 and M00811) and nicotinate degradation (M00622) according to KEGG,1 during fermentation was constructed using the annotated enzymes and their metabolic pathways (Figure 9). The types and abundances of bacterial enzymes were higher than those of fungi. The enzymes associated with nicotine degradation were less abundant than those associated with nicotinate degradation. In the nicotine degradation pathway, EC:1.5.99.4 (nicotine dehydrogenase subunit A/B/C, ndhA/B/C), EC:1.4.2.2 (nicotine dehydrogenase, nox/nicA2), EC:1.5.3.5 ((S)-6-hydroxynicotine oxidase, nctB), EC:1.4.2.3 (pseudooxynicotine dehydrogenase, pao), EC:1.5.3.6 ((R)-6-hydroxynicotine oxidase, 6-hdno), EC:1.2.1.83 (3-succinoylsemialdehyde-pyridine dehydrogenase, sap), and EC:1.5.99 (3-succinoylpyridine monooxygenase) were not detected in any sample. The abundance of EC:1.5.99.14 (6-hydroxypseudooxynicotine dehydrogenase subunit alpha, kdhA), EC:1.14.13.10 (2,6-dihydroxypyridine 3-monooxygenase), and EC:1.5.3.19 (4-methylaminobutanoate oxidase (formaldehyde-forming)) increased in J0 and gradually decreased during fermentation. In the nicotinate degradation pathway, the abundance of EC:1.13.11.9 (2,5-dihydroxypyridine 5,6-dioxygenase, nicX), EC:3.5.1.106 (N-formylmaleamate deformylase, nicD), and EC:3.5.1.107 (maleamate amidohydrolase, nicF) showed the same trend. The abundance of microbial enzymes annotated to succinate semialdehyde transferred into the gamma-aminobutyrate (GABA) shunt, dicarboxylate-hydroxybutyrate cycle, and hydroxypropionate-hydroxybutylate cycle, and fumarate transferred into the citrate cycle, urea cycle, tyrosine degradation, reductive citrate cycle, dicarboxylate-hydroxybutyrate cycle, hydroxypropionate bicycle, incomplete reductive citrate cycle, and arginine biosynthesis were higher than the abundance of enzymes involved in nicotine degradation and nicotinate degradation pathways.
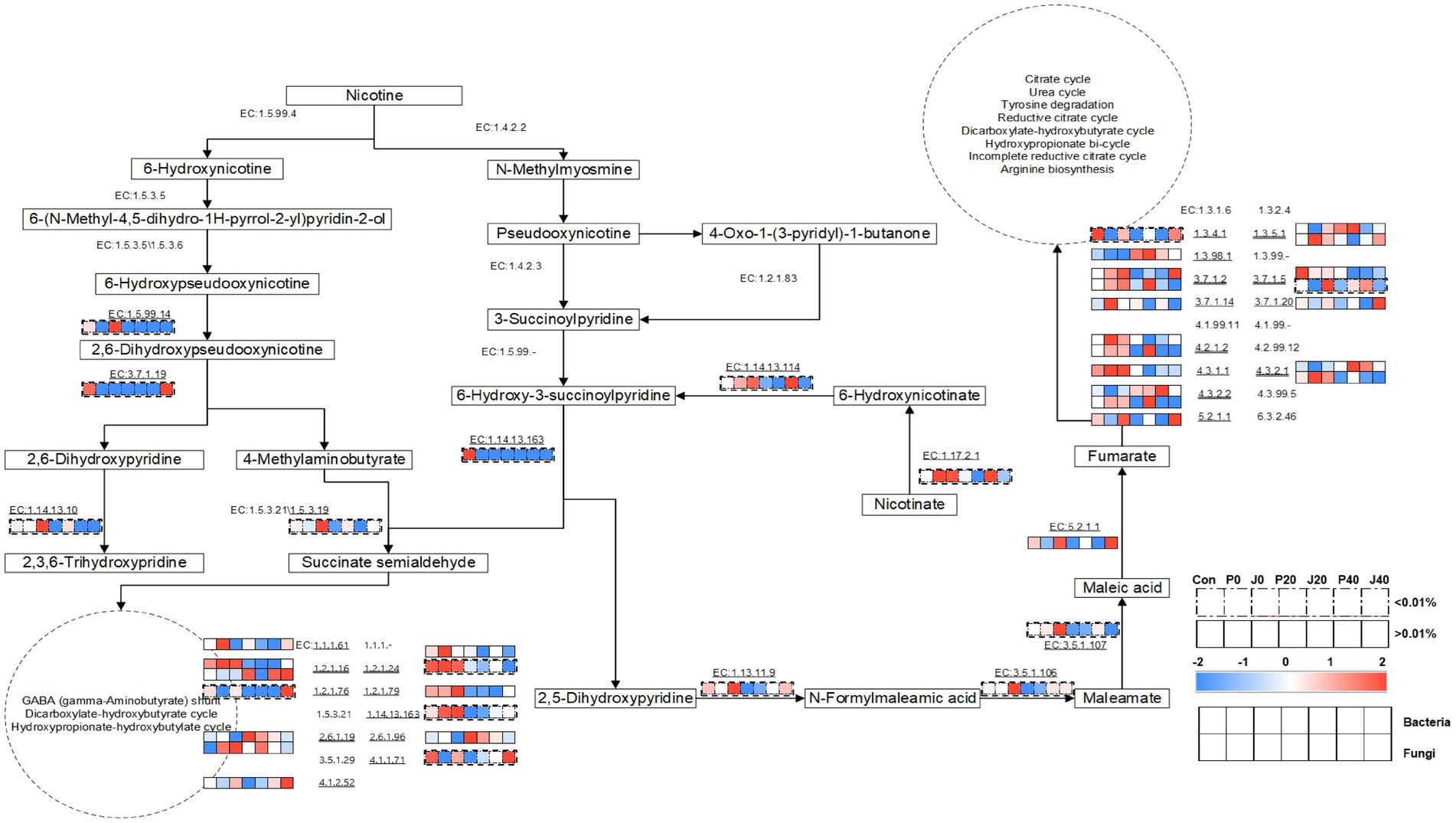
Figure 9. Metabolic pathways of nicotine degradation and nicotinate degradation and the expression levels of the genes encoding related enzymes in CFLs according to Encyclopedia of Genes and Genomes (KEGG) (Jimenez et al., 2008; Qiu et al., 2012).
3.8. Correlation analysis of the predominant microbes and VFCs
Spearman’s correlation coefficients (p < 0.05) were used to establish significant associations between the 25 dominant microorganisms and 34 VFCs (Table 3). Acinetobacter (M1), Aerococcus (M2), and Oceanobacillus (M14) were not significantly correlated with VFCs. Moreover, 2-Ethyl-6-methylpyrazine, 2-phenylethanol, 4′-methylacetophenone, acetoin, acetophenone, and styrene were not significantly correlated with microorganisms. Graphs of the positive and negative-correlation networks were visualized using Gephi, as shown in Figures 10A,B, respectively. The modularity index of the positive correlation graph was 0.452 (>0.4), suggesting that the network had a modular structure. Twenty genera were significantly correlated with 21 VFCs values. A total of 41 notes and 72 edges (pairs of significant and robust correlations) were identified. There were six highly connected genera (degree ≥5), including Pantoea, Paracoccus, Stenotrophomonas, Mycosphaerella, Stemphylium, and unclassified Eurotiales, belonging to Pseudomonadota and Ascomycota. Seven highly connected VFCs, 1-octen-3-one, geranylacetone, sulcatone, 2,3-butanedione, benzeneacetaldehyde, 2-hexenal, and decanal, belong to plastochrome degradation products, Maillard reaction products, aromatic amino acid degradation products, and others. Nicotinic degradation products, cotinine, and 2,4,6-collidine were significantly and positively correlated with Burkholderia-Caballeronia-Paraburkholderia and Prauserella, Mycosphaerella, and Stemphylium, respectively. Gamma-valerolactone was significantly and positively correlated with Mycosphaerella and unclassified Eurotiales; geranylacetone with Pantoea; and unclassified Eurotiales, Alternaria, Paracoccus, and Stemphylium, neophytadiene, and megastigmatrienone with Pantoea.
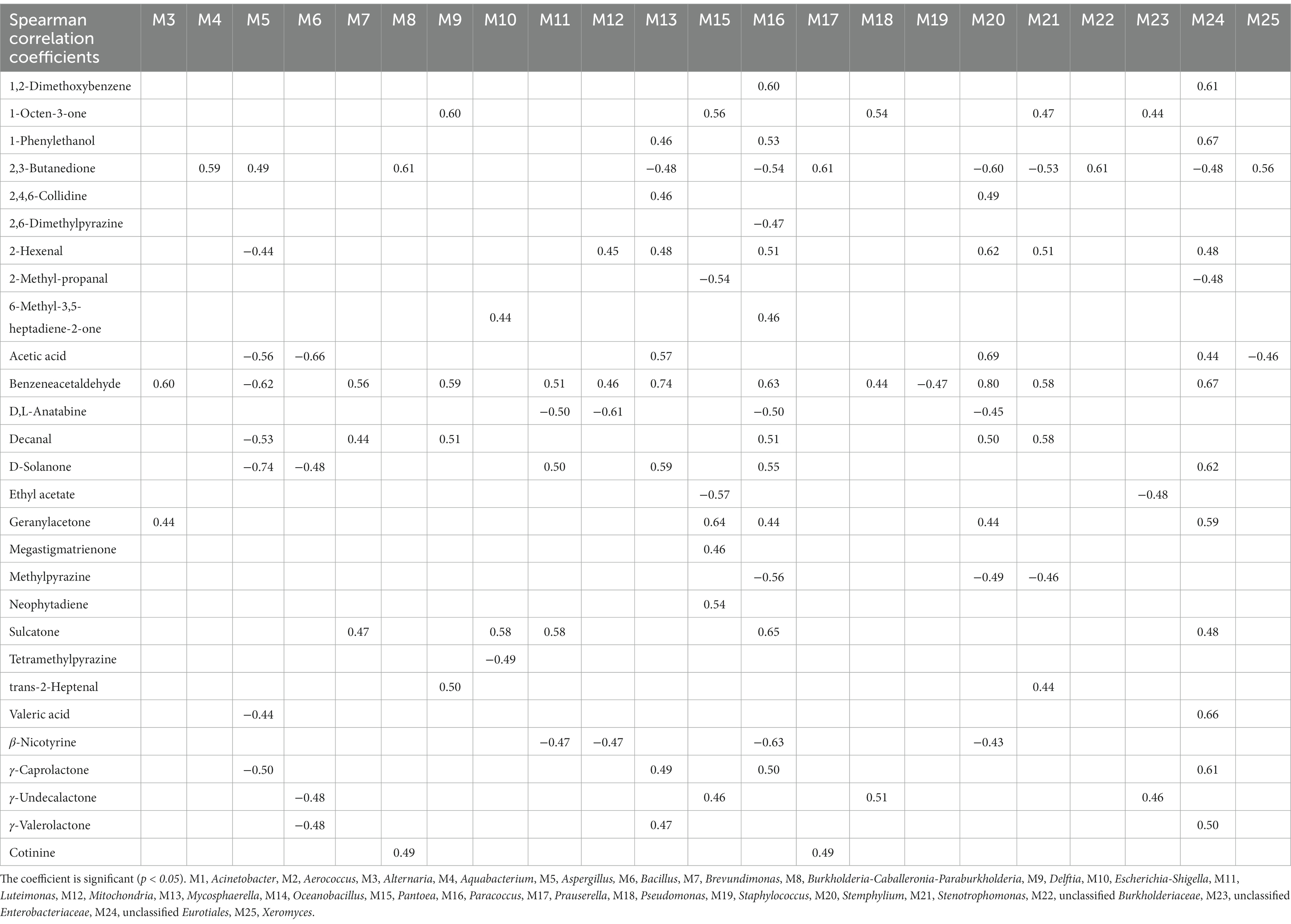
Table 3. Spearman’s correlation coefficients between representative microbes and core volatile flavor compounds.
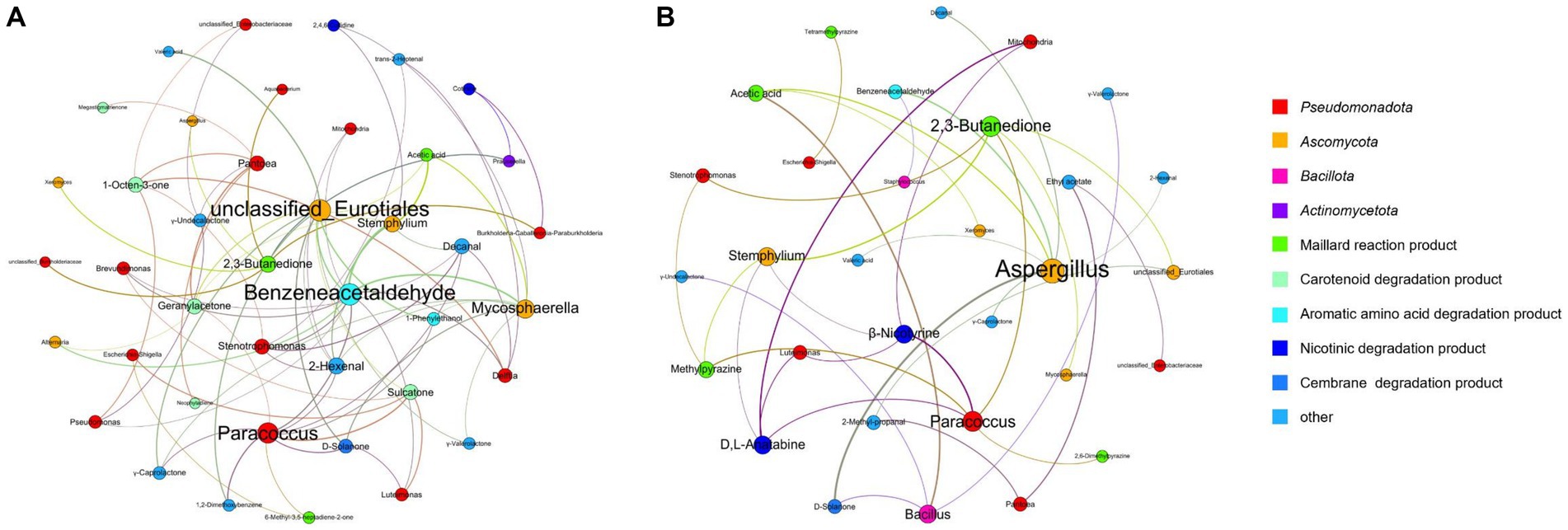
Figure 10. Co-occurrence networks of representative microbes and core VFCs in CFLs based on correlation analysis, positive (A) and negative (B) correlation.
The modularity index of the negative correlation graph was 0.605 (>0.4), suggesting that the network had a modular structure. Fourteen genera significantly correlated with 17 VFCs. A total of 31 notes and 35 edges (pairs with significant and robust correlations) were identified. There were three highly connected notes (degree ≥5), including Aspergillus, Paracoccus, and 2,3-butanedione. Aspergillus was significantly and negatively correlated with D-solanone, acetic acid, 2-hexenal, benzeneacetaldehyde, valeric acid, γ-caprolactone, and decanal, which are cembrane degradation products and Maillard reaction products. 2,3-Butanedione significantly and negatively correlated with Mycosphaerella, Stemphylium, unclassified Eurotiales, Paracoccus, and Stenotrophomonas, belonging to Ascomycota and Pseudomonadota. The nicotinic degradation products, β-nicotyrine, and D,L-anatabine were significantly and negatively correlated with Stemphylium, Paracoccus, Mitochondria, and Luteimonas. Gamma-valerolactone was significantly and negatively correlated with Bacillus; ethyl acetate with Pantoea and unclassified Enterobacteriaceae; 2,6-dimethylpyrazine with Paracoccus; methylpyrazine with Paracoccus, Stemphylium, and Stenotrophomonas; and tetramethylpyrazine with Escherichia-Shigella.
4. Discussion
Tobacco leaves are the most widely planted nonfood crops worldwide. The quality of tobacco leaves is affected by the breed, climate, soil, and culture conditions. Due to the poor quality of cigar tobacco grown in China at present, developing a new measure to improve their quality would have important economic benefits for both tobacco farmers and manufacturers. In the present study, the addition of an edible medicinal fungus, T. aurantialba SCT-F3 fermented broth as the fermentation medium improved the quality of CFLs. According to the results of sensory evaluation, the mellow aroma, richness, maturity, and sweetness of fermented CFLs increased, irritation decreased, and the sense of balance improved. The addition of fermentation media significantly changed the total alkaloid, total nitrogen, reduced sugar, and total sugar contents. The CFLs’ total sugar and reduced sugar contents significantly increased at the beginning of fermentation. The fermented broth of T. aurantialba contains not only a large amount of monosaccharides, such as mannose, glucose, and galactose (Deng et al., 2017), but also amylase and cellulase, which can decompose starch and cellulose (Chen et al., 2021), and might be the cause of this phenomenon. At the end of fermentation, there were no significant difference in the reducing sugar and total sugar content of all samples, and there was no significant difference in the total alkaloid content of the samples with added culture medium. Microbes consume nicotine and aniline compounds, and alkaloid compounds produced by microbial metabolism or protein decomposition, which may cause the total alkaloid content first decreased and then increased during fermentation. The fluctuation of total sugar and reducing sugar content during the fermentation process is due to the degradation of glycogen, the utilization of microorganisms, and the Maillard reaction (Ye et al., 2019). Total nitrogen and nicotine are related to smoke concentration and pungency, and sugar is related to sweetness (Hu et al., 2022). However, within a certain concentration range, the contents of chemical components, total alkaloids, total nitrogen, reducing sugar, and total sugar may not directly reflect the sensory qualities of CFLs. Therefore, we measured VFCs to explore the effects of T. aurantialba SCT-F3 fermentation medium on the quality of CFLs.
Aromatic amino acid degradation products, plastochrome degradation products, cembrane degradation products, Maillard reaction products, and nicotine degradation products are especially important for forming characteristic tobacco flavors and aromas (Xie, 2009; Yin et al., 2012). Four VFCs from the Maillard reaction, 2,3-butanedione with creamy fragrance, methylpyrazine with sweet fragrance, 2,6-dimethylpyrazine and 2-ethyl-6-methylpyrazine with baked potato fragrance (Yan and Zhao, 2008) increased in CFLs fermented with T. aurantialba SCT-F3 fermentation medium. Fermentation led to the increase in nicotine degradation products, including β-nicotyrine, D,L-anatabine, and cotinine. VFCs from T. aurantialba SCT-F3 broth increased in unfermented CFLs added T. aurantialba SCT-F3 fermentation medium. However, the increase in VFCs did not significantly improve the sensory quality scores. Some VFCs in CFLs gradually decreased or increased as fermentation progressed; however, the sensory quality continuously increased. Additionally, through PLS analysis of conventional chemical constituents, VFCs, and sensory evaluations, it was found that in the complex aroma system by our study, 2-ethyl-6-methylpyrazine, methylpyrazine, D,L-anatabine, β-nicotyrine, nicotinic degradation products and total nitrogen had a significantly positive contribution to the sensory quality. In contrast, 1-phenylethanol with rose flavor, geranylacetone with green flavor, megastigmatrienone with flowery and woody flavor, D-solanone enhancing the fragrance of tobacco, 6-methyl-3,5-heptadiene-2-one with cinnamon aroma, 1,2-dimethoxybenzene with pleasant odor, and γ-caprolactone with herbal and sweet flavor showed the opposite trend. Although higher content of VFCs would endow CFLs with more fragrant flavor, the coordination and balance of VFCs in tobacco leaves determine the sensory quality of tobacco leaves. A significant increase or decrease in a VFC might break the balance (Zhang et al., 2021). However, there is no criteria for evaluating coordination and balance to measure the quality of cigar tobacco yet. Further study is needed to establish the criteria for evaluating coordination and balance of chemical composition and VFCs to reduce sensory evaluators workload and provide objective criterion.
The effects of T. aurantialba SCT-F3 fermentation medium and fermentation on the microbial community of CFLs were amplified using Illumina high-throughput sequencing. The addition of the fermentation medium significantly changed the microbes’ richness, diversity, and structure. Fungal communities have more stable structures than bacterial communities. Aspergillus was the dominant fungus, mainly clustered in CFLs, and may play an important role in maintaining the stability of fungal communities. Aspergillus species, filamentous fungi with GRAS status, are widely used in the food industry to produce multiple enzymes such as amylases, proteases, lipases, cellulases, and aroma compounds (Raveendran et al., 2018; Kumar et al., 2021; Chilakamarry et al., 2022). It is worth noting that in the fermentation process of CFLs, avoiding Aspergillus sp. mycelium formation affects the appearance quality of CFLs, while applying their properties of enzyme and aroma production is very important.
Changes in the microbial communities of the CFLs may be separated into three distinct phases. Phase I is the initial disturbance period. In this phase, the endogenous microbial community and its functions changed dramatically with the addition of fermentation media. Nutrients, including sugars, proteins, ketones, aldehydes, alcohols, and esters from T. aurantialba SCT-F3 fermentation medium facilitated the growth of 31 microbial genera, which led to an increase in diversity and species richness. Furthermore, the activity of 20 microbial functional genes increased, including nucleotide biosynthesis, amino acid biosynthesis, fatty acid and lipid biosynthesis, fermentation, nicotine degradation, and nicotinate degradation. This indicates that adding T. aurantialba SCT-F3 accelerated the VFCs of CFLs and shortened the fermentation time (Cai et al., 2022). Phase II was the transitional period. The loss of diversity and species richness likely resulted from the interactions between microorganisms, such as competition and antagonism (Tao et al., 2014). Functional genes related to nucleotide biosynthesis, amino acid biosynthesis, fatty acid, and lipid biosynthesis, and fermentation further increased, indicating that substance consumption and new substance synthesis occurred in CFLs during this period. Phase III was relatively stable. The microbial community gradually stabilized owing to the complete suppression of external disturbances (Wu et al., 2016). The abundance of functional genes was low, indicating that the metabolic activity of microorganisms has also become less active.
VFCs are affected by microbial metabolism (Yang et al., 2021). Network analysis has shown that microbes in CFLs are closely related to VFCs, consistent with previous study findings (Zhang et al., 2020a,b; Zheng et al., 2022a,b). Aspergillus sp. produces aromatic compounds (Chilakamarry et al., 2022); this was positively related to 2,3-butanedione in our study. Alternaria, a cosmopolitan fungal genus, is one of the dominant resident fungi in tobacco leaves (Chattopadhyay et al., 2021) and is positively related to benzene acetaldehyde with a fruity and sweet aroma (Koan Sik et al., 2006), and geranylacetone. Pseudomonas was positively related to 1-octen-3-one with a mushroom flavor (Bauer et al., 2022), γ-undecalactone with a sweet peach flavor (Chen et al., 2022), and benzeneacetaldehyde with a hyacinth aroma. Achromobacter, Cellulomonas, Enterobacter, Arthrobacter, Alcaligene s, Pseudomonas, and Aspergillus have been reported to degrade nicotine (Meng et al., 2010). In our study, Burkholderia-Caballeronia-Paraburkholderia and Prauserella were positively correlated with cotinine and Mycosphaerella and Stemphylium were positively correlated with 2,4,6-collidine. Although microbial enzymes that catabolize nicotine to 6-hydroxynicotine (EC:1.5.99.4) or N-methylmyosmine (EC: 1.4.2.2) were not detected, enzymes associated with nicotine degradation and the nicotinate degradation pathway increased after the addition of T. aurantialba SCT-F3 fermentation medium. Microbial enzymes mainly participate in succinate semi-aldehyde-transfer and fumarate-transfer pathways. Hence, it is speculated that microbial enzymes in CFLs catalyze the increase of β-nicotyrine, D,L-anatabine, and cotinine during fermentation; some intermediates of these four strains participate in the transformation process of nitrogen-containing substances and some strains use nitrogen-containing substances in the metabolic process.
5. Conclusion
When spontaneous fermentation cannot meet the demands of consumers, medium fermentation is an important processing method for improving the sensory quality of cigar tobacco leaves. Our present study demonstrates a new microbial medium fermentation method for improving the quality of CFLs. The addition of the microbial medium produced by Tremella aurantialba SCT-F3 significantly affected the chemical components and VFCs, mainly increasing the content of Maillard reaction products and nicotine degradation products. The fermentation medium changed the microbial community’s structure and improved CFL microbial activity. We also revealed the contributions of chemical components and VFCs to sensory evaluation and the association between predominant microbes and VFCs.
Data availability statement
The data presented in the study are deposited in the NCBI BioProject repository, accession number PRJNA999475.
Author contributions
QiZ: Conceptualization, Data curation, Formal analysis, Methodology, Software, Writing – original draft, Writing – review & editing. SY: Investigation, Methodology, Resources, Writing – review & editing. ZY: Investigation, Methodology, Resources, Writing – review & editing. TZ: Investigation, Methodology, Resources, Writing – review & editing. PL: Investigation, Methodology, Resources, Writing – review & editing. QuZ: Investigation, Methodology, Resources, Writing – review & editing. WC: Investigation, Methodology, Resources, Writing – review & editing. YW: Investigation, Methodology, Resources, Writing – review & editing. JZ: Funding acquisition, Supervision, Writing – review & editing. XJ: Investigation, Methodology, Resources, Writing – review & editing. DL: Resources, Supervision, Writing – review & editing.
Funding
The author(s) declare financial support was received for the research, authorship, and/or publication of this article. This work was supported by China National Tobacco Company (No. 110202101062(XJ-11) and 110202201032(XJ-03)), and China Tobacco Sichuan Industrial Co., Ltd. (No. rtx201820).
Conflict of interest
Authors QiZ, SY, ZY, PL, QuZ, WC, YW, XJ, and DL were employed by the company China Tobacco Sichuan Industrial Co., Ltd.
The remaining authors declare that the research was conducted in the absence of any commercial or financial relationships that could be construed as a potential conflict of interest. The authors declare that this study received funding from China National Tobacco Company and China Tobacco Sichuan Industrial Co., Ltd. The funder had the following involvement in the study: QZ, SY, ZY, PL, QZ, and WC were involved in the study design, collection, analysis and data analysis. QZ, XJ, and DL were involved in the preparation of the manuscript and decision to publish.
Publisher’s note
All claims expressed in this article are solely those of the authors and do not necessarily represent those of their affiliated organizations, or those of the publisher, the editors and the reviewers. Any product that may be evaluated in this article, or claim that may be made by its manufacturer, is not guaranteed or endorsed by the publisher.
Supplementary material
The Supplementary material for this article can be found online at: https://www.frontiersin.org/articles/10.3389/fmicb.2023.1267916/full#supplementary-material
Abbreviations
CFL, Cigar filler leaf; OTU, Operational taxonomic unit; PLS, Partial least-squares; VFC, Volatile flavor compound.
Footnotes
References
Bauer, P., Ortner, E., and Buettner, A. (2022). Influence of elongation and desaturation on chemosensory properties in acrylates and their corresponding 1-alken-3-ones. Anal. Bioanal. Chem. 414, 8009–8022. doi: 10.1007/s00216-022-04332-9
Cai, W., Hu, W., Li, P., Liu, J., Zhang, Q., Zhou, Q., et al. (2022). Effects of fermentation medium on cigar filler. Front. Bioeng. Biotechnol. 10:1069796. doi: 10.3389/fbioe.2022.1069796
Caporaso, J. G., Kuczynski, J., Stombaugh, J., Bittinger, K., Bushman, F. D., Costello, E. K., et al. (2010). QIIME allows analysis of high-throughput community sequencing data. Nat. Methods 7, 335–336. doi: 10.1038/nmeth.f.303
Chattopadhyay, S., Malayil, L., Mongodin, E. F., and Sapkota, A. R. (2021). A roadmap from unknowns to knowns: advancing our understanding of the microbiomes of commercially available tobacco products. Appl. Microbiol. Biotechnol. 105, 2633–2645. doi: 10.1007/s00253-021-11183-4
Chen, C., Liu, Z., Yu, H., Xu, Z., and Tian, H. (2022). Flavoromic determination of lactones in cheddar cheese by GC-MS-olfactometry, aroma extract dilution analysis, aroma recombination and omission analysis. Food Chem. 368:130736. doi: 10.1016/j.foodchem.2021.130736
Chen, Z., Yu, J., Feng, Y., Ma, M., Yue, W., and Guo, X. (2021). Transcriptome different analysis of Tremella aurantialba at mycelium and fruiting body stages. Biotechnol. Bull. 37, 73–84. doi: 10.13560/j.cnki.biotech.bull.1985.2020-1038
Chilakamarry, C. R., Mimi Sakinah, A. M., Zularisam, A. W., Sirohi, R., Khilji, I. A., Ahmad, N., et al. (2022). Advances in solid-state fermentation for bioconversion of agricultural wastes to value-added products: opportunities and challenges. Bioresour. Technol. 343:126065. doi: 10.1016/j.biortech.2021.126065
China Tobacco Sichuan Industrial Co., Ltd. (2021). The cigar handbook. Beijing: Huaxia Publishing House.
Deng, C., Fu, H., Shang, J., Qiao, Z., Zhao, C., and Chen, J. (2017). Preparation, chemical analysis and biological activity of polysaccharides from fermented Tremella aurantialba. J. Food Sci. Biotechnol. 36, 67–73. doi: 10.3969/j.issn.1673-1689.2017.01.012
Deng, C., Sun, Y., Fu, H., Zhang, S., Chen, J., and Xu, X. (2016). Antioxidant and immunostimulatory activities of polysaccharides extracted from Tremella aurantialba mycelia. Mol. Med. Rep. 14, 4857–4864. doi: 10.3892/mmr.2016.5794
Douglas, G. M., Maffei, V. J., Zaneveld, J., Yurgel, S. N., and Langille, M. G. I. J. C. S. H. L. (2019). PICRUSt2: An improved and extensible approach for metagenome inference. BioRxiv [Epubh ahead of preprint] doi:doi: 10.1101/672295
Hu, W., Cai, W., Zheng, Z., Liu, Y., Luo, C., Xue, F., et al. (2022). Study on the chemical compositions and microbial communities of cigar tobacco leaves fermented with exogenous additive. Sci. Rep. 12:19182. doi: 10.1038/s41598-022-23419-y
Jia, Y., Liu, Y., Hu, W., Cai, W., Zheng, Z., Luo, C., et al. (2023). Development of Candida autochthonous starter for cigar fermentation via dissecting the microbiome. Front. Microbiol. 14:1138877. doi: 10.3389/fmicb.2023.1138877
Jimenez, J. I., Canales, A., Jimenez-Barbero, J., Ginalski, K., Rychlewski, L., Garcia, J. L., et al. (2008). Deciphering the genetic determinants for aerobic nicotinic acid degradation: the nic cluster from Pseudomonas putida KT2440. Proc. Natl. Acad. Sci. U.S.A. 105, 11329–11334. doi: 10.1073/pnas.0802273105
Koan Sik, W., Seo Young, H., Hyang-Sik, Y., Junsoo, L., Heon-Sang, J., and Haeng-Ran, K. (2006). Aroma characteristics of byeolmijang with optional ingredients. J. Korean Soc. Food Sci. Nutr. 35, 738–746. doi: 10.3746/jkfn.2006.35.6.738
Kumar, V., Ahluwalia, V., Saran, S., Kumar, J., Patel, A. K., and Singhania, R. R. (2021). Recent developments on solid-state fermentation for production of microbial secondary metabolites: challenges and solutions. Bioresour. Technol. 323:124566. doi: 10.1016/j.biortech.2020.124566
Li, N., Wang, C., Zeng, D., and Liu, Y. (2012). Isolation and identification of bacillus cereus and its application in cigar leaf fermentation. Acta Tabacaria Sin. 18, 65–69. doi: 10.3969/j.issn.1004-5708.2012.02.013
Lin, C., Cui, H., Wang, X., Wang, H., Xia, S., Hayat, K., et al. (2020). Regulating water binding capacity and improving porous carbohydrate matrix's humectant and moisture proof functions by mixture of sucrose ester and Polygonatum sibiricum polysaccharide. Int. J. Biol. Macromol. 147, 667–674. doi: 10.1016/j.ijbiomac.2020.01.101
Meng, X. J., Lu, L. L., Gu, G. F., and Xiao, M. (2010). A novel pathway for nicotine degradation by aspergillus oryzae 112822 isolated from tobacco leaves. Res. Microbiol. 161, 626–633. doi: 10.1016/j.resmic.2010.05.017
Parada, A. E., Needham, D. M., and Fuhrman, J. A. (2016). Every base matters: assessing small subunit rRNA primers for marine microbiomes with mock communities, time series and global field samples. Environ. Microbiol. 18, 1403–1414. doi: 10.1111/1462-2920.13023
Qiu, J., Ma, Y., Wen, Y., Chen, L., Wu, L., and Liu, W. (2012). Functional identification of two novel genes from Pseudomonas sp strain HZN6 involved in the catabolism of nicotine. Appl. Environ. Microbiol. 78, 2154–2160. doi: 10.1128/aem.07025-11
Raveendran, S., Parameswaran, B., Ummalyma, S. B., Abraham, A., Mathew, A. K., Madhavan, A., et al. (2018). Applications of microbial enzymes in food industry. Food Technol. Biotechnol. 56, 16–30. doi: 10.17113/ftb.56.01.18.5491
Rognes, T., Flouri, T., Nichols, B., Quince, C., and Mahe, F. (2016). VSEARCH: a versatile open source tool for metagenomics. PeerJ 4:e2584. doi: 10.7717/peerj.2584
Sun, T., Wang, R., Sun, D., Li, S., Xu, H., Qiu, Y., et al. (2020). High-efficiency production of Tremella aurantialba polysaccharide through basidiospore fermentation. Bioresour. Technol. 318:124268. doi: 10.1016/j.biortech.2020.124268
Tao, Y., Li, J., Rui, J., Xu, Z., Zhou, Y., Hu, X., et al. (2014). Prokaryotic communities in pit mud from different-aged cellars used for the production of Chinese strong-flavored liquor. Appl. Environ. Microbiol. 80, 2254–2260. doi: 10.1128/AEM.04070-13
Usyk, M., Zolnik, C. P., Patel, H., Levi, M. H., and Burk, R. D. (2017). Novel ITS1 fungal primers for characterization of the Mycobiome. mSphere 2:e00488-17. doi: 10.1128/mSphere.00488-17
Wu, Q., Kong, Y., and Xu, Y. (2016). Flavor profile of Chinese liquor is altered by interactions of intrinsic and extrinsic microbes. Appl. Environ. Microbiol. 82, 422–430. doi: 10.1128/AEM.02518-15
Xie, G., Kong, X., Kang, J., Su, N., Fei, J., and Luo, G. (2021). Fungal community succession contributes to product maturity during the co-composting of chicken manure and crop residues. Bioresour. Technol. 328:124845. doi: 10.1016/j.biortech.2021.124845
Yang, M., Huang, J., Zhou, R., Qi, Q., Peng, C., Zhang, L., et al. (2021). Characterizing the microbial community of Pixian Doubanjiang and analysing the metabolic pathway of major flavour metabolites. Lwt 143:111170. doi: 10.1016/j.lwt.2021.111170
Ye, J., Wang, L., Yang, F., Yan, J., Yang, X., Ma, K., et al. (2019). Improving quality of reconstituted tobacco via fermenting extract of its raw material with Paenibacillus sp. Acta Tabacaria Sin. 25, 33–38. doi: 10.16472/j.chinatobacco.2018.097
Yin, G., Xu, S., Ruan, X., Wei, J., Liu, J., and Hou, D. (2012). Changes of degradation products of carotenoids and cembranoids in B4F flue-cured tobacco during redrying. Tobacco Sci Technol. 10, 61–64. doi: 10.3969/j.issn.1002-0861.2012.10.014
Zhang, Q., Geng, Z., Deng, Y., Luo, C., Li, D., and Ding, Z. (2021). Effects of Ophiocordyceps sinensis fermentation on sensory qualities and chemical components of tobacco leaves from the minor materials area. Acta Tabacaria Sin. 27, 1–9. doi: 10.16472/j.chinatobacco.2020.278
Zhang, Q., Geng, Z., Li, D., and Ding, Z. (2020a). Characterization and discrimination of microbial community and co-occurrence patterns in fresh and strong flavor style flue-cured tobacco leaves. Microbiology 9:e965. doi: 10.1002/mbo3.965
Zhang, Q., Luo, C., Li, D., and Cai, W. (2020b). Research progress in curing and fermentation technology for cigar tobacco leaf production. Acta Tabacaria Sin. 26, 1–6. doi: 10.16472/j.chinatobacco.2019.339
Zheng, T., Zhang, Q., Li, P., Wu, X., Liu, Y., Yang, Z., et al. (2022a). Analysis of microbial community, volatile flavor compounds, and flavor of cigar tobacco leaves from different regions. Front. Microbiol. 13:907270. doi: 10.3389/fmicb.2022.907270
Zheng, T., Zhang, Q., Wu, Q., Li, D., Wu, X., Li, P., et al. (2022b). Effects of inoculation with Acinetobacter on fermentation of cigar tobacco leaves. Front. Microbiol. 13:911791. doi: 10.3389/fmicb.2022.911791
Zhu, H., Cao, C., Zhang, S., Zhang, Y., and Zou, W. (2011). pH-control modes in a 5-L stirred-tank bioreactor for cell biomass and exopolysaccharide production by Tremella fuciformis spore. Bioresour. Technol. 102, 9175–9178. doi: 10.1016/j.biortech.2011.06.086
Keywords: cigar filler leaf (CFL), Tremella aurantialba , fermentation medium, microbial community, volatile flavor compound
Citation: Zhang Q, Yang S, Yang Z, Zheng T, Li P, Zhou Q, Cai W, Wang Y, Zhang J, Ji X and Li D (2023) Effects of a novel microbial fermentation medium produced by Tremella aurantialba SCT-F3 on cigar filler leaf. Front. Microbiol. 14:1267916. doi: 10.3389/fmicb.2023.1267916
Edited by:
Ming Jun Zhu, South China University of Technology, ChinaReviewed by:
Guangsen Fan, Beijing Technology and Business University, ChinaBinbin Hu, Yunnan Academy of Tobacco Agricultural Sciences, China
Copyright © 2023 Zhang, Yang, Yang, Zheng, Li, Zhou, Cai, Wang, Zhang, Ji and Li. This is an open-access article distributed under the terms of the Creative Commons Attribution License (CC BY). The use, distribution or reproduction in other forums is permitted, provided the original author(s) and the copyright owner(s) are credited and that the original publication in this journal is cited, in accordance with accepted academic practice. No use, distribution or reproduction is permitted which does not comply with these terms.
*Correspondence: Xiaoying Ji, aml4eWNoZW5AMTYzLmNvbQ==; Dongliang Li, MzYwMTg4MjI4QHFxLmNvbQ==